Monitoring of the microcirculation in children undergoing major abdominal and thoracic surgery: A pilot study
Abstract
BACKGROUND:
Monitoring of the macrocirculation during surgery provides limited information on the quality of organ perfusion.
OBJECTIVE:
We investigated the feasibility of perioperative microcirculatory measurements in children.
METHODS:
Sublingual microvessels were visualized by handheld videomicroscopy in 11 children (19 mo – 10 yrs) undergoing surgery > 120 min at four time points: T0) after induction of anesthesia; T1) before end of anesthesia, T2) 6 h post surgery and T3) 24 h post surgery.
RESULTS:
Measurements were feasible in all children at T0 and T1. At T2 and T3, imaging was restricted to 6 and 4 infants, respectively, due to respiratory compromise and missing cooperation. The capillary density was reduced at T1 compared to T0 (8.1 mm/mm2 [4.0-17.0] vs. 10.6 mm/mm2 [5.1-19.3]; p = 0.01), and inversely related to norepinephrine dose (Pearson r = -0.65; p = 0.04). Microvascular flow and serum glycocalyx makers Syndecan-1 and Hyaluronan increased significantly from T0 to T1.
CONCLUSION:
Perioperative microcirculatory monitoring in children requires a high amount of personal and logistic resources still limiting its routine use. Major surgery is associated with microvascular alterations and glycocalyx perturbation. The possible consequences on patient outcome need further evaluation. Efforts should concentrate on the development of next generation devices designed to facilitate microcirculatory monitoring in children.
1Introduction
Major surgery in children is often accompanied by hemodynamic compromise counteracted with fluid- and catecholamine treatment with the goal to preserve organ perfusion and tissue oxygenation [1]. Traditionally, parameters of the macrocirculation such as blood pressure and heart rate are used to guide treatment decision and monitor treatment success [2]. However, it was demonstrated in critical ill adults and children that correction of blood pressure and cardiac output may not be paralleled by an equal increase in microcirculatory perfusion [3–6]. The uncoupling of macro- and microcirculation, a condition termed “loss of hemodynamic coherence”, can result in persistent microcirculatory dysfunction associated with organ failure and adverse outcome [7]. Indeed, measures aiming at hemodynamic stabilization of the patient such as fluid resuscitation and high doses of vasopressors may even contribute to and worsen loss of hemodynamic coherence. Therefore, resuscitation strategies focusing on optimization of perfusion instead of blood pressure may help to improve outcome [8].
To assess the state of the microcirculation and organ perfusion, surrogate parameters such as capillary refill time, urine production and serum lactate levels are used, however these parameters are confounded by various factors thus limiting their diagnostic value [1]. The development of Handheld Vital Microscopy (HVM), has enabled direct visualization of microvessels in the skin and mucous membranes allowing microcirculatory monitoring during surgery [9]. In children, HVM has been successfully applied to evaluate microvessel density and blood flow as well as endothelial glycocalyx in acute and chronic conditions such as sepsis, respiratory failure and diabetes mellitus [10–12]. With respect to perioperative microcirculatory alterations, there is still very limited data in children concentrating on cardiac surgery [13–15]. In principle, HVM is easy to learn and apply, however it requires cooperation of the patient which hinders its use in younger children. Furthermore, in the perioperative setting, access to the patient is limited and measurements need to fit into the perioperative routine which is usually strictly timed. In this pilot study, we investigated the feasibility of HVM to monitor the sublingual microcirculation in children undergoing major abdominal and thoracic surgery and the effect of surgery on microcirculatory parameters.
2Methods
2.1Study design and patient recruitment
The study was conducted as a single-center prospective observational study at the Children’s University Hospital of the Ludwig-Maximilians-University, Munich, Germany. Children planned for major abdominal or thoracic surgery were recruited over a period of 12 months and included whenever a researcher of the team was available. Exclusion criteria included duration of surgery < 120 min, septic shock, cranio-cerebral injury, cardiovascular surgery, and acute arrhythmias. Induction medication included propofol in combination with sevofluran and atracurium. For maintenance of anesthesia all children received sevoflurane except for one child who was kept on propofol. All children had arterial blood pressure monitoring and central venous catheters. Administration and choice of fluids and blood products as well as vasoactive drugs was up to the discretion of the anesthesiologist during surgery and the intensive care team after transfer to the PICU.
2.2Ethical approval
The study was approved by the ethics committee of the medical faculty of the Ludwig-Maximilian-University, Munich, Germany (Approval No. 319-16). Written informed consent was obtained from the parents or legal guardians and the patients as far as possible according to age. The experiments were carried out in accordance with the Declaration of Helsinki.
2.3Recordings and analysis of the microcirculation
Intravital recordings of the sublingual microcirculation were performed using Sidestream Dark Field (SDF) imaging technology as previously described [16, 17]. Images were obtained with the Microscan videomicroscope (5x-magnification; Microvision Medical, Amsterdam, Netherlands) by two researchers not involved in patient care. Consensus guidelines for image acquisition and quality criteria for off-line analysis were followed as far as possible [18, 19]. This included prior training of the persons performing measurements, recording of at least 3 sites for microcirculatory analyses and 3 sites for glycocalyx measurement and efforts to minimize pressure artefacts and guarantee sufficient image quality. Recordings were planned at the following time points: T0 = before surgery after induction of anesthesia, T1 = immediately after surgery before end of anesthesia, T2 = 6 h post surgery, T3 = 24 h post surgery.
The following microcirculatory parameters were assessed using the Automated Vascular Analysis program (AVA 3.2, MicroVision Medical Amsterdam, The Netherlands):
– Total Vessel Density (TVD)=cumulative length of all vessels per field of view in mm/mm2
– Diameter distribution = percentage of total vessel length of small (<10 μm), medium (10-25 μm) and large (>25 μm) vessels.
– Microcirculatory Flow Index (MFI)=semiquantitative measure of blood flow quality. In every quadrant of the image, a flow quality score is assigned for each vessel category. Flow qualities were defined as 0 = no flow, 1 = intermittent, 2 = sluggish, 3 = continuous (normal), and 4 = hyperdynamic flow [20]. The MFI was calculated for every vessel category as mean of the flow qualities of every quadrant and the mean of the vessel categories was taken for further statistical analysis.
2.4Analysis of the endothelial glycocalyx
The endothelial glycocalyx was evaluated locally by SDF-imaging and globally by quantification of circulating glycocalyx components Syndecan-1 and Hyaluronan. A specialized software (GlycoCheck, Microvascular Health Solutions Inc., Salt Lake City, UT, USA) was used to calculate the Perfused Boundary Region (PBR; μm) as a surrogate for the microvascular glycocalyx thickness in SDF-recordings of sublingual vessels as described before [21]. Briefly, the program measures the width of the red blood cell column (RBCW) within vessels of 5-25 μm diameter based on the light intensity profile along perpendicular vessel line markers. The PBR representing the most luminal part of the endothelial glycocalyx penetrable to erythrocytes, is defined as ½ x (maximum – median RBCW). An intact thick glycocalyx limits lateral movements of red blood cells, leading to a small PBR. When the glycocalyx is shed or disturbed, red blood cells can move closer to the vessel wall, represented by an increase in PBR.
Serum syndecan-1 and hyaluronan were measured before (T0) and immediately after surgery (T1) via enzyme-linked immunosorbent assays (Human sCD138 (Syndecan-1) ELISA Kit (Diaclone SAS, Besancon Cedex, France) and Hyaluronan Enzyme-Linked Immunosorbent Assay Kit (Echelon Biosciences Inc., Salt Lake City, UT), using a microplate reader SPECTROstar Omega (BMG LABTECH, Ortenberg, Germany) according to manufacturers’ instructions. Assessing the assay reproducibility, we noted that the range of intra-assay coefficient of variation (CV) amounted to 0.54-12.8%, being higher only in one sample for Hyaluronan with a CV of 21%. In relation to the range of the standard curves (syndecan-1 : 8-256 ng/ml; Hyaluronan: 50-1600 ng/ml), we identified one post-surgery sample exceeding the highest standard point of the calibration curve for syndecan-1 even though the sample was diluted for the analysis (dilution factor 1.5). This value was taken for the statistical analysis as 256 ng/ml, which is the highest concentration considered within the calibration curve for syndecan-1 and therefore reliable. The values for Syndecan-1 and Hyaluronan were corrected for individual total protein concentrations to account for postoperative hemodilution. For this purpose, the total protein concentration at T0 was defined as being 100% and the Syndecan-1 and Hyaluronan values obtained at T1 were divided by the ratio of post/preoperative total protein concentration.
2.5Clinical and laboratory data
For every patient age, gender, body weight and height, main diagnosis, surgical procedure and duration of surgery were noted. Furthermore, at every measurement time point blood pressure, heart rate, body temperature, fluid and volume balance, urine output, doses of vasoactive drugs and respiratory support were registered. Laboratory data was obtained by arterial blood gas analysis (pH, pO2, pCO2, Base Excess, HCO3, serum lactate) and routine blood work from venous samples (hemoglobin, hematocrit, platelet- and leukocyte count, total serum protein, creatinine, C-reactive Protein and blood glucose).
2.6Statistical evaluation
For each parameter, the mean of the repeated measurements per time point and child was used. Statistical analyses and graphical presentation were performed with GraphPad Prism 8.2 (GraphPad Software, San Diego, CA, USA). Normal distribution was tested with the Shapiro-Wilk test. Comparison between two measurement time points was done with a paired t-test or a Wilcoxon matched-pairs signed rank test, as appropriate. Comparison of > two measurement time points was done with a repeated measures ANOVA, or a Friedman test followed by Tuckey’s multiple comparison test or by Dunn’s multiple comparisons test, respectively. Simple correlation between two parameters was tested with Pearson correlation. A p-value<0.05 was considered as statistically significant.
3Results
3.1Patient and surgical characteristics
During the study period a total of 38 children were scheduled for major abdominal or thoracic surgery. Of these, 11 were recruited in the study. Main reasons for missing recruitment were logistic reasons (no researcher available to perform measurements), parental refusal of study participation and language barriers. Patient demographics, diagnoses and information concerning the surgical procedure are provided in Table 1. Mean age of participants was 74 months (median 78 months; range: 19-120) and mean weight was 21±12 kg (Median 16.5 kg) with 55% of the children being female. The mean duration of the surgery was 314±118 min (median 271 min).
Table 1
Patient and surgical characteristics
Patient | Age (months) | Gender | Weight (kg) | Diagnosis | Surgical procedure | Duration of surgery (min) |
1 | 35 | Male | 11.5 | Neuroblastoma | Tumor resection | 493 |
2 | 118 | Female | 34 | FNH | Segmental resection | 440 |
3 | 120 | Female | 29 | FNH | Segmental resection | 433 |
4 | 39 | Female | 15 | Nephroblastoma | Tumor resection | 222 |
5 | 114 | Female | 22 | Caustic esophageal stenosis | Esophagus replacement | 405 |
6 | 119 | Male | 38 | Pheochromocytoma | Nephrectomy (left) | 251 |
7 | 78 | Female | 16.5 | Embryonal RMS | Tumor resection | 150 |
8 | 104 | Female | 39 | Phakomatosis liver | Hemihepatectomy | 201 |
9 | 34 | Male | 8.8 | IMT | Partial resection | 271 |
10 | 35 | Male | 10 | IMT | Partial resection | 208 |
11 | 19 | Male | 8 | Neuroblastoma | Tumor resection | 381 |
FNH = Focal nodular hyperplasia, IMT = inflammatory myofibroblastic tumor, RMS = rhabdomyosarcoma.
3.2Feasibility of microcirculatory measurements
In all children microcirculatory measurements were obtained at T0 and T1. However, due to logistic obstacles and time restraints we were not able to acquire the targeted number of recordings in all patients and some recordings had to be excluded from further analysis due to insufficient image quality. Suppl. Table 1 provides detailed information on the number of recordings per child and measurement time point. Measurements at T0 were performed at the anesthesia induction room (Fig. 1), while measurement at T1 needed to take place at the operating room as children were directly transferred to the PICU after completion of surgery without going to the recovery room. Post-surgery measurements at T2 und T3 were successful only in 6 and 4 children, respectively (for details see Suppl. Table 1). The main reasons hindering recordings were respiratory instability and arousal in children still intubated and agitation/missing cooperation in extubated children.
Fig. 1
Picture of the anesthesia induction room with the “microcirculation-imaging unit” including SDF-camera, computer, and monitor. To allow flexible recordings and changes between different locations (induction room, operating room, PICU), the equipment was placed on a mobile instrument card and provided with an uninterruptible power supply.
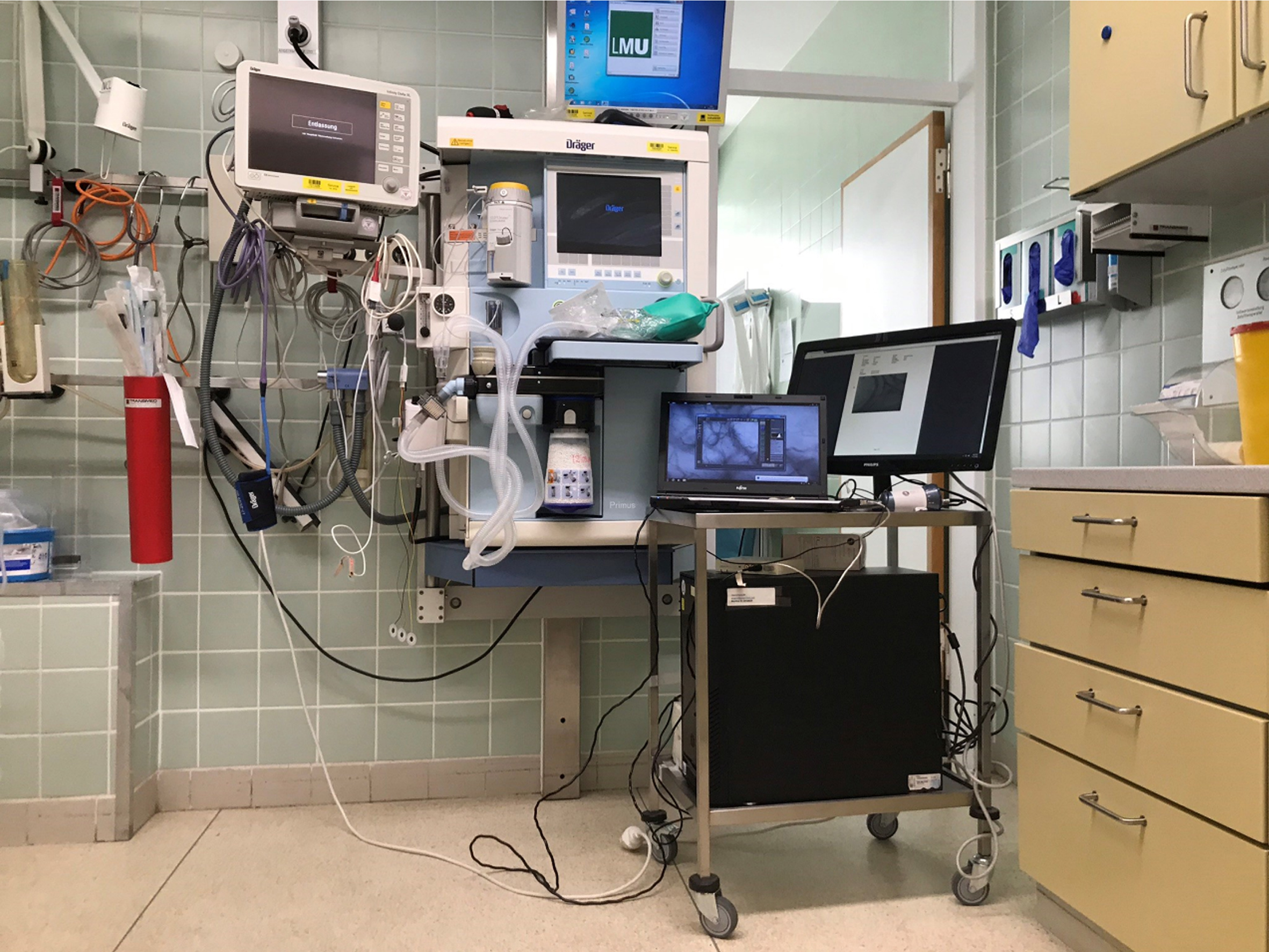
3.3Clinical and laboratory data
During surgery, children received on average 16 ml/kg bw/h infusion of crystalloid solutions. The average blood loss was 28.5 ml/kg, and for volume replacement an average of 15 ml/kg bw colloidal solutions and 33 ml/kg bw blood products (erythrocyte concentrate, fresh frozen plasma and platelet concentrate) were administered (Suppl. Table 2). The amount of crystalloids (ml/kg/h) was indirectly correlated with the body weight of the children (Pearson r = -0.72, p = 0.001), but not with the duration of surgery. By contrast, the amount of colloids and blood products (ml/kg bw) showed direct correlation with the duration of surgery (Pearson r = 0.64, p = 0.03) and the intraoperative blood loss (Pearson r = 0.85, p = 0.0009), but not with the bodyweight of the children.
None of the children was treated with vasoactive agents prior to surgery. All children received a continuous infusion of norephinephrine during surgery and 5 children were treated with single doses of cafedrine/theodrenaline after induction of anesthesia. The average dose of norepinephrine (0.11 μg/kg/min; range 0.006 – 0.35) was significantly associated with the amount of crystalloids administered during surgery (Pearson r = 0.71, p = 0.001).
Mean arterial blood pressure (MAP) and heart rate (HR) did not change significantly from T0 to T1 (MAP 64±11 mmHg vs. 69±12 mmHg; HR 107±17 bmp vs. 110±13 bpm), whereas body temperature was significantly higher at T1 compared to T0 (36.4±0.7 °C vs. 37.1±0.8 °C; p = 0.035). With respect to laboratory data, we observed a significant rise of serum lactate and creatinine from T0 to T1, while platelet count and total serum protein were significantly reduced. No significant change in hemoglobin and hematocrit was seen (Suppl. Table 3).
3.4Microcirculatory data
Due to missing recordings at T2 and T3, we chose to perform two separate analyses. First, we analyzed data of all children (n = 11) including only the time points T0 and T1. Secondly, we analyzed the subgroup of children with measurements available for the timepoints T0 to T2 (n = 6). We also plotted the data of children with measurements available for all timepoints (n = 4), however did not perform a statistical analysis due to the low n-number (Supplemental Fig. 1).
TVD and MFI for all vessel categories combined did not significantly change. However, when looking at the density of small vessels (<10 μm), we saw a significant decrease from T0 to T1 (Fig. 2A). Similarly, the MFI in small vessels showed a significant change with increased values at T1 compared to T0 (Fig. 2B). Analysis of the vessel diameter distribution confirmed the reduction of small sized vessels after surgery by 15% compared to pre-surgery values, whereas medium sized vessels (10-25 μm diameter) increased by 22% (Fig. 2C). Looking at the subgroup of patients with recordings available at T2, these changes seem to be acute and transient as both, small vessel density, MIF and vessel diameter distribution showed a return towards preoperative values already six hours after surgery (Fig. 2D-F).
Fig. 2
Perioperative changes of microcirculatory parameters in sublingual vessels. Panels A-C include data of all children (n = 11), panels D-F include data from a subgroup of children (n = 6) with recordings available at timepoint T2. Small vessel density (A) decreased and the microvascular flow in small vessels (B) increased significantly from T0 to T1. The proportion of small vessels (<10 μm diameter) decreased significantly during surgery, while the proportion of medium vessels (10-25 μm diameter) increased significantly (C). Follow-up recordings 6 h post-surgery (T2) demonstrate a return of small vessel density (D), MFI (E), and diameter distribution (F) towards preoperative values.
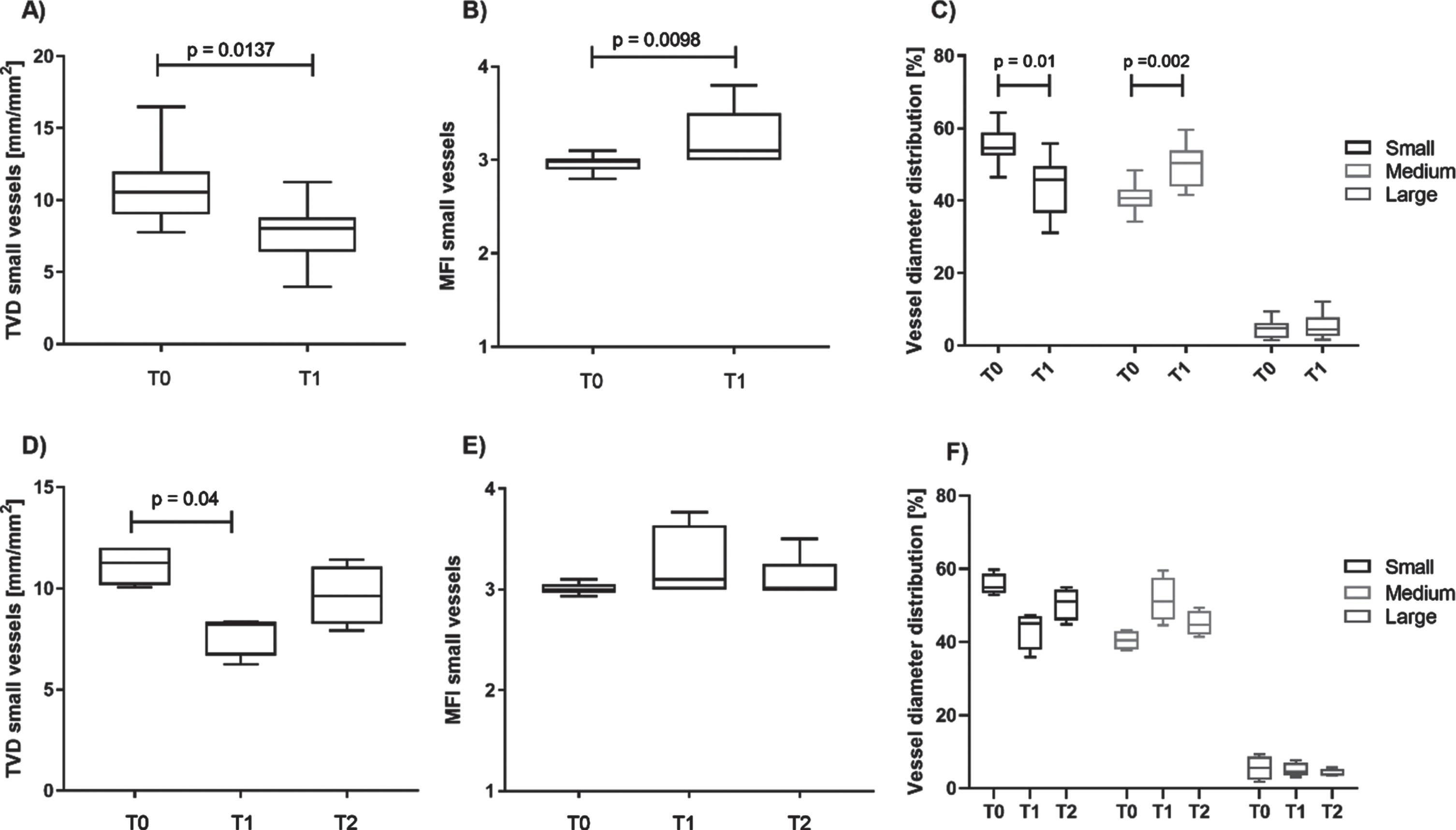
There was no significant change in the PBR as surrogate for local endothelial glycocalyx from T0 to T1 (Fig. 3A and B). By contrast, we found a significant rise in the concentration of Syndecan-1 and Hyaluronan in the serum at T1 (Fig. 3C and D).
Fig. 3
Perioperative evaluation of parameters of the endothelial glycocalyx. No significant change in the perfused boundary region (PBR) in sublingual vessels was observed from T0 to T1 (A) nor in the subgroup of children with recordings for timepoints T0 to T2 (B). Circulating serum levels of Syndecan-1 (C) and Hyaluronan (D) increased during surgery (values are corrected for total serum protein).
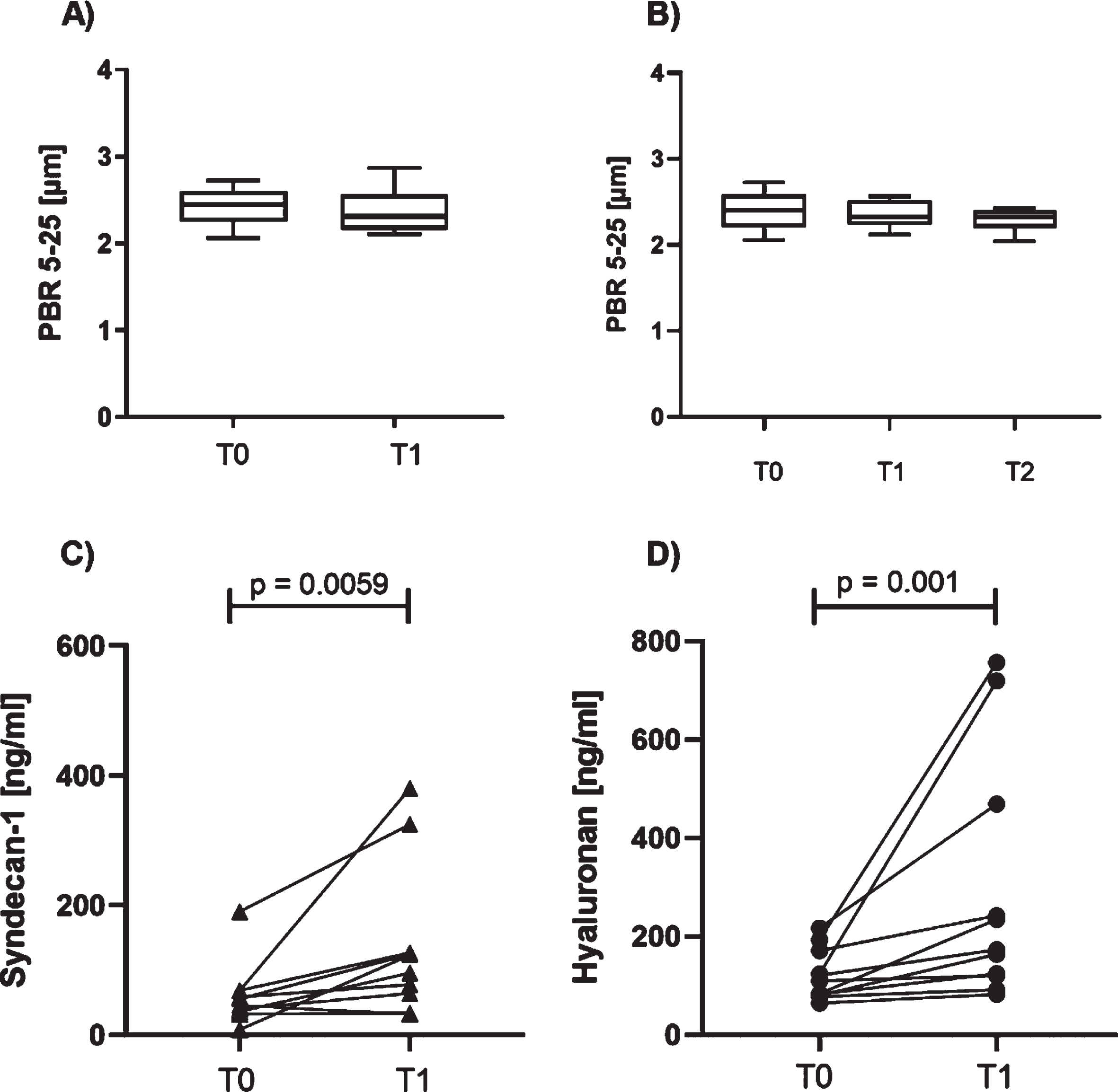
3.5Correlation of microcirculatory parameters and clinical variables
None of the microcirculatory parameters showed a correlation with blood pressure or heart rate. Interestingly, while we found a significant correlation of the small vessel density with PaO2 at T0 (Pearson r = 0.67, p = 0.025), this association was lost at T1 (r=-0.02, p = 0.9). However, the small vessel density at T1 was inversely related to the norepinephrine dose (Pearson r = -0.65 und p = 0.04). Furthermore, the small vessel MFI at T0 as the only microcirculatory variable was negatively associated with pre- and postoperative lactate levels (Pearson r = -0.78, p = 0.004 and r = -0.64, p = 0.037, respectively). The relative increase in % in serum Syndecan-1 from T0 to T1 showed a positive correlation with the dose of norepinephrine (Pearson r = 0.66, p = 0.037) and with the postoperative level of Hyaluronan (r = 0.76; p = 0.011). No other correlation of Syndecan-1 levels with microcirculatory parameters was observed. We did not find an association of microcirculatory data with the type and amount of resuscitation fluids infused during surgery nor with other laboratory parameters such as serum creatinine.
4Discussion
The aim of this pilot study was to evaluate the feasibility of sublingual microcirculatory measurements in the perioperative setting and to provide first data on the effect of major surgery on microcirculatory parameters in children.
The rationale to monitoring the microcirculation is based on the recognition that in critically ill patients improvement of macrohemodynamic parameters such as blood pressure are not necessarily followed by an increase of organ perfusion and tissue oxygenation [7]. Therefore, direct information on the condition of the microcirculation may help to guide resuscitation strategies and improve patient outcome [22–24].
Microcirculatory monitoring in critically ill patients and especially in children can be challenging. HVM has been designed to image the microcirculation in adults and its use in the pediatric population is limited by body size and lacking cooperation. Gonz
We observed significant changes in sublingual microcirculatory parameters following major surgery with a reduction in the small vessel density and the proportion of small vessels. As oxygen and nutrient exchange with the tissue happens at the level of the smallest vessels (i.e. capillaries), these changes might lead to tissue hypoxia and contribute to postoperative organ failure [26–28]. Jhanji and colleagues reported a significantly reduced functional capillary density and a lower proportion of perfused small vessels in adults who developed complications after major abdominal surgery compared to patients with uncomplicated postoperative course [29]. A persistent reduction in the functional small vessel density was associated with adverse outcome and mortality in critically ill adults and children [10, 30, 31]. As a limitation of our study, we were not able to investigate the relationship between microcirculatory alterations and postoperative outcome due to the low number of patients.
The effect of surgery on microvascular flow seems to be more heterogenous. A recent meta-analysis by Chalkias and colleagues on studies investigating perioperative changes of the sublingual microcirculation found an overall decrease in MFI [17]. However, there was a high degree of heterogeneity between studies, and some of the studies also found higher microvascular flow in the immediate postoperative period as seen in our cohort [32, 33]. Likely, variations in the measurement time point, the type of surgery inducing different degrees of inflammation as response to surgical trauma and possible transient bacteremia during abdominal surgery and the use of vasoactive drugs contribute to these differences. In view of the decreased proportion of small vessels at T1, the increased microvascular flow may be consequence of the lower microvessel cross sectional area. Furthermore, changes in hematocrit can significantly influence erythrocyte velocity in the microcirculation and tissue oxygenation [34, 35]. The observation of a variable association of the vessel density with PaO2 and of the pre-surgery MFI with lactate levels emphasizes the complex nature of microcirculatory regulation and its interplay with tissue metabolism, which are subject to various influences including known factors such as reduced oxygen consumption during post-aggression metabolism [36] as well as unknown factors.
The changes in small vessel density and in MFI occurred independent of blood pressure and heart rate confirming the concept of loss of hemodynamic coherence that has been described before in critically ill pediatric patients [1]. Actually, some of the measures aiming at improvement of macrohemodynamic variables may even worsen microcirculatory dysfunction, such as excessive fluid administration and high doses of vasopressors. In line with this notion, we found an inverse relationship between the small vessel density and the amount of intraoperative infused norepinephrine. As demonstrated by Dubin et al, targeting blood pressure by use of escalating doses of norepinephrine in patients with septic shock did not improve microcirculatory flow index but tended to decrease capillary perfused density [37]. Yet, the microcirculatory changes observed in our patients cannot be solely attributed to the vasoconstrictive effect of norepinephrine since measurements 6 hours post-surgery showed a near normalization of microcirculatory parameters despite ongoing use of norepinephrine in these children.
In the present study, we did not observe perioperative changes in PBR, a parameter of local microvascular glycocalyx thickness. This contrasts with an earlier study of our group on children undergoing cardiac surgery [13]. However, shedding of the glycocalyx in cardiovascular surgery is likely related to the use of cardiopulmonary bypass and not the surgical procedure itself [13, 38]. Looking at the amount of circulating glycocalyx components revealed a significant postoperative increase in the levels of Syndecan-1 and Hyaluronan. Likely, PBR shedding occurs mainly in the organs affected during surgery and therefore is not reflected in local PBR measurements of the sublingual microcirculation. Intravenous fluid therapy and volume overload have been associated with glycocalyx shedding [39, 40]. Apart from the amount of resuscitation fluid administered, the extent of glycocalyx shedding also depends on the type of fluid used. In an animal model of hemorrhagic shock, shedding of Syndecan-1 and Hyaluronan was most pronounced after resuscitation with crystalloid solution whereas blood or blood derived products affected glycocalyx thickness the least [41]. In our study, children received on average 16 ml/kg bw/h i.v. crystalloids which resembles a moderate intraoperative fluid regimen [42]. By contrast, the amount of infused colloids (mainly albumin 5%) and blood products with a mean of 15 ml/kg bw and 33 ml/kg bw, respectively, was rather high. Apart from the beneficial profile of blood derived products compared to crystalloids with respect to glycocalyx shedding, albumin has been suggested to directly contribute to glycocalyx restoration [43]. Therefore, stable PBR in combination with increased levels of circulating glycocalyx components might be expression of a high glycocalyx turn over with constant rebuilding on a local microvascular level.
Interestingly, the increase in serum Syndecan-1 was directly related to the dose of norepinephrine applied during surgery. Due to the observational character of our study we can only speculate about the nature of this association. The norepinephrine dose was significantly related to the amount of crystalloids infused during surgery and thus might go hand in hand with the possible negative effects of crystalloids on glycocalyx integrity. However, we did not find a direct association of circulating Syndecan-1 levels with fluid or volume therapy. It is intriguing to argue the other way around i.e. that loss of the glycocalyx during surgery alters vascular reactivity and permeability resulting in hemodynamic instability that is answered by higher norepinephrine doses and fluid administration. In support of this hypothesis, Saoraya et al. recently reported that Syndecan-1 levels in septic patients were predictive of subsequent fluid administration over the next 72 h and correlated with the maximum dose of vasopressors [44]. However, the causal relationship as well as the underlying pathomechanism still need to be established.
While most pediatric studies so far have evaluated the sublingual microcirculation, HVM can also be used to monitor the intestinal microcirculation during surgery [45]. One of the questions that should be addressed in future studies is whether direct assessment of the intestinal perfusion possibly in combination with techniques to judge tissue oxygenation such as near-infrared spectroscopy (NIRS) [46] is helpful to guide surgery and anesthesia and prevent complications. Furthermore, the value of targeting microcirculatory parameters to guide perioperative hemodynamic management and the potential of microcirculatory monitoring to identify patients at risk of adverse postoperative outcome need to be studied prospectively.
5Conclusion
Our study demonstrates that sublingual microcirculatory measurements in children undergoing major surgery are challenging, require a high personal and logistic effort for realization and may be hampered by respiratory instability and lacking cooperation in the further postoperative course. Surgery is associated with transient alterations of the microcirculation, however the consequences for patient outcome deserve further investigation. Next generation devices designed to facilitate microcirculatory monitoring in children and scores integrating different microvascular parameters in a semi-quantitative realtime fashion instead of time-consuming off-line analysis of recordings may help to advance the use of SDF-Imaging in clinical routine.
Acknowledgments
We thank the patients and their families for participation and the teams of the operating room and the PICU for supporting this study.
Supplementary material
[1] The supplementary material is available in the electronic version of this article: https://dx.doi.org/10.3233/CH-221617.
References
[1] | Erdem O , Kuiper JW , Tibboel D . Hemodynamic coherence in critically ill pediatric patients. Best Pract Res Clin Anaesthesiol. (2016) ;30: (4):499–510. |
[2] | Pour-Ghaz I , Manolukas T , Foray N , Raja J , Rawal A , Ibebuogu UN , et al. Accuracy of non-invasive and minimally invasive hemodynamic monitoring: where do we stand? Ann Transl Med. (2019) ;7: (17):421. |
[3] | den Uil CA , Lagrand WK , van der Ent M , Nieman K , Struijs A , Jewbali LS , et al. Conventional hemodynamic resuscitation may fail to optimize tissue perfusion: an observational study on the effects of dobutamine, enoximone, and norepinephrine in patients with acute myocardial infarction complicated by cardiogenic shock. PLoS One. (2014) ;9: (8):e103978. |
[4] | Tachon G , Harrois A , Tanaka S , Kato H , Huet O , Pottecher J , et al. Microcirculatory alterations in traumatic hemorrhagic shock. Crit Care Med. (2014) ;42: (6):1433–41. |
[5] | Buijs EA , Reiss IK , Kraemer U , Andrinopoulou ER , Zwiers AJ , Ince C , et al. Increasing mean arterial blood pressure and heart rate with catecholaminergic drugs does not improve the microcirculation in children with congenital diaphragmatic hernia: a prospective cohort study. Pediatr Crit Care Med. (2014) ;15: (4):343–54. |
[6] | Knaut M , Matschke K , Plotze K , Steinmann C , Mrowietz C , Jung F . Cutaneous and muscular microcirculation in patients with terminal heart failure awaiting transplantation. Clin Hemorheol Microcirc. (2012) ;52: (2-4):217–27. |
[7] | Ince C . Hemodynamic coherence and the rationale for monitoring the microcirculation. Crit Care. (2015) ;19 Suppl 3: :S8. |
[8] | Gazmuri RJ , de Gomez CA . From a pressure-guided to a perfusion-centered resuscitation strategy in septic shock: Critical literature review and illustrative case. J Crit Care. (2020) ;56: :294–304. |
[9] | Nam K , Jeon Y . Microcirculation during surgery. Anesth Pain Med (Seoul). (2022) ;17: (1):24–34. |
[10] | Top AP , Ince C , de Meij N , van Dijk M , Tibboel D . Persistent low microcirculatory vessel density in nonsurvivors of sepsis in pediatric intensive care. Crit Care Med. (2011) ;39: (1):8–13. |
[11] | Top AP , Ince C , Schouwenberg PH , Tibboel D . Inhaled nitric oxide improves systemic microcirculation in infants with hypoxemic respiratory failure. Pediatr Crit Care Med. (2011) ;12: (6):e271–4. |
[12] | Nussbaum C , Cavalcanti Fernandes Heringa A , Mormanova Z , Puchwein-Schwepcke AF , Bechtold-Dalla Pozza S , Genzel-Boroviczeny O . Early microvascular changes with loss of the glycocalyx in children with type 1 diabetes. J Pediatr. (2014) ;164: (3):584-9e1. |
[13] | Nussbaum C , Haberer A , Tiefenthaller A , Januszewska K , Chappell D , Brettner F , et al. Perturbation of the microvascular glycocalyx and perfusion in infants after cardiopulmonary bypass. J Thorac Cardiovasc Surg. (2015) ;150: (6):1474-81e1. |
[14] | Scolletta S , Marianello D , Isgro G , Dapoto A , Terranova V , Franchi F , et al. Microcirculatory changes in children undergoing cardiac surgery: a prospective observational study. Br J Anaesth. (2016) ;117: (2):206–13. |
[15] | Gonzalez Cortes R , Urbano Villaescusa J , Solana Garcia MJ , Lopez Gonzalez J , Fernandez Lafever SN , Ramirez Gomez B , et al. Microcirculatory Changes in Pediatric Patients During Congenital Heart Defect Corrective Surgery. J Cardiovasc Transl Res. (2021) ;14: (6):1173–85. |
[16] | Puchwein-Schwepcke A , Grzybowski AK , Genzel-Boroviczeny O , Nussbaum C . Effects of Prematurity on the Cutaneous Microcirculatory Network in the First Weeks of Life. Front Pediatr. (2019) ;7: :198. |
[17] | Chalkias A , Papagiannakis N , Mavrovounis G , Kolonia K , Mermiri M , Pantazopoulos I , et al. Sublingual microcirculatory alterations during the immediate and early postoperative period: A systematic review and meta-analysis. Clin Hemorheol Microcirc. (2022) ;80: (3):253–65. |
[18] | Ince C , Boerma EC , Cecconi M , De Backer D , Shapiro NI , Duranteau J , et al. Second consensus on the assessment of sublingual microcirculation in critically ill patients: results from a task force of the European Society of Intensive Care Medicine. Intensive Care Med. (2018) ;44: (3):281–99. |
[19] | Massey MJ , Larochelle E , Najarro G , Karmacharla A , Arnold R , Trzeciak S , et al. The microcirculation image quality score: development and preliminary evaluation of a proposed approach to grading quality of image acquisition for bedside videomicroscopy. J Crit Care. (2013) ;28: (6):913–7. |
[20] | De Backer D , Hollenberg S , Boerma C , Goedhart P , Buchele G , Ospina-Tascon G , et al. How to evaluate the microcirculation: report of a round table conference. Crit Care. (2007) ;11: (5):R101. |
[21] | Puchwein-Schwepcke A , Artmann S , Rajwich L , Genzel-Boroviczeny O , Nussbaum C . Effect of gestational age and postnatal age on the endothelial glycocalyx in neonates. Sci Rep. (2021) ;11: (1):3133. |
[22] | Zhou Q , Dai C , Zhu Y , Han T , Zhou J , Zhao L , et al. The effectiveness and feasibility of fluid resuscitation directed by microcirculation monitoring in patients with septic shock: a randomized controlled trial. Ann Palliat Med. (2021) ;10: (8):9069–77. |
[23] | Trzeciak S , McCoy JV , Phillip Dellinger R , Arnold RC , Rizzuto M , Abate NL , et al. Early increases in microcirculatory perfusion during protocol-directed resuscitation are associated with reduced multi-organ failure at 24h in patients with sepsis. Intensive Care Med. (2008) ;34: (12):2210–7. |
[24] | Harrois A , Dupic L , Duranteau J . Targeting the microcirculation in resuscitation of acutely unwell patients. Curr Opin Crit Care. (2011) ;17: (3):303–7. |
[25] | Gonzalez R , Lopez J , Urbano J , Solana MJ , Fernandez SN , Santiago MJ , et al. Evaluation of sublingual microcirculation in a paediatric intensive care unit: prospective observational study about its feasibility and utility. BMC Pediatr. (2017) ;17: (1):75. |
[26] | Ellis CG , Bateman RM , Sharpe MD , Sibbald WJ , Gill R . Effect of a maldistribution of microvascular blood flow on capillary O(2) extraction in sepsis. Am J Physiol Heart Circ Physiol. (2002) ;282: (1):H156–64. |
[27] | Greenwood JC , Jang DH , Hallisey SD , Gutsche JT , Horak J , Acker MA , et al. Severe Impairment of Microcirculatory Perfused Vessel Density Is Associated With Postoperative Lactate and Acute Organ Injury After Cardiac Surgery. J Cardiothorac Vasc Anesth. (2021) ;35: (1):106–15. |
[28] | Domizi R , Damiani E , Scorcella C , Carsetti A , Castagnani R , Vannicola S , et al. Association between sublingual microcirculation, tissue perfusion and organ failure in major trauma: A subgroup analysis of a prospective observational study. PLoS One. (2019) ;14: (3):e0213085. |
[29] | Jhanji S , Lee C , Watson D , Hinds C , Pearse RM . Microvascular flow and tissue oxygenation after major abdominal surgery: association with post-operative complications. Intensive Care Med. (2009) ;35: (4):671–7. |
[30] | Sakr Y , Dubois MJ , De Backer D , Creteur J , Vincent JL . Persistent microcirculatory alterations are associated with organ failure and death in patients with septic shock. Crit Care Med. (2004) ;32: (9):1825–31. |
[31] | van Genderen ME , Lima A , Akkerhuis M , Bakker J , van Bommel J . Persistent peripheral and microcirculatory perfusion alterations after out-of-hospital cardiac arrest are associated with poor survival. Crit Care Med. (2012) ;40: (8):2287–94. |
[32] | Bansch P , Flisberg P , Bentzer P . Changes in the sublingual microcirculation during major abdominal surgery and post-operative morbidity. Acta Anaesthesiol Scand. (2014) ;58: (1):89–97. |
[33] | Arnold RC , Dellinger RP , Parrillo JE , Chansky ME , Lotano VE , McCoy JV , et al. Discordance between microcirculatory alterations and arterial pressure in patients with hemodynamic instability. J Crit Care. (2012) ;27: (5):531 e1–7. |
[34] | Hiebl B , Mrowietz C , Ploetze K , Matschke K , Jung F . Critical hematocrit and oxygen partial pressure in the beating heart of pigs. Microvasc Res. (2010) ;80: (3):389–93. |
[35] | Jung F , Mrowietz C , Hiebl B , Franke RP , Pindur G , Sternitzky R . Influence of rheological parameters on the velocity of erythrocytes passing nailfold capillaries in humans. Clin Hemorheol Microcirc. (2011) ;48: (1):129–39. |
[36] | Finnerty CC , Mabvuure NT , Ali A , Kozar RA , Herndon DN . The surgically induced stress response. JPEN J Parenter Enteral Nutr. (2013) ;37: (5 Suppl):21S–9S. |
[37] | Dubin A , Pozo MO , Casabella CA , Palizas F Jr , MuriasG, MoseincoMC, et al. Increasing arterial blood pressure with norepinephrine does not improve microcirculatory blood flow: a prospective study. Crit Care. (2009) ;13: (3):R92. |
[38] | Koning NJ , Vonk AB , Vink H , Boer C . Side-by-Side Alterations in Glycocalyx Thickness and Perfused Microvascular Density During Acute Microcirculatory Alterations in Cardiac Surgery. Microcirculation. (2016) ;23: (1):69–74. |
[39] | Chappell D , Bruegger D , Potzel J , Jacob M , Brettner F , Vogeser M , et al. Hypervolemia increases release of atrial natriuretic peptide and shedding of the endothelial glycocalyx. Crit Care. (2014) ;18: (5):538. |
[40] | Hippensteel JA , Uchimido R , Tyler PD , Burke RC , Han X , Zhang F , et al. Intravenous fluid resuscitation is associated with septic endothelial glycocalyx degradation. Crit Care. (2019) ;23: (1):259. |
[41] | Torres Filho IP , Torres LN , Salgado C , Dubick MA . Plasma syndecan-1 and heparan sulfate correlate with microvascular glycocalyx degradation in hemorrhaged rats after different resuscitation fluids. Am J Physiol Heart Circ Physiol. (2016) ;310: (11):H1468–78. |
[42] | Sumpelmann R , Becke K , Brenner S , Breschan C , Eich C , Hohne C , et al. Perioperative intravenous fluid therapy in children: guidelines from the Association of the Scientific Medical Societies in Germany. Paediatr Anaesth. (2017) ;27: (1):10–8. |
[43] | Becker BF , Chappell D , Bruegger D , Annecke T , Jacob M . Therapeutic strategies targeting the endothelial glycocalyx: acute deficits, but great potential. Cardiovasc Res. (2010) ;87: (2):300–10. |
[44] | Saoraya J , Wongsamita L , Srisawat N , Musikatavorn K . Plasma syndecan-1 is associated with fluid requirements and clinical outcomes in emergency department patients with sepsis. Am J Emerg Med. (2021) ;42: :83–9. |
[45] | Uz Z , Ince C , Shen L , Ergin B , van Gulik TM . Real-time observation of microcirculatory leukocytes in patients undergoing major liver resection. Sci Rep. (2021) ;11: (1):4563. |
[46] | Kuik SJ , Kalteren WS , Mebius MJ , Bos AF , Hulscher JBF , Kooi EMW . Predicting intestinal recovery after necrotizing enterocolitis in preterm infants. Pediatr Res. (2020) ;87: (5):903–9. |