Diagnosis, management, and prevention of malfunctions in anesthesia machines
Abstract
BACKGROUND:
The anesthesia machine serves as a vital piece of lifesaving equipment.
OBJECTIVE:
To analyze incidents of failures in the Primus anesthesia machine and address these malfunctions to reduce recurrence of failure, save maintenance costs, enhance safety, and improve overall efficiency.
METHODS:
We conducted an analysis on the records pertaining to the maintenance and parts replacement of the Primus anesthesia machines used in the Department of Anaesthesiology at Shanghai Chest Hospital over the past two years to identify the most common causes of failure. This included an assessment of the damaged parts and degree of damage, as well as a review of factors that caused the fault.
RESULTS:
The main cause of the faults in the anesthesia machine was found to be air leakage and excessive humidity in the central air supply of the medical crane. The logistics department was instructed to increase inspections to check and ensure the quality of the central gas supply and ensure gas safety.
CONCLUSION:
Summarizing the methods for dealing with anesthesia machine faults can save hospitals a lot of money, ensure normal hospital and department maintenance, and provide a reference to repair such faults. The use of Internet of Things platform technology can continuously develop the direction of digitalization, automation, and intelligent management in each stage of the “whole life cycle” of anesthesia machine equipment.
1.Introduction
The anesthesia machine serves as a vital piece of lifesaving equipment. It is crucial that the rescue bag-mask be linked to the patient’s oxygen supply in time in case of a problem during surgery. The anesthesia equipment should be replaced with a backup unit promptly if the problem cannot be fixed. In order to quickly diagnose the problem and implement the appropriate fix, it is essential that the appropriate personnel have a thorough understanding of the gas circuit. Correct diagnosis and suitable patient treatment rely heavily on the accuracy and functioning of the medical equipment, in addition to the expertise and experience of the physician [1]. In our research, we illustrate how to close these gaps with concrete instances. The nine case studies presented in this paper illustrate the nuances of the problem, highlight the progress that has been made, and highlight the difficulties that still remain [2].
The Primus anesthesia machine is a top-of-the-line high performance anesthesia equipment used in various surgical procedures, offering comprehensive perioperative ventilation and anesthesia functions. It supports a range of clinical ventilation modes, including manual/autonomous ventilation (Man. Spont function), controlled ventilation (volume-controlled ventilation or VCV, and pressure-controlled ventilation or PCV), Autoflow, synchronous intermittent mandatory ventilation (SIMV), and pressure support ventilation (PSV). The machine also has its own gas monitoring system feature, compatible with multiple inhaled anesthetic agents (sevoflurane, desflurane, and isoflurane), and can provide manual ventilation and maintain anesthesia depth in case of machine failure. The Primus machine is designed with both air and electric circuit components, providing an ideal ventilation mode which can significantly improve the safety of clinical anesthesia [1].
The fault analysis and maintenance of the Primus anesthesia machine is crucial to its safe use and optimal performance. By identifying the cause of faults and conducting preventative maintenance, we can enhance the safe use and performance of the machine [2]. In this paper we present an analysis of details of faults and replaced parts of the Primus machine over the past two years (as shown in Table 1), highlighting the causes for malfunction and offering solutions.
Table 1
Data pertaining to malfunctions (faults) of the Primus anesthesia machine
Fault number | Number of faults caused by leakage | Machine status at the time of fault | Description of the fault | Maintenance work |
---|---|---|---|---|
66 | 22 (33.3%) | In use | MINUTE VOL. LOW | Replace the flow sensor and tighten the interface of the suction/exhalation port |
2 (3.3%) | CIRCLE LEAK | Confirm and reconnect the threaded pipe | ||
1 (1.5%) | VOLUME NOT ATTAINED | Confirm and reconnect the threaded pipe | ||
2 (3.3%) | PINSP. NOT ATTAINED | Reinstall the circuit and clean the rubber parts at the circuit’s joints components | ||
5 (7.6%) | FG LOW OR LEAK | Replace the original device for air supply | ||
1 (1.5%) | FG LOW OR LEAK | Reinstall the circuit and remove the blockage of the waste discharge system | ||
2 (3.3%) | During the | failed:Valves/ventilator | Replace valves and pressure sensors | |
9 (13.6%) | self-test | failed:Leakage (man/spon) | Reinstall the sodium lime tank and the circuit | |
1 (1.5%) | failed:Leakage (system) | Tighten the interface of the suction/exhalation port, confirm and reconnect the threaded pipe, and replace the pressure sensor |
2.Materials and methods
2.1The Primus anesthesia machine ventilation mode [3]
The Primus anesthesia machine has both a gas circuit and an electric circuit component. In our analysis of repair records over the past two years, we found that the majority of faults occurred in the air circuit. Hence, we present an introduction to the air circuit system (Fig. 1). The gas circuit system consists of a gas supply and delivery system, an anesthesia gas volatilization tank, a respiratory circuit, an anesthesia respirator, a monitoring and alarm system, and an anesthesia exhaust gas clean-up system.
Figure 1.
Working drawing of the Primus anesthesia machine.
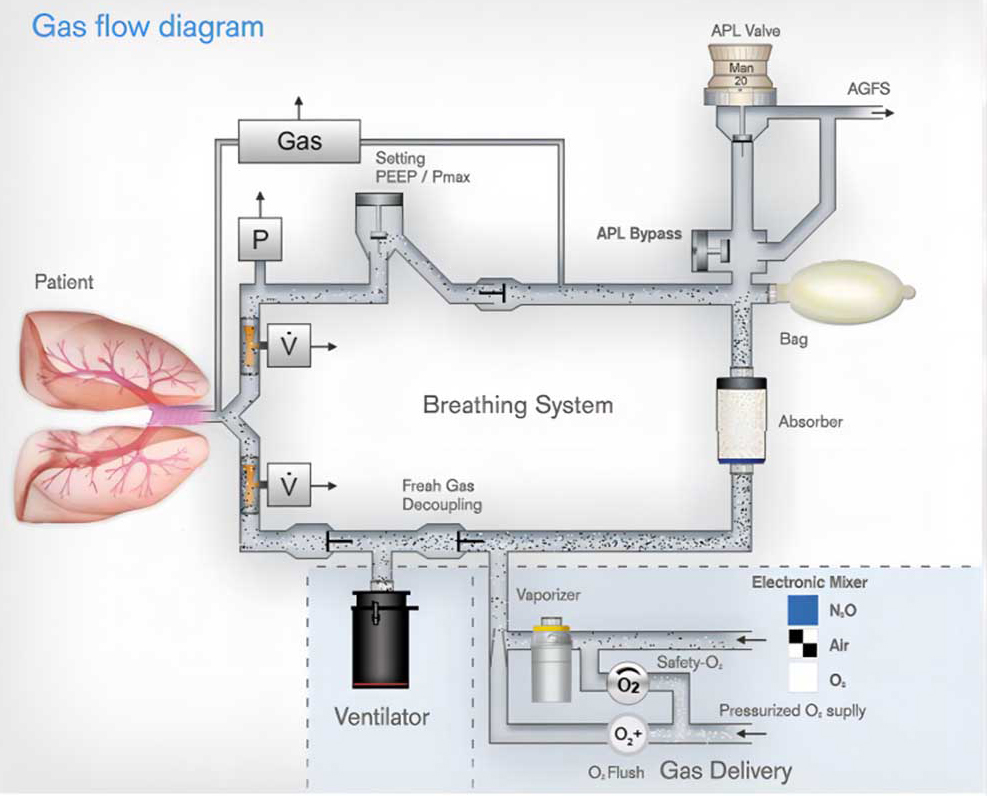
The Primus machine has a fresh gas isolation valve that allows fresh gas to flow out of the air storage bag and prevents it from entering the patient circuit during the inspiratory phase. In the expiratory phase, the fresh gas, the gas in the air storage bag and the patient’s exhaled gas, all flow directly into the ventilator. The fresh gas isolation valve allows for more accurate tidal volumes independent of flow sensor monitoring and ensures accurate tidal volume delivery even if the flow sensor fails. The electric control system of the Primus machine ensures precise gas delivery, and the return circuit compliance requirements are low, while its semi-closed circuit can make the effective fresh gas flow less than the minute ventilation volume. The Primus machine thus has several advantages: the flow of fresh gas is greater than that of the patient’ s intake, it minimizes the amount of gas and anesthetics used, helps reduce environmental pollution, and improve respiratory humidification function.
2.2Samples analyzed
We analyzed 96 different anesthesia machines utilized in Shanghai Chest Hospital, and found that 51 of them Primus anesthesia machines being used in a variety of operation rooms, representing 53% of the total, thus serving as the main equipment in the anesthesia department. The Primus machines offer ease of use in clinical settings, with clearly visible and adjustable parameters. To ensure proper functioning, repair and maintenance personnel must carefully follow the self-test process and pass all parameters before regular use. Technical proficiency and patience are required.
3.Results
3.1Fault analysis and countermeasures
3.1.1Fault pertaining to air leakage
3.1.1.1 Description of the fault
During thoracic surgery with one-lung ventilation, the machine issued alarms for “circuit leakage” and “low minute ventilation” in “Vol.” mode (machine-controlled mode) and only delivered 100 ml though it was set to a tidal volume of 300 ml. The patient’s end-tidal carbon dioxide (etco2) rose to 58 mmHg and the oxygen saturation (Spo2) dropped to 92.
3.1.1.2 Analysis of the fault
In spite of passing the self-test, “circuit leakage” and “low minute ventilation” in the machine-controlled mode can be caused by two reasons: 1) Decreased tidal volume (V
3.1.1.3 Fault remediation
The procedure was thoracic surgery with one-lung ventilation, and double-lumen endobronchial tubes were used for one-lung ventilation. We first investigated if the anesthesia machine fault was caused by the operation mode. Using a bronchofibroscope, we checked whether the double-lumen tube had deviated from its position and whether the cuff had air leakage. Once we confirmed that these were correct, we performed manual ventilation using the bag-mask ventilation to ensure the safety of the procedure and connected pure oxygen (5L) flow to ventilate the patient. Then we closed the inhalation volatilization tank and connected the simulated lung to select the “Vol” machine-controlled mode for simulated ventilation.
We found that the fault persisted, and the simulated ventilation was not working. We next checked the respiratory circuit and artificial nasal sampling tube and found no damage in these. The engineer checked with the anesthesiologist and learning that the sodium lime was changed during the operation, he opened the sodium lime tank and found that part of the old, discolored sodium lime residue was stuck on the sealing ring. Despite wiping it clean with gauze and reinstalling the sodium lime tank, the leak persisted.
After raising the manual control “APL” valve and continuing manual ventilation, air leakage was heard near the APL valve. It was determined that a slipped ring at the at the end of the circuit connecting with the anesthesia machine had caused the air leakage. After replacing the slipped ring and checking the pipelines, hoses, and filters for any abnormalities, the machine functioned normally.
Air leaks in the anesthesia machine can negatively impact the accuracy of tidal volume and cause ventilation failure due to internal circuit valve malfunctions or malfunctioning of the monitoring of respiratory parameters and alarm systems due to sensor failure [4, 5, 6]. Such problems may not pose immediate direct threats to patient safety but can significantly impact the quality of anesthesia during surgery. Early detection and prompt resolution of these faults is crucial for maintaining safe and controlled procedures. Clinical engineers should thoroughly inspect the anesthesia machine according to the established self-test procedure to ensure full functionality before use.
In the case of equipment malfunction during this surgery, the patient’s spontaneous ventilation was secured as a first priority. Once patient safety was ensured, we systematically investigated the probable causes for the machine-controlled air leakage, starting with excluding any surgical factors and thoroughly screening each component of the external circuit of the machine, including the volatilization tank, respiratory circuit, all interfaces, and various parts of the sodium lime tank. Timely execution of emergency repairs must be carried out efficiently and in a well-organized manner, ensuring the patient’s continued ventilation with monitoring of the functioning of the simulated lung [7].
3.2Fault pertaining to excessive humidity of the central air supply
3.2.1Description of the fault
From May to August 2020, the self-test sensors of several Primus anesthesia machines detected yellow alarm failures that did not impact the machines’ normal operation. Subsequently, the sound of water dripping could be heard when operating the machine and it was suspected that water might have seeped into the instrument. The operation of the machine was immediately stopped, the standby machine was connected so as to not affect the normal work in the operating room, and the manufacturer’s engineer was contacted for maintenance assistance. After opening the rear cover of the machine, it was found that the sound was coming from the air pressure reducing valve. The valve was replaced and there was no further sound, but the self-test sensor remained yellow. Subsequently, we checked for faults in the fresh gas flow valve, positive end-expiratory pressure (PEEP) valve, suction valve, and exhalation valve and replaced these, resulting in the resolution of the fault.
3.2.2Analysis of the fault
There were several consecutive failures that occurred in the water inlet of the pressure reducing valve. As seen in the gas circuit diagram of the pressure reducing valve, the fault was identified in the gas source and gas circuit where air and oxygen were mixed (Fig. 2).
Figure 2.
Pathway diagram of the Primus anesthesia machine.
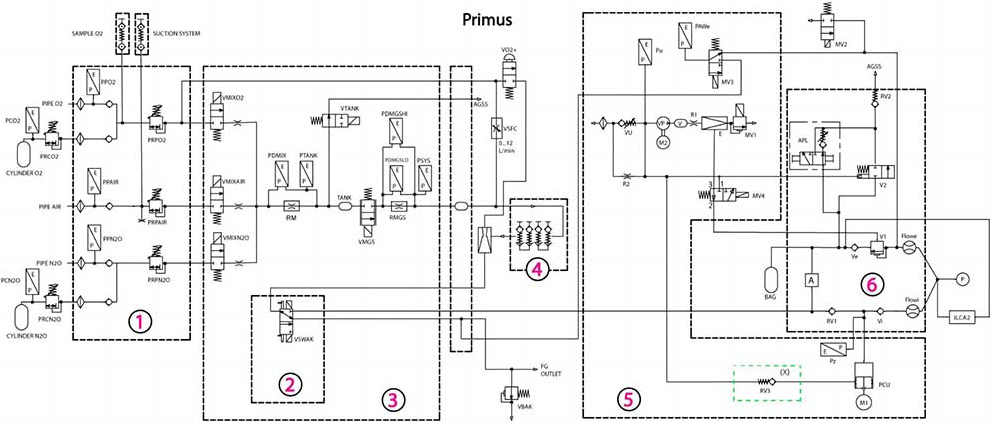
Analysis of the central air supply quality revealed water mist emission during the weekend overhaul of the medical crane, even when the central air source was disconnected. We studied the gas circuit diagram of the anesthesia machine and found that several failures of the anesthesia machine were caused by water entering the pressure reducing valve due to the excess water content in the central air source.
Further investigating this, we found that the large temperature difference between indoor and outdoor environments in the hot summer weather caused the compressed air to enter the indoor air-conditioning environment from the outdoor hot environment, and this caused water condensation when the warmer compressed air came in contact with the colder air. This led to excessive humidity in the central air source, causing water to enter the pressure reducing valve and leading to several failures in the anesthesia machine. The air source pressure increased to 5.0 Kpa, and the high pressure of air entering the anesthesia machine from medical crane caused damage to various sensors and pressure reducing valves.
3.2.3Fault remediation
The hospital’s operating rooms receive gas supply from the air compressor room and central oxygen station, with corresponding interfaces in the operating rooms and wards provided by the Logistics Support Department. We recommended that the Logistics Department conduct periodic inspections more frequently to regularly check the quality of the central supply of gas and ensure gas safety and that their personnel should be contacted immediately in case of any issues.
Additionally, another aspect to be noted is that the anesthesia machine’s crucial parts are made of copper and are susceptible to corrosion and gas leakage caused by water coming in contact with verdigris (copper oxide) [8]. To prolong the machine’s service life, the outside and inside of the machine must be kept dry after each use.
A yellow alarm fault in the internal circuit of the Primus anesthesia machine was still detected. After thoroughly investigating the humidity of the central air supply and air source in the operating room, it was found that four anesthesia machines had serious water seepage issues. The fresh gas flow valve, PEEP valve, suction valve, and discharge valve in the air circuit were checked and replaced if damaged by the water seepage [9]. The anesthesia machine was run for 8 hours after self-testing to ensure it as working as per normal parameters and indicators before resuming regular operations.
4.Discussion
4.1Summary
Based on the Primus service manual and the actual situation, we checked all the parts one by one for faults, and the faulty parts were replaced or ordered in time so as to not disrupt normal clinical operations. In case of similar faults occurring repeatedly, it is important to take corresponding measures based on experience and ensure the safe use of anesthesia machine and reduce maintenance cost [10, 11].
From a procurement standpoint, it is important to focus on preventive maintenance measures during the process of tender procurement itself, to include technical parameters such as bacterial/viral filters, biennial replacement of aeration and gas control, checking the O-ring between the respiratory system valve plate and the rolling film cover plate, triennial replacement of the lower rolling membrane under ventilator
4.2Future prospects
4.2.1Hardware device support
As part of the inspection system that was established, the central air supply station was equipped with air conditioning to maintain the same temperature as the operating room and other measures were taken up to prevent malfunctions in the anesthesia machine due to air source factors. To enhance the accuracy of various parameters of the anesthesia machine, quality control testing was conducted using Fluke’s equipment testing tools [12, 13]. These steps aimed to further strengthen and ensure the safety of the anesthesia machine.
4.2.2Software system support
From the perspective of asset supervision, when procuring maintenance for equipment, there is no extra costs for upgrading software and hardware systems, allowing real-time analysis of equipment performance [14, 15]. The rationale for this is as follows: Connecting small and medium-sized medical equipment like anesthesia machines to an Internet of Things management platform via information technology can realize remote and dynamic monitoring and management, provide real-time insights into equipment performance and status of functions, and the use of big data analysis and artificial intelligence can support hospital managements to make accurate decisions [16, 17]. Adopting a standardized approach to conformity assessment tests for PMS anesthesia machines, in addition to improving the reliability of the devices, is the first step in managing the digital transformation of these devices in healthcare facilities, opening up the possibility of using artificial intelligence [18].
By utilizing scientific and technological means, not only can the normal operations and routine maintenance of hospital equipment be ensured, but also software upgrades and hardware support can be guaranteed throughout the equipment’s lifespan. This will reduce the cost of managing and operating medical devices and improve efficiency, as well as reduce the maintenance time required for medical equipment and the workload of clinical engineers. By compiling data from active anesthesia machines, the integration of the Internet of Things and blockchain technology can facilitate the establishment of a closed loop between the medical equipment and various information systems within the hospital [18, 19, 20]. The limitation of this study is the small sample size, which should be addressed in future studies.
5.Conclusion
A crucial piece of hospital equipment is the anesthesia machine. Hospitals may save a lot of money by reviewing a summary of the techniques used to deal with faults in anesthesia machines, which can also serve as a reference for repairing such faults and assure the regular maintenance operations of hospitals and departments. The Internet of Things platform technology is used to advance digitalization, automation, and intelligent management throughout the “whole life cycle” of anesthesia machine equipment from the perspective of various hospital management departments, thereby improving the quality and safety of surgical services provided by hospitals.
That is to say, it is possible to accomplish comprehensive real-time monitoring, record keeping, and control and administration of medical anesthetic machines thanks to digital information technology. Medical anesthesia machines equipped with a remote monitoring system can report malfunctioning components and initiate preventative maintenance management with a simple click of a button. The medical anesthesia machine has a safety lock, a protective cover, and other safeguards to ensure the user’s and the patient’s safety.
Competing interests
The authors declare that they have no competing interests.
Funding
No external funding was received to conduct this study.
Availability of data and materials
All data generated or analysed during this study is included in this article. Further enquiries can be directed to the corresponding author.
Acknowledgments
The authors would like to acknowledge the hard and dedicated work of all staff that implemented the intervention and evaluation components of the study.
References
[1] | Badnjevic A, et al. Measurement in medicine – Past, present, future. First Conference on Medical and Biological Engineering in Bosnia and Herzegovina (CMBEBIH 2015). (2015) . |
[2] | Badnjevic A. Evidence-based Maintenance of Medical Devices: Current Shortage and Pathway Towards Solution. 1 Jan. 2023: : 293-305. |
[3] | Vande Lune SA, Lantry JH, Mason PE, et al. Universal anesthesia machine: Clinical application in an austere, resource-limited environment. Mil Med. (2020) ; 185: (5-6): e550-e556. |
[4] | Singh M, Nath G. Artificial intelligence and anesthesia: A narrative review. Saudi J Anaesth. (2022) ; 16: (1): 86-93. |
[5] | Ning XY. Measurement comparison of tidal volume of anesthesia machine. Metrology and Measurement Technique. (2020) ; 47: (3): 51-52. |
[6] | Chen TR, Feng D, Jing JQ. Basic structure and fault analysis of anesthesia machine [Chinese]. Science and Technology & Innovation. (2020) ; (14): 124-125. |
[7] | Thoben C, Dennhardt N, Krauß T, Sümpelmann R, Zimmermann S, Rüffert H, Heiderich S. Preparation of anaesthesia workstation for trigger-free anaesthesia: An observational laboratory study. Eur J Anaesthesiol. (2019) ; 36: (11): 851-856. |
[8] | Chen L, Wang H, Guo D, et al. Application of PDCA circulation method in improving the safety of anesthesia machine. China Medical Devices. (2018) ; 33: (4): 143-146. |
[9] | Hernandez-Meza G, Izzetoglu M, Osbakken M, Green M, Abubakar H, Izzetoglu K. Investigation of optical neuro-monitoring technique for detection of maintenance and emergence states during general anesthesia. J Clin Monit Comput. (2018) ; 32: (1): 147-163. |
[10] | Brattwall M, Warrén-Stomberg M, Hesselvik F, Jakobsson J. Brief review: Theory and practice of minimal fresh gas flow anesthesia. Can J Anaesth. (2012) ; 59: (8): 785-797. |
[11] | Gurbeta L, Dzemic Z, Bego T, Sejdic E, Badnjevic A. Testing of anesthesia machines and defibrillators in healthcare institutions. J Med Syst. (2017) ; 41: (9): 133. |
[12] | Lv L. Discussion on the management of medical equipment archives in hospitals [Chinese]. Money China. (2016) ; 14: : 348. |
[13] | Miao Z, Wen QP. Infection control of anesthesia machine for patients with novel coronavirus pneumonia [Chinese]. Journal of Dalian Medical University. (2020) ; 42: (2): 172-176. |
[14] | Sampson JB, Lee BH, Koka R, Chima AM, Jackson EV, Ogbuagu OO, Tran TP, Rosen MA. Human factors evaluation of the universal anaesthesia machine: Assessing equipment with high-fidelity simulation prior to deployment in a resource-constrained environment. J Natl Med Assoc. (2019) ; 111: (5): 490-499. |
[15] | Santangelo B, Robin A, Simpson K, Potier J, Guichardant M, Portier K. The modification and performance of a large animal anesthesia machine (Tafonius®) in order to deliver xenon to a horse. Front Vet Sci. (2017) ; 4: : 162. |
[16] | Altınışık U, Altınışık HB, Şimşek T, et al. Evaluation of the approaches of the anaesthesiologists on maintenance of anaesthesia machines. Turk J Anaesthesiol Reanim. (2016) ; 44: (3): 134-141. |
[17] | Badnjević A, Avdihodžić H, Gurbeta Pokvić L. Artificial intelligence in medical devices: Past, present and future. Psychiatria Danubina. (2021) ; 33: (Suppl 2): 101-106. |
[18] | Badnjevic A, Deumic A, Dzemic Z, Pokvic LG. A novel method for conformity assessment testing of anaesthesia machines for post-market surveillance purposes. Technol Health Care. (2023) ; 31: (1): 377-387. |
[19] | Figueiredo DBS, Aun AG, Lara JR, Garofalo NA, Teixeira-Neto FJ, Braz LG, Braz MG. Measurement of anesthetic pollution in veterinary operating rooms for small animals: Isoflurane pollution in a university veterinary hospital. Braz J Anesthesiol. (2021) ; 71: (5): 517-522. |
[20] | Tobin JM, Barras WP, Bree S, et al. Anesthesia for trauma patients. Mil Med. (2018) ; 183: (suppl_2): 32-35. |