A precision neurorehabilitation using SSEP for early detection of sensory deficit and restoration of the motor recovery in balance, gait and activities of daily living in acute stroke
Abstract
BACKGROUND:
Impaired sensory in acute stroke patients results in dynamic balance, gait and activities of daily living (ADL) impairment.
OBJECTIVE:
The aim of present study was to examine the correlation between somatosensory-evoked potential (SSEP) parameters and motor recovery in balance, gait and ADL performance in hemiparetic stroke survivors.
METHODS:
One hundred and one participants with hemiparetic stroke (43 males, 58 females; mean age, 6538
RESULTS:
ANOVA revealed the significant difference (
CONCLUSIONS:
Our research provides therapeutic evidence that correlation of somatosensory functions on motor recovery, balance, gait, and ADL in patients with hemiplegic stroke.
1.Introduction
The integrity of the sensory function is an important biomarker for prediction of the motor recovery and associated balance, gait, and activities of daily living (ADL) after hemiparetic stroke. The extent of initial sensory impairment was closely correlated with motor and functional recovery [1]. Furthermore, proprioception deficit was the strongest determinant factor for balance, ambulation, and ADL in stroke patients. Neurophysiologically, the proprioceptive information includes kinaesthesia, joint movement and joint position sense to maintain the center of mass (COM) within the base of support (BOS) during standing and dynamic walking as well as during ADL. Such sensory inputs are transmitted via the medial lemniscus and the ipsilateral dorsal column tracts to the frontoparietal sensory-motor cortex [2], which in turn executes accurate motor commands for optimal balance and locomotor movement to occur during ADL.
Conventionally, clinical sensory tests include joint position, kinesthetic senses, and tactile senses. However, such sensory tests are not sensitive, but qualitative, and difficult to administer to the acute stroke patients with cognitive impairment. To overcome the shortcoming of the qualitative clinical sensory tests, the somatosensory evoked potential (SSEP) has recently gained a widespread acceptance as a powerful clinical decision-making test to determine the reliability of sensory related to proprioceptive function more precisely than the current clinical tests. SSEP allows detecting the electrophysiological reaction of the central nervous system (CNS) in response to stimulate an electrical sensory of posterior tibial nerve [3]. In fact, recent SSEP studies showed that SSEP provided accurate prognostic appraisal for motor recovery in dynamic standing balance, gait, ADL in acute stroke patients [4, 5, 6]. Nevertheless, it remains unknown that the degree or severity of the sensory impairment function is associated with clinical outcomes of functional motor recovery in sitting balance and postural control in acute stroke patients. Certainly, a precision rehabilitation medicine involves early detection of sensory deficit, which help clinicians to specifically target the sensori-motor integration to better improve sensory function during the acute phase of the neurorehabilitation process, thereby enhancing postural control in sitting and standing position as well as dynamic walking and ADL. Hence, the purpose of the current study was to determine the correlation between the degree of sensory impairment and functional motor recovery in balance (TIS, BBS), gait (FAC), and ADL (Barthel index) in acute stroke patients. The hypothesis was that the degree of sensory impairment affects the prognostic to functional outcome measures in acute stroke patients. In addition, there is a correlation between degree of sensory impairment and functional motor recovery.
2.Methods
2.1Subjects
Eleven participants with hemiparetic stroke (mean age
2.2Somatosensory evoked potential test
Electrophysiological SSEP testing of the central nervous system (CNS) was measured with the Electro Synergy system (Viasys Healthcare; San Diego, CA, USA) to provide real-time information about changes in brain electrical activity in response to therapeutic interventions. Specifically, SSEP measures evoked conduction of an electrical stimulus along the posterior tibial nerve, which is considered an important prediction biomarker to estimate the potential balance, gait, ADL, and lower extremity motor recovery. A bar-electrode was stimulated the posterior tibial nerve. The cathode was placed in the middle position between the achilles tendon and medial malleolus. The cathode was stimulated the posterior tibial nerve with a current of 30 mA [5].
The anode was attached 2 cm distally from the cathode. The signal was recorded by attaching the electrode guidelines into the Fz and attaching the electrode into the Cz as per the 10–20 system (Fig. 1) [7]. The bandpass filter was from 20 to 2000 Hz. The SSEP was measured sensory normal if P37 was more than 41.7 ms, it is normal conversely, if P37 is less than 41.7 ms, sensory impairment, the absence of signal was classified as sensory absent group [8]. According to the standard SSEP data, 33 stroke patients were allocated into the sensory normal group; 36 stroke individuals into the sensory impaired group; 32 stroke individuals were categorized into the sensory absent group.
Figure 1.
A: Bar-electrodes, B: reference electrodes (Fz, Cz).
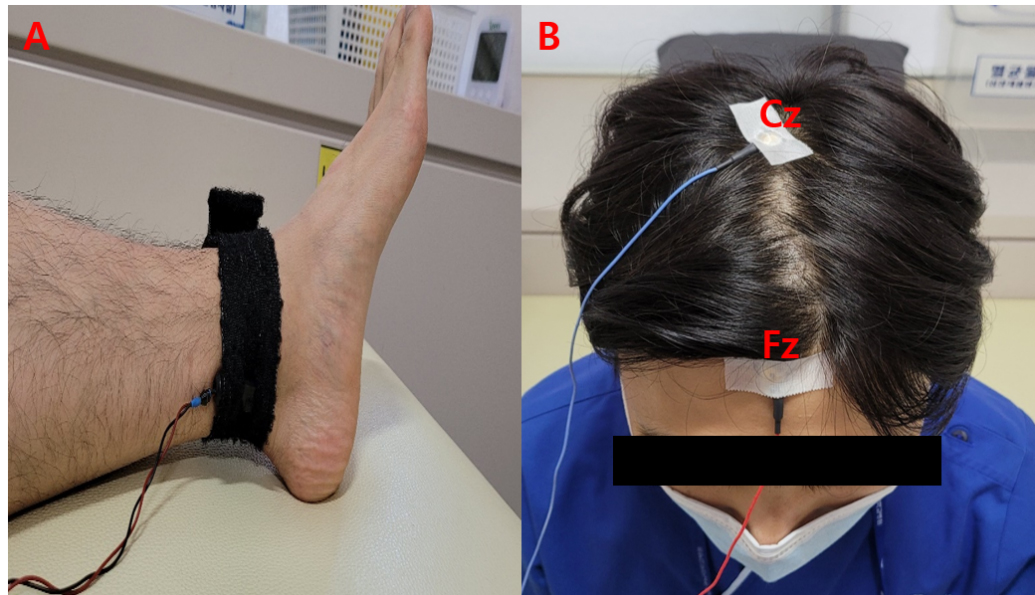
2.3Clinical tests
All participants underwent standardized clinical tests including MBI, FMA, TIS, BBS, and FAC before and after intervention.
2.3.1MBI
MBI is a performance measure to examine an ability to perform ADL, functional mobility; gait in stroke patients. The MBI test is comprised of 10 items, with each item score ranging from 1 to 5 levels (1: Total dependence, 2: Severe dependence, 3: Moderate dependence, 4: Slight dependence, 5: Independence). The maximum score is 100 points [9]. The validity and reliability are reported to be good in stroke patients,
2.3.2FMA
The FMA is a quantitative measure of motor recovery after stroke, which includes the five domains measured as follows: motor function (upper extremity 66 point; lower extremity 34 point), sensory function (24 point), balance (14 point), joint range of motion (44 point), joint pain (44 point). However, in the present study, the lower motor function domain was tested only to determine the relationship between the lower extremity motor recovery function and SSEP in the posterior tibial nerve. Each domain is classified on a 3 point scale (0
2.3.3TIS
TIS is a performance measure which includes the motor impairment of the trunk related with balance on sitting, and trunk movement coordination in hemiparetic stroke patients. The evaluation domain consists of sitting balance on static (3 items), sitting balance on dynamic (10 items), and coordination (4 items), and the total score is 23 points. This scale proved to be excellent reliability for stroke patients (
2.3.4BBS
BBS is a performance evaluate which is designed to assess standing balance in stroke population [13]. This clinical outcome measure consists of a total of 14 items. Each item is to be given scores ranging from 0 point (cannot perform) to 4 points (normal performance) and the full score is 56 points this scale proved to be excellent reliability for stroke patients (ICC
2.3.5FAC
The FAC is categorized patients according to motor abilities necessary for functional ambulation. The FAC has six levels ranging from 0 (non-functional ambulation) to 5 (independent). FAC has excellent reliability in patients with hemiplegia after a stroke (Kappa
2.3.6Intervention
All participants underwent conventional neurodevelopmental rehabilitation intervention for 30 minutes per a day, 5 days a week over a 4 weeks period were recruited for the present study.
2.4Data analysis
The parametric tests included paired t-tests to analyze intervention-related changes before and after the treatment in each group. Analyses of variance (ANOVA) were applied to verify the group difference among the three groups after the treatment. Post-hoc analyses (Scheffe test) were used if significant change was obtained. The Kolmogorov-Smirnov test was analyzed to test for normal distribution. Levene’s test was performed to analyze the homogeneity of variances. The collected data were statistically analyzed using the SPSS Window 25.0 program (SPSS Inc., Chicago, IL, USA). Statistical significance level
3.Results
Table 1 presents the clinical characteristics of the subjects. The Levene’s test didn’t show a significant difference in the baseline characteristics of the subjects, supporting the group homogeneity.
Table 1
Clinical characteristics of the subjects
Sensory normal group ( | Sensory impaired group ( | Sensory absent group ( |
| |
---|---|---|---|---|
Sex | ||||
Male | 12 | 16 | 15 | 0.18 |
Female | 21 | 20 | 17 | |
Lesion | ||||
Cortex | 22 | 26 | 22 | 0.60 |
Subcortex | 11 | 10 | 10 | |
Stroke type | ||||
Infraction | 19 | 18 | 18 | 0.68 |
Hemorrhage | 14 | 18 | 14 | |
Paretic side | ||||
Right | 23 | 21 | 20 | 0.13 |
Left | 10 | 15 | 12 | |
Age | 64.36 | 68.63 | 62.78 | 0.78 |
MMSE | 20.36 | 21.83 | 21.93 | 0.49 |
Onset (month) | 2.18 | 1.86 | 1.93 | 0.52 |
Table 2
Comparison of clinical tests (
Sensory normal group ( | Sensory impaired group ( | Sensory absent group ( | ANOVA | |
---|---|---|---|---|
MBI (score) | ||||
Before | 47.30 | 36.5 | 30.25 | |
After | 61.96 | 46.5 | 37.84 | 9.84 |
Paired | 6.46 | 5.25 | 3.71 | |
FMA (score) | ||||
Before | 21.78 | 15.75 | 11 | |
After | 27.36 | 20.33 | 14.31 | 31.49 |
Paired | 5.04 | 6.59 | 3.74 | |
TIS (score) | ||||
Before | 13.51 | 11.05 | 8.75 | |
After | 19.66 | 16.13 | 12.28 | 14.68 |
Paired | 9.46 | 7.89 | 6.92 | |
BBS (score) | ||||
Before | 23.57 | 11.38 | 10 | |
After | 42.03 | 23.25 | 16.87 | 26.68 |
Paired | 9.22 | 7.38 | 6.08 | |
FAC (grade) | ||||
Before | 2 | 1.19 | 0.71 | |
After | 3.51 | 2.25 | 1.31 | 29.57 |
Paired | 10 | 10.86 | 5.46 |
Note. Mean
Table 2 shows the comparative clinical data across the three groups pre and post intervention. That all the three groups showed significant differences between before and after the study (
4.Discussion
The current research indicated a correlation of the effects of baseline somatosensory functions in the individuals who had the acute stroke patients to their motor recovery and associated balance, gait and ADL after stroke rehabilitation. As anticipated, Clinical evaluation demonstrated that NSG in acute stroke patients exhibited grater improvements in motor function, sitting balance, standing balance, gait performance and ADL than those who had impaired somatosensory function. These results are consistent with previous researches [16, 17] that participants with normal somatosensory functions examined greater improvement of motor recovery function evaluated by BBS, FAC and MBI score compared to participants with impaired somatosensory function after stroke inpatient rehabilitation. Other study [18] were reported that improvement of lower limb motor function (FMA) when somatosensory evoked potential is normal. Dynamic standing balance, gait, ADL in patients with acute stroke has been due to various variables, including sensory impairment, muscle weakness, movement coordination and dexterity deficit. These problems were eventually caused by sensory dysfunction. In case, impaired sensory ability didn’t transfer precise information to primary motor cortex. Inadequate input of sensory information leads to inefficient and uncontrolled movements. These results have affected for motor performance, standing balance, gait, and ADL. However, there was no study effect of sitting position on postural control according to the severity of lower extremity sensation in SSEP. In the present study, there was a significant difference in the postural control of sitting position among the groups according to degree of sensory impairment. The spine of the body consists of active and passive structures: the passive structures of the pelvis and thoracolumbar spine and the active parts of the trunk muscles [19]. Akuthota and Nadler [20] portrayed the core with the abdominals in the front, the paraspinals and gluteus in the behind, the diaphragm as the roof and the pelvic floor and hip girdle musculature as the bottom side. The hip girdle musculature plays an essential role within the kinetic chain, especially for all movement, in stability of the trunk and pelvis and in shifting force from the lower extremities to the pelvis and spine. Most stroke patients had a decreased sensory ability. Thus, altered somatosensory input spread from the included limb to the primary motor cortex by ascending pathways could be generated unacceptable motor output to the spinal cord by descending pathways in SIG and SAG [21]. Incorrect motor output of the lower extremity leads to changes in the CNS, resulting in decreased dynamic balance, postural regulation, and increasing postural sway [22]. Hence, meaningful sensory input provides valuable feedback to the dynamic balance control making it an important mechanism in supporting the function of the somatosensory system overall [23]. These results of study indicate that the level of posterior tibial nerve impairment can provide to motor function, balance, gait and ADL after stroke. Clinically, this study presents insights into improving functional ability in acute stroke survivors.
5.Conclusions
The current study suggested a correlation of baseline somatosensory functions in the individuals who had an acute stroke patient to their motor recovery and associated balance, gait and ADL after stroke rehabilitation. As anticipated, the normal sensory function group showed superior improvements in motor recovery, balance, gait and ADL than patients with impaired and absent somatosensory function group.
Conflict of interest
None to report.
References
[1] | Turville ML, Matyas TA, Blennerhassett JM, Carey LM. Initial severity of somatosensory impairment influences response to upper limb sensory retraining post-stroke. NeuroRehabilitation. (2018) ; 43: (4): 413-23. |
[2] | Nuwer MR. Fundamentals of evoked potentials and common clinical applications today. Electroencephalography and Clinical Neurophysiology. (1998) ; 106: (2): 142-8. |
[3] | Regan D. Some characteristics of average steady-state and transient responses evoked by modulated light. Electroencephalography and Clinical Neurophysiology. (1966) ; 20: (3): 238-48. |
[4] | Lee SY, Kim BR, Han EY. Association between evoked potentials and balance recovery in subacute hemiparetic stroke patients. Annals of Rehabilitation Medicine. (2015) ; 39: (3): 451. |
[5] | Yoon HS, Cha YJ, Sohn MK, You JSH. Effect of rehabilitation on the somatosensory evoked potentials and gait performance of hemiparetic stroke patients. Technology and Health Care. (2018) ; 26: (S1): 145-50. |
[6] | Pollock A, Baer G, Campbell P, Choo PL, Forster A, Morris J, et al. Physical rehabilitation approaches for the recovery of function and mobility following stroke. Cochrane Database of Systematic Reviews. (2014) (4). |
[7] | Tzvetanov P, Rousseff RT, Atanassova P. Prognostic value of median and tibial somatosensory evoked potentials in acute stroke. Neuroscience Letters. (2005) ; 380: (1-2): 99-104. |
[8] | Miura T, Sonoo M, Shimizu T. Establishment of standard values for the latency, interval and amplitude parameters of tibial nerve somatosensory evoked potentials (SEPs). Clinical Neurophysiology. (2003) ; 114: (7): 1367-78. |
[9] | Shah S, Vanclay F, Cooper B. Improving the sensitivity of the Barthel Index for stroke rehabilitation. Journal of Clinical Epidemiology. (1989) ; 42: (8): 703-9. |
[10] | Rollnik J. The early rehabilitation Barthel index (ERBI). Die Rehabilitation. (2011) ; 50: (6): 408-11. |
[11] | Duncan PW, Propst M, Nelson SG. Reliability of the Fugl-Meyer assessment of sensorimotor recovery following cerebrovascular accident. Physical Therapy. (1983) ; 63: (10): 1606-10. |
[12] | Verheyden G, Nieuwboer A, Feys H, Thijs V, Vaes K, De Weerdt W. Discriminant ability of the Trunk Impairment Scale: a comparison between stroke patients and healthy individuals. Disability and Rehabilitation. (2005) ; 27: (17): 1023-8. |
[13] | Berg K, Wood-Dauphinee S, Williams J. The Balance Scale: reliability assessment with elderly residents and patients with an acute stroke. Scandinavian Journal of Rehabilitation Medicine. (1995) ; 27: (1): 27-36. |
[14] | Mao H-F, Hsueh I-P, Tang P-F, Sheu C-F, Hsieh C-L. Analysis and comparison of the psychometric properties of three balance measures for stroke patients. Stroke. (2002) ; 33: (4): 1022-7. |
[15] | Viosca E, Martínez JL, Almagro PL, Gracia A, González C. Proposal and validation of a new functional ambulation classification scale for clinical use. Archives of Physical Medicine and Rehabilitation. (2005) ; 86: (6): 1234-8. |
[16] | Hwang P, Sohn MK, Kim CS, Jee S. Tibial somatosensory evoked potential can prognosticate for ambulatory function in subacute hemiplegic stroke. Journal of Clinical Neuroscience. (2016) ; 26: : 122-5. |
[17] | Tzvetanov P, Rousseff RT. Median SSEP changes in hemiplegic stroke: long-term predictive values regarding ADL recovery. NeuroRehabilitation. (2003) ; 18: (4): 317-24. |
[18] | Kim SW, Kim SB, Lee SY, Koh SE, Lee JM, Lee JY. Motor evoked potentials and somatosensory evoked potentials of upper and lower extremities for prediction of functional recovery in stroke. Brain & Neurorehabilitation. (2012) ; 5: (1): 24-31. |
[19] | Willard F, Vleeming A, Schuenke M, Danneels L, Schleip R. The thoracolumbar fascia: anatomy, function and clinical considerations. Journal of Anatomy. (2012) ; 221: (6): 507-36. |
[20] | Akuthota V, Nadler SF. Core strengthening. Archives of Physical Medicine and Rehabilitation. (2004) ; 85: : 86-92. |
[21] | Canedo A. Primary motor cortex influences on the descending and ascending systems. Progress in Neurobiology. (1997) ; 51: (3): 287-335. |
[22] | Ivanenko Y, Gurfinkel VS. Human postural control. Frontiers in Neuroscience. (2018) ; 12: : 171. |
[23] | Woo MT, Davids K, Liukkonen J, Orth D, Chow JY, Jaakkola T. Effects of different lower-limb sensory stimulation strategies on postural regulation – a systematic review and meta-analysis. PloS One. (2017) ; 12: (3): e0174522. |