Exercise-related blood pressure response is related to autonomic modulation in young adults: A new extension study
Abstract
BACKGROUND:
The delayed blood pressure recovery (BPR) at post-exercise has been in association with a major risk of cardiovascular disease and death.
OBJECTIVE:
The study focused on evaluating the systolic and diastolic blood pressure recovery (SBPR, DBPR) and the autonomic modulation following treadmill exercise in healthy young adults. Although considerable literature had been published about BPR and HRV, the association between BPR and ultra-short-term HRV has not yet been completely described.
METHODS:
Fifteen subjects performed exercise with three different intensities on a treadmill, the speed was 6 km/h, 9 km/h, 12 km/h, respectively. SBP and DBP was measured per 30 s in each trial. The synchronous 5-min electrocardiogram (ECG) signals were recorded and HRV
RESULTS:
The intraclass correlation coefficient (ICC) values and the Bland-Altman plots indicated good consistency and repeatability between HRV
CONCLUSIONS:
These observations represented a new insight into the cardiovascular regulation at post-exercise, which could contribute to physical exercise areas in the future.
1.Introduction
Prehypertension or hypertension is a main risk factor of cardiovascular diseases, i.e., coronary artery disease, stroke and diabetes [1, 2]. The exaggerated BP response to exercise was reported to have a high incidence of hypertension due to the early alternations in cardiovascular hemodynamic and endothelial dysfunction [3, 4]. Thus, a practical clinical technique to evaluate cardiovascular health is the assessment of blood pressure recovery (BPR) at post-exercise. BP response to exercise is frequently used to evaluate various aspects of cardiovascular modulation [5, 6].
Heart rate variability (HRV) is a common and useful tool in assessing autonomic nervous system function, which is used in many academic areas [7]. Short-term HRV is believed to be an independent factor of some chronic diseases [8]. It is considered that the increasing mortality of cardiovascular diseases is related to the sudden changes in antonomic regulation at post-exercise, which could be reflected by HRV parameters [9, 10, 11]. Due to the prevalence of HRV in physical exercise, more literatures aim to pay attention to the application of accurate HRV assessment in exercise. For example, it was reported that a larger convertion in sympathetic-vagal balance was resulted from higher intersity exercise in Parekh’s study [12]. Besides, it was supported that HRV monitor could help to track the exercise program of training adaptation training adaptation in exercise training, and set the appropriate physical training loads to improve the performance for athletes [13, 14, 15].
BPR and HRV responses to post-exercise are related to the intensity of aerobic exercise, particularly in the first minutes of recovery [12, 16]. Lewis et al. [17] found that the prolonged sympathetic predominance and slow parasympathetic reactivation at post-exercise contributed to a delayed BPR. Therefore, the investigation of the impact of exercise on autonomic modulation and BPR may provide further insight into physical exercise areas.
To our knowledge, most of the literature which bases on studying BPR and HRV at post-exercise is independent. The purpose of this study was to investigate the effects of treadmill post-exercise on autonomic nervous modulation and BPR. Instead of using the standard HRV
2.Methods
2.1Subjects
Fifteen healthy were recruited to take part in this study. The measurement of the average age and the body mass index (BMI) was 22.3
2.2Experimental procedure
Before ECG signal acquisition, subjects were asked to seat quietly and breathe naturally for 10 min. Then, ECG signal was collected for 5-min duration as a baseline (Rest). Subsequently, subjects were ask to take the warm-up phase for 60 s on the treadmill, which was to ensure that the sensors were firmly attached and subjects could be familiar with the procedure. Later, ECG signals were recorded for other three 5-min periods as soon as each performance was ended. The treadmill speed was adjusted to 6 km/h (Post-E1), 9 km/h (Post-E2) and 12 km/h (Post-E3), respectively. To elimilate the impacts on the repeated measurements of HRV, the initial BP and HR prior to each trial was recorded to guarantee that cardiovascular modulation had returned to Rest condition level. Participants were allowed to maintain stable to reduce the motion artifact. Each trial lasted for 3 mins with the duration of 30 mins in-between trials and were performed in a random order.
2.3Blood pressure monitoring
Brachial cuff-BP was measured by a digital BP monitor device (HEM-7211, Omron Healthcare, Japan). Subjects were required to sit quitely with the cuff wrapped around the right arm at the same level between its center and the subject’s heart. Cuff-BP was measured every 30 s and 10 cuff-BPs were recorded for BPR assessment in each trial. The values that each cuff-BP minus the mean BP at Rest condition were taken as BPR.
Specifically,
(1)
2.4Data acquistition and HRV measures
In this study, the Power-Lab/16sp system (Castle Hill, AD Instruments, Australia) was used to collect and amplify the ECG signal. The frequency was set at 1 kHz. Subsequently, the signals were filtered by a 45-Hz low-pass filter and a 1-Hz high-pass filter. The ectopic beats were visually identified, then, the abnormal data were replace by linear interpolation of adjacent RR intervals. The normal cardiac intervals were automatically measured and exported for HRV analysis.
Due to the 30 s consumption for the measurement of each cuff-BP, 30 s ultra-short-term HRV analysis was used to correlate with the BPR in this study. The consecutive HRV
2.5Statistical analysis
The software SPSS Statistics (version 24.0) was used for the statistical analysis. All indices were presented as mean
3.Results
3.1Ultra-short term HRV30s as a surrogate of standard HRV5min
The results of initinal BP and HR prior to each trial are summarized as mean
Table 1
The variation of HR and BP in repeated measurement prior to each trial, and the intraclass correlation coefficient (ICC) in four trials
Rest | Post-E1 | Post-E2 | Post-E3 | ICC | ||
---|---|---|---|---|---|---|
HR | 77.29 | 76.23 | 76.20 | 76.92 | 0.990 |
0.943 |
SBP | 116.76 | 116.07 | 118.21 | 117.64 | 0.940 |
0.831 |
DBP | 71.54 | 68.79 | 69.14 | 71.28 | 0.731 |
0.906 |
Note:
Figure 1.
The Bland-Altman analysis between HRV
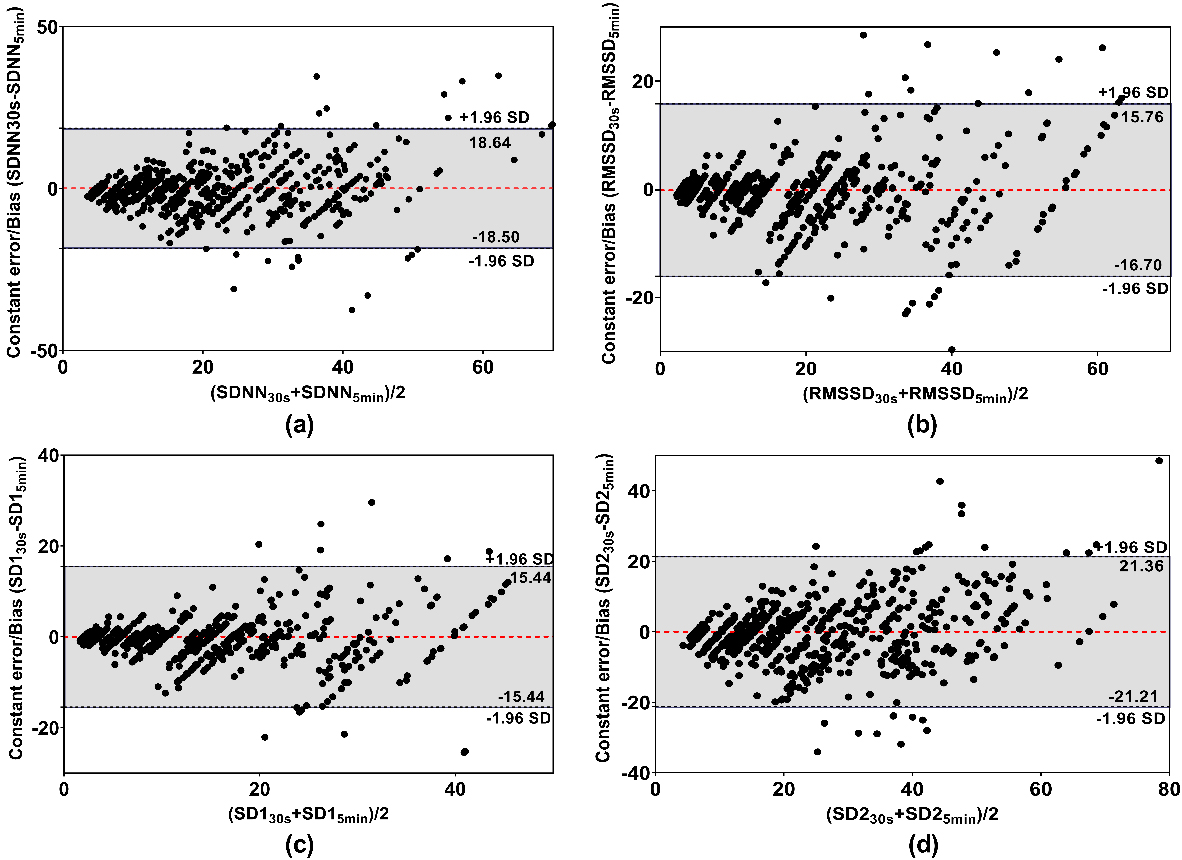
Table 2
The ICC results between HRV
SDNN | RMSSD | SD1 | SD2 | |
---|---|---|---|---|
Rest |
0.890 |
0.876 |
0.877 | 0.718 |
Post-E1 |
0.767 |
0.821 |
0.784 | 0.708 |
Post-E2 |
0.796 |
0.753 |
0.754 |
0.756 |
Post-E3 |
0.772 |
0.837 |
0.823 |
0.818 |
Note: ICC was used for analyzing the repeatability and consistency between HRV
Bland-Altman plots of the differences between HRV
Continuous short-term HRV
Figure 2.
The continuous time-domain HRV
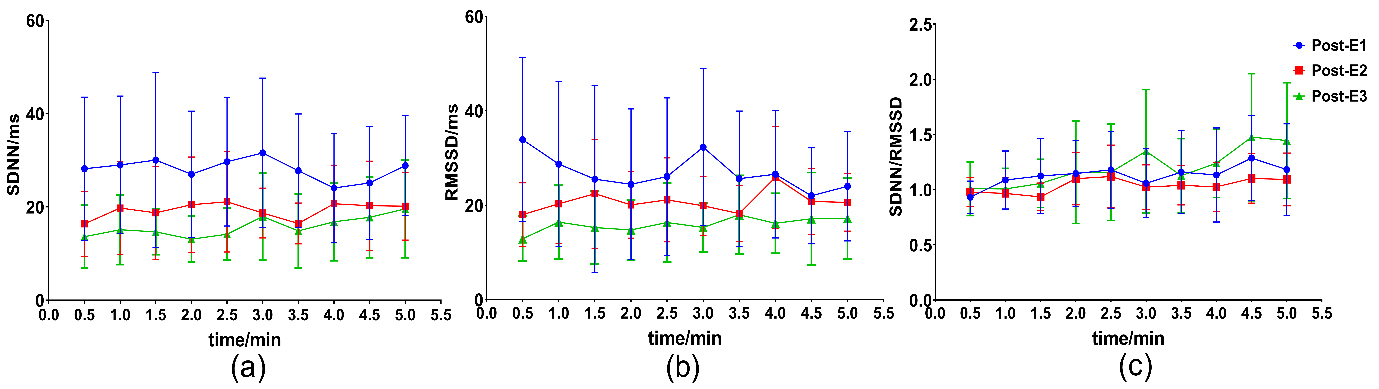
Figure 3.
The continuous non-linear HRV
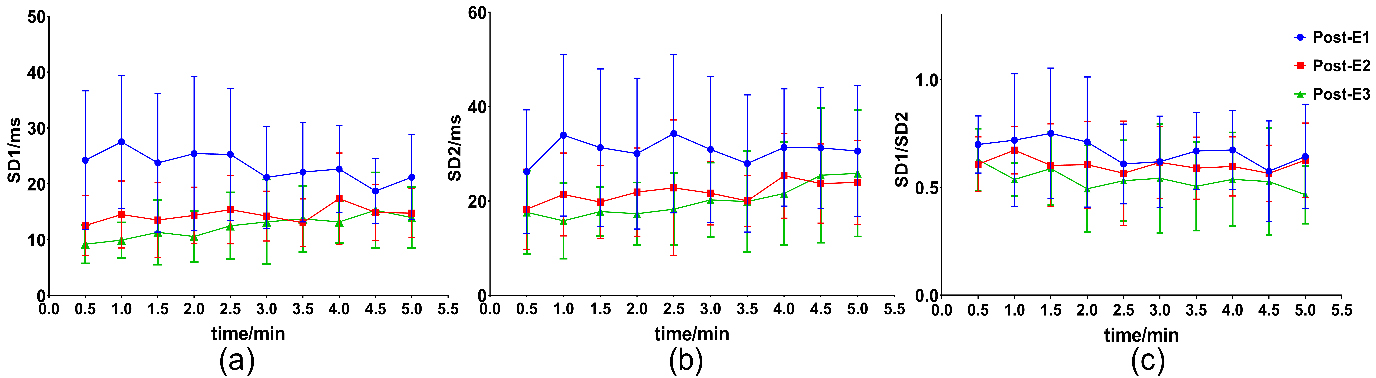
3.2BPR and the relationship with HRV30s
The results of simultaneous SBPR and DBPR are illustrated in Fig. 4. As expected, SBPR and DBPR generally decreased and returned to the Rest level in nearly 4
There were correlations between HRV
Table 3
Spearman correlation coefficients between HRV
SDNN | RMSSD | SDNN/RMSSD | SD1 | SD2 | SD1/SD2 | |
---|---|---|---|---|---|---|
SBPR | ||||||
Post-E1 | 0.295 |
0.691 |
|
0.626 |
0.607 | |
Post-E2 |
|
|
0.618 | |||
Post-E3 |
|
0.733 |
| 0.740 |
|
0.709 |
DBPR | ||||||
Post-E1 | 0.221 | 0.572 |
|
0.732 |
0.679 | |
Post-E2 |
|
|
0.635 | |||
Post-E3 |
|
|
0.905 |
|
0.730 |
Figure 4.
The continuous blood pressure recovery at post-exercise trials. (a) is systolic BP recovery (SBPR), (b) is diastolic BP recovery (DBPR).
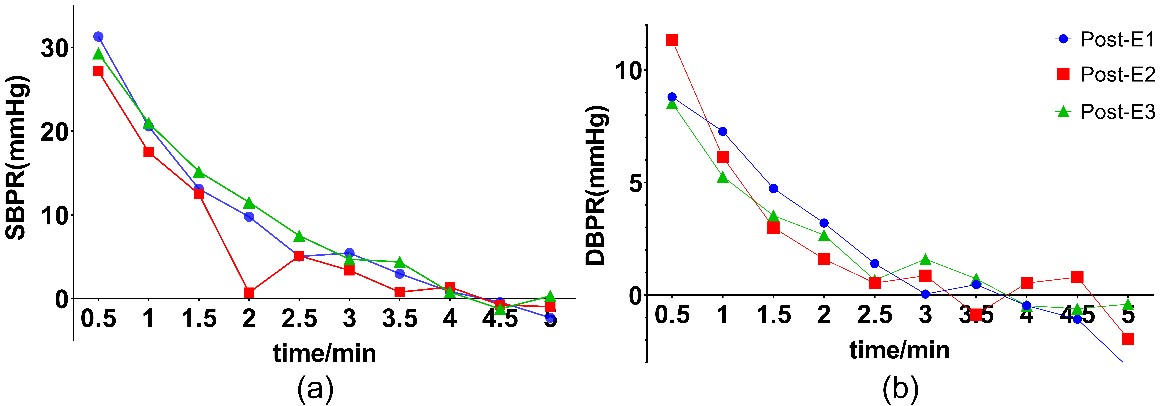
Figure 5.
The Spearman correlation between SBPR and HRV
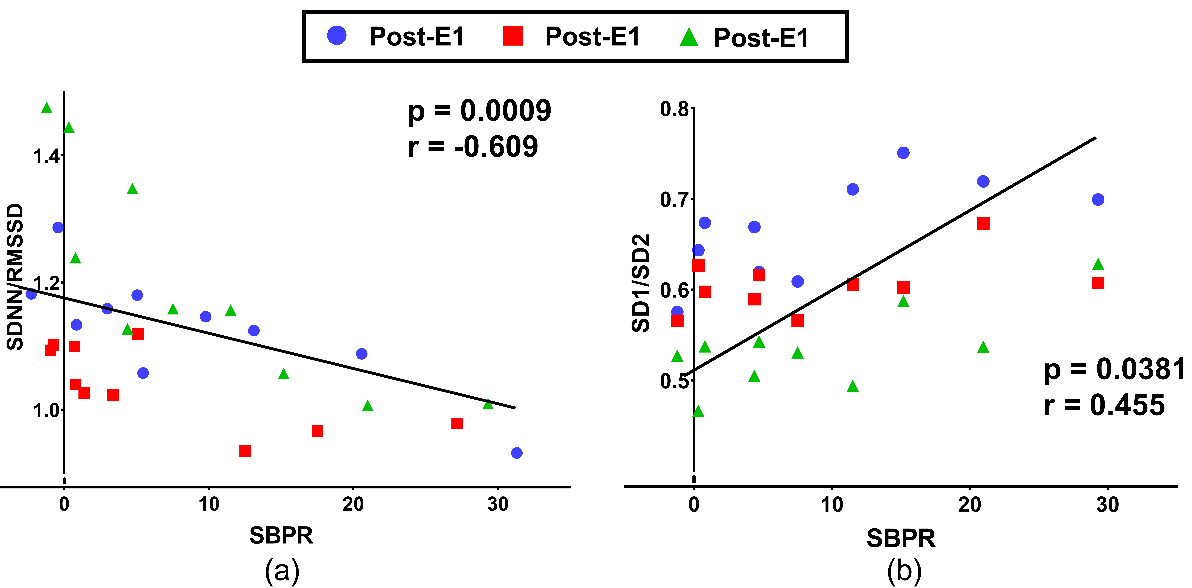
Figure 6.
The Spearman correlation between DBPR and HRV
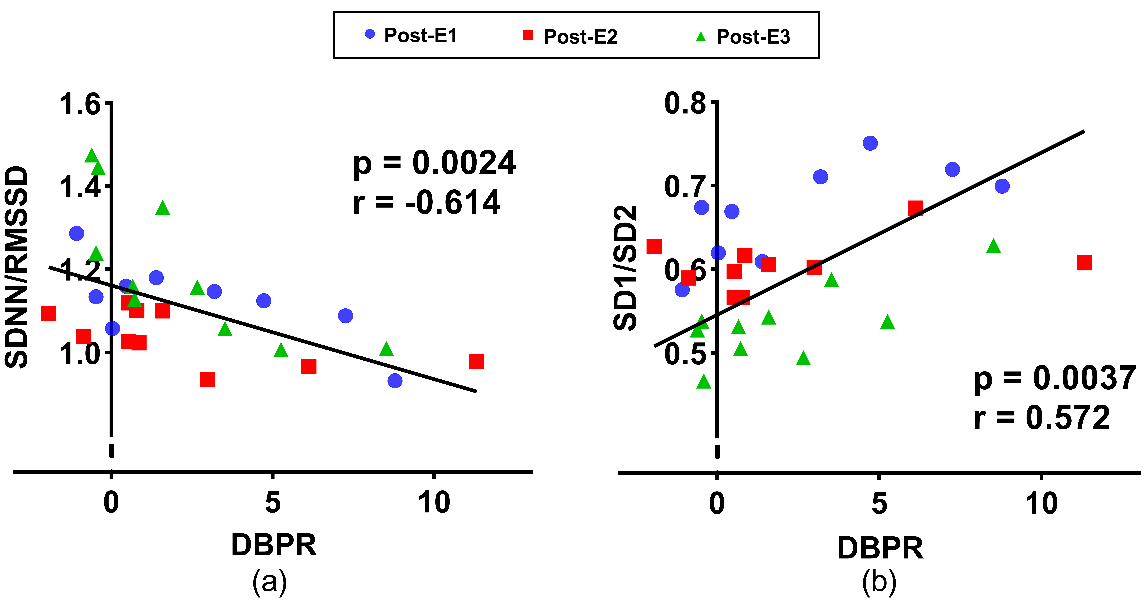
4.Discussion
In the present study, we investigated the effects of treadmill exercise on cardiovascular modulation and BPR during the recovery period. The continuous autonomic response was evaluated by ultra-short-term HRV
Physical exercise could lead to great alteration in stroke volume and the increase of peripheral vasoconstriction. Three major mechanisms were thought to be liable for neurocardiovascular regulation during physical exercise, i.e., activation of the cerebral nervous system, the baroreceptor afferents and the activity from chemical and mechanical receptors [18, 19, 20, 21]. The neural circuits in the medulla are activated by these mechanisms so as to regulate the balance of the sympathetic and parasympathetic tone. However, some factors can influence the physiological responses to exercise for each mechanism, i.e., exercise mode and intensity, muscle fiber type or recruited muscle mass [22]. The responses were also reflected in the autonomic alteration, as assessed via HRV parameters. Time-domain parameters including SDNN, RMSSD and ratio SDNN/RMSSD, and non-linear parameters including SD1, SD2 and ratio SD1/SD2 were utilized in the present study. SDNN and SD2 were affected by both sympathetic and parasympathetic activities [23]; RMSSD and SD1 were considered to represent vagal modulation [7]; while ratio SDNN/RMSSD and ratio SD1/SD2 could be used as an indicator of sympathovagal balance [7, 24].
Instead of using the standard HRV
Furthermore, the decreased SDNN
Physical exercise-related blood pressure response was demonstrated to have prognostic implications in clinical study. The exaggerated BP response to exercise was caused by the increased peripheral vascular resistance and impaired capacity of vasodilatation in the early period of hypertension [4, 31]. BPR was extensively studied to assess the cardiovascular system. The whole exercise period was dynamic with various changes of physiological conditions. Treadmill exercise required a lot of muscle mass participation, which could produced a volume load on cardiovascular system. In general, the increase of vascular conductance and the decrease of vascular resistance were usually greater than the elevations of cardiac output after dynamic exercise, which indicated that the BPR was triggered by vasodilation [32]. On the other hand, there was an interesting phenomenon in our study: SBP had recovered to rest level at the 4
Besides, it had been proposed that the cerebral nervous mechanism played an important role in cardiovascular modulation, which was related to the vasodilation at post-exercise as a feed-forward system [34]. That was the reason that the correlation between HRV
The major limitations of this study are the lack of different age ranges, i.e., younger or older paticipants, which indicated that the results could not be precisely extrapolated for a wider population. Additionally, only one exercise mode was performed to investigate the correlation between HRV
5.Conclusions
In summary, exercise-related high blood pressure was a common phenomenon, which had a great relationship with autonomic modulation and vasoconstriction. The ultra-short-term HRV
Acknowledgments
The authors would like to express their gratitude to the volunteers who participated in this study and generously contributed their time.
Conflict of interest
All authors read and approved the final manuscript. The authors declare that they have no conflict of interest.
References
[1] | Townsend RR, Wilkinson IB, Schiffrin EL, Avolio AP, Chirinos JA, Cockcroft JR, et al. Recommendations for improving and standardizing vascular research on arterial stiffness: a scientific statement from the american heart association. Hypertension. (2015) ; 66: (3): 698-722. |
[2] | Lewington S, Clarke R, Qizilbash N, Peto R, Collins R, Prospective Studies C. Age-specific relevance of usual blood pressure to vascular mortality: a meta-analysis of individual data for one million adults in 61 prospective studies. Lancet. (2002) ; 360: (9349): 1903-13. |
[3] | Guo XF, Zhang XY, Guo L, Li Z, Zheng LQ, Yu SS, et al. Association between pre-hypertension and cardiovascular outcomes: a systematic review and meta-analysis of prospective studies. Curr Hypertens Rep. (2013) ; 15: (6): 703-16. |
[4] | Turmel J, Bougault V, Boulet LP, Poirier P. Exaggerated blood pressure response to exercise in athletes: dysmetabolism or altered autonomic nervous system modulation? Blood Press Monit. (2012) ; 17: (5): 184-92. |
[5] | Charansonney OL. Exercise testing in the 21st century: from an “old” diagnostic to a novel health risk assessment tool. Discov Med. (2012) ; 14: (77): 247-52. |
[6] | Pecanha T, Bartels R, Brito LC, Paula-Ribeiro M, Oliveira RS, Goldberger JJ. Methods of assessment of the post-exercise cardiac autonomic recovery: a methodological review. Int J Cardiol. (2017) ; 227: : 795-802. |
[7] | Heart rate variability. Standards of measurement, physiological interpretation, and clinical use. Task Force of the European Society of Cardiology and the North American Society of Pacing and Electrophysiology. Eur Heart J. (1996) ; 17: (3): 354-81. |
[8] | Okutucu S, Karakulak UN, Aytemir K, Oto A. Heart rate recovery: a practical clinical indicator of abnormal cardiac autonomic function. Expert Rev Cardiovasc Ther. (2011) ; 9: (11): 1417-30. |
[9] | Kannankeril PJ, Le FK, Kadish AH, Goldberger JJ. Parasympathetic effects on heart rate recovery after exercise. J Investig Med. (2004) ; 52: (6): 394-401. |
[10] | Tulppo MP, Kiviniemi AM, Hautala AJ, Kallio M, Seppanen T, Tiinanen S, et al. Sympatho-vagal interaction in the recovery phase of exercise. Clin Physiol Funct Imaging. (2011) ; 31: (4): 272-81. |
[11] | Curtis BM, O’Keefe JH, Jr. Autonomic tone as a cardiovascular risk factor: the dangers of chronic fight or flight. Mayo Clin Proc. (2002) ; 77: (1): 45-54. |
[12] | Parekh A, Lee CM. Heart rate variability after isocaloric exercise bouts of different intensities. Med Sci Sports Exerc. (2005) ; 37: (4): 599-605. |
[13] | Hemingway H, Shipley M, Brunner E, Britton A, Malik M, Marmot M. Does autonomic function link social position to coronary risk? The whitehall II study. Circulation. (2005) ; 111: (23): 3071-7. |
[14] | Amano M, Kanda T, Ue H, Moritani T. Exercise training and autonomic nervous system activity in obese individuals. Med Sci Sports Exerc. (2001) ; 33: (8): 1287-91. |
[15] | Oliveira RS, Leicht AS, Bishop D, Barbero-Alvarez JC, Nakamura FY. Seasonal changes in physical performance and heart rate variability in high level futsal players. Int J Sports Med. (2013) ; 34: (5): 424-30. |
[16] | Goldberger JJ, Le FK, Lahiri M, Kannankeril PJ, Ng J, Kadish AH. Assessment of parasympathetic reactivation after exercise. Am J Physiol Heart Circ Physiol. (2006) ; 290: (6): H2446-52. |
[17] | Lewis GD, Gona P, Larson MG, Plehn JF, Benjamin EJ, O’Donnell CJ, et al. Exercise blood pressure and the risk of incident cardiovascular disease (from the Framingham Heart Study). Am J Cardiol. (2008) ; 101: (11): 1614-20. |
[18] | Liang N, Nakamoto T, Mochizuki S, Matsukawa K. Differential contribution of central command to the cardiovascular responses during static exercise of ankle dorsal and plantar flexion in humans. J Appl Physiol (1985). (2011) ; 110: (3): 670-80. |
[19] | Ichinose M, Delliaux S, Watanabe K, Fujii N, Nishiyasu T. Evaluation of muscle metaboreflex function through graded reduction in forearm blood flow during rhythmic handgrip exercise in humans. Am J Physiol Heart Circ Physiol. (2011) ; 301: (2): H609-16. |
[20] | Matsukawa K. Central command: control of cardiac sympathetic and vagal efferent nerve activity and the arterial baroreflex during spontaneous motor behaviour in animals. Exp Physiol. (2012) ; 97: (1): 20-8. |
[21] | Iellamo F. Neural mechanisms of cardiovascular regulation during exercise. Auton Neurosci. (2001) ; 90: (1-2): 66-75. |
[22] | Leicht AS, Sinclair WH, Spinks WL. Effect of exercise mode on heart rate variability during steady state exercise. Eur J Appl Physiol. (2008) ; 102: (2): 195-204. |
[23] | De Vito G, Galloway SDR, Nimmo MA, Maas P, McMurray JJV. Effects of central sympathetic inhibition on heart rate variability during steady-state exercise in healthy humans. Clin Physiol Funct I. (2002) ; 22: (1): 32-8. |
[24] | Kang JH, Kim JK, Hong SH, Lee CH, Choi BY. Heart rate variability for quantification of autonomic dysfunction in fibromyalgia. Ann Rehabil Med. (2016) ; 40: (2): 301-9. |
[25] | Simoes RP, Castello-Simoes V, Mendes RG, Archiza B, Dos Santos DA, Bonjorno JC, Jr., et al. Identification of anaerobic threshold by analysis of heart rate variability during discontinuous dynamic and resistance exercise protocols in healthy older men. Clin Physiol Funct Imaging. (2014) ; 34: (2): 98-108. |
[26] | Baek HJ, Cho CH, Cho J, Woo JM. Reliability of ultra-short-term analysis as a surrogate of standard 5-min analysis of heart rate variability. Telemed J E Health. (2015) ; 21: (5): 404-14. |
[27] | Munoz ML, van Roon A, Riese H, Thio C, Oostenbroek E, Westrik I, et al. Validity of (ultra-)short recordings for heart rate variability measurements. Plos One. (2015) ; 10: (9): e0138921. |
[28] | Fisher JP, Seifert T, Hartwich D, Young CN, Secher NH, Fadel PJ. Autonomic control of heart rate by metabolically sensitive skeletal muscle afferents in humans. J Physiol. (2010) ; 588: (Pt 7): 1117-27. |
[29] | Spranger MD, Krishnan AC, Levy PD, O’Leary DS, Smith SA. Blood flow restriction training and the exercise pressor reflex: a call for concern. Am J Physiol Heart Circ Physiol. (2015) ; 309: (9): H1440-52. |
[30] | Buchheit M, Laursen PB, Ahmaidi S. Parasympathetic reactivation after repeated sprint exercise. Am J Physiol Heart Circ Physiol. (2007) ; 293: (1): H133-41. |
[31] | Julius S. Abnormalities of autonomic nervous control in human hypertension. Cardiovasc Drugs Ther. (1994) ; 8: (Suppl 1): 11-20. |
[32] | Halliwill JR. Mechanisms and clinical implications of post-exercise hypotension in humans. Exerc Sport Sci Rev. (2001) ; 29: (2): 65-70. |
[33] | Romero SA, Minson CT, Halliwill JR. The cardiovascular system after exercise. J Appl Physiol (1985). (2017) ; 122: (4): 925-32. |
[34] | Halliwill JR, Taylor JA, Eckberg DL. Impaired sympathetic vascular regulation in humans after acute dynamic exercise. J Physiol. (1996) ; 495: (Pt 1): 279-88. |
[35] | Ainslie PN, Duffin J. Integration of cerebrovascular CO2 reactivity and chemoreflex control of breathing: mechanisms of regulation, measurement, and interpretation. Am J Physiol Regul Integr Comp Physiol. (2009) ; 296: (5): R1473-95. |
[36] | Ott MM, Nuding SC, Segers LS, Lindsey BG, Morris KF. Ventrolateral medullary functional connectivity and the respiratory and central chemoreceptor-evoked modulation of retrotrapezoid-parafacial neurons. J Neurophysiol. (2011) ; 105: (6): 2960-75. |
[37] | Schmidt E, Despas F, Le Traon A, Czosnyka M, Pickard J, Rahmouni K, et al. Intracranial pressure is a physiological stress that determines sympathetic nervous system activity. Fluids and Barriers of the CNS. (2015) ; 12: (Suppl 1). |
[38] | Spyer KM, Gourine AV. Chemosensory pathways in the brainstem controlling cardiorespiratory activity. Philos Trans R Soc Lond B Biol Sci. (2009) ; 364: (1529): 2603-10. |
[39] | Heffernan KS, Kelly EE, Collier SR, Fernhall B. Cardiac autonomic modulation during recovery from acute endurance versus resistance exercise. Eur J Cardiovasc Prev Rehabil. (2006) ; 13: (1): 80-6. |