Impact of black raspberries on the normal and malignant Apc deficient murine gut microbiome
Abstract
BACKGROUND:
In animals and humans, black raspberries (BRBs) have chemo-preventative effects against Wnt driven colorectal cancer (CRC). While BRBs have made it into clinical trials, the exact mechanisms of BRB action remain unclear. Potentially the chemo-preventative properties are linked to their impact on the gut microbiome, as diet is known to influence the microbial diversity of the gut and plays a key role in regulating intestinal homeostasis and the aetiology of CRC.
OBJECTIVE:
To determine the impact of a BRB diet on the microbial biodiversity of the wild-type and malignant mouse intestine.
METHODS:
Adult mice in which Wnt driven tumourigenesis could be initiated by conditional deletion of Apc in the intestinal stem cell (Lgr5CreERT2) were administered a 10% BRB diet. Total DNA from faecal pellets pre- and post-BRB exposure was used for longitudinal metataxonomic analysis of the V1 to V3 regions of the 16S rRNA gene.
RESULTS:
Individually BRB intervention and Apc loss alter the microbial community of the gut. In combination, the microbiome changes observed in the Apc deficient intestine are attenuated upon administration of a BRB diet.
CONCLUSIONS:
Our results suggest that BRB intervention may protectively regulate the gut microbiota in the healthy and malignant intestine.
Abbreviations
CRC | Colorectal cancer |
BRB | Black raspberry |
HFD | High-fat diet |
ISC | Intestinal stem cell |
UC | Ulcerative colitis |
SCFA | Short chain fatty acids |
AIN-76A diet | American Institute of Nutrition-76A diet |
IP | Intraperitoneal |
d.p.i | Days post induction |
PCA | Principle component analysis |
F1 | Faecal sample 1 |
F2 | Faecal sample 2 |
F3 | Faecal sample 3 |
FAP | Familial adenomatous polyposis |
NS | Not significant |
DP | Difference between mean proportions. |
1Introduction
It is widely accepted that several factors contribute to the development of sporadic colorectal cancer (CRC) [1]; a leading cause of cancer death worldwide [2]. There is substantial evidence that genetic and epigenetic alterations [1], inflammatory bowel diseases [3], and environmental factors e.g. diet [4], contribute to the aetiology of CRC. Worldwide it is estimated that ∼30–50% of all cancer cases [4] and 54% of UK CRC cases could have been prevented through lifestyle changes [5], emphasising the importance of cancer prevention. The impact of certain diets on the progression of CRC are beginning to be understood. For example, high-fat diets (HFD), a major contributor to obesity, have been shown to increase the risk of developing CRC by impacting on the number and tumourigenic potential of intestinal stem cells (ISCs) [6], the cell-of-origin of CRC [7]. In contrast, several studies have documented the beneficial effects of high fibre diets against CRC [8]. Dietary fibre is fermented by colonic microbes to produce short chain fatty acids (SCFAs) such as butyrate, which is a vital source of energy for colonocytes [9, 10]. Moreover, several studies have documented the beneficial effects of the berry fruit, including black raspberries (BRB), against CRC in humans and in animal models. Specifically, diets containing BRBs have been shown to reduce ulcerative colitis (UC) in rodent models (inflammatory disease) [11] and reduce CRC tumour and polyp burdens in both mice and humans [12–15]. While these studies have advanced our understanding of the impact of BRBs on CRC and have made it into clinical trials, the exact mechanisms of action remain unclear. However, it is likely that the gut microbiome plays a role, as diet is known to influence the diversity of the gut bacteria in both animals and humans [16]. This interaction between dietary intake and the microbiota has been well studied [17, 18] and it has been demonstrated in mice that the constant exposure of the large intestine to ∼103 different commensal bacterial species, a total of ∼1014 cells, effects immune and inflammatory responses and cancer predisposition [9, 19–21]. The microbiome has an essential role in regulating normal gut homeostasis and there is a plethora of evidence indicating that specific microbial profiles may underpin many inflammatory diseases such as UC and Crohn’s disease [22], metabolic diseases such as diabetes [23] and is linked to obesity [24], all of which have a predisposition to CRC. For example, it has been shown that obese individuals have reduced numbers of Bacteroidetes and a decreased microbial diversity and genetic abundance, while the levels of Firmicutes are elevated in these obese individuals [25, 26]. In contrast, healthy lean individuals have a greater microbial biodiversity and an increased proportion of Bacteroidetes to Firmicutes [25, 26]. Interest in the gut microbiome and their associated metabonomes in human disease including cancer [8, 27], has placed emphasis on the need to further understand the complex underlying mechanisms involved in physiological but also pathophysiological interactions between diet-host-microbiome.While many studies have shown that consumption of certain dietary fibres and phytonutrients impacts the microbial diversity and function of the gut microbiota, there is limited understanding on the impact of the CRC chemo-preventative fruit, BRBs, on the gastrointestinal microbiome. Here, using a longitudinal study design we examined the impact of a black raspberry-based gut microbiome modulation in a CRC setting using the Lgr5CreERT2 Apcfl/fl mouse model of CRC, which drives tumourigenesis from the intestinal stem cell. In this study, we aimed to further our knowledge of the impact of BRB consumption on the gut microbiota in a wild type and intestine at the earliest stages of malignancy to determine whether the microbiome is likely to play a role in the chemo-preventative properties of BRBs.
2Methods
2.1Black raspberry (BRB) diet
The 10% BRB diet was produced by Dyets Inc, USA. In brief, freeze-dried BRB powder [28] (purchased from Berrihealth USA), was incorporated into purified American Institute of Nutrition (AIN)-76A (protein, 20.8 kcal%; carbohydrate, 67.7 kcal%; and fat, 11.5 kcal%) animal diet pellets by 10% w/w concentration at the expense of sucrose. The BRB and control (AIN-76A) diets were stored at 4°C until being administered to the animals.
2.2Animals and ethics
All animal procedures were conducted in accordance with institutional animal care and NC3R(UK) ARRIVE guidelines. Work was approved under a UK Home Office Project license (30/3279; protocols 6 & 7) issued under the Animals (Scientific Procedures) Act 1986. The animals were maintained on an outbred background and were housed in a standard facility. In brief, mice were maintained in conventional open top cages, with 2/2 spruce fir tree bedding (IPS Ltd) under a 12 hr light cycle, with water and RM3(E) diet (expanded diet, Special Diet Services UK), ad libitum, provided for nutritional support. To enrich the environment, sunflower seeds (at weaning only, LBS Ltd), nestlets (IPS Ltd), disposable envirotubes (IPS Ltd) and small chewsticks (Labdiet-IPS Ltd) were provided. At weaning age, mice were genotyped as previously described, for the Lgr5CreERT2 transgene [29] and for the targeted Apcfl/fl allele [30]. Genotyping conditions are available upon request. Adult Lgr5CreERT2 Apcfl/fl mice (11–15 weeks, N = 4 per cohort with 2 males and 2 females in each group) were randomly assigned to either control (AIN-76A) or treatment (BRB) groups. Before administering the respective diets, faecal samples (F1) were collected from individual mice and stored at –80°C. After two weeks on their respective diets, a second faecal sample (F2) was collected prior to inducing Apc loss in the intestinal stem cells (ISC). Lgr5CreERT2 Apcfl/fl mice were induced by intraperitoneal (IP) injection with 80 mg/kg Tamoxifen dissolved in corn oil (Sigma, UK) once daily for 4 consecutive days. This results in homozygous loss of the Apc allele in the ISC which leads to adenoma formation as previously described [7]. Twenty days post induction (d.p.i), a final faecal sample (F3) was collected prior to sacrifice. Mice were weighed three times a week from the start of the study and remained on their respective diets throughout. A schematic representation of the animal bioassay and comparisons analysed are shown in Fig. 1 and Table 1 respectively.
Fig.1
Schematic representation of BRB exposure, recombination of Apcfl/fl alleles and longitudinal faecal sampling regime.
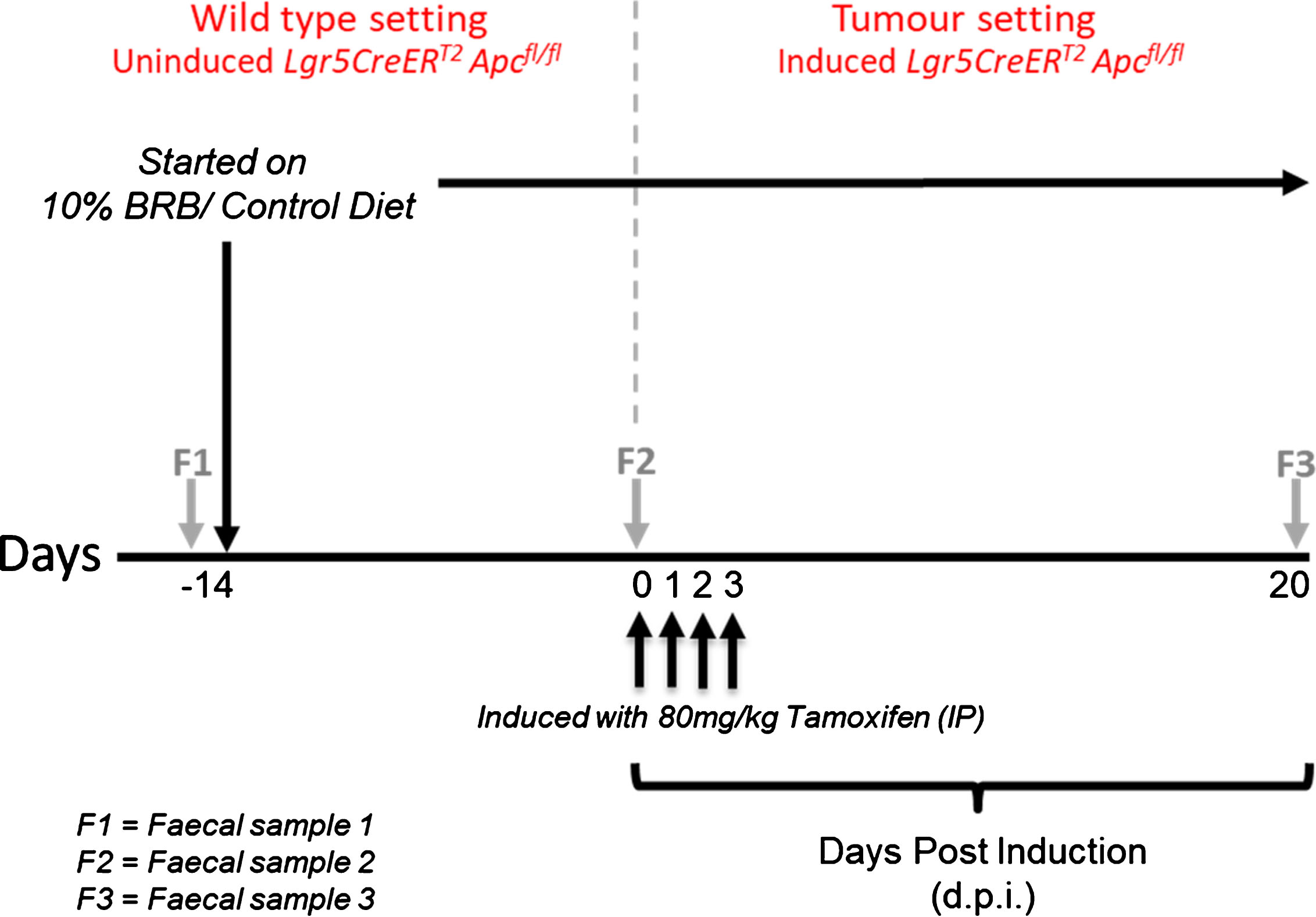
Table 1
Summary of the comparisons analysed in this longitudinal study
Figure | Comparsion | d.p.i. | Diet | N number |
2 | F1 from all uninduced Lgr5CreERT2 Apcfl/fl mice prior to start of experiment | –14 days | In house chow | 8 mice (4 put on control diet and 4 put on 10% BRB diet after faecal sampling of F1) |
3 | F2 from uninduced Apcfl/fl mice | day 0 (day of induction) | AIN-76A control diet vs 10% BRB diet | 4 mice per diet cohort |
5 | F2 from uninduced Apcfl/fl and F3 from induced Apcfl/fl mice | day 0 vs day 20 | AIN-76A control diet | 4 mice per faecal timepoint |
6 | F2 from uninduced Apcfl/fl and F3 from induced Apcfl/fl mice | day 0 vs day 20 | 10% BRB diet | 4 mice per faecal timepoint |
7 | F3 from induced Apcfl/fl mice | day 20 | AIN-76A control diet vs 10% BRB diet | 4 mice per diet cohort |
F1 = faecal sample 1, F2 = faecal sample 2, F3 = faecal sample 3.
2.3DNA extraction
All faecal pellets were stored at –80°C immediately until required. Total bacterial DNA was extracted from the faecal samples using the FastDNA® SPIN Kit for Soil and the FastPrep® Instrument (MP Biomedicals, Santa Ana, CA), as per manufacturer details. DNA concentrations were quantified using the Nanodrop2000 (Thermo Scientific, UK).
2.4Sequencing and analysis of data
Total DNA extracted from faecal pellets was used for metataxonomic analysis of the V1 to V3 regions of the 16S rRNA gene. Sequence data was provided by Research and Testing Laboratory (Lubbock, TX, USA) as described previously [31]. The data was analysed using the Mothur program v 1.37.0 and the MiSeq pipeline [32].
2.5Statistics
Differences in the OTU data at all taxonomic levels (phylum to genus) was tested for the different interventions using STAMP [33] using White’s non-parametric test and multiple test correction using the Benjamini-Hochberg FDR test, error bars are 95% confidence intervals using the DP:bootstrap method (DP = difference between mean proportions). Analysis of difference between clusters was tested using the PERMANOVA test (Adonis command in Vegan package) in R. A Shapiro-Wilk normality test was used to determine whether mouse body weights at specific time points (–14, 0 and 20 d.p.i) were normally distributed. To test for significance in % body weight change, an unpaired t-test was used, error bars represent standard error of mean. Statistical significance was defined as P≤0.05.
3Results
3.1Consumption of a BRB enriched diet alters the composition of the gut microbiome
Previous studies have demonstrated the impact of a 10% freeze-dried BRB diet on the composition and diversity of mouse gut microbial communities [34]. Due to the natural variations that exist in murine gut microbiomes between animal facilities we first sought to characterise the microbiome in our mice prior to onset of cancer.Faecal pellets were collected from wild type (uninduced Lgr5CreERT2 Apcfl/fl(N = 8)) mice prior to AIN-76A control or BRB (ad libitum) dietary modification (F1; Fig. 1). At this stage (F1), PCA of mice bacterial community structure indicated no clear separation of the microbial communities’ present in the faeces (Fig. 2, square represents mice that will be fed AIN-76A control diet and circle represents mice that will be fed BRB diet). As individual microbiomes were comparable, mice were exposed to control or BRB diets ad libitum for two weeks. Analysis of faecal samples at the F2 sampling point (Fig. 1) from uninduced Apcfl/flmice 14 days after BRB dietary modification, demonstrated a clear shift in the composition of the microbiome in a ‘wild type’ setting (Fig. 3A). No individual taxonomic group, from phylum to genus level, were found to be altered when an FDR was applied, however pre-FDR analysis shows trends to support the hypothesis that BRB diet is associated with leanness after BRB fed mice appeared to have reduced Firmicutes and increased levels of Bacteriodetes compared to control (Fig. 3B). To ensure there were no adverse effects of BRB intervention on murine body weight and to monitor symptoms of intestinal tumourigenesis, all mice were weighed throughout the study (Fig. 4A). There was no significant difference in mouse % body weight at day 0, 14 and 20 d.p.i between control or BRB fed mice (Fig. 4B), however, on average BRB-fed mice appear to have slightly lower body weights then control fed mice throughout the study (Fig. 4A).
Fig.2
Composition of the gut microbiota of communities are equivalent prior to BRB dietary modification. A principal co-ordinate analysis (PCA) of unweighted unifrac distances indicating no clear separation of the microbial communities’ present in each animal. This result was supported by PERMANOVA analysis in R. Faecal samples of 4 uninduced control Lgr5CreERT2 Apcfl/fl () and 4 uninduced experimental Lgr5CreERT2Apcfl/fl (
) mice taken at the F1 sampling point (prior to commencement of BRB dietary modification).
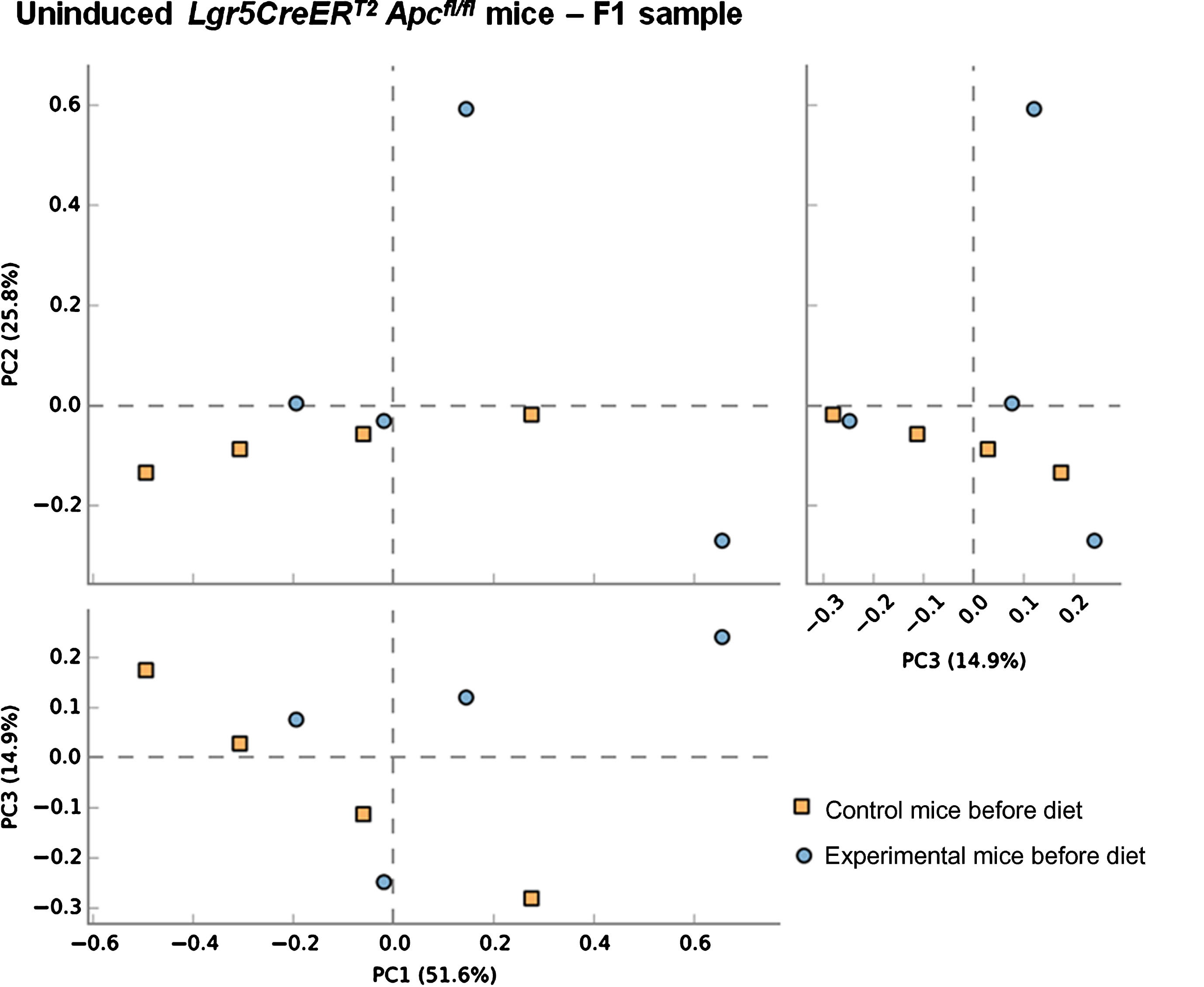
Fig.3
Consumption of a BRB diet alters microbial composition in the uninduced Lgr5CreERT2 Apcfl/flintestine. A) Comparison of faecal samples taken at the F2 (; AIN-76A control diet) and F2 (
; BRB diet) sampling point from the uninduced Lgr5CreERT2 Apcfl/fl cohort. A PCA plot of the communities, based on the genera in each sample, indicates separation of the two groups when PC1 vs PC2 and PC1 vs PC3 are plotted, this result was supported by a PERMANOVA analysis in R. B) Pre-FDR analysis highlights 2 week feeding of BRBs resulted in an increased abundance of Bacteroidetes and reduced Firmicutes compared to control fed mice however, when an FDR is applied no one genus was shown to alter due to the feeding intervention, but the whole community shifted its composition in response to the BRB diet. N = 4 mice per cohort, error bars represent 95% confidence intervals using the DP:bootstrap method (DP = difference between mean proportions).
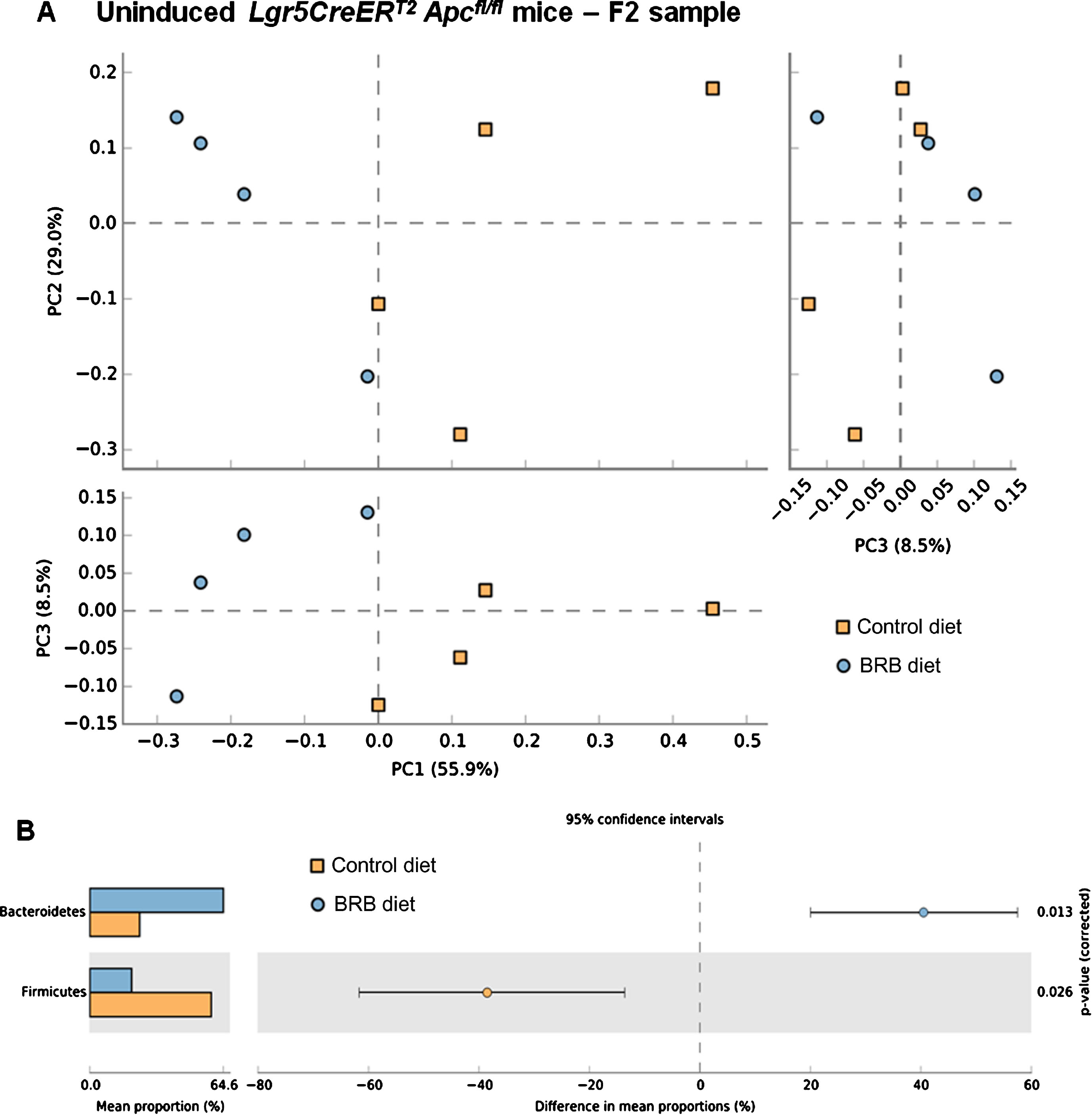
Fig.4
Feeding of 10% BRB diet does not alter mouse body weight over time. (A) A line graph showing % change in body weight over time. At time point – 14 days uninduced Lgr5CreERT2 Apcfl/fl mice (N = 4 per cohort) were administered either control or BRB diet. On day 0 mice were weighed prior to Tamoxifen induction of Cre-mediated Apc loss from the intestinal stem cell compartment. Mice were weighed continually until day 20 post induction where mice were culled and sampled. (B) Scatterplots showing at –14, 0 and 20 days post induction there was no significant difference in % mouse body weight of BRB fed mice compared to control fed mice. N = 4 mice per diet cohort, error bars represent standard error of mean, NS = not significant as determined by a paired t-test.
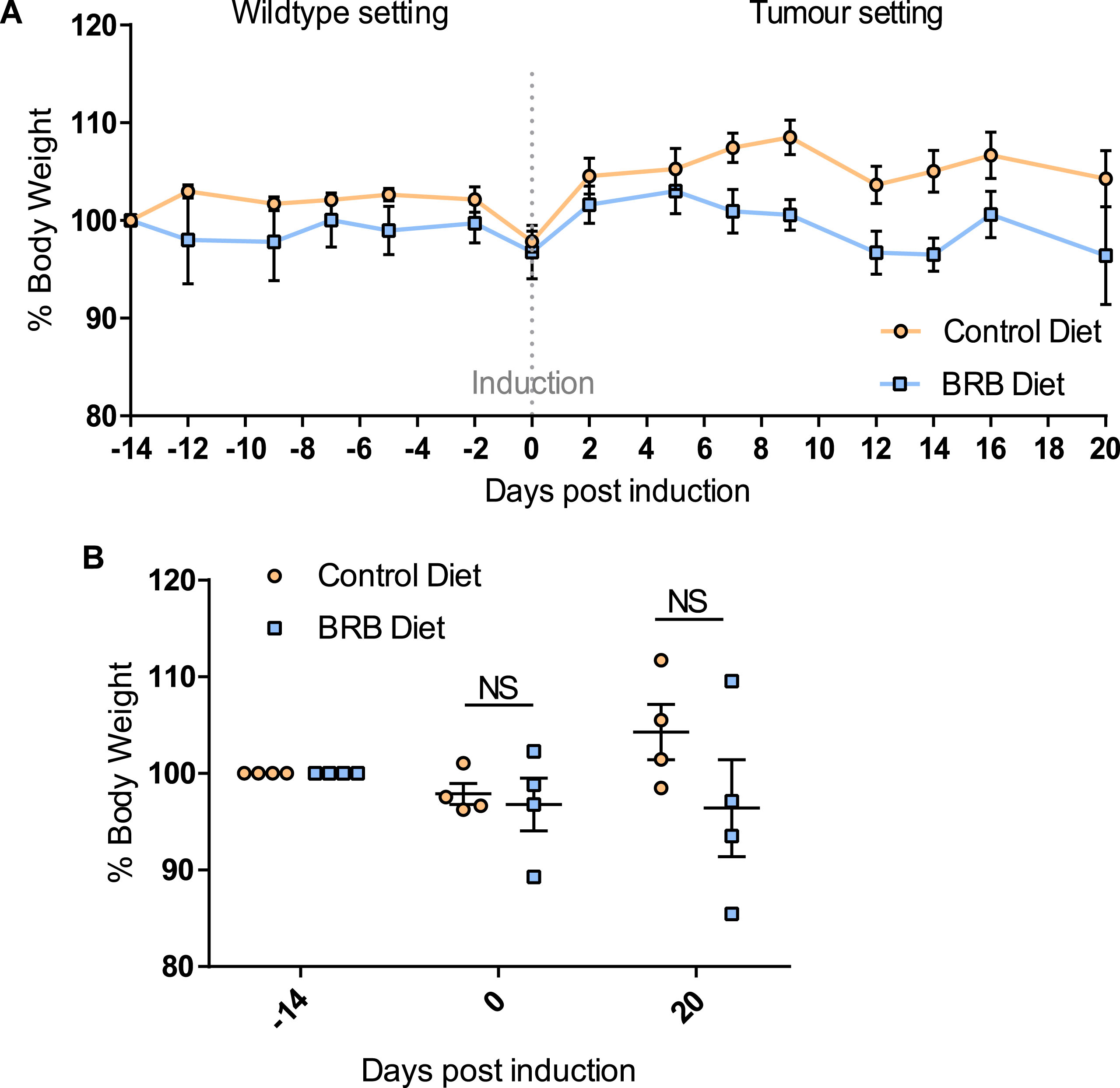
3.2Loss of Apc from the intestine stem cell alters the composition of the gut microbiome
Prior to analysis of the effects of BRBs during tumourigenesis we first analysed the impact of ISC Apc loss on the microbiome, which mimics the initiation stages of colorectal tumourigenesis [7]. PCA analysis demonstrated a clear shift in the composition of the bacterial communities in control fed Lgr5CreERT2Apcfl/fl20 days following the deletion of Apc in the ISC (comparing F2 and F3) (Fig. 5A), providing evidence that the tumourigenic process immediately impacts the gut microbiome. Further analysis at taxonomic class levels reflects increases in Bacteroides and Clostirdium_XVIII species are the main contributors to the indicated community changes observed (Fig. 5B). However, due to the small number of samples (N = 4) the changes failed to reach statistical significance when an FDR was applied.
Fig.5
Loss of Apc from the ISC alters the microbial composition of the Lgr5CreERT2 Apcfl/fl intestine. (A) A PCA plot comparing faecal communities of control diet fed Lgr5CreERT2 Apcfl/fl mice before (; F2) and 20 days following Apc ISC deletion (
; F3) demonstrates a clear separation of the two groups when PC1 vs PC2 and PC1 vs PC3 are plotted, further supported by PERMANOVA analysis in R. (B) Classification of sequences by taxonomy at the class levels indicates trends to support the changes in overall community structure but application of a FDR indicates the changes are not significant at the genus level. P values are pre-FDR corrected, error bars represent 95% confidence intervals using the DP:bootstrap method. N = 4 mice fed control diet per faecal sampling time point, note this is longitudinal faecal sampling from the same mice at different time points.
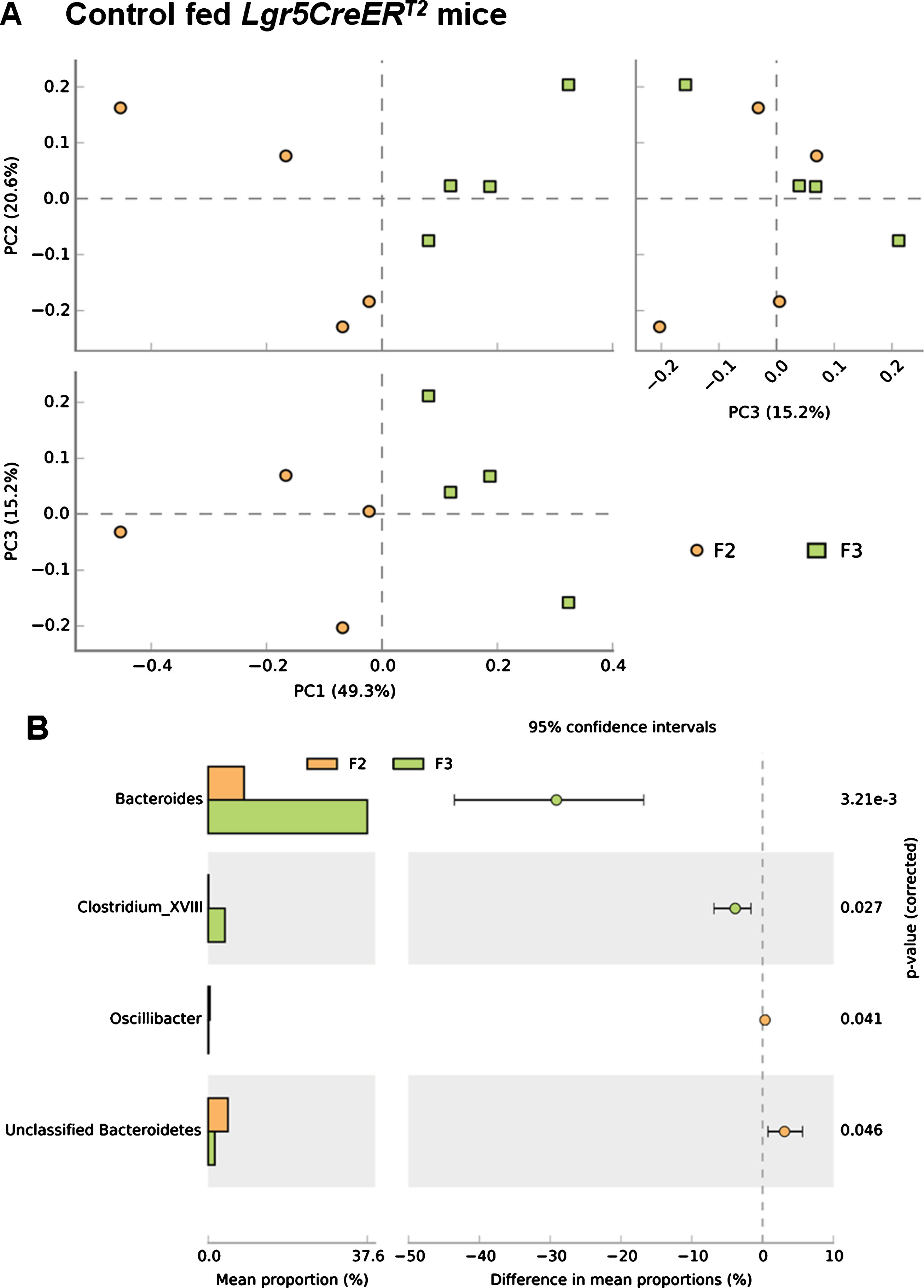
3.3Two week BRB feeding prior to Apc loss minimizes the impact of ISC Apc loss on the composition of the gut microbiome
We next sought to address whether BRB intervention prior to the onset of intestinal tumourigenesis by induction of ISC Apc loss influences the microbiome of mice 20 days post induction. Comparison of F2 (square) and F3 (circle) faecal samples from BRB fed Lgr5CreERT2 Apcfl/fl mice shows a slight alteration to the gut microbiome composition (Fig. 6). These changes in bacterial composition appear to be less than the change that occurs from ISC Apc loss in the control diet setting (Fig. 5), which indicates that BRBs can attenuate the cancer-induced microbiome alterations. Finally, we wanted to evaluate whether there were any alterations to the gut cancer microbiome as a result of BRB exposure. Surprisingly, PCA analysis on F3 samples from mice showed there were no differences in the gut microbiome composition in tumour bearing Lgr5CreERT2 Apcfl/fl mice fed control or BRB diets at 20 days post Apc loss (Fig. 7).
Fig.6
Consumption of BRB diet prior to the loss of Apc from the ISC reduces the alterations to the microbial composition of the Lgr5CreERT2 Apcfl/fl intestine. A PCA plot comparing faecal communities of BRB diet fed Lgr5CreERT2 Apcfl/fl mice before (; F2) and 20 days following Apc ISC deletion (
; F3) demonstrates a clear separation of the two groups when PC1 vs PC3 and PC2 vs PC3 are plotted, further supported by PERMANOVA analysis in R. N = 4 mice fed BRB diet per faecal sampling time point, note this is longitudinal faecal sampling from the same mice at different time points.
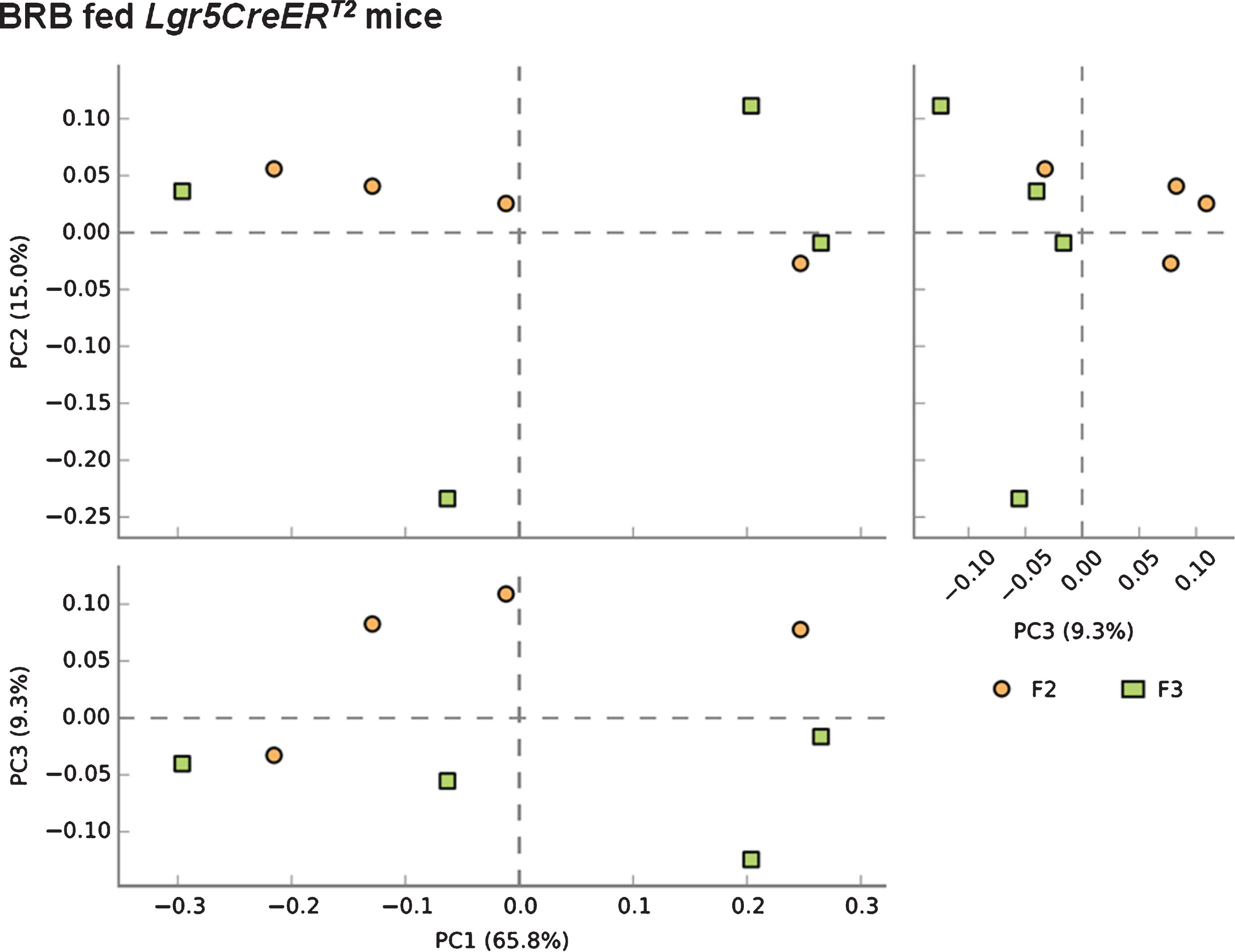
Fig.7
Consumption of a BRB diet does not alter microbial composition in the Lgr5CreERT2Apcfl/fl intestine 20 days post ISC Apc loss. Comparison of faecal samples taken at the F3 (; AIN-76A control diet) and F3 (
; BRB diet) sampling point from the Lgr5CreERT2Apcfl/fl cohort. A PCA plot of the communities for animals fed a BRB indicating no separation of the two groups, this result was supported by PERMANOVA analysis in R. Indicating these mice were refractory to BRB induced microbiome changes. N = 4 mice per cohort.
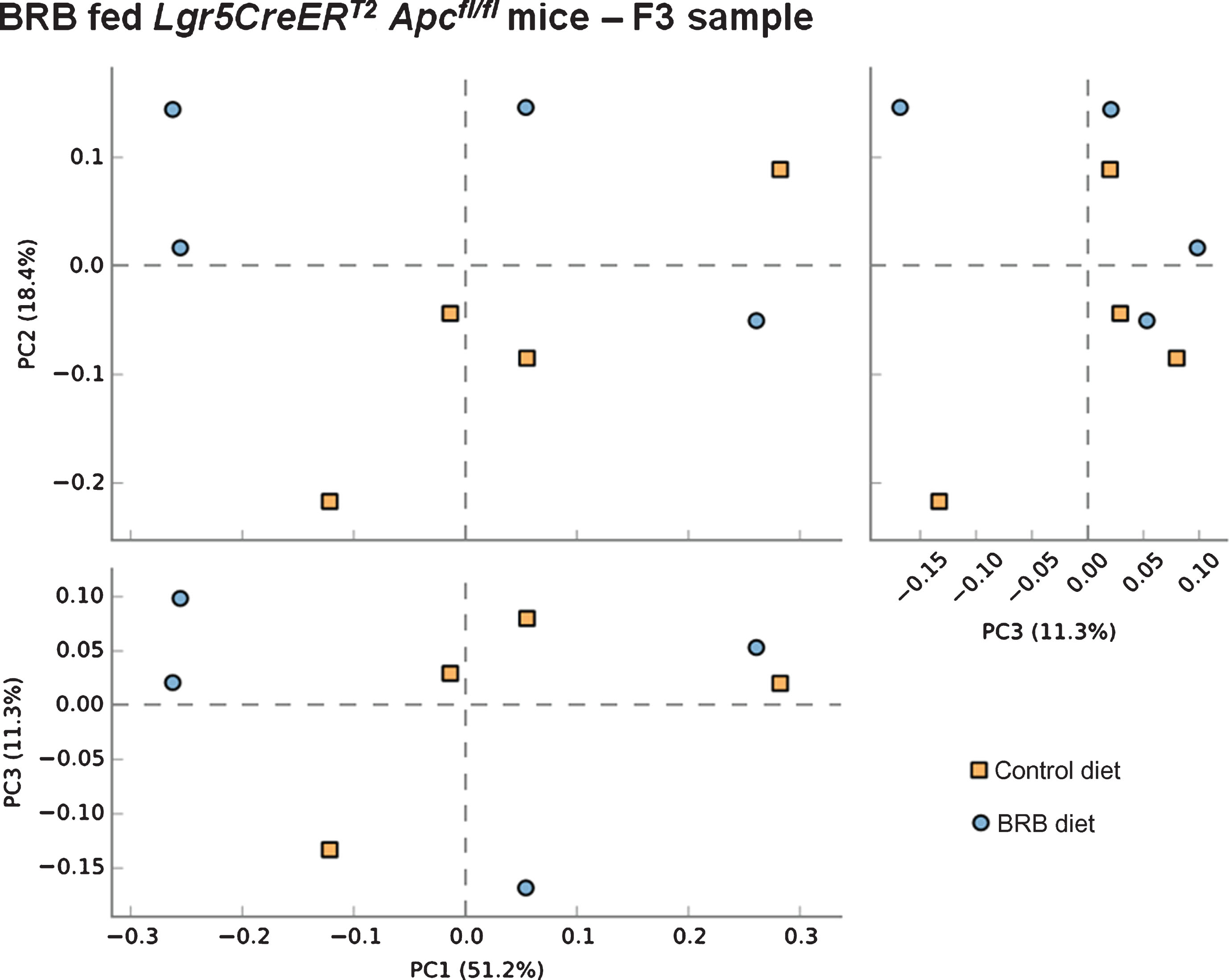
4Discussion
Chemoprevention refers to the use of naturally occurring or synthetic agents to reverse, suppress or delay the development of carcinogenesis [35, 36]. One of the growing concerns in the cancer field is the increase in cancer incidence globally, where there is now substantial evidence that shows the Western lifestyle is strongly attributable to several cancer types, in particular colon and liver cancer [4]. In the UK alone, it is estimated that over half of CRCs could have been prevented through lifestyle changes [5]. In recent years several studies have highlighted the benefits of natural food sources (most of which are rich fibre sources) and plant-based chemicals on human health and thus, these may be able to be modified for preventative strategies. For example, in a randomized, controlled feeding study in healthy humans, addition of broccoli to the diet at 200 g/day decreased the abundance of Firmicutes and increased the relative abundance of Bacteroidetes and Bacteroides compared to control [37]. This ratio of Bacteroides/Firmicutes is associated with leaner body weights [25, 26]. In addition, BRBs have previously been shown to reduce tumour/polyp burden in CRC and FAP patients and in murine models of CRC [12–14, 38]. The chemo-preventative effects of BRBs have been attributed to several biological processes such as, reducing inflammation, inhibiting the Wnt signaling pathway (the main driver of CRC), and altering the epigenome to reactivate silenced Wnt pathway antagonists [11–15, 38, 39]. There is now a growing body of evidence indicating that our diet impacts on the gut microbiome. The commensal gut microbiota has a mutualistic role within our body such that it regulates gut homeostasis, aids digestion of indigestible fibre and helps maintain the immune system [40]. However, changes to the composition of the gut microbiota is known to result in dysbiosis which is strongly linked to several diseases including obesity, inflammatory bowel diseases and ultimately CRC [22–24, 41, 42]. Due to the impact of diet on the gut microbiome it is possible that the chemo-preventative effects of BRBs are in part due to their effect on the gastrointestinal microbiota as previous studies have reported that BRBs influence the normal gut microbiota of healthy mice and rats [43, 44]. Thus, we sought to investigate what effect BRB feeding had on the microbiome in a ‘wild type’ intestine and what effect BRBs had on the microbiome of a transgenic murine model of CRC at the early stages of colorectal tumourigenesis. Herein, we report that intervention with BRB diet in mice alters the microbiome of the normal healthy gut. Additionally, we show that the gut microbiome is influenced by onset of tumourigenesis, and this modification to the gut microbiota may be minimised in the context of BRB feeding.
It has previously been documented that the murine gut microbiota can differ depending on their location (i.e. which institution they are in), inter and intra-species heterogeneity, cage effects, diet, age and stress [45, 46]. Thus, to minimise the variation in the microbiota composition of the mice at the start of the experiment, we used age-matched litter mates from one mouse colony, which were in the same room on the same rack within the animal facility. In order to accurately track the changes in the microbiome we sampled faeces from the same mice at 3 time points throughout the study, to 1) determine the impact of 2-week feeding on BRB diet on the ‘normal’ gut microbiome, 2) to determine the impact of Apc loss in the ISC population, which represents the initiation stage of CRC and 3) to evaluate the effect of BRBs on the gut microbiome in mice after the onset of tumourigenesis when cancerous lesions are present in the small intestine. Our results indicate that before the commencement of the control AIN-76A diet or control diet supplemented with 10% freeze-dried BRBs, the composition of the gut microbiota was similar among all 8 healthy wild type mice (uninduced Lgr5CreERT2 Apcfl/fl) (Fig. 2). This highlights that any changes in the microbiota communities from subsequent faecal samples is from the BRB dietary modification and/or the onset of tumourigenesis. Previous studies have demonstrated that feeding of freeze-dried BRBs and their anthocyanin-derivatives alter the microbial community of healthy wild type mice and rats [43, 44]. In support of this data, we also report herein, that 2-week feeding of BRB diet alters the composition of the murine gut microbiome compared to control diet (Fig. 3A). However, due to the small number of 4 mice per cohort, we were not able to detect any significant microbial alterations at the Phyla or Genus level, unlike the previous studies which indicated BRBs reduce the abundance of Firmicutes and increased Bacteroidetes compared to the control diet [44], which has been observed in leaner individuals and mice [25, 47]. However, we report a similar trend of Bacteroidetes to Firmicutes ratio pre-FDR analysis (Fig. 3B). Although not significantly altered after 2 weeks on the BRB diet, healthy mice with BRB intervention also appear to have a lower body weight on average than control fed mice, even after the onset of tumourigenesis (Fig. 4A), which may be associated to the whole community shift in the microbiome and the preferential Bacteroidetes/ Firmicutes ratio.
It is now well established that the gut microbiome is substantially altered in cancer patients, although it is unknown whether microbial changes have causative features or are a consequence of cancer onset. Several studies have documented that CRC patients tend to have more Fusobacteria, Proteobacteria, Clostridium, Dialister, Peptostreptococcus, unclassified Clostridiales and Oscillospira than control patients [48, 49]. However, some studies have reported contradictory findings on Bacteroides levels in CRC patients. While Bacteroides are usually found to be reduced in abundance in CRC patients compared to control patients [50], another group reported that Bacteroides were increased in CRC patients [51]. It is likely that the composition of microbial communities is influenced by several factors including tumour burden and the stage of the disease and thus, results from all microbiome studies should be carefully interpreted. In our study, we report that 20 days after onset of colorectal tumourigenesis through the loss of the tumour suppressor gene Apc from the ISC compartment, resulted in a shift in the microbial community (Fig. 5A). Contrary to studies which associate increased Bacteroides with healthy weights [25, 26, 50] and thus reduced risk of obesity and CRC, we report similar trends to Sobhani et al. (2011) of increased Bacteroides in tumour bearing mice (Fig. 5B). However, when an FDR was applied, these changes were not found to be significant, highlighting the need for larger cohort sizes in the future.
We next looked to evaluate whether addition of BRBs to the diet prior to tumour onset, offsets the changes to the microbial community seen in the malignant setting. We report that the microbial composition at 20 d.p.i. in BRB-fed tumour bearing mice does shift from the composition observed in the same mice prior to tumour onset (Fig. 6), however, this change in microbial community appears to be less than the shift seen in the control diet tumour setting (Fig. 5A). This suggests that BRB intervention may limit the cancer-induced microbial changes and thus, may exert a protective effect against CRC progression, however, this needs to be investigated further. When analysing the effects of BRBs on the microbiome on tumour-bearing mice at 20 d.p.i compared to control fed tumour mice we report there were no alterations to the microbial community (Fig. 7). This was somewhat surprising, given that the shift in the microbiome in BRB-fed mice following Apc loss appeared to be less drastic than the shift in control fed mice following Apc loss. However, as previously eluded to, the composition of microbial communities can be influenced by tumour burden and the stage of the disease. As it has been previously reported in both animal models and human patients that BRBs protect against CRC and FAP disease by reducing tumour/polyp burden [12, 14], it is possible that the lack of change in microbial communities may reflect differences in tumour burden in our mice. Therefore, future studies should evaluate tumour burden in these cohorts. It is also important to note, that while animal models have been invaluable in deciphering the mechanisms that cause cancer and have advanced our understanding of the microbiome, they do not fully recapitulate the human body, such that the site of tumourigenesis is different between human and mouse [52], the baseline microbiomes would be different as a result of mice having a vegetarian diet unlike many humans [53], and the number of tumours that form in Lgr5CreERT2 Apcfl/fl mice is not representative of the in vivo human setting, such that the induction regime used in this study results in a large tumour burden throughout the entire small intestine and colon [7]. Thus, in order to accurately decipher microbial changes and possible chemo-preventative mechanisms in the context of BRB feeding in the healthy and malignant gut, future studies using human faecal samples are essential.
As demonstrated above, the mouse gut microbiome was substantially changed by BRBs in both the healthy intestine and following tumour onset, which may have protective effects against CRC initiation and progression. However, understanding the specific interactions between the host-gut microbiome and in the context of dietary modification would enable us to develop better therapeutic strategies, thus it is important that future studies investigate potential mechanisms in both animal models but more importantly in humans. Specifically, through qRT-PCR it would be useful to characterise the effects of BRBs on specific microbes at the Genus level, and on the respective gut metabolomes in control and BRB fed mice in the healthy and malignant intestine. In particular analysing the levels of SCFAs, for example butyrate – a microbial bi-product which is the main source of colonocyte energy, that has been shown to be a potent histone deacetylase inhibitor (epigenetic regulator), anti-inflammatory and have protective effects against CRC [54], would shed light on the impact of the microbiome and the metabolome in CRC prevention. Identifying the specific microbiota altered in the context of BRB intervention may enable us to correlate any metabolic changes to particular butyrate-producing phyla, allowing us to underpin the more intricate mechanisms involved in dietary cancer chemoprevention. In addition, given the known disturbances in epithelial barrier integrity and mucosal layers of the malignant gut [55] it would be interesting to evaluate if BRBs protect against CRC and dysbiosis by protectively regulating the epithelial barrier. Several studies have reported in CRC cell lines that butyrate stimulates mucus production and maintains epithelial integrity [56, 57], thus it may be possible that increases in butyrate-producing microbiota may protect the intestinal epithelial from insult. Commonly associated with defects in the intestinal epithelial barrier, and a common driver of CRC is the onset of inflammation due to the presence of bacteria in the colonic tissue [58]. Previously, BRBs have been shown to reduce inflammation in murine models of ulcerative colitis [11] and CRC [12], in rat models of oesophageal cancer [59], and enhance natural killer cell immunology in CRC patients [60]. Specifically, in mouse models of oesophageal cancer, BRBs were found to increase the expression of the anti-inflammatory cytokine IL-10 [59], which is associated with regulatory T cells that dampen inflammation and maintain immune homeostasis [61], and thus, has roles in reducing cancer risk. Moreover, it has been shown that the infiltration of bacteria to the colonic epithelium of adenoma-bearing mice induces, expression of the pro-inflammatory cytokine Th17 [58]. IL-10 is required to suppress Th17 cell-mediated inflammation [62]. It may be possible that BRB intervention modifies the microbiome to induce the expression of IL-10 to reduce Th17-mediated inflammation. However, in the study by Bi et al. (2010), 12-week feeding of BRBs was shown to reduce the expression of IL-10 in the Muc2–/– murine model of inflammation driven CRC [12], opposite of the effects of BRBs on IL-10 expression in oesophageal cancer, suggesting model-specific phenotypes. This again, highlights the importance of performing studies on human tissue to faithfully understand the effects and mechanisms of BRB intervention for CRC. Despite this, future studies using mouse models should also investigate the role of BRBs on microbiome-mediated inflammatory responses for chemoprevention.
5Conclusion
In summary, the current study demonstrated that BRB intervention was able to alter the composition of the gut microbiome in healthy and intestinal tumour-bearing mice. These alterations in the microbial communities’ present in the bowel in the context of BRB chemoprevention, may elicit protective roles against colorectal carcinogenesis, and the microbiome itself may serve a predictive biomarker to monitor at risk patients of CRC development.
Conflict of interest
The authors have no conflict of interest to report.
Author Contributions
Study design, acquisition of data, analysis, and interpretation of data: Stephanie May, Grace McDermott, Julian R Marchesi and Lee Parry.
Manuscript writing and revising: Stephanie May, Julian R Marchesi, Lee Parry.
Acknowledgments
The work was funded by Tenovus Cancer Care (PhD2013/L25), the World Cancer Research Fund (2013/1006), Rosetrees Foundation (CM401), Cancer Research UK Programme grant (C1295/ A15937)) and a philanthropic donation from Mr Lyndon and Mrs Shirley Ann Wood from the Moorhouse Group Ltd (LP). JRM and the Division of Integrative Systems Medicine and Digestive Disease at Imperial College London receives financial support from the National Institute of Health Research (NIHR) Imperial Biomedical Research Centre (BRC) based at Imperial College Healthcare NHS Trust and Imperial College London.
References
[1] | Yamagishi H , Kuroda H , Imai Y , Hiraishi H . Molecular pathogenesis of sporadic colorectal cancers. Chinese Journal of Cancer. (2016) ;35: :4. DOI: 10.1186/s40880-015-0066-y |
[2] | Ferlay J , Soerjomataram I , Dikshit R , Eser S , Mathers C , Rebelo M , et al. Cancer incidence and mortality worldwide: Sources, methods and major patterns in GLOBOCAN 2012. International Journal of Cancer. (2015) ;136: (5):E359–E86. DOI: 10.1002/ijc.29210 |
[3] | Axelrad JE , Lichtiger S , Yajnik V . Inflammatory bowel disease and cancer: The role of inflammation, immunosuppression, and cancer treatment. World Journal of Gastroenterology. (2016) ;22: (20):4794-801. DOI: 10.3748/wjg.v22.i20.4794 |
[4] | World Cancer Research Fund/American Institute for Cancer Research. Diet, Nutrition, Physical Activity and Cancer: A Global Perspective. Continuous Update Project Expert Report. A summary of the Third Expert Report 2018. ISBN 978-1-912259-47-2. Accessed on 29/11/2018. Available at www.wcrf.org/sites/default/files/Summary-third-expert-report.pdf |
[5] | Brown KF , Rumgay H , Dunlop C , Ryan M , Quartly F , Cox A , et al. The fraction of cancer attributable to modifiable risk factors in England, Wales, Scotland, Northern Ireland, and the United Kingdom in 2015. British Journal of Cancer. (2018) ;118: (8):1130–41. DOI: 10.1038/s41416-018-0029-6 |
[6] | Beyaz S , Mana MD , Roper J , Kedrin D , Saadatpour A , Hong S-J , et al. High-fat diet enhances stemness and tumorigenicity of intestinal progenitors. Nature. (2016) ;531: (7592):53–8. DOI: 10.1038/nature17173 |
[7] | Barker N , Ridgway RA , van Es JH , van de Wetering M , Begthel H , van den Born M , et al. Crypt stem cells as the cells-of-origin of intestinal cancer. Nature. (2009) ;457: (7229):608–11. DOI: 10.1038/nature07602 |
[8] | O’Keefe SJD , Li JV , Lahti L , Ou J , Carbonero F , Mohammed K , et al. Fat, fibre and cancer risk in African Americans and rural Africans. Nature Communications. (2015) ;6: :6342. DOI: 10.1038/ncomms7342 |
[9] | Alexander JL , Scott AJ , Pouncey AL , Marchesi J , Kinross J , Teare J . Colorectal carcinogenesis: An archetype of gut microbiota-host interaction. Ecancermedicalscience. (2018) ;12: :865. DOI: 10.3332/ecancer.2018.865 |
[10] | Cummings JH , Pomare EW , Branch WJ , Naylor CP , Macfarlane GT . Short chain fatty acids in human large intestine, portal, hepatic and venous blood. Gut. (1987) ;28: (10):1221–7. |
[11] | Wang L-S , Kuo C-T , Stoner K , Yearsley M , Oshima K , Yu J , et al. Dietary black raspberries modulate DNA methylation in dextran sodium sulfate (DSS)-induced ulcerative colitis. Carcinogenesis. (2013) ;34: (12):2842–50. DOI: 10.1093/carcin/bgt310 |
[12] | Bi X , Fang W , Wang L-S , Stoner GD , Yang W . Black Raspberries Inhibit Intestinal Tumorigenesis in Apc1638+/– and Muc2–/– Mouse Models of Colorectal Cancer. Cancer Prevention Research. (2010) ;3: :1443–50. DOI: 10.1158/1940-6207.CAPR-10-0124 |
[13] | Wang L-S , Arnold M , Huang Y-W , Sardo C , Seguin C , Martin E , et al. Modulation of Genetic and Epigenetic Biomarkers of Colorectal Cancer in Humans by Black Raspberries: A Phase I Pilot Study. Clinical Cancer Research. (2011) ;17: (3):598. DOI: 10.1158/1078-0432.CCR-10-1260 |
[14] | Wang L-S , Burke CA , Hasson H , Kuo C-T , Molmenti CLS , Seguin C , et al. A Phase Ib Study of the Effects of Black Raspberries on Rectal Polyps in Patients with Familial Adenomatous Polyposis. Cancer Prevention Research. (2014) ;7: (7):666. DOI: 10.1158/1940-6207.CAPR-14-0052 |
[15] | Wang L-S , Kuo C-T , Huang THM , Yearsley M , Oshima K , Stoner GD , et al. Black Raspberries Protectively Regulate Methylation of Wnt Pathway Genes in Precancerous Colon Tissue. Cancer prevention research (Philadelphia, Pa). (2013) ;6: (12):1317–27. DOI: 10.1158/1940-6207.CAPR-13-0077 |
[16] | Sonnenburg JL , Bäckhed F . Diet-microbiota interactions as moderators of human metabolism. Nature. (2016) ;535: (7610):56–64. DOI: 10.1038/nature18846 |
[17] | Turnbaugh PJ , Ridaura VK , Faith JJ , Rey FE , Knight R , Gordon JI . The effect of diet on the human gut microbiome: A metagenomic analysis in humanized gnotobiotic mice. Sci Transl Med. (2009) ;1: (6):6ra14. DOI: 10.1126/scitranslmed.3000322 |
[18] | De Filippo C , Cavalieri D , Di Paola M , Ramazzotti M , Poullet JB , Massart S , et al. Impact of diet in shaping gut microbiota revealed by a comparative study in children from Europe and rural Africa. Proc Natl Acad Sci U S A. (2010) ;107: (33):14691–6. DOI: 10.1073/pnas.1005963107 |
[19] | Clarke TB , Davis KM , Lysenko ES , Zhou AY , Yu Y , Weiser JN . Recognition of peptidoglycan from the microbiota by Nod1 enhances systemic innate immunity. Nat Med. (2010) ;16: (2):228–31. DOI: 10.1038/nm.2087 |
[20] | Wen L , Ley RE , Volchkov PY , Stranges PB , Avanesyan L , Stonebraker AC , et al. Innate immunity and intestinal microbiota in the development of Type 1 diabetes. Nature. (2008) ;455: (7216):1109–13. DOI: 10.1038/nature07336 |
[21] | Maslowski KM , Vieira AT , Ng A , Kranich J , Sierro F , Yu D , et al. Regulation of inflammatory responses by gut microbiota and chemoattractant receptor GPR43. Nature. (2009) ;461: (7268):1282–6. DOI: 10.1038/nature08530 |
[22] | Frank DN , St Amand AL , Feldman RA , Boedeker EC , Harpaz N , Pace NR . Molecular-phylogenetic characterization of microbial community imbalances in human inflammatory bowel diseases. Proceedings of the National Academy of Sciences of the United States of America. (2007) ;104: (34):13780–5. DOI: 10.1073/pnas.0706625104 |
[23] | Qin J , Li Y , Cai Z , Li S , Zhu J , Zhang F , et al. A metagenome-wide association study of gut microbiota in type 2 diabetes. Nature. (2012) ;490: :55. DOI: 10.1038/nature1145 |
[24] | Zhang H , DiBaise JK , Zuccolo A , Kudrna D , Braidotti M , Yu Y , et al. Human gut microbiota in obesity and after gastric bypass. Proceedings of the National Academy of Sciences of the United States of America. (2009) ;106: (7):2365–70. DOI: 10.1073/pnas.0812600106 |
[25] | Le Chatelier E , Nielsen T , Qin J , Prifti E , Hildebrand F , Falony G , et al. Richness of human gut microbiome correlates with metabolic markers. Nature. (2013) ;500: :541. DOI: 10.1038/nature12506 |
[26] | Bervoets L , Van Hoorenbeeck K , Kortleven I , Van Noten C , Hens N , Vael C , et al. Differences in gut microbiota composition between obese and lean children: A cross-sectional study. Gut Pathogens. (2013) ;5: (1):10. DOI: 10.1186/1757-4749-5-10 |
[27] | Ou J , Carbonero F , Zoetendal EG , DeLany JP , Wang M , Newton K , et al. Diet, microbiota, and microbial metabolites in colon cancer risk in rural Africans and African Americans. The American Journal of Clinical Nutrition. (2013) ;98: (1):111–20. DOI: 10.3945/ajcn.112.056689 |
[28] | Stoner GD . Foodstuffs for preventing cancer: The preclinical and clinical development of berries. Cancer Prev Res (Phila). (2009) ;2: (3):187–94. DOI: 10.1158/1940-6207.CAPR-08-0226 |
[29] | Barker N , van Es JH , Kuipers J , Kujala P , van den Born M , Cozijnsen M , et al. Identification of stem cells in small intestine and colon by marker gene Lgr5. Nature. (2007) ;449: (7165):1003–7. DOI: 10.1038/nature06196 |
[30] | Shibata H , Toyama K , Shioya H , Ito M , Hirota M , Hasegawa S , et al. Rapid colorectal adenoma formation initiated by conditional targeting of the Apc gene. Science. (1997) ;278: (5335):120–3. DOI: 10.1126/science.278.5335.120 |
[31] | MacIntyre DA , Chandiramani M , Lee YS , Kindinger L , Smith A , Angelopoulos N , et al. The vaginal microbiome during pregnancy and the postpartum period in a European population. Sci Rep. (2015) ;5: :8988. DOI: 10.1038/srep08988 |
[32] | Mitra A , MacIntyre DA , Mahajan V , Lee YS , Smith A , Marchesi JR , et al. Comparison of vaginal microbiota sampling techniques: Cytobrush versus swab. Sci Rep. (2017) ;7: (1):9802. DOI: 10.1038/s41598-017-09844-4 |
[33] | Parks DH , Tyson GW , Hugenholtz P , Beiko RG . STAMP: Statistical analysis of taxonomic and functional profiles. Bioinformatics. (2014) ;30: (21):3123–4. DOI: 10.1093/bioinformatics/btu494 |
[34] | Tu P , Bian X , Chi L , Gao B , Ru H , Knobloch TJ , et al. Characterization of the Functional Changes in Mouse Gut Microbiome Associated with Increased. ACS Omega. (2018) ;3: (9):10927–37. DOI: 10.1021/acsomega.8b00064 |
[35] | Steward WP , Brown K . Cancer chemoprevention: A rapidly evolving field. British Journal of Cancer. (2013) ;109: :1–7. DOI: 10.1038/bjc.2013.280 |
[36] | Sporn MB , Newton DL . Chemoprevention of cancer with retinoids. Fed Proc. (1979) ;38: (11):2528–34. DOI: 10.1016/0090-8258(81)90086-X |
[37] | Kaczmarek JL , Liu X , Charron CS , Novotny JA , Jeffery EH , Seifried HE , et al. Broccoli consumption affects the human gastrointestinal microbiota. The Journal of Nutritional Biochemistry. (2019) ;63: :27–34. DOI: 10.1016/j.jnutbio.2018.09.015 |
[38] | Chen L , Jiang B , Zhong C , Guo J , Zhang L , Mu T , et al. Chemoprevention of colorectal cancer by black raspberry anthocyanins involved the modulation of gut microbiota and SFRP2 demethylation. Carcinogenesis. (2018) ;39: (3):471–81. DOI: 10.1093/carcin/bgy009 |
[39] | Wang L-S , Kuo C-T , Cho S-J , Seguin C , Siddiqui J , Stoner K , et al. Black Raspberry-Derived Anthocyanins Demethylate Tumor Suppressor Genes Through the Inhibition of DNMT1 and DNMT3B in Colon Cancer Cells. Nutrition and Cancer. (2013) ;65: (1):118–25. DOI: 10.1080/01635581.2013.741759 |
[40] | Valdes AM , Walter J , Segal E , Spector TD . Role of the gut microbiota in nutrition and health. BMJ. (2018) ;361. DOI: 10.1136/bmj.k2179 |
[41] | Kostic AD , Chun E , Robertson L , Glickman JN , Gallini CA , Michaud M , et al. Fusobacterium nucleatum potentiates intestinal tumorigenesis and modulates the tumor-immune microenvironment. Cell Host & Microbe. (2013) ;14: (2):207–15. DOI: 10.1016/j.chom.2013.07.007 |
[42] | Castellarin M , Warren RL , Freeman JD , Dreolini L , Krzywinski M , Strauss J , et al. Fusobacterium nucleatum infection is prevalent in human colorectal carcinoma. Genome Research. (2012) ;22: (2):299–306. DOI: 10.1101/gr.126516.111 |
[43] | Pan P , Lam V , Salzman N , Huang Y-W , Yu J , Zhang J , et al. Black raspberries and their anthocyanin and fiber fractions alter the composition and diversity of gut microbiota in F-344 rats. Nutrition and Cancer. (2017) ;69: (6):943–51. DOI: 10.1080/01635581.2017.1340491 |
[44] | Tu P , Bian X , Chi L , Gao B , Ru H , Knobloch TJ , et al. Characterization of the Functional Changes in Mouse Gut Microbiome Associated with Increased Akkermansia muciniphila Population Modulated by Dietary Black Raspberries. ACS Omega. (2018) ;3: (9):10927–37. DOI: 10.1021/acsomega.8b00064 |
[45] | Friswell MK , Gika H , Stratford IJ , Theodoridis G , Telfer B , Wilson ID , et al. Site and strain-specific variation in gut microbiota profiles and metabolism in experimental mice. PloS One. (2010) ;5: (1):e8584–e. DOI: 10.1371/journal.pone.0008584 |
[46] | Laukens D , Brinkman BM , Raes J , De Vos M , Vandenabeele P . Heterogeneity of the gut microbiome in mice: Guidelines for optimizing experimental design. FEMS Microbiology Reviews. (2016) ;40: (1):117–32. DOI: 10.1093/femsre/fuv036 |
[47] | Turnbaugh PJ , Ley RE , Mahowald MA , Magrini V , Mardis ER , Gordon JI . An obesity-associated gut microbiome with increased capacity for energy harvest. Nature. (2006) ;444: :1027. DOI: 10.1038/nature05414 |
[48] | Hibberd AA , Lyra A , Ouwehand AC , Rolny P , Lindegren H , Cedgård L , et al. Intestinal microbiota is altered in patients with colon cancer and modified by probiotic intervention. BMJ Open Gastroenterology. (2017) ;4: (1). DOI: 10.1136/bmjgast-2017-000145 |
[49] | Wong SH , Kwong TNY , Chow T-C , Luk AKC , Dai RZW , Nakatsu G , et al. Quantitation of faecal Fusobacterium improves faecal immunochemical test in detecting advanced colorectal neoplasia. Gut. (2017) ;66: (8):1441. DOI: 10.1136/gutjnl-2016-312766 |
[50] | Shen XJ , Rawls JF , Randall T , Burcal L , Mpande CN , Jenkins N , et al. Molecular characterization of mucosal adherent bacteria and associations with colorectal adenomas. Gut Microbes. (2010) ;1: (3):138–47. DOI: 10.4161/gmic.1.3.12360 |
[51] | Sobhani I , Tap J , Roudot-Thoraval F , Roperch JP , Letulle S , Langella P , et al. Microbial dysbiosis in colorectal cancer (CRC) patients. PloS one. (2011) ;6: (1):e16393–e. DOI: 10.1371/journal.pone.0016393 |
[52] | Jackstadt R , Sansom OJ . Mouse models of intestinal cancer. The Journal of Pathology. (2016) ;238: (2):141–51. DOI: 10.1002/path.4645 |
[53] | Nguyen TLA , Vieira-Silva S , Liston A , Raes J . How informative is the mouse for human gut microbiota research? Disease Models & Mechanisms. (2015) ;8: (1):1–16. DOI: 10.1242/dmm.017400 |
[54] | Donohoe DR , Holley D , Collins LB , Montgomery SA , Whitmore AC , Hillhouse A , et al. A gnotobiotic mouse model demonstrates that dietary fiber protects against colorectal tumorigenesis in a microbiota- and butyrate-dependent manner. Cancer Discov. (2014) ;4: (12):1387–97. DOI: 10.1158/2159-8290.CD-14-0501 |
[55] | Landy J , Ronde E , English N , Clark SK , Hart AL , Knight SC , et al. Tight junctions in inflammatory bowel diseases and inflammatory bowel disease associated colorectal cancer. World Journal of Gastroenterology. (2016) ;22: (11):3117–26. DOI: 10.3748/wjg.v22.i11.3117 |
[56] | Hatayama H , Iwashita J , Kuwajima A , Abe T . The short chain fatty acid, butyrate, stimulates MUC2 mucin production in the human colon cancer cell line, LS174T. Biochemical and Biophysical Research Communications. (2007) ;356: (3):599–603. DOI: 10.1016/j.bbrc.2007.03.025 |
[57] | Peng L , Li Z-R , Green RS , Holzman IR , Lin J . Butyrate enhances the intestinal barrier by facilitating tight junction assembly via activation of AMP-activated protein kinase in Caco-2 cell monolayers. The Journal of Nutrition. (2009) ;139: (9):1619–25. DOI: 10.3945/jn.109.104638 |
[58] | Grivennikov SI , Wang K , Mucida D , Stewart CA , Schnabl B , Jauch D , et al. Adenoma-linked barrier defects and microbial products drive IL-23/IL-17-mediated tumour growth. Nature. (2012) ;491: (7423):254–8. DOI: 10.1038/nature11465 |
[59] | Peiffer DS , Wang L-S , Zimmerman NP , Ransom BWS , Carmella SG , Kuo C-T , et al. Dietary Consumption of Black Raspberries or Their Anthocyanin Constituents Alters Innate Immune Cell Trafficking in Esophageal Cancer. Cancer Immunology Research. (2016) ;4: (1):72. DOI: 10.1158/2326-6066.CIR-15-0091 |
[60] | Pan P , Kang S , Wang Y , Liu K , Oshima K , Huang Y-W , et al. Black Raspberries Enhance Natural Killer Cell Infiltration into the Colon and Suppress the Progression of Colorectal Cancer. Frontiers in Immunology. (2017) ;8: :997. DOI: 10.3389/fimmu.2017.00997 |
[61] | Takeuchi Y , Nishikawa H . Roles of regulatory T cells in cancer immunity. International Immunology. (2016) ;28: (8):401–9. DOI: 10.1093/intimm/dxw025 |
[62] | Chaudhry A , Samstein RM , Treuting P , Liang Y , Pils MC , Heinrich J-M , et al. Interleukin-10 signaling in regulatory T cells is required for suppression of Th17 cell-mediated inflammation. Immunity. (2011) ;34: (4):566–78. DOI: 10.1016/j.immuni.2011.03.018 |