Design of an ergonomic gestural interface for professional road cycling
Abstract
BACKGROUND:
Connected bike computers can support professional cyclists in achieving better performances but interacting with them requires taking their hands off the handlebar compromising focus and safety.
OBJECTIVE:
This research aims at exploring the design of an ergonomic interface based on micro-gestures that can allow cyclists to interact with a device while holding the handlebar.
METHODS:
Three different studies were conducted with seven professional cyclists adopting the gesture-elicitation technique. One study aimed at eliciting free micro-gestures; a second to evaluate gestures recognizable with a smart glove; the last focused on the gestures recognized through an interactive armband.
RESULTS:
The analysis of the micro-gestures elicited during these studies allowed producing a first set of guidelines to design gestural interfaces for drop-bars (a specific type of handlebar for road bikes). These guidelines suggest which fingers to use and how to design their movement in order to provide an ergonomic interface. It also introduces the principle of symmetry for the attribution of symbols to symmetric referents. Finally, it provides suggestions on the design of the interactive drop-bar.
CONCLUSIONS:
The guidelines provided in this paper can support the design of gestural interfaces for professional cyclists that can enhance performance and increase safety.
1Introduction
In sports, there is a constant tension toward continuous improvement and breaking world records [1]. Indeed, performance in road bicycle racing improved by 6.38% in the last 20 years [2]. The main factors enabling the enhancement of performance over time consist of better coaching, nutrition, talent identification and training [1]. Another additional factor, which is less understood, is technology [1]. It has been shown that technology in cycling played a major role in improving performance, at least in particular specialties [1]. However, technology encompasses different disciplines; for instance, advances in materials for the construction of lighter bikes and the progress in optimization of aerodynamics greatly impacted performance. Another emerging technological domain applied to cycling is the development of information and communication systems; a popular example is the recent introduction of two-way radios allowing communication with coaches and team-mates [2]. Another important example concerns the quantification and tracking of performance during training and racing through the use of pervasive sensors [3–5]. These sensors are often used for post-training analysis but cyclists are also avid users of systems that can display immediate feedback during the athletic performance [6]. There are multiple types of system that can track the cyclist’s performance and provide immediate feedback; the most popular type is the so-called connected bike computer. Other types include different kinds of wearable systems, such as smart watches and smart glasses. Semi-professional and professional athletes training for road cycling tend to prefer the connected bike computers over the other solutions because the most advanced versions do not just integrate the GPS but they support also the connection to multiple sensors, from the heart-rate monitor to the power meter; in particular, the latter plays a crucial role for the improvement of these athletes’ training. Connected bike computers are usually placed in the middle of the handlebar on a specific mounting system and their interface is composed of a display with some buttons or a touch-sensitive screen for the interaction. Unfortunately, both pressing a button or tapping on the touch-sensitive screen to interact with the computer require the cyclist to shift her/his focus from the road to the device and, most importantly, to take at least one hand off the handlebar to physically touch the device. This action can be dangerous and can distract the athlete, who has to keep her/his focus in order to maintain good performances. A valid alternative to the aforementioned classical interfaces can be found in the gestural interaction, especially via gestures that can be performed at a distance from the device, as already studied in other similar contexts, e.g., automotive [7]. In particular, it is worth exploring the possibilities of developing a system that can enable the cyclist to perform ergonomic micro-gestures while keeping her/his hands on the handlebar [8]. Such a solution allowing the cyclist to interact with a specific device, i.e., a connected bike computer, without having to release her/his grasp on the handlebar can enhance performance and, most importantly, increase safety. The cyclist can, indeed, enhance her/his performance because the distraction is reduced and she/he can keep her/his focus on the road and on her/his effort while just glancing at the screen to read the information without having to coordinate her/his movement for pressing the button or tapping on the correct area of the touchscreen; moreover, the movement to reach the connected bike computer requires the cyclist to change her/his posture, which can compromise her/his aerodynamic posture. This solution allows also increasing safety because the athlete can keep both her/his hands on the handlebar while interacting with a device, which translates in a better balance and in a shorter reaction time in case of accident.
Although it is clear that an interface enabling cyclists to interact with their connected bike computers through gestures performed directly on the handles would increase performance and safety, there is still no work exploring the design of such interaction. This paper presents a gesture elicitation study aiming at discovering the most convenient and ergonomic gestures that professional cyclists would like to perform in order to interact with a screen on the handlebar. In particular, three different sessions were performed to analyze the gestures that can be performed with a smart glove, with a smart armband and the design of the ideal interface based on the, so-called, micro-gestures. The discussion of the results of these elicitation-studies provides a first set of guidelines for the design of micro-gestures with reference to the fingers to use and type of movement to perform. Moreover, it shows some design principles concerning the interactive zones of a handlebar and the concept of symmetry for the association symbols to symmetric referents.
2Related work
There are growing numbers of smartphone applications and devices specifically designed for cyclists. Many of them are designed to be used just before or after the cycling activity. This approach is referred at as “stop-to-interact” paradigm [9], which is sufficient for some applications but recent research shows that for cyclists it is becoming more and more important to keep interacting also during the cycling activity [10]. The possibility to interact with digital content through smartphones or specific devices without stopping the cycling activity was defined in [11] as “truly mobile interaction” and, in order to enable such experiences that support locomotion in a seamless manner, the interaction design “has to respect the distinctive nature of cycling as a mode of transport and needs to carefully interweave moments of interaction with it” [12]. Since the interaction with button and touchscreens placed on the devices, which are either placed in the middle of the handlebar or worn on the wrist, require the users to move their hands off the handles, researchers started investigating gestures as a means for a more convenient interaction form. It is, indeed, possible to find in the scientific literature multiple examples of gestural interfaces to augment the interaction possibilities of cyclists without having to interrupt their locomotion; for instance, Dancu et al. presented a system allowing to use “natural” arm gestures to control a signaling system [13]. The aforementioned example of system requires the cyclist to take her/his hand off the handlebar in order to perform the gestures that can control the projected signaling interface. Another work explored a similar solution to support the communication in groups of cyclists through the display of information on the LEDs sewn on their t-shirts but with a fundamental difference: the system was designed to recognize gestures performed while grasping the handlebar [14]. The possibility of interacting while keeping the correct posture with both hands on the handlebar is important because, as highlighted in the previous section, it allows increasing safety and, for athletes, also performance.
The previous examples present two different interfaces that were designed by researchers without the direct involvement of end users in the process. This approach can lead to the creation of an interface that is not ideal for the final users since the set of gestures is arbitrarily designed by researchers without referring to what users perceive as simple to perform and remember. Participatory design, intended here as a creative activity shared between researchers and end users who work together on equal grounds in the design development process [15], allows co-creating with end users a gesture set that can increase user’s acceptance and enhance the ergonomics of the interaction. In particular, the user elicitation technique, which is explained in the next section, has been demonstrated to be an effective method for the design of gestures that respond to users’ needs in different contexts: e.g., interacting with tabletops [16], with smartphones [17] and with the steering wheel [18]. In our literature review, we found two studies that reportedly investigated through user elicitation the design of gestural interfaces for seamless interaction during the cycling activity. For instance, Hochleitner et al. created a gesture set with the movements that the users indicated as the most convenient to perform while cycling and analyzed the zones preferred for such interaction [19]; in particular, this second part of the study stated that users prefer to interact with their fingers directly on the handlebar. This result confirms the popular choice made by many cyclists, from amateurs to professionals, to place their connected bike computer and smartphones in the center of the handlebar. The second study we mentioned concerns Tan et al.’s work, which explored the applicability for micro-gestures performed directly on the handlebar by creating a glove prototype and devising a taxonomy with 10 possible micro-gestures [20]. This work is particularly interesting because it represents the sole example of co-designed gestural interface for on-bike interaction that focuses also on safety concerns, such as reducing the attention shift and minimizing the hands’ displacement to interact with smart devices while cycling. Unfortunately, these micro-gestures were specifically designed to be performed on a flat handlebar and this implies the impossibility of applying them to handlebars with different shapes. Indeed, several types of bicycle handlebars exist (e.g., bullhorn, butterfly, riser, BMX, porteur, cruiser, condorino, moustache etc.) and they are characterized by substantially different shapes, which require specific hands and command positions. In road bicycle racing, the most traditional type of handlebar is the dropped handlebar, also called drop-bar (Fig. 1), which ensures an optimal posture with reference to aerodynamics [21]. The shape of the drop-bar is peculiar and, as depicted in Fig. 2, it allows for four different hand postures: the hoods, the hooks, the drops and the tops [22]. The hoods position refers to keeping hands on top of the rubbery brake hoods, wrapped around them firmly. The drops position involves holding on to the parts of the bars that curve; if the cyclist’s hands is behind the brake levers, then it is called hooks. The tops position consists of holding both hands on the tops, the flat part of the handlebar. The recommended positions to assume on road bikes to find the right balance between ergonomics and aerodynamics are with the hands on the brake hoods and the arms slightly flexed, the torso should flex to 45° in relation to the top tube and with the hands in the drops, the torso and top tube angle should be about 30° [23]. In this paper, we want to fill the gap in the current scientific literature presenting a study that investigates which micro-gestures professional athletes would like to perform in order to interact with a device while cycling and using a drop-bar.
Fig. 1
Picture showing the five different zones of a handlebar for road bikes, called drop-bar: tops, hoods, ramps, hooks and drops.
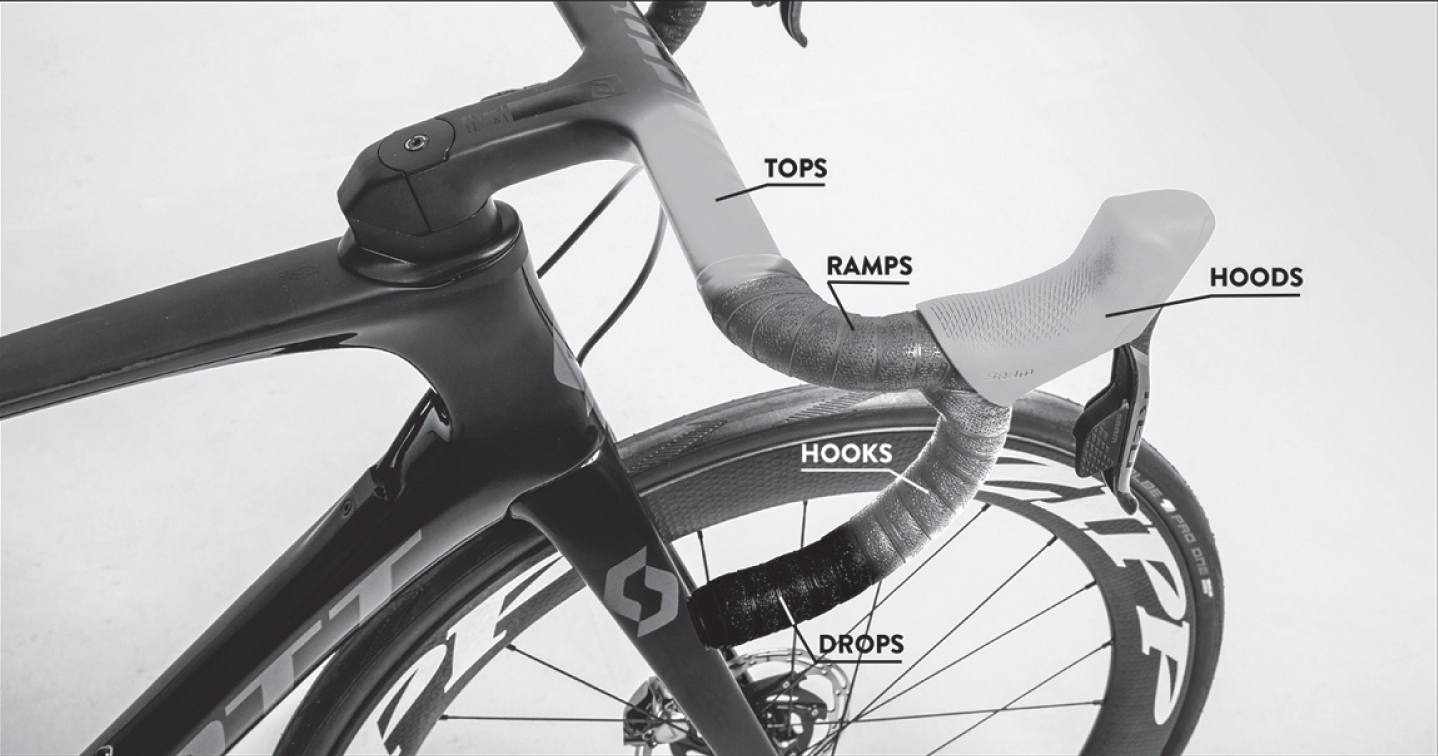
Fig. 2
This image presents the five possible hand positions on a drop-bar: tops, hoods, ramps, hooks and drops positions.
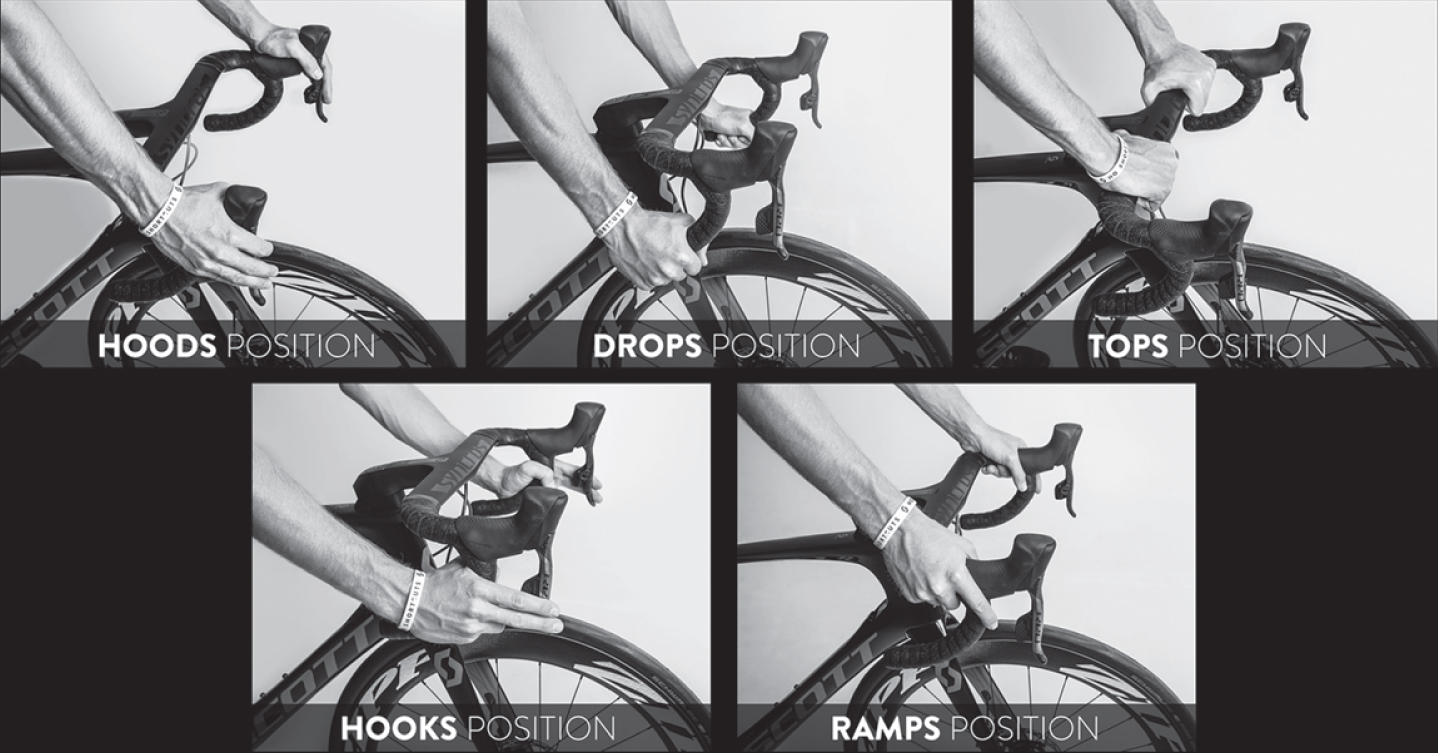
3Methodology
In the field of participatory design, a technique called “gesture elicitation” emerged to become one of the most popular approaches for the design of gestural interaction. Designing gestures following this technique implies involving directly the end users, which are “individually shown the desired effect of an action (called a referent) and asked to propose the gesture (called a symbol) that would bring that effect about” [24]. After collecting all the answers of the users participating in the study, the researchers have to formalize and reconcile the different elicited symbols in order to create a single canonical gesture set that will be adopted for the interface design.
This research work is composed of three different gesture-elicitation studies: the first for the design of free micro-gestures, i.e., without any technological constraints, following the standard methodology; the second study concerned the evaluation of gestures using a smart glove; the third for the recognition of gestures using an interactive armband. The last two studies are not proper elicitation studies meaning that they provide users with a set of available gestures (symbols) and they have just to choose the ones to assign to the presented referents. The setup of the experiment and the test participants were the same for all of the three studies. The setup was composed of a road bike with drop-bars in front of a camera to record the performed movements at the Scott Sports SA headquarters (Fig. 3). The participants were seven professional or semi-professional cyclists (aged between 29 and 37 years, all males), who voluntarily took part in the experiment. All of them are right-handed and have several years of experience as road cyclists. They were asked to sit on a road bike held with a stand, and to assume their preferred posture and hand position on the drop-bars. The actual order of the studies conducted with the participants was: the elicitation of free micro-gestures was first in order to avoid a possible bias due to the gestures imposed by the systems used for the other two studies; the second and third studies were selected randomly tossing a coin.
Fig. 3
Picture depicting the setup for the user-elicitation studies.
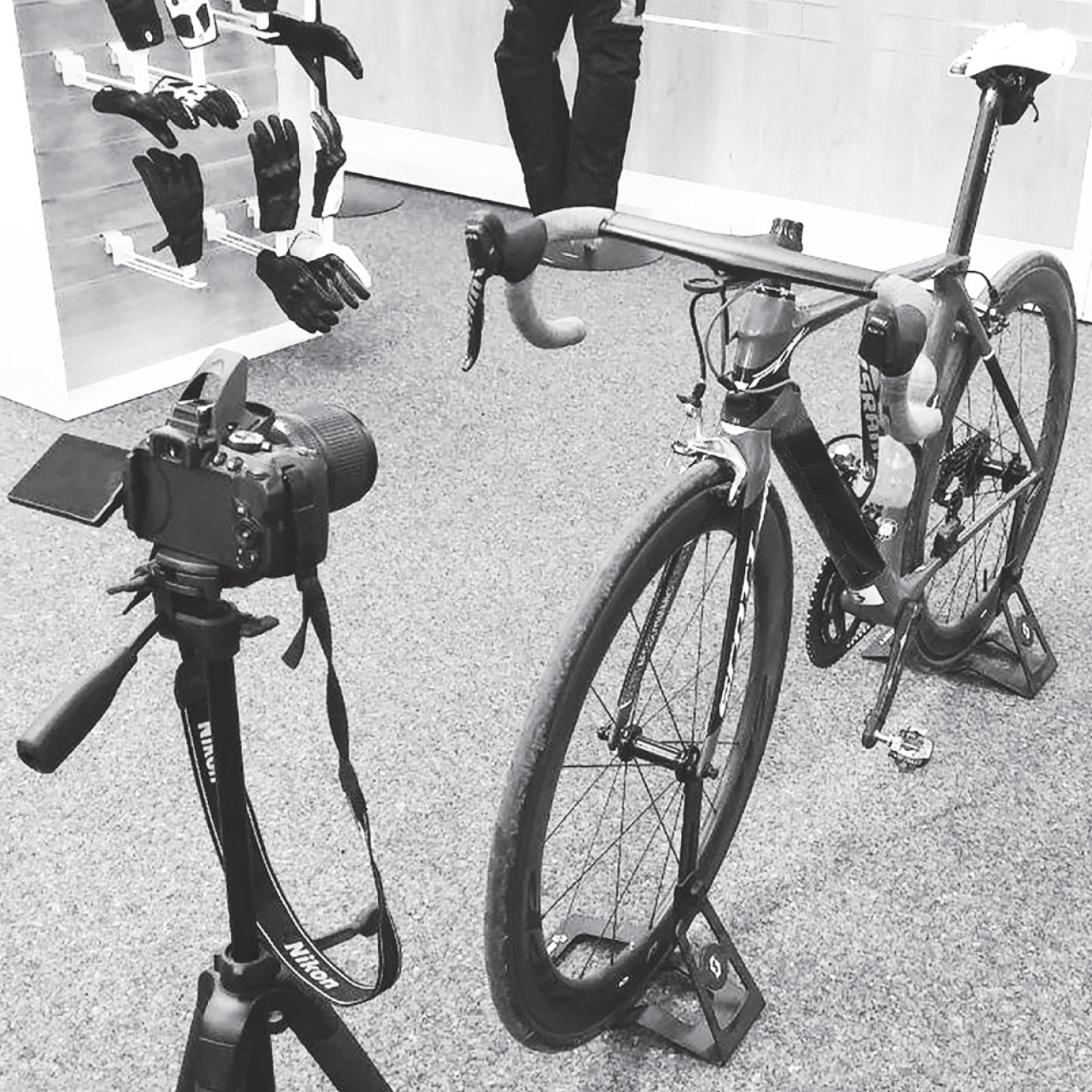
After the presentation of the objective of the study, the participants were shown the mobile application that it was possible to control with the gestures and this was done on a smartphone placed on the handlebar. In order to facilitate the retention of the different actions during the experiment, the list of possible actions was displayed on a laptop computer in front of them. This is the list of possible actions within the application: 1) Swipe Left, 2) Swipe Right, 3) Open Camera, 4) Close Camera, 5) Switch Camera, 6) Take Picture, 7) Zoom In, 8) Zoom Out. These actions were used as referents during the three elicitation studies.
The tests were designed in accordance with European and National legislation regarding user safety and privacy.
3.1Free micro-gestures
In this first study, the users were asked to elicit a gesture for every referent in complete freedom: the cyclists could perform any gesture as symbol to control the application remotely without considering any technological constraints or references. Users could choose to perform gestures anywhere on the handlebar, with either hand and in any valid way. A gesture was valid if well-defined and if it was performed while grasping the handlebar. This last criterion constrained all the elicited gestures to fall into the category of micro-gestures.
3.2Gestures with smart glove
One of the elicitation studies was conducted with the GoGlove, which is a smart glove that allows users to control the connected Bluetooth-devices (e.g., smartphones) without touching them [25]. This glove integrates a magnet on the thumb and specific sensors on the fingertips of index, middle and ring fingers and one on the proximal phalange of the index finger, as depicted in Fig. 3. Therefore, the GoGlove allows recognizing only eight gestures based on the tap of the thumb with the other sensorized fingers, i.e., four single taps: thumb + index, thumb + middle, thumb + ring, thumb + index-phalange; four double taps: thumb + index, thumb + middle, thumb + ring, thumb + index-phalange. The participants were instructed to execute these gestures while holding the handlebar. The participants were allowed to use either hand.
In this gesture elicitation study, the symbols (these eight gestures) are already constrained by the technology and the users are asked to associate them to the afore-mentioned referents (the eight actions of the mobile applications).
3.3Gestures with interactive armband
This gesture-elicitation study was conducted with the Myo armband. This interactive armband, produced by Thalmic Labs and currently discontinued, is a wearable gesture and motion control device that lets the user take command of her/his phone, computer and software via Bluetooth [26]. This armband translates the muscles’ electrical activity into motion controls. It consists of eight rectangular blocks, linked together with an elastic rubber and to be worn directly against the skin, as shown in Fig. 5. This is because the electromyography-integrated sensors can read the electrical activity of muscles and detect hand gestures combined with inertial sensors that record the movements of the arm. The Myo armband is capable of recognizing five gestures at default, i.e., fist, double tap, finger spread, wave left and wave right. These gestures were performed while grasping the handlebar similarly to the vocabulary presented in [27], where the user was holding a steering wheel. Therefore, the fist gesture, which is an isometric contraction, was performed as a squeeze of the handlebar. The wave left and wave right were performed as wrist extension and wrist flexion. The double tap also was performed while holding the handlebar as in the study with the GoGlove. The finger extension remained unchanged.
Fig. 4
The GoGlove wearable device [28], which integrates sensors on fingertips for the recognition of single and double tap gestures and a Bluetooth module for wireless connection to other devices.
![The GoGlove wearable device [28], which integrates sensors on fingertips for the recognition of single and double tap gestures and a Bluetooth module for wireless connection to other devices.](https://ip.ios.semcs.net:443/media/wor/2020/66-4/wor-66-4-wor203238/wor-66-wor203238-g004.jpg)
Fig. 5
The Myo armand [29], which integrates electromyographic and inertial sensors for the recognition of five gestures, and a Bluetooth module for wireless connection to other devices.
![The Myo armand [29], which integrates electromyographic and inertial sensors for the recognition of five gestures, and a Bluetooth module for wireless connection to other devices.](https://ip.ios.semcs.net:443/media/wor/2020/66-4/wor-66-4-wor203238/wor-66-wor203238-g005.jpg)
In this study, similarly to the one with the smart glove, the participants had to associate to the previously presented referents, five symbols that were imposed by the technological choice. The participants were allowed to use either hand.
4Results
The results collected during the three different studies are presented in the following three subsections.
4.1Results with free micro-gestures
The cyclists have all preferred the hoods position, which refers to keeping hands on top of the rubbery brake hoods, wrapped around them firmly. As it is considered the most comfortable for cycling posture, all the micro-gestures were performed in this position.
During this study, every user had to elicit eight micro-gestures; therefore, in total there were 56 elicited micro-gestures. Figure 6 depicts how the participants used their hands to perform these micro-gestures, while Fig. 7 reports, for each finger, the number of micro-gestures it was involved in (considering that a single micro-gesture can use multiple fingers at the same time). The right hand was the most used with 33 out of 56 micro-gestures (60%), while the left hand alone was used just in 16 micro-gestures (28%), and both hands were used 7 times (12%). The most used finger was the index, which was involved in 31 out of 56 micro-gestures (55%), of which 19 times with the right hand. The second most used finger was the thumb with 23 occurrences (50%), of which 14 with the right hand alone. The middle finger was used 18 times (32%), the ring finger 14 (25%) and the little finger 12 (21%). The sum of the times the fingers were used is bigger than the total of micro-gestures (56) because multiple fingers could be used together in the same micro-gesture.
Fig. 6
Participants were allowed to use either hand during the micro-gesture elicitation study and this graph reports the frequency of use of their hands.
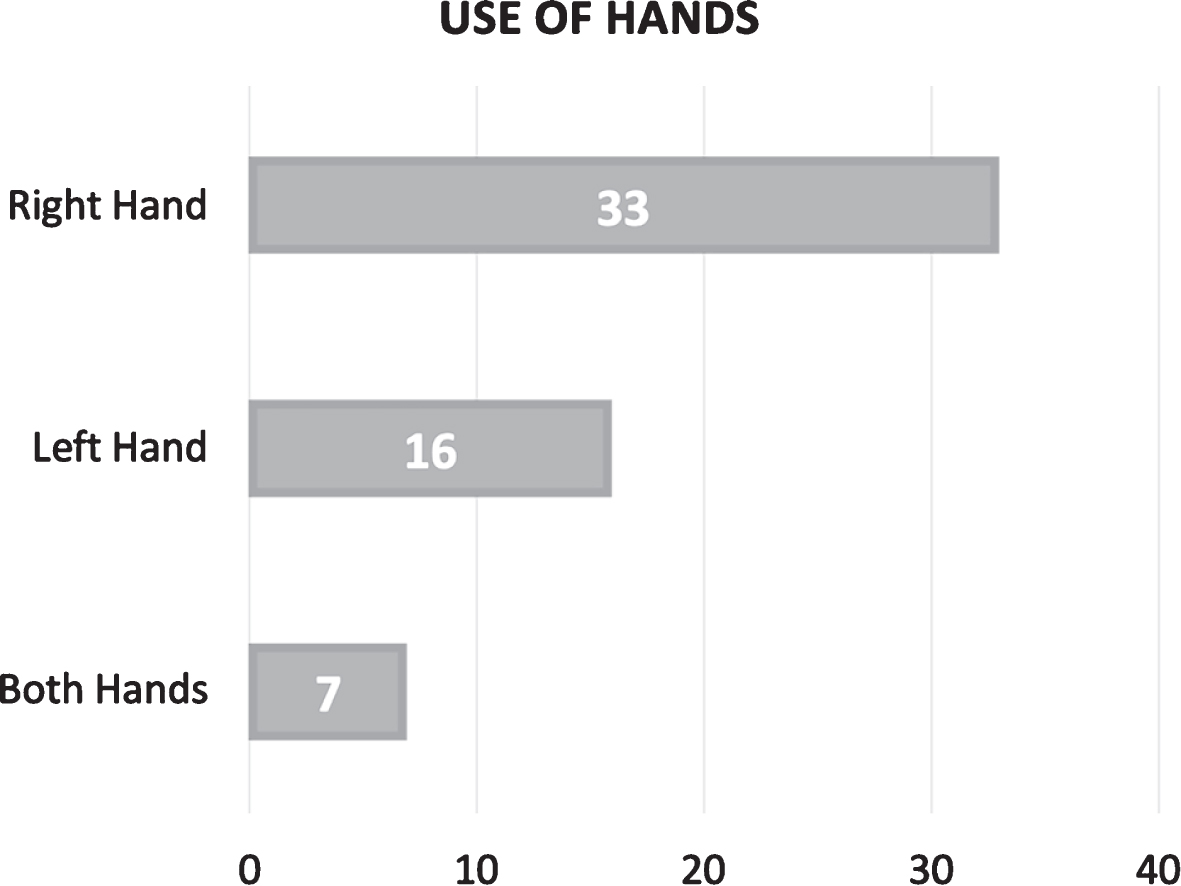
Fig. 7
This graph shows the frequency of use of each finger, also in combination with others, for each elicited micro-gesture and with reference to the hand.
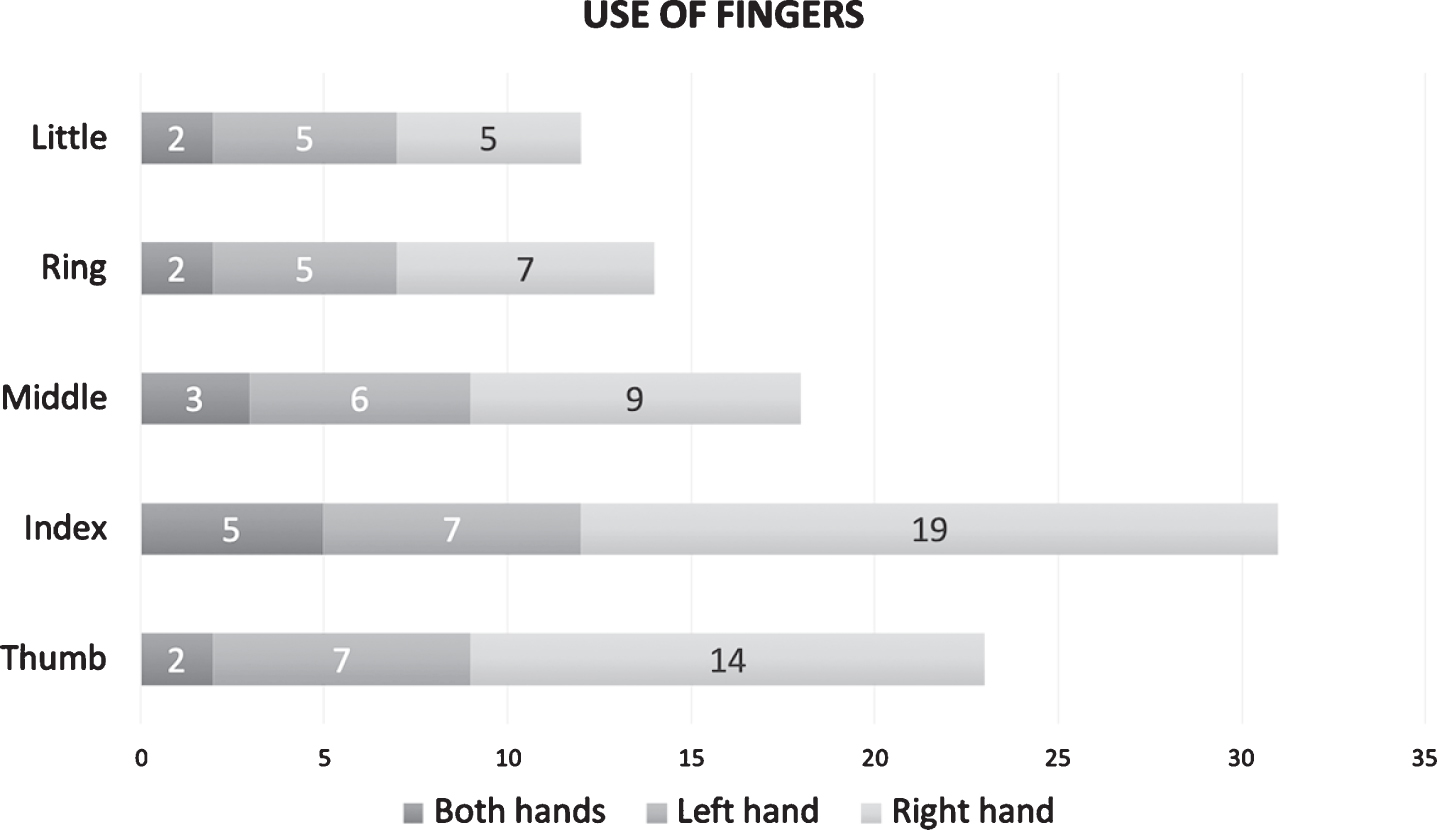
As shown in Fig. 8, the finger frequency changes when considering the number of micro-gestures performed with the exclusive use of one or a specific combination of multiple fingers. The most popular symbol concerns micro-gestures performed only with the thumb finger, which constituted the 28% of the total (16/56). Micro-gestures using only the index finger come in second and were 20% of the total (11/56). Micro-gestures using index, middle, ring and little fingers together were 14% of the total (8/56). Finally, symbols involving a combination of index and middle fingers represented the 11% of the total (6/56). The micro-gestures with these four fingers are relatively broad gestures but are considered safe by cyclists. They are characterized by the simultaneous movement of the four fingers in different directions. Other elicited micro-gestures represented the 27% of the total (15/56), which represent a miscellaneous of gestures that cannot constitute a separate category since they were hardly used.
Fig. 8
This graph reports the frequency of micro-gestures performed with the exclusive use of one or a specific combination of multiple fingers.
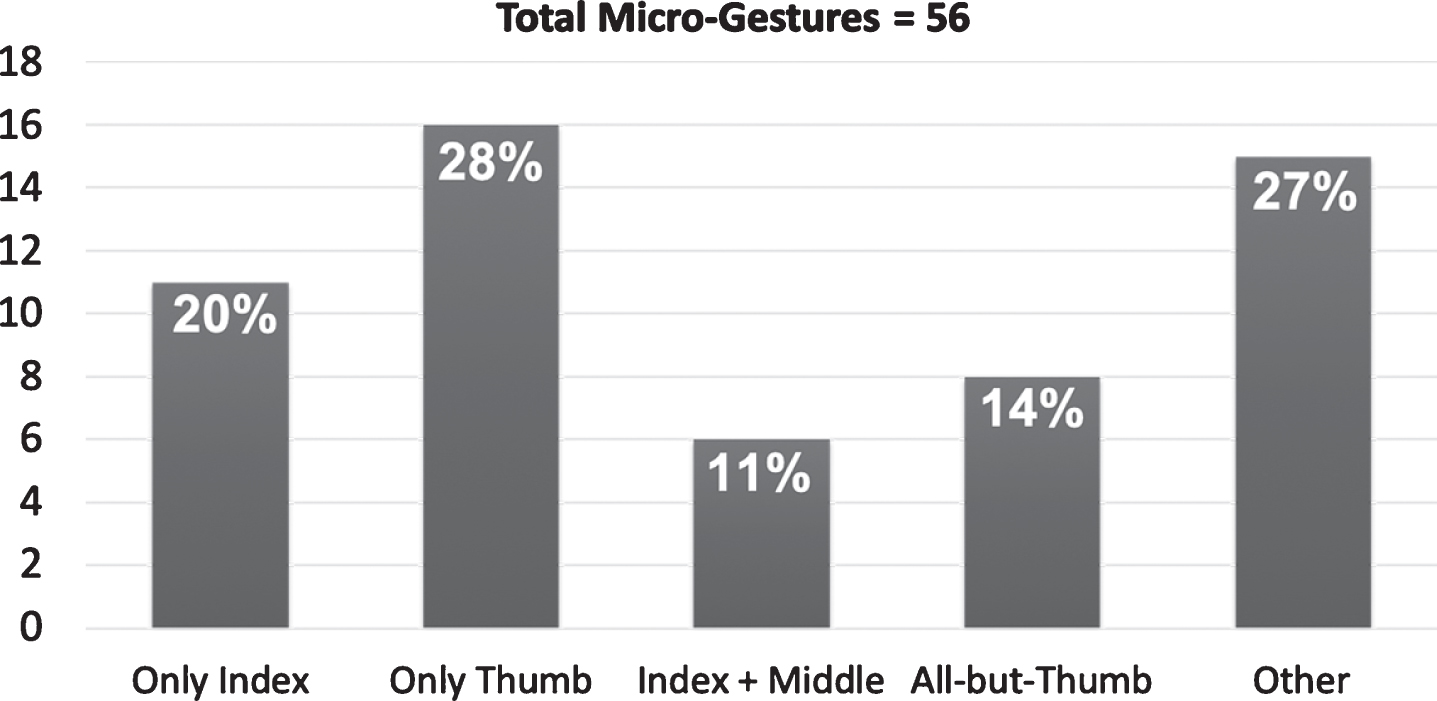
During this study, it emerged that all the micro-gestures can be classified by the type of movement into three categories:
1. Press micro-gesture: gestures in which one or more fingers tap in different handlebar’s parts.
2. Swipe micro-gesture: fingers like thumb or index are sliding on the break lever or on some portions of the handlebar.
3. Extension micro-gestures: the fingers are separated from the normal riding grip for a short period of time.
The most frequent micro-gestures are reported with reference to the categories previously described in Fig. 9. Neglecting the “other” group, which includes all the micro-gestures that were too rare to constitute a separate class, it is possible to note that pressing the thumb alone on a part of the handlebar was the most popular symbol among all the cyclists with 10 out of 56 (≅18%). The second most frequent micro-gesture involved pressing the index, with 9 out of 56 occurrences (16%). Three different gestures are equally placed as the third most elicited micro-gesture with 5 out of 56 preferences (≅9%), which are: thumb extension, index and middle fingers extended together, and the extension of all fingers but the thumb (called hereafter all-but-thumb). A detailed description of all the free micro-gestures elicited in this study can be found in [28].
Fig. 9
This graph shows the frequency of the unique micro-gestures elicited during this study with reference to the category of movement, i.e., press, swipe and extension.
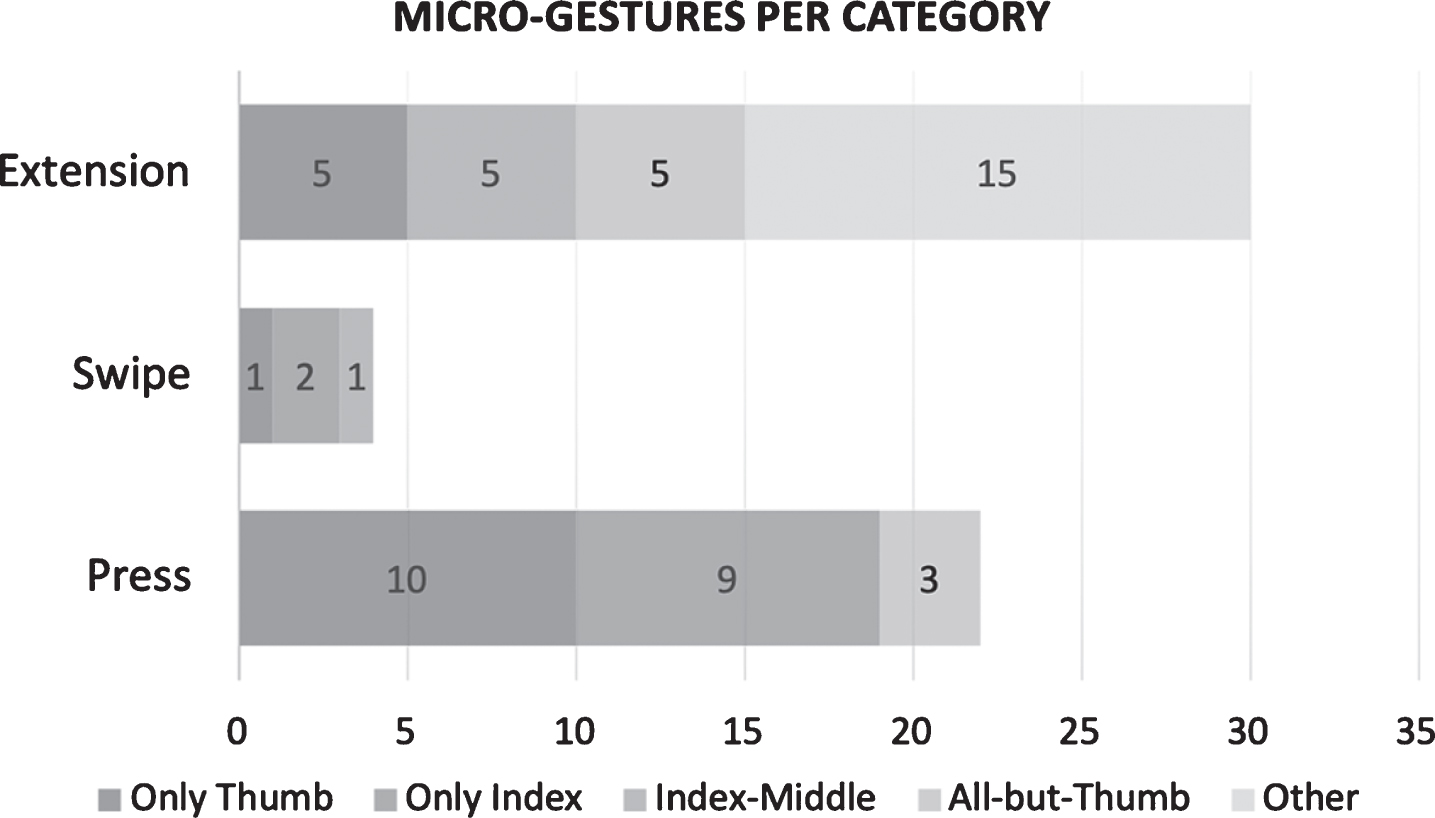
4.2Results with smart glove
In this study conducted with the GoGlove wearable device, five out of seven users chose to perform the gestures on hooks position and two in the tops position.
In the total of 56 elicited gestures, 34 were single tap and 22 double tap gestures (Fig. 10). The index finger gesture was chosen 29 out of 56 times as symbol, which constitutes the 53%. The ring gesture was the second preference with 11 preferences (19%). The middle finger was selected 10 times (18%). The phalange was the least favorite with 6 preferences (10%). It is interesting to notice as difference between the use of the middle and ring that the ring finger was preferred as single tap while this was indifferent for the middle ring.
Fig. 10
This graph depicts the distribution of the use of fingers with the smart glove, GoGlove [28], with reference to the kind of gesture: single tap or double tap. The index was the most used finger and the single tap the preferred movement.
![This graph depicts the distribution of the use of fingers with the smart glove, GoGlove [28], with reference to the kind of gesture: single tap or double tap. The index was the most used finger and the single tap the preferred movement.](https://ip.ios.semcs.net:443/media/wor/2020/66-4/wor-66-4-wor203238/wor-66-wor203238-g010.jpg)
Table 1 shows the results of this elicitation study for the attribution of the symbols (i.e., available gestures) to the presented referents (i.e., actions in the mobile application). For four referents there is a clear preference for the kind of gesture that is considered to be the most appropriate: single tap with the ring finger to open the camera and single tap with the index to take a picture. The single tap with the index was also chosen to swipe left and to zoom in. These results show the clear preference for the index and the ring fingers and for the single tap to interact with the application. These results will be further discussed in the next section.
Table 1
Rows represent the referents (actions in the app) and the columns represent the symbols (available gestures using the GoGlove wearable device). The maximum score for each symbol for a given referent is seven (the number of participants)
Single Tap Phalange | Single Tap Index | Single Tap Middle | Single Tap Ring | Double Tap Phalange | Double Tap Index | Double Tap Middle | Double Tap Ring | |
Swipe Left | 1 | 4 | 0 | 0 | 0 | 2 | 0 | 0 |
Swipe Right | 0 | 2 | 1 | 0 | 0 | 3 | 1 | 0 |
Open Camera | 1 | 0 | 1 | 5 | 0 | 0 | 0 | 0 |
Close Camera | 0 | 1 | 0 | 3 | 0 | 2 | 0 | 1 |
Switch Camera | 1 | 0 | 2 | 0 | 1 | 1 | 1 | 1 |
Take Picture | 1 | 5 | 0 | 0 | 0 | 0 | 0 | 1 |
Zoom In | 0 | 4 | 0 | 0 | 0 | 3 | 0 | 0 |
Zoom Out | 1 | 0 | 1 | 0 | 0 | 2 | 3 | 0 |
4.3Results with interactive armband
In this study conducted with the Myo armband, as with the GoGlove, five out of seven users performed the gestures on hooks position and two in the tops position.
Table 2 reports the results of this study and it shows that the vast majority chose to associate the wave left gesture to swipe left and the wave right gesture to swipe right. The majority also associated the fist gesture with taking a picture. It is interesting to note that wave left and wave right were also consistently used for the zoom in and zoom out actions. These results will be further discussed in the next section.
Table 2
Rows represent the referents (actions in the app) and the columns represent the symbols (available gestures using the Myo armband). The maximum score for each symbol for a given referent is seven (the number of participants)
Fist | Wave Left | Wave Right | Fingers Spread | Double Tap | |
Swipe Left | 0 | 5 | 2 | 0 | 0 |
Swipe Right | 0 | 2 | 5 | 0 | 0 |
Open Camera | 2 | 0 | 0 | 2 | 3 |
Close Camera | 2 | 1 | 1 | 2 | 1 |
Switch Camera | 1 | 2 | 0 | 3 | 1 |
Take Picture | 4 | 0 | 0 | 0 | 3 |
Zoom In | 1 | 3 | 1 | 1 | 1 |
Zoom Out | 1 | 1 | 3 | 2 | 0 |
5Discussion
During these studies, all cyclists were concerned about their own safety while performing their elicited gestures. For this reason, the choice of micro-gestures, intended as small movements performed while holding the handlebar to interact at a distance with connected bike computers while cycling, was unanimously approved. The participants reasoned aloud while showing the chosen gestures and they revealed that some micro-gestures were suggested just because they seemed more intuitive and convenient to perform. Therefore, the main criteria that guided the cyclists’ choices were based on the ergonomics of the movements to be performed while holding the handlebar and the cognitive effort required to remember the gesture. However, there are some patterns that emerged from the analysis of these results that can be used to propose a first set of guidelines for the design of interfaces based on micro-gestures performed on a drop-bar.
5.1Hand anatomy
A clear predominance of the index and thumb on the other three fingers can be observed. While the index and the thumb fingers were the most used, the movement of the ring and the little finger alone was defined by the cyclists as “uncomfortable”. Apparently, the number of fingers moved does not affect the difficulty of the gesture as long as the fingers involved are easy to move together. Indeed, two out of four groups of symbols implied the use of multiple fingers, i.e., index + middle and all-but-thumb (Fig. 8). Other combinations of finger extension can be difficult to perform, for instance, stretching the ring and middle fingers separately. Stretching the little or the index finger is much easier because of human hand’s anatomic architecture.
There is a set of constraints to consider when designing universal gestures. For example, as explained in [8], it is easy to tap the thumb on the middle and ring fingers while holding the handlebar, but depending on the bar diameter and the hand size, tapping the thumb with the index or the little finger can be difficult. This was observed also during the study with the GoGlove, which confirmed the tap of thumb + middle and thumb + ring fingers as easy to perform while holding a handlebar. However, the difference between the study reported by Wolf et al. in [8] about micro-gestures on the steering wheel and the one presented in this paper concerns the use of the index. The tap of thumb + index was the dominant gesture while using the GoGlove on the drop-bar and this is easily explained thanks to the bar dimension: the handlebar is usually smaller than a steering wheel rendering the aforementioned gesture convenient.
The results show clearly that the most popular micro-gestures were pressing the thumb and pressing the index (Fig. 9). The extension of thumb, index + middle and all-but-thumb follow in this order. At the end of the list, with a low number of preferences, there are the all-but-thumb press and the index swipe. For the use alone, the thumb plays a crucial role for micro-gestures according to these participants. The index is already well solicited during cycling because it is used for the brake and gear levers but nonetheless it was used in multiple elicited gestures. Therefore, these fingers should be the main parts of the hand for the design of these micro-gestures, followed by the combination of all-but-thumb and index + middle.
Another important contribution of this study consists in the creation of a taxonomy for the types of movement made with the different fingers (Fig. 9). Indeed, it was possible to identify three precise categories of elicited gestures: extension, press and swipe. These categories should be used as reference when designing micro-gestures performed on the drop-bar, prioritizing the press and extension movements.
5.2Handlebar shape
The design of micro-gestures must also consider the specific zones of the handlebar they are performed on. When cyclists were asked where they preferred to perform the free micro-gestures, all of them answered on the hoods, which is the stationary body of a brake lever: the part that attaches to the handlebars. This term is normally used when describing brake levers intended for use with drop-bars, where the hood is intended to act as an alternate hand rest. When asked why, all of them answered that this is area of the drop-bar where they can position their hands on while cycling and that allows accessing rapidly the bike commands, i.e., brake and gear levers. This consideration is directly linked to the cyclists’ concern about safety. Indeed, the expression of their needs for interacting with connected bike computers without taking their hands off the hoods is due to the awareness that having their hands in that position allows them to access brakes faster in case of accident. Using a set of gestures recognized by commercial devices such as the GoGlove and Myo while holding the handlebar is not ideal. The single tap and the double tap recognized by the GoGlove are both difficult to perform in the hoods position. Indeed, five out of seven cyclists preferred performing them on the hooks position and the remaining on the tops. This is easily explained by the fact that in those two zones, hooks and tops, the handlebar is more or less round shaped and tapping the fingertips while holding a bar is still a convenient movement to perform, which is not possible holding the hoods. The five gestures recognizable by default using the Myo armband presented similar problems of ergonomics on the hoods position. Indeed, also in this case five out of seven cyclists chose to perform these gestures on the hooks and the other two on the tops. As for the GoGlove, tapping the fingertips was a convenient gesture to perform while holding the tops or hooks; performing the fist gesture is also easy because it is squeezing the handlebar (it can be considered a motionless gesture since it consists of an isometric contraction); similarly, the wave left and wave right were performed as wrist extension and wrist flexion while holding the bar. However, all of these aforementioned gestures are convenient on tops and hooks but not on the hoods because of its shape. Only the finger extension could be performed on the hoods and, indeed, a similar gesture was suggested by the cyclists during the elicitation of free gestures: the all-but-thumb extension.
The peculiar shape of the drop-bar and especially of the hoods requires designing ad hoc gestures that can be performed while holding the handlebar in a way that can allow rapid access to the brake levers. Another fundamental factor determining the right design of these gestures is represented by the categories resulted from this study. It differs to design a gesture based on pression or swiping rather than based on the extension of fingers since the first two require touching a specific area of the handlebar and the latter needs free area of movement. Considering the gesture category and the part of the hand implied in the movement allows tracing some specific zones of interest on the drop-bar, which are the ones accessible on the hoods position and that should be the target of future systems for the recognition of gestures while cycling as it is depicted in Fig. 11. Recognizing pressure or swiping implies integrating touch-sensitive sensors on the handlebar surface. For the thumb and index fingers, this means on the side parts of the hoods; for the other fingers, this means sensorizing the hooks. Recognizing extension gestures would require other types of technology: it would be possible to embed video-based sensors on the handlebar, otherwise wearing electromyographic and inertial sensors is a valid alternative. Current shapes of drop-bars allow for performing the index-middle and all-but-thumb extension since the area around the hands on the hoods is completely clear and the hand posture is comfortable.
Fig. 11
This image depicts the suggested design for the interactive zones for an ergonomic interface based on micro-gestures on a drop-bar. It is possible to note that these zones are placed around the hoods, since this was the position that received the consensus from the participants and was indicated not only the most popular but also the safest because it allows to access the gear and brake levers rapidly.
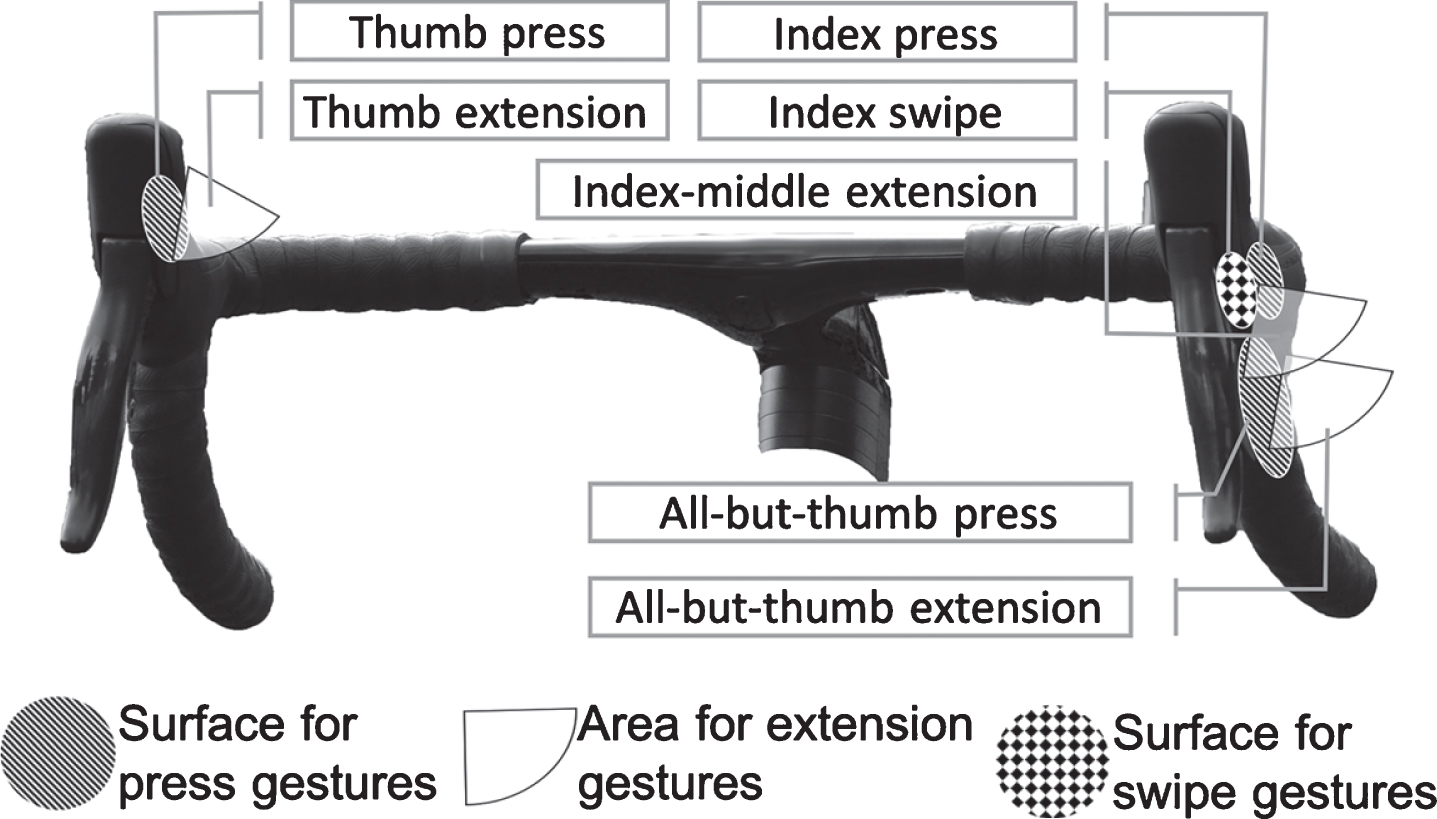
5.3Symmetry
The spatial reference is not only important for the ergonomics of the posture and of the movements but also on a cognitive level. For instance, during the free gesture elicitation-study, it was also possible to note that “symmetric” referents implied symmetric symbols, i.e., the paired gestures swipe left/right, zoom in/out and open/close camera resulted having almost identical (pairwise) results for the chosen fingers and gesture types. In order to elicit a gesture for the swipe left or a swipe right action, five out of seven users chose to use respectively the right and the left hand. Four users elicited the gestures only with the thumb finger, while the remaining one used the little finger. The four gestures that involved the movement of the thumb are divided equally in tap gesture and extension gesture. For the zoom in/out, three users elicited the same gestures that would do on a touchscreen and were found to be very similar to pinch-in and pinch-out gestures, except that they were performed freehand and, therefore, excluded from the analysis. Three out of seven participants used also symmetric gestures for starting and closing the camera function. This effect could be observed also in the study with the Myo armband, where participants chose to attribute wave left gesture to swipe left and the wave right gesture to swipe right, plus wave left and wave right were also suggested for the zoom in and zoom out actions. The results enforce the analysis based on the free gestures about the crucial role that symmetry can play for users in order to easily associate symbols to referents.
The considerations concerning the symmetric correspondence of symbols and referents should be applied also to the design of the drop-bar: the interactive zones of the handlebar should be symmetrical. Figure 11 depicts these zones only on one side for the sake of clarity, but they should be replicated on both left and right hoods, and the same should be done for the hooks.
5.4Study limitations
This study is the first work exploring the design of a tangible gestural interface integrated in a road bike. As such, it presents some limitations: the sample size of participants is limited to seven, which is insufficient for a statistical significance of this study. Moreover, all of the athletes who took part in this study were working in the same company, which can introduce a cultural bias. All the gesture elicitation sessions were conducted in a laboratory setting using a real road bike but as a stationary bicycle: the next study will be designed to test these gestural interfaces while actually cycling on the road. Finally, the shape of the handlebar can vary greatly and, for this reason, the results of this study can be applied only to a drop-bar with a classic shape (see Fig. 3), which can differ from some of the most recent models. Moreover, this study focused on the hoods position, therefore the final design recommendations are limited to this posture.
6Conclusion
Technological innovation in bike design is evolving fast, both for urban use [29] and sports [3]. The latter, in particular, requires continuous improvement to constantly push the limits of performance. In this context, connected bike computers are gaining importance since they can provide the athletes with instant feedback, which allows improving performance. However, these devices are cumbersome to use while cycling since they require the user to take her/his hands off the handlebar to press their buttons or tap on their touchscreen. Gestures that can be performed while grasping the handlebar performing small movements (called micro-gestures) and that can control these devices at a distance are a possible solution to this problem allowing for enhanced performance and safety. The current scientific literature falls short on studies focused on the design of gestural interfaces for drop-bars and this research aims at providing a first set of principles to guide the design of such interfaces. It is important to start with some fundamental guidelines concerning the hand anatomy: the thumb and the index were the preferred fingers for the elicited micro-gestures. It is possible also to design other gestures involving the use of all-but-thumb and index + middle movements. It is important to consider the anatomic constraints for some movements and other possible issues depending on the hand size compared to the drop-bar size. For the design of the finger movement, this elicitation study provided also a taxonomy based on three categories: press, extension and swipe. These movements are all well suited to be performed on the hoods position, which was unanimously elected the standard posture. The preferred combination of the aforementioned fingers and movement categories are namely: thumb press, index press, thumb extension, index + middle extension, all-but-thumb extension, all-but-thumb press, and index swipe.
The design of the handlebar is crucial for the ergonomic use of bikes [30]. Therefore, further guidelines focused on the design of the drop-bar are needed. In this paper, a number of preliminary guidelines are reported in order to show where the interactive zones should be designed; more specifically, they should be around the hoods and on a part of the hooks (see Fig. 11), and they should be symmetric between left and right.
Finally, the design of the gestures should be symmetric in order to assign symmetric symbols to symmetric referents.
Conflict of interest
None to report.
References
[1] | Haake SJ . The impact of technology on sporting performance in Olympic sports. Journal of Sports Sciences. (2009) ;27: (13):1421–31. |
[2] | El Helou N , Berthelot G , Thibault V , Tafflet M , Nassif H , Campion F , Hermine O , Toussaint JF . Tour de France, Giro, Vuelta, and classic European races show a unique progression of road cycling speed in the last 20 years. Journal of Sports Sciences. (2010) ;28: (7):789–96. |
[3] | Broker J , Crawley J . Advanced sport technologies: Enhancing Olympic performance. In: ISBS-Conference Proceedings Archive 2001 (Vol. 1, No. 1). |
[4] | Wilson BD . Development in video technology for coaching. Sports Technology. (2008) ;1: (1):34–40. |
[5] | Gharghan SK , Nordin R , Ismail M . A survey on energy efficient wireless sensor networks for bicycle performance monitoring application. Journal of Sensors. 2014. |
[6] | Le A , Jaitner T , Litz L . Sensor-based training optimization of a cyclist group. In 7th International Conference on Hybrid Intelligent Systems (HIS 2007) 2007; pp. 265-270. IEEE. |
[7] | Angelini L , Baumgartner J , Carrino F , Carrino S , Caon M , Khaled OA , Sauer J , Lalanne D , Mugellini E , Sonderegger A . A comparison of three interaction modalities in the car: gestures, voice and touch. In Actes de la 28ième conférence francophone sur l’Interaction Homme-Machine. 2016; pp. 188-196 ACM. |
[8] | Wolf K . Microgestures—enabling gesture input with busy hands. In Peripheral Interaction 2016; (pp. 95-116). Springer, Cham. |
[9] | Marshall J , Tennent P . Mobile interaction does not exist. In CHI’13 Extended Abstracts on Human Factors in Computing Systems 2013; (pp. 2069-2078). ACM. |
[10] | de Waard D , Westerhuis F , Lewis-Evans B . More screen operation than calling: the results of observing cyclists’ behaviour while using mobile phones. Accident Analysis & Prevention. (2015) ;76: :42–8. |
[11] | Marshall J , Dancu A , Mueller FF . Interaction in motion: designing truly mobile interaction. In: Proceedings of the 2016 ACM Conference on Designing Interactive Systems. 2016; (pp. 215-228). ACM. |
[12] | Rowland D , Flintham M , Oppermann L , Marshall J , Chamberlain A , Koleva B , Benford S , Perez C . Ubikequitous computing: designing interactive experiences for cyclists. In: Proceedings of the 11th International Conference on Human-Computer Interaction with Mobile Devices and Services. 2009; (p. 21). ACM. |
[13] | Dancu A , Vechev V , Ünlüer AA , Nilson S , Nygren O , Eliasson S , Barjonet JE , Marshall J , Fjeld M . Gesture bike: examining projection surfaces and turn signal systems for urban cycling. In: Proceedings of the 2015 international conference on interactive tabletops & surfaces. 2015; (pp. 151-159). ACM. |
[14] | Kräuter N , Lösing S , Bauer G , Schwering L , Seuter M . Supporting safety in cycling groups using LED-augmented gestures. In: Proceedings of the 2016 ACM international joint conference on pervasive and ubiquitous computing: adjunct. 2016; (pp. 889-892). ACM. |
[15] | Sanders EB , Stappers PJ . Co-creation and the new landscapes of design. Co-design. (2008) ;4: (1):5–18. |
[16] | Wobbrock JO , Morris MR , Wilson AD . User-defined gestures for surface computing. In: Proceedings of the SIGCHI Conference on Human Factors in Computing Systems. 2009; (pp. 1083-1092). ACM. |
[17] | Ruiz J , Li Y , Lank E . User-defined motion gestures for mobile interaction. In: Proceedings of the SIGCHI Conference on Human Factors in Computing Systems. 2011; (pp. 197-206). ACM. |
[18] | Angelini L , Carrino F , Carrino S , Caon M , Khaled OA , Baumgartner J , Sonderegger A , Lalanne D , Mugellini E . Gesturing on the Steering Wheel: a User-elicited taxonomy. In: Proceedings of the 6th international conference on automotive user interfaces and interactive vehicular applications. 2014; (pp. 1-8). ACM. |
[19] | Hochleitner W , Sellitsch D , Rammer D , Aschauer A , Mattheiss E , Regal G , Tscheligi M . No need to stop: exploring smartphone interaction paradigms while cycling. In: Proceedings of the 16th international conference on mobile and ubiquitous multimedia. 2017; (pp. 177-187). ACM. |
[20] | Tan Y , Yoon SH , Ramani K . BikeGesture: user elicitation and performance of micro hand gesture as input for cycling. In: Proceedings of the 2017 CHI Conference Extended Abstracts on Human Factors in Computing Systems. (2017) ; (pp. 2147–2154). ACM. |
[21] | Lukes RA , Chin SB , Haake SJ . The understanding and development of cycling aerodynamics. Sports Engineering. (2005) ;8: (2):59–74. |
[22] | Slane J , Timmerman M , Ploeg HL , Thelen DG . The influence of glove and hand position on pressure over the ulnar nerve during cycling. Clinical Biomechanics. (2011) ;26: (6):642–8. |
[23] | Silberman MR , Webner D , Collina S , Shiple BJ . Road bicycle fit. Clinical Journal of Sport Medicine. (2005) ;15: (4):271–6. |
[24] | Morris MR , Danielescu A , Drucker S , Fisher D , Lee B , Wobbrock JO . Reducing legacy bias in gesture elicitation studies. Interactions. (2014) ;21: (3):40–45. |
[25] | GoGlove | The Ultimate Activity Accessory [Internet]. Goglove.io. 2019 [cited 1 March 2019]. Available from: http://goglove.io/ |
[26] | Rawat S , Vats S , Kumar P . Evaluating and exploring the MYO ARMBAND. In 2016 International Conference System Modeling & Advancement in Research Trends (SMART) (2016) ; (pp. 115–120). IEEE. |
[27] | Angelini L , Caon M , Carrino F , Carrino S , Lalanne D , Khaled OA , Mugellini E . Wheelsense: Enabling tangible gestures on the steering wheel for in-car natural interaction. In International Conference on Human-Computer Interaction. (2013) ; (pp. 531–540). Springer, Berlin, Heidelberg. |
[28] | Caon M , Süsse R , Grelier B , Khaled OA , Mugellini E . Gesturing on the Handlebar: A User-Elicitation Study for On-Bike Gestural Interaction. In: Congress of the International Ergonomics Association. 2018; (pp. 429-439). Springer, Cham. |
[29] | Tosi F , Belli A , Rinaldi A , Tucci G . The Intermodal Bike: multi-modal integration of cycling mobility through product and process innovations in bicycle design. Work. (2012) ;41: (Supplement 1):1501–6. |
[30] | Chen YL , He KC . Changes in human cervical and lumbar spine curves while bicycling with different handlebar heights. Work. (2012) ;41: (Supplement 1):5826–7. |