Effect of additional dead space using end-tidal CO2 measurement on ventilating preterm infants: An experimental study
Abstract
BACKGROUND:
Dead space is the part of the airway where no gas exchange takes place. Any increase in dead space volume has a proportional effect on the required tidal volume and thus on the risk of ventilation-induced lung injury. Inserts that increase dead space are therefore not used in small preterm infants. This includes end-tidal CO2 measurement.
OBJECTIVE:
The aim of this study was to investigate the effect of the end-tidal CO2 measurement adapter on ventilation.
METHODS:
In an experimental setup, an end-tidal CO2 measurement adapter, three different pneumotachographs (PNT-A, PNT-B, PNT-Neo), and a closed suction adapter were combined in varying set-ups. The time required for CO2 elimination by a CO2-flooded preterm infant test lung was measured.
RESULTS:
PNT-A prolonged CO2 elimination time by 0.9 s (
CONCLUSION:
The flow sensor had the greatest influence on ventilatory effort, while end-tidal CO2 measurement had only a moderate effect. The increased ventilatory effort levied by the CO2 measurement was dependent on the flow sensor selected. The use of closed suctioning more negatively impacted ventilatory effort than did end-tidal CO2 measurement.
1.Introduction
Survival of small preterm infants has improved significantly over the past decades [1]. During the same period, the incidence of chronic lung injury in this population has increased [2]. Ventilation and volume trauma therefore remain relevant risk factors for neonates [3]. Instrumental dead space has a major impact by increasing tidal volume and thus ventilatory effort [4, 5]. Technical solutions to eliminate dead space exist in theory [6, 7, 8], but have not yet been integrated into ventilators. Currently, the only viable solutions aim at keeping dead space at a minimum. Therefore, technical installations between the Y-piece of the ventilation tubes and the endotracheal tube (ETT) that disproportionately increase the dead space should be avoided.
End-tidal CO2 (EtCO2) measurement is a non-invasive method for testing the efficiency of neonatal ventilation. Despite supporting literature [12], it is avoided in small preterm infants, due to imposed dead space. The aim of this study was to investigate the effect of the installation of a MicrostreamTM EtCO2 monitoring device in combination with other equipment in the ventilation system on the ventilatory effort of infants.
2.Materials and methods
The CO2 elimination time of a test lung flooded with CO2 was measured using different components of the ventilation system. The experimental setup, shown in Fig. 1, was taken from a previous study [4].
Figure 1.
Experimental setup.
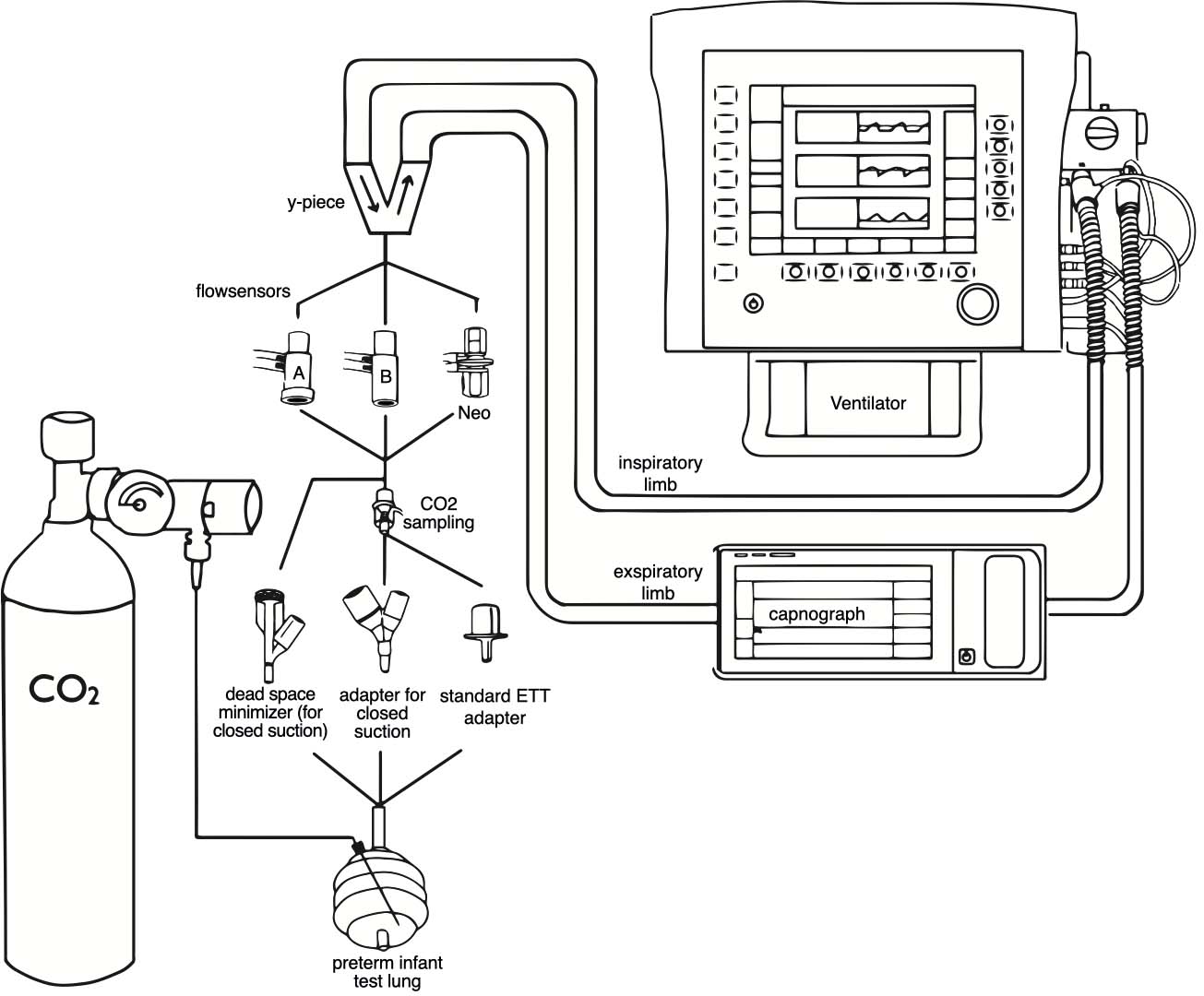
A Sophie ventilator (F. Stephan GmbH, Gackenbach, Germany) equipped with a patient hose system for open care operation was used. To eliminate their possible interference, the integrated humidification and warming devices were switched off and the trigger mechanisms were disabled. To simulate the lungs of a premature infant weighing 1000 g, a test lung with a volume of 50 ml and a compliance of 0.6 ml/mbar (Drägerwerk AG & Co. KGaA, Lübeck, Germany) was connected to the ventilator. The lung was flooded with CO2 via a perfusor needle (original perfusor syringe OPS 50 ml Luer Lock, Braun Medical, Melsungen, Germany) and a supply line connected to a CO2 cylinder (medical gas; 100% CO2). A flow meter set at 5 l/min was used to down-regulate the CO2 flow. A CO2 measurement was established in the expiratory limb of the breathing tube (IntelliVue X3 with MicrostreamTM module; Philips, Amsterdam, The Netherlands).
In each experiment, the test lung was attached to the Y piece of the breathing tube with one of three different endotracheal tube (ETT) connectors, without or via one of three flow sensors. Each combination was tested with and without additional EtCO2 measurement. When compatible, the adapter for EtCO2 measurement (Microstream FilterLine H set, CO2 sampling line for infants/newborns, and airway adapter for humid environment; Oridion, Jerusalem, Israel) was installed between the ETT-connector and the flow sensor.
The following adapters and flow sensors were tested:
1. Pneumotachograph-A (PNT-A) (F. Stephan GmbH, Gackenbach, Germany)
2. Pneumotachograph-B (PNT-B) (F. Stephan GmbH, Gackenbach, Germany)
3. Pneumotachograph-Neo (PNT-Neo) (F. Stephan GmbH, Gackenbach, Germany)
4. Standard ETT connector (Safety Rüschelit I.D. 3.0 mm; Willy Rüsch, Kernen, Germany)
5. ETT connector for closed suction (Closed suction system for neonates/pediatrics, 3.0 mm Y-adapter; Halyard Health, Alpharetta, USA)
6. Dead space minimized ETT connector for closed suctioning (3.0 mm; F. Stephan GmbH, Gackenbach, Germany)
The adapters were used in their usual positions. All possible combinations of the above adapters were tested. Due to the physical shape of each adapter, not every flow sensor could be combined with every tube adapter or the CO2 measurement adapter. A ranking according to the size of the flow sensors is shown in Fig. 2. A total of nineteen possible combinations were made and each test condition was repeated twenty times.
Figure 2.
CO2 elimination time in seconds for the different flow sensors with adapters, No-PNT: without Pneumotachograph, PNT-A: Pneumotachograph-A, PNT-B: Pneumotachograph-B, PNT-Neo: Pneumotachograph-Neo, ET: Standard ETT connector, dsm: Dead space minimised ETT connector for closed suctioning, afcs: ETT connector for closed suction, CO2s: adapter for EtCO2 measurement.
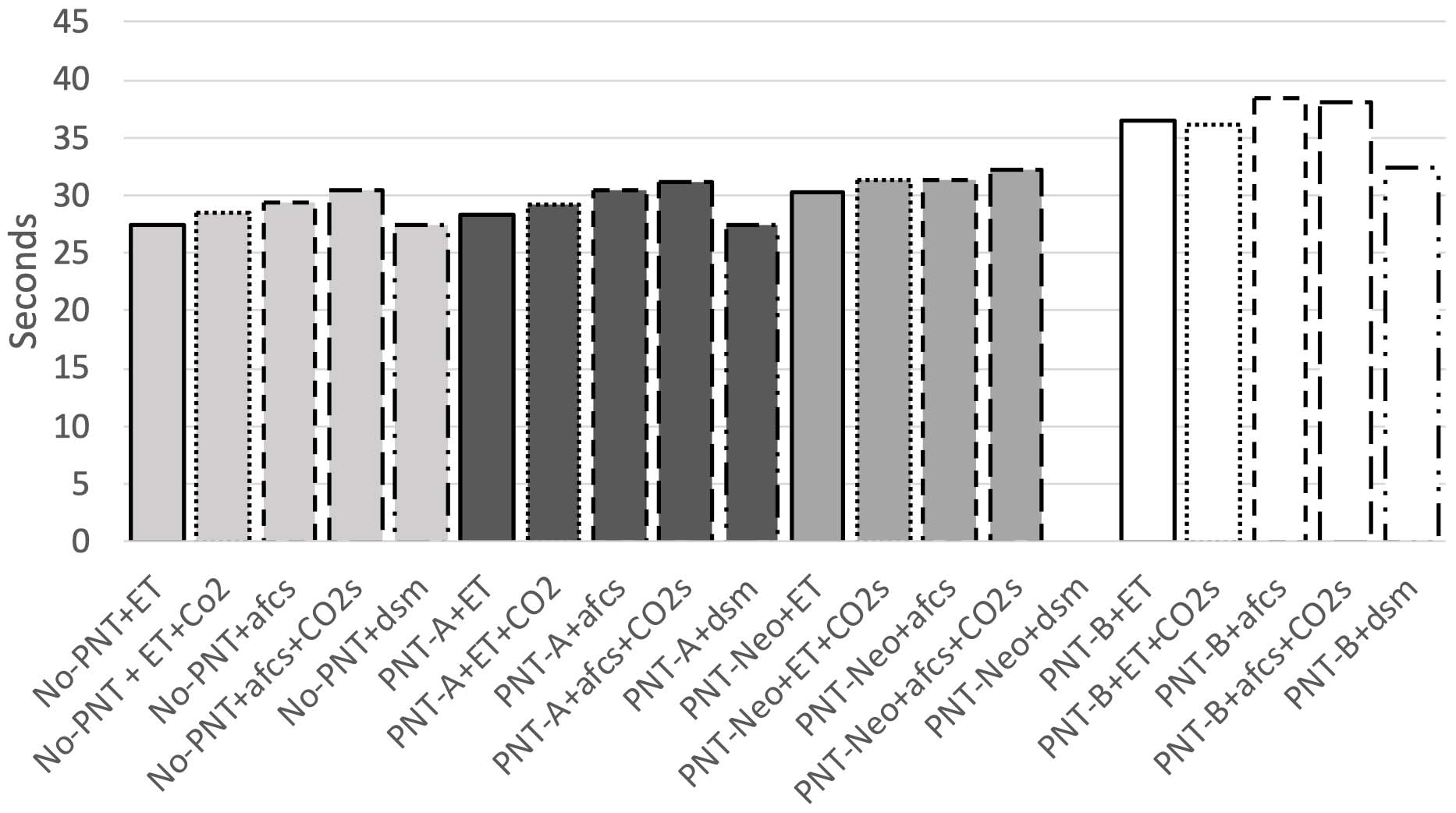
At the start of each experiment, the test lung was flooded with 5 L/min of CO2. During this time, the ventilator was in continuous positive airway pressure (CPAP) mode with a flow of 10 L/min and positive end-expiratory pressure (PEEP) of 5 mbar. CO2 delivery was stopped after 30 seconds. The CPAP mode was exited, flushing excess CO2 from the tube and Y-piece. When the CO2 sensor in the expiratory limb detected no further CO2 flow, intermittent mandatory ventilation (IMV) mode was selected on the ventilator. Ventilation was set at a rate of 60 breaths per minute, a peak inspiratory pressure (PIP) of 16 mbar, a PEEP of 5 mbar and a flow of 10 L/min. This corresponds to a tidal volume of 6 ml. CO2 concentrations were displayed as waves on the monitor connected to the CO2 sensor. The height of the CO2 curves decreased with each breath. The time taken to clear CO2 from the test lung was measured. The time from the start of ventilation until the value fell below the threshold of 10 mmHg was evaluated. The threshold of 10 was used because it was easier to assess than the exact drop in CO2 to zero.
Results were expressed as the mean (
The experiments did not involve animals or human subjects; therefore, no ethical review or consent for participation or publication was required.
3.Results
In the absence of a flow sensor and with a standard ETT adapter, CO2 elimination time without EtCO2 was 27.48 s (
When the PNT-A flow sensor and standard ETT adapter were combined, CO2 elimination time without EtCO2 was 28.40 s (
In the experimental set up of PNT-Neo with the standard ETT adapter, CO2 elimination time was 30.36 s (
With PNT-B flow sensor and the standard ETT adapter, the CO2 elimination time was 36.46 s (
The results are graphically summarized in Fig. 2.
4.Discussion
The present work revealed that the use of the MicrostreamTM EtCO2 adapter does not necessarily lead to an increase in CO2 elimination time and thus to an increase in ventilatory effort. This was largely dictated by the dead space within the different flow sensors. The larger the flow sensors and the more adapters combined, the longer the CO2 washout time. In the case of the flow sensor with the largest dead space (PNT-B), use of the EtCO2 adapter actually reduced CO2 elimination time. In the other test series, the installation of EtCO2 increased the elimination time by 3 to 4%. This could be due to the effect of washing out the dead space by aspirating breathing gas. With the MicrostreamTM EtCO2 process, only 50 ml/min is aspirated. With a tidal volume of 6 ml and a frequency of 60 ppm, this gives a minute volume of 360 ml/min and translates to only a fraction of the minute volume being removed. In the study by Schöber et al., a reduction in CO2 of approximately 15% was achieved by withdrawing 400 ml/min of inspiratory gas [7]. This is a multiple of the amount withdrawn here. Accordingly, the effect was greater. However, the issue in this paper is not the washout of the total instrumental dead space, but only the washout of the instrumental dead space of the EtCO2 adapter. Therefore, it seems plausible that the washout of the small volume of only 50 ml/min in this study also has an effect on CO2 elimination. As this study only examined the adapter in combination with a running measurement (
Remarkably, we have demonstrated that the dead space sizes of the different pneumotachygraphs corresponded to CO2 washout times. This is also consistent with the results of the study by Wald et al. [4] where the CO2 washout time was shortest in the absence of a flow sensor. The results of our study show that the dead space of the PNT-Neo is slightly larger than the dead space of the PNT-A, but significantly smaller than the dead space of the PNT-B. In principle, therefore, the PNT-Neo could also be used for small premature infants.
These results also support the clinical relevance of dead space previously discussed by Nassabeh-Montazami et al. In this work, it was shown that dead space increases the required tidal volume in smaller preterm infants weighing well below 1000 g [9]. In our study, the lungs of a preterm infant weighing approximately 1000 g were simulated. As the adapters used here are also used for smaller infants with lower tidal volumes, the effect shown should be correspondingly greater.
Closed suctioning has been used in neonatology for many years [10]. Previously, closed and open suctioning were compared in terms of effectiveness and imposed pain [11]. Newly presented in this study is a comparison of dead space and its effect on ventilatory effort. Closed suctioning increases CO2 elimination time, even in ventilating without a flow sensor. This means that the flow turbulence from the Y-piece is not able to wash out all the dead space. Overall, with a 3–7% increase of the CO2 elimination time, the negative effect of closed suction is twice that of EtCO2 measurment. The reduction of the CO2 elimination time by using a dead space optimized approach for closed suction is correspondingly impressive. However, this does not apply to all flow sensors.
Though closed suctioning, in contrast to EtCO2 measurement, is standard in treatment of small, preterm infants [10], our data showed that in comparison to the adapter for closed suctioning, the adapter for EtCO2 measurement had relatively little effect on dead space. Post-market surveillance of medical devices is needed to collect such information. This could pinpoint dissimilarities in devices from various manufacturers, how well devices function or are maintained, and even the extent of their influence on medical procedures [13, 14].
A limitation of this study is that the CO2 elimination time measured cannot be readily extrapolated as ventilation effort. It is established though that mechanical ventilation contributes to chronic lung injury via direct physical damage [3], and therefore can be presumed that a higher CO2 elimination time correlates with increased injury and a lower CO2 elimination time imposes less physical stress on the lungs. Consequently, the results can be considered representative of changes in ventilatory effort.
An additional hindrance was that dead space in the device or adapter could not be provided in ml. This was partly because the manufacturers did not explicitly state the dead space for each device. Secondly, dead space cannot be determined simply by measuring the internal cavity. Complex flow measurements are required to determine the actual dead space of an adapter. Areas of turbulence or even stagnant air, such as behind projections that occur when multiple adapters are joined together, cannot be evaluated as dead space. For this reason, dead space has only been evaluated in relation to the other devices or adapters. However, it can be assumed that a long CO2 elimination time also correlates with a large dead space [4].
5.Conclusion
In this experiment, the dead space of technical attachments after the Y-piece in the ventilation circuit increases the ventilation effort in small preterm infants. The flow sensor has the greatest influence on ventilation effort. The installation of an EtCO2 adapter does not lead to a further increase in ventilatory effort in all cases and overall, its effect on CO2 elimination time is considerably less than the effect of closed suction. Therefore, EtCO2 measurement should be possible even in relatively small preterm infants if necessary.
Availability of data and material
The raw data from the in vitro simulations are available from the corresponding author upon reasonable request.
Funding
This study was conducted without funding.
Author contributions
The publication was prepared as part of the diploma thesis of Linda Mur which was supervised by Martin Wald. All authors actively contributed to the publication. Linda Mur collected data and co-wrote the paper with Martin Wald, who planned the research. Natalee Annon-Eberharter, as a native speaker, revised and corrected the text in English.
Acknowledgments
The ventilator and flow sensors were provided free of charge by the manufacturer or suppliers for the duration of the study. Data collection and analysis were performed without any influence from the manufacturers or suppliers. We thank them for their trust and cooperation.
Conflict of interest
Martin Wald has been a consultant for the companies Fritz Stephan GmbH and medin Medical Innovations GmbH and has given paid lectures for Medtronic Österreich GmbH in the last three years. In addition, he has organized workshops himself that were sponsored by the above-mentioned companies. All other authors have no conflicts of interest to declare.
References
[1] | Bell EF, Hintz SR, Hansen NI, Bann CM, Wyckoff MH, DeMauro SB, et al. Mortality, in-hospital morbidity, care practices, and 2-year outcomes for extremely preterm infants in the US, 2013–2018. JAMA [Internet]. (2022) ; 327: (3): 248-63. Available from: https://jamanetwork.com/journals/jama/articlepdf/2788142/jama_bell_2022_oi_210140_1654285730.66359.pdf. |
[2] | Stoll BJ, Hansen NI, Bell EF, Walsh MC, Carlo WA, Shankaran S, et al. Trends in care practices, morbidity, and mortality of extremely preterm neonates, 1993–2012. JAMA. (2015) ; 314: (10): 1039-51. |
[3] | Sahni M, Bhandari V. Recent advances in understanding and management of bronchopulmonary dysplasia. F1000Res [Internet]. 20200714th ed. (2020) ; 9. Available from: https://f1000researchdata.s3.amazonaws.com/manuscripts/27962/9fc43b29-d423-40c3-9f8e-baeb64247fc6_25338_-_vineet_bhandari.pdf?doi=10.12688/f1000research.25338.1&numberOfBrowsableCollections=87&numberOfBrowsableInstitutionalCollections=4&numberOfBrowsableGateways=49. |
[4] | Wald M, Jeitler V, Lawrenz K, Weninger M, Kirchner L. Effect of the Y-piece of the ventilation circuit on ventilation requirements in extremely low birth weight infants. Intensive Care Med [Internet]. (2005) ; 31: (8): 1095-100. Available from: https://www.ncbi.nlm.nih.gov/pubmed/15999252. |
[5] | Keszler M, Nassabeh-Montazami S, Abubakar K. Evolution of tidal volume requirement during the first 3 weeks of life in infants < 800 g ventilated with Volume Guarantee. Arch Dis Child Fetal Neonatal Ed [Internet]. 20081205th ed. (2009) ; 94: (4): F279-82. Available from: https://fn.bmj.com/content/94/4/F279. |
[6] | Wald M, Kalous P, Lawrenz K, Jeitler V, Weninger M, Kirchner L. Dead-space washout by split-flow ventilation. A new method to reduce ventilation needs in premature infants. Intensive Care Med. 20050419th ed. (2005) ; 31: (5): 674-9. |
[7] | Schöber M, Bohnhorst B, Annon-Eberharter N, Wald M. Dead space washout by intentional leakage flow during conventional ventilation of premature infants-an experimental study. Pediatr Pulmonol [Internet]. 20220620th ed. (2022) ; 57: (9): 1998-2002. Available from: https://onlinelibrary.wiley.com/doi/pdfdirect/10.1002/ppul.25906?download=true. |
[8] | Danan C, Tauzin M, Jung C, Carbonnier B, Dassieu G, Decobert F, et al. Instrumental dead space: A glass ceiling for extremely low birth weight preterm infants? A dead space washout bench study. Pediatr Pulmonol [Internet]. (2023) . Available from: doi: 10.1002/ppul.26353. |
[9] | Nassabeh-Montazami S, Abubakar KM, Keszler M. The impact of instrumental dead-space in volume-targeted ventilation of the extremely low birth weight (ELBW) infant. Pediatr Pulmonol [Internet]. (2009) ; 44: (2): 128-33. Available from: https://www.ncbi.nlm.nih.gov/pubmed/19061234. |
[10] | Cordero L, Sananes M, Ayers LW. Comparison of a closed (Trach Care MAC) with an open endotracheal suction system in small premature infants. J Perinatol [Internet]. (2000) ; 20: (3): 151-6. Available from: https://www.nature.com/articles/7200330. |
[11] | Cardoso JM, Kusahara DM, Guinsburg R, Pedreira ML. Randomized crossover trial of endotracheal tube suctioning systems use in newborns. Nurs Crit Care [Internet]. 20150316th ed. (2017) ; 22: (5): 276-83. Available from: doi: 10.1111/nicc.12170. |
[12] | Lopez E, Grabar S, Barbier A, Krauss B, Jarreau PH, Moriette G. Detection of carbon dioxide thresholds using low-flow sidestream capnography in ventilated preterm infants. Intensive Care Med [Internet]. (2009) ; 35: (11): 1942-9. Available from: doi: 10.1007/s00134-009-1647-5. |
[13] | Krieger TJ, Wald M. Volume-targeted ventilation in the neonate: Benchmarking ventilators on an active lung model. Pediatr Crit Care Med. (2017) ; 18: (3): 241-248. doi: 10.1097/PCC.0000000000001088. |
[14] | Badnjevic A, Gurbeta L, Jimenez ER, Iadanza E. Testing of mechanical ventilators and infant incubators in healthcare institutions. Technology and Health Care [Internet]. (2017) ; 25: (2): 237-50. Available from: doi: 10.3233/THC-161269. |