Balance performance when responding to visual stimuli in patients with Benign Paroxysmal Positional Vertigo (BPPV)
Abstract
BACKGROUND:
Patients with Benign Paroxysmal Positional Vertigo (BPPV) often report a sensation of disequilibrium before treatment with the canalith repositioning maneuvers. Apart from vestibular information, visual input also contributes to balance control. How visual stimuli influence balance control in BPPV patients is unclear.
OBJECTIVE:
To investigate the influence of visual stimuli on balance performance in BPPV patients.
METHODS:
Three groups of participants, patients with BPPV, and healthy young and older adults, were instructed to stand under three conditions: 1) eyes open (EO); 2) while watching a static picture via a video display; and 3) while watching a rotating visual scene. Antero-posterior (AP), lateral (ML), and total sway path during standing were analyzed.
RESULTS:
In all conditions, patients with BPPV showed significantly larger AP, ML and total sway path than young, whereas older adults only showed significantly larger AP and total sway path than young adults. During the visual stimuli, all participants exhibited significantly increased AP and total sway while watching a static image and a moving scene as compared with the EO condition.
CONCLUSIONS:
Patients with BPPV have similar balance control to older adults, but poorer balance control than young adults. The reduced lack of standing balance control in the coronal plane of patients with BPPV, might affect balance strategy after external perturbations.
1Introduction
Benign paroxysmal positional vertigo (BPPV) is one of the most commonly seen vestibular disorders in neurology, and ear, nose and throat (ENT) clinics [29, 33]. It is characterized by recurrent and very short periods of intense vertigo no longer than one minute with a quick change of head position [9, 22]. During a vertigo attack, patients typically experience rotational vertigo and an inability in maintaining upright balance [8, 9]. Between the vertigo attacks, although rotational vertigo may be absent, many patients may still complain of a continual sensation of disequilibrium in an upright position [8, 19].
The control of standing balance requires sensory inputs from the visual, somatosensory and vestibular systems and appropriate motor commands to the musculoskeletal system [2, 4, 35, 44]. Vestibular disorders can disrupt sensory control of posture [36] as well as vestibular reflexes involving postural muscle responses [1], leading to balance problems and increased risk of falls [16, 28, 34, 40]. Although unsteadiness is a common complaint in patients with BPPV, our understanding is limited. As symptoms of BPPV may resolve spontaneously in most cases, most patients would tend to slow down their daily movement until the symptoms have resolved. During this period of time, patients might have altered their balance performance because of the vestibular disorders, which might increase the risk of falling [30]. There have been studies investigating balance performance in people with vestibular disorders [12, 44]. There was evidence showing that balance performance of BPPV patients could be effectively ameliorated by a canalith repositioning manoeuver [5, 11, 13, 31, 32]. However, it is unclear how BPPV patients would respond to an external perturbation, especially in the active phase before treatment with the canalith repositioning maneuvers.
Studies examining balance performance were often tested in a perturbation paradigm using a moving platform or unstable surface to provide an external physical perturbation directly to the body [3]. Another form of introducing balance disturbances is via sensory interference, such as a visual disturbance. Of all the different sensory inputs, vision provides reference information about the orientation of the body related to the outer world. Therefore, when visual information is deprived, maintaining upright balance becomes more challenging, as indicated by increased postural sway compared to the condition when visual information is available [38]. Another visual condition different from deprived vision is movement of the outer environment when one is in a static position. This visual condition is more common in the daily life than deprived vision, such as standing on a platform watching a train passing by or watching moving traffic while waiting to cross the road. This kind of motion of the environment normally creates an illusion of self-motion and produces sensory conflicts [6]. Because of these sensory conflicts, increased postural adjustments were found in healthy adults when watching moving scenes [17].
It is not clear to what extent balance performance is influenced by BPPV when patients are exposed to a visual stimulus, particularly in the active phase. Therefore, the purpose of this study was to investigate balance performance in response to a visual stimulus in patients with BPPV in the active phase, compared with healthy young and older adults.
2Methods
2.1Participants
Patients with a history suggestive of position related vertigo (i.e. brief vertigo episodes triggered by head movements such as lying down, getting up or turning over in bed, or looking up/down) in the past month (BPPV group) were recruited from the Neurology and ENT department of National Cheng Kung University Hospital, Taiwan. If the patients did not show typical positional nystagmus following the Dix-Hallpike manoeuvers, they were excluded from the analysis. Patients with central nervous system disease or other conditions that could affect balance were also excluded. All the patients had normal caloric and electronystagmography (ENG) test results.
Two other groups of young (age 20–25 years) and older participants (age > 60 years) with no history of vestibular or neurological disease were recruited from the university and nearby communities and served as control groups. Written consent was obtained from all participants as approved by the ethics committee of the institution where the study was conducted.
2.2Sensorimotor assessment
Visual acuity was examined using a standard printed Snellen eye chart with the participant seated 20 feet away[24]. Binocular visual contrast sensitivity was examined using the Melbourne Edge Test [46]. Correct identification of the orientation of the edges on the patches indicates a measure of contrast sensitivity in decibel units. Both visual tests were tested with corrected vision if the participant wore corrective glasses during daily walking.
Plantar cutaneous sensitivity was examined on a total of six sites for the sole of both feet, including the first and fifth metatarsal heads and the center of the heel, using the Semmes-Weinstein monofilaments (Patterson Company, IL, USA). The Semmes-Weinstein monofilaments consist of 20 filaments with different thickness of nylon string. Participants were tested in the sitting position with the eyes closed to eliminate visual cues. During the testing, the filament was applied perpendicularly and slowly to the test site for 1.5 seconds until the filament bowed. The participant was asked to indicate if the pressure was felt. The thinnest filament that the participant was able to perceive was recorded as the Plantar cutaneous sensitivity.
Bilateral hand-grip strength was assessed using a calibrated JAMAR hand dynamometer (Sammons Preston Rolyan, IL, USA). Participants exerted maximal grip for three seconds in a sitting position with the tested arm by the side, elbow flexed at 90°, and the forearm in neutral position. The maximal isometric strength of bilateral hip flexors, knee extensors, and ankle dorsiflexors was measured using a handheld dynamometer (MicroFET2, HOGGAN Health Industries, UT, USA) following standardized manual muscle testing procedures [20]. Bilateral ankle plantarflexor strength was tested using the standardized manual muscle testing with a 25-level grade [20].
2.3Balance tasks
All participants were asked to stand with the feet shoulder-width apart on a force plate (Kistler model 9286A, Winterthur, Switzerland). A head-mounted video display (Prober, Karlton 4), which included two 0.72-inch LCD panel, was used for image projection to provide either a static image or moving visual scenes for visual disturbance. The sides of the goggles were covered to avoid peripheral vision. Each of the following three standing conditions were recorded for 30 seconds: 1) eyes open without goggles (EO); 2) while watching a static picture of a seashore via the video display; and 3) while watching a moving visual scene that created an illusion of self-rotating around the seashore. The tests were performed in the order of EO, static image, and moving visual scene.
2.4Data reduction and analysis
Center of pressure (COP) was analyzed from the force plate recordings during each of the tasks. Signals were sampled at 1000 Hz.The following sway parameters were calculated from the COP data and normalized to the foot length (average of both feet lengths): i) antero-posterior (AP) sway path, ii) lateral (ML) sway path, and iii) total sway path, which combines the length of AP and ML sway.
In order to investigate balance strategy under different visual conditions in different subject groups, all the aforementioned sway parameters (AP, ML, and total sway) were further divided into three time phases: i) Phase I: 1–10 seconds, ii) Phase II: 11–20 seconds, and iii) Phase III: 21–30 seconds of sway path.
The SPSS version 17 (SPSS Inc., Chicago, IL) was used for all of the statistical analyses. Averaged values of bilateral visual acuity, touch-pressure sensitivity and muscle strength were used for analysis. One-way analysis of variance (ANOVA) was used to compare the demographic data, visual acuity, visual contrast sensitivity, plantar cutaneous sensitivity and all the muscle strength data except plantarflexor strength among the three groups. The Kruskal-Wallis test was used to compare ankle plantarflexor strength among the three groups. Multivariate repeated-measures ANOVA (MANOVA) with Bonferroni post-hoc tests were performed on factors “condition” (EO, static image, and moving visual scene) and “group” (young, older, and BPPV) within each phase on the AP, ML, and total sway path parameters. All significance levels were set at p < 0.05.
3Results
Thirty healthy young subjects and 30 healthy older subjects completed the tests. Thirty-five patients with a history suggestive of position related vertigo were recruited for testing. Five of the patients showed negative Dix-Hallpike testing results and were excluded from the analysis. The other 30 patients were diagnosed with unilateral posterior semicircular canal BPPV. The demographic data are shown in Table 1. The young group was significantly taller and had significantly lower BMI than the other two groups. The young group also had significantly longer foot length than the BPPV group. The affected semicircular canals of the BPPV patients were right posterior in 19, and left posterior in 11 patients.
Table 1
Demographic data and physical examinations
Group | Young (N = 30) | Older (N = 30) | BPPV (N = 30) |
Basic data | |||
Age (yrs) | 22.1±2.0 | 66.0±4.4† | 49.7±15.8‡ |
Gender (F/M) | 17/13 | 15/15 | 23/7 |
Height (cm) | 167.1±6.7 | 160.0±7.6† | 161.0±8.2‡ |
Weight (kg) | 58.2±7.1 | 60.2±11.1 | 58.5±8.6 |
BMI (kg/m2) | 20.81±1.71 | 23.41±3.29† | 22.54±2.58‡ |
Mean foot length (cm) | 23.9±1.4 | 23.3±1.5 | 23.0±1.1‡ |
Sensory function | |||
Visual acuity (/50) | 24.0±10.5 | 37.4±23.6† | 26.8±12.1‡ |
Visual contrast sensitivity (dB) | 23.6±1.0 | 21.9±2.3† | 21.9±2.1‡ |
Great toe sensitivity (mg) | 3.32±0.33 | 3.90±0.27† | 3.62±0.48‡* |
5th toe sensitivity (mg) | 3.39±0.28 | 4.05±0.28† | 3.75±0.46‡* |
Heel sensitivity (mg) | 3.67±0.36 | 4.14±0.33† | 4.00±0.35‡ |
Strength | |||
Grip (kg) | 30.3±8.7 | 27.7±7.9 | 25.6±8.3 |
Hip flexor (kg) | 19.6±5.5 | 15.5±4.7† | 14.8±5.2‡ |
Knee extensor (kg) | 20.0±4.5 | 18.4±4.2 | 17.9±4.4 |
Ankle dorsiflexor (kg) | 22.9±5.3 | 21.3±4.9 | 20.0±4.3 |
Ankle Plantarflexor (times) | 23.0±3.4 | 17.5±8.3† | 17.4±7.4‡ |
All values are mean±SD. BMI = Body Mass Index, Mean foot length is the average value of both feet length. †Significant difference between the young and older group. ‡Significant difference between the young and BPPV group. *Significant difference between the older and BPPV group.
3.1Sensorimotor function
Significant differences in visual acuity (F = 5.510, p = 0.006) and visual contrast sensitivity (F = 7.838, p = 0.001) were noted among the three groups (Table 1). The young group had better visual acuity and visual contrast sensitivity than the older and BPPV groups. Significant differences were also noted in plantar cutaneous sensitivity over the distal end of the metatarsals of the great and 5th toes and the heels among the three groups (great toe: F = 18.820, p < 0.001, 5th toe: F = 26.683, p < 0.001, heel: F = 14.006, p < 0.001; Table 1). The young group showed better plantar cutaneous sensitivity than the other two groups over the three sites. The BPPV group showed better plantar cutaneous sensitivity than the older group over the distal end of the metatarsals of the great and 5th toes. In addition, the young group had significantly larger hip flexor (F = 7.482, p = 0.001) and ankle plantarflexor (X2 = 11.453, p = 0.003) strength than the other two groups (Table 1).
3.2Standing balance
MANOVA showed significant main effects of group (Phase I: F = 3.963, p = 0.001; Phase II: F = 4.335, p < 0.001; Phase III: F = 3.885, p = 0.001; Fig. 1) and condition (Phase I: F = 15.140, p < 0.001; Phase II: F = 8.593, p < 0.001; Phase III: F = 2.756, p = 0.017; Fig. 1) in all three phases.
Fig.1
(A) AP, (B) ML, and (C) total sway path of the three phases of EO, static image, and moving visual scenes condition in the three groups. All values are mean values in % foot length. †Significant difference between older and young group. ‡Significant difference between BPPV and young group. *Significant difference between Static image and EO condition. ¥Significant difference between Moving visual scene and EO condition. §Significant difference between Moving visual scene and Static image condition.
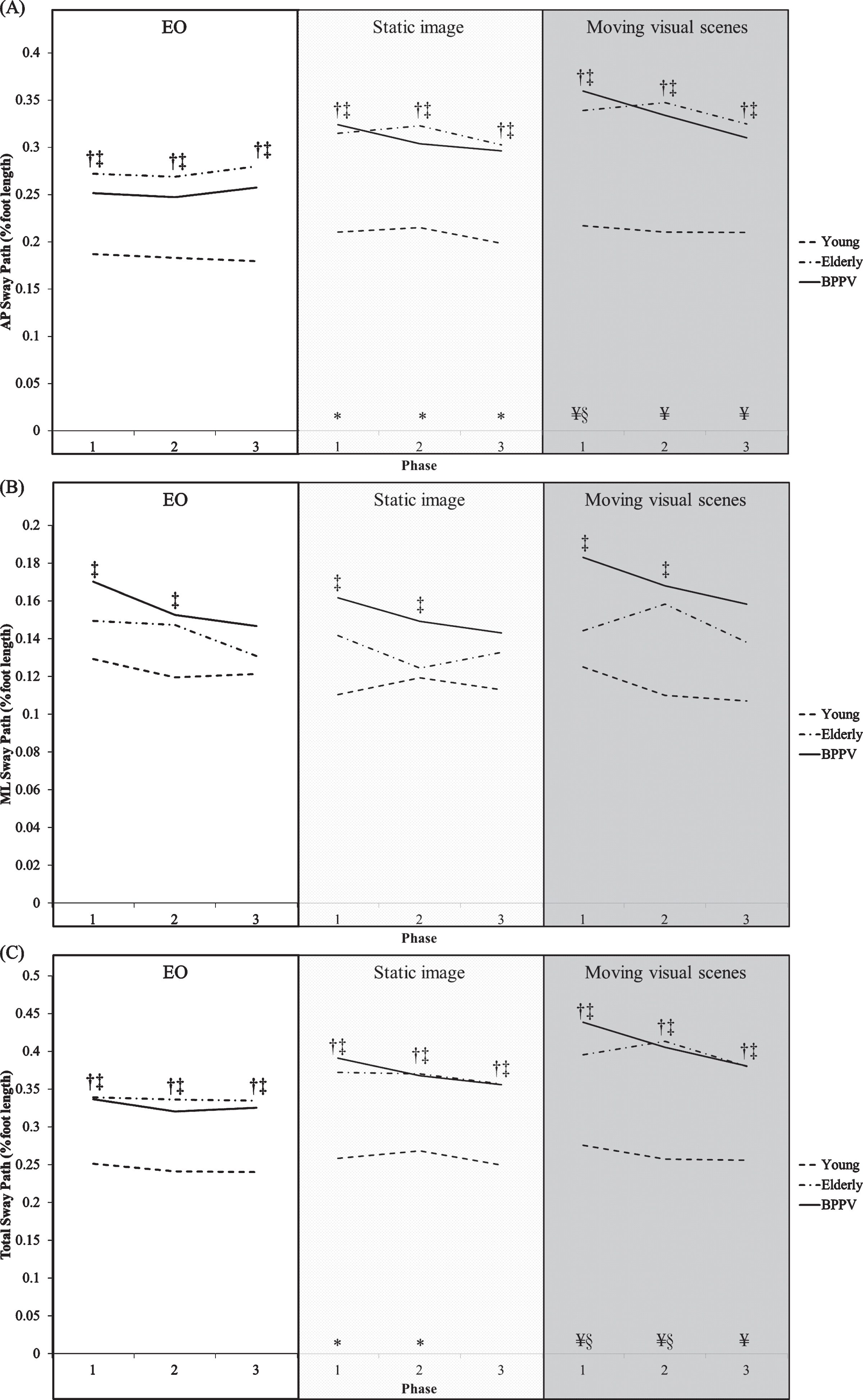
Post hoc test showed that both the older and BPPV group demonstrated significantly larger AP and total sway path than the young group in all three phases of all the three conditions (Fig. 1). The BPPV group also demonstrated significantly larger ML sway path than the young group in Phase I and II of all the three conditions (Fig. 1). No significant difference in AP, ML and total sway path was found between BPPV and older group in all three phases of all the three conditions.
Comparing the AP sway path among the three conditions, all three groups showed significantly larger AP sway path in both the static image and moving scenes condition than the EO condition in all three phases, and significantly larger AP sway path in the moving scenes condition than the static image condition in Phase I (Fig. 1). In addition, when comparing to the EO condition, all three groups showed significantly larger total sway path in the moving scenes condition in all three phases and in the static image condition in Phase I and II (Fig. 1). The total sway path in the moving scenes condition was also significantly larger than in the static image condition in Phase I and II in all three groups (Fig. 1). No significant difference in ML sway path among the three conditions was found in all three groups.
4Discussion
This study examined the influence of visual stimulation on postural stability in patients with BPPV before treatment with canalith repositioning maneuvers and older adults within different time phases, compared with healthy young adults. Overall, both the BPPV patients and older adults had larger AP and total postural sway than the young group in all conditions. The BPPV patients also exhibited larger ML postural sway in the first two phases of all conditions than the young group. Furthermore, both the static image and moving visual scenes condition affected the postural stability in the AP direction and total postural sway. The influence of the static image was mainly in the first two phases, whereas the influence of the moving visual scenes lasted throughout the three phases.
Previous studies found that the number of hair cells and neurons in the vestibular system significantly decrease in older adults over 50 years old [25, 37]. This decrease might result in age-related deterioration in vestibular function in the older population. The age-related peripheral vestibular function deterioration could further affect the processing of visual, vestibular, and somatosensory inputs in the central nervous system, and motor outputs, which is an essential element in standing postural control [45]. Combined with muscle strength decline and neural processing slowness due to aging may further lead to a balance deficit [21, 43]. It was also reported that healthy older adults rely more on visual information during standing postural control, compared with healthy young adults [10, 15]. Thus, older adults may show postural instability when there is a visual disturbance, such as movement of the visual scene, or when there is no visual reference, such as wearing goggles covering the whole visual field.
The aging effect might not be a main factor influencing postural control in the BPPV patients. Inspecting possible factors that could affect balance performance, the BPPV patients in our study had better plantar cutaneous sensitivity than the older adults, but similar visual acuity, visual contrast sensitivity, and lower extremity muscle strength to the older adults. These vision and cutaneous sensitivity differences could partly explain the increased postural sway in the AP direction in patients with BPPV compared with the young adults, but similar AP sway path to the older adults. However, the increased ML postural sway in the patients with BPPV, which was not found in the older adults, might indicate that such vestibular dysfunction might further decrease postural stability, especially in the coronal plane. Lateral stability is crucial when using a stepping strategy for balance recovery after external perturbations [39], and highly associated with number of falls [26, 27]. Further studies are needed to determine if the increased ML postural sway would be related with a higher risk of falling for patients in the active phase of BPPV.
There are two possible reasons that might cause such increased postural sway in patients with BPPV. First, the vestibular signal generated between the two vestibular systems might be imbalanced because of the otoconia falling into the semicircular canal in only one side and producing abnormal vestibular signals [11]. This could further impair the vestibulospinal tracts output and thus influence balance control. Second, a majority of patients with BPPV often complain of a feeling of disequilibrium, especially in an upright position. Such self-perceived postural instability might also make postural control more difficult for patients with BPPV.
With regard to the visual stimuli, watching the moving visual scenes via the goggles was intended to create an illusion of self-movement and to cause conflicting sensory inputs, and thus examine whether postural control would be influenced by the visual disturbance. The young adults in our study showed larger postural sway during the visual disturbance than the EO condition, which is consistent with previous findings that visual motion stimuli induce postural adjustments [23]. Furthermore, as patients with peripheral or central vestibular dysfunction were found to show a higher level of visual dependence than healthy controls [7, 18], patients with BPPV were supposed to exhibit larger postural sway than healthy young controls when there were conflicting sensory inputs caused by visual interference. Our study further found that the patients with BPPV had a similar amount of postural sway as the older adults when watching the moving visual scenes. When available sensory inputs, such as proprioception for older adults and vestibular for BPPV patients, are unable to provide precise information, vision becomes a relatively more reliable sensory input during postural control. Both patients with BPPV in the active phase and older adults might have re-weighted the sensory inputs to be more visually dependent when maintaining upright stance [42].
In addition, the results demonstrated that when watching the moving visual scenes or a static image via the goggles, there was a trend showing that the amount of postural sway decreased with time, especially in the patients with BPPV. The patients with BPPV had larger postural sway in the first phase, but decreased postural sway in the second and third phases. This finding might suggest that patients with BPPV change their balance control strategy to react to the initially increased postural sway when there is a visual disturbance. It was found that when one could anticipate the influence of perturbation, the central nervous system would regulate the muscle activities to counteract the destabilizing effect of the perturbations [41]. Therefore, the patients might use a strategy to stiffen their body for maintaining a static standing posture and thus showed a decreasing trend of postural sway. However, this strategy would reduce the flexibility of the body to react to perturbations [14], and might increase the risk of falling.
5Conclusion
The findings of this study suggest that patients with BPPV might have become more visually dependent when maintaining upright stance in the active phase of BPPV, similar to older adults. Increased postural sway in both the AP and ML directions in patients with BPPV could possibly place the patients in a higher risk for falling. In order to counteract such increased postural sway, they may stiffen their body to maintain postural stability. Therefore, postural stability training in patients with BPPV might help them adopt appropriate balance control strategies when facing an external perturbation.
Acknowledgments
The authors thank the Ministry of Science and Technology, Taiwan for supporting this study (MOST 103-2314-B-006-082-), and Yi-Ting Chen and Yu-Ting Liao for help in data collection.
References
[1] | Allum J.H. and Ledin T. , Recovery of vestibulo-ocular reflex-function in subjects with an acute unilateral peripheral vestibular deficit, J Vestib Res 9: ((1999) ), 135–144. |
[2] | Balaguer Garcia R. , Pitarch Corresa S. , Baydal Bertomeu J.M. and Morales Suarez-Varela M.M. , Static posturography with dynamic tests. Usefulness of biomechanical parameters in assessing vestibular patients, Acta Otorrinolaringol Esp 63: ((2012) ), 332–338. |
[3] | Bardy B.G. , Oullier O. , Lagarde J. and Stoffregen T.A. , On perturbation and pattern coexistence in postural coordination dynamics, J Mot Behav 39: ((2007) ), 326–336. |
[4] | Black F.O. , Wall C. 3rd and Nashner L.M. , Effects of visual and support surface orientation references upon postural control in vestibular deficient subjects, Acta Otolaryngol 95: ((1983) ), 199–201. |
[5] | Blatt P.J. , Georgakakis G.A. , Herdman S.J. , Clendaniel R.A. and Tusa R.J. , The effect of the canalith repositioning maneuver on resolving postural instability in patients with benign paroxysmal positional vertigo, Am J Otol 21: ((2000) ), 356–363. |
[6] | Bronstein A.M. , Suppression of visually evoked postural responses, Exp Brain Res 63: ((1986) ), 655–658. |
[7] | Bronstein A.M. , Visual vertigo syndrome: clinical and posturography findings, J Neurol Neurosurg Psychiatry 59: ((1995) ), 472–476. |
[8] | Bronstein A.M. , Oxford textbook of vertigo and imbalance, Oxford University Press, Oxford, UK, (2013) . |
[9] | Bronstein A.M. and Lempert T. , Dizziness: A Practical Approach to Diagnosis and Management, Cambridge University Press, Cambridge, (2007) . |
[10] | Bugnariu N. and Fung J. , Aging and selective sensorimotor strategies in the regulation of upright balance, J Neuroeng Rehabil 4: ((2007) ), 19. |
[11] | Cohen H.S. and Kimball K.T. , Effectiveness of treatments for benign paroxysmal positional vertigo of the posterior canal, Otol Neurotol 26: ((2005) ), 1034–1040. |
[12] | Cohen H.S. , Mulavara A.P. , Peters B.T. , Sangi-Haghpeykar H. and Bloomberg J.J. , Standing balance tests for screening people with vestibular impairments, Laryngoscope 124: ((2014) ), 545–550. |
[13] | Cohen H.S. and Sangi-Haghpeykar H. , Canalith repositioning variations for benign paroxysmal positional vertigo, Otolaryngol Head Neck Surg 143: ((2010) ), 405–412. |
[14] | Di Giulio I. , Baltzopoulos V. , Maganaris C.N. and Loram I.D. , Human standing: does the control strategy preprogram a rigid knee? J Appl Physiol (1985) 114: ((2013) ), 1717–1729. |
[15] | Faraldo-Garcia A. , Santos-Perez S. , Crujeiras-Casais R. , Labella-Caballero T. and Soto-Varela A. , Influence of age and gender in the sensory analysis of balance control, Eur Arch Otorhinolaryngol 269: ((2012) ), 673–677. |
[16] | Gananca F.F. , Gazzola J.M. , Gananca C.F. , Caovilla H.H. , Gananca M.M. and Cruz O.L. , Elderly falls associated with benign paroxysmal positional vertigo, Braz J Otorhinolaryngol 76: ((2010) ), 113–120. |
[17] | Guerraz M. and Bronstein A.M. , Mechanisms underlying visually induced body sway, Neurosci Lett 443: ((2008) ), 12–16. |
[18] | Guerraz M. , Yardley L. , Bertholon P. , Pollak L. , Rudge P. , Gresty M.A. and Bronstein A.M. , Visual vertigo: symptom assessment, spatial orientation and postural control, Brain 124: ((2001) ), 1646–1656. |
[19] | Herdman S.J. , Vestibular Rehabilitation, F. A. Davis Company, Philadelphia, PA, 2007. |
[20] | Hislop H.J. and Montgomery J. , Daniels and Worthingham’s muscle testing: techniques of manual examination, Saunders Elsevier, St. Louis, Mo, 2007. |
[21] | Horak F.B. , Shupert C.L. and Mirka A. , Components of postural dyscontrol in the elderly: a review, Neurobiol Aging 10: ((1989) ), 727–738. |
[22] | Kentala E. and Pyykko I. , Vertigo in patients with benign paroxysmal positional vertigo, Acta Otolaryngol Suppl 543: ((2000) ), 20–22. |
[23] | Lestienne F. , Soechting J. and Berthoz A. , Postural readjustments induced by linear motion of visual scenes, Exp Brain Res 28: ((1977) ), 363–384. |
[24] | Levenson J.H. and Kozarsky A. , Visual Acuity, in: Clinical Methods: The History, Physical, and Laboratory Examinations, H.K. Walker, W.D. Hall andJ.W. Hurst eds., Butterworths, Boston, 1990. |
[25] | Lopez I. , Ishiyama G. , Tang Y. , Tokita J. , Baloh R.W. and Ishiyama A. , Regional estimates of hair cells and supporting cells in the human crista ampullaris, J Neurosci Res 82: ((2005) ), 421–431. |
[26] | Lord S.R. , Rogers M.W. , Howland A. and Fitzpatrick R. , Lateral stability, sensorimotor function and falls in older people, J Am Geriatr Soc 47: ((1999) ), 1077–1081. |
[27] | Maki B.E. , Holliday P.J. and Topper A.K. , A prospective study of postural balance and risk of falling in an ambulatory and independent elderly population,M, J Gerontol 49: ((1994) ), 72–84. |
[28] | McDonnell M.N. and Hillier S.L. , Vestibular rehabilitation for unilateral peripheral vestibular dysfunction, Cochrane Database Syst Rev 1: ((2015) ), CD005397. |
[29] | Mizukoshi K. , Watanabe Y. , Shojaku H. , Okubo J. and Watanabe I. , Epidemiological studies on benign paroxysmal positional vertigo in Japan, Acta Otolaryngol Suppl 447: ((1988) ), 67–72. |
[30] | Muir S.W. , Berg K. , Chesworth B.M. , Klar N. and Speechley M. , Modifiable Risk Factors Identify People Who Transition from Non-fallers to Fallers in Community-Dwelling Older Adults: A Prospective Study, Physiother Can 62: ((2010) ), 358–367. |
[31] | Mulavara A.P. , Cohen H.S. , Peters B.T. , Sangi-Haghpeykar H. and Bloomberg J.J. , New analyses of the sensory organization test compared to the clinical test of sensory integration and balance in patients with benign paroxysmal positional vertigo, Laryngoscope 123: ((2013) ), 2276–2280. |
[32] | Nair M.A. , Mulavara A.P. , Bloomberg J.J. , Sangi-Haghpeykar H. and Cohen H.S. , Visual dependence and spatial orientation in benign paroxysmal positional vertigo, J Vestib Res 27: ((2018) ), 279–286. |
[33] | Nedzelski J.M. , Barber H.O. and McIlmoyl L. , Diagnoses in a dizziness unit, J Otolaryngol 15: ((1986) ), 101–104. |
[34] | Neuhauser H.K. , von Brevern M. , Radtke A. , Lezius F. , Feldmann M. , Ziese T. and Lempert T. , Epidemiology of vestibular vertigo: a neurotologic survey of the general population, Neurology 65: ((2005) ), 898–904. |
[35] | Peterka R.J. , Sensorimotor integration in human postural control, J Neurophysiol 88: ((2002) ), 1097–1118. |
[36] | Peterka R.J. , Statler K.D. , Wrisley D.M. and Horak F.B. , Postural compensation for unilateral vestibular loss, Front Neurol 2: ((2011) ), 57. |
[37] | Richter E. , Quantitative study of human Scarpa’s ganglion and vestibular sensory epithelia, Acta Otolaryngol 90: ((1980) ), 199–208. |
[38] | Ring C. , Nayak U.S. and Isaacs B. , The effect of visual deprivation and proprioceptive change on postural sway in healthy adults, J Am Geriatr Soc 37: ((1989) ), 745–749. |
[39] | Rogers M.W. , Hedman L.D. , Johnson M.E. , Cain T.D. and Hanke T.A. , Lateral stability during forward-induced stepping for dynamic balance recovery in young and older adults,M, J Gerontol A Biol Sci Med Sci 56: ((2001) ), 589–594. |
[40] | Schlick C. , Schniepp R. , Loidl V. , Wuehr M. , Hesselbarth K. and Jahn K. , Falls and fear of falling in vertigo and balance disorders: A controlled cross-sectional study, J Vestib Res 25: ((2016) ), 241–251. |
[41] | Schmid M. and Sozzi S. , Temporal features of postural adaptation strategy to prolonged and repeatable balance perturbation, Neurosci Lett 628: ((2016) ), 110–115. |
[42] | Speers R.A. , Kuo A.D. and Horak F.B. , Contributions of altered sensation and feedback responses to changes in coordination of postural control due to aging, Gait Posture 16: ((2002) ), 20–30. |
[43] | Sturnieks D.L. , St George R. and Lord S.R. , Balance disorders in the elderly, Neurophysiol Clin 38: ((2008) ), 467–478. |
[44] | Talebi H. , Karimi M.T. , Abtahi S.H. and Fereshtenejad N. , Static Balance in Patients with Vestibular Impairments: A Preliminary Study, Scientifica (Cairo) 2016: ((2016) ), 6539858. |
[45] | Teasdale N. , Stelmach G.E. and Breunig A. , Postural sway characteristics of the elderly under normal and altered visual and support surface conditions, J Gerontol 46: ((1991) ), B238–244. |
[46] | Verbaken J.H. and Johnston A.W. , Population norms for edge contrast sensitivity, Am J Optom Physiol Opt 63: ((1986) ), 724–732. |