Multi-radical (ORACMR5) antioxidant capacity of selected berries and effects of food processing
Abstract
BACKGROUND: Fruits and berries are known to contain relatively high amounts of antioxidant/bioactive compounds. Several methods have been used for measurement of antioxidant capacity (AC), but not all methods have direct relevance to in vivo antioxidant status.
OBJECTIVE: Determine AC in berry/fruit samples, processed berry products, and purified compounds by utilizing 5 different biologically relevant free radical/oxidant sources.
METHODS: Samples were assayed for AC capacity using 5 different free radical/oxidant sources: peroxyl radical (ORAC), hydroxyl radical (HORAC), peroxynitrite (NORAC), superoxide anion (SORAC) and singlet oxygen (SOAC)]. Total AC (sum of AC with 5 individual radicals) was expressed as Oxygen Radical Absorption Capacity using Multiple Radicals (ORACMR5).
RESULTS: SOAC contributed more than 60% of ORACMR5 in blackberries, sweet and tart cherries; and no detectable levels of SOAC were found in strawberries, black currants and raspberries. Whole fruit purees of mango, wild blueberry and cherry contained 95, 85 and 67% respectively of total ORACMR5 from SOAC. However, freeze dried wild blueberry powder from same production season had only 28% as SOAC and 42 and 22% as ORAC and HORAC. Blueberry/Pomegranate and Mango/Pineapple smoothies had 67% and 77% of ORACMR5 as SOAC.
CONCLUSIONS: The antioxidant quenching potential using 5 different radical/oxidant sources of different berries and fruits varied widely and understanding this variation may be helpful in understanding health benefits of different berries and foods.
1Background
Oxygen is critical to sustenance of life in all living organisms. However, catalytic reactions involving oxygen can also result in the formation of several free radicals (Fig. 1). Formation of superoxide anion and the hydroxyl radical occurs during various biochemical process, which are usually kept in check by cellular antioxidant mechanisms designed to quench free radicals. Superoxide dismutases are enzymes that catalyze the dismutation of superoxide anion to hydrogen peroxide and molecular oxygen. Reduction of hydrogen peroxides in cells is accomplished via the catalase enzyme to water and oxygen. Cycloxygenases, nitric oxide synthases and prostaglandin synthases are other sources of reactive oxygen species (ROS) [1]. ROS are oxygen-based chemical intermediates with high reactivity. In situations of excess production of ROS relative to antioxidant defenses, a condition termed oxidative stress exists. The balance between production of ROS and systems meant to mitigate ROS is termed ‘redox state.’ An extreme prooxidant status in a cell can lead to progressive loss of cellular function and eventual death. In order to prevent this, cells have developed mechanisms to detect changes in redox status and turn on the antioxidant machinery of the cell [1]. However, situations occur, i.e diseases conditions such as inflammation, diabetes, arthritis, atherosclerosis, cancer, infections, and other external stimulators such as radiation, drugs, drug metabolites or environmental pollutants, in which the intracellular antioxidant mechanisms are not able to correct the altered redox state. In these situations, antioxidant compounds are beneficial in preventing or reducing oxidative damage to cellular macromolecules. Recent published data were reviewed [2] in which increased consumption of dietary antioxidants, measured by the Oxygen Radical Absorbance Capacity (ORAC) or Total Phenolics by the Folin assay, were associated with improvements in health outcomes. It is clear that dietary bioactives/antioxidants have health effects in vivo, although it is also clear that some of the mechanisms of action may not be through a direct antioxidant mechanism, but through a signaling event to turn on antioxidant machinery in the cell. Nuclear factor-kappaβ is one notable factor in this regard [1]. Another major mechanism in the cellular defense against oxidative or electrophilic stress is activation of the Nrf2-antioxidant response element signaling pathway, which controls the expression of genes whose protein products are involved in the detoxification and elimination of reactive oxidants and electrophilic agents through conjugative reactions and by enhancing cellular antioxidant capacity [3]. Berries have been shown to be an excellent source of dietary antioxidants in the diet. After strawberry intake, a moderate increase in fasting plasma antioxidant capacity and vitamin C was observed, together with a significant increase in the lag phase preceding plasma lipid oxidation [4]. Increased intake of strawberries for only 2 weeks was shown to be sufficient to attenuate mononuclear cell mortality after ex vivo exposure to a single acute oxidative challenge suggesting that a regular consumption of strawberries may enhance body defenses against oxidative challenges [4]. Consumption of 75 g of blueberries significantly reduced postprandial oxidation when consumed with a typical high-carbohydrate, low-fat breakfast [5]. This is the first report that has demonstrated that increased serum antioxidant capacity derived from blueberries is not attributable to the fructose or ascorbate content of blueberries.
Antioxidant capacity assays provide measures of relative amounts of ‘bioactives’ in botanicals, with potential for expressing bioactivity in vivo through antioxidant mechanisms. Dietary antioxidant intake in published studies up to this point in time has relied primarily upon data derived using the peroxyl radical as the oxidant source in the Oxygen Radical Absorption Capacity (ORAC) assay developed by Ou, Huang, Prior et al. [6–8] and expanded into a food database by Wu and coworkers [9]. Recently, methods of assay have been developed using other biologically relevant radical/oxidant sources for assessing in vitro antioxidant capacity/reactivity. In addition to the original ORAC assay which used the peroxyl radical, a new panel of antioxidant assays has been developed by chemists at Brunswick Laboratories (Southborough, MA), and at International Chemistry Testing (Milford, MA), using the reactive oxygen species, superoxide anion, hydroxyl radical, and singlet oxygen, and the reactive nitrogen species, peroxynitrite. This panel of assays has led to the term, Oxygen Radical Absorbance Capacity using Multiple Radicals (ORACMR5) which is a sum of the antioxidant capacity against 5 of the reactive species found in the human body: ROS (peroxyl radical, hydroxyl radical, superoxide anion, singlet oxygen), and reactive nitrogen species (peroxynitrite). Utilizing ORACMR5, foods and dietary compounds, including berries can be identified that have significant capacity to efficiently quench other ROS. Because superoxide anion seems to be pivotal in the formation of other free radicals (Fig. 1), ORACMR5 provides the opportunity for the first time to identify berries and other foods that have the potential for efficient quenching of superoxide anion and thereby decreasing the oxidative load from other free radicals.
Berries are often processed and marketed in various forms utilized in baking, jam, or jelly or pureed for juice/pulp manufacture. Depending upon the processing method(s), antioxidant capacity using the peroxyl radical in the ORAC assay can be altered [10–12], but no data are available on the effects of processing on food antioxidants using multiple radical sources.
The objective of this study was to determine the relative antioxidant capacity of selected freeze dried berries, some processed berries/fruits into smoothies, juice concentrates or purees, and purified compounds using ORACMR5.
2Methods
2.1Antioxidant capacity methods
ORACMR5 methods described by Nemezer and Ou [13] were used for antioxidant capacity assay using peroxyl (ORAC), hydroxyl (HORAC), superoxide anion (SORAC), peroxynitrite (NORAC), and singlet oxygen (SOAC) radicals/oxidants. Peroxyl radical scavenging capacity (ORAC) was measured as previously reported [6, 14]. Fluorescein was used as the fluorescent probe. Hydroxyl radical scavenging capacity (HORAC) assay was performed according to a report by Ou et al. [15]. Peroxynitrite scavenging capacity (NORAC) was determined by monitoring the oxidation of 2,3,6-tribromo-4,5-dihydroxybenzyl methyl ether (DHR-123) [16].
Superoxide anion scavenging assay (SORAC) was performed as described by Zhang et al. [17]. Hydroethidine (HE) was used to measure the O2– scavenging capacity. The mixture of xanthine and xanthine oxidase was used to generate O2– radicals. Nonfluorescent HE was oxidized by O2– to form a species of unknown structure that emits fluorescence signal at 586 nm. Addition of superoxide dismutase (SOD) inhibits the HE oxidation.
Singlet oxygen scavenging capacity (SOAC) assay was designed to accurately quantify singlet oxygen activity in a high throughput fashion (Ou et al., unpublished data, ICT, Milford, MA). Singlet oxygen is generated in ethanol by the molybdate-catalyzed disproportionation of hydrogen peroxide at 37 °C. Hydroethidine (HE), a non-fluorescent probe, was oxidized by singlet oxygen to form oxyethidium which exhibits a strong fluorescence signal at 590 nm. Therefore, the inhibition of HE’s fluorescence increase in the presence of antioxidant is an index of antioxidant capacity. Results are expressed as “μmole TE (micromole Trolox equivalent)/gram or liter”. Using the conversion factor of 1 μmole vitamin E eq/μmole TE, the final results were calculated.
Total ORACMR5 (Sum of peroxyl (ORAC), hydroxyl (HORAC), superoxide anion (SORAC), peroxynitrite (NORAC), and singlet oxygen (SOAC) radicals/oxidants) expressed as μmoles TE per g freeze dried berry or per serving for berry smoothies and purees.
2.2Pure phenolic/antioxidant compounds
Twenty three pure compounds were purchased from commercial vendors for the analysis of ORACMR5 as follows: β-carotene, protocatechuic acid, ferulic acid (Alfa Aesar/Thermo Fisher Scientific Inc., Waltham, MA); lycopene, astaxanthin, quercetin, rutin, baicalin, catechin, epicatechin, epicatechingallate, epigallocatechin (EGC), EGC gallate, naringenin, neohesperidin, gallic acid, caffeic acid, chlorogenic acid, Vitamin C (Sigma-Aldrich Corp., St. Louis, MO); Kaempferol, (INDOFINE Chemical Company, Inc., Hillsborough, NJ); hesperetin, resveratrol (Quality Phytochemicals LLC, New Jersey); procyanidin A2, (Extrasynthese,Genay,Cedex, France). Samples were analyzed in triplicate and data were expressed as μmoles Trolox Equivalents (TE) per mg of pure compound.
2.3Processed samples
Processed samples for analysis were obtained from commercial sources. Smoothies [Blueberry/pomegranate (BPS) and Mango/pineapple MPIS)] were obtained from a local McDonalds restaurant. BPS contained the following ingredients: in a low-fat Yogurt, Blueberry Puree, Water, Clarified Demineralized Pineapple Juice Concentrate, Raspberry Puree, Apple Juice Concentrate, Pomegranate Juice Concentrate, and contained 1% or less of Natural (Plant Source) and Artificial Flavors, Cellulose Powder, Peach Juice Concentrate, Pear Juice Concentrate, Citric Acid, Lemon Juice Concentrate, Xanthan Gum, Pectin, Fruit and Vegetable Juice For Color [18].
The Mango Pineapple Fruit smoothie (MPS) base contained the following ingredients in a low fat yogurt: Water, Clarified Demineralized Pineapple Juice Concentrate, Mango Puree Concentrate, Pineapple Juice Concentrate, Orange Juice Concentrate, Pineapple Puree (Pineapple and Ascorbic Acid), Apple Juice Concentrate, Contained less than 1% : Cellulose Powder, Natural (Plant Source) and Artificial Flavors, Xanthan Gum, Pectin, Citric Acid, Fruit and Vegetable Juice for Color, Turmeric Extract (Color) [19].
Whole berry/fruit Fruition purees [Mango Passion Fruition (MPF); Wild Blueberry Fruition (WBF); Cherry Fruition (CF) were obtained from Wyman’s of Maine (Milbridge, ME). The Fruition products are 100% of either mango, wild blueberry or cherry that have been “gently pressed and flash frozen”.
Aronia Juice concentrate was obtained from Mae’s Health and Wellness, LLC, Omaha, NE. This product is a highly concentrated version of the raw aronia berry in a convenient juice form. The product is stored frozen.
2.4Percent polymeric color analysis
Percent polymeric color (PC) of purees was determined using the spectrophotometric assay of Giusti and Wrolstad [20]. Sample extracts were diluted with water in order to have an absorbance reading between 0.5 and 1.0 at 512 nm. For analysis, 0.2 mL of 0.90 M potassium metabisulfite was added to 2.8 mL diluted sample (bisulfite bleached sample) and 0.2 mL of DI water was added to 2.8 mL diluted sample (non-bleached, control sample). After equilibrating for 15 min, but not more than 1 h, samples were evaluated at λ= 700 nm, 512 nm, and 420 nm. Color density was calculated using the control sample according to the following formula:
Color Density = [(A420 nm – A700 nm) + (A512 nm – A700 nm)]×Dilution Factor
Polymeric color was determined using the bisulfite-bleached sample using the following formula:
Polymeric Color = [(A420 nm – A700 nm) + (A512 nm – A700 nm)]×Dilution Factor
Percent polymeric color was calculated using the formula:
% Polymeric color = (polymeric color/color density)×100
3Results and discussion
Freeze dried samples of 14 different berries/fruits were measured for antioxidant capacity using assays that test for efficacy against 5 different radical/oxidant sources [peroxyl (ORAC), hydroxyl (HORAC), peroxynitrite (NORAC), superoxide anion (SORAC) and singlet oxygen (SOAC)] with the total of the 5 expressed as ORACMR5 (Table 1). Total ORACMR5 ranged from 475 to 3003 μmoles Trolox Equivalents per g dry weight (TE/g DM) for the berries analyzed, however, the antioxidant capacity for different radical sources varied considerably. Singlet O2 antioxidant capacity contributed to more than 60% of the ORACMR5 in blackberries, sweet and tart cherries, but ORACMR5 in blackberries was more than 3 times higher than in sweet and tart cherries. No detectable singlet O2 antioxidant oxygen capacity (AOC) was found in strawberries, black currants and raspberries (Table 1, Fig. 2). SOARC was found in all berries tested (Table 1; Fig. 3) with organically grown blueberries having the highest SORAC of the berries tested (Fig. 3).
Antioxidant protection against the hydroxyl radical was predominate in Aronia juice concentrate and Açaí (Table 1). Antioxidant protection against the peroxyl radical (ORAC) was predominate in blueberry, elderberry, bilberry and black currant but ranged from 356 to 989 μmoles TE/g DM in the different berries. There were 2 samples of wild blueberry which were composite samples collected in 2 different production years (2013 and 2014). The composite for each year was prepared by compiling samples of blueberries from different fields and then the mixture was freeze dried and ground into a powder. Differences were observed between the production years with the 2014 production year lower in total ORACMR5 than 2013 production year but we do not have an explanation for the relatively large change between years.
Although previous in vivo studies have been based upon dietary intake of ORAC using the peroxyl radical, it seems clear that consideration must be given to other important radical/oxidant sources that are prevalent in vivo that are used in ORACMR5. Total ORACMR5 may be important in terms of in vivo protection against oxidative stress, but the balance of antioxidants in berries and foods may be equally or more important.
3.1Processing effects on ORACMR5
The effects of processing berries into whole fruit purees or smoothies on the antioxidant capacity were also investigated using ORACMR5 methods. Whole fruit Fruition purees of mango, wild blueberry and cherry contained 95, 85 and 67% respectively of the total ORACMR5 as singlet oxygen (SOAC) radical antioxidant protection (Table 2). However, freeze dried fresh wild blueberry from the same production season (2014) (Table 2) had only 28% as SOAC and 42 and 22% as ORAC and HORAC, respectively. Data from fresh wild blueberries (Wild BB 2014) are presented as a comparison to that of Wild Blueberry puree in the radial plot (Fig. 4). The remarkable observation is the relatively high singlet oxygen capacity in processed (pressed/pureed) blueberry samples had 50% lower amounts for ORAC (peroxyl radical) in the processed samples relative to the freeze dried wild blueberry sample. Comparing the unprocessed freeze dried wild blueberry to the wild blueberry Fruition, on a μmole basis, the increase in SOAC more than accounts for by the decrease in ORAC and HORAC such that the total ORACMR5 is 1721 compared to 1206 μmoles TE/g DM for the Fruition compared to the freeze dried wild blueberry. Although with processing there did not appear to be a decline in total ORACMR5 in the blueberry Fruition compared to whole blueberry, there was a definite shift in the amount of protection from peroxyl (ORAC) and perhaps from hydroxyl radicals (HORAC) into protection against singlet oxygen. The explanation for this shift is not clear. Processing did not cause a large increase in polymeric color (∼18%) (Table 2), as we have observed these levels in freeze dried wild blueberries. There does not appear to be any major shift in the anthocyanin profile in the Wild Blueberry Fruition (data not presented).
Mango and Cherry Fruition samples also had antioxidant capacity primarily against singlet oxygen (95 and 85% respectively) (Table 2). Unprocessed samples of mango and cherry were not available to compare with the processed samples. Sweet and tart cherries were shown to have 54% and 67%, respectively, of the ORACMR5 as SOAC. Blackberries were also been shown to have high SOAC (66% of total) and a quite high ORACMR5 (Table 1).
Commercially available Blueberry/Pomegranate and Mango/Pineapple smoothies had 70% and 77% of total ORACMR5 as SOAC, while antioxidant capacity against the peroxyl radical (ORAC) was 25% and 12%, respectively (Fig. 5, Table 3). The ORAC from a serving of the blueberry/pomegranate smoothie provided 2213 μmoles TE of ORAC (Table 3) which is equivalent to the estimated average of a serving of fruits and vegetables [9]. The Mango/Pineapple smoothie had a lower ORAC but higher SOAC and ORACMR5 than the blueberry/pomegranate smoothie (Table 3, Fig. 5). On a per serving basis (87 mL), the Fruition products had total ORACMR5 of 19,000 to ∼37,000 umoles TE and the smoothies had values of ∼9,000 ORACMR5 but mostly as SOAC. A serving of either of these smoothies would make a significant contribution to the daily antioxidant capacity intake in the diet. The Fruition products had higher ORACMR5 relative to calories in a serving compared to the Blueberry/Pomegranate and Mango/Pineapple smoothies. This can be important since the higher the caloric intake the more ROS will likely be generated. Additional studies are needed to determine the processing conditions responsible for the changes in antioxidant capacity within ORACMR5.
3.2ORACMR5 of pure phytochemicals
Purified polyphenolic compounds found in many berries were also analyzed for ORACMR5 antioxidant capacity and the data is expressed as μmoles TE per mg of pure compound (Table 4) and SOAC and SORAC are presented in Figs. 6 and 7 and expressed as μmoles TE per μmole pure compound. There were large differences in antioxidant protection against the 5 oxidant sources. More than 95% of the ORACMR5 in vitamin C was as SORAC, (Tables 4). Using the peroxyl radical in the ORAC method, vitamin C was shown to have a relative value of 0.95 compared to Trolox [7], but levels in this study were non-detectable (Table 4).
The flavanols as a group had major SORAC protection as well as gallic acid (Table 4, Fig. 7). Green tea polyphenols were studied by Sato et al. [21] to determine structural characteristics for superoxide anion radical scavenging. Some structures among the green tea polyphenols were shown to actually produce superoxide anion as well as other structural characteristics had superoxide scavenging activity. In some cases the scavenging activity was stronger than the production activity. A net scavenging activity was observed for epigallocatechin-gallate, methyl-gallate, gallocatechin-epigallocatechin and epigallocatechin-epicatechin [21]. However, using our conditions of analysis, epigallocatechin and gallic acid were shown to have the highest superoxide anion quenching of the polyphenols (Table 4, Fig. 7) but epigallocatechin acted as a superoxide anion generator under the conditions used by Sato et al. [21]. Indoxyl sulfate has been shown to be an endogenous antioxidant with superoxide anion scavenging activity generated from activated neutrophils and also it has been shown to eliminate hydroxyl radicals [22]. Catecholamines have also been shown to have a direct effect on scavenging superoxide anion radicals [23]. Superoxide anion has been shown to be detrimental in some health related conditions. Physical frailty in older people is associated with superoxide anion overproduction by NADPH oxidase and low-grade chronic inflammation [24]. Diabetes-induced breakdown of the blood-retinal barrier (BRB) has been linked to hyperglycemia-induced expression of vascular endothelial growth factor (VEGF) and is likely mediated by an increase in oxidative stress mediated by superoxide anion [25]. Findings by Folbergrova and coworkers [26] suggested that increased superoxide generation precedes neuronal degeneration and may thus play a causative role in neuronal injury in the occurrence of oxidative stress in brain of immature rats during seizures. This may have clinical relevance for an approach for the treatment of epilepsy in children, suggesting that substances with antioxidant properties combined with the conventional therapies might provide a beneficial effect [26].
Astazanthin, beta-carotene and kaempferol had more than 90% of the ORACMR5 was as SOAC (Table 4). Astaxanthin is classified as axanthophyll. Singlet oxygen is produced by leukocytes during inflammatory and biochemical reactions and deactivated by producing reactive oxygen species and peroxides. It is generally accepted that free radical quenching is a key requirement for an efficient antioxidant. However, the antioxidant capacity does not depend solely on the efficiency of quenching/removal of the oxidizing free radical but also on the reactivity and the lifetime of the products of the quenching reaction [27]. While carotenoids are generally accepted as ‘antioxidants’, the reactivity/lifetime of such carotenoid radicals may lead to a switch from anti- to pro-oxidant behavior and such redox-controlled reactions may lead to deleterious as well as beneficial health effects [27]. Bohm et al. [27] have shown that in a mixture of carotenoids all carotenoid radical cations will be converted by lycopene back to the parent carotenoid and the lycopene may be sacrificed. It was noted that the radical cations arising from the three carotenoids present in the human macular (lutein, zeaxanthin and mesozeaxanthin) are all repaired efficiently by lycopene but not by β-carotene. Astaxanthin is being marketed as one of the most powerful antioxidants found in nature primarily because, unlike some other types of antioxidants, astaxanthin never becomes a pro-oxidant in the body so it can never cause harmful oxidation. Based upon the limited number of pure compounds that we analyzed, astaxanthin is definitely one that has the highest capacities for quenching singlet oxygen (Table 5), but it has low capacity for quenching the other radicals. Lycopene and beta-carotene have much lower SOAC and ORACMR5 than astaxanthin (Table 4, Fig. 6). The interaction of carotenoids with singlet oxygen species is important for the understanding of many important aspects of life such as photosynthesis, vision, various medical treatments from dermatology to cancer, as well as understanding any possible deleterious reactions affecting man.
Of the pure compounds analyzed (Table 4), procyanidin dimer-A2 had extremely high ORAC levels and asta xanthin had higher ORAC levels than most of the pure compounds analyzed (Table 4). Of the berries, blueberries, elderberries and Açaí fruit pulp had relatively high ORAC levels (Table 1) which has been documented previously [9]. ORAC levels in numerous fruits and vegetables have been published previously [9].
Nitric oxide is an important intracellular messenger molecule that activates guanylyl cyclase to convert guanosine triphosphate to cyclic GMP which causes smooth muscle relaxation [28]. Nitric oxide converts rapidly to NO2 under aerobic conditions (Fig. 1). Nitric oxide is known to have beneficial effects on human health [29]. Its removal by antioxidants may lead to detrimental effects on health. In contrast, NO2 is a strong radical oxidant and known only to cause damage to biomolecules and human health [30]. NO2 can be converted to peroxynitrite or nitric oxide can react with superoxide anion to form peroxynitrite (Fig. 1). Peroxynitrite oxidizes protein thiols forming disulfide and can nitrate tyrosyl groups in proteins to form nitrated proteins leading to either a loss or gain of enzymatic activity or interfere with signal transduction mechanisms. Peroxynitrite (NORAC) protection was relatively low in all berry samples tested (<1–5% of ORACMR5 (Table 1). Most of the pure compounds tested also had relatively low antioxidant protection against peroxynitrite except baicalin and procyanidin dimer-A2 which had NORAC values which were 31.5 and 8.4% of ORACMR5, respectively (Table 4). Baicalin is a polyphenol flavonoid (flavone) naturally extracted from Scutellaria sp. (skullcap) including Scutellariabaicalensis and Scutellarialateriflora. It has numerous health promoting properties, including anti-inflammatory, calming, and sleep promoting benefits, and is contained in plants which have been used for centuries in traditional medicine.
Baicalin and procyanidin dimer-A2 were also compounds with relatively higher HORAC levels (Table 4). Berries that had relatively higher levels of HORAC included Aronia juice concentrate, Açaí and wild blueberry particularly from the 2013 production year. There is no data available suggesting that any of these berries have biacalin or procyanidin dimer-A2. Procyanidin dimer-A2 had much higher levels of ORAC and NORAC than HORAC (Table 4).
3.3Dietary antioxidant intake and health effects
Data available from the published literature [2] indicates that daily intakes in the range of 10–14,000 umoles TE antioxidant capacity against the peroxyl radical (ORAC) is sufficient to have beneficial health effects. A recent study [13] using a supplement mixture of berries and other ingredients that provided 44,000 units of ORACMR5 and 10,700 units of ORAC, was effective in deceasing oxidative stress following a meal. This study is the first reported that combined a mixture of antioxidant sources to arrive at a reasonable balance between the antioxidants and also to demonstrate an in vivo effect on lowering free radicals in the plasma following a meal.
In addition to studies referenced in a recent review [2], more recent studies have also demonstrated that increased intake of dietary antioxidants/phytochemicals can improve health outcomes. Increased dietary antioxidant intake was associated with less oxidative stress among men diagnosed with prostate cancer [31] as indicated by lower urinary 8-isoprostane concentrations. Ingestion of anthocyanin-rich beverages improved antioxidant enzyme activities and plasma antioxidant capacity, thus protecting the body against oxidative stress, a hallmark of ongoing atherosclerosis [32]. Diets naturally rich in polyphenols positively influence fasting and postprandial triglyceride-rich lipoprotein (TRL) concentrations and reduced oxidative stress. Marine long chain n-3 polyunsaturated fatty acids reduced TRLs of exogenous origin. Through their effects on postprandial lipemia and oxidative stress, polyphenols may favorably affect cardiovascular disease risk [33].
Ingestion of a phytonutrient-poor food and its individual fat/protein or sugar components increased plasma oxidative activity, which was not observed after ingestion of a kilojoule-equivalent phytonutrient-rich food [34]. A decrease in resting luminol enhanced whole blood chemiluminescence was observed following regular strawberry consumption which may suppress baseline formation of oxidants by circulating phagocytes and decrease the risk of systemic imbalance between oxidants and anti-oxidants and be one of mechanisms of the health-promoting effects of strawberry consumption [35]. The major antioxidant protection in strawberries is from peroxyl, hydroxyl and superoxide-anion radicals (Table 1).
Attenuated oxidative and inflammatory responses were observed with Montmorency tart cherry concentrate consumption which may be efficacious in combating post-exercise oxidative and inflammatory cascades that can contribute to cellular disruption. Additionally, consumption of Montmorency tart cherry concentrate may be beneficial in repeated days of cycling and conceivably in other sporting scenario’s where back-to-back performances are required [36].
4Conclusions
Data obtained using ORACMR5 provides new insights into diet planning and food selection. The recommendation for several years has been to consume a variety of fruits and vegetables. The information from ORACMR5 analysis provides additional support and reasons for this recommendation as we gain a better understanding of different types of antioxidants in different foods. Raspberries, black currants, strawberries and boysenberries had 30–50% of their total ORACRMR5 as superoxide anion antioxidant capacity (SORAC), but absolute values per g DM were higher for other berries that had higher total ORACMR5 levels (Organic blueberry and Blackberry). Consumption of higher levels of SOARC in foods should provide greater potential to suppress the oxidative load from other radicals.
Blackberries were shown to have quite high levels of singlet oxygen antioxidant capacity (SOAC), which has not been reported previously, although it is not clear what compound or group of compounds are responsible for this high SOAC capacity.
Peroxynitrite antioxidant capacity is relatively low in all foods tested. Whether this is related to the possibility that removal of too much nitric oxide may have detrimental health effects warrants further investigation.
Total ORACMR5 can vary considerably between berries and also between production years based upon data presented from wild blueberry, a finding which likely exists with other berries when growing conditions vary from year to year. Based upon the concept of selecting a balance of antioxidants based upon ORACMR5, one can select one or a combination of berries that provide antioxidant protection from multiple radical sources. Additional clinical trials will be needed in order to document that health benefits indeed do result from consumption of adequate levels and a balance of antioxidants represented in ORACMR5. Results from an initial clinical trial appear encouraging [13]. Consumption of berries in the diet either individually and/or in combination can be important components in a diet that provide a balance in protection from oxidative stress from multiple oxidant sources within the body.
Processing of berries/fruits into smoothies or fresh fruit purees appears to cause a shift in antioxidant capacity to singlet oxygen protection relative to the protection from other radicals. Consumption of a serving of berry smoothies or purees/Fruition products can make a significant contribution to total ORACMR5 consumption in the diet but may need to be combined with other berries or foods to provide a greater “balance” in antioxidant capacity.
References
[1] | Shankar K , Mehendale HM . Oxidative Stress, In: Wexler P, editor. Encyclopedia of Toxicology (Third Edition). Oxford: Academic Press; (2014) . pp. 735–7, http://dx.doi.org/10.1016/B978-0-12-386454-3.00345-6 |
[2] | Prior RL . Oxygen radical absorbance capacity (ORAC): New horizons in relating dietary antioxidants/bioactives and health benefits. Journal of Functional Foods. (2014) ; (on line)(12: ), http://dx.doi/10.1016/j.jff.2014.12.018 |
[3] | Nguyen T , Nioi P , Pickett CB . The Nrf2-antioxidant response element signaling pathway and its activation by oxidative stress. J Biol Chem. (2009) ;284: (20):13291–5, http://dx.doi.org/10.1074/jbc.R900010200 |
[4] | Tulipani S , Armeni T , Giampieri F , Alvarez-Suarez JM , Gonzalez-Paramas AM , Santos-Buelga C , et al. Strawberry intake increases blood fluid, erythrocyte and mononuclear cell defenses against oxidative challenge. Food Chem. (2014) ;156: :87–93, http://dx.doi.org/10.1016/j.foodchem.2014.01.098 |
[5] | Blacker BC , Snyder SM , Eggett DL , Parker TL . Consumption of blueberries with a high-carbohydrate, low-fat breakfast decreases postprandial serum markers of oxidation. Br J Nutr. (2012) ;109: (09):1–8, http://dx.doi.org/10.1017/s0007114512003650 |
[6] | Huang D , Ou B , Hampsch-Woodill M , Flanagan JA , Prior RL . High-throughput assay of oxygen radical absorbance capacity (ORAC) using a multichannel liquid handling system coupled with a microplate fluorescence reader in 96-well format. J Agric Food Chem. (2002) ;50: (16):4437–44, http://dx.doi.org/10.1021/jf0201529 |
[7] | Ou B , Hampsch-Woodill M , Prior RL . Development and validation of an improved oxygen radical absorbance capacity assay using fluorescein as the fluorescent probe. J Agric Food Chem. (2001) ;49: (10):4619–26, http://dx.doi.org/10.1021/jf010586o">http://dx.doi.org/10.1021/jf010586o |
[8] | Huang D , Ou B , Prior RL . The chemistry behind antioxidant capacity assays. J Agric Food Chem. (2005) ;53: (6):1841–56, http://dx.doi.org/10.1021/jf030723c |
[9] | Wu X , Beecher GR , Holden JM , Haytowitz DB , Gebhardt SE , Prior RL . Lipophilic and hydrophilic antioxidant capacities of common foods in the United States. J Agric Food Chem. (2004) ;52: (12):4026–37, http://dx.doi.org/10.1021/jf049696w |
[10] | Brownmiller C , Howard LR , Prior RL . Processing and storage effects on monomeric anthocyanins, percent polymeric color, and antioxidant capacity of processed blueberry products. J Food Sci. (2008) ;73: (5):H72–H9, http://dx.doi.org/10.1111/j.1750-3841.2008.00761.x |
[11] | Hager TJ , Howard LR , Prior RL . Processing and storage effects on monomeric anthocyanins, percent polymeric color, and antioxidant capacity of processed blackberry products. J Agric Food Chem. (2008) ;56: (3):689–95, http://dx.doi.org/10.1021/jf071994g |
[12] | Howard LR , Prior RL , Liyanage R , Lay JO . Processing and storage effect on berry polyphenols: Challenges and implications for bioactive properties. J Agric Food Chem. (2012) ;60: (27):6678–93, http://dx.doi.org/10.1021/jf2046575 |
[13] | Nemzer B , Chang T , Xie Z , Pietrzkowski Z , Reyes T , Ou B . Decrease of free radical concentrations in humans following consumption of a high antioxidant capacity natural product. Food Science and Nutrition. (2014) ;2: (6):647–54, http://dx.doi.org/10.1002/fsn3.146 |
[14] | Ou B , Chang T , Huang D , Prior RL . Determination of Total Antioxidant Capacity by Oxygen Radical Absorbance Capacity (ORAC) using fluorescein as the fluorescence probe: First action 2013.23. J of AOAC International. (2013) ;96: (6)1372–6, http://dx.doi.org/10.5740/jaoacint.13-175 |
[15] | Ou B , Hampsch-Woodill M , Flanagan J , Deemer EK , Prior RL , Huang D . Novel fluorometric assay for hydroxyl radical prevention capacity using fluorescein as the probe. J Agric Food Chem (2002) ;50: (10):2772–7, http://dx.doi.org/10.1021/jf011480w |
[16] | Chung HY , Choi HR , Park HJ , Choi JS , Choi WC . Peroxynitrite Scavenging and Cytoprotective Activity of 2,3,6-Tribromo-4,5-dihydroxybenzyl Methyl Ether from the Marine Alga Symphyocladia latiuscula. J Agric Food Chem. (2001) ;49: (8):3614–21, http://dx.doi.org/10.1021/jf0101206 |
[17] | Zhang L , Huang D , Kondo M , Fan E , Ji H , Kou Y , et al. Novel high-throughput assay for antioxidant capacity against superoxide anion. J Agric Food Chem. (2009) ;57: (7):2661–7, http://dx.doi.org/10.1021/jf8033368 |
[18] | NA. Blueberry-Pomegranate Smoothie 2015 [cited 12/01], Available from: http://www.mcdonalds.com/us/en/food/product_nutrition.mccafe.3810.blueberry-pomegranate-smoothie-small.html |
[19] | NA. Mango-Pineapple Smoothie 2015 [cited 12/01], Available from: http://www.mcdonalds.com/us/en/food/product_nutrition.mccafe.2788.mango-pineapple-smoothie-small.html |
[20] | Giusti MM , Wrolstad RE . Characterization and measurement of anthocyanins with UV-visible spectroscopy, In: Wrolstad RE, editor. Current Protocols in Food Chemistry. New York, NY: John Wiley & Sons; (2001) . p. F1.2.1–F.2.13. |
[21] | Sato M , Toyazaki H , Yoshioka Y , Yokoi N , Yamasaki T . Structural characteristics for superoxide anion radical scavenging and productive activities of green tea polyphenols including proanthocyanidin dimers. Chem Pharm Bull (Tokyo) (2010) ;58: (1), 98–102. |
[22] | Miyamoto Y , Iwao Y , Tasaki Y , Sato K , Ishima Y , Watanabe H , et al. The uremic solute indoxyl sulfate acts as an antioxidant against superoxide anion radicals under normal-physiological conditions. FEBS Lett. (2010) ;584: (13):2816–20, http://dx.doi.org/10.1016/j.febslet.2010.04.046 |
[23] | Kladna A , Berczynski P , Kruk I , Michalska T , Aboul-Enein HY . Superoxide anion radical scavenging property of catecholamines. Luminescence. (2013) ;28: (4):450–5, http://dx.doi.org/10.1002/bio.2475 |
[24] | Baptista G , Dupuy AM , Jaussent A , Durant R , Ventura E , Sauguet P , et al. Low-grade chronic inflammation and superoxide anion production by NADPH oxidase are the main determinants of physical frailty in older adults. Free Radic Res. (2012) ;46: (9):1108–14, http://dx.doi.org/10.3109/10715762.2012.692784 |
[25] | El-Remessy AB , Franklin T , Ghaley N , Yang J , Brands MW , Caldwell RB , et al. Diabetes-induced superoxide anion and breakdown of the blood-retinal barrier: Role of the VEGF/uPAR pathway. PLoS One (2013) ;8: (8):e71868, http://dx.doi.org/10.1371/journal.pone.0071868 |
[26] | Folbergrova J , Otahal J , Druga R . Brain superoxide anion formation in immature rats during seizures: Protection by selected compounds. Exp Neurol. (2012) ;233: (1):421–9, http://dx.doi.org/10.1016/j.expneurol.2011.11.009 |
[27] | Bohm F , Edge R , Truscott TG . Interactions of dietary carotenoids with singlet oxygen (1O2) and free radicals: Potential effects for human health. Acta Biochim Pol (2012) ;59: (1), 27–30. |
[28] | Kalyanaraman B . Teaching the basics of redox biology to medical and graduate students: Oxidants, antioxidants and disease mechanisms. Redox Biol. (2013) ;1: (1):244–57, http://dx.doi.org/10.1016/j.redox.2013.01.014 |
[29] | Moncada S , Higgs A . The L-arginine-nitric oxide pathway. The New England Journal Of Medicine (1993) ;329: (27), 2002–12. |
[30] | Kirsch M , Korth H-G , Sustmann R , de Groot H . The pathobiochemistry of nitrogen dioxide. Biological Chemistry (2002) ;383: (3-4), 389–99. |
[31] | Vance TM , Azabdaftari G , Pop EA , Lee SG , Su LJ , Fontham ET , et al. Intake of dietary antioxidants is inversely associated with biomarkers of oxidative stress among men with prostate cancer. Br J Nutr. (2015) :1–7, http://dx.doi.org/10.1017/s0007114515004249 |
[32] | Kuntz S , Kunz C , Herrmann J , Borsch CH , Abel G , Frohling B , et al. Anthocyanins from fruit juices improve the antioxidant status of healthy young female volunteers without affecting anti-inflammatory parameters: Results from the randomised, double-blind, placebo-controlled, cross-over ANTHONIA (ANTHOcyanins in Nutrition Investigation Alliance) study. Br J Nutr. (2014) ;112: (6):925–36, http://dx.doi.org/10.1017/s0007114514001482 |
[33] | Annuzzi G , Bozzetto L , Costabile G , Giacco R , Mangione A , Anniballi G , et al. Diets naturally rich in polyphenols improve fasting and postprandial dyslipidemia and reduce oxidative stress: A randomized controlled trial. Am J Clin Nutr. (2014) ;99: (3):463–71, http://dx.doi.org/10.3945/ajcn.113.073445 |
[34] | Khor A , Grant R , Tung C , Guest J , Pope B , Morris M , et al. Postprandial oxidative stress is increased after a phytonutrient-poor food but not after a kilojoule-matched phytonutrient-rich food. Nutr Res. (2014) ;34: (5):391–400, http://dx.doi.org/10.1016/j.nutres.2014.04.005 |
[35] | Bialasiewicz P , Prymont-Przyminska A , Zwolinska A , Sarniak A , Wlodarczyk A , Krol M , et al. Addition of strawberries to the usual diet decreases resting chemiluminescence of fasting blood in healthy subjects-possible health-promoting effect of these fruits consumption. J Am Coll Nutr. (2014) ;33: (4):274–87, http://dx.doi.org/10.1080/07315724.2013.870502 |
[36] | Bell PG , Walshe IH , Davison GW , Stevenson E , Howatson G . Montmorency cherries reduce the oxidative stress and inflammatory responses to repeated days high-intensity stochastic cycling. Nutrients. (2014) ;6: (2):829–43, http://dx.doi.org/10.3390/nu6020829 |
[37] | Yang J , Ou B , Wise ML , Chu Y . In vitro total antioxidant capacity and anti-inflammatory activity of three common oat-derived avenanthramides. Food Chem. (2014) ;160: : 338–45, http://dx.doi.org/10.1016/j.foodchem.2014.03.059 |
[38] | Yan Y , Tan CK , Wu H , Wang S , Huang D . Nitrogen dioxide absorbance capacity of flavanols quantified by a NO(2)-selective fluorescent probe. J Agric Food Chem. (2014) ;62: (23):5253–8, http://dx.doi.org/10.1021/jf5001925 |
Figures and Tables
Fig.1
Reactive oxygen and nitrogen species: Generation and their reactions. Adapted from Kalyanaraman, 2013 [28] and Yan et al. [38].
![Reactive oxygen and nitrogen species: Generation and their reactions. Adapted from Kalyanaraman, 2013 [28] and Yan et al. [38].](https://ip.ios.semcs.net:443/media/jbr/2016/6-2/jbr-6-2-jbr127/jbr-6-jbr127-g001.jpg)
Fig.2
Singlet Oxygen Antioxidant Capacity (SOAC) of freeze dried berries/fruits expressed as μmoles TE per g dry matter (DM).
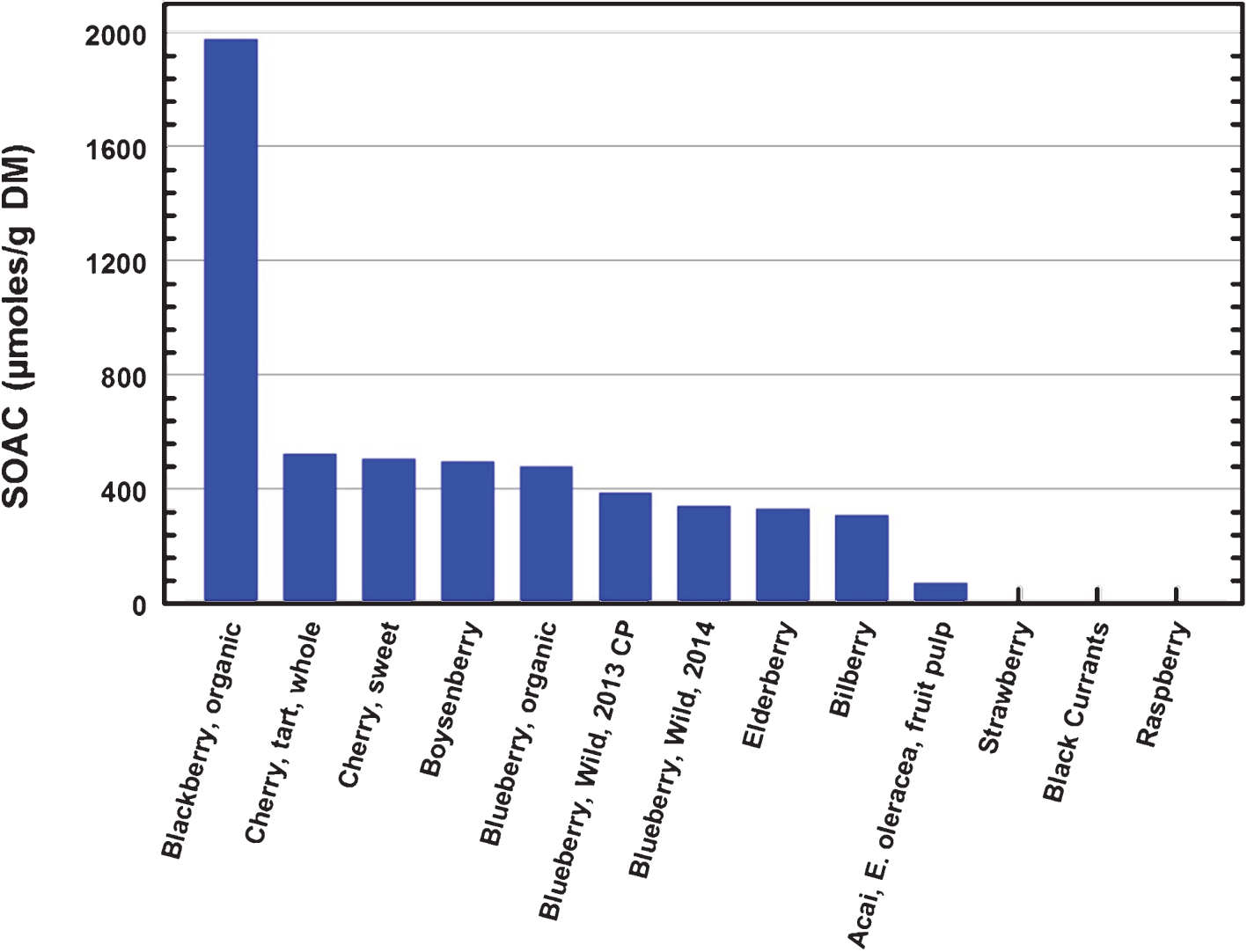
Fig.3
Superoxide Oxygen Radical Antioxidant Capacity (SORAC) of freeze dried berries/fruits expressed as μmoles TE per g dry matter (DM).
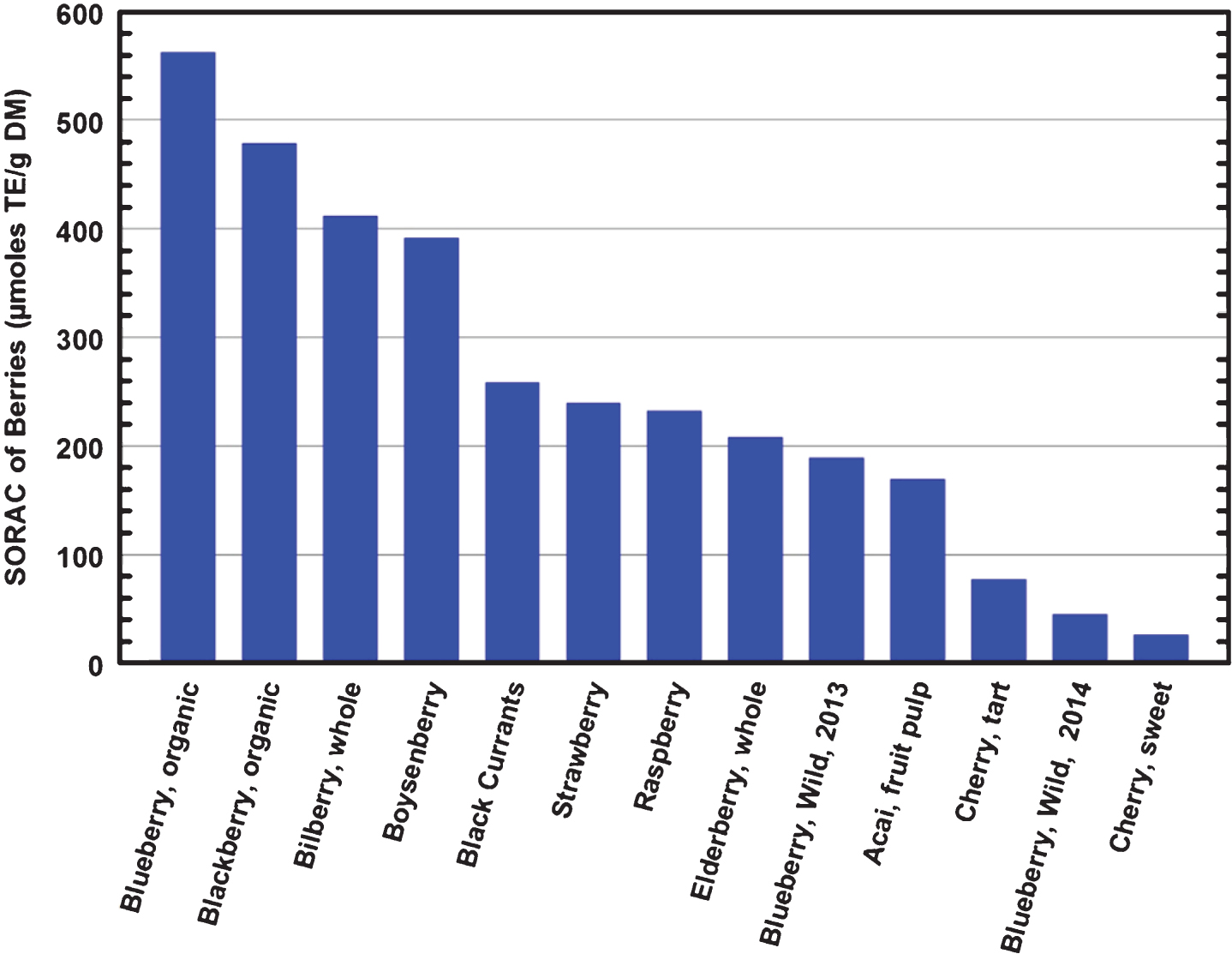
Fig.4
Radial plot comparison of Wild Blueberry (WB) Passion Fruition and Wild Blueberry freeze dried powder (WB FD) (2013). Antioxidant data expressed as μmole TE/g dry matter (DM). Values for each radical are indicated on the respective radial arm.
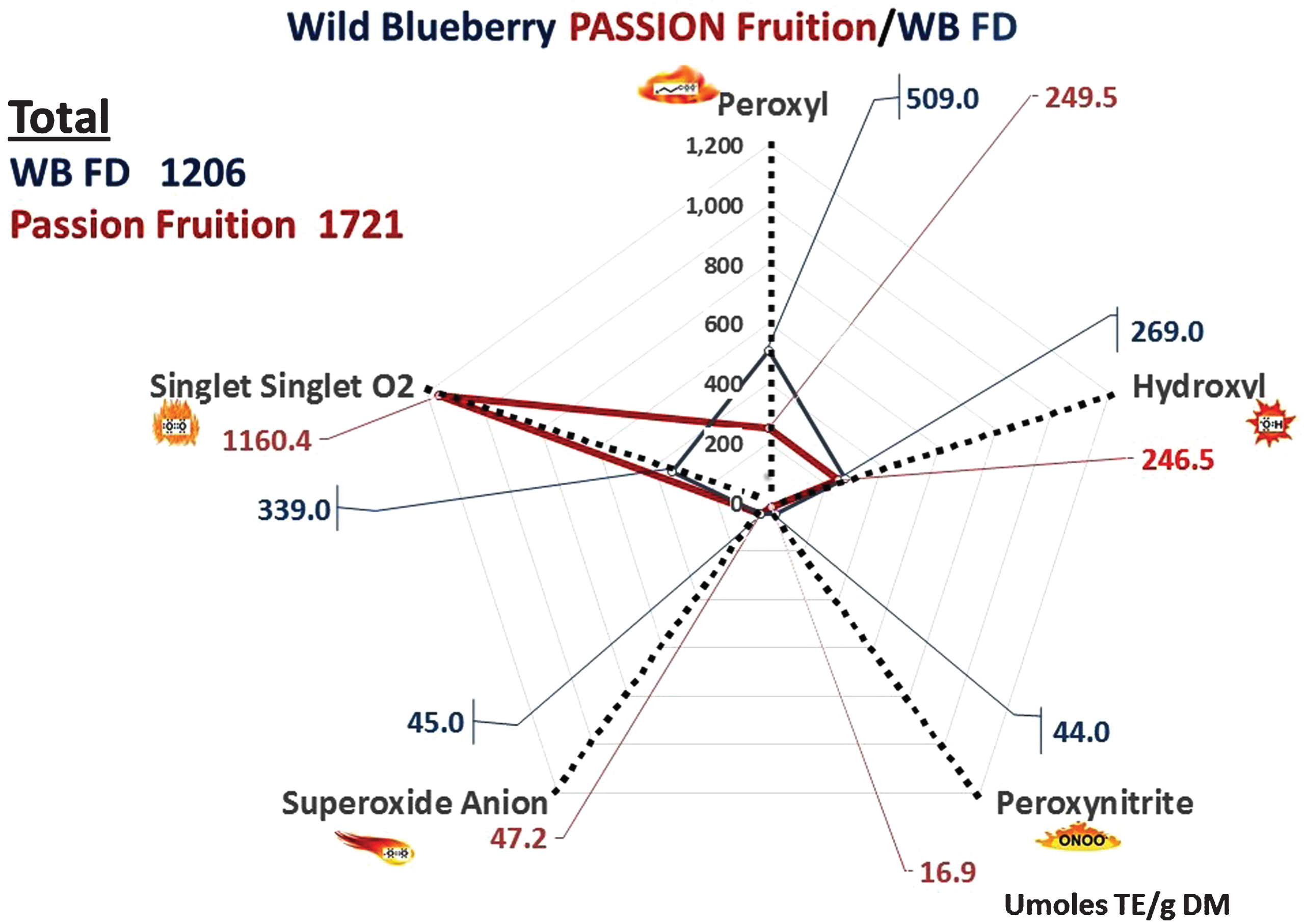
Fig.5
Radial plot comparison of blueberry/pomegranate and Mango/pineapple smoothes. Antioxidant data expressed as μmole TE/serving (265 g). Values for each radical indicated on the respective radial arm.
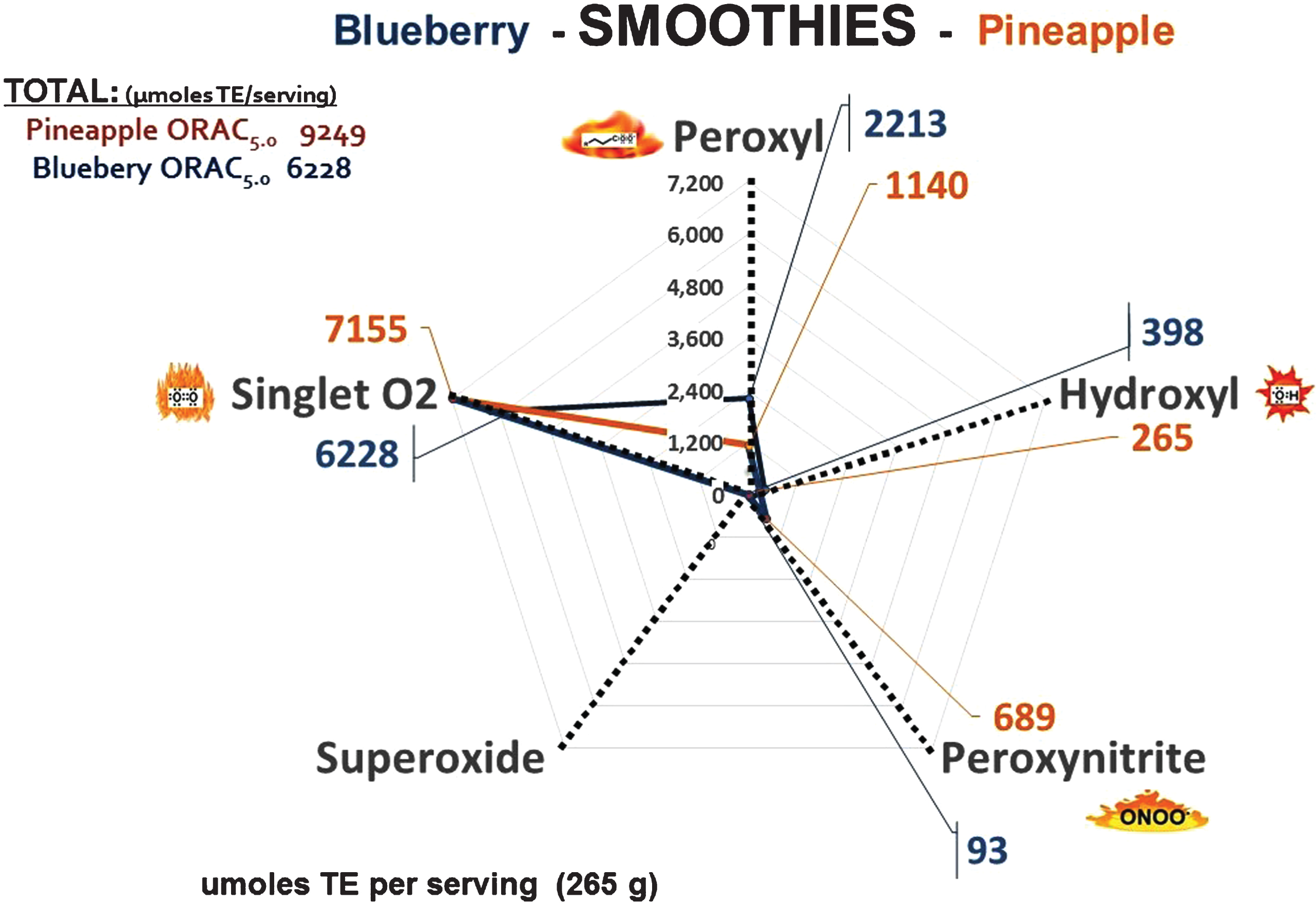
Fig.6
Singlet Oxygen Antioxidant Capacity (SOAC) of pure compounds expressed as μmoles TE per μmole pure compound.
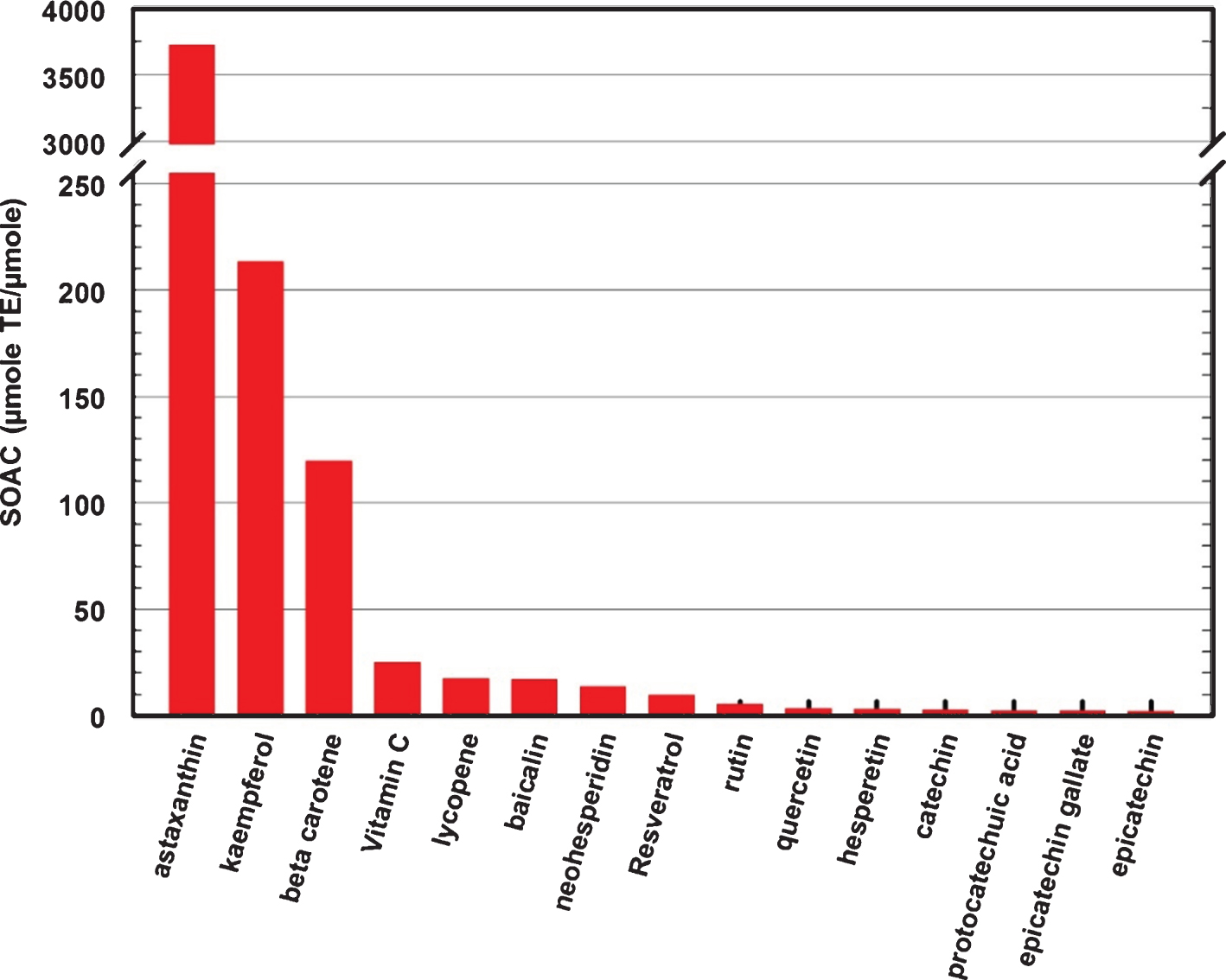
Fig.7
Superoxide Radical Antioxidant Capacity (SORAC) of pure compounds expressed as μmoles TE per μmole pure compound.
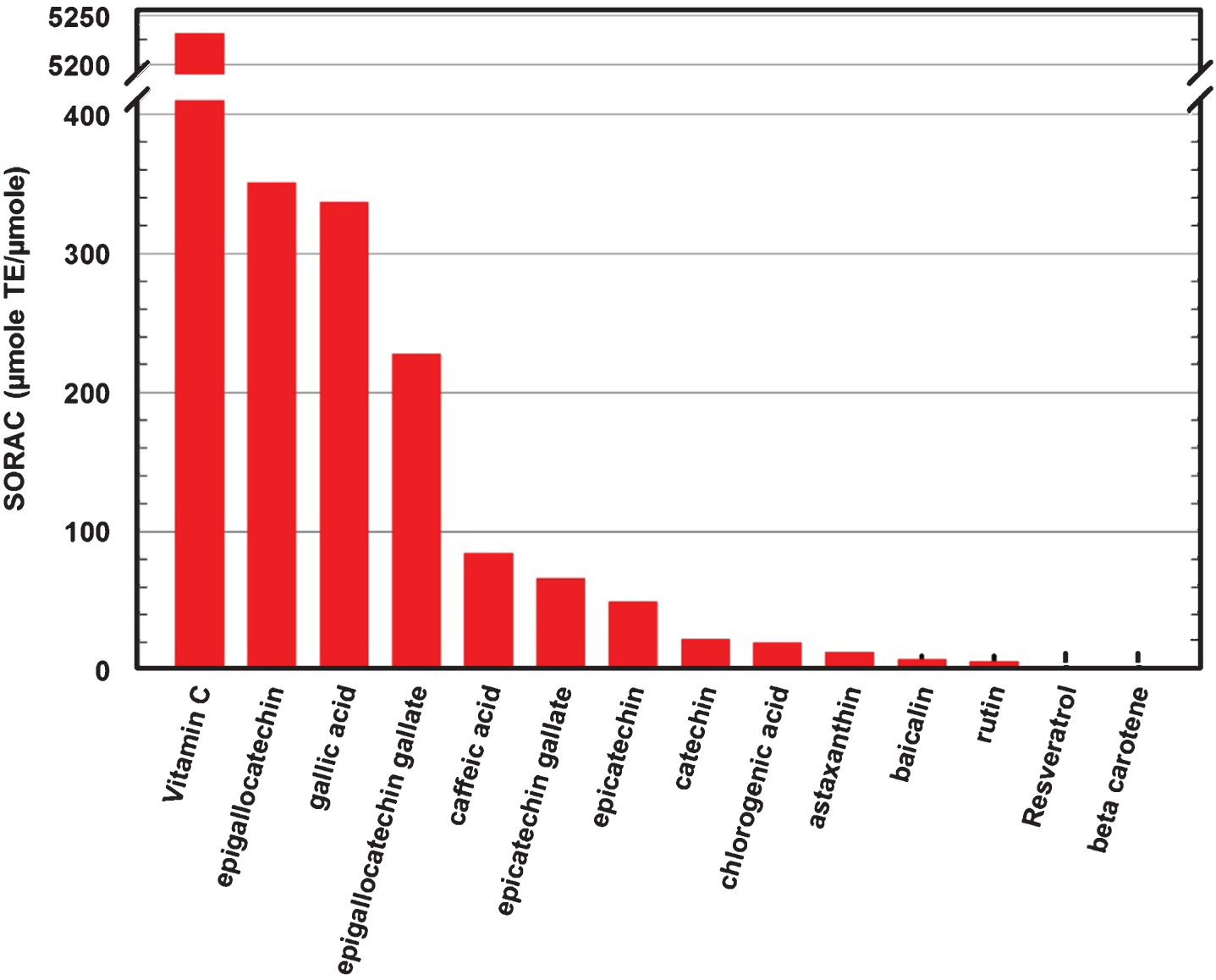
Table 1
Antioxidant Capacitya using Multiple Radicals (ORAC, HORAC, SORAC, NORAC and SOAC), Total ORACMR5 of selected Fruits and Berries
BERRY/FRUIT | Peroxyl (ORAC) | Hydroxyl (HORAC) | Peroxy-Nitrite NORAC | Superoxide Anion (SOARC) | Singlet Oxygen (SOAC) | TOTAL (ORACMR5) |
Blackberry, organic | 423 | 99 | 31 | 478 | 1971 | 3003 |
Aronia Juice Concentrate | 418 | 1007 | 59 | 674 | 416 | 2575 |
Acai E, oleracea Fruit pulp | 986 | 1357 | 17 | 169 | 71 | 2600 |
Blueberry, Wild 2013 | 880 | 823 | 71 | 189 | 386 | 2349 |
Blueberry, Wild, 2014 | 509 | 269 | 44 | 45 | 339 | 1206 |
Blueberry, Organic | 618 | 105 | 47 | 562 | 477 | 1809 |
Elderberry | 953 | 143 | 60 | 208 | 329 | 1694 |
Bilberry | 767 | 112 | 58 | 411 | 308 | 1655 |
Boysenberry | 216 | 76 | 35 | 391 | 495 | 1212 |
Cherry, Sweet | 202 | 125 | 6 | 26 | 504 | 864 |
Cherry, Tart | 149 | 16 | 10 | 77 | 520 | 772 |
Black Currants | 389 | 65 | 31 | 258 | 0 | 742 |
Strawberry | 356 | 101 | 26 | 239 | 0 | 722 |
Raspberry | 161 | 60 | 22 | 232 | 0 | 475 |
aAntioxidant capacity expressed as μmoles Trolox Equivalents per g Dry Matter, except for Aronia Juice. concentrate, which is expressed as μmoles Trolox Equivalents per mL from 2 production years. Predominate AC from the different radical/oxidant sources within a berry/fruit is highlighted in bold.
Table 2
Antioxidant Capacity of Mango, Cherry and Wild Blueberry whole fruit Fruitions and freeze dried Whole Blueberries. Antioxidant Capacity (ORAC, HORAC, SORAC, NORAC and SOAC) and Total ORACMR5 expressed as μmoles TE per g Dry Matter or per serving. The per cent contribution of to the total ORACMR5 is presented in a separate column
ITEM | Mango Fruition | Cherry Fruition | Wild Blueberry Fruition | Wild Blueberry | |||||||
/g DM | /Servinga | % b | /g DM | /Servinga | % b | /g DM | /Servinga | % b | /g DM | % b | |
ORAC | 28.2 | 282 | 1.5 | 149.6 | 2219 | 6.3 | 249.2 | 3826 | 14.5 | 509 | 42.0 |
HORAC | 68.4 | 686 | 3.6 | 173.3 | 2571 | 7.3 | 246.5 | 3780 | 14.3 | 269 | 22.0 |
NORAC | 2.2 | 22 | 0.1 | 7.6 | 113 | 0.3 | 16.9 | 259 | 1.0 | 44 | 4.0 |
SORAC | 0.0 | 0.0 | 0.0 | 25.0 | 371 | 1.1 | 47.2 | 724 | 2.7 | 45 | 4.0 |
SOAC | 1829 | 18329 | 95.0 | 2004 | 29723 | 84.9 | 1160 | 17780 | 67.4 | 339 | 28.0 |
ORACMR5 | 1928 | 19319 | 100.0 | 2360 | 34997 | 100.0 | 1721 | 26389 | 100.0 | 1206 | 100.0 |
Total Phenolicsc | 1.02 | — | — | 1.16 | — | — | 2.10 | — | — | — | — |
Total ACNc | 0.375 | — | — | 0.3 | — | — | 1.34 | — | — | — | — |
Polymeric Color, % | — | — | — | 37.2 | — | — | 18.3 | — | — | — | — |
Calories | — | 50 | — | — | 50 | — | — | 50 | — | 0.57 | — |
Sugars | — | 11 | — | — | 10 | — | — | 6 | — | — | — |
aServing size used was 87 mL. Antioxidant Capacity data expressed as μmoles Trolox Equivalents per serving. bPercent contribution to total ORACMR5 . cTotal anthocyanins (ACN) and total phenolic concentrations expressed as mg/g Dry matter.
Table 3
Antioxidant capacity of smoothies (Blueberry/Pomegranate and Mango/Pineapple). (Total ORACMR5 expressed as μmoles TE per serving) and the per centcontribution of ORAC, HORAC, SORAC, NORAC and SOAC to the totalORACMR5
ITEM | Blueberry/Pomgranatec | Mango/Pineappled | ||
/Servinga | % b | /Servinga | % b | |
ORAC | 2213 | 24.8 | 1140 | 12.3 |
HORAC | 398 | 4.5 | 265 | 2.9 |
NORAC | 93 | 1.0 | 689 | 7.4 |
SORAC | 0 | 0.0 | 0 | 0.0 |
SOAC | 6228 | 69.7 | 7155 | 77.4 |
ORACMR5 | 8931 | 100.0 | 9249 | 100.0 |
Calories | 220 | — | 210 | — |
Table 4
Antioxidant Capacity (ORAC, HORAC, SORAC, NORAC and SOAC and Total ORACMR5) of purified phenolic compounds expressed as μmoles TE per mg of pure compound
COMPOUND | ORAC | HORAC | NORAC | SORAC | SOAC | ORACMR5 |
CAROTENOIDS | ||||||
beta carotene | 21.74 | 0.34 | 0.18 | 0.37 | 222.85 | 245.47 |
lycopene | 8.88 | 11.66 | 1.99 | 0.00 | 32.63 | 55.17 |
astaxanthin | 227.28 | 13.44 | 0.85 | 21.98 | 6237.85 | 6501.41 |
FLAVONOLS | ||||||
quercetin | 14.49 | 5.92 | 3.44 | 0.00 | 11.41 | 35.27 |
kaempferol | 18.24 | 5.97 | 3.14 | 0.00 | 744.82 | 772.18 |
Rutin (quercetin-glycoside) | 7.01 | 7.37 | 2.67 | 10.43 | 9.04 | 36.53 |
FLAVONE | ||||||
baicalin | 30.22 | 716.89 | 370.42 | 18.28 | 38.33 | 1174.14 |
FLAVANOLS | ||||||
catechin | 22.05 | 7.41 | 5.13 | 77.34 | 9.75 | 121.68 |
epicatechin | 66.32 | 16.67 | 4.75 | 171.12 | 7.20 | 266.06 |
Epicatechin gallate | 8.14 | 5.15 | 0.36 | 150.39 | 5.33 | 169.38 |
Epigallocatechin (EGC) | 8.16 | 6.43 | 2.78 | 1146.06 | 2.91 | 1166.34 |
EGC gallate | 10.78 | 6.76 | 4.08 | 496.67 | 3.40 | 521.70 |
FLAVANONES | ||||||
naringenin | 35.37 | 4.44 | 0.62 | 0.00 | 1.54 | 41.98 |
hesperetin | 63.09 | 21.47 | 2.55 | 0.00 | 10.35 | 97.46 |
Neohesperidin(-glycoside) | 49.68 | 10.66 | 0.08 | 0.00 | 22.70 | 83.12 |
PROANTHOCYANIDIN | ||||||
Procyanidin dimer - A2 | 66320.90 | 464.34 | 6140.50 | 0.00 | 0.00 | 72925.74 |
PHENOLIC ACID | ||||||
protocatechuic acid | 33.80 | 6.75 | 1.36 | 0.00 | 17.65 | 59.56 |
gallic acid | 5.29 | 5.88 | 2.41 | 1980.37 | 2.35 | 1996.30 |
HYDROXYCINNAMIC ACID | ||||||
caffeic acid | 24.26 | 8.38 | 3.27 | 467.47 | 8.71 | 512.10 |
chlorogenic acid | 8.86 | 3.67 | 2.48 | 56.62 | 2.03 | 73.66 |
ferulic acid | 19.42 | 7.00 | 5.10 | 0.00 | 5.00 | 36.52 |
OTHER | ||||||
Vitamin C | 0.00 | 5.39 | 1.14 | 29707.02 | 142.23 | 29855.78 |
Resveratrol | 76.41 | 20.77 | 2.63 | 4.95 | 42.98 | 147.74 |
Avenanthramide 2ca | 36.82 | 16.24 | 3.04 | 47.73 | 5.06 | 108.88 |
Avenanthramide 2fa | 17.86 | 17.02 | 1.04 | 15.33 | 17.36 | 68.61 |
Avenanthramide 2pa | 23.30 | 19.92 | 1.08 | 8.33 | 20.09 | 72.72 |
aData from Yang et al., 2014 [37].