The efficacy of low molecular weight heparin is reduced in COVID-19
Abstract
BACKGROUND:
A significant degree of mortality and morbidity in COVID-19 is through thromboembolic complications, only partially mitigated by anticoagulant therapy. Reliable markers of infection severity are not fully established.
OBJECTIVES:
This study investigated whether visco-elastic biomarkers predict disease severity on presentation to the Emergency Department (ED) and how they measure response to anticoagulation
METHODS:
Patients testing positive for COVID-19 at a large University Teaching Hospital ED were recruited at presentation. Multiple blood samples were taken throughout hospital admission to monitor disease progression with end outcome recorded. Visco-elastic markers, fractal dimension (df) and Time to Gel Point (TGP) which measure the properties of the incipient clot were compared in patients with and without anticoagulation by Low Molecular Weight Heparin (LMWH).
RESULTS:
TGP and df did not predict severity of infection with COVID-19. Although LMWH prolonged TGP, there was no change in df indicating LMWH did not change clot microstructure.
CONCLUSIONS:
Therapeutic efficacy of LMWH appears blunted in COVID-19 infection. This may be due to the inflammatory state creating a resistance to LMWH activity, which may explain why LMWH appears less effective in COVID-19 compared to other disease states. COVID-19 was not predicted by visco-elastic testing at the time of ED presentation.
1Introduction
COVID-19 infection is frequently complicated by thromboembolic events. Beyond the acute lung inflammation and hypoxia, these events appear to be a major contributor to mortality and morbidity. There are a range of pathological processes implicated in this, Pulmonary Emboli (PE), and coronary thrombi have been observed without any evidence of preceding Deep Venous Thrombosis (DVT) suggesting de novo intravascular clot formation. Post-mortem findings in COVID-19 involve a wide range of thromboembolic complications including myocardial infarction (MI), PE and end organ microthrombi leading to multi-organ failure [1–3]. Techniques such as Thromboelastography (TEG) and Rotational Thromboelastometry (ROTEM) have been used to describe COVID-19 associated coagulopathy, and while a growing body of evidence suggests microthrombotic complications are caused as a result of fibrinolytic shutdown and an inability to break down clot mass [4–6], a range of other coagulopathic patterns were reported across studies [7, 8]. This heterogeneity in data indicates alternative measures of coagulation are necessary to fully examine the pathological effects of COVID-19.
Low Molecular Weight Heparin (LMWH) is routinely used as prophylactic treatment against Venous Thromboembolism (VTE), but in COVID-19 appears to be insufficient in preventing VTE [9]. Previous studies have demonstrated a significantly higher dosing of anticoagulant is required to prevent VTE in COVID-19 patients with a correspondingly higher target Anti-Xa concentration [10, 11] and while this failure to achieve target Anti-Xa levels is more prevalent in patients on Intensive Care Units (ICUs), than on general medical wards, there remains a considerable cohort on general wards also under-anticoagulated as assessed by Anti-Xa levels [12, 13]. The certainty of these findings and the strength of recommendations possible from them has been limited as to date the majority of studies have either been small scale or non-peer reviewed articles. As we move further away from the earliest phases of the pandemic, there is a need to strengthen the evidence base with larger studies. Additionally, the majority of these studies tend to focus on a comparison of anticoagulant dose vs target Anti-Xa levels. Consequently, there is relatively little data investigating how established markers of coagulation respond to anticoagulant therapy [9].
A range of techniques have been trialled to assess the severity of COVID-19 at early stages of the disease, and while they have each provided some utility, none have provided sufficient sensitivity and specificity to stand alone as a severity prognosticator. National Early Warning Score 2 (NEWS2) is a score generated from basic physiological measurements and was updated from an earlier National Early Warning Score (NEWS). Prior to the COVID-19 pandemic, NEWS was shown to be predictive of death, unanticipated ICU admission or cardiac arrest for patients already in hospital [14] and is also validated for use in the Emergency Department (ED) at the time of first presentation [15]. When specifically evaluated for its utility in COVID-19 patients, NEWS2 had good predictive value for assessing death or ICU admission within 24 hours of vital sign measurement without the need for additional covariates, or adjusting the weighting of parameters from the original scoring [16]. In much smaller scale studies designed to evaluate use of NEWS2 at the time of arrival to the ED for COVID-19 patients, NEWS2 on admission was closely associated with severe disease, defined as death whilst an inpatient, or admission to ICU at any stage of hospital stay [17]. While NEWS2 therefore appears to be a useful tool for the ED clinician in predicting risk of severe disease due to COVID-19, by definition it requires physiological deterioration in order to predict severity. Therefore a tool that is sensitive prior to deterioration is desirable.
Extensive work has gone into exploring the relationship between viral load and disease severity. While it appears that there is a link between older age and viral load, there has been no established relationship between severity and viral load [18]. Radiological techniques such as plain film or CT have also been assessed, and while there are a number of features which may indicate severe disease, imaging may also be unremarkable in early stages [19].
A range of laboratory markers have also been assessed, and an extensive list of markers have been associated with severity of disease, this includes D-dimer, white blood cell counts, CRP, renal function, liver function and cardiac markers [20, 21]. However, as these are markers, they are not direct targets for therapy and therefore there remains a need to establish modifiable testing parameters through which a goal directed therapy can be pursued. Other coagulation parameters might provide a direct target for therapy. As dysregulation of the coagulation system drives thrombogenicity, modifying these parameters might directly reduce the thrombogenic effect.
The aims of this study were therefore twofold, to evaluate whether visco-elastic measurements of clot quality and microstructure can predict severity of disease due to COVID-19 in the early stages of infection, and to describe changes to the therapeutic effect of heparin in COVID-19 infection.
2Methodology
120 patients presenting to a single University Hospital ED with respiratory or febrile symptoms suspicious for COVID-19 infection were recruited, and tested for COVID-19 via PCR virology. Patients were excluded if they were on anticoagulant medication including warfarin and direct oral anticoagulants (DOACs) such as apixaban or rivaroxaban, or if they declined to participate. This study occurred before the roll out of COVID-19 vaccinations in the UK, and as such all patients were unvaccinated.
On arrival patients had COVID-19 PCR virology performed, once a PCR result was reported for a patient, further investigations were only performed if the result was positive for COVID-19. 96 patients tested positive for COVID-19, 1 patient was later found to have been on warfarin and was retrospectively removed from analysis.
On recruitment to the trial, patients had routine haematological and biochemical blood samples drawn. Alongside these, ROTEM and Rheometry were performed by the investigating team. Patients testing positive for COVID-19 on PCR virology and requiring admission received LMWH as per standard treatment protocols at the time. The time this was administered was recorded and patients stratified into those who had blood sampling before receiving LMWH and those who had already been given LMWH when recruited to the study. Clinical pathways also included corticosteroid therapy for any patient with a supplemental oxygen requirement, the standard therapy was dexamethasone orally or intra-venously unless the responsible clinician deemed another corticosteroid more appropriate. Patients were followed up through their admission with their length of stay and outcome recorded. The investigating team had no influence on the clinical decision making for patients in the study. Patients were retrospectively grouped based on their outcome. Those who died or required an ICU admission were classed as Critical, those who required a hospital stay of 5 days or more classed as Severe, and those discharged before 5 days were classed as Mild-Moderate.
Repeat blood sampling was performed at 24 hours, 3-5 days and 7 days if the patient remained in hospital at this time. Discharge decisions were made by the clinicians responsible for the patient with no input from the investigating team. Beyond 7 days no further blood samples were collected for the study even if the patient remained in hospital.
Blood samples were taken from the antecubital vein where possible using a 21 G butterfly needle. Two 2.7 mL samples were immediately transferred into PET 0.109M 3.2% citrated vacutainers (Becton Dickinson, Plymouth, UK Ref: 363095). Routine coagulation studies were performed on the first citrated vacutainer including PT, APTT, Clauss fibrinogen and were measured using a Sysmex CA1500 analyser within two hours of collection. D-dimer analysis was carried out using Latex immunoturbidimetric assay (Instrumentation Laboratory, Warrington, UK). 4 ml was collected into a plastic dipotassium EDTA vacuette (Becton Dickinson, Plymouth, UK Ref 367839) for FBC analysis using a Sysmex XE 2100 (Sysmex UK, Milton Keynes, UK). 5 mL was collected into a SST II Advance (Becton Dickinson, Plymouth, UK Ref 367954), testing of U&Es, LFTs, Bone Profile and CRP were performed on a Roche-Cobas 8000 Modular Analyser (Roche Diagnostics Ltd, UK).
The second citrated sample was used for Rotational Thromboelastometry, performed using a ROTEM Delta Whole Blood Haemostasis System (TEM Innovations GmbH, 2011) with testing carried out according to manufacturer recommendations and analysed on ROTEM Delta Software v1.6.3. The markers selected for analysis were Extem and Intem Clotting Time (CT), Clot Formation Time (CFT), Maximum Clot Firmness (MCF), Alpha Angle (Alpha) and Maximum Lysis (ML). This was designed to evaluate clot formation kinetics, mechanical structure and clot breakdown, mirroring the values selected by other studies. This data has been more fully reported in a separate article [4].
A 6.6 mL aliquot of whole blood was immediately loaded into a TA instruments AR-G2 controlled-stress rheometer (TA Instruments, New Castle, DE, USA) at 37°C (±0.1°C) for visco-elastic testing. This technique has been fully described previously in the literature, has been validated as a reliable technique for describing the visco-elastic properties of blood and acts as a compound marker of clot initiation, the templating effect and mechanical properties of the mature clot [22–24]. Briefly, visco-elastic measurement is performed using small amplitude oscillatory shear measurements at varying frequencies. The gel point is defined as the transition point from visco-elastic liquid to visco-elastic solid and is indicative of formation of the incipient clot. Time to Gel Point (TGP) measures the time taken to reach this transition. A mathematical relationship exists between the visco-elastic properties at the gel point and a structural parameter, the Fractal Dimension (df) which quantitatively describes the framework of the incipient clot –a higher value represents a denser and more branched fibrin network, whereas a lower df represents a looser and more open fibrin network. The incipient clot mass scales as a power-law relationship with df as illustrated in Fig. 1 [22]. Herein the incipient clot mass is normalised to a value of 1 for df = 1.707. Crucially df has been shown to be sensitive to the effects of in-vitro administration of heparin to whole blood in healthy volunteers, with increased levels of heparin, as measured by anti-Factor Xa, leading to lower values of df representative of incipient clots with significantly less mass [22].
Fig. 1
A graph displaying the relationship between df and the Normalised Mass, where a Normalised Mass of 1.00 is set at the mean df for the study cohort (1.707).
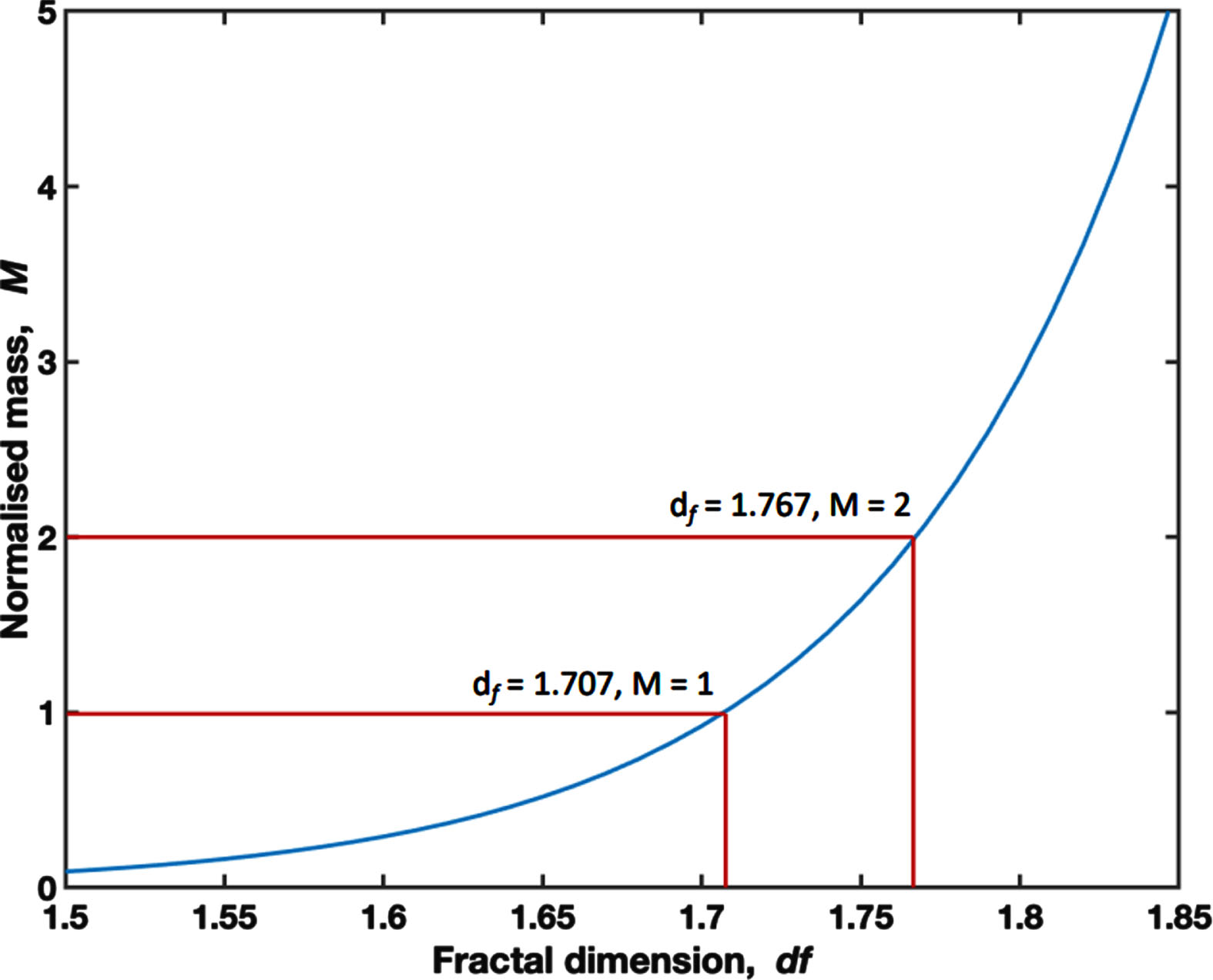
Statistical analysis was performed on IBM SPSS Statistics v28.0.0.0(190), Shapiro-Wilk testing was performed to assess for normality, and t-tests or ANOVA were used to assess for differences in groups when appropriate. These data are presented as a mean±standard deviation. Where non-parametric testing was appropriate, data is presented as a median±interquartile range and Mann-Witney or Kruskal-Wallis testing performed in place of a t-test or ANOVA respectively. Statistical significance throughout the paper is defined as p < 0.05. In graphs *, ** and *** signify p < 0.05, p≤0.01 and p≤0.001 respectively. Where comparisons between 3 groups indicate 1 group is significantly different to both other groups, but those two groups do not have a significant difference, the significant differences are denoted by a and b next to the values which differ with the p value indicated below.
Graphs and tables were produced in GraphPad Prism 9 v9.3.1(471).
Ethical approval was given by the South West Wales Research Ethics Committee (Wales REC 6), IRAS 216266, REC reference 17/WA/0123 [25].
3Results
Table 1a shows descriptive characteristics for each group. Age was significantly different in the mild-moderate group compared with both other groups, and is a well-known risk factor for disease severity. There was no significant difference in the age of the severe and critical groups. The relatively low proportion of patients with a previous CVA or PE is likely reflective of the fact that taking anticoagulant medication was an exclusion criteria for the study. Table 1b details the results of the first blood samples taken from each patient. The only significant difference in routine blood testing was CRP, which was significant different in the mild-moderate group compared to both other groups (p = 0.009 vs severe, p = 0.002 vs critical), the severe and critical groups did not have a statistically significant difference. Previously published data from this study cohort demonstrated the critical outcome group had a significantly reduced capacity for fibrinolysis compared to the other outcome groups [4], whilst other ROTEM parameters were not different across the outcome groups. Comparisons across the sampling time points indicated ROTEM parameters did not change throughout hospital admission in any outcome group.
Table 1a
Patient cohort characteristics for each group
Mild-Moderate | Severe | Critical | |
n | 29 | 46 | 20 |
Age (yrs) | 50.0±14.6ab | 64.3±12.5a | 69.2±13.6b |
Male % | 38 | 39 | 55 |
Diabetes % | 17 | 22 | 25 |
COPD % | 3 | 4 | 30 |
Hypertension % | 14 | 30 | 50 |
IHD / Angina % | 7 | 4 | 20 |
Heart failure % | 3 | 0 | 0 |
Hypercholesterolaemia | 7 | 7 | 5 |
CVA % | 0 | 2 | 5 |
PE / DVT % | 3 | 2 | 5 |
Cancer % | 7 | 11 | 5 |
Received LMWH before recruitment % | 31 | 39 | 30 |
a and b, p < 0.001.
Table 1b
Standard blood testing results taken on arrival to the ED
Mild-Moderate | Severe | Critical | |
Full Blood Count | |||
Haemoglobin (g/dL) | 139±16 | 134±18 | 137±18 |
Platelets (x109/L) | 238±74 | 282±97 | 228±80 |
White Cell Count | 6.7 (5.15 - 8.55) | 7.25 (5.325 - 9.850) | 7.9 (6.525 - 11.075) |
Neutrophils | 4.9 (3.55 - 7.05) | 6.15 (4.15 - 8.65) | 6.4 (4.85 - 10.4) |
Coagulation Screen | |||
PT (s) | 10.6 (10.2 - 11.15) | 10.6 (10.4 - 11.0) | 10.9 (10.225 - 11.2) |
APTT (s) | 23.8 (21.7 - 25.45) | 24.6 (20.35 - 25.6) | 24.05 (22.3 - 26.9) |
Fibrinogen (g/L) | 5.3 (4.1 - 6.15) | 5.65 (4.8 - 6.625) | 5.9 (4.025 - 6.35) |
D-dimer (μg/L) | 731 (544 - 1456.5) | 1149 (597.5 - 2182.75) | 981.5 (632 - 1520.75) |
Anti-Xa (units/mL) | 0.03 (0.01 - 0.0775) | 0.04 (0.01 - 0.275) | 0.04 (0.01 - 0.1675) |
Inflammatory Markers | |||
CRP (mg/L) | 44 (5 –101)ab | 88 (38.25 –229)a | 149.5 (63.5 - 200.25)b |
a and b, p < 0.01.
Figure 2 compares the df and TGP values on arrival between the groups. There were no differences in either df or TGP between the difference outcome groups.
Fig. 2
Bar chart of df and box plot of TGP based on outcome severity. No significant differences were detected.
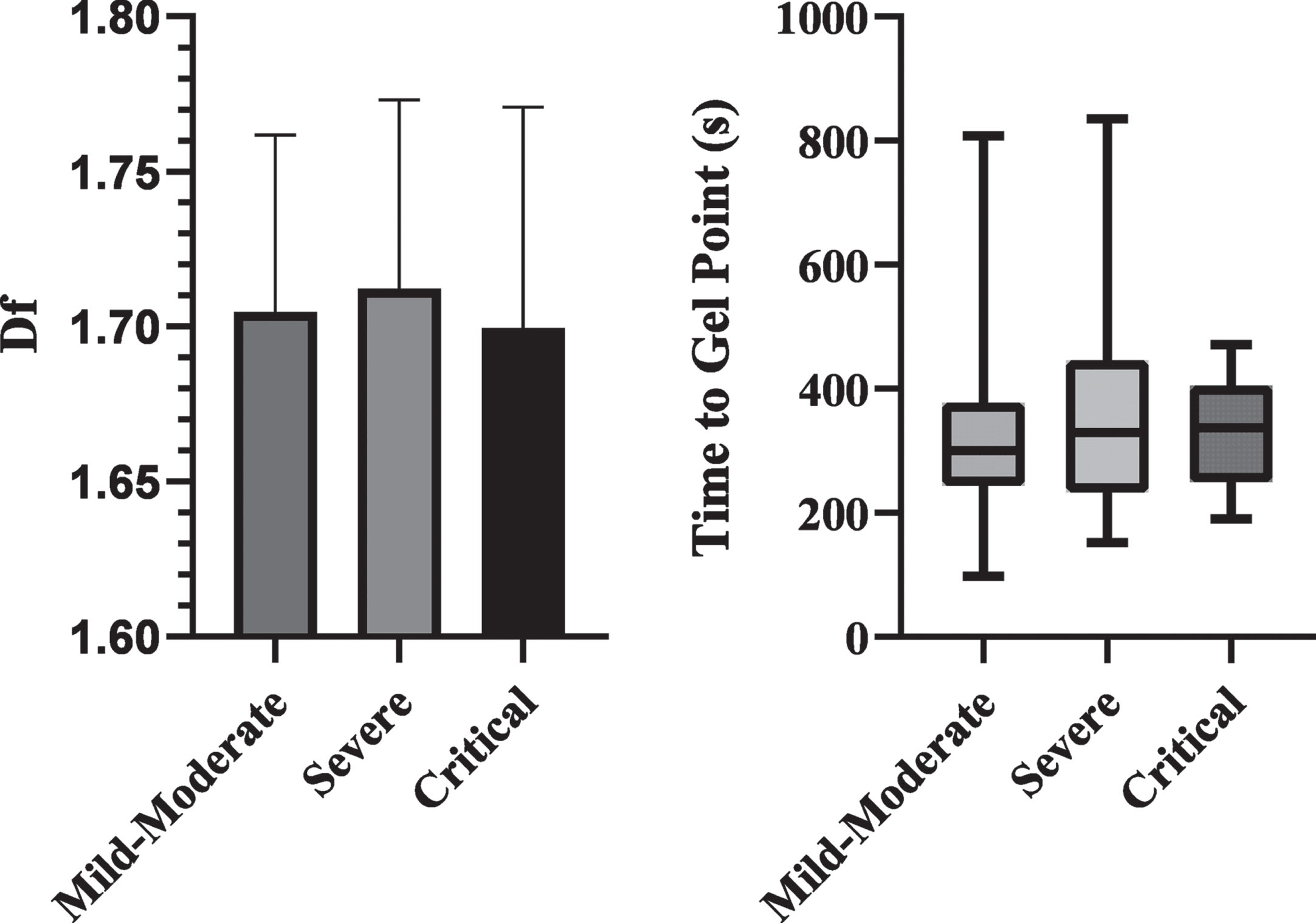
The patients who had received LMWH before blood sampling were compared to those who had been given no anti-coagulation before blood sampling and is shown in Fig. 3. In the LMWH group, mean time between administration of LMWH and blood sampling was 8.7 hours. Anti-Xa levels were significantly different between the groups (0.299 vs 0.031, p < 0.001). Figure 3 shows the df and TGP between these groups. There was no significant difference in the df between these two groups, but TGP was significantly prolonged in those who had received LMWH, 409 s vs 301 s (p < 0.001). Full details are shown in Table 2.
Fig. 3
Bar chart of df and box plot of TGP comparing Covid positive patients who had received LMWH before blood sampling against those with no anticoagulation. df was not significant. TGP was significantly prolonged in the LMWH group (p<0.001).
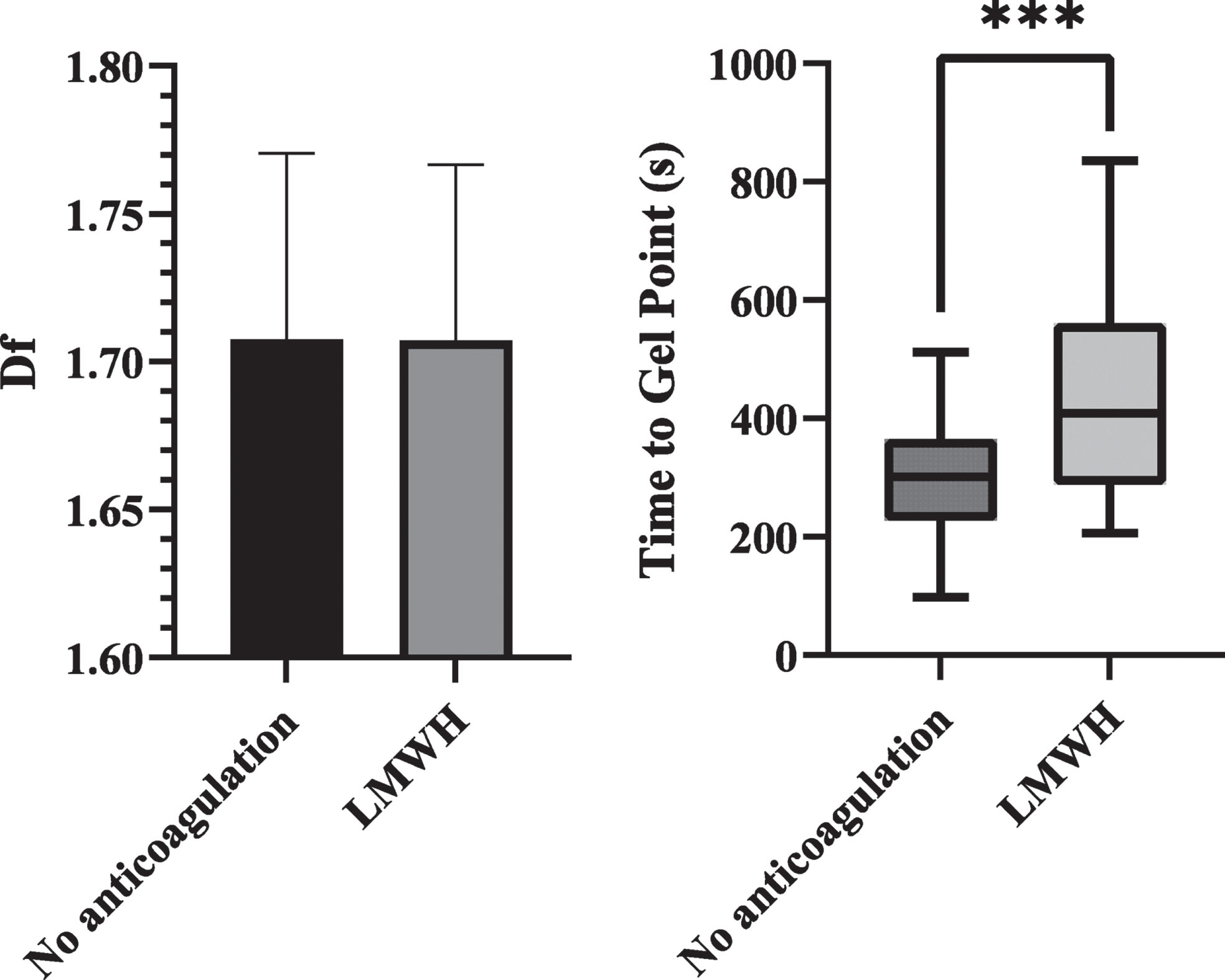
Table 2
Comparisons of df and TGP in patients who had received LMWH before blood sampling vs patients who had received no anticoagulation at the time of sampling
No Anticoagulation | LMWH | |
n | 59 | 32 |
df | 1.71±0.06 | 1.70±0.06 |
TGP (s) | 301 (227 - 365)*** | 409 (288 - 561)*** |
Anti-Xa (units/mL) | 0.031 (0.010 - 0.060)*** | 0.299 (0.130 - 0.578)*** |
Anti-Xa levels were compared to TGP, df and Normalised Mass. These values include sampling at all time points within the study. Once patients were commenced on LMWH it was continued throughout the study with no doses missed. These results are shown in Fig. 4. A positive relationship was observed between TGP and Anti-Xa (R2 =0.020, P = 0.03), whilst no relationship was seen between Anti-Xa and either df or Normalised Mass.
Fig. 4
Scatterplots of df, TGP and Normalised Mass against Anti-Xa levels. There was a very weak relationship between TGP and Anti-Xa, but no significant correlation between Anti-Xa and df and TGP.
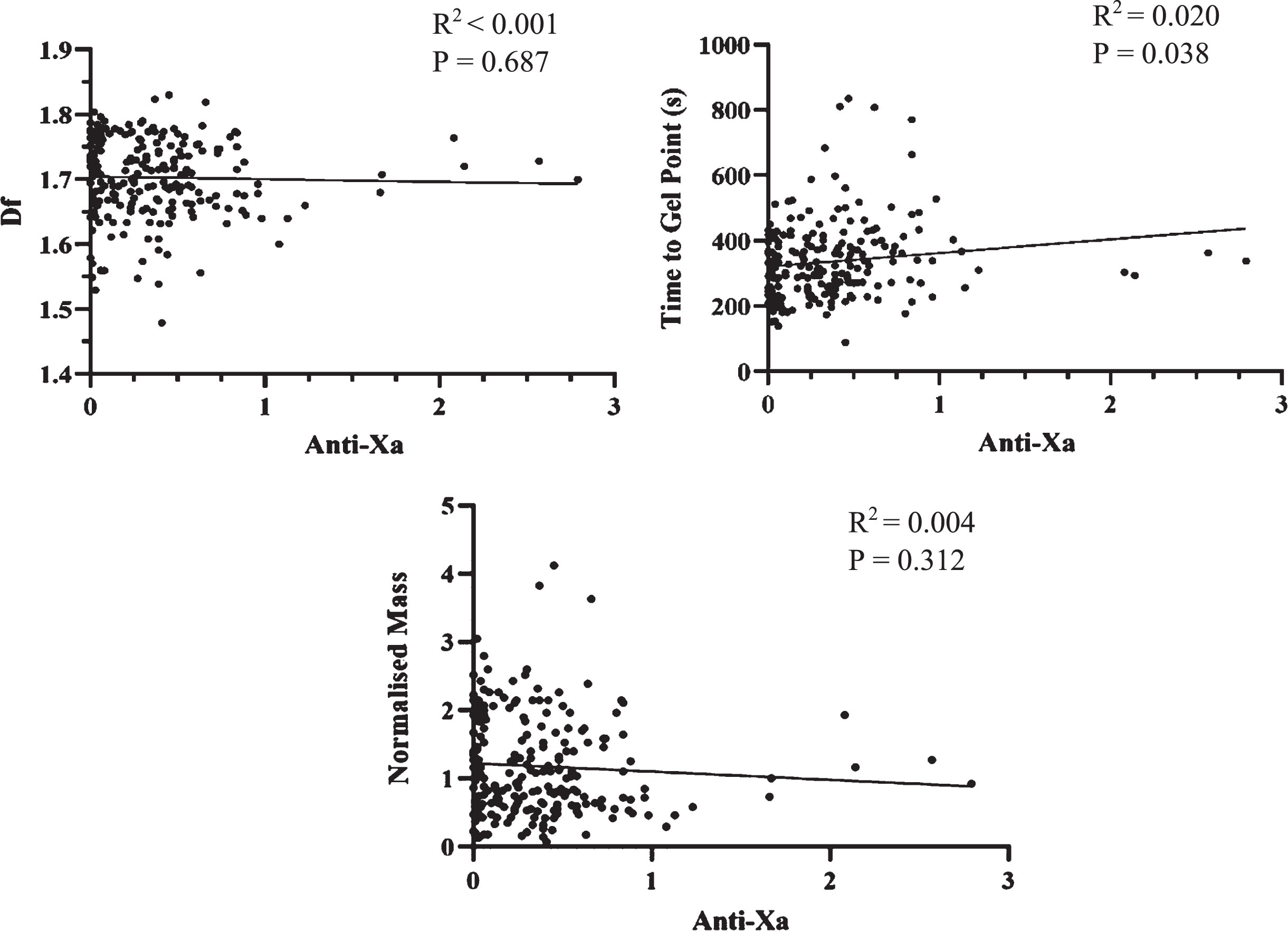
Normalised Mass was compared against ROTEM and this data is seen in Fig. 5. In the context of clot lysis, it is appropriate to compare ROTEM findings to Normalised Mass in addition to df. There was a significant negative correlation between Normalised Mass and ROTEM clot lysis markers. As a greater Normalised Mass is indicative of a denser clot, this indicates rate of clot lysis is related to clot density.
Fig. 5
Scatterplots of Normalised Mass against Extem and Intem Maximum Lysis (%). A statistically significant negative correlation was seen in both cases (p<0.05).
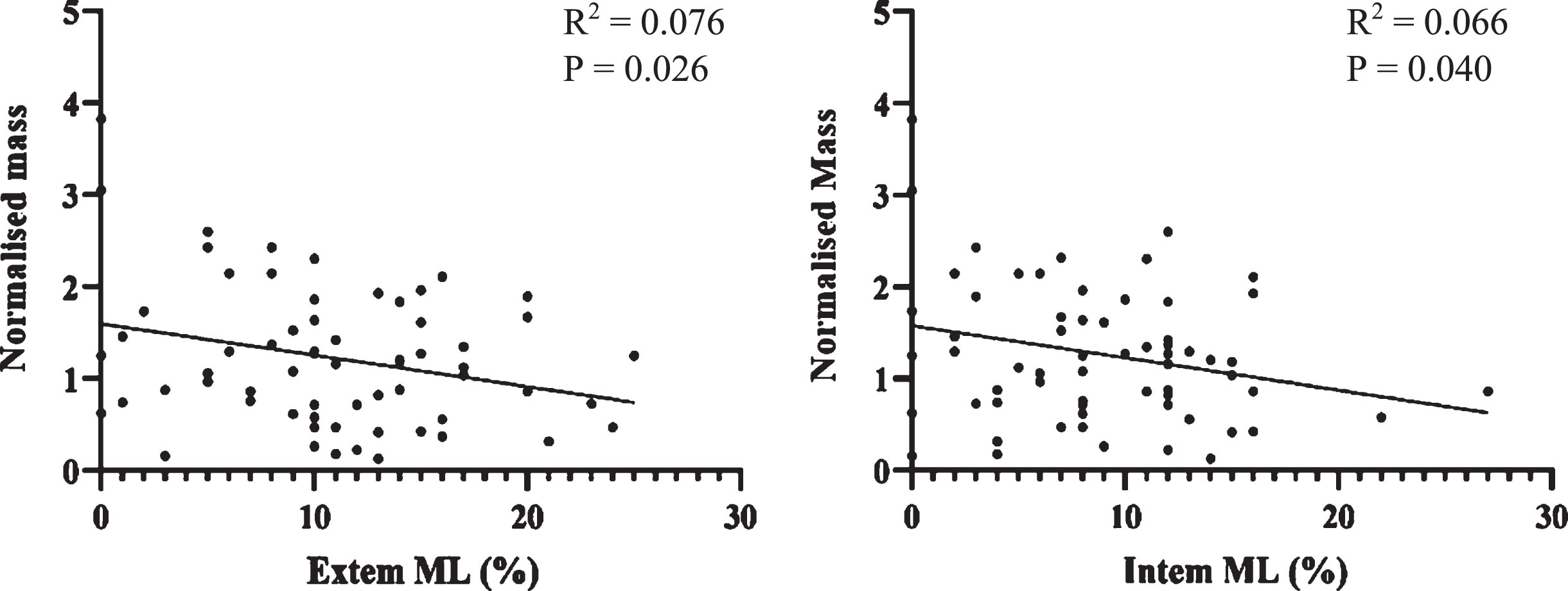
As previously published, Table 3 describes the results of CTPAs performed on patients in the study, and their detection rates for PE [4]. These CTPAs were performed at the discretion of the clinical team caring for the patient independently of the research team. This data was not a primary outcome of the study, however is included for completeness and is broadly comparable to previous systematic reviews on similar patient cohorts [26].
Table 3
A table showing the percentage of patients in each severity group who had a CTPA during admission, and the percentage which were positive
Mild-moderate | Severe | Critical | |
CTPAs performed | 34% | 30% | 40% |
Positive rate | 20% | 21% | 25% |
4Discussion
In this study, there has been no evidence that assessment of clot microstructure, quality and strength in using novel dynamic visco-elastic markers such as df at the time of presentation predicts the severity of the subsequent disease progression in COVID-19. This is further evidence that despite a significant burden of thromboembolic disease in COVID-19, it is atypical in how this disease is reflected by coagulation assays. In sepsis, it appears the initial trend in visco-elastic testing is towards a hypercoagulable state with dense clots and shortened clot formation times, but that with deterioration to septic shock, there is a reversal of this trend to weak clots generated by a very loose fibrin network [27]. However, in COVID-19 there appears to be no differences in clot microstructure as measured by df, even in severe disease with significant thromboembolic complications. Mortality and morbidity in COVID-19 do not appear to follow the standard model of mortality in septic shock due to bacterial pathogens, and as such it may not be appropriate to mirror strategies for treating sepsis in the management of severe COVID-19.
Fractal analysis describes microstructure of the incipient clot at the gel point where the clot transitions from fluid like behaviour to solid like behaviour. The microstructure at this stage goes on to establish the template for the mature clot which follows. The negative correlation of Normalised Mass with maximum clot lysis on ROTEM (Fig. 5) is of significance because it reinforces this link between the incipient clot and its mechanical and elastic properties once formed as a mature clot.
However, there is ample evidence to suggest that in COVID-19 there is a reduced efficacy of LMWH in these patients. This is counter to previous findings in healthy volunteers that in vitro heparin alters microstructure [20]. These studies also demonstrated a consistent relationship between Anti-Xa and both df and TGP, this relationship does not appear to be maintained in COVID-19 infection. While a relationship between Anti-Xa and TGP is maintained, there is no relationship between Anti-Xa and df. Although the previous study administered heparin in vitro, whilst this study measured df following the in vivo administration of LMWH, we believe the lack of alteration to clot microstructure following administration of LMWH is more likely a pathological effect of COVID-19 infection.
In blood, a lower df represents a looser fibrin network with fewer branch points, and as such, a developing clot will take longer to transition from displaying fluid-like to solid-like properties. The pro-inflammatory state seen in COVID-19 appears to disrupt the widely held relationship seen in polymers –both biological and non-biological - between df and TGP in which a change in one variable predictably corresponds with a change in the other. A prolonged TGP would ordinarily be accompanied by a lower df, and yet in COVID-19, LMWH is able to prolong TGP without a change in df. Across a range of disease states, this relationship between df and TGP has been maintained even when the pathophysiology of disease leads to a coagulopathy. COVID-19 appears to be an outlier in disrupting this relationship. Studies in healthy volunteers with ex-vitro addition of heparin also demonstrated reciprocal changes to df and TGP, so it does not appear likely that heparin is the causative agent for this disruption. The only previous instance of a similar finding was in patients receiving warfarin therapy following first episode of VTE [28]. Comparing these patients to patients receiving warfarin for non-VTE indications (e.g atrial fibrillation or metallic heart valve), there was a significant difference in df but not in TGP. In both VTE and non-VTE patients, TGP was appropriately prolonged by warfarin. But only in the non-VTE group was df reduced below the normal healthy range. Further analysis of the VTE group indicated a sub-group of patients in which df remained high despite warfarin therapy, and patients who subsequently suffered recurrent VTE despite anticoagulation were all from this sub-group in which df remained high following warfarin therapy. The factor which uncoupled df from TGP in recurrent VTE was not identified, recurrence was not due to sub-therapeutic INR readings. Both the VTE study and this work in COVID-19 would indicate there are pathways by which coagulation can be modified which remain poorly understood.
It is not known what factor has led to the disruption of the relationship between df and TGP. But understanding this change may offer new insights into thromboembolic diseases and provide a better understanding of the reasons why anticoagulant therapies are unsuccessful in the treatment or prevention of thromboembolic disease in some patients. This additional understanding may lead on to the identification of new targets for pharmacological agents, or the development of additional biomarkers in coagulation.
Although this study has not linked this reduction in efficacy with an increased incidence of PE or DVT, it was not powered or designed to do so. While there has been a link between increased thrombogenicity and patient outcome in COVID-19, an important step would be to link these via pathological processes such as PE and DVT. This follow up work may provide evidence to warrant studies into LMWH dosing in COVID-19 patients and whether more aggressive anticoagulation strategies are of benefit.
As seen across a wide range of the literature, demographic factors such as age and co-morbidity, and inflammatory markers such as CRP continue to be predictive of severe disease and death.
5Limitations
Some patients were not admitted to ICU at the discretion of the clinical team if pre-existing co-morbidity and functional status indicated an ICU admission would not be in their best interests. This may mean some patients were misclassified based on the classification criteria used. In some cases this did not affect the analysis, e.g a patient who was not admitted to ICU, and subsequent died on the ward and therefore would be classed as critical in either case. However, there were other patients whose physiological condition would normally indicate an ICU admission, but due to baseline functional status were not escalated to ICU, and then survived a ward based stay in hospital. In these instances critically unwell patients were downclassed as a severe case meaning a type II statistical error was more likely.
While the study did not collect blood beyond day 7 of admission, there were some patients who either died later in their stay, or deteriorated later in their admission and only then required an ICU admission. In these patients it is therefore unknown whether visco-elastic testing was deranged as their clinical condition worsened. While the majority of deaths occurred within or close to the first 7 days, the longest period between admission and death was 25 days.
6Conclusions
At the time of presentation to the ED, visco-elastic testing does not predict the severity of COVID-19 infection. However, there is significant evidence to suggest that LMWH therapy does not induce the same effect in COVID-19 patients as it would in other cohorts. It is likely that this explains some of the additional burden of thromboembolic disease seen in these patients. Further studies are required to translate this laboratory finding into clinical practice.
Additionally, the coagulopathy of COVID-19 appears to induce unique pathophysiological changes to clot microstructure. Understanding how these changes are generated may offer crucial insights into thromboembolic diseases and the possibility to develop new biomarkers in coagulation or provide additional pharmaceutical targets to treat disease.
Acknowledgments
We received a Welsh Government Sêr Cymru III grant to undertake this research - MA/KW/1457/20. WG Project Number: 32. We would also like to thank the clinical staff in Morriston Hospital Emergency Department and the Ed Major Critical Care Unit for their assistance in identifying participants and facilitating sample collection.
References
[1] | Iba T , Levy JH , Levi M , Connors JM , Thachil J . Coagulopathy of Coronavirus Disease 2019, Crit Care Med (2020) ;48: (9):1358–1364. doi: 10.1097/CCM.0000000000004458. |
[2] | Jung EM , Stroszczynski C , Jung F . Contrast enhanced ultrasonography (CEUS) to detect abdominal microcirculatory disorders in severe cases of COVID-19 infection: First experience, Clin Hemorheol Microcirc (2020) ;74: (4):353–361. doi: 10.3233/CH-209003. |
[3] | Chen T , Wu D , Chen H , Yan W , Yang D , Chen G .et al. Clinical characteristics of 113 deceased patients with coronavirus retrospective study, BMJ. (2020) ;368: :m1295. doi: 10.1136/bmj.m1091. |
[4] | Watson O , Pillai S , Howard M , Zaldua JC , Whitley J , Burgess B . et al., Impaired fibrinolysis in severe Covid-19 infection is detectable in early stages of the disease. Clinl Haemorheol Microcirc. (2022). DOI: 10.3233/CH-221491. |
[5] | Creel-Bulos C , Auld SC , Caridi-Scheible M , Barker NA , Friend S , Gaddh M . Fibrinolysis Shutdown and Thrombosis in a COVID-19 ICU, Shock (2021) ;55: (3):316–320. doi: 10.1097/SHK.0000000000001635. |
[6] | Wright FL , Vogler TO , Moore EE , Moore HB , Wohlauer MV , Urban S . et al., Fibrinolysis Shutdown Correlation with Thromboembolic Events in Severe COVID-19 Infection, J Am Coll Surg (2020) ;231: (2):193–203. doi: 10.1016/j.jamcollsurg.2020.05.007. |
[7] | Hartmann J , Ergang A , Mason D , Dias JD . The Role of TEG Analysis in Patients with COVID-19 Associated Coagulopathy: A Systematic Review, Diagnostics (Basel). (2021) ;11: (2):172. doi: 10.3390/diagnostics11020172. |
[8] | Bareille M , Hardy M , Douxfils J , Roullet S , Lasne D , Levy JH . et al., Viscoelastometric Testing to Assess Hemostasis of COVID- A Systematic Review, J Clin Med (2021) ;10: (8):1740. doi: 10.3390/jcm10081740. |
[9] | Stattin K , Lipcsey M , Andersson H , et al. Inadequate prophylactic effect of low-molecular weight heparin in critically ill COVID-19 patients, J Crit Care (2020) ;60: :249–252. doi: 10.1016/j.jcrc.2020.08.026. |
[10] | Vlot EA , Van den Dool EJ , Hackeng CM , Sohne M , Noordzij PG , Van Dongen EPA . Anti Xa activity after high dose LMWH thrombosis prophylaxis in covid 19 patients at the intensive care unit, Thromb Res (2020) ;196: :1–3. doi: 10.1016/j.thromres.2020.07.035. |
[11] | Streng AS , Delnoij TSR , Mulder MMG , et al., Monitoring of Unfractionated Heparin in Severe COVID- An Observational Study of Patients on CRRT and ECMO, TH Open.-. Published Nov 19 (2020) ;4: (4):e365–e375. Published 2020 Nov 19. doi: 10.1055/s-0040-1719083. |
[12] | Trunfio M , Salvador E , Cabodi D , et al., Anti-Xa monitoring improves low-molecular-weight heparin effectiveness in patients with SARS-CoV-2 infection, Thromb Res (2020) ;196: :432–434. doi: 10.1016/j.thromres.2020.09.039. |
[13] | Kofteridis DP , Ioannou P , Kondili E , Chamilos G , Filippatos TD . Personalized prophylactic anticoagulation in hospitalized patients with Covid-19 - The role of anti-Xa monitoring, Clin Microbiol Infect (2021) ;27: (8):1188–1189. doi: 10.1016/j.cmi.2021.04.024. |
[14] | Smith GB , Prytherch DR , Meredith P , Schmidt PE , Featherstone PI . The ability of the National Early Warning Score (NEWS) to discriminate patients at risk of early cardiac arrest, unanticipated intensive care unit admission, and death, Resuscitation (2013) ;84: (4):465–470. doi: 10.1016/j.resuscitation.2012.12.016. |
[15] | Kim I , Song H , Kim HJ , et al. Use of the National Early Warning Score for predicting in-hospital mortality in older adults admitted to the emergency department, Clin Exp Emerg Med (2020) ;7: (1):61–66. doi: 10.15441/ceem.19.036. |
[16] | Kostakis I , Smith GB , Prytherch D , et al. The performance of the National Early Warning Score and National Early Warning Score 2 in hospitalised patients infected by the severe acute respiratory syndrome coronavirus 2 (SARS-CoV-2), Resuscitation (2021) ;159: :150–157. doi: 10.1016/j.resuscitation.2020.10.039. |
[17] | Myrstad M , Ihle-Hansen H , Tveita AA , et al. National Early Warning Score 2 (NEWS2) on admission predicts severe disease and in-hospital mortality from Covid-19 - a prospective cohort study, Scand J Trauma Resusc Emerg Med. (2020) ;28: (1):66. Published Jul 13 doi: 10.1186/s13049-020-00764-3. |
[18] | Dadras O , Afsahi AM , Pashaei Z , et al. The relationship between COVID-19 viral load and disease severity: A systematic review, Immun Inflamm Dis. (2022) ;10: (3):e580. doi: 10.1002/iid3.580. |
[19] | Wong HYF , Lam HYS , Fong AH , et al. Frequency and Distribution of Chest Radiographic Findings in Patients Positive for COVID-19, Radiology (2020) ;296: (2):E72–E78. doi: 10.1148/radiol.2020201160. |
[20] | Alzoughool F , Alanagreh L , Abumweis S , Atoum M . Cerebrovascular comorbidity, high blood levels of C-reactive protein and D-dimer are associated with disease outcomes in COVID-19 patients, Clin Hemorheol Microcirc. (2021) ;77: (3):311–322. doi: 10.3233/CH-201002. |
[21] | Gallo Marin B , Aghagoli G , Lavine K , et al. Predictors of COVID-19 severity: A literature review, Rev Med Virol (2021) ;31: (1):1–10. doi: 10.1002/rmv.2146. |
[22] | Evans PA , Hawkins K , Morris RH , et al. Gel point and fractal microstructure of incipient blood clots are significant new markers of hemostasis for healthy and anticoagulated blood, Blood. (2010) ;116: (17):3341–3346. doi: 10.1182/blood-2010-02-269324. |
[23] | Lawrence MJ , Marsden N , Kaczynski J , et al. An Investigation Into the Effects of In Vitro Dilution With Different Colloid Resuscitation Fluids on Clot Microstructure Formation, Anesth Analg (2016) ;123: (5):1081–1088. doi: 10.1213/ANE.0000000000001536. |
[24] | Lawrence MJ , Kumar S , Hawkins K , et al. A new structural biomarker that quantifies and predicts changes in clot strength and quality in a model of progressive haemodilution, Thromb Res (2014) ;134: (2):488–494. doi: 10.1016/j.thromres.2014.05.039. |
[25] | Health Research Authority. UK [https://www.hra.nhs.uk]. Retrieved 30/05/2022. Available from: https://www.hra.nhs.uk/planning-and-improving-research/application-summaries/research-summaries/thrombogenicity-in-acute-exacerbation-of-copd/ |
[26] | Roncon L , Zuin M , Barco S , Valerio L , Zuliani G , Zonzin P . et al. Incidence of acute pulmonary embolism in COVID-19 patients: Systematic review and meta-analysis, Eur J Intern Med (2020) ;82: :29–37. doi: 10.1016/j.ejim.2020.09.006. |
[27] | Davies GR , Pillai S , Lawrence M , et al. The effect of sepsis and its inflammatory response on mechanical clot characteristics: a prospective observational study, Intensive Care Med. (2016) ;42: (12):1990–1998. doi: 10.1007/s00134-016-4496-z. |
[28] | Lawrence MJ , Sabra A , Mills G , et al. A new biomarker quantifies differences in clot microstructure in patients with venous thromboembolism, Br J Haematol (2015) ;168: (4):571–575. doi: 10.1111/bjh.13173. |