Transcranial direct current stimulation in physical therapy treatment for adults after stroke: A systematic review
Abstract
BACKGROUND:
Stroke is a clinical syndrome that can cause neurological disorders due to a reduction or interruption in the blood flow at the brain level. Transcranial direct current stimulation (TDCS) is a non-invasive electrotherapy technique with the ability to modulate the function of nervous tissue.
OBJECTIVE:
The aim of this review is to analyze the effects derived from the application of the TDCS for post-stroke patients on functionality and mobility.
METHODS:
The data search was conducted in PubMed, PEDro, Cochrane Library, Web of Science and Scopus between July and August 2023. The search focused on randomized clinical trials conducted in the period of 2019–2023, and according to the selection criteria, seven studies were obtained.
RESULTS:
The results found are mainly focused on the analysis of the scales Fugl-Meyer Assessment for Upper Extremity and Wolf Motor Function Test.
CONCLUSION:
The application of TDCS presents benefits in post-stroke individuals on functionality, mobility and other secondary studied variables.
1Introduction
Stroke or cerebral vascular accident (CVA) refers to a clinical syndrome in which a decrease in the supply of nutrients and oxygen occurs due to a reduction or interruption in the blood flow at the brain level (Guzik & Bushnell, 2017; Ku, Chen, Yang, Lai, & Wang, 2020; Savia, 2020). The appearance of a neurological deficit can be caused owing to the situation previously quoted. The prevalence of suffering a stroke increases with age and it is prevalence is higher in men than in women (Savia, 2020). A clinical sign of note in most patients is the presence of sensorimotor alterations in the opposed body side to the brain injury (Baer & Durward, 2004). Two types of strokes are distinguished in relation to the nature of the brain lesion: ischemic and hemorrhagic. The first one is more frequent and less lethal compared to hemorrhagic stroke (Baer & Durward, 2004; Sepúlveda-Contreras, 2020).
Physiotherapy can focus on several aspects including, for example, increasing strength and range of motion, coordination and balance, reducing spasticity, improving gait ability and independence and quality of life (Iqbal, Arsh, Hammad, Haq, & Darain, 2020; Kim & Jang, 2021; Marquez-Chin & Popovic, 2020; Pérez-De La Cruz, 2021). Thus, the main goal of physiotherapy in the stoke treatment is to restore the highest possible functionality, both motor and sensory skills (Pearce, O’Donnell, Pimentel, Blake, & Mackenzie, 2023).
Transcranial direct current stimulation (TDCS) is a non-invasive electrotherapy technique intended for the treatment of conditions in the central nervous system, especially in chronic diseases (Charvet, Shaw, Bikson, Woods, & Knotkova, 2020; Lefaucheur et al., 2017; Pinto, Teixeira Costa, Duarte, & Fregni, 2018; Woods et al., 2016). This intervention represents a novel alternative with the ability to normalize or modulate the function of nervous tissue (Allman et al., 2016; Ayache & Chalah, 2020; Viganò, Toscano, Puledda, & Di Piero, 2019). TDCS therapy consists on the application of an electric current low amplitude monophasic directly on the scalp, which allows modulating the excitability of certain cortical areas depending on the placement and polarity of the electrodes (Caulfield et al., 2020; Charvet et al., 2020; Woods et al., 2016). Therefore, this technique could improve the post-stroke rehabilitation process, taking into account that the recovery of motor skills could be considered dependent on neuroplasticity (Duan, Liu, Yang, Huang, & Shen, 2023). The aim of this review is to analyze the effects derived from the application of the TDCS for post-stroke patients on the functionality and mobility, and the second purpose is to compare the characteristics of the TDCS treatment options. This research seeks to continue and update the results of other previous reviews focused on this therapy (Bornheim et al., 2022; Dong et al., 2021; Orrù, Conversano, Hitchcott, & Gemignani, 2020).
2Materials and methods
2.1Review protocol and search strategy
The search was conducted following the Preferred Reporting for Systematic Reviews and Meta-Analyses (PRISMA) guidelines and was registered with the International Prospective Register of Systematic Reviews (PROSPERO) with ID code: CRD42023458319. The approach to the issue was made based on the PICO question format: in adults post stroke (P = population), is TDCS treatment, alone or combined with other physical therapy (I = intervention) compared to no intervention, standard physical therapy or with no treatment to compare (C = comparison); effective to improve functionality or mobility (O = outcomes)?
The search was carried out between July and August 2023 using Pubmed, PEDro, Cochrane Library, Web of Science and Scopus. The descriptors of structured language to define the search strategy were established through the DeCS/MeSH platform, the following terms were selected: “physical therapy”; “rehabilitation”; “stroke”; and “transcranial direct current stimulation”. The final search formula was established using Boolean operators (AND/OR): “transcranial direct current stimulation AND stroke AND (physical therapy OR rehabilitation)” in those databases in which it was possible (Pubmed, PEDro, Cochrane Library, Web of Science and Scopus), or through two searches: “transcranial direct current stimulation AND stroke AND physical therapy” and “transcranial direct current stimulation AND stroke AND rehabilitation” in PEDro.
2.2Eligibility criteria, study selection and data collection process
The search was limited to randomized clinical trials performed in adults who suffered a stroke episode and conducted in the period of 2019–2023, besides only those articles written in English were selected. After eliminating duplicate references and excluding systematic reviews, pilot studies, case reports and study projects, and articles older than 5 years or written in a language other than English, we proceeded to screen by reading the title and abstract.
Those articles whose intervention focused on the application of TDCS in the treatment of stroke sequelae on mobility and functionality were selected. All those articles focused on other pathologies, other techniques or non-physiotherapeutic interventions, treatments not carried out in adults or developed with non-human experimental models, or interventions focused on the analysis of the effects at the cognitive level, vision, aphasia, or dysphagia were excluded.
The search and screened the articles was realized for two independent reviewers, these reviewers applied inclusion/exclusion criteria, and later, another reviewer supervised the systematic review, quality assessment, and data extraction. The heterogeneity of the results invited us to opt for a qualitative analysis of the studies found.
From each study, information was extracted regarding the number of individuals in the total sample and in each group, the study variables and the follow-up time, the intervention parameters, and the results obtained.
2.3Quality assessment of studies
The selection of studies was conditioned on their methodological quality, based on the PEDro score, because this database is the updated reference for the quality assessment of interventions evidence in physical therapy. The PEDro scale has excellent reliability for use in systematic reviews of randomized clinical trials (Maher, Sherrington, Herbert, Moseley, & Elkins, 2003). The scale includes 11 items, through which scores are obtained with a final range of 0 to 10 to evaluate the methodological quality of randomized clinical trials; the items included in the PEDro scale can be seen in Appendix A. The scores were obtained from the PEDro database and later revised.
Only those clinical trials with a score equal to or greater than 7 on the PEDro scale were selected to guarantee high methodological quality.
3Results
3.1Study selection
The PRISMA flow diagram (Fig. 1) illustrates the different stages of conduction and selection of the systematic review. The selection was made according to the stated eligibility criteria. Finally, 7 studies of the 2651 articles were selected.
Fig. 1
PRISMA flow diagram. Identification of the results obtained from the databases.
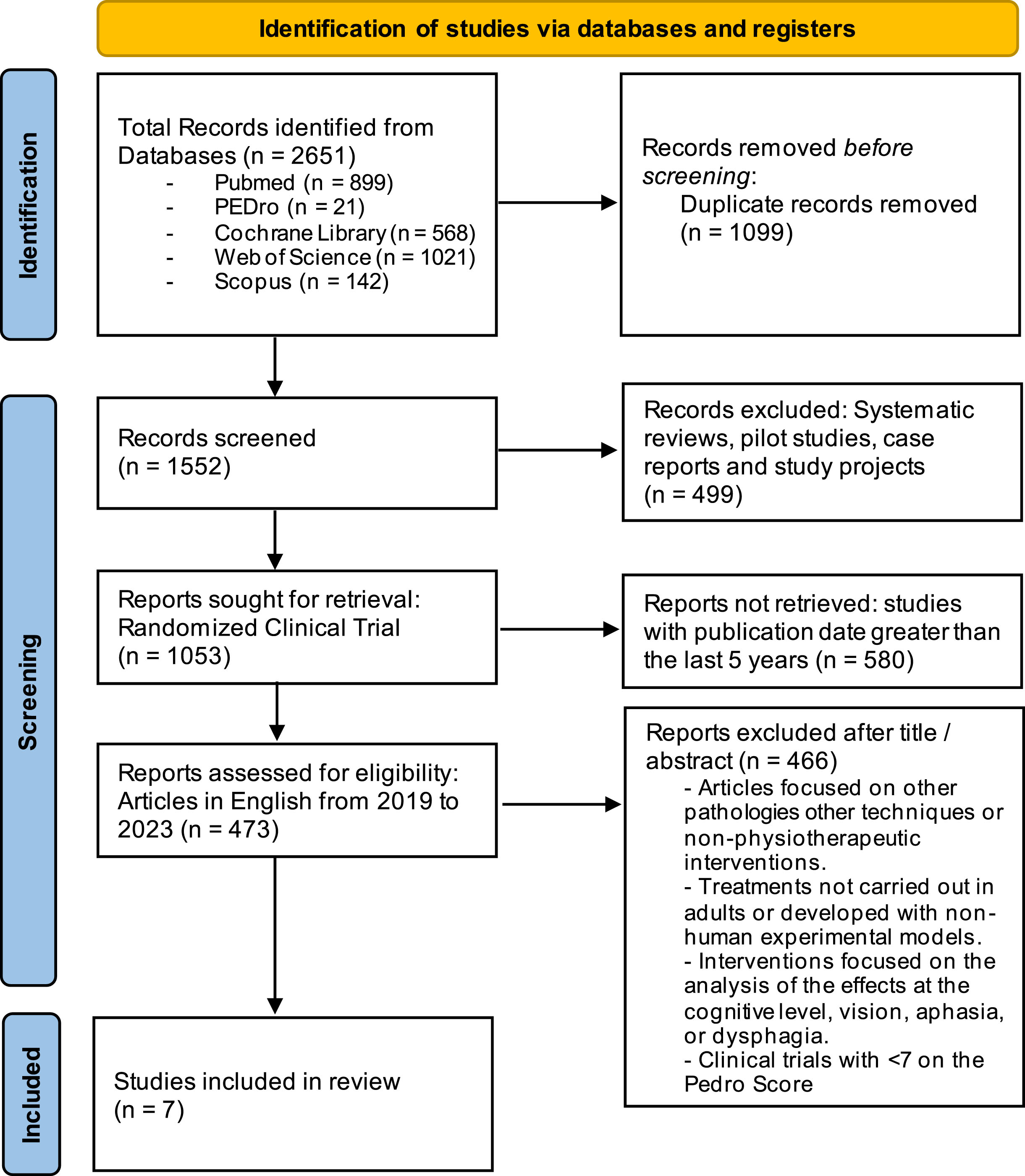
3.2Sample population characteristics and Methodological quality assessment
The total sample size of the set of studies analyzed was composed of 340 subjects who completed each clinical trial. The average age of the total sample was 58,92 years, with 61,17% men; the majority of randomized clinical trials have a predominance of ischemic strokes, the distribution of the hemi paretic affected side was homogeneous and both acute and chronic patients were included. Table 1 represents the data related to the number of individuals and groups of each investigation, the variables analyzed in each study and the follow-up time. In addition, Table 1 contains the score obtained on the PEDro scale to analyze the methodological quality, all selected articles show a level from good to excellent with scores higher than 7 (Andressa de Souza et al., 2021; Ehsani, Mortezanejad, Yosephi, Daniali, & Jaberzadeh, 2022; Kashoo et al., 2022; Klomjai et al., 2022; Llorens et al., 2021; Pires et al., 2023; Tedla et al., 2022) and even reaching a score of 9 (Tedla et al., 2022) or 10 (Andressa de Souza et al., 2021) in some cases. Appendix B includes the localization where studies were conducted.
Table 1
Characteristics of the clinical trials included in the systematic review
Author (year) | Participants | PEDro | Outcomes | Assessment time |
and groups | score | measurements | ||
(Andressa de Souza et al., 2021) | N = 26, (13/13) | 10/10 | Shoulder Pain (VAS) | 2 post-intervention evaluations: |
SPADI | post-treatment and 1 month follow-up | |||
DASH | ||||
FMA –UE | ||||
Shoulder ROM | ||||
Grip strength | ||||
SSQOL | ||||
BDI | ||||
PSQI | ||||
(Ehsani et al., 2022) | N = 32, (12/10/10) | 7/10 | Spasticity (MAS) | 2 post-intervention evaluations: |
BBS | post-treatment and 1 month follow-up | |||
EMG | ||||
(Kashoo et al., 2022) | N = 64, (32/32) | 8/10 | FMA –UE | 1 post-intervention evaluations |
ARAT | ||||
(Klomjai et al., 2022) | N = 78, (20/18/20/20) | 8/10 | FMA –UE/LE | 2 post-intervention evaluations: |
WMFT | post-treatment and 1 month follow-up | |||
FTSTS | ||||
TUG | ||||
MFV | ||||
(Llorens et al., 2021) | N = 29, (14/15) | 7/10 | FMA –UE | 1 post-intervention evaluation |
WMFT | ||||
NSA | ||||
(Pires et al., 2023) | N = 57, (20/20/17) | 8/10 | FMA –UE | 2 post-intervention evaluations: |
GPOCS | after 5 and 10 sessions | |||
(Tedla et al., 2022) | N = 54, (18/18/18) | 9/10 | TIS | 1 post-intervention evaluation |
FMA –UE | ||||
WMFT | ||||
TMWT | ||||
SSQOL |
Abbreviations. ARAT: Action Research Arm Test; BBS: Berg Balance Scale; BDI: Beck Depression Inventory; DASH: Disabilities of the Arm, Shoulder and Hand questionnaire; EMG: electromyography; FMA: Fugl-Meyer Assessment of Motor Recovery after Stroke Scale; FTSTS: Five-Times Sit-To-Stand; GPOCS: Global Perception of Change Scale; LE: lower extremity; MAS: Modified Ashworth Scale; MFV: Mean Blood Flow Velocity; NSA: Nottingham Sensory Assessment; ROM: Range of Motion; PSQI: Pittsburgh Sleep Quality Index; SPADI: Shoulder Pain and Disability Index; SSQOL: Stroke Specific Quality of Life Scale; TIS: Trunk Impairment Scale; TMWT: Ten Meter Walk Test; TUG: Timed Up and Go; UE: upper extremity; VAS: Visual Analogic Scale; WMFT: Wolf Motor Function Test.
3.3Outcomes measurements and assessment time
Functionality was the most analyzed variable in most of the studies, especially through the Fugl-Meyer Assessment of Motor Recovery after Stroke Scale for Upper Extremity (FMA –UE), considering that almost all clinical trials analyzed the effects on deficits in the upper limb. Specifically, this variable was included in 6 of the articles (Andressa de Souza et al., 2021; Kashoo et al., 2022; Klomjai et al., 2022; Llorens et al., 2021; Pires et al., 2023; Tedla et al., 2022), likewise, the variable Wolf Motor Function Test (WMFT) was also normally used (Klomjai et al., 2022; Llorens et al., 2021; Tedla et al., 2022). The effects on gait, balance, and lower limb functionality have also been studied (Ehsani et al., 2022; Klomjai et al., 2022; Tedla et al., 2022), as well as spasticity associated with electromyographic recording (EMG) (Ehsani et al., 2022), and shoulder pain, strength, and range of motion (ROM) (Andressa de Souza et al., 2021). Quality of life values (SSQOL: Stroke Specific Quality of Life Scale) (Andressa de Souza et al., 2021; Tedla et al., 2022), Beck Depression Inventory (BDI), Pittsburgh Sleep Quality Index (PSQI) (Andressa de Souza et al., 2021), Global Perception of Change Scale (GPCS) (Pires et al., 2023) and Haemodynamic response (MFV: Mean Blood Flow Velocity) (Klomjai et al., 2022) were also included as outcome measures.
The follow-up time ranged between 1 and 2 post-intervention assessments, usually at the end of the intervention period (Kashoo et al., 2022; Llorens et al., 2021; Pires et al., 2023; Tedla et al., 2022) with a maximum follow-up of one month (Andressa de Souza et al., 2021; Ehsani et al., 2022; Klomjai et al., 2022). All information on the variables included in each study and follow-up times is available in Table 1.
3.4Interventions protocols and effects of treatments
Table 2 shows the characteristics of the treatments of each randomized clinical trial and its most notable results. The TDCS treatment protocols included in the selected articles show interventions of between 5 (Klomjai et al., 2022) and 24/25 sessions (Llorens et al., 2021; Tedla et al., 2022), most of them consisting of 10 sessions in 2 weeks (Andressa de Souza et al., 2021; Ehsani et al., 2022; Kashoo et al., 2022; Pires et al., 2023).
Table 2
Interventions, procedures, and results of the clinical trials based on the application of TDCS in the physical treatment of stroke
Author (year) | TDCS intervention protocols and alternative treatments | Results |
(Andressa de Souza et al., 2021) | 10 sessions in 2 weeks: 20’ passive physiotherapy+20’ active upper extremity exercise | ↓ VAS |
with simultaneous TDCS or sham TDCS (Anodal C3/C4 affected hemisphere | ↓ SPADI | |
application 2mA) | ↓ DASH | |
=FMA –UE | ||
↑ ROM | ||
=Grip strength | ||
↑ SSQOL | ||
(Ehsani et al., 2022) | 10 sessions in 2 weeks: 20’ stretching and balance training (all groups)+20’ TDCS | ↓ MAS |
Anodal M1 affected hemisphere application 1mA or sham TDCS (only TDCS or sham | ↑ BBS | |
TDCS groups) | ↓ EMG LG | |
↑ EMG TA | ||
(Kashoo et al., 2022) | 10 sessions in 2 weeks: 30’ TDCS Anodal C3/C4 affected hemisphere application 1,5mA | ↑ FMA –UE |
or sham TDCS+30’ upper extremity exercise combined with motor imagery | ↑ ARAT | |
(simultaneous to TDCS or sham TDCS) | ||
(Klomjai et al., 2022) | 5 sessions in consecutive days: 20’ TDCS application 1,5mA (4 groups: Anodal C3/C4 | ↑ FMA –UE/LE |
affected hemisphere or Cathodal C3/C4 non-affected hemisphere or Dual-anodal | ↑ WMFT | |
C3/C4 affected hemisphere and cathodal C3/C4 non-affected hemisphere- or sham | ↑ FTSTS | |
current)+60’ conventional physiotherapy (all groups) | ↑ TUG | |
=MFV | ||
(Llorens et al., 2021) | 25 sessions (3 to 5 times a week): 30’ TDCS Anodal C3/C4 affected hemisphere | ↑ FMA –UE |
application 2mA (TDCS group) with VR+30’ conventional physical therapy on the | ↑ WMFT | |
affected joints of the hemiparetic side (both groups) | =NSA | |
(Pires et al., 2023) | 10 sessions in 2 weeks: 20’ TDCS application 2mA (3 groups: Anodal C3/C4 affected | ↑ FMA –UE |
hemisphere or Cathodal C3/C4 non-affected hemisphere or sham current with Anodal | ||
electrodes colocation)+45’ upper extremity conventional physiotherapy | ||
(Tedla et al., 2022) | 24 sessions in 6 weeks: 90’ for each session; 3 groups (Conventional physical therapy | ↑ TIS |
group includes only upper extremity, trunk and lower extremity exercise; Sham TDCS | ↑ FMA –UE | |
group includes 20’ sham TDCS+30’ trunk targeted PNF+40’ conventional physical | ↑ WMFT | |
therapy; and TDCS group includes 20’ TDCS Anodal -anodal C3/C4 affected | ↑ TMWT | |
hemisphere and cathodal C3/C4 non-affected hemisphere-application 2mA+30’ trunk | ↑ SSQOL | |
targeted PNF+40’ conventional physical therapy) |
Abbreviations. ARAT: Action Research Arm Test; BBS: Berg Balance Scale; BDI: Beck Depression Inventory; C3/C4: positions of International 10/20 positioning Electroencephalogram System; DASH: Disabilities of the Arm, Shoulder and Hand questionnaire; EMG: electromyography; FMA: Fugl-Meyer Assessment of Motor Recovery after Stroke Scale; FTSTS: Five-Times Sit-To-Stand; GPOCS: Global Perception of Change Scale; LE: lower extremity; LG: lateral gastrocnemius muscle; M1: primary motor cortex; MAS: Modified Ashworth Scale; NSA: Nottingham Sensory Assessment; ROM: Range of Motion; PSQI: Pittsburgh Sleep Quality Index; PNF: proprioceptive neuromuscular facilitation; SPADI: Shoulder Pain and Disability Index; SSQOL: Stroke Specific Quality of Life Scale; TA: tibialis anterior muscle; TIS: Trunk Impairment Scale; TDCS: transcranial direct current stimulation; TMWT: Ten Meter Walk Test; TUG: Timed Up and Go; UE: upper extremity; VAS: Visual Analogic Scale; VR: virtual reality; WMFT: Wolf Motor Function Test.
In all investigations, the placement of the electrodes was oriented towards the stimulation of the primary motor cortex M1, specifically placing the electrodes at points C3/C4 (International 10/20 positioning Electroencephalogram System) on affected hemisphere (Andressa de Souza et al., 2021; Ehsani et al., 2022; Kashoo et al., 2022; Klomjai et al., 2022; Llorens et al., 2021; Pires et al., 2023; Tedla et al., 2022). Usually, the application of the technique was carried out in the modality of Anodal TDCS combined with a conventional physiotherapy program with mobility and balance exercises (Andressa de Souza et al., 2021; Ehsani et al., 2022; Kashoo et al., 2022; Klomjai et al., 2022; Llorens et al., 2021; Pires et al., 2023; Tedla et al., 2022), motor imagery exercises (Kashoo et al., 2022) or virtual reality (Llorens et al., 2021) compared to the application of Cathodal TDCS (Klomjai et al., 2022; Pires et al., 2023), Dual TDCS (Klomjai et al., 2022) sham TDCS treatment (Andressa de Souza et al., 2021; Ehsani et al., 2022; Kashoo et al., 2022; Klomjai et al., 2022; Pires et al., 2023; Tedla et al., 2022) or conventional physical therapy protocol (Ehsani et al., 2022; Llorens et al., 2021; Tedla et al., 2022).
The application time of the TDCS treatment was from 20 minutes (Andressa de Souza et al., 2021; Ehsani et al., 2022; Klomjai et al., 2022; Pires et al., 2023; Tedla et al., 2022) to 30 minutes (Kashoo et al., 2022; Llorens et al., 2021), with an intensity of 1mA (Ehsani et al., 2022), 1,5mA (Kashoo et al., 2022; Klomjai et al., 2022) or 2mA (Andressa de Souza et al., 2021; Llorens et al., 2021; Pires et al., 2023; Tedla et al., 2022). For the sham groups TDCS treatment was applied for 30 second and then the electrodes remained in the same position during the supposed current passage time (Andressa de Souza et al., 2021; Ehsani et al., 2022; Kashoo et al., 2022; Klomjai et al., 2022; Pires et al., 2023; Tedla et al., 2022).
The conventional physiotherapy treatments included were mainly directed at the upper extremity (Andressa de Souza et al., 2021; Kashoo et al., 2022; Pires et al., 2023; Tedla et al., 2022), trunk proprioceptive neuromuscular facilitation (PNF) (Tedla et al., 2022) and passive techniques on hemi paretic side (Andressa de Souza et al., 2021; Llorens et al., 2021).
Positive effects of TDCS treatment have been considered, highlighting some aspects of the results of each article. In comparison between TDCS and simultaneous exercise versus sham TDCS and the same exercise protocol (Andressa de Souza et al., 2021), TDCS treatment provides benefits in several variables as reducing pain, increasing range of motion, motor function and quality of life. Grip strength and the FMA –UE scale did not experiment changes after treatment. However, positive effects were also collected from the sham TDCS protocol intervention and exercise group and intergroup analysis was not possible as there were significant pre-intervention differences.
Specific changes on the FMA –UE were the most studied, statistically significant results have been observed for the Anodal TDCS application (Kashoo et al., 2022; Klomjai et al., 2022; Llorens et al., 2021; Pires et al., 2023; Tedla et al., 2022), in the same way the effects on the WMFT were positive in three of the investigations (Klomjai et al., 2022; Llorens et al., 2021; Tedla et al., 2022). In the modalities of intervention based on the cathodal application, favorable results were obtained, superior to the sham TDCS but below the anodal intervention (Pires et al., 2023). Furthermore, with respect to the functionality of the lower limbs FMA –LE, the dual TDCS modality may be more convenient (Klomjai et al., 2022).
On the other hand, it is necessary to highlight the significant changes in SSQOL (Andressa de Souza et al., 2021; Tedla et al., 2022) and BBS (Ehsani et al., 2022) derived from the TDCS application. No post-treatment changes were found for the hemodynamic variables (MFV) (Klomjai et al., 2022) and the results on the Nottingham Sensory Assessment (NSA) were not remarkable either (Llorens et al., 2021).
4Discussion
The results offered by the randomized clinical trials analyzed suggest that it is possible to find beneficial effects of the application of TDCS in post-stroke treatment. However, the heterogeneity in the clinical characteristics of post-stroke patients may condition the effects to be achieved.
The sample consisted of adult individuals who had suffered a stroke, who were subjected to different treatment alternatives based on the stimulation of the primary motor cortex (M1) with the intention of achieving changes in the motor skills of these patients through a phenomenon of neuromodulation (Carvalho et al., 2018; Cruccu et al., 2016). In general, it is possible to draw conclusions similar to the results obtained in other previous studies along the same lines (Allman et al., 2016).
The studies included ischemic and hemorrhagic post-stroke patients and in different phases of recovery from the moment of the stroke, so that the results could be conditioned since it is estimated that an early intervention can be more favorable (Garrido M et al., 2023; Zhao, Liu, Sun, & Li, 2022), and that TDCS can promote faster acquisition of motor skills (Kunaratnam et al., 2022). In addition, it may be interesting to individualize the treatment dose to the characteristics of each patient (Beaulieu, Blanchette, Mercier, Bernard-Larocque, & Milot, 2019), and perform the intervention at home (Prathum et al., 2022), this is one of the advantages of the technique.
With respect to the application parameters used, most of the articles coincide in making use of the Anodal TDCS, placing the anode on points C3/C4 to stimulate M1, with an application time from 20 to 30 minutes and a maximum intensity of 2mA. The intensity applied in all studies coincides with safety recommendations and with the guidelines applied in other recent research (Youssef, Mohamed, & Hamdy, 2023; Zhu et al., 2017). In this sense, the investigations of Klomjai et al. (Klomjai et al., 2022) and Tedla et al. (Tedla et al., 2022) are notable because this studies included treatment with Cathodal TDCS or Dual application with anodal electrode on C3/C4 affected hemisphere and cathodal electrode on C3/C4 non-affected hemisphere. Overall, anodal stimulation was more effective than cathodal TDCS. Despite this, it is convenient to analyze the effects derived from the cathodal modulation treatment (Ahdab et al., 2019; Duan et al., 2023; Geiger, Roche, Vlachos, Cattagni, & Zory, 2019; Salazar et al., 2020).
The shortest intervention was the one developed by Klomjai et al., 2022, and the longest were those by Llorens et al., 2021 and Tedla et al., 2022. The duration and follow-up of the study conditions its prospective, the results can be very different in the short and long term (Cattagni et al., 2019; Geiger et al., 2019).
Within the results, it is noteworthy that all the interventions are accompanied by a conventional exercise-based intervention, which in many cases offers positive results, even though it consists in sham TDCS. This suggests the importance of including exercise for therapeutic purposes in post-stroke physiotherapy treatment, as advocated in other studies (Bornheim, Croisier, Maquet, & Kaux, 2020; Graef et al., 2016; Massaferri et al., 2023). Therefore, it would be convenient to analyze the independent effects of TDCS and exercise individually. That is, future research should study the contribution of exercise to transcranial electrotherapy or the increase in effect size derived from the addition of TDCS to the effects of therapeutic exercise.
In view of the variables studied and the results obtained, it is possible to point out that most of the investigations focus on changes in motor skills and coordination of the upper limb. Most of the articles used in this review agree about the use of FMA to study possible changes after TDCS treatment. In one of the investigations (Andressa de Souza et al., 2021) does not obtain differences pre and post treatment. On the other hand, other articles (Kashoo et al., 2022; Klomjai et al., 2022; Llorens et al., 2021; Pires et al., 2023; Tedla et al., 2022) refer getting significant improvements in FMA after TDCS treatment. In relation with these latest investigations, two of them (Kashoo et al., 2022; Llorens et al., 2021) only used anodal TDCS, while the rest (Klomjai et al., 2022; Pires et al., 2023; Tedla et al., 2022) also used cathodic and dual TDCS to compare these several types of currents and know which of them offers better results in the treatment of upper extremity after suffering a stroke. All of them agreed on that the best current is anodic TDCS, although it is necessary to highlight that dual TDCS achieves good results, primarily in the lower limb recover (Klomjai et al., 2022).
Among the variables analyzed, it also makes sense to talk about shoulder pain, which is very common in post-stroke patients (Ito et al., 2023; Lin, Shen, Chang, Wu, & Özçakar, 2023). The VAS scale was used in one of the articles (Andressa de Souza et al., 2021), the results obtained on the levels of shoulder pain after the application of the TDCS are one of the main findings of this study and confirm the performance of this therapy on chronic pain (Brighina et al., 2019; DaSilva et al., 2012; Stilling, Monchi, Amoozegar, & Debert, 2019; Zortea et al., 2019). This circumstance is important since it represents an alternative to pharmacological treatment (Moisset & Lefaucheur, 2019), taking into account that post-stroke patients usually suffer sensory hypersensitivity and follow significant drug treatment (de Sain et al., 2023).
Furthermore, Andressa de Souza et al. (Andressa de Souza et al., 2021) included assessment with DASH and SPADI scales, these variables relate shoulder pain to specific capacity for specific activities or movements.
On the contrary, lower limb’s results after the application of TDCS have barely been studied, only some articles have obtained results on this matter. One of the articles, Ehsani et al. (Ehsani et al., 2022) conduct the Modified Ashworth Scale (MAS) of plantar flexors and electromyography (EMG) in Lateral Gastrocnemius (LG) and Tibialis Anterior (TA) muscles and passed the Berg Balance Scale (BBS), obtaining a decrease in the EMG activity of LG muscle and a rising in the EMG activity of TA muscle, An improvement in balance and a reduction in spasticity in the plantar flexors. Besides, another article (Klomjai et al., 2022) uses the FMA-Lower Extremity and obtains significant results in the improvement of motor function after treatment with TDCS, specifically with dual current, followed by one hour of physiotherapy.
These studies do not only support other researches on gait and the lower extremities (Klomjai et al., 2018; Ojardias et al., 2020), also confirm that the integration of TDCS with physiotherapy prolongs all the observed effects. In addition, the combination of TDCS with virtual reality, Llorens et al.(Llorens et al., 2021) is novel.
Finally, the differences between the monitoring variables assessed in each investigation determine the results of this review. Despite the high methodological quality of the studies analyzed, there are some limitations derived from the characteristics of the sample and the follow-up times. The difficulty of blinding patients and evaluators is another of the limitations that this type of research could present, however, the characteristics of this low-intensity electrotherapy technique allow a short interval of current (30 seconds) to be applied at the start of the sham treatment time.
With a view to future lines of research, it may be convenient to establish divisions between the age groups of the sample’s participants and especially related on the nature of the stroke, due to the origin of the stroke, ischemic or hemorrhagic, could condition the results of the treatment. As well as, it would be advisable to study in depth if the passage of time since the appearance of the stroke and the acute or chronic phase where the patient is, modify the response of the TDCS treatment.
In the same course of action, it is room for assess the regularity between treatment sessions and, above all the necessity of carry through the check in more long-term, just as the adverse effects and the neurophysiological processes that happens in the nervous system during the TDCS treatment application.
5Conclusions
TDCS treatment offers positive results in improving functionality in post-stroke patients. Most of the studies focus on the upper extremity evaluation through Fugl-Meyer Assessment of Motor Recovery after Stroke Scale, furthermore, it is possible to report benefits on Wolf Motor Function Test and other related variables such as range of motion, spasticity and pain.
The most widespread treatment modality is the Anodal TDCS application, these interventions are frequently accompanied by therapeutic exercise programs and conventional physiotherapy. Therefore, the stimulation program should be considered a complement to therapies that encourage the patient’s active role.
There is consensus on establishing TDCS interventions with intensities from 1 to 2mA, treatment time of at least 20 minutes and placing the electrodes at points C3/C4.
Future research should consider the possibility of analyzing the effects in a long-term follow-up, as well as the need to observe the changes depending on the ischemic or hemorrhagic origin of the lesion. In addition, it is necessary to study the neurophysiological changes that occur in the nervous system during the TDCS treatment.
Author contributions
Conceptualization, J.G-R. and C.A-M.; methodology, J.G-R. and M.R.-H.; software, P.G.-R.; validation, R.M-V., M.J.V.-G. and M.R.-H.; formal analysis, P.G.-R; investigation, J.G.-R. and C.A.-M.; resources, M.J.V.-G.; data curation, R.M.-V.; writing— original draft preparation, P.G.-R. and C.A.-M.; writing— review and editing, J.G-R. and M.R.-H.; visualization, M.J.V.-G. and R.M.-V.; supervision, M.R.-H. and J.G.-R.; project administration, M.R-H. All authors read and agreed to the published version of the manuscript.
Funding
This research received no external funding.
Ethical approval
Not applicable.
Informed consent
Not applicable.
Data availability statement
Not applicable.
Conflicts of interest
The authors declare no conflict of interest.
References
1 | Ahdab, R. , Mansour, A. G. , Khazen, G. , El-Khoury, C. , Sabbouh, T.M. , Salem, M. , Riachi, N. ((2019) ). Cathodal Transcranial Direct Current Stimulation of the Occipital cortex in Episodic Migraine: A Randomized Sham-Controlled Crossover Study. Journal of Clinical Medicine, 9: (1), 60. https://doi.org/10.3390/jcm9010060 |
2 | Allman, C. , Amadi, U. , Winkler, A. M. , Wilkins, L. , Filippini, N. , Kischka, U. , Johansen-Berg, H. ((2016) ). Ipsilesional anodal tDCS enhances the functional benefits of rehabilitation in patients after stroke. Science Translational Medicine, 8: (330). https://doi.org/10.1126/scitranslmed.aad5651 |
3 | Andressa de Souza, J. , Ferrari Corrêa, J. C. , Marduy, A. , Dall’Agnol, L. , Gomes de Sousa, M. H. , Nunes da Silva, V. , Corrêa, F. I. ((2021) ). To Combine or Not to Combine Physical Therapy With tDCS for Stroke With Shoulder Pain? Analysis From a Combination Randomized ClinicalTrial for Rehabilitation of Painful Shoulder in Stroke. Frontiers in Pain Research, 2: , 696547. https://doi.org/10.3389/FPAIN.2021.696547 |
4 | Ayache, S. S. , Chalah, M. A. ((2020) ). Transcranial Direct Current Stimulation and Migraine-The Beginning of a Long Journey. Journal of Clinical Medicine, 9: (4), 1194. https://doi.org/10.3390/jcm9041194 |
5 | Baer, G. , Durward, B. (2004). Stroke. In M. Stokes (Ed.), Physical Management in Neurological Rehabilitation (2nd ed., pp. 75-101). Elsevier. |
6 | Beaulieu, L. D. , Blanchette, A. K. , Mercier, C. , Bernard-Larocque, V. , Milot, M. H. ((2019) ). Efficacy, safety, and tolerability of bilateral transcranial direct current stimulation combined to a resistance training program in chronic stroke survivors: A double-blind, randomized, placebo-controlled pilot study. Restorative Neurology and Neuroscience, 37: (4), 333–346. https://doi.org/10.3233/RNN-190908 |
7 | Bornheim, S. , Croisier, J. L. , Maquet, P. , Kaux, J. F. ((2020) ). Transcranial direct current stimulation associated with physical-therapy in acute stroke patients -Arandomized, triple blind, sham-controlled study. Brain Stimulation, 13: (2), 329–336. https://doi.org/10.1016/J.BRS.2019.10.019 |
8 | Bornheim, S. , Thibaut, A. , Beaudart, C. , Maquet, P. , Croisier, J. L. , Kaux, J. F. ((2022) ). Evaluating the effects of tDCS in stroke patients using functional outcomes: a systematic review. Disability and Rehabilitation, 44: (1), 13–23. https://doi.org/10.1080/09638288.2020.1759703 |
9 | Brighina, F. , Curatolo, M. , Cosentino, G. , De Tommaso, M. , Battaglia, G. , Sarzi-Puttini, P. C. , Fierro, B. ((2019) ). Brain Modulation by Electric Currents in Fibromyalgia:AStructured Review on Non-invasive Approach With Transcranial Electrical Stimulation. Frontiers in Human Neuroscience, 13: (40). https://doi.org/10.3389/fnhum.2019.00040 |
10 | Carvalho, F. , Brietzke, A. P. , Gasparin, A. , Dos Santos, F. P. , Vercelino, R. , Ballester, R. F. , Caumo, W. ((2018) ). Home-based transcranial direct current stimulation device development: An updated protocol used at home in healthy subjects and fibromyalgia patients. Journal of Visualized Experiments, 2018: (137), 1–9. https://doi.org/10.3791/57614 |
11 | Cattagni, T. , Geiger, M. , Supiot, A. , de Mazancourt, P. , Pradon, D. , Zory, R. , Roche, N. ((2019) ). A single session of anodal transcranial direct current stimulation applied over the affected primary motor cortex does not alter gait parameters in chronic stroke survivors. Neurophysiologie Clinique=Clinical Neurophysiology, 49: (4), 283–293. https://doi.org/10.1016/J.NEUCLI.2019.07.012 |
12 | Caulfield, K. A. , Badran, B. W. , DeVries, W. H. , Summers, P. M. , Kofmehl, E. , Li, X. , George, M. S. ((2020) ). Transcranial electrical stimulation motor threshold can estimate individualized tDCS dosage from reverse-calculation electric-field modeling. Brain Stimulation, 13: (4), 961–969. https://doi.org/10.1016/j.brs.2020.04.007 |
13 | Charvet, L. E. , Shaw, M. T. , Bikson, M. , Woods, A. J. , Knotkova, H. ((2020) ). Supervised transcranial direct current stimulation (tDCS) at home: A guide for clinical research and practice. Brain Stimulation, 13: (3), 686–693. https://doi.org/10.1016/j.brs.2020.02.011 |
14 | Cruccu, G. , Garcia-Larrea, L. , Hansson, P. , Keindl, M. , Lefaucheur, J.P. , Paulus, W. , Attal, N. ((2016) ). EAN guidelines on central neurostimulation therapy in chronic pain conditions. European Journal of Neurology, 23: (10), 1489–1499. https://doi.org/10.1111/ene.13103 |
15 | DaSilva, A. F. , Mendonca, M. E. , Zaghi, S. , Lopes, M. , Dossantos, M. F. , Spierings, E. L. , Fregni, F. ((2012) ). TDCS-induced analgesia and electrical fields in pain-related neural networks in chronic migraine. Headache, 52: (8), 1283–1295. https://doi.org/10.1111/j.1526-4610.2012.02141.x |
16 | de Sain, A. , Pellikaan, L. W. , van Voskuilen, J. , Migdis, M. , Sommers-Spijkerman, M. P. , Visser-Meily, J. M. , Huenges Wajer, I. M. (2023). Sensory hypersensitivity after acquired brain injury: the patient perspective. Disability and Rehabilitation, 1-8. https://doi.org/10.1080/09638288.2023.2251401 |
17 | Dong, K. , Meng, S. , Guo, Z. , Zhang, R. , Xu, P. , Yuan, E. , Lian, T. ((2021) ). The Effects of Transcranial Direct Current Stimulation on Balance and Gait in Stroke Patients: A Systematic Review and Meta-Analysis. Frontiers in Neurology, 12: , 650925. https://doi.org/10.3389/FNEUR.2021.650925/BIBTEX |
18 | Duan, Q. , Liu, W. , Yang, J. , Huang, B. , Shen, J. (2023). Effect of Cathodal Transcranial Direct Current Stimulation for Lower Limb Subacute Stroke Rehabilitation. Neural Plasticity, 2023. https://doi.org/10.1155/2023/1863686 |
19 | Ehsani, F. , Mortezanejad, M. , Yosephi, M. H. , Daniali, S. , Jaberzadeh, S. ((2022) ). The effects of concurrent M1 anodal tDCS and physical therapy interventions on function of ankle muscles in patients with stroke: a randomized, double-blinded sham-controlled trial study. Neurological Sciences, 43: (3), 1893–1901. https://doi.org/10.1007/S10072-021-05503-9 |
20 | Garrido M, M. , Álvarez E, E. , Acevedo P, F. , Moyano V,Á. , Castillo N, N. , Cavada Ch, G. ((2023) ). Early transcranial direct current stimulation with modified constraint-induced movement therapy for motor and functional upper limb recovery in hospitalized patients with stroke: A randomized, multicentre, double-blind, clinical trial. Brain Stimulation, 16: (1), 40–47. https://doi.org/10.1016/J.BRS.2022.12.008 |
21 | Geiger, M. , Roche, N. , Vlachos, E. , Cattagni, T. , Zory, R. ((2019) ). Acute effects of bi-hemispheric transcranial direct current stimulation on the neuromuscular function of patients with chronic stroke: A randomized controlled study. Clinical Biomechanics (Bristol, Avon), 70: , 1–7. https://doi.org/10.1016/J.CLINBIOMECH.2019.07.022 |
22 | Graef, P. , Michaelsen, S. M. , Dadalt, M. L. R. , Rodrigues, D.A.M.S. , Pereira, F. , Pagnussat, A. de S. ((2016) ). Effects of functional and analytical strength training on upper-extremity activity after stroke: a randomized controlled trial. Brazilian Journal of Physical Therapy, 20: (6), 543–552. https://doi.org/10.1590/BJPT-RBF.2014.0187 |
23 | Guzik, A. , Bushnell, C. (2017). Stroke Epidemiology and Risk Factor Management. Continuum, 23(1, Cerebrovascular Disease), 15-39. https://doi.org/10.1212/CON.0000000000000416 |
24 | Iqbal, M. , Arsh Hammad, S. , Haq, I. , Darain, H. ((2020) ). Comparison of dual task specific training and conventional physical therapy in ambulation of hemiplegic stroke patients:Arandomized controlled trial. JPMA. The Journal of the Pakistan Medical Association, 70: (1). https://doi.org/10.47391/JPMA.10443 |
25 | Ito, D. , Kawakami, M. , Kuwahara, W. , Yamada, Y. , Kondo, K. , Tsuji, T. ((2023) ). Parameter mapping of hemiplegic shoulder electrical stimulation for motor function: A scoping review. NeuroRehabilitation, 53: (1), 19–32. https://doi.org/10.3233/NRE-220301 |
26 | Kashoo, F. Z. , Al-Baradie, R. S. , Alzahrani, M. , Alanazi, A. , Manzar, M. D. , Gugnani, A. , Chahal, A. ((2022) ). Effect of Transcranial Direct Current Stimulation Augmented with Motor Imagery and Upper-Limb Functional Training for Upper-Limb Stroke Rehabilitation: A Prospective Randomized Controlled Trial. International Journal of Environmental Research and Public Health, 19: , 15199. https://doi.org/10.3390/IJERPH192215199 |
27 | Kim, K. H. , Jang, S. H. ((2021) ). Effects of Task-Specific Training after Cognitive Sensorimotor Exercise on Proprioception, Spasticity, and Gait Speed in Stroke Patients: A Randomized Controlled Study. Medicina (Lithuania), 57: (10). https://doi.org/10.3390/MEDICINA57101098 |
28 | Klomjai, W. , Aneksan, B. , Chotik-Anuchit, S. , Jitkaew, P. , Chaichanudomsuk, K. , Piriyaprasarth, P. , Hiengkaew, V. ((2022) ). Effects of Different Montages of Transcranial Direct Current Stimulation on Haemodynamic Responses and Motor Performance in Acute Stroke: A Randomized Controlled Trial. Journal of Rehabilitation Medicine, 54: , jrm00331. https://doi.org/10.2340/JRM.V54.3208 |
29 | Klomjai, W. , Aneksan, B. , Pheungphrarattanatrai, A. , Chantanachai, T. , Choowong, N. , Bunleukhet, S. , Hiengkaew, V. ((2018) ). Effect of single-session dual-tDCS before physical therapy on lower-limb performance in sub-acute stroke patients: A randomized sham-controlled crossover study. Annals of Physical and Rehabilitation Medicine, 61: (5), 286–291. https://doi.org/10.1016/J.REHAB.2018.04.005 |
30 | Ku, P. H. , Chen, S. F. , Yang, Y. R. , Lai, T. C. , Wang, R. Y. ((2020) ). The effects of Ai Chi for balance in individuals with chronic stroke: a randomized controlled trial. Scientific Reports, 10: (1). https://doi.org/10.1038/S41598-020-58098-0 |
31 | Kunaratnam, N. , Saumer, T. M. , Kuan, G. , Holmes, Z. , Swarbrick, D. , Kiss, A. , Chen, J. L. ((2022) ). Transcranial direct current stimulation leads to faster acquisition of motor skills, but effects are not maintained at retention. PloS One, 17: (9). https://doi.org/10.1371/JOURNAL.PONE.0269851 |
32 | Lefaucheur J. P. , Antal A. , Ayache S. S. , Benninger D. H. , Brunelin J. , Cogiamanian F. , Paulus W. (2017, January 1). Evidence-based guidelines on the therapeutic use of transcranial direct current stimulation (tDCS). Clinical Neurophysiology. Elsevier Ireland Ltd. https://doi.org/10.1016/j.clinph.2016.10.087 |
33 | Lin, T.-Y. , Shen, P.-C. , Chang, K.-V. , Wu, W.-T. , Özcakar, L. ((2023) ). Shoulder ultrasound imaging in the poststroke population: a systematic review and meta-analysis. Journal of Rehabilitation Medicine, 55: , jrm13432. https://doi.org/10.2340/JRM.V55.13432 |
34 | Llorens, R. , Fuentes, M. A. , Borrego, A. , Latorre, J. , Alcañiz, M. , Colomer, C. , Noé, E. ((2021) ). Effectiveness of a combined transcranial direct current stimulation and virtual reality-based intervention on upper limb function in chronic individuals post-stroke with persistent severe hemiparesis: a randomized controlled trial. Journal of Neuroengineering and Rehabilitation, 18: , 108. https://doi.org/10.1186/S12984-021-00896-2 |
35 | Maher, C. G. , Sherrington, C. , Herbert, R. D. , Moseley, A. M. , Elkins, M. ((2003) ). Reliability of the PEDro Scale for Rating Quality of Randomized Controlled Trials. Physical Therapy, 83: (8), 713–721. https://doi.org/10.1093/PTJ/83.8.713 |
36 | Marquez-Chin, C. , Popovic, M. R. ((2020) ). Functional electrical stimulation therapy for restoration of motor function after spinal cord injury and stroke: A review. BioMedical Engineering Online, 19: (1), 1–25. https://doi.org/10.1186/s12938-020-00773-4 |
37 | Massaferri, R. , Montenegro, R. , de Freitas Fonseca, G. , Bernardes, W. , Cunha, F. A. , Farinatti, P. ((2023) ). Multimodal physical training combined with tDCS improves physical fitness components in people after stroke: a double-blind randomized controlled trial. Topics in Stroke Rehabilitation, 30: (7). https://doi.org/10.1080/10749357.2023.2165260 |
38 | Moisset, X. , Lefaucheur, J.-P. ((2019) ). Non pharmacological treatment for neuropathic pain: Invasive and non-invasive cortical stimulation. Revue Neurologique, 175: (1-2), 51–58. https://doi.org/10.1016/j.neurol.2018.09.014 |
39 | Ojardias, E. , Azé, O. D. , Luneau, D. , Mednieks, J. , Condemine, A. , Rimaud, D. , Giraux, P. ((2020) ). The Effects of Anodal Transcranial Direct Current Stimulation on the Walking Performance of Chronic Hemiplegic Patients. Neuromodulation: Journal of the International Neuromodulation Society, 23: (3), 373–379. https://doi.org/10.1111/NER.12962 |
40 | Orrù, G. , Conversano, C. , Hitchcott, P. K. , Gemignani, A. ((2020) ). Motor stroke recovery after tDCS:Asystematic review. Reviews in the Neurosciences, 31: (2), 201–218. https://doi.org/10.1515/REVNEURO-2019-0047/ASSET/GRAPHIC/JREVNEURO-2019-0047_CV_004.JPG |
41 | Pearce, G. , O’Donnell, J. , Pimentel, R. , Blake, E. , Mackenzie, L. ((2023) ). Interventions to Facilitate Return to Work after Stroke: A Systematic Review. International Journal of Environmental Research and Public Health, 20: (15). https://doi.org/10.3390/IJERPH20156469 |
42 | Pérez-De La Cruz, S. ((2021) ). Comparison between Three Therapeutic Options for the Treatment of Balance and Gait in Stroke: A Randomized Controlled Trial. International Journal of Environmental Research and Public Health, 18: (2), 1–11. https://doi.org/10.3390/IJERPH18020426 |
43 | Pinto, C. B. , Teixeira Costa, B. , Duarte, D. , Fregni, F. (2018). Transcranial Direct Current Stimulation as a Therapeutic Tool for Chronic Pain. Journal of ECT. https://doi.org/10.1097/YCT.0000000000000518 |
44 | Pires, R. , Baltar, A. , Sanchez, M. P. , Antonino, G. B. , Brito, R. , Berenguer-Rocha, M. , Monte-Silva, K. ((2023) ). Do Higher Transcranial Direct Current Stimulation Doses Lead to Greater Gains in Upper Limb Motor Function in Post-Stroke Patients? International Journal of Environmental Research and Public Health, 20: (2). https://doi.org/10.3390/IJERPH20021279 |
45 | Prathum, T. , Piriyaprasarth, P. , Aneksan, B. , Hiengkaew, V. , Pankhaew, T. , Vachalathiti, R. , Klomjai, W. ((2022) ). Effects of home-based dual-hemispheric transcranial direct current stimulation combined with exercise on upper and lower limb motor performance in patients with chronic stroke. Disability and Rehabilitation, 44: (15), 3868–3879. https://doi.org/10.1080/09638288.2021.1891464 |
46 | Salazar, A. P. , Cimolin, V. , Schifino, G. P. , Rech, K. D. , Marchese, R. R. , Pagnussat, A. S. ((2020) ). Bicephalic transcranial direct current stimulation combined with functional electrical stimulation for upper-limb stroke rehabilitation: A double-blind randomized controlled trial. Annals of Physical and Rehabilitation Medicine, 63: (1), 4–11. https://doi.org/10.1016/J.REHAB.2019.05.004 |
47 | Savia, A. ((2020) ). Nuevas perspectivas en el manejo prehospitalario del accidente cerebrovascular. Neurologya Argentina, 12: (4), 260–270. https://doi.org/10.1016/j.neuarg.2020.07.004 |
48 | Sepúlveda-Contreras, J. ((2020) ). Caracterizacion de pacientes con accidente cerebrovascular ingresados en un hospital de baja complejidad en Chile. Universidad y Salud, 23: (1), 8–12. https://doi.org/10.22267/RUS.212301.208 |
49 | Stilling, J. M. , Monchi, O. , Amoozegar, F. , Debert, C. T. (2019). Transcranial Magnetic and Direct Current Stimulation (TMS/tDCS) for the Treatment of Headache: A Systematic Review. Headache. https://doi.org/10.1111/head.13479 |
50 | Tedla, J. S. , Rodrigues, E. , Ferreira, A. S. , Vicente, J. , Reddy, R. S. , Gular, K. , Dixit, S. ((2022) ). Transcranial direct current stimulation combined with trunktargeted, proprioceptive neuromuscular facilitation in subacute stroke: a randomized controlled trial. PeerJ, 10: , e13329. https://doi.org/10.7717/PEERJ.13329/SUPP-3 |
51 | Viganò, A. , Toscano, M. , Puledda, F. , Di Piero, V. (2019). Treating chronic migraine with neuromodulation: The role of neurophysiological abnormalities and maladaptive plasticity. Frontiers in Pharmacology. https://doi.org/10.3389/fphar.2019.00032 |
52 | Woods, A. J. , Antal, A. , Bikson, M. , Boggio, P. S. , Brunoni, A. R. , Celnik, P. , Nitsche, M. A. (2016). A technical guide to tDCS, and related non-invasive brain stimulation tools. Clinical Neurophysiology. Elsevier Ireland Ltd. https://doi.org/10.1016/j.clinph.2015.11.012 |
53 | Youssef, H. , Mohamed, N.A.E.H. , Hamdy, M. ((2023) ). Comparison of bihemispheric and unihemispheric M1 transcranial direct current stimulations during physical therapy in subacute stroke patients: A randomized controlled trial. Neurophysiologie Clinique=Clinical Neurophysiology, 53: (3). https://doi.org/10.1016/J.NEUCLI.2023.102895 |
54 | Zhao, L. , Liu, Z. , Sun, Q. , Li, H. ((2022) ). Effect of transcranial direct current stimulation combined with a smart hand joint training device on hand dysfunction in patients with early stroke. Folia Neuropathologica, 60: (2), 177–184. https://doi.org/10.5114/FN.2022.117534 |
55 | Zhu, C. , Yu, B. , Zhang, W. , Chen, W. , Qi, Q. , Miao, Y. ((2017) ). Effiectiveness and safety of transcranial direct current stimulation in fibromyalgia: A systematic review and meta-analysis. Journal of Rehabilitation Medicine, 49: (1), 2–9. https://doi.org/10.2340/16501977-2179 |
56 | Zortea, M. , Ramalho, L. , Alves, R.L. , Alves da, C.F.S. , Braulio, G. , Torres da, I.L.S. , Caumo, W. ((2019) ). Transcranial Direct Current Stimulation to Improve the Dysfunction of Descending Pain Modulatory System Related to Opioids in Chronic Non-cancer Pain: An Integrative Review of Neurobiology and Meta-Analysis. Frontiers in Neuroscience, 13: , 1218. https://doi.org/10.3389/fnins.2019.01218 |
Appendix A
Appendix A indicates the PEDro Scale score details of each randomized clinical trial included in the systematic review.
The PEDro Scale assesses the following sections: eligibility criteria were specified; subjects were randomly allocated to groups (in a crossover study, subjects were randomly allocated an order in which treatments were received); allocation was concealed; the groups were similar at baseline regarding the most important prognostic indicators; there was blinding of all subjects; there was blinding of all therapists who administered the therapy; there was blinding of all assessors who measured at least one key outcome; measures of at least one key outcome were obtained from more than 85% of the subjects initially allocated to groups; all subjects for whom outcome measures were available received the treatment or control condition as allocated, or, where this was not the case, data for at least one key outcome was analyzed by “intention to treat”; the results of between-group statistical comparisons are reported for at least one key outcome; the study provides both point measures and measures of variability for at least one key outcome.
Table A1
PEDro Score
Author (year) | PEDro scale score details |
(Andressa de Souza et al., 2021) | Eligibility criteria: No; Random allocation: Yes; Concealed allocation: Yes; Baseline comparability: Yes; Blind subjects: Yes; Blind therapists: Yes; Blind assessors: Yes; Adequate follow-up: Yes; Intention-to-treat analysis: Yes; Between-group comparisons: Yes; Point estimates and variability: Yes. |
(Ehsani et al., 2022) | Eligibility criteria: No; Random allocation: Yes; Concealed allocation: No; Baseline comparability: Yes; Blind subjects: No; Blind therapists: No; Blind assessors: Yes; Adequate follow-up: Yes; Intention-to-treat analysis: Yes; Between-group comparisons: Yes; Point estimates and variability: Yes. |
(Kashoo et al., 2022) | Eligibility criteria: Yes; Random allocation: Yes; Concealed allocation: No; Baseline comparability: Yes; Blind subjects: Yes; Blind therapists: No; Blind assessors: Yes; Adequate follow-up: Yes; Intention-to-treat analysis: Yes; Between-group comparisons: Yes; Point estimates and variability: Yes. |
(Klomjai et al., 2022) | Eligibility criteria: Yes; Random allocation: Yes; Concealed allocation: Yes; Baseline comparability: Yes; Blind subjects: Yes; Blind therapists: No; Blind assessors: Yes; Adequate follow-up: Yes; Intention-to-treat analysis: No; Between-group comparisons: Yes; Point estimates and variability: Yes. |
(Llorens et al., 2021) | Eligibility criteria: Yes; Random allocation: Yes; Concealed allocation: Yes; Baseline comparability: Yes; Blind subjects: No; Blind therapists: No; Blind assessors: Yes; Adequate follow-up: Yes; Intention-to-treat analysis: No; Between-group comparisons: Yes; Point estimates and variability: Yes. |
(Pires et al., 2023) | Eligibility criteria: Yes; Random allocation: Yes; Concealed allocation: Yes; Baseline comparability: Yes; Blind subjects: No; Blind therapists: No; Blind assessors: Yes; Adequate follow-up: Yes; Intention-to-treat analysis: Yes; Between-group comparisons: Yes; Point estimates and variability: Yes. |
(Tedla et al., 2022) | Eligibility criteria: Yes; Random allocation: Yes; Concealed allocation: Yes; Baseline comparability: Yes; Blind subjects: Yes; Blind therapists: No; Blind assessors: Yes; Adequate follow-up: Yes; Intention-to-treat analysis: Yes; Between-group comparisons: Yes; Point estimates and variability: Yes. |
Appendix B
Appendix B show more characteristics of each randomized clinical trial included in the systematic review.
Table B1
Specific details of studies
Author (year) | Institution or clinical center and country |
(Andressa de Souza et al., 2021) | Nove de Julho University, São Paulo, Brazil |
(Ehsani et al., 2022) | Semnan University of Medical Sciences, Semnan, Iran |
(Kashoo et al., 2022) | University Hospital of the National Institute of Medical Sciences, Rajasthan in Jaipur, India |
(Klomjai et al., 2022) | Siriraj Hospital, Bangkok, Thailand |
(Llorens et al., 2021) | Hospital Vithas Valencia al Mar, Valencia, Spain |
(Pires et al., 2023) | Laboratory of Applied Neuroscience (LANA), Universidade Federal de Pernambuco, Brazil |
(Tedla et al., 2022) | King Khalid University, Abha, Aseer, Saudi Arabia |