Profiling the phenolic acids, flavonoids and tannins in skunk currants (Ribes glandulosum) of Northern Québec, Canada
Abstract
BACKGROUND:
Skunk currant is widely dispersed across North America and a feature of some traditional North American indigenous diets. Whereas many wild and cultivated berries have attracting interest related to their antioxidant phenolic metabolites and putative health benefits in humans, very few data are available concerning skunk currant phytochemistry.
OBJECTIVE:
Provide the first metabolic profile of skunk currant fruits with a focus on phenolic and polyphenolic compounds, owing to their emerging implications in human health.
METHODS:
Skunk currants were harvested in Nunavik, Québec. Flavonols, flavan-3-ols, and phenolic acids were characterized using a targeted approach with reverse-phase ultra-high pressure liquid chromatography coupled with tandem mass spectrometry. Ellagitannins and anthocyanins were measured using reverse-phase HPLC following acid hydrolysis and employing diode array detection. Proanthocyanidins and sugars were detected with normal-phase HPLC.
RESULTS:
A total of 11 phenolic acids and 11 flavonoids, including three cyanidins and three quercetin glycosides were identified. Both condensed (proanthocyanidins) and hydrolysable (ellagitannins) tannins were also detected at 162 mg and 75 mg per 100 g extract, respectively. The cumulative amount of detected phenolic and polyphenolic metabolites totaled 622.6 mg/100 g extract (63.4 mg/100 g berry FW).
CONCLUSIONS:
Skunk currant is a source of many bioactive phenolic and polyphenolic compounds. Appearing richer in phenolics than some cultivated varieties, the wild northern varieties of North America warrant additional study.
1Introduction
Ribes glandulosum, known commonly as skunk currant or hairy berry, is widely dispersed across all ten provinces and three territories of Canada as well as parts of the United States. Despite the subjectively unpleasant odour of ripe berries that gives the plant its name, R. glandulosum keeps company among other wild berries in the traditional diet of several North American Indigenous peoples. Relative to most other Ribes species within its range, however, both ethnobotanical records and scientific study of skunk currant and its uses are sparse. While raw Ribes species were sometimes consumed by the Algonquin in southwestern Quebec and the Woods Cree (Nihithawak) in east-central Saskatchewan [1], the consumption of skunk currants appears most common among the Inuit and First Nations of northern Québec and Labrador. In Nunavik, Quebec, Inuit call the fruits mirqualik (mirquq means “hairy” in Inuktitut) and consider them edible, nutritious and – according to some – delicious [2]. In Nain, the fruits (mikulik) are eaten raw and made into jam [2]. Referring to the plant as wopeoaminanatuk, the Cree of Eeyou Istchee consider the fruits edible but not highly valued. The limited reports of skunk currant consumption in southern regions may reflect the increased diversity of wild fruits in warmer, less harsh climates, but may also reflect cultural preferences. Although the fruits have no reported traditional medicinal applications, a compound decoction of the roots was used for back pain and “female weakness” and a decoction of the stems was used to prevent blood clotting after birth [3, 4].
While little is known about the chemistry or potential health benefits of skunk currant, a growing body of scientific evidence supports the protective effects of berry phytochemicals, particularly phenolic metabolites, against age-related chronic diseases [5]. For example, epidemiological data reveals that the consumption of strawberries, blueberries, and/or flavonoids correlates with reduced risk of myocardial infarction, type 2 diabetes, and cognitive decline [6– 8]. Only one study has investigated the chemistry of R. glandulosum fruits to date. Analysing the sugar, organic acid, and total phenolic content of 25 berry species grown in Slovenia, Mikulic-Petkovsek et al. [9] reported that cultivated “white currants” (R. glandulosum) were among the lowest in terms of total sugars and total phenolics yet relatively rich in total organic acid content. North American skunk currants, however, are red – indicative of anthocyanins linked specifically to the health promoting benefits of berries [7, 8, 10] – and, in northern Québec, grow in high UV conditions known to activate phenolic biosynthesis [11]. Indeed, in a study comparing the total phenolics as well as antiglycation and antioxidant activities of 12 berry species from Northern Québec, skunk currant ranked in the top five for most measures, and scored significantly better than the closely related R. triste [12]. As such, the chemistry of wild North American R. glandulosum likely differs substantially from European cultivars.
The present work provides a targeted characterization of phenolic metabolites in skunk currant fruits harvested in Nunavik, Northern Québec. Focusing on compounds believed to contribute positively to human health, we profiled phenolic acids, flavonols, anthocyanins, ellagitannins and proanthocyanidins, as well as sugars. We aimed to report both well-established and emerging compounds of interest to human health as well as contribute to the growing body of evidence supporting the value of traditional foods and traditional indigenous diets in the management and prevention of chronic illnesses.
2Materials and methods
2.1Chemical standards and reagents
Analytical standards and reagents were purchased from Sigma-Aldrich (St. Louis, MO, USA), except cyanidin 3-glucoside, which was purchased from Extrasynthèse (Genay, France). Liquid chromatography grade solvents and acids were purchased from EMD Millipore Chemicals (Billerica, MA, USA) and Anachemia (Montreal, QC, Canada), respectively.
2.2Plant materials and extraction
The fruits of R. glandulosum were harvested in September 2012 near Kangiqsualujjuaq, on the east coast of Ungava Bay at the mouth of the George River, in Nunavik, Québec. The berries were firm, bright red, and covered with small glandular hairs. After harvest, the samples were stored (– 20°C) and transported (< – 4°C) frozen until experimental use.
Frozen berries (20 g) were extracted overnight with 30 mL of ethanol, under agitation. After centrifugation (4000 rpm, 5 min at 4°C), the supernatant was removed and refrigerated while the pellet was resuspended in 20 mL of ethanol, then extracted and centrifuged again. The pooled supernatants were filtered using Whatman 1 filter paper, concentrated by rotoevaporation then lyophilized.
2.3Phytochemical analyses
For more detailed reports of the methods described below, please refer to previous publications [13, 14]. In addition to total phenolic content as measured using the Folin-Ciocalteu method, we report the individual and cumulative concentrations of all identified compounds across the five classes of phenolics (phenolic acid, flavonols & flavan-3-ols, anthocyanins, proanthocyanidins, and ellagitannins).
2.3.1Total phenolic content
Total phenolic content (TPC) in fruit extract was measured via the Folin-Ciocalteu method using gallic acid as standard. Extract solutions (20μl in 20% methanol 0.1% trifluoroacetic acid [TFA]) were mixed with 100μl of 10-fold diluted Folin-Ciocalteu reagent and 80μl of sodium carbonate solution (75 g/l). The absorbance was measured at 765 nm after 1 h of incubation at room temperature using a BMG Labtech Fluostar Omega microplate reader (Offenburg, Germany). TPC values are expressed as gallic acid equivalents (GAE) per unit sample weight.
2.3.2Phenolic acids, flavonols & flavan-3-ols
Selected phenolic acids, flavonols, and flavan-3-ols were characterized in fruit extract by reverse-phase ultra-high pressure liquid chromatography with coupled tandem mass spectrometry (UHPLC-MS/MS) with negative ionization and using a targeted library of standards, as previously described [13]. This approach allows for rapid separation, detection and identification of most flavonoids, including glycosides most commonly found in berries. Compounds were quantified relative to high-purity reference standards, when available. Chlorogenic acid (5-caffeoylquinic acid, 5-CQA) was used to quantify its 3- and 4-CQA isomers. Glycosylated flavonols and phenolic acids were quantified based on their corresponding aglycones (kaempferol or quercetin and coumaric or caffeic acid, respectively).
2.3.3Anthocyanins
Requiring low pH to achieve good peak resolution and separation, anthocyanins were characterized independently of related flavonoids using reverse-phase HPLC with diode array detection (DAD) and data acquisition at 520 nm wavelength [13]. The compounds were identified based on their retention time and quantified (at 520 nm) using cyanidin 3-glucoside.
2.3.4Proanthocyanidins
As described by Dudonné et al. [13] proanthocyanidins were analyzed by normal-phase HPLC with fluorescence detection at 230 nm and 321 nm excitation and emission wavelengths, respectively. Separating proanthocyanidins according to size (monomers, dimers, trimers, etc.) rather than polarity, we quantified proanthocyanidins relative to injected (–)-epicatechin reference standard, then applied a correction factor based on fluorescence response of each degree of polymerization (DP; from 1 to >10) [13]. Results reflect the total proanthocyanidin content but not the types of monomer or linkage patterns. Note that the flavan-3-ols (–)-epicatechin and (+)-catechin, detected as described in section 2.3.2, make up the majority (but not all) of proanthocyanidin monomers.
2.3.5Total ellagitannins
The structural complexity and diversity of ellagitannins put their individual characterization beyond the scope of this study. Accordingly, total ellagitannins content of fruit extract was quantified as free ellagic acid, following acid hydrolysis, using reverse-phase HPLC as described by Dudonné et al. [13]. Briefly, the extract was solubilized in 50% methanol containing 10 mM ascorbic acid and 1.2 M hydrochloric acid incubated in darkness for 2 h at 85°C. Chromatographic data were acquired at 250 nm and ellagic acid was used for quantification.
2.4Sugar analysis
Berry extract was dissolved in 80% ethanol, vortexed for 2 min then heated for 10 min at 70°C. After centrifugation (3000 rpm for 5 min), an aliquot of the supernatant was evaporated. The pellet was resuspended in ultrapure water, filtered and analyzed by HPLC using a refractometer as detector. External glucose and fructose standards were used to quantify their respective concentrations.
2.5Antioxidant activity
Skunk currant extract was evaluated using the Oxygen Radical Absorbing Capacity (ORAC) assay following established protocols (Gillespie et al., 2007; Harris et al., 2011). In 96-well black clear-bottom microplates, 0.08μM fluorescein was added to 75 mM PBS (blank), Trolox standard (6.25– 50μM) or berry extract (0– 25 mg/mL) and allowed to equilibrate for 10 minutes at 37°C. 2,2-azobis(2-methylpropionamidine) dihydrochloride (AAPH) (150 mM) was then added to each well with fluorescence subsequently monitored at excitation and emission wavelengths of 485 nm and 530 nm, respectively, every 3 minutes for 90 minutes. Final results were calculated as μM Trolox equivalents (TE) per 100 g fresh fruit.
2.6Statistical analysis
Differences in quantified levels of phenolic acids were analyzed by ANOVA with Tukey HSD post hoc analysis using GraphPad Prism 7 (GraphPad Software, La Jolla, CA). Berry extracts were tested in triplicate (n = 3) with a significance level of 95% (p < 0.05).
3Results
Fresh skunk currants were approximately 86% water, by weight. Extract yield was 71.5% relative to dry powdered fruits and 10.2% relative to fresh weight. In total, we identified several phenolic acids and flavonoids, quantified proanthocyanidins with DP ranging from 1 to 7 and estimated the total ellagitannin content. TPC was measured by Folin-Ciocalteu method and the value was 35.9±0.1 mg GAE/g extract (365 mg GAE/100 g fresh material). The Antioxidant Radical Absorbance Capacity (ORAC) value of skunk current extract was determined to be 2108±49 TE per 100 g fresh fruit.
Table 1 presents the cumulative amounts of the individually quantified phenolics according to structural class: phenolic acids, ellagitannins, proanthocyanidins, anthocyanins, and flavonols. Among the identified compounds, we characterized primarily phenolic acids, followed by flavonols. Ellagitannins and anthocyanins were the least abundant phenolic compounds detected in skunk currant (Table 1).
Table 1
Overall phenolic composition of R. glandulosum.
Total phenolic content | Anthocyanins | Flavonoids | Phenolic acids | Proanthocyanidins | Ellagitannins | |
mg/100 g extract | 3589±42.3 | 59.6±0.01 | 143±5.24 | 226±5.50 | 163±2.09 | 74.3±3.98 |
mg/100 g fresh fruit | 365.1±4.31 | 6.07±0.01 | 14.6±0.53 | 23.0±0.56 | 16.5±0.21 | 7.56±0.40 |
The total phenolic content estimated by Folin-Ciocalteu assay is presented along with the total amounts of each identified compound within the targeted classes of (poly)phenolics (mean±SEM, n = 3).
3.1Phenolic acids
Identified phenolic acids cumulatively amounted to 226 mg/100 g of berry extract or 23.0 mg/100 g FW. Shikimic acid is by far the most abundant phenolic acid; it is present in quantities nearly five-fold greater than the next most abundant phenolic acid, 5-caffeoylquinic acid (chlorogenic acid) (Fig. 1). Para-coumaric acid, caffeic acid, 4-hydroxybenzoic acid and 3,4-dihydroxybenzoic acid were detected in quantities less than 1 mg/100 g extract.
Fig.1
Phenolic acid composition of R. glandulosum (mg acid/100 g extract). The seven most abundant phenolic acids are represented with the measured content per 100 g of extract (mean±SEM, n = 3). Shikimic acid is significantly more abundant than all other phenolic acids (* = p < 0.05, ANOVA post hoc Tukey HSD). The remaining phenolic acids detected were present in quantities less than 1 mg/100 g extract; these acids include 4-hydroxybenzoic acid, p-coumaric acid, caffeic acid, and 3,4-dihydroxybenzoic acid.
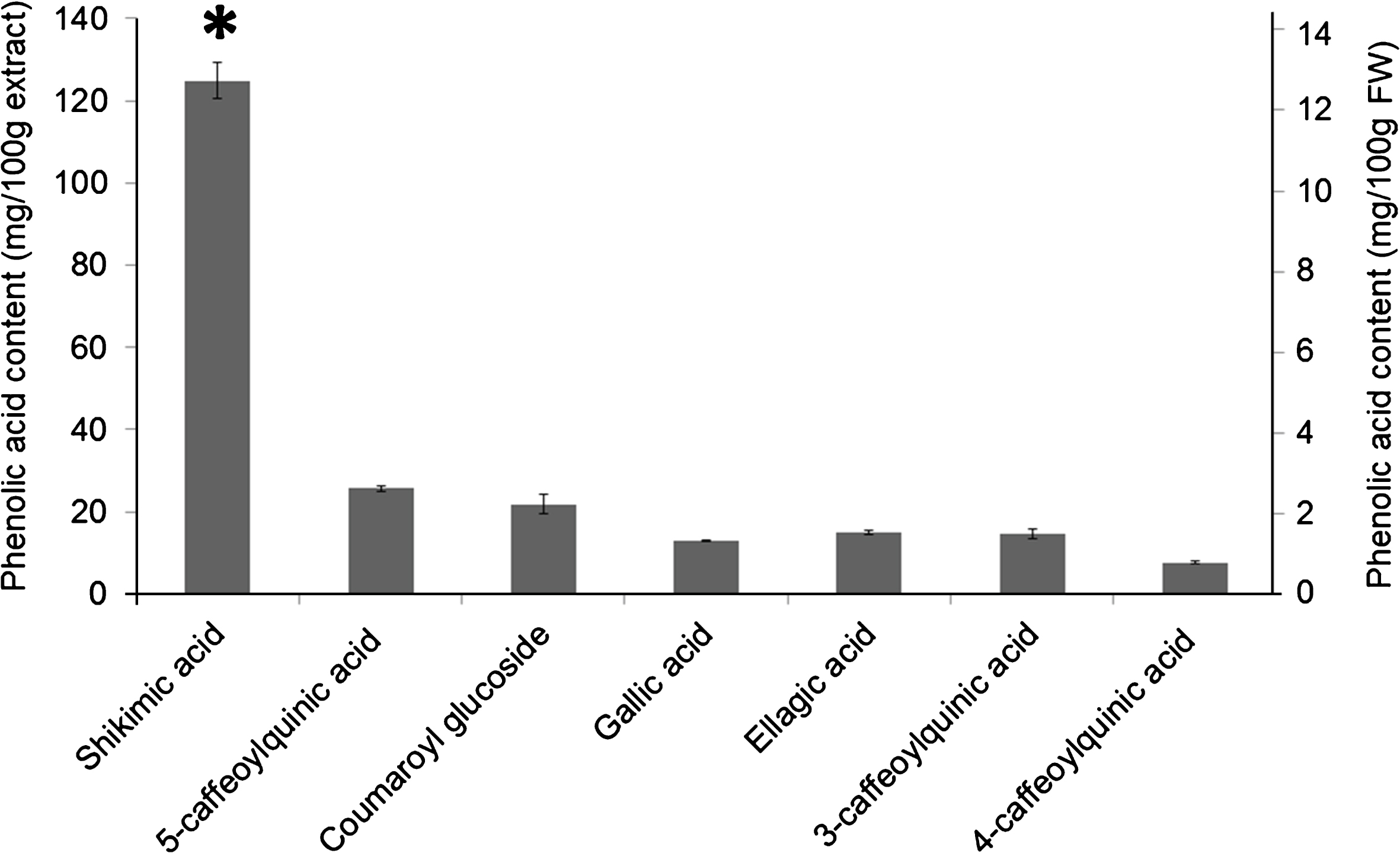
3.2Flavonoids (including anthocyanins)
Flavonols, primarily quercetin and kaempferol glycosides, and flavan-3-ols make up 0.14% of the total extract. Phlorizin, a dihydrochalcone glycoside, was detected in very small amounts. R. glandulosum fruits also contained 59.59 mg of anthocyanins/100 g extract. Cyanidin 3-glucoside is the most abundant anthocyanin, making up 87% of the total anthocyanin content, followed by cyanidin 3-rutinoside (11.8%) and small amounts of an unidentified cyanidin derivative (Table 2).
Table 2
Detailed composition of targeted phenolic classes.
CAS # | Detection criteria* | mg/100 g extract | mg/100 g fresh fruit | |
Flavonols | ||||
Quercetin | 117-39-5 | 301 m/z | <1 | <0.1 |
Quercetin-galactoside | 482-36-0 | 463/301 m/z | 41.0±1.44 | 4.17±0.15 |
Quercetin-rhamnoside | 522-12-3 | 447/301 m/z | 15.4±0.78 | 1.57±0.08 |
Kaempferol-glucoside | 520-18-3 | 447/285 m/z | 38.3±0.57 | 3.90±0.06 |
Rutin | 153-18-4 | 609/463/301 m/z | 4.21±0.79 | 0.428±0.08 |
Dihydrochalcones | ||||
Phlorizin | 60-81-1 | 435/273 | <1 | <0.1 |
Anthocyanins | ||||
Not identified | 520 nm | 0.60±0.06 | 0.067±0.01 | |
Cyanidin 3-glucoside | 7084-24-4 | 520 nm | 52.0±0.20 | 5.82±0.02 |
Cyanidin 3-rutinoside | 18719-76-1 | 520 nm | 7.02±0.13 | 0.786±0.01 |
Proanthocyanidins | ||||
Monomers (flavan-3-ols) | 230/321 nm | 56.1±12.7 | 5.71±1.29 | |
(+)-Catechin** | 154-23-4 | 289 m/z | (33.1±3.06) | (3.37±0.31) |
(–)-Epicatechin** | 490-46-0 | 289 m/z | (10.2±1.83) | (1.04±0.19) |
Dimers | 230/321 nm | 57.4±16.0 | 5.84±1.62 | |
Trimers | 230/321 nm | 20.8±0.97 | 2.12±0.10 | |
Tetramers | 230/321 nm | 12.2±1.43 | 1.24±0.15 | |
Pentamers | 230/321 nm | 7.56±0.95 | 0.77±0.10 | |
Hexamers | 230/321 nm | 4.36±1.54 | 0.44±0.16 | |
Heptamers | 230/321 nm | 1.93±0.86 | 0.20±0.09 | |
Octamers | 230/321 nm | <1 | <0.1 | |
Nonamers | 230/321 nm | <1 | <0.1 | |
Decamers | 230/321 nm | <1 | <0.1 | |
Polymers (DP>10) | 230/321 nm | ND | ND |
Anthocyanins, flavonoids, phenolic acids, proanthocyanidins, and ellagitannins are divided into subclasses (where applicable) and presented with individual compounds’ measured quantity in extract and in fresh fruit (mean±SEM, n = 3) * Compound detection and identification based on retention time and peak wavelength of visible light absorption (nm, anthocyanins), fluorescence excitation and emission wavelengths (nm, proanthocyanidins) or mass charge ratio (m/z, flavonols, flavan-3-ols), relative to reference standards. ** The flavan-3-ols (+)-catechin and (–)-epicatechin are the most common procyanidin monomers; their respective levels (in brackets) contribute to the total proanthocyanidin monomer level.
3.3Tannins
Our analyses revealed both proanthocyanidins (condensed tannins) and ellagitannins (hydrolysable tannins). In total, skunk currant extract contained 162.56±2.09 mg of proanthocyanidins/100 g of extract (Table 2). Monomers and dimers make up 69.8% of skunk currant PACs. Like most berries, there is a pronounced trend of decreasing occurrence as the degree of polymerization increases. Trimers account for a further 12.8% of the proanthocyanidins content, and tetramers, pentamers, and hexamers collective account for another 14.8%. This leaves less than 3% of all the PACs as higher complexity polymers.
Based on ellagic acid residues freed through acid hydrolysis, approximately 74.3±3.98 mg of ellagitannins were quantified per 100 g extract.
3.4Sugars
With no available data for on North American skunk currants, we also measured sugar content. The extract contained 40.3±4.0 g of sugars/100 g of extract (4.1±0.4 g/100 g FW). Following hydrolysis, 16.5±0.2 g of this total were found to be glucose and the remaining 23.8±3.8 g were characterized as fructose. No other monosaccharides were detected.
4Discussion
A variety of berries and small fruits are linked to positive modulation of human health, especially with respect to metabolic syndrome. There is a strong body of evidence supporting the beneficial effects of berries with regard to regulating inflammation, LDL-cholesterol, insulin resistance and lipid metabolism [15– 18]. These bioactivities are typically attributed to the complex phenolic makeup of berries, including anthocyanins and other flavonoids as well as proanthocyanidins [5, 18]. Skunk currants grow much further North than other North American Ribes species, where they contribute more commonly to traditional indigenous diets and from where we collected our samples. Our data demonstrate that, like other berries in the Canadian North, R. glandulosum fruits contain a range of bioactive phenolic metabolites. However, relative to other edible northern berries such as black crowberries (Empetrum nigrum) and lingonberries (Vaccinium vitis-idaea), they are generally less rich in proanthocyanidins and anthocyanins [14]. Ellagitannin content in skunk currants (7.56 mg/100 mg fresh fruit) is greater than most northern berries by a factor of 10 or higher but is still less than half of levels reported in alpine bearberry (19.4 mg ellagitannin/100 mg FW) [14]. Ellagitannins are an emerging class of interest in bioactive phytochemicals, particularly as anti-inflammatory agents and positive modulators of gut microbiota [19, 20]. Further investigation will reveal if skunk currant elicits similar effects in vivo.
Although very little other published data are available, two studies report limited results on the phytochemistry of R. glandulosum: one comparing the TPC, phenolic acids and sugars of a cultivated variety (“white currants”) among 24 other types of European berries [9], and the other comparing TPC and various measures of radical scavenging activity of wild skunk currant among 12 North American berry species [12]. While our TPC results (by Folin-Ciocalteu method) are comparable with the North American data, the white currant cultivar contained 40.8 mg GAE/100 mg in fresh weight (0.0408% TPC), approximately nine-fold fewer phenolics than reported here (0.365% TPC). Our samples also contained more sugars, nearly 9X more glucose and 12X more fructose/100 g fresh weight [9].
Our results also differed from white currants in terms of shikimic acid content, which was more than four times more abundant in currants from northern Québec yet the principle phenolic acid reported in both studies [9]. In skunk currants, shikimic acid levels were 2.6 times higher (by weight) than all the chlorogenic acid derivates combined, and considerably higher than those of many other northern berries [14]. Note that our analytical methods were not optimized for detecting the organic acids reported in white currant by Mikulic-Petkovsek et al. [9], which may have been present yet not detected.
These differences in reported phytochemistry may be attributed to numerous factors, including methodological limitations, but most notably genetic and environmental differences between the colourless cultivar and wild-harvested R. glandulosum. Variation in these characteristics between cultivars and growing regions is well-documented, for example between cultivars of blueberries and between black currant varieties local to specific regions of Sweden, which demonstrated a 2-fold difference in total phenols [21, 22]. The limited sampling for this study (one collection site) unquestionably underestimated the variability, and most likely the diversity, of wild skunk currant phytochemistry across its North American range. Analysing other parts of R. glandulosum, as well as fruits from other regions at other times, may reveal a wider variety or greater abundance of target metabolites such as shikimic acid.
Shikimic acid is the precursor in the commercial synthesis of oseltamivir phosphate, marketed under the brand name Tamiflu, one of very few neuraminidase inhibitors that reduce the severity of the avian flu H5N1 [23]. Presently, shikimic acid is extracted from the seeds of Illicium verum, Chinese star anise, whose harvest is subject to weather conditions and requires six years to grow to fruiting stage [24]. Although efforts are underway to produce shikimic acid via fermentation with recombinant microorganisms, none of these alternative methods have yet proved commercially viable. Shikimic acid is considerably less abundant in skunk currants than in Illicium verum, at approximately 0.1% w/w whereas star anise is approximately 8% shikimic acid w/w. However, the wide availability and greater palatability of R. glandulosum compared to Illicium verum may make it a viable dietary supplement, if not a supplemental target source for the world supply of oseltamivir should other ecotypes or plant parts prove more productive than the collected northern fruits.
Several of the identified polyphenolics also offer potential benefits to human health, though less likely as a precursor for drug synthesis. For example, the cyanidin, quercetin, and caffeic acid derivates found in skunk currants have been identified as the active antiglycation agents in other berries and medicinal plants [12, 26, 27] and – together with the tannins – contribute to the reported ORAC value, which compare with those of other red currants, red and black grapes, as well as apples and oranges [28]. In addition to radical scavenging capacity, these molecules – as well as other structurally related anthocyanins, flavonols, flavonals and polymeric ellagitannins and procyanidins – elicit multiple protective effects (e.g. anti-inflammatory, neuroprotective, cardioprotective) in experimental models and even human studies [6, 8, 29– 31].
5Conclusions
The presented research provides a thorough profile of phenolic compounds of interest to human health identified in R. glandulosum fruit. Based on recent studies on other northern berries with overlapping chemistry, these fruits warrant study in experimental models of metabolic disease [20, 30]. The plant also deserves attention as a potential source of shikimic acid. Reporting low sugar content, our results begin to fill the remaining knowledge gap regarding the nutritional value of skunk currants and complement the broader objective of supporting traditional foods as a tool in preventing and managing chronic illnesses among North American Indigenous peoples.
Acknowledgments
We thank the Inuit of Nunavik for welcoming us into their communities and sharing their knowledge and experiences around berries with ML, AC & CH. Financial support was provided by an ArcticNet grant to ML (2011– 2015) and a Natural Sciences and Engineering Research Council of Canada grant to CSH. We also want to thank and recognize the late Dr Eric Dewailly, a major contributor to research on Inuit populations’ health, the Principal Investigator of the ArcticNet project, and a mentor to the team.
REFERENCES
[1] | Black MJ . Algonquin Ethnobotany: An Interpretation of Aboriginal Adaptation in South Western Quebec. Ottawa. National Museums of Canada; (1980) . |
[2] | Cuerrier A . the Elders of Kangiqsualujjuaq. The botanical knowledge of the Inuit of Kangiqsualujjuaq. Nunavik. Avataq Cultural Institute; (2012) . |
[3] | Leighton AL . Wild Plant Use by theWoods Cree (Nihithawak) of East-Central Saskatchewan. Ottawa. National Museums of Canada; (1985) . |
[4] | Densmore F . Uses of plants by the Chippewa Indians, Washington. U.S. G.P.O.; (1928) . |
[5] | Paredes-López O , Cervantes-Ceja ML , Vigna-Pérez M , Hernández-Pérez T . Berries: Improving human health and healthy aging, and promoting quality life – A review. Plant Foods Hum Nutr. (2010) ;65: (3):299–308. doi: 10.1007/s11130-010-0177-1 |
[6] | Devore EE , Kang JH , Breteler MMB , Grodstein F . Dietary intakes of berries and flavonoids in relation to cognitive decline. Ann Neurol. (2012) ;72: (1):135–43. doi: 10.1002/ana.23594 |
[7] | Wedick NM , Pan A , Cassidy A , Rimm EB , Sampson L , Rosner B , Willett W , Hu FB , Sun Q , van Dam RM . Dietary flavonoid intakes and risk of type 2 diabetes in US men and women. Am J Clin Nutr. (2012) ;95: , 925–33. doi: 10.3945/ajcn.111.028894 |
[8] | Blumberg JB , Camesano TA , Cassidy A , Kris-Etherton P , Howell A , Manach C , Ostertag LM , Sies H , Skulas-Ray A , Vita JA . Cranberries and their bioactive constituents in human health. Adv Nutr. (2013) ;4: , 618–32. doi: 10.3945/an.113.004473 |
[9] | Mikulic-Petkovsek M , Schmitzer V , Slatnar A , Stampar F , Veberic R . Composition of sugars, organic acids, and total phenolics in 25 wild or cultivated berry species. J Food Sci.. (2012) ;77: (10):C1064–C70. doi: 10.1111/j.1750-3841.2012.02896.x |
[10] | Kellogg J , Wang J , Flint C , Ribnicky D , Kuhn P , De Mejia EG , Raskin I , Lila MA . Alaskan wild berry resources and human health under the cloud of climate change. J Agric Food Chem. (2010) ;58: (7):3884–3900. doi: 10.1021/jf902693r |
[11] | Hahlbrock K , Scheel D . Physiology and molecular biology of phenylpropanoid metabolism. Annu Rev Plant Biol. (1989) ;40: (1):347–69. |
[12] | Harris CS , Cuerrier A , Lamont E , Haddad PS , Arnason JT , Bennett SAL , Johns T . Investigating wild berries as a dietary approach to reducing the formation of advanced glycation endproducts: Chemical correlates of in vitro antiglycation activity. Plant Foods Hum Nutr. (2014) ;69: (1):71–7. doi: 10.1007/s11130-014-0403-3 |
[13] | Dudonné S , Dubé P , Pilon G , Marette A , Jacques H , Weisnagel J , Desjardins Y . Modulation of strawberry/cranberry phenolic compounds glucuronidation by co-supplementation with onion: Characterization of phenolic metabolites in rat plasma using an optimized μSPE– UHPLC-MS/MS method. J Agric Food Chem. (2014) ;62: (14):3244–56. doi: 10.1021/jf404965z |
[14] | Dudonné S , Dubé P , Anhê FF , Pilon G , Marette A , Lucas M , Lemire M , Harris CS , Dewailly E , Desjardins Y . Comprehensive analysis of phenolic compounds and abscisic acid profiles of twelve native Canadian berries. J Food Compos Anal. (2015) ;44: , 214–24. doi: 10.1016/j.jfca.2015.09.003 |
[15] | LilaMA . Impact of bioflavonoids from berryfruits on biomarkers of metabolic syndrome. Func Foods Health Dis. (2011) ;2: , 13–24. |
[16] | Basu A , Lyons TJ . Strawberries, blueberries, and cranberries in the metabolic syndrome: Clinical perspectives. J Agric Food Chem. (2012) ;60: (23):5687–92. doi: 10.1021/jf203488k |
[17] | Burns Kraft TF , Dey M , Rogers RB , Ribnicky DM , Gipp DM , Cefalu WT , Raskin I , Lila MA . Phytochemical composition and metabolic performance-enhancing activity of dietary berries traditionally used by Native North Americans. J Agric Food Chem. (2008) ;56: (3):654–60. doi: 10.1021/jf071999d |
[18] | Lila A , Kellogg J , Grace M , Yousef GG , Burns Kraft TF , Rogers RB . Stressed for success: How the berry’s wild origins result in multifaceted health protections. Acta Hortic. (1017) )23–43. doi: 10.17660/ActaHortic.2014.1017.1 |
[19] | Etxeberria U , Fernández-Quintela A , Milagro FI , Aguirre L , Alfredo Martínez J , Portillo MP . Impact of polyphenols and polyphenol-rich dietary sources on gut microbiota composition. J Agric Food Chem.. (2013) ;61: (40):9517–33. doi: 10.1021/jf402506c |
[20] | Fernando AF , Varin TV , Le Barz M , Pilon G , Dudonné S , Trottier J , St-Pierre P , Harris CS , Lucas M , Lemire M , Dewailly É , Barbier O , Desjardins Y , Roy D , Marette A . Arctic berry extracts target the gut-liver axis to alleviate metabolic endotoxemia, insulin resistance and hepatic steatosis in diet-induced obese mice. Diabetologia. (2017) . Epub, doi: 10.1007/s00125-017-4520-z |
[21] | Scalzo J , Miller S , Edwards C , Meekings J , Alspach P . Variation in phytochemical composition and fruit traits of blueberry cultivars and advanced breeding selections in New Zealand. Acta Hortic. (2009) ;810: , 823–30. doi: 10.17660/ActaHortic.2009.810.109 |
[22] | Vagiri M , Rumpunen K , Johansson E . Quality traits in black currant (Ribes nigrum L.) as affected by genotype and location. Acta Hortic. (1117) )207–12. doi: 10.17660/ActaHortic.2016.1117.33 |
[23] | Centers for Disease Control and Prevention (US). Information on avian influenza [Internet]. Atlanta: Centers for Disease Control and Prevention; 2017 Apr 13 [cited 2017 Aug 9]. Available from: https://www.cdc.gov/flu/avianflu/ |
[24] | Ghosh S , Chisti Y , Banerjee UC . Production of shikimic acid. Biotechnol Adv. (2012) ;30: (6):1425–31. doi: 10.1016/j.biotechadv.2012.03.001 |
[25] | Liu H , Li Q , Zhang Y , Zhou Y . Analysis of (–)-shikimic acid in chinese star anise by GC– MS with selected ion monitoring. Chromatographia. (2009) ;69: (3-4):339–44. doi: 10.1365/s10337-008-0898-6 |
[26] | Harris CS , Beaulieu L , Fraser M , McIntyre KL , Owen PL , Martineau LC , Cuerrier A , Johns T , Haddad PS , Bennett SAL , Arnason JT . Inhibition of advanced glycation end product formation by medicinal plant extracts correlates with phenolic metabolites and antioxidant activity. Planta Med. (2011) ;77: (2):196–204. doi: 10.1055/s-0030-1250161 |
[27] | Beaulieu L , Harris CS , Saleem A , Cuerrier A , Haddad PS , Martineau LC , Bennett SAL , Arnason JT . Inhibitory effect of the Cree traditional medicine wiishichimanaanh (Vaccinium vitis-idaea) on advanced glycation endproduct formation: Identification of active principles. Phytother Res. (2010) ;24: (5):741–47. doi: 10.1002/ptr.3025 |
[28] | Haytowitz DB , Seema B . USDA database for the oxygen radical absorbance capacity (ORAC) of selected foods, Release 2. US Department of Agriculture 2010: 10-48. Available at http://www.ars.usda.gov/Services/docs.htm; date of use: December 2017. |
[29] | Stull AJ , Cash KC , Johnson WD , Champagne CM , Cefalu WT . Bioactives in blueberries improve insulin sensitivity in obese, insulin-resistant men and women. J Nutr. (2010) ;140: (10):1764–8. doi: 10.3945/jn.110.125336 |
[30] | Eid HM , Ouchfoun M , Brault A , Vallerand D , Musallam L , Arnason JT , Haddad PS . Lingonberry (Vaccinium vitis-idaea L.) Exhibits Antidiabetic Activities in a Mouse Model of Diet-Induced Obesity. Evidence-Based Complementary and Alternative Medicine (2014) :645812 [10 p] |
[31] | Esposito D , Chen A , Grace MH , Komarnytsky S , Lila MA . Inhibitory effects of wild blueberry anthocyanins and other flavonoids on biomarkers of acute and chronic inflammation in vitro. J Agric Food Chem. (2014) ;62: (29):7022–8. doi: 10.1021/jf4051599 |