Anti-IL-8 monoclonal antibodies inhibits the autophagic activity and cancer stem cells maintenance within breast cancer tumor microenvironment1
Abstract
BACKGROUND:
Breast cancer tumor microenvironment (TME) is a promising target for immunotherapy. Autophagy, and cancer stem cells (CSCs) maintenance are essential processes involved in tumorigenesis, tumor survival, invasion, and treatment resistance. Overexpression of angiogenic chemokine interleukin-8 (IL-8) in breast cancer TME is associated with oncogenic signaling pathways, increased tumor growth, metastasis, and poor prognosis.
OBJECTIVE:
Thus, we aimed to investigate the possible anti-tumor effect of neutralizing antibodies against IL-8 by evaluating its efficacy on autophagic activity and breast CSC maintenance.
METHODS:
IL-8 monoclonal antibody supplemented tumor tissue culture systems from 15 females undergoing mastectomy were used to evaluate the expression of LC3B as a specific biomarker of autophagy and CD44, CD24 as cell surface markers of breast CSCs using immunofluorescence technique.
RESULTS:
Our results revealed that anti-IL-8 mAb significantly decreased the level of LC3B in the cultured tumor tissues compared to its non-significant decrease in the normal breast tissues.
Anti-IL-8 mAb also significantly decreased the CD44 expression in either breast tumors or normal cultured tissues. While it caused a non-significant decrease in CD24 expression in cultured breast tumor tissue and a significant decrease in its expression in the corresponding normal ones.
CONCLUSIONS:
Anti-IL-8 monoclonal antibody exhibits promising immunotherapeutic properties through targeting both autophagy and CSCs maintenance within breast cancer TME.
1.Introduction
Breast cancer is the most common cancer affecting women worldwide. It remains the main cause of cancer-related deaths in women, despite the advanced screening methods for early detection and the development of novel treatment strategies [1].
Autophagy is a distinct type of programmed cell death. It is a tightly regulated catabolic process that has a major role in cell regeneration and homeostasis [2]. It was reported that autophagy plays a dual role in the development of cancer cells, where at early stages of tumor development, it acts as an inhibitor of tumorigenesis, but in advanced stages, it is involved in the preservation, growth, and proliferation of tumor cells [3]. LC3B is a specific biomarker of autophagy, that is associated with the formation of autophagosomes. LC3B synthesis and activation are controlled by autophagy-associated genes such as ATG3, and ATG7. Its expression is reported to be upregulated in different malignancies making it a useful indicator for autophagy monitoring [4].
The tumor microenvironment (TME) plays an important role in tumor progression. The vital role of its resident cells and cytokines was reported to promote tumor invasion and metastasis [5]. Cancer stem cells (CSCs) are one of the most vital members within the TME, which have the ability of self-renewal, proliferation, and differentiation. They are known to be involved in the pathogenesis of tumors including initiation, progression, metastasis, recurrence, and chemoresistance [6].
Breast cancer stem cells (BCSCs) are characterized by high expression of the transmembrane glycoprotein CD44 and a low or no expression of the glycosylated mucin-type protein (CD44+/CD24−). CD44 is a glycoprotein located on the cell surface which is known to play a vital role in cell–cell interactions, cell adhesion, and migration. CD24 is a cell surface protein responsible for signal transduction. The percentage of both markers varies among different types of breast cancers and breast cancer cell lines [7]. CD44+CD24−BCSCs that exhibit enhanced invasive properties are located at the invasive edge of breast tumors and are characterized as mesenchymal-like BCSCs [8].
Interleukin-8 (IL-8) is a pro-inflammatory, angiogenic chemokine that plays a fundamental role in immune modulation within breast cancer TME [9]. Overexpression of IL-8 is associated with the oncogenic signaling pathways, increased tumor growth, metastasis, and poor prognosis [10]. Moreover, the cytokine profile of tumor-associated macrophages showed that IL-8 is the most abundant cytokine released in advanced breast cancer patients [10]. In this regard, utilizing neutralizing antibody against IL-8 was revealed to decrease tumor growth, angiogenesis, and metastasis [11].
However, the studies that investigate the link between CSCs, autophagy and IL-8 in breast cancer TME are limited. Therefore, we aimed in the current study to investigate the autophagic activities and CSC maintenance in our own designed ex vivo breast cancer tumor tissue culture system and to evaluate the effect of supplementing the system with anti-IL-8 monoclonal antibodies (mAbs) on both parameters.
2.Subjects and methods
2.1.Subjects
The current study was approved by the ethical committee of the medical research institute, Alexandria University, Egypt and confines to the provisions of the declaration of Helsinki. It included 15 females who were scheduled for modified radical mastectomy for histologically proved breast cancer. Immune-compromised patients and those with immunologic mediated disorders were excluded. Full history was taken from each patient, in addition, the tumor size and different clinicopathological parameters was recorded after surgery.
2.2.Methods
2.2.1.Tissue culture of tumor and normal breast tissues
Fresh tissue samples of breast tumor/normal (peritumoral tissue obtained from the same excised breast from a distant area outside the safety margins) were obtained from each patient immediately after surgery and maintained separately in RPMI organ transportation medium until use for the tissue culture.
Under aseptic conditions, the breast tissue was sliced and added to a 96-well culture plate according to the following design:
Breast tumor tissues cultured in complete MEBM media.
Breast tumor tissues cultured in complete MEBM media supplemented with 1 μg/ml anti-IL8-mAb.
Breast normal tissues (peritumoral tissue obtained from the same excised breast from a distant area outside the safety margins) in complete MEBM media.
Breast normal tissues (peritumoral tissue obtained from the same excised breast from a distant area outside the safety margins) cultured in complete MEBM media supplemented with 1 μg /ml anti IL-8 mAb.
The 96 well culture plates were then incubated at 37 °C in a constant atmosphere of 5% CO2 for 72 hours. Afterward, they were fixed in 10% phosphate-buffered formalin PH 7.4 for 24 hours. Then they were dehydrated, and preserved in paraffin-embedded blocks.
2.2.2.Tissue preparation for immunofluorescence assay
Tissue sections were deparaffinized, rehydrated and then immersed in retrieval sodium citrate buffer 0.01 M, pH: 6.0, heated at 100 °C, and washed with PBS. Slides were finally incubated with 1% fetal calf serum (Thermo Biochemicals, USA) for half an hour to reduce the non-specific binding of the secondary antibody [12]. Expression of LC3B was detected as a specific biomarker of autophagy while CD44 and CD24 were detected as cell surface markers of BCSCs.
2.2.3.Immunofluorescence detection of LC3B
Immunofluorescence detection of LC3B expression in fixed sections of cultured tumor/normal tissues was done by the deparaffinization of the tissue slides as previously described, followed by the incubation of the slides with LC3B primary monoclonal antibody (Invitrogen Thermofisher scientific, USA) (with dilution 1:100) overnight at 4 °C. The slides were then washed and incubated with the secondary antibody Alexaflour 488-conjugated anti-rat IgG (minimal cross-reaction to human, mouse, horse, bovine, and rabbit serum proteins) (Jackson Laboratories, USA) in a dark room for one hour, washed three times by PBS and finally mounted by fluorescent mounting medium containing DAPI (eBioscience, UK).
2.2.4.Immunofluorescence detection of CD44 and CD24 expression
Immunofluorescence detection of CD44 and CD24 expression in fixed sections of cultured tumor/normal tissues was done by performing simultaneous double immunofluorescence staining according to the manufactures’ manual [13]. The two primary antibodies were mixed in phosphate buffer saline (CD24 at 1:100, CD44 at 1:50) (ThermoFisher laboratories, USA), applied on the tissues and then incubated at 4 °C overnight.
The secondary antibodies, Alexaflour 488-conjugated anti-mouse IgG (ThermoFisher laboratories, USA) and Alexaflour 594-conjugated anti-rat IgG (ThermoFisher laboratories, USA) were mixed and diluted in PBS (1:300). The mixture was then added to the tissue sections, incubated in the dark for 60 minutes and then washed twice in PBS for 5 minutes. Finally the slides were mounted by fluorescent mounting medium containing DAPI (eBioscience, UK).
2.2.5.Visual assessment of immunofluorescence staining
Visual assessment of the immunofluorescence stain was done by examining the slides using correct excitation and filters on a fluorescence microscope (lieca, Italy). The slides were then photographed and the fluorescence intensity of the stain was calculated using ImageJ photos analyzing software as follows [14]:
2.2.6.Statistical analysis
Statistical analysis of the results was carried out using IBM software package version 22.0 (Armonk, NY: IBM Corp) [15]. Correlations were used to analyze different parameters. Results were considered to be significantly judged at the 5% level (p < 0.05).
Mean, median, and standard deviation tests were used for the samples’ descriptive analysis, and the Wilcoxon Signed Ranks test was used to test the significance of the difference between the control and the test groups. Statistical tests were used to evaluate the correlation between the different assessed parameters and the clinicopathological parameters of the patients as follows:
The Spearman Rho test (r): between two continuous data sets.
The Kendall rank correlation coefficient (𝜏): between continuous and ordinal data sets.
The Point-Biserial Correlation test (r): between continuous data and nominal data sets.
3.Results
3.1.Clinicopathological parameters
The patients’ clinicopathological parameters descriptive analysis revealed that, the age of the patients enrolled in the current study ranged from 41 to 71 years old with a mean age of 56.47 ± 8.37. The majority of the patients (93.33%) had invasive ductal carcinoma while only one patient had invasive lobular carcinoma, all of which were grade II. 80% of patients showed vascular invasion of tumor cells, but on the other hand, 66% of the patients only demonstrated tumor cell invasion for axillary lymph nodes. The excised tumor size of the patients ranged from 2 to 8 cm with a mean size of 4.37 ± 2.09.
Regarding hormonal receptor expression, 84.33% of patients enrolled in the current study showed positive expression for estrogen and progesterone hormonal receptors with different intensities (Table 1).
Table 1
The clinicopathological data of the patients enrolled in the study
Clinicopathological parameters | No | % |
Age | ||
<50 | 4 | 26.67 |
50–60 | 6 | 40.00 |
≥60 | 5 | 33.33 |
Min.–Max. | 41.00–71.00 | |
Mean ± SD | 56.47 ± 8.37 | |
Median | 59 | |
Histopathologic type | ||
Invasive lobular carcinoma | 1 | 6.67 |
Invasive ductal carcinoma | 14 | 93.33 |
Histologic grade | ||
II | 15 | 100.0 |
Vascular invasion | ||
Negative vascular invasion | 3 | 20.00 |
Positive vascular invasion | 12 | 80.00 |
Tumor size | ||
≤2 cm | 3 | 20.00 |
>2–5 cm | 7 | 46.67 |
>5 cm | 5 | 33.33 |
Min. – Max. | 2.00–8.00 | |
Mean ± SD | 4.37 ± 2.09 | |
Median | 4.00 | |
L.N invasion | ||
Negative L.N. | 5 | 33.33 |
Positive L.N. | 10 | 66.67 |
Min.–Max. | 0–21 | |
Mean ± SD | 4 ± 5.92 | |
Median | 2.00 | |
Pathological stage | ||
I | 3 | 20.0 |
II | 7 | 46.7 |
III | 5 | 33.3 |
Hormonal status | ||
ER | ||
Negative | 2 | 16.67 |
+ | 2 | 16.67 |
++ | 4 | 33.33 |
+++ | 4 | 33.33 |
PR | ||
Negative | 2 | 16.67 |
+ | 4 | 33.33 |
++ | 4 | 33.33 |
+++ | 2 | 16.67 |
[i] L.N.: Lymph node. ER: Estrogen receptor expression. PR: Progesterone receptor expression.
3.2.Immunofluorescence detection of autophagy according to detected LC3B expression level
3.2.1.Autophagy levels in anti-IL-8 mAb treated breast tumor and normal tissues versus control tissue cultures
A significant decrease in the level of autophagy was detected in the tumor tissues cultured in the presence of anti-IL-8 mAb compared to those cultured in the absence of anti-IL-8 mAb (p = 0.002). On the other hand, no significant difference was detected between the level of autophagy in breast normal tissues either cultured in the presence or absence of anti-IL-8 mAb (p = 0.820) (Table 2 Figs 1,2,3).
Table 2
LC3B fluorescence intensity in the cultured tumor and normal breast tissue
Florescence intensity (pixel/μm2) | T (n = 15) | T + anti-IL-8 (n = 15) | N (n = 15) | N + anti-IL-8 (n = 15) |
>2 | 20% | 80% | 73.3% | 73.3% |
2–<5 | 40% | 20% | 20% | 20% |
5–<10 | 26.6% | 0% | 6.7% | 6.7% |
>10 | 13.4% | 0% | 0% | 0% |
MIN–MAX | 0.073–14.08 | 0.087–3.847 | 0.054–8.145 | 0.061–6.89 |
Mean ± SD | 5.03 ± 4.01 | 1.56 ± 0.95 | 1.89 ± 1.89 | 1.85 ± 1.65 |
Z(P) | −3.124∗ (0.002∗) | −0.227 (0.820) |
[i] Z = Wilcoxon signed ranks test. ∗: Statistically significant at p ≤ 0.05.
Fig. 1.
Graphical representation of autophagy level via LC3b expression within the tumor and normal tissue culture systems. Data presented as mean ± SD. ∗Significant from tumor tissue cultured alone (T) (T versus T + anti-IL-8 p = 0.002) & (T versus N p = 0.05). no significant difference observed between (N versus N + anti-IL-8 p = 0.82) or (T + anti-IL-8 versus N + anti-IL-8 p = 0.865).
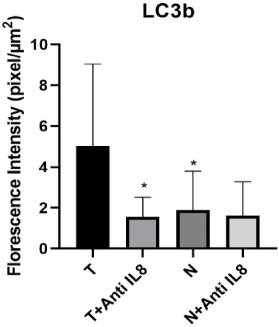
Fig. 2.
Representative image of the immunofluorescence analysis of LC3B expression in breast tumor tissue culture system examined and imaged by Leica Confocal microscope (Confocal microscopy 200×). a,b,c immunofluorescence staining in Tumor tissues cultured without anti-IL8 mab. a: DAPI nuclear staining (blue). b: LC3B staining showing fluorescence intensity >10 pixel/μm2 for Alexafluor 488 staining (Green) indicating high LC3B expression. c: Merged image. d,e,f: immunofluorescence staining in tumor tissues cultured with anti-IL8 mab. d: DAPI nuclear staining (blue). e: LC3B staining showing fluorescence intensity <2 pixel/μm2 for Alexafluor 488 staining (Green) indicating low LC3B expression. c: Merged image.
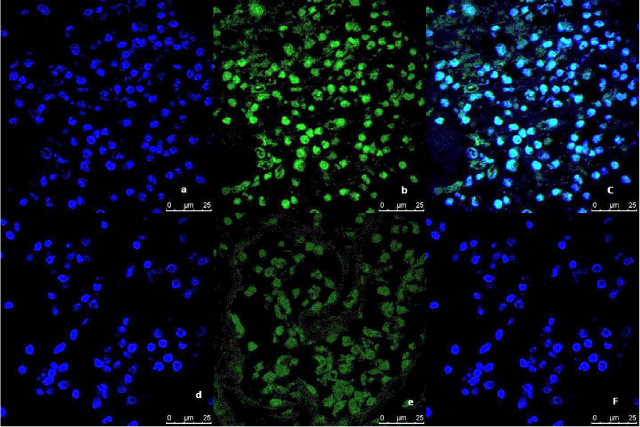
Fig. 3.
Representative image of the immunofluorescence analysis of LC3B expression in breast normal tissue culture system, examined and imaged by Leica Confocal microscope (Confocal microscopy 200×). a,b,c: immunofluorescence staining in normal tissues cultured without anti-IL8 mab. a: DAPI nuclear staining (blue). b: LC3B staining showing fluorescence intensity <2 pixel/μm2 for Alexafluor 488 staining (Green) indicating low LC3B expression. c: Merged image. d,e,f: immunofluorescence staining in normal tissues cultured with anti-IL8 mab. d: DAPI nuclear staining (blue). e: LC3B staining showing fluorescence intensity <2 pixel/μm2 for Alexafluor 488 staining (Green) indicating low LC3B expression. c: Merged image.
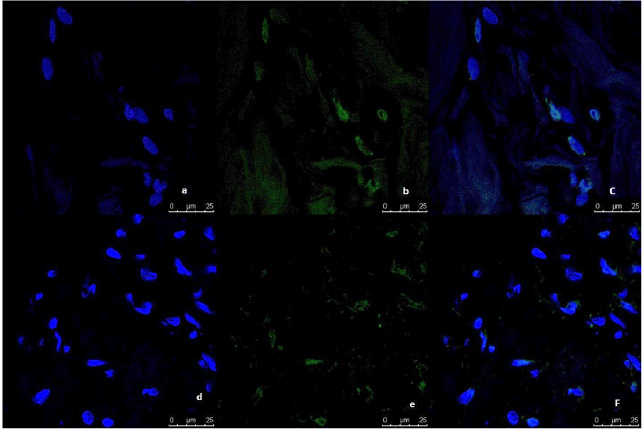
3.3.Autophagy level within the different tissue cultures vs their normal counterparts
According to detected LC3B fluorescence intensity, the study results showed a significant increase in the autophagy levels in breast tumor tissues cultured without anti-IL-8 mAb compared to the corresponding normal ones (T vs N) (p = 0.005).
In addition, no significant difference was detected in the autophagy levels between breast tumor tissues cultured in the presence of anti-IL-8 mAb and breast normal tissue cultured with anti-IL-8 mab (p = 0.865) (Figs 1,2,3).
3.4.Assessment of breast cancer stem cells maintenance according to detected cell surface markers CD44/CD24 expression level
3.4.1.CD44 fluorescence intensity in tumor and normal cultured breast tissues
The results of the immunofluorescence assessment of CD44 expression showed a statistically significant decrease in the level of CD44 expression in the breast tumor tissues cultured in the presence of anti-IL-8 mAb compared to tumor tissues cultured in the absence of anti-IL-8 mAb (p = 0.012). Similarly, a statistically significant decrease in the CD44 expression was also noticed in the corresponding normal tissue cultures supplemented with anti-IL-8 mAb compared to the un-supplemented ones (p = 0.023) (Table 3, Figs 4,5,6).
Table 3
CD44 & CD24 expression levels in cultured tumor and normal breast tissue cultured in the presence or absence of anti-IL-8 monoclonal antibodies
Florescence intensity (pixel/μm2) | CD44 expression (n = 15) | CD24 expression (n = 15) | ||||||
T | T + anti-IL-8 | N | N + anti-IL-8 | T | T + anti-IL-8 | N | N + anti-IL-8 | |
< 2 | 20% | 66.67% | 53.34% | 93.34% | 40% | 60% | 66.67% | 93.33% |
2– < 5 | 40% | 26.67% | 13.33% | 6.66% | 20% | 20% | 13.33% | 0% |
5– < 10 | 6.67% | 6.66% | 20% | 0 | 33.33% | 13.33% | 6.67% | 0% |
> 10 | 13.33% | 0% | 13.33% | 0 | 6.67% | 6.67% | 13.33% | 6.67% |
MIN–MAX | 0.120–15.388 | 0.049–6.856 | 0.039–9.450 | 0.059–3.59 | 0.041–11.61 | 0.054–11.34 | 0.050–11.29 | 0.041–13.7 |
Mean ± SD | 5.241 ± 4.609 | 2.137 ± 1.755 | 3.325 ± 3.129 | 1.165 ± 0.87 | 3.840 ± 3.62 | 2.716 ± 3.07 | 2.845 ± 3.970 | 1.157 ± 3.5 |
Z(P) | −2.499∗ (0.012∗) | −2.272∗ (0.023∗) | −0.852 (0.394) | −2.329∗ (0.020∗) |
[i] Z = Wilcoxon signed ranks test. ∗: Statistically significant at p ≤ 0.05.
Fig. 4.
Graphical representation of CSCs maintenance level via CD44/CD24 expression within the tumor and normal tissue culture systems. Data presented as mean ± SD. CD44: ∗significant from T, #significant from N, no significant difference observed between (T + anti-IL-8 versus N + anti-IL-8). CD24: ∗significant from N, no significant difference observed between (T versus T + anti-IL-8) or (T + anti-IL8 versus N + anti-IL8).
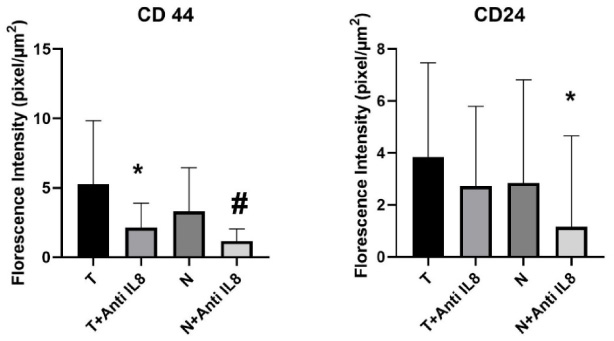
Fig. 5.
Representative image of immunofluorescence analysis of CD44/CD24 expression in breast tumor tissue culture systems, examined and imaged by Leica Confocal microscope (Confocal microscopy 200×). a,b,c,d: Immunofluorescence staining in tumor tissues cultured without anti-IL8 mab. a: DAPI nuclear staining (blue). b: CD44 staining showing fluorescence intensity 5–10 pixel/μm2 for Alexafluor 596 staining (red) indicating moderate CD44 expression. c: CD24 staining showing fluorescence intensity <2 pixel/μm2 for Alexafluor 488 staining (Green) indicating low CD24 expression. d: Merged image. e,f,g,h: Immunofluorescence staining in normal tissues cultured with Anti-IL8 mab. e: DAPI nuclear staining (blue) f: CD44 staining showing fluorescence intensity <2 pixel/μm2 for Alexafluor 596 staining (red) indicating low CD44 expression. g: CD24 staining showing fluorescence intensity 2–5 pixel/μm2 for Alexafluor 488 staining (Green) indicating mild CD24 expression. d: Merged image.
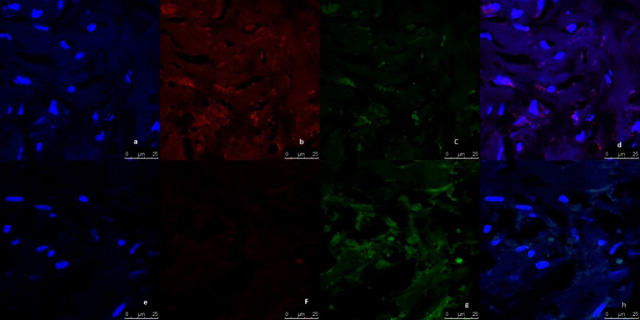
Fig. 6.
Representative image of immunofluorescence analysis of CD44/CD24 expression in breast normal tissue culture systems, examined and imaged by Leica Confocal microscope (Confocal microscopy 200×). a,b,c,d immunofluorescence staining in normal tissues cultured without anti-IL8 mab. a: DAPI nuclear staining (blue). b: CD44 staining showing fluorescence intensity <2 pixel/μm2 for Alexafluor 596 staining (red) indicating low CD44 expression. c: CD24 staining showing fluorescence intensity <2 pixel/μm2 for Alexafluor 488 staining (Green) indicating low CD24 expression. d: Merged image. e,f,g,h: immunofluorescence staining in normal tissues cultured with Anti-IL8 mab. e: DAPI nuclear staining (blue). f:CD44 staining showing fluorescence intensity <2 pixel/μm2 for Alexafluor 596 staining (red) indicating low CD44 expression. g: CD24 staining showing fluorescence intensity <2 pixel/μm2 for Alexafluor 488 staining (Green) indicating low CD24 expression. d: Merged image.
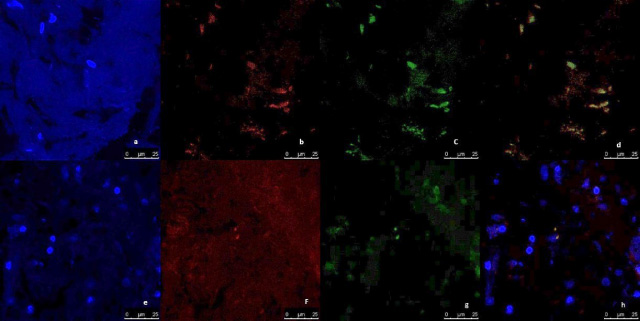
3.4.2.CD44 fluorescence intensity in cultured tumor tissues versus their normal counterparts
No significant difference was observed in the expression level of CD44 in tumor tissues cultured in the presence of anti-IL-8 mAb compared to corresponding normal tissues (T + anti-IL-8 versus N + anti-IL-8). Also, no significant difference was observed in the CD44 expression in tumor cultured in the absence of anti-IL-8 mAb compared to corresponding normal ones (T versus N) (p = 0.069 & p = 0.191 respectively) (Fig. 4).
3.4.3.CD24 fluorescence intensity in cultured breast tumor and normal tissues
No significant difference was observed in the CD24 expression levels in the breast tumor tissues cultured with anti-IL-8 mAb compared to those cultured without anti-IL-8 mab (p = 0.394). Normal breast tissue cultures showed a significant decrease in the level CD24 expression in cultures supplemented with anti-IL-8 mAb compared to the un-supplemented ones (p = 0.020) (Table 2, Figs 4,5,6).
3.4.4.CD24 fluorescence intensity in the breast tumor tissues versus their normal counterparts
No significant difference was also observed in the expression level of CD24 between tumor and normal tissues cultured without IL-8 mab, and as well, between tumor and normal tissues cultured in the presence of anti-IL-8 mab (p = 0.650 & p = 0.053 respectively) (Fig. 4).
3.4.5.Correlation between autophagy and cancer stem cells maintenance within the cultured breast tumor and normal tissues
Statistical tests done to reveal the correlation between the studied different parameters showed no meaningful correlation between autophagy level & CD44 expression within the breast tumor tissue culture supplemented with anti-IL-8 mAb (r = 0.04643, p = 0.869), as well as, between autophagy level & CD24 expression within the tumor tissues cultured with anti-IL-8 mAb (r = −0.18929, p = 0.49926).
Similar results were observed within the breast normal tissue cultures where no correlation was observed between autophagy level & CD44 expression (r = 0.11628, p = 0.6798) and between autophagy level & CD24 expression within the breast normal tissues cultured with anti-IL8 mab (r = −0.125, p = 0.65714) (Table 4).
Table 4
Statistical correlation between immunofluorescence intensity LC3B & expression level of CD44 and CD24 levels within the breast tumor and normal culture systems supplemented with anti-IL-8 mab
LC3B expression | Immunofluorescence intensity | ||||
CD44 | CD24 | ||||
T + anti-IL-8 | N + anti-IL-8 | T + anti-IL-8 | N + anti-IL-8 | ||
T + anti-IL-8 | r | 0.04643 | – | −0.18929 | – |
p | 0.869 | – | 0.49926 | – | |
N + anti-IL-8 | r | – | 0.11628 | – | −0.125 |
p | – | 0.6798 | – | 0.65714 |
[i] ∗p value for spearman rho test. ∗∗Statistically significant at p ≤ 0.05.
3.4.6.Correlations between the levels of autophagy, cell surface markers CD44/CD24 expression, and clinicopathological parameters among breast cancer patients
The correlation between the levels of autophagy, cell surface markers CD44/CD24 expression, and clinicopathological parameters among breast cancer patients is summarized in Table 5.
Table 5
Statistical correlation between the expression of Lc3B, CD44, and CD24 expression and some of the clinicopathological parameters among breast cancer patients enrolled in the current study
Clinicopathological parameters | Untreated tumor tissues | Anti-IL-8 treated tumor tissues | ||||||||||
LC3B (pixel/μm2) | CD44 (pixel/μm2 ) | CD24 (pixel/μm2 ) | LC3B (pixel/μm2 ) | CD44 (pixel/μm2 ) | CD24 (pixel/μm2 ) | |||||||
r/𝜏 | p | r/𝜏 | p | r/𝜏 | p | r/𝜏 | p | r/𝜏 | p | r/𝜏 | p | |
Age (r) | −0.570 | 0.027∗ | 0.290 | 0.294 | −0.021 | 0.939 | −0.308 | 0.264 | −0.295 | 0.285 | 0.084 | 0.766 |
No. of LN involved (r) | −0.118 | 0.676 | −0.100 | 0.722 | −0.447 | 0.095 | −0.284 | 0.304 | −0.100 | 0.722 | −0.341 | 0.214 |
Total no of LN (r) | 0.396 | 0.144 | −0.718 | 0.003* | −0.379 | 0.164 | −0.057 | 0.839 | 0.133 | 0.637 | −0.348 | 0.203 |
Tumor size (r) | −0.204 | 0.465 | 0.203 | 0.468 | −0.129 | 0.647 | −0.254 | 0.361 | 0.151 | 0.592 | 0.305 | 0.270 |
Pathological stage | −0.087 | 0.758 | 0.309 | 0.263 | −0.235 | 0.399 | −0.189 | 0.500 | 0.039 | 0.891 | 0.058 | 0.838 |
Vascular invasion (r)∗∗∗ | −0.07 | 0.786 | 0.051 | 0.855 | 0.2 | 0.455 | 0.463 | 0.082 | 0.015 | 0.955 | 0.174 | 0.532 |
ER status (𝜏) | 0.240 | 0.252 | 0.199 | 0.568 | 0.336 | 0.107 | −0.380 | 0.069 | −0.314 | 0.132 | 0.119 | 0.560 |
PR status (𝜏) | 0.109 | 0.603 | 0.011 | 0.599 | 0.163 | 0.034** | −0.271 | 0.194 | −0.358 | 0.086 | 0.314 | 0.132 |
[i] ∗p-value for spearman rho test, statistically significant at p ≤ 0.05. ∗∗p-value for Kendall rank correlation coefficient, statistically significant at p ≤ 0.05. ∗∗∗Point-Biserial correlation test (r).
Statistical correlations done in the untreated tumor tissue cultures groups revealed a significant association between LC3B expression and the patient’s age (p = 0.027), between CD44 expression and the number of involved lymph nodes (p = 0.003), and between CD24 expression and PR status (p = 0.034). Moreover, the statistical correlations done within the treated tumor tissue culture group didn’t reveal any significant association with any of the clinicopathological parameters (Table 5).
4.Discussion
The interactions between the cellular populations of TME with each other (such as immune cells, cancer cells and cancer stem cells) or with the TME molecular components such as cytokines and cell surface molecules, decide the fate of disease prognosis, cancer invasion, metastasis, recurrence and also treatment resistance. Massive studies focused on cancer stem cells (CSCs) maintenance and programmed cell death including apoptosis and autophagy as main players in the immune modulations within the TME [16].
There is a controversy regarding the role of autophagy in cancer. Many studies have reported that autophagy can either promote or inhibit tumorigenesis [17], depending on many factors such as tumor type and stage [18]. The results of the current study revealed that the level of detected LC3B fluorescence intensity showed a significant increase in control breast tumor tissues compared to the corresponding normal ones. Putting into consideration that the majority of cases enrolled in the current study were invasive ductal carcinoma stage 2, our result comes in line with previous studies reported that autophagy has tumor suppressor properties in early tumorigenesis by different mechanisms including the activation of P53 gene pathway [19] and, Beclin-1 and Atg12 autophagy genes mediated apoptosis activation [20,21], but once the tumor is established autophagy is reported to promote tumorigenesis and tumor survival [22].
The possible mechanisms of autophagy tumor-promoting activities include, prevention of cancer DNA damage [22], sustaining cancer cell survival during starvation, inhibition of inflammation, and supporting tumor metastasis [23]. Several studies associated the overexpression of LC3B autophagy marker with various cancer types metastasis including breast cancer [24], which emphasizes, along with the previous findings, the importance of autophagy as a target for immunotherapy.
In addition, the decreased LC3B expression in the breast tumor tissues cultured in the presence of anti-IL-8 mAb compared to control cultured tumor tissues (p = 0.002), demonstrates that targeting autophagy within the TME-mimicking tissue culture system in the current study by anti-IL-8 mAb significantly decreased the autophagic activities. Accordingly, enriching the breast cancer TME by anti-IL-8 mAb could be considered an effective immunotherapeutic strategy.
The vital role of IL-8 as an important chemokine in enhancing the recruitment of suppressive immune cells such as T regs and MDSCs to the TME has been well established [25]. In addition, using anti-IL-8 mAb to neutralize the tumor-supporting activities of IL-8, by preventing the binding of IL-8 to its receptors CXCR1 and CXCR2, which in turn inhibits the downstream activation of IL-8 mediated signaling, is suggested to be an effective immunotherapeutic strategy [25]. Moreover, previous preclinical and clinical trials showed that blocking IL-8 activities using anti-IL-8 mAb has promising antitumor effects, by inhibiting metastasis through different mechanisms, such as, modulating the myeloid cells infiltration within the TME [26], down-regulation of matrix metalloproteases and NF kβ [27], and enhancing immune-mediated lysis of tumor cells via NK cells and cytotoxic T lymphocytes [26].
Noteworthy, the current study is the first study that investigates the effect of IL-8 blockade on autophagy in breast cancer TME. The link between autophagy and IL-8 in breast cancer TME is not fully studied so far. But several studies reported the role of IL-8 in the induction of autophagy in other physiological and pathological conditions, such as the role of autophagy in the induction of inflammation and inflammatory cytokines production including IL-6 & IL-8 in hepatitis B virus via the activation of NF kβ pathway [28], the ability of IL-8 to enhance the autophagy of neutrophils in the synovial fluid in rheumatoid arthritis [29], and its ability to induce autophagy in bone marrow mesenchymal stem cells via Akt-STAT3 pathway [30]. In addition, the fact that anti-IL-8 mAb is well known for its activity to prevent metastasis [9], gives it the upper hand on other autophagy inhibitors such as chloroquine, that was reported to induce tumor regression in only non-metastatic cancers, with no efficacy in the metastatic forms [31].
Furthermore, the insignificant difference between the detected autophagy levels in cultured breast normal tissues, either in the presence or absence of anti-IL-8 mAb refers to the specificity and safety of using anti-IL-8 mAb as it does not affect the level of autophagy in breast normal tissues.
Regarding CD44 expression detected in our breast tumor tissues culture system, the assessed immunofluorescent intensity for CD44 showed a significant decrease in its expression level within the breast tumor tissues cultured in the presence of anti-IL-8 mAb compared to the control tumor tissues. This decreased level of CD44 expression indicates the effectiveness of the anti-IL-8 mAb treatment in reducing the stemness of the tumor cells within the breast TME.
Several studies reported the role of IL-8 in the maintenance of stemness and induction of epithelial-mesenchymal transition (EMT), by activating the downstream signaling pathways such as PLC-PKC, PI3K-AKT, Wnt/β-catenin, FAK/Src and MAPK pathways in different types of cancers [9,32,33].
Similarly, our results also revealed a significant decrease in the CD44 expression in the breast normal tissue cultures supplemented with anti-IL-8 mAb compared to those detected in un-supplemented ones. This result indicates that anti-IL-8 mAb can decrease the level of CD44 expression and maintenance of stemness within the TME not only in established tumors but also in early initiating ones. Keeping in mind that the normal breast tissues used for the breast normal tissue cultures designed in the current study are obtained from the same excised breast containing the primary tumor. So, it is expected that these breast normal tissues may contain CD44-expressing cancer-initiating cells. Moreover, the treatment by anti-IL-8 monoclonal antibody acted on the cancer-initiating cells in the breast normal tissues in the same way as it acted on the CD44 and CD24 expression in tumor cells. this observation is beneficial in considering anti-IL-8 monoclonal antibodies as a promising immunotherapeutic agent to target cancer stem cells and stemness properties within breast tumor tissues in addition to its proven previously metastatic blocking effect.
Previous studies reported that C44+/CD24−BCSCs are much more abundant at the invasive edge of the breast tumor rather than its core supporting the suggestion that normal breast tissues adjacent to the primary tumor may contain C44+/CD24− BCSCs [8].
CD24 is a glycosyl-phosphatidyl-inositol (GPI)-anchored glycoprotein, that is reported to have vital functions on different immune cells. In addition, it was reported to be over-expressed on different cancer cells and its expression is correlated with cancer progression and poor prognosis. Moreover, expression of CD24 on triple-negative breast cancer cell lines was observed to inhibit cancer cell phagocytosis leading to cancer progression and invasion [34].
The contradicted findings reported in the current study concerning the expression of CD24 as a marker of stemness within the breast cancer TME come in line with previous studies’ findings. Several studies had reported that breast CSCs population express C44+/CD24− surface markers and that their abundance is correlated with recurrence, metastasis, and therapy resistance [35,36]. Unlike CD44, CD24 was reported to be more expressed on differentiated breast cancer cells rather than progenitor cancer stem cells [37]. It was also reported that CD24+ cells are more liable for radiation-induced chromosomal instability than CD24− cells [38].
On the other hand, some studies reported that CD24 contributes to breast cancer cell stemness, invasion, and metastasis. Where its knockdown results in a decrease in the expression of the Notch1 signaling pathway, which in turn decreases breast cancer cell invasion, migration, and therapy resistance [39].
Several studies investigated the role of autophagy in CSC maintenance and the link between both and cancer metastasis. It was demonstrated that autophagy is upregulated in breast CSCs compared to breast cancer cell lines and transgenic mice, which gives the breast CSCs population its hemostatic, therapy resistance and increased migration potential [2]. The detailed mechanisms of that link are not fully elucidated yet. Recent studies suggested different signaling pathways that may induce autophagy-related CSCs maintenance such as PI3K/Akt/mTOR signaling pathway [40], P53/Zeb1 axis [41], and NF-kβ pathway [42].
Recently, Bie et al. (2021) reported that the interleukin-17 signaling cascade promotes the maintenance of CSCs via inducing Beclin-1 ubiquitination, resulting in their progression and self-renewal [43]. Taking into consideration that IL-17 expression is positively correlated to the expression of IL-6, IL-8, and VEGF expression in the TME [44]. Thus, the blockade of IL-8 within the TME in the current study, possibly targets the IL-17 pathway resulting in the decreased autophagy & CSCs population observed.
In addition, the TGF-β signaling pathway was reported to be involved in the induction of EMT, CSCs maintenance as well as autophagy by different mechanisms. It is reported to induce EMT by SMAD, NOTCH, WNT, INTEGRIN, and STAT3 pathways [45], and autophagy by increasing the expression of autophagy-related genes such as BECLIN1, ATG5, and ATG7 [46]. This pathway is also linked with IL-8 where TGF-β was reported to induce IL-8 and macrophage chemo-attractant protein-1 (MCP-1) production in inflammatory conditions [47].
According to the findings of the current study, we can conclude that the ability of anti-IL-8 mAb to target autophagy and CSCs within the breast cancer TME makes it a promising immunotherapeutic agent for breast cancer. Finally, the achievement of consequent studies using a higher number of breast cancer patients of different stages of the disease is recommended to properly identify the targeted patient populations of the proposed strategy. In addition, further studies including the effect of anti-IL8 on the different cells within the TME and the intracellular signaling pathways are very recommended.
Ethical statement and consent to participate
The current study was approved by the ethical committee of the medical research institute, Alexandria University, Egypt and confines to the provisions of the declaration of Helsinki. A written informed consent was provided by all subjects under study.
Conflict of interest
All of the authors declared no conflict of interest.
Data availability
The data supporting the findings of this study are available on request from the corresponding author. The data are not publicly available due to privacy.
5.Funding
This study is not funded by any institution or a funding body.
Author contribution
Abou Shousha SA conceived and designed the study. All the authors contributed to the material preparation, data collection, and analysis. The first draft of the manuscript was written by Abou Shousha SA, Medhat E, and Shahine Y and all authors commented on previous versions of the manuscript. All authors read and approved the final manuscript.
References
[1] | Monnot GC, , Romero P, Rationale for immunological approaches to breast cancer therapy, Breast, 37: : 187–195, (2018) . |
[2] | Nazio F, , Bordi M, , Cianfanelli V, , Locatelli F, , Cecconi F, Autophagy and cancer stem cells: Molecular mechanisms and therapeutic applications, Cell Death & Differentiation, 26: (4): 690–702, (2019) . |
[3] | Alizadeh J, , Lorzadeh S, , Ghavami S, Autophagy and cancer metastasis: A Trojan horse, J Invest Med, 69: (6): 1145–1147, (2021) . |
[4] | Meng YC, , Lou XL, , Yang LY, , Li D, , Hou YQ, Role of the autophagy-related marker LC3 expression in hepatocellular carcinoma: A meta-analysis, J Cancer Res Clin Oncol, 146: : 1103–1113, (2020) . |
[5] | Xiao Y, , Yu D, Tumor microenvironment as a therapeutic target in cancer, Pharmacology & Therapeutics, 221: : 107753, (2021) . |
[6] | Zeng X, , Liu C, , Yao J, , Wan H, , Wan G, , Li Y , Breast cancer stem cells, heterogeneity, targeting therapies and therapeutic implications, Pharmacological Res, 163: : 105320, (2021) . |
[7] | Gyan E, , Owiredu WKBA, , Fondjo LA, , Jackson AM, , Green AR, , Rahman GA, A review of the racial heterogeneity of breast cancer stem cells, Gene, 796–797, : (2021) . |
[8] | Vikram R, , Chou WC, , Hung SC, , Shen CY, Tumorigenic and metastatic role of CD44−/low/CD24−/low cells in luminal breast cancer, Cancers, 12: (5): 1239, (2020) . |
[9] | Abou Shousha SA, , Hussein B, , Shahine Y, , Fadali G, , Zohir M, , Hamed Y , Angiogenic activities of interleukin-8, vascular endothelial growth factor and matrix metalloproteinase-9 in breast cancer, Egypt J Immunol, 29: (3): 54–63, (2022) . . PMID: 35758969. |
[10] | Lin L, , Li L, , Ma G, , Kang Y, , Wang X, , He J, Overexpression of IL-8 and Wnt2 is associated with prognosis of gastric cancer, Folia Histochemica et Cytobiologica, 60: (1): 66–73, (2022) . |
[11] | Hajizadeh F, , Aghebati Maleki L, , Alexander M, , Mikhailova MV, , Masjedi A, , Ahmadpour M , Tumor-associated neutrophils as new players in immunosuppressive process of the tumor microenvironment in breast cancer, Life Sci, 264: : 118699, (2021) .. |
[12] | Abou-shousha S, , Moaz M, , Sheta M, , Motawea MA, An approach to breast cancer immunotherapy: The apoptotic activity of recombinant anti-interleukin-6 monoclonal antibodies in intact tumor microenvironment of breast carcinoma, Scand J Immunol, 83: : 427–437, (2016) . |
[13] | Abou Shousha S, , Baheeg S, , Ghoneim H, , Zoheir M, , Hemida M, , Shahine Y, The effect of Fas/FasL pathway blocking on apoptosis and stemness within breast cancer tumor microenvironment (preclinical study), Breast Disease, 42: (1): 163–176, (2023) . |
[14] | Jensen EC, Quantitative analysis of histological staining and fluorescence using ImageJ, Anatomical Record, 296: (3): 378–381, (2013) . |
[15] | Kirkpatrick LA, , Brooke CF, , A Simple Guide to IBM SPSS Statistics, 2015. |
[16] | Long JS, , Ryan KM, New frontiers in promoting tumour cell death: Targeting apoptosis, necroptosis and autophagy, Oncogene [Internet], 31: (49): 5045–5060, (2012) . |
[17] | Rodrigues BLC, , Lallo MA, , Perez EC, The controversial role of autophagy in tumor development: A systematic review, Immunol Invest, 49: (4): 386–396, (2019) . |
[18] | Chavez-Dominguez R, , Perez-Medina M, , Lopez-Gonzalez JS, , Galicia-Velasco M, , Aguilar-Cazares D, The double-edge sword of autophagy in cancer: From tumor suppression to pro-tumor activity, Front Oncol, 10: : 578418, (2020) . |
[19] | Xu J, , Patel NH, , Gewirtz DA, Triangular relationship between p53, autophagy, and chemotherapy resistance, Int J Mol Sci, 21: (23): 1–27, (2020) . |
[20] | Wang Y, , Xie J, , Wang H, , Huang H, , Xie P, Beclin-1 suppresses gastric cancer progression by promoting apoptosis and reducing cell migration, Oncol Lett, 14: (6): 6857–6862, (2017) . |
[21] | Rubinstein AD, , Eisenstein M, , Ber Y, , Bialik S, , Kimchi A, The autophagy protein ATG12 associates with antiapoptotic BCL-2 family members to promote mitochondrial apoptosis, Mol Cell, 44: (5): 698–709, (2011) . |
[22] | Guo JY, , Xia B, , White E, Autophagy-mediated tumor promotion, Cell, 155: : 1621–1635, (2013) . |
[23] | Ariosa AR, , Lahiri V, , Lei Y, , Yang Y, , Yin Z, , Zhang Z , A perspective on the role of autophagy in cancer, Biochim Biophys Acta Mol Basis Dis, 1867: (12): 166262, (2021) . |
[24] | Zhao H, , Yang M, , Zhao J, , Wang J, , Zhang Y, , Zhang Q, High expression of LC3B is associated with progression and poor outcome in triple-negative breast cancer, Med Oncol, 30: (1): 475, (2013) . |
[25] | Briukhovetska D, , Dörr J, , Endres S, , Libby P, , Dinarello CA, , Kobold S, Interleukins in cancer: From biology to therapy, Nat Rev Cancer, 21: : 481–499, (2021) . |
[26] | Li P, , Rozich N, , Wang J, , Wang J, , Xu Y, , Herbst B , Anti-IL-8 antibody activates myeloid cells and potentiates the anti-tumor activity of anti-PD-1 antibody in the humanized pancreatic cancer murine model, Cancer Lett, 539: : 215722, (2022) . |
[27] | Mian BM, , Dinney CPN, , Bermejo CE, , Sweeney P, , Tellez C, , Yang XD , Fully human anti-interleukin 8 antibody inhibits tumor growth in orthotopic bladder cancer xenografts via down-regulation of matrix metalloproteases and nuclear factor-κB, Clin Cancer Res, 9: (8): 3167–3175, (2003) . |
[28] | Luo M, , Wong S, , Chan M , LYJ of cellular, 2015 undefined. Autophagy mediates HBx-induced nuclear factor-κB activation and release of IL-6, IL-8, and CXCL2 in hepatocytes, Wiley Online Library, 230: (10): 2382–2389, (2015) . |
[29] | An Q, , Yan W, , Zhao Y, , Yu K, Enhanced neutrophil autophagy and increased concentrations of IL-6, IL-8, IL-10 and MCP-1 in rheumatoid arthritis, Int Immunopharmacol, 65: : 119–128, (2018) . |
[30] | Lei S, , Shanqiang Z, , Xiaodong Z, , Yuting Z, , Liping X, , Yang J , Enhancing the ability of autophagy and proliferation of bone marrow mesenchymal stem cells by interleukin-8 through Akt-STAT3 pathway in hypoxic environment, Sheng Wu Gong Cheng Xue Bao, 32: (10): 1422–1432, (2016) . |
[31] | Barnard RA, , Regan DP, , Hansen RJ, , Maycotte P, , Thorburn A, , Gustafson DL, Autophagy inhibition delays early but not late-stage metastatic disease, J Pharmacol Exp Ther, 358: (2): 282–293, (2016) . |
[32] | Tang KH, , Ma S, , Lee TK, , Chan YP, , Kwan PS, , Tong CM , CD133 + liver tumor-initiating cells promote tumor angiogenesis, growth, and self-renewal through neurotensin/interleukin-8/CXCL1 signaling, Hepatology, 55: (3): 807–820, (2012) . |
[33] | Wen J, , Zhao Z, , Huang L , LWJ of cellular and, 2020 undefined. IL-8 promotes cell migration through regulating EMT by activating the Wnt/β-catenin pathway in ovarian cancer, Wiley Online Library, 24: (2): 24, (2020) . |
[34] | Altevogt P, , Sammar M, , Hüser L, , Kristiansen G, Novel insights into the function of CD24: A driving force in cancer, Int J Cancer, 148: (3): 546–559, (2021) . |
[35] | Mendez LE, , Srinivasan M, , Hamouda RK, , Ambedkar B, , Arzoun H, , Sahib I , Evaluation of CD44+/CD24−and aldehyde dehydrogenase enzyme markers in cancer stem cells as prognostic indicators for triple-negative breast cancer, Cureus, 14: (8)(2022) . |
[36] | Denisov EV, , Skryabin NA, , Gerashchenko TS, , Tashireva LA, , Wilhelm J, , Buldakov MA , Clinically relevant morphological structures in breast cancer represent transcriptionally distinct tumor cell populations with varied degrees of epithelial-mesenchymal transition and CD44+CD24− stemness, Oncotarget, 8: (37): 61163, (2017) . |
[37] | Bradley CA, CD24–a novel’don’t eat me’signal, Nat Rev Cancer, 19: (10): 541–542, (2019) . |
[38] | Jang MH, , Kang HJ, , Jang KS, , Paik SS, , Kim WS, Clinicopathological analysis of CD44 and CD24 expression in invasive breast cancer, Oncol Lett [Internet], 12: (4): 2728–2733, (2016) . . [cited 2022 Feb 26]. Available from: http://www.spandidos-publications.com/10.3892/ol.2016.4987/abstract. |
[39] | Lim J, , Lee KM, , Shim J, , Shin I, CD24 regulates stemness and the epithelial to mesenchymal transition through modulation of Notch1 mRNA stability by p38MAPK, Arch Biochem Biophys, 558: : 120–126, (2014) . |
[40] | Lu Q, , Wang WW, , Zhang MZ, , Ma ZX, , Qiu XR, , Shen M , ROS induces epithelial-mesenchymal transition via the TGF-β1/PI3K/AKT/mTOR pathway in diabetic nephropathy, Exp Ther Med, 17: (1): 835–846, (2019) . |
[41] | Wang J, , Liu D, , Sun Z, , Ye T, , Li J, , Zeng B , Autophagy augments the self-renewal of lung cancer stem cells by the degradation of ubiquitinated p53, Cell Death & Disease, 12: (1): 1–12, (2021) . |
[42] | Niu T, , Tian Y, , Wang G, , Guo G, , Tong Y, , Shi Y, Inhibition of ROS-NF-κB-dependent autophagy enhances Hypocrellin A united LED red light-induced apoptosis in squamous carcinoma A431 cells, Cell Signal, 69: : 109550, (2020) . |
[43] | Bie Q, , Song H, , Chen X, , Yang X, , Shi S, , Zhang L , IL-17B/IL-17RB signaling cascade contributes to self-renewal and tumorigenesis of cancer stem cells by regulating Beclin-1 ubiquitination, Oncogene, 40: (12): 2200–2216, (2021) . |
[44] | Huang Q, , Duan L, , Qian X, , Fan J, , Lv Z, , Zhang X , IL-17 promotes angiogenic factors IL-6, IL-8, and vegf production via Stat1 in lung adenocarcinoma, Sci Rep, 6: (1): 1–13, (2016) . |
[45] | Hao Y, , Baker D, , Ten DP, TGF-β-mediated epithelial-mesenchymal transition and cancer metastasis, Int J Mol Sci, 20: : 2019. |
[46] | Suzuki HI, , Kiyono K, , Miyazono K, Regulation of autophagy by transforming growth factor-β (TGFβ) signaling, Autophagy, 6: (5): 645–647, (2010) . |
[47] | Qi W, , Chen X, , Polhill TS, , Sumual S, , Twigg S, , Gilbert RE , TGF-β1 induces IL-8 and MCP-1 through a connective tissue growth factor-independent pathway, Am J Physiol Renal Physiol, 290: (3): 703–709, (2006) . doi:10.1152/ajprenal.00254.2005. |