Cortical activity, kinematics and trunk muscles activity response to pelvis movements during unstable sitting
Abstract
BACKGROUND:
Balance control is a leading component of human motor activities and its impairment is associated with an increased risk of falling, lower back pain due to impaired motor control mechanism. Prolonged sitting position at workplace is one of the risk factors of reduced postural control and lower back pain.
OBJECTIVE:
To evaluate theta and alpha waves cortical activity, trunk muscles activity and kinematics in static sitting, dynamic sitting on different platforms: simple wobble board (WB) and wobble board on bearing surface (WBB).
METHODS:
The kinematics of body segments, electromyography of five trunk muscles, electroencephalography of 32 scalp electrodes were measured during balance tasks in sitting position for 17 subjects with continuous seated position at workplace.
RESULTS:
Cortical power on WBB1 increase in fronto – central (
CONCLUSIONS:
The study shows that WBB can increase personalized sitting and improve trunk motor control during hours of prolonged sitting.
1.Introduction
A potential mechanism which is associated with lower back pain is known as insufficient lumbar stability [1], reduced proprioception, which causing the impaired postural control [2]. Trunk postural control is often assessed when using unstable seats to perform the sitting tasks [3]. The larger center-of-pressure while sitting on unstable seat indicates the poorer postural control [3, 4]. Balance control is a leading component of human every day motor activities such as standing, walking or sitting and its impairment is associated with an increased risk of falling [5, 6], functional independence [6], lower back pain due to impaired motor control mechanism. A complex interplay between the sensory and the motor systems allows a good control on posture and balance [5]. Efficient control of the center of mass by proper muscle activation is essential in maintaining a good equilibrium in standing and sitting. Thus, reduced somatosensory input can affect the postural control in lower back pain.
In many studies there are talking and experimenting about increment of dynamic sitting via destabilization of support surface [6, 7, 8] and mostly this dynamic sitting or standing is explained as external perturbation [6] to reach physiological changes. Also, other dynamic sitting is reached via internal perturbation [9, 10] when individuals should maintain balance using external force (muscles). The important features of unstable chairs with movable seat are that it forces of the pelvis movement and increases muscle activity [6].
Moreover, studies analyzing electroencephalography (EEG) signals during external balance perturbations showed higher demands on balance control. This is associated with higher theta activity located in the medial fronto – central cortical area [11, 7]. Theta activity increment during balance tasks is not only in the fronto – central cortex but also include centro – parietal regions [11] which is involved in sensory integration and sensorimotor coordination. The functional significance of frontal and parietal brain areas involvement during balance control is supported by longitudinal studies on the effects of balance training on neural structure [12]. Gray matter volume increased in frontal and parietal cortical areas after six weeks of continuous balance training and this was correlated with improvements in balance performance [12]. Also, it is important to mention, that subjects explored and evaluated different movement coordination patterns to improve performance in the dynamic balance tasks [12]. Various components of motor learning as a means to induce neuroplasticity [13]. Here we focus on evaluation of the theta and alpha waves cortical activity, trunk muscles activity and kinematics in static sitting, dynamic sitting on different platforms: simple wobble board (WB) and wobble board on bearing surface (WBB). We hypothesize that on custom – made wobble board on bearing surface pelvic motions will be more challenging according to trunk muscles activity, cortical activity, and kinematics parameters, comparing with simple wobble board.
2.Materials and methods
2.1Participants
Seventeen subjects with non-specific lower back pain and continuous seated position at workplace were involved in the study. The subjects were 28
All participants were verbally informed about the protocol and read and signed a consent form. Ethical approval (2020-06-17, No. BE-2-34) was obtained from the Regional ethics committee (Lithuanian University of Health Sciences). The Helsinki Declaration (1964) and its later amendments were also followed.
2.2Protocol overview
Three different equipment were used for experiment: regular chair, wobble board (WB) and custom designed wobble board on bearing surface (WBB) (Fig. 1). The tasks were divided into 3 groups: static sitting, anterior and posterior pelvis movement on wobble board (WB1) and on wobble board on bearing surface (WBB1), lateral – medial pelvis movement on wobble board (WB2) and on wobble board on bearing surface (WBB2). There were 5 tasks and each of them was performed once and lasted for 60 s with the break between them. During these tasks simultaneously the kinematics of body segments, electromyography (EMG) of five trunk muscles, electroencephalography (EEG) of 32 scalp electrodes were measured. Before the balancing movements, all subjects sat quietly for 30 s, Fig. A.1. While subjects performed the tasks in the different experimental conditions, a 3D motion capture system was used to record the trajectory of the calibrated anatomical systems technique. Reflective markers were placed on the spine, arms, pelvis, knees, ankles and feet. The locations of the reflective markers were collected using 10 high-speed infrared cameras recording at 300 Hz For one person five tasks were assigned and 5 trunk muscles activity, 13 EEG electrodes and 3 kinematic points were analyzed after experimental measurements. The EMG, EEG and kinematic data were recorded simultaneously.
Figure 1.
Experimental protocol.
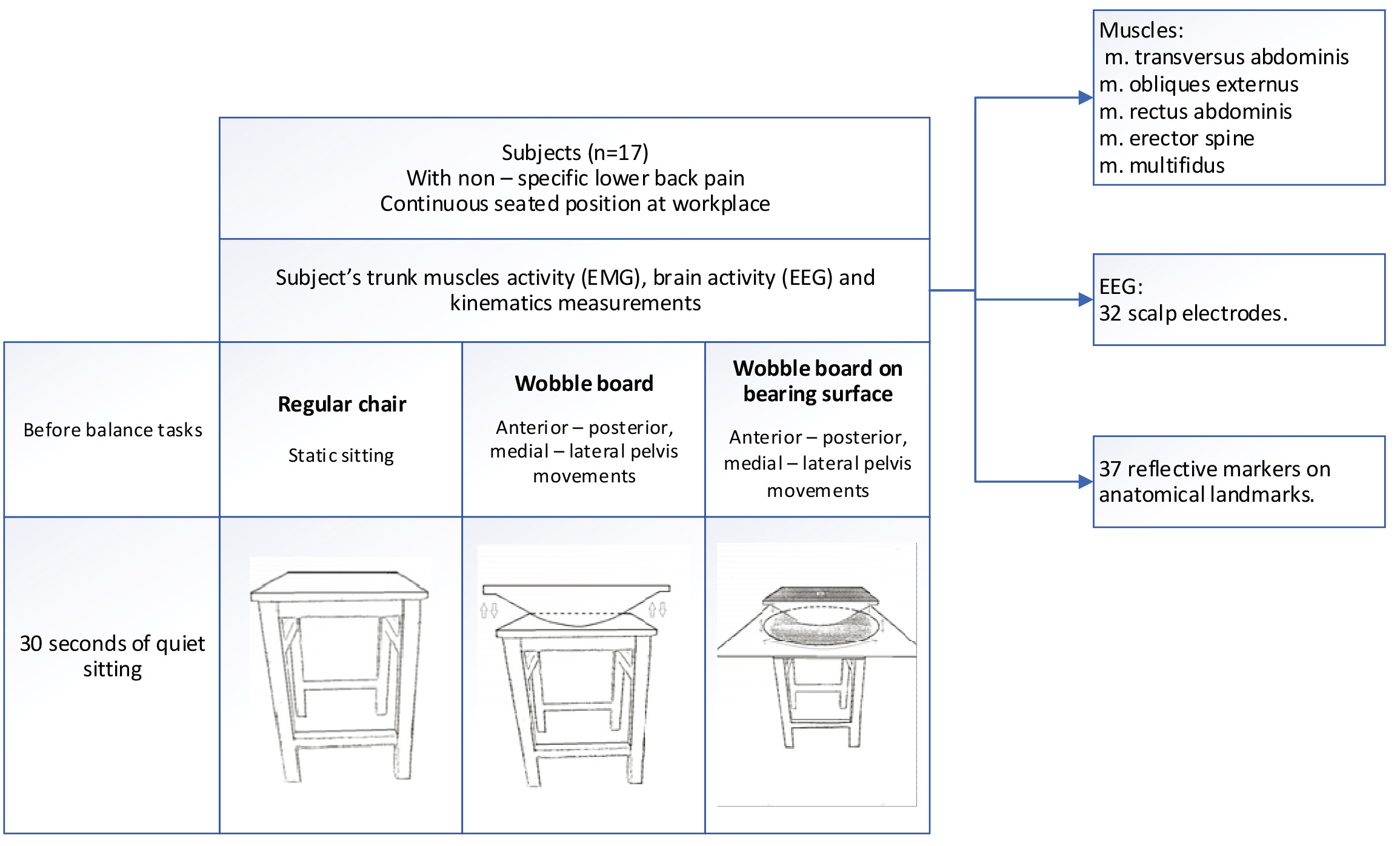
2.3Data acquisition
2.3.1Platform movements
A custom – made wobble board on bearing surface was made, Fig. 2 which was showed in a previous research [14]. This unstable seat has 3 degrees of freedom (DOF), anterior – posterior, lateral – medial movements. Wobble board on bearing surface is made with 6 small bearings beneath the top surface and can move freely in all directions.
Figure 2.
a) A simple wobble board and b) wobble board placed on bearing surface.
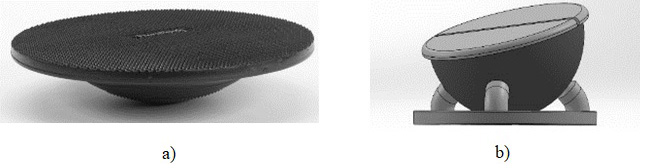
2.3.2Electromyography data acquisition processing and analysis
The trunk muscles activity was measured during the balance tasks by surface electromyography (sEMG) (Noraxon MR3.6). Bipolar sEMG activity was recorded using surface electrodes from the right side of: m. transversus abdominis (TA) 2 cm inferior and medial to the anterior superior iliac spine and parallel to the fibers of the TA [15], m. obliques externus (OE), m. rectus abdominis (RA) 4 cm to the side from the umbilicus, lower border of electrode at umbilicus level [15], m. erector spine (thoraco – lumbar part) (ES) 5 cm lateral to T9 spinous process [16], m. multifidus (MF) aligned with a line from caudal tip posterior spina iliaca superior to the interspace between L1 and L2 interspace at the level of L5 spinous process [17]. Before the surface electrodes placement on the skin was shaved, swabbed and rubbed with alcohol to reduce skin impedance. The sampling rate was set to 1500 Hz.
For further calculations and results comparison between participants, maximum voluntary isometric contraction (MVIC) was performed for each muscle and the sEMG amplitude recorded at the same time. MVIC was performed for every muscle three times, trial lasts 5 seconds with the rest interval between every trial. Mean MVIC EMG amplitude was calculated for each of the three, 5 seconds windows and these data were used for proportion calculations to get the %MVIC. The data obtained from the task procedures of the pelvis were converted to percent according proportion calculation.
After measurement, the offline data processing of electromyography was done for all subjects. sEMG data were band-pass filtered (the frequency range 5–500 Hz) then full wave rectified and smoothened. The amplitude of sEMG data was normalized using the mean dynamic activity method [18].
2.3.3Electroencephalography data acquisition processing and analysis
Electroencephalography was recorded from 32 scalp locations overlying the whole scalp (Fp1, Fp2, F7, F3, Fz, F4, F8, FC5, FC1, FC2, FC6, AF3, AF4, T7, C3, Cz, C4, T8, CP5, CP1, CP2, CP6, P7, P3, Pz, P4, P8, PO7, PO3, PO4, PO8, Oz) [7] and equally distributed over both hemispheres according to the International 10:20-system, using a wireless EEG amplifier, g. Nautilus (g.tec medical engineering GmbH, Austria). Electrode PO8 was placed directly on mastoid bone beneath the EEG cap. The referent position was placed at the right ear. Active electrode sites were prepared by rubbing the scalp with a blunt-tipped needle, which was subsequently used to apply conductive electrode gel (g.gamma gel). Mastoid electrode site was additionally prepared by scrubbing the skin with an alcohol pad [8]. Impedances at primary electrode sites (i.e., mastoids) were
During measurement and after that data were collected and processed using g.BSanalyze software package for Matlab. The EEG data were first high-pass filtered (Butterworth filter, highpass: 0.5 Hz) to remove noise in the lower frequencies. A semiautomatic algorithm was used to exclude segments contaminated by artifacts. For the remaining segmented data of each balance condition an Independent Component Analysis (ICA) algorithm implemented in g.BSanalyze was used for ICA decomposition [7]. Segmented data were then baseline corrected.
To investigate cortical activity changes on two different unstable surfaces, spectral power values for theta (3–7 Hz) and alpha (8–13Hz) frequency bands were calculated (Hanning window).
According to previous studies [7, 20] regions of interest were defined as follows: frontal (F3, Fz, F4), fronto – central (FC1, FC2, C3, Cz, C4), centro – parietal (CP1, CP2, P3, Pz, P4).
2.3.4Kinematic data
Three-dimensional kinematic data were recorded by motion capture with 10 cameras (Oqus7+ Qualisys AB, Gothenburg, Sweden) and the Qualysis Track Manager software at a sampling frequency of 300 Hz. The Helen-Hayes marker set was used for measuring full – body kinematics, Fig. 3. For this study two anatomical points were of major interest: pelvis point – anterior superior iliac spine (ASIS) and spine region point 10
Figure 3.
Full body kinematics. Anatomical points amplitude of movement while sitting on wobble board on bearing surface (WBB) and wobble board (WB) at the same time performing lateral – medial and anterior – posterior movements.
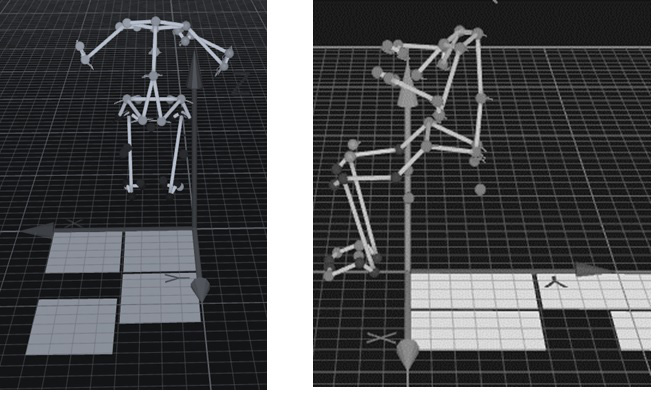
2.4Statistical analysis
The data of 17 participants were analysed with statistical package SPSS version 23.0. For a small sample, the nonparametric Wilcoxon test was used. The data level for statistical significance was set at
3.Results
3.1Electromyography (EMG)
3.1.1Muscles activity change on different sitting surfaces
The increment of MF, EO and TA muscles activity were observed on WBB1 compared with WB1, Fig. B.1, however these results were not statistically significant. Except ES muscle, which activity on WBB1 was 2.45 (3.35
Figure 4.
Muscles activity change in anterior – posterior motion on wobble board (WB1) and muscles activity in anterior – posterior motion on wobble board on bearing surface (WBB1).
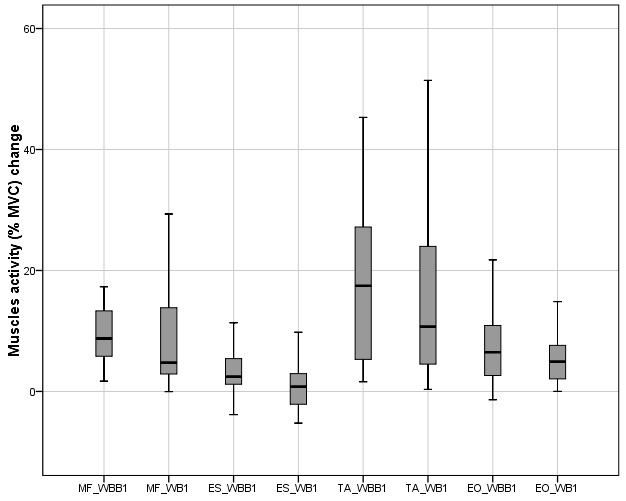
Figure 5.
Muscles activity change in lateral – medial motion on wobble board (WB2) and muscles activity in lateral – medial motion on wobble board on bearing surface (WBB2).
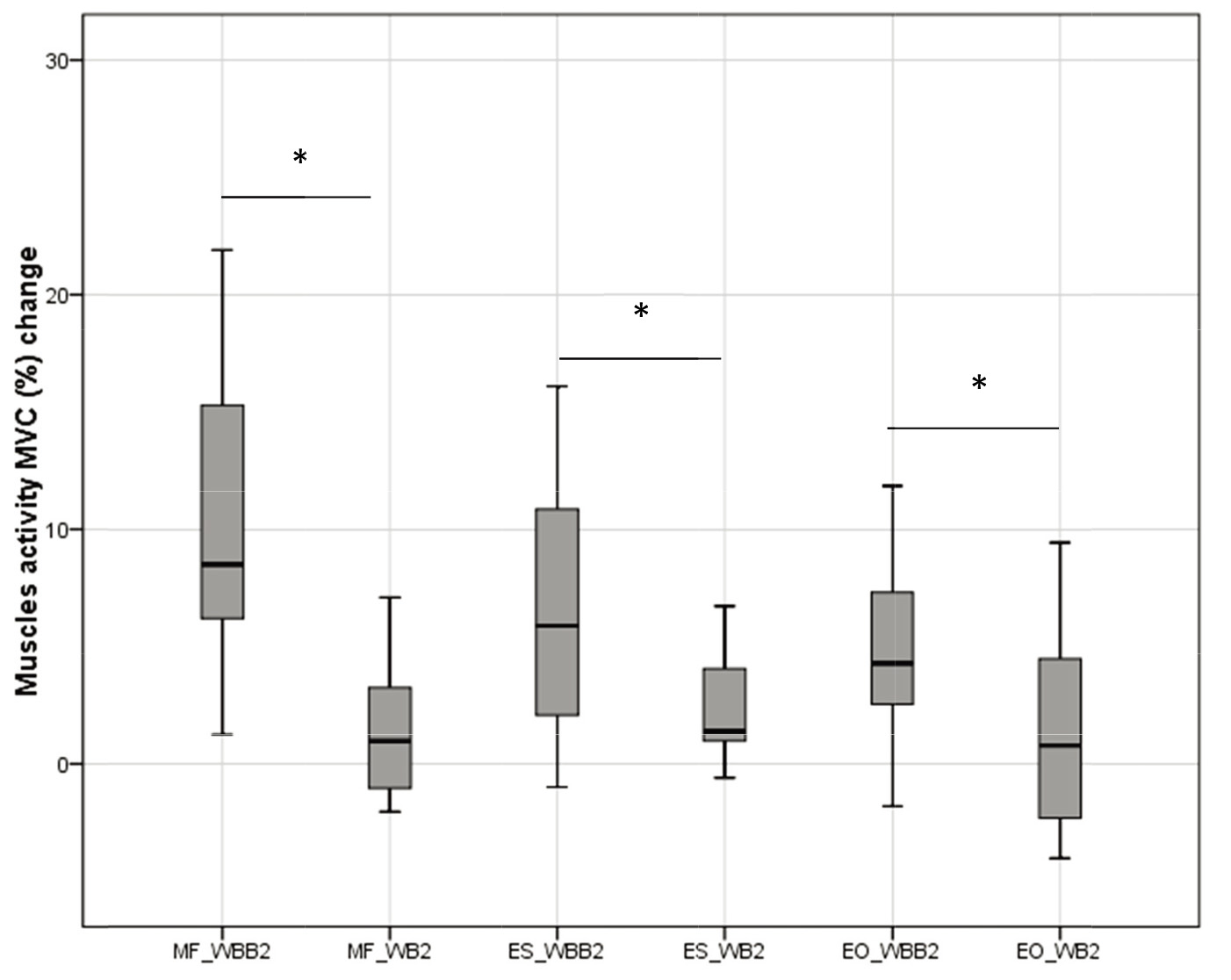
Significant differences of MF, ES and EO activity change on a lateral – medial pelvic movement in sitting on WB and WBB was observed, Fig. 4. Muscles activity change was measured from static sitting. The MF muscle activity on WBB2 was 8.52 (10.79
3.2Electroencephalography (EEG)
3.2.1Alpha power spectral density (PSD)
Alpha wave power spectral density (PSD) was calculated in two different motions: anterior – posterior and medial – lateral on different sitting surfaces: wobble board and wobble board on bearing surface. These results were compared from the static sitting and represented in Table 1.
Table 1
PSD of alpha wave in frontal, fronto – central, centro – parietal regions. The comparison was made next: Static sitting (SS) with anterior – posterior movement on wobble board on bearing surface (WBB1); Static sitting (SS) with anterior – posterior movement on wobble board (WB1); Static sitting (SS) with lateral – medial movement on wobble board on bearing surface (WBB2); Static sitting (SS) with lateral – medial movement on wobble board (WB2). Significant interactions are highlighted in bold type
Frontal region | Fronto – central region | Centro – parietal region | ||||
---|---|---|---|---|---|---|
Median | Median | Median | ||||
WBB1 |
163.85 (210.07 | 0.007 |
200.88 (220.46 | 0.01 |
163.93 (162.27 | 0.041 |
WB1 | 99.08 (134.67 | 0.05 |
175.06 (199.12 | 0.019 | 122.34 (131.52 | 0.182 |
WBB2 |
113.47 (154.62 | 0.004 |
119.05 (201.50 | 0.015 |
110.98 (218.34 | 0.028 |
WB2 | 106.47 (140.30 | 0.131 |
132.34 (163.34 | 0.034 | 115.11 (150.02 | 0.152 |
SS | 58.87 (72.61 | 91.87 (93.23 | 82.92 (88.92 |
3.2.2Theta power spectral density (PSD)
Theta wave power spectral density was calculated in two different motions: anterior – posterior and medial – lateral on different sitting surfaces: wobble board and wobble board on bearing surface. These results were compared from the static sitting and represented in Table 2.
Table 2
PSD of theta wave in frontal, fronto – central, centro – parietal regions. The comparison was made next: Static sitting (SS) with anterior – posterior movement on wobble board on bearing surface (WBB1); Static sitting (SS) with anterior – posterior movement on wobble board (WB1); Static sitting (SS) with lateral – medial movement on wobble board on bearing surface (WBB2); Static sitting (SS) with lateral – medial movement on wobble board (WB2). Significant interactions are highlighted in bold type
Frontal region | Fronto – central region | Centro – parietal region | ||||
---|---|---|---|---|---|---|
Median | Median | Median | ||||
WBB1 |
159.88 (223.69 | 0.005 |
218.94 (226.64 | 0.005 |
122.87 (220.90 | 0.022 |
WB1 |
109.08 (145.23 | 0.033 |
147.15 (192.28 | 0.011 |
129.24 (206.92 | 0.002 |
WBB2 |
110.66 (221.19 | 0.005 |
141.91 (267.26 | 0.005 |
247.01 (287.61 | 0.022 |
WB2 |
99.16 (161.58 | 0.033 |
127.66 (167.49 | 0.011 |
132.06 (172.78 | 0.002 |
SS | 60.73 (74.30 | 55.81 (75.86 | 62.94 (68.32 |
3.3Kinematics
Significant differences (
Figure 6.
Mean of ASIS and Th10 amplitude of movement in anterior – posterior pelvic motion on wobble board on bearing surface (WBB1) and wobble board (WB1).
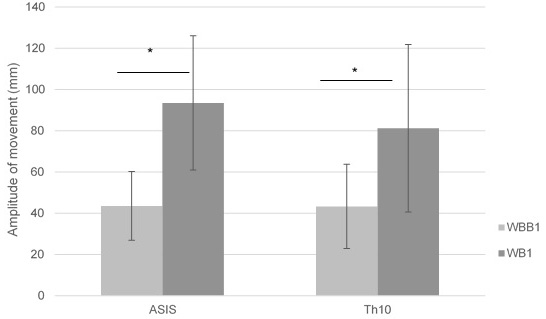
Significant differences (
Figure 7.
Mean of ASIS and Th10 amplitude of movement in lateral – medial pelvic motion on wobble board on bearing surface (WBB2) and wobble board (WB2).
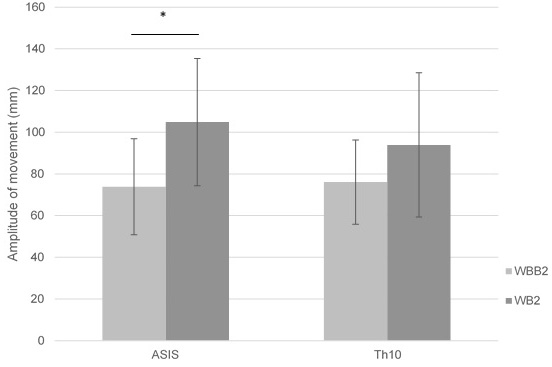
3.4Correlation analysis
Correlation analysis was done comparing Fz, Cz and Pz electrodes PSD and amplitude of movement of ASIS and Th10 anatomical points in two different movements anterior – posterior pelvic motion as well as in lateral – medial pelvic motion on two different platforms. There were no significant correlations on wobble board. Also, we did not find any significant correlations in anterior – posterior pelvic movement.
Figure 8.
a) Correlations between theta power on electrode Pz and ASIS anatomical points amplitude of movement on WBB in lateral – medial movement. b) Correlations between theta power on electrode Pz and Th10 anatomical points amplitude of movement on WBB in lateral – medial movement.
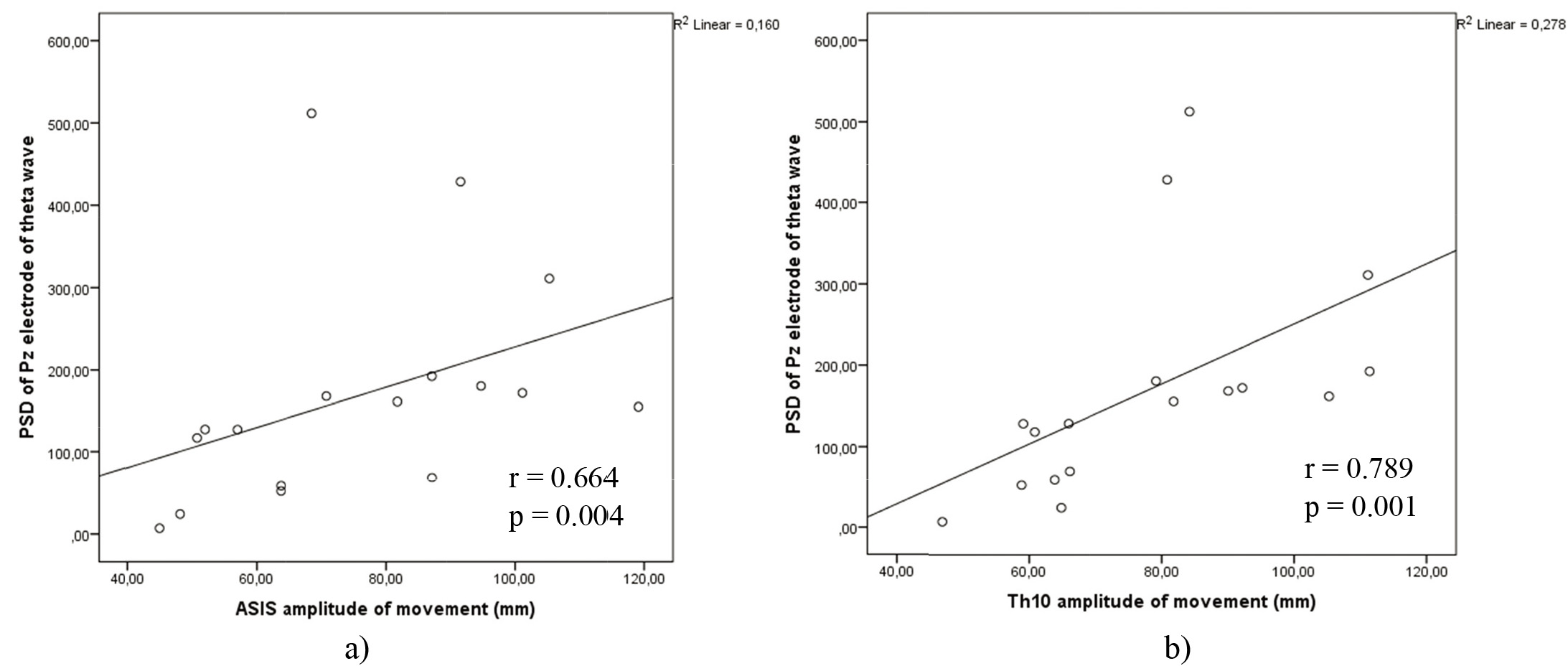
Figure 9.
a) Correlations between alpha power on electrode Fz and ASIS anatomical points amplitude of movement on WBB in lateral – medial movement. b) Correlations between alpha power on electrode Pz and Th10 anatomical points amplitude of movement on WBB in lateral – medial movement.
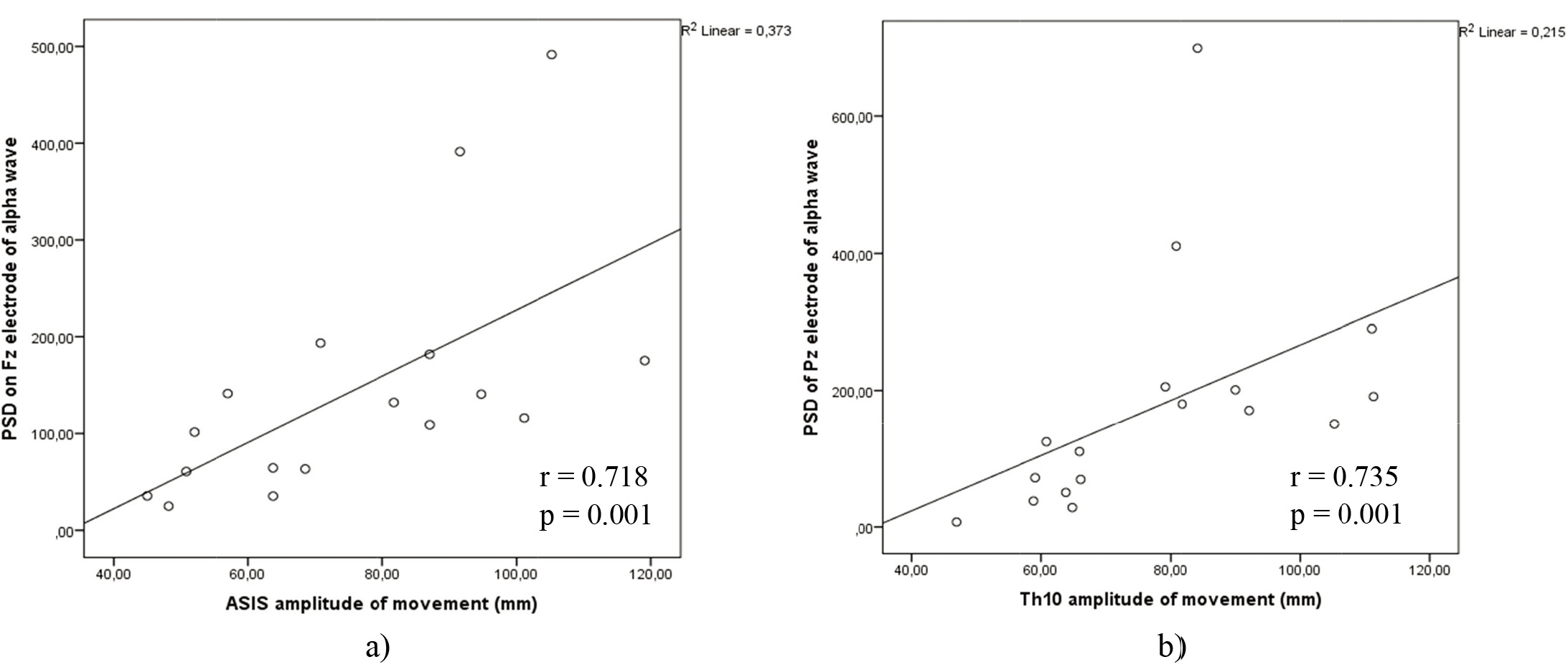
However, the significant correlations displayed in Fig. 8a and b, were seen in lateral – medial movement on WBB of theta power on Pz electrode position with Th10 amplitude of movement (
Alpha power of Fz electrode correlated with Th10 amplitude of movement in lateral – medial motion on WBB (
4.Discussion
The purpose of this research was to evaluate and compare subjects’ trunk muscles activity, cortical theta and alpha activity and kinematic body segments movement in static sitting and when sitting on different platforms: simple wobble board and wobble board placed on bearing surface. Scientific literature lacks research where sitting balance was analyzed as a factor that induces cortical activity. Only in a few studies position transition from sitting to standing was analyzed [21, 22, 23, 24]. However, different sitting conditions are still little analyzed. In the present study cortical theta activity was observed on two different surfaces: wobble board and wobble board on bearing surface when performing two movements anterior – posterior and lateral – medial pelvic movements. We hypothesized that on custom made wobble board on bearing surface pelvic motions will be more challenging according to trunk muscles, cortical activity and kinematic parameters, compared with simple wobble board.
The cortical theta power showed higher activity in fronto – central and centro – parietal regions. Moreover, when two different sitting surfaces were compared: static sitting and WBB, static sitting and WB, we can see that theta activity increased on both surfaces compared with static sitting (
Another important aspect in balance tasks occurs in alpha wave excitation. When two different sitting surfaces were compared: static sitting and WBB, static sitting and WB, it could be seen that alpha activity increased on WBB compared from static sitting (
The amplitude of movement of anatomical points ASIS and Th10 can be seen lower on wobble board on bearing surface compared with wobble board in anterior – posterior and lateral – medial movements (
The activity increment in MF, ES and EO muscles were observed in lateral – medial movement on wobble board placed on bearing surface compared with wobble board (
This study is not without limitations. For instance, the sample size could be bigger, also it is important to evaluate eyes-closed condition in EEG measurements, like other studies did. Further research is needed to overcome these limitations.
5.Conclusions
The results of the present study indicate a global increase in theta and alpha waves PSD when performing anterior – posterior and medial – lateral movements on wobble board and on wobble board on bearing surface, which means that the balance maintenance in sitting position on WB and WBB was challenging. However, theta and alpha waves power in medial – lateral movement on wobble board on bearing surface was found to be strongly correlated to anatomical points amplitude of movement, probably reflecting higher demands on task which evokes balance perturbation. Furthermore, lateral – medial movement on wobble board on bearing surface increase more muscles activity compared with anterior – posterior movement. As previously said, the anterior – posterior movement is more stabilizing and participants with non – specific lower back pain have impaired trunk motor control and stabilization, which less is needed in lateral – medial movement. This study provides new insights of how efficiently improve trunk motor control during hours of prolonged sitting, when neuromuscular control of pelvis and lumbar spine are affected and people with non-specific lower back pain cannot move pelvis isolated, from the findings we can see that WBB can help to feel and increase the pelvis movements. Wobble board on bearing surface could be adjusted to increase trunk muscles activity, especially for those people who have impaired neuromuscular control in lumbo-pelvic region. However, more studies need to be performed, with bigger sample size and including eyes-open, eyes-closed condition, more accurately evaluate cortical response.
Acknowledgments
The authors would like to thank neurologist Vaidotas Gudžiūnas and physiotherapist Simona Puskunigytė-Krasuckienė.
Conflict of interest
None to report.
Funding
The authors received no financial support for the research, authorship, and/or publication of this article.
References
[1] | Andreopoulou G, Maaswinkel E, Cofré Lizama LE, van Dieën JH. Effects of support surface stability on feedback control of trunk posture. Exp Brain Res. (2015) ; 233: (4): 1079–87. |
[2] | AU – WilliamsAD., AU – VetteAH., A Vibrotactile Feedback Device for Seated Balance Assessment and Training, JoVE [Internet]. 2019; (143): e58611. Available from: https://www.jove.com/video/58611. |
[3] | Acasio JC, Nussbaum MA, Hendershot BD. Trunk-pelvic coordination during unstable sitting with varying task demand: A methodological study. J Biomech [Internet]. (2021) ; 118: : 110299. Available from: doi: 10.1016/j.jbiomech.2021.110299. |
[4] | Acasio JC, Butowicz CM, Golyski PR, Nussbaum MA, Hendershot BD. Associations between trunk postural control in walking and unstable sitting at various levels of task demand. J Biomech [Internet]. (2018) ; 75: : 181–5. Available from: doi: 10.1016/j.jbiomech.2018.05.006. |
[5] | Mierau A, Pester B, Hülsdünker T, Schiecke K, Strüder HK, Witte H. Cortical correlates of human balance control. Brain Topogr. (2017) ; 30: (4): 434–46. |
[6] | Williams AD, Kumawat A, Agarwal K, Boser QA, Rouhani H, Vette AH. An instrumented wobble board for assessing and training dynamic sitting balance. 2017 IEEE Int Conf Syst Man, Cybern SMC 2017. 2017; 2017-Janua: 2249–54. |
[7] | Hülsdünker T, Mierau A, Strüder HK. Higher Balance Task Demands are Associated with an Increase in Individual Alpha Peak Frequency. (2016) ; 9: (January): 1–12. |
[8] | Payne AM, Hajcak G, Ting LH. Dissociation of muscle and cortical response scaling to balance perturbation acceleration. J Neurophysiol. (2019) ; 121: (3): 867–80. |
[9] | Slobounov S, Hallett M, Stanhope S, Shibasaki H. Role of cerebral cortex in human postural control: An EEG study. Clin Neurophysiol. (2005) ; 116: (2): 315–23. |
[10] | Larivière C, Preuss R, Ludvig D, Henry SM. Is postural control during unstable sitting a proxy measure for determinants associated with lumbar stability? J Biomech. (2020) ; 102: : 1–9. |
[11] | Hülsdünker T, Mierau A, Neeb C, Kleinöder H, Strüder HK. Cortical processes associated with continuous balance control as revealed by EEG spectral power. Neurosci Lett [Internet]. (2015) ; 592: : 1–5. Available from: http://www.sciencedirect.com/science/article/pii/S0304394015001639. |
[12] | Taubert M, Draganski B, Anwander A, Müller K, Horstmann A, Villringer A, et al. Dynamic properties of human brain structure: Learning-related changes in cortical areas and associated fiber connections. J Neurosci. (2010) ; 30: (35): 11670–7. |
[13] | Diekfuss JA, Grooms DR, Hogg JA, Singh H, Slutsky-Ganesh AB, Bonnette S, et al. Targeted Application of Motor Learning Theory to Leverage Youth Neuroplasticity for Enhanced Injury-Resistance and Exercise Performance: OPTIMAL PREP. J Sci Sport Exerc [Internet]. (2021) ; (0123456789). Available from: doi: 10.1007/s42978-020-00085-y. |
[14] | Aleknaite-dambrauskiene I, Domeika A, Gudžiūnas V, Zaveckas V. Pilot Study of Wobble Seat on Trunk Muscles Activity and Kinematics. (2021) ; 27: (2): 155–8. |
[15] | Flowers DW, Brewer W, Ellison J, Mitchell K, Frilot C. Transversus abdominis activation does not alter gait impairments in patients with and without knee osteoarthritis. Clin Biomech [Internet]. (2021) ; 82: (December 2020): 105270. Available from: doi: 10.1016/j.clinbiomech.2020.105270. |
[16] | McCook DT, Vicenzino B, Hodges PW. Activity of deep abdominal muscles increases during submaximal flexion and extension efforts but antagonist co-contraction remains unchanged. J Electromyogr Kinesiol [Internet]. (2009) ; 19: (5): 754–62. Available from: doi: 10.1016/j.jelekin.2007.11.002. |
[17] | Recommendations for sensor locations in trunk or (lower) back muscles [Internet]. Available from: http://wwwseniam.org/. |
[18] | Freddolini M, Strike S, Lee RYW. The role of trunk muscles in sitting balance control in people with low back pain. J Electromyogr Kinesiol [Internet]. (2014) ; 24: (6): 947–53. Available from: doi: 10.1016/j.jelekin.2014.09.009. |
[19] | Attia M, Hettiarachchi I, Hossny M, Nahavandi S. A time domain classification of steady-state visual evoked potentials using deep recurrent-convolutional neural networks. Proc – Int Symp Biomed Imaging. (2018) ; 2018-April: (Isbi): 766–9. |
[20] | Slobounov S, Hallett M, Cao C, Newell K. Modulation of cortical activity as a result of voluntary postural sway direction: An EEG study. Neurosci Lett [Internet]. 2008/07/12. (2008) Sep 19; 442: (3): 309–13. Available from: https://pubmed.ncbi.nlm.nih.gov/18639613. |
[21] | De Sanctis P, Butler JS, Malcolm BR, Foxe JJ. Recalibration of inhibitory control systems during walking-related dual-task interference: A Mobile Brain-Body Imaging (MOBI) Study. Neuroimage. (2014) ; 94: : 55–64. |
[22] | Malcolm BR, Foxe JJ, Butler JS, De P, Tishman DR, Neurophysiology C, et al. HHS Public Access. (2016) ; 230–42. |
[23] | Mochizuki G, Sibley KM, Cheung HJ, Camilleri JM, McIlroy WE. Generalizability of perturbation-evoked cortical potentials: Independence from sensory, motor and overall postural state. Neurosci Lett. (2009) ; 451: (1): 40–4. |
[24] | Bulea TC, Prasad S, Kilicarslan A, Contreras-Vidal JL. Sitting and standing intention can be decoded from scalp EEG recorded prior to movement execution. Front Neurosci. (2014) ; 8: (Nov): 1–19. |
[25] | Sipp AR, Gwin JT, Makeig S, Ferris DP. Loss of balance during balance beam walking elicits a multifocal theta band electrocortical response. J Neurophysiol. (2013) ; 110: (9): 2050–60. |
[26] | Varghese JP, Beyer KB, Williams L, Miyasike-daSilva V, McIlroy WE. Standing still: Is there a role for the cortex? Neurosci Lett [Internet]. (2015) ; 590: : 18–23. Available from: doi: 10.1016/j.neulet.2015.01.055. |
[27] | Slobounov SM, Teel E, Newell KM. Modulation of cortical activity in response to visually induced postural perturbation: Combined VR and EEG study. Neurosci Lett [Internet]. (2013) ; 547: : 6–9. Available from: doi: 10.1016/j.neulet.2013.05.001. |
[28] | Wittenberg E, Thompson J, Nam CS, Franz JR. Neuroimaging of human balance control: A systematic review. Front Hum Neurosci. (2017) ; 11: (April): 1–25. |
[29] | Varghese JP, Marlin A, Beyer KB, Staines WR, Mochizuki G, McIlroy WE. Frequency characteristics of cortical activity associated with perturbations to upright stability. Neurosci Lett [Internet]. (2014) ; 578: : 33–8. Available from: doi: 10.1016/j.neulet.2014.06.017. |
[30] | Katahira K, Yamazaki Y, Yamaoka C, Ozaki H, Nakagawa S, Nagata N. EEG correlates of the flow state: A combination of increased frontal theta and moderate frontocentral alpha rhythm in the mental arithmetic task. Front Psychol. (2018) ; 9: (MAR): 1–11. |
[31] | Tse YYF, Petrofsky JS, Berk L, Daher N, Lohman E, Laymon MS, et al. Postural sway and rhythmic electroencephalography analysis of cortical activation during eight balance training tasks. Med Sci Monit [Internet]. (2013) Mar 8; 19: : 175–86. Available from: https://www.ncbi.nlm.nih.gov/pubmed/23470794. |
[32] | Roberts BWR, Vette AH. A kinematics recommendation for trunk stability and control assessments during unstable sitting. Med Eng Phys. (2019) ; 73: : 73–6. |
[33] | Roberts BWR, Gholibeigian F, Lewicke J, Vette AH. Spatial and temporal relation of kinematics and muscle activity during unstable sitting. J Electromyogr Kinesiol [Internet]. (2020) ; 52: (November 2019): 102418. Available from: doi: 10.1016/j.jelekin.2020.102418. |
[34] | O’Sullivan K, McCarthy R, White A, O’Sullivan L, Dankaerts W. Lumbar posture and trunk muscle activation during a typing task when sitting on a novel dynamic ergonomic chair. Ergonomics. (2012) ; 55: (12): 1586–95. |
[35] | Kingma I, van Dieën JH. Static and dynamic postural loadings during computer work in females: Sitting on an office chair versus sitting on an exercise ball. Appl Ergon. (2009) Mar; 40: (2): 199–205. |
[36] | Gregory DE, Dunk NM, Callaghan JP. Stability ball versus office chair: Comparison of muscle activation and lumbar spine posture during prolonged sitting. Hum Factors [Internet]. (2006) Mar 1; 48: (1): 142–53. Available from: doi: 10.1518/001872006776412243. |
[37] | O’Sullivan K, O’Sullivan P, O’Keeffe M, O’Sullivan L, Dankaerts W. The effect of dynamic sitting on trunk muscle activation: A systematic review. Appl Ergon [Internet]. (2013) ; 44: (4): 628–35. Available from: doi: 10.1016/j.apergo.2012.12.006. |