Cocoa supplementation reduces amyloid-beta1–42 (Aβ1–42) induced deficits in a transgenic C. elegans
Abstract
BACKGROUND:
Cocoa, a significant contributor of polyphenols to the western diet has been shown to be effective against Aβ induced toxicity in vitro. However, the effects of long-term cocoa supplementation on Aβ induced behavioural deficits, particularly on the short-term memory loss observed in human AD are not well defined.
OBJECTIVE:
This study characterized the phenotype of a pan-neuronal Aβ expressing C. elegans strain and investigated the effects of long-term cocoa supplementation on Aβ induced behavioural deficits including short-term memory loss and lifespan.
METHODS:
Cocoa powder was supplemented to the E. coli OP50 diet of C. elegans starting from L1 stage until they die. Neuronally controlled processes including locomotion, learning and memory were studied at different stages of the lifespan. In addition, lifespan was evaluated with different cocoa doses. Aβ fibril levels were determined with Thioflavin T.
RESULTS:
Aβ expressing worms showed a reduced growth, a reduced maximum speed at old age, short-term memory deficits at middle age and a reduced lifespan. Cocoa-supplementation reversed the deficits in growth, maximum speed, short-term memory loss and lifespan to reach similar levels to control counterparts while reducing the Aβ fibril levels.
CONCLUSIONS:
Long-term cocoa supplementation seemed to improve Aβ induced deficits in C. elegans.
1Introduction
Alzheimer’s disease (AD) is a progressive neurodegenerative disorder which is characterized by a gradual loss of memory and cognitive skills [1]. Pathological hallmarks of AD include extracellular deposition of amyloid-β (Aβ) plaques and intracellular aggregation of neurofibrillary tangles in the brain. Being the most common cause of dementiaworldwide, AD accounts for 50–80%of all dementia cases [2]. Prevalence of AD increases with age and it is becoming increasingly common as global older population is escalating. To date, there is no pharmaceutical intervention that has been shown to cure this multifactorial disease or halt the disease progression.
It has been shown that healthy dietary pattern is one of the key protective factors for AD [3]. Higher adherence to a diet rich in natural plant-based foods has shown to reduce the incidence of neurodegenerative diseases [4, 5]. One possible explanation for this reduction is the action of polyphenols in such foods through different mechanisms [6]. Cocoa and its products are known to be rich sources of polyphenols which significantly contribute to the total antioxidant capacity of the diet [7, 8]. Several studies report beneficial effects of cocoa peptides, cocoa extracts and cocoa polyphenols in Aβ induced toxicity in AD [9–12]. However, how cocoa consumption affects Aβ induced behavioural deficits, in particular learning and memory and lifespan are not well-defined.
C. elegans is an inexpensive in vivo model which has been widely used to study Aβ toxicity [13–17]. Even though C. elegans lack Aβ and β-secretase and do not exhibit human pathological and behavioural symptoms of AD, several transgenic strains that express human Aβ have been developed for pharmacological evaluation and mechanistic studies [18]. In this study, we selected a C. elegans strain expressing constitutive pan-neuronal Aβ1–42 which displays an age-related onset of behavioural dysfunction, such as that observed in human AD [16]. Therefore, the aim of this study was to characterize the phenotype of this neuronal Aβ1–42 expressing strain and to determine the effects of long-term cocoa supplementation on Aβ induced behavioural deficits and the lifespan.
2Materials and methods
2.1C. elegans strains and maintenance
Transgenic [GRU101 gnals1 (myo-2p::yfp), GRU102 gnals2 (myo-2p::YFP+unc-119p::Abeta1–42)] C. elegans and Escherichia coli (E. coli) OP50 were obtained from Caenorhabditis Genetics Center (Minneapolis, MN, USA). Worms were maintained on nematode growth medium (NGM) plates carrying a lawn of E. coli OP50. Worm maintenance as well as all the experiments were carried out at 20°C.
2.2Obtaining synchronous cultures
Age-synchronized worms were obtained by bleaching gravid adults. Briefly, gravid hermaphrodites were washed off from an NGM plate with M9 buffer and made the final volume to 3.5 ml. Bleaching solution (bleach: 5N NaOH = 2 : 1) was added to the worm suspension at a volume of 1.5 ml. The suspension was mixed by vortexing the tube five seconds and keeping further 30 seconds on rest until all the worm bodies got dissolved. The suspension was centrifuged (2 min at 1300 g) to pellet the released eggs and the supernatant was poured off. M9 buffer was added to a final volume of 5 ml, centrifuged again and the supernatant was removed. This step was repeated at least three times to completely get rid of the bleaching solution. The egg pellet was resuspended in 3 ml of M9 and kept for 48 hours on a shaker for hatching.
2.3Cocoa treatment
A commercially available cocoa powder, which was an unsweetened dry powder processed with alkali was used for the study. A cocoa suspension was made to the desired final concentration in M9 buffer to make it spreadable. For all the experiments except lifespan, a single dose of cocoa which is 5 mg of cocoa powder suspended in 1 ml of M9 was used. For the lifespan experiment, five different doses of cocoa were prepared by suspending 1 mg, 2 mg, 3 mg, 4 mg and 5 mg cocoa in 1 ml of M9 buffer. Concentrated E. coli OP50 was prepared by suspending 1 g of E. coli pellet in 16 ml of M9 buffer. Concentrated E. coli was first added to NGM plates and was grown overnight at 20°C. Cocoa suspension was added on the top of E. coli lawn at a ratio of 1 : 2 volume, E. coli: cocoa suspension (for small plates: 100 μl of E. coli and 200 μl of cocoa, for medium plates: 200 μl of E.coli and 400 μl of cocoa). Worms received cocoa starting from L1 stage.
2.4Growth and locomotion
Age synchronized worms were washed off from both treatment and control plates and collected in a 15 ml falcon tube. Once worms settled to the bottom, supernatant was removed and 5 ml of M9 was added to wash off the food and repeated twice. Thereafter, 100 μl of worm suspension was placed on plain agar plates in the absence of food. Plates were left in the laminar flow cabinet for 5 min to dry out the liquid. Then, they were placed under a stereo microscope (SMZ745T; Nikon, Japan equipped with G-AL 1.0 x objective) and videos were captured using NIS Elements imaging software (version 5.01, Japan) and a digital camera (DS-Fi2; Nikon, Japan) with settings at 50 frames per second, 30 second duration per video and resolution of 640×480 pixels. The scale bar (μm) in the imaging software was used to convert pixels into μm. Worms were tracked and analysed via WormLab 3.1 software (MBF Bioscience, Williston, VT USA). Worms tracked over a minimum of 10 seconds were included in the final analysis. Mean body length (μm), mean area (μm2), mean speed (μm/s), maximum speed (μm/s), and maximum amplitude (μm) were reported. According to the WormLab software, speed is defined as the distance per second travelled by the worm along its central axis. Maximum amplitude is the maximum centroid displacement over an entire track.
2.5Determination of motility classes at old age
Motility classes of worms were determined as previously described (19). Age synchronized worms were sorted by class (A, B or C) at day 12 and re-scored for class every day until death. Animals move constantly away from the touch stimulus and leave sinusoidal tracks in the E. coli lawn were categorized into the class “A”. Worms do not move unless prodded and leave non-sinusoidal tracks were categorized as class “B”. Worms do not move even prodded but, exhibit head and/or tail movements or twitch in response to touch were categorized as class “C”.
2.6Learning and short-term associative memory
Naive chemotaxis, learning and short-term associative memory were determined based on C. elegans positive butanone learning assay (Fig. 1A) [20]. Age-synchronized worms (approximately 1000) were grown on high growth media plates with food until they reached the desired age. Chemotaxis assay (Fig. 1B) was performed with approximately 200 worms. Remaining were starved for 1 hr and at the end of starvation period, worms were associated with food and chemo-attractant butanone for 1 hr. Chemotaxis assay was repeated to test 1x (massed) associative learning immediately after conditioning (t = 0 time point). Rest of the worms were transferred equally to two NGM plates seeded with E. coli and incubated at 20°C. After 1 hr, and 2 hrs from food-butanone association, chemotaxis assay was performed with the worms on two plates (t = 1 hr and t = 2 hr time points). Chemotaxis index (CI) was calculated as, CI = [(Number of worms at butanone) –(Number of worms at ethanol)]/ (Total number of worms). The learning index (LI) at different time points was calculated as, LI = Chemotaxis indext –Chemotaxis indexNaive. Dementia index (short-term associative memory loss) was calculated after 1 hr and 2 hrs from the food-butanone association (Dementia indext = Learning index0 - Learning indext).
Fig. 1
Learning and short-term associative assay workflow, adapted from (20). (A) Short-term associative memory assay: Starved worms were conditioned with butanone for 1 h and some of them were immediately tested for 1X learning (t = 0) and the rest were transferred onto holding plates for 1 h and 2 hrs (B) Chemotaxis assay: Briefly, 1 μl of 2M NaN3 was added to the butanone (left) and EtOH (right) spots. Then, 1 μl of 10%Butanone in 95%EtOH and 95%EtOH were added to the butanone and EtOH spots. Worms (200) in M9 buffer were added to the origin. KimWipe absorbed M9 and released worms. Worms moved to either butanone or EtOH spots and were paralysed.
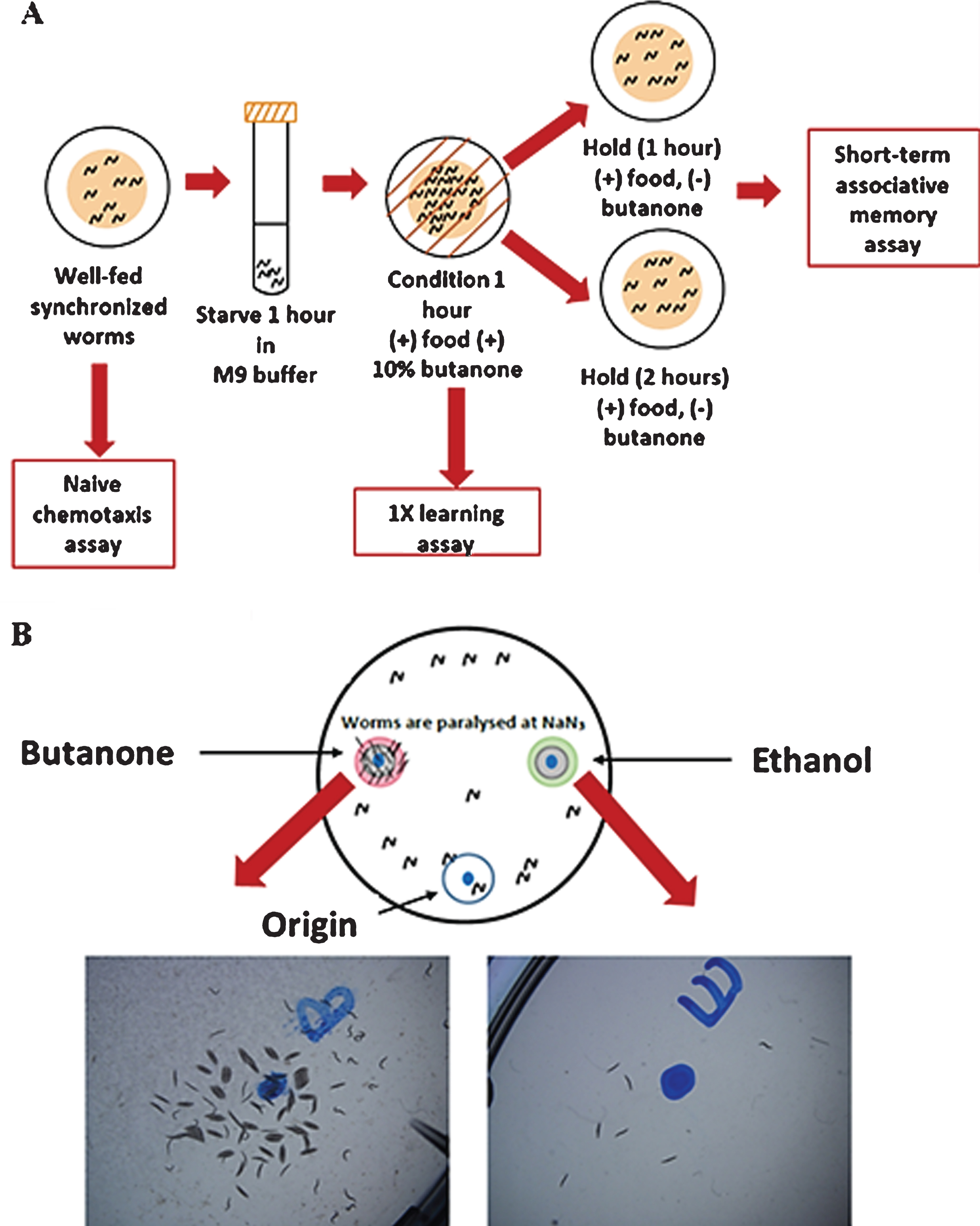
2.7Lifespan assay
Lifespan assay was performed as described in reference [21]. Floxuridine (FUdR) was not used to avoid progeny production and instead worms were transferred everyday onto fresh plates until they stop laying eggs (until day 9). Thereafter, worms were transferred every other day. Worms that were crawled off from the plates were excluded from the analysis. Survival curves were plotted. Mean, median and maximum lifespan were reported.
2.8Quantitative staining of Aβ fibrils with Thioflavin-T
Quantitative staining of Aβ fibrils with Thioflavin-T (ThT) was performed according to a previously described method [22] with slight modifications. At day 4 and day 8 worms were collected by washing the plate with 1 ml of M9 buffer and transferred into an Eppendorf tube. The worms were pelleted by centrifugation at 14 k rpm for 2 min and the supernatant was poured off. Worms were washed two more times with M9 to get rid of food. Worm pellet was resuspended in 500 μl of M9 in a microcentrifuge tube, snap frozen in liquid nitrogen and stored at –80°C until use. Worms were thawed and homogenized by using Precellys®24 Homogenizer (Bertin technologies) and Precellys® lysing kit: 03961-1-004 (0.5 mm glass beads) at 6400 rpm, 2x10 secs twice for a total time of 40 secs in 500 μl of M9. Homogenized worms were centrifuged at 14k rpm for 2 min and the supernatant was collected into a new tube. The concentration of total soluble protein in each sample was quantified by bicinchoninic acid assay (Pierce™ BCA Protein Assay Kit). Equal amount of protein was used from each sample and triplicates were performed for each. Each replicate was mixed with 10 μl of M9 buffer and 2 μl of 1 mM ThT (Sigma) in a final volume of 100 μl. Fluorescence intensity was measured by a fluorescence plate reader (CLARIOstar multi-mode plate reader, BMG LABTECH) using excitation at 440 nm and emission at 482 nm and averaged from three independent experiments.
2.9Statistical analysis
All statistical analyses were performed in IBM SPSS® statistics software (version 24). Data were expressed as mean±standard error of mean (SEM). Differences between groups for body length, mean area, locomotion, chemotaxis and worm classes (at day 12) were analysed using general linear model, multivariate test. Differences between Aβ levels were calculated using general linear model, univariate test. Differences between the number of days to reach motility group B/C from A was determined using t-Test. Survival function was estimated using Kaplan-Meier curves. Survival curves were compared using log rank (Mantel-Cox) test. Maximum lifespan was the average lifespan of top 10 longest-lived worms. Differences between groups for maximum lifespan were determined using one-way ANOVA with post-tests (Tukey’s test). P < 0.05 was considered significant.
3Results
3.1Cocoa supplementation reversed the reduced growth in pan-neuronal Aβ1–42 expressing worms
We measured body length (μm) and area (μm2) of worms starting from day 2 (after 24 hours from introducing L1 worms to food) till day 8 as indicators of worm growth and development. Results showed that Aβ1–42 expressing worms (GRU102) were significantly shorter than control (GRU101) worms across all the time points starting from day 3 (P < 0.05, Fig. 2A). Cocoa supplementation significantly increased the body length of GRU102 worms to reach similar levels to GRU101 worms starting from day 4 (Fig. 2B). GRU102 worms were significantly thinner than GRU101 worms across all the time points (P < 0.05, Fig. 2C). Cocoa supplementation increased the thickness of GRU102 worms to reach similar levels to GRU101 worms at day 8 (Fig. 2D).
Fig. 2
Body length (μm) and area (μm2) of control and cocoa supplemented GRU102 (Aβ1–42) and GRU101 (control) worms. Experiments were performed in triplicate. Values are expressed in mean±SEM (n≥44). *P<0.05 for the indicated comparison (calculated using multivariate test, general linear model). (A) GRU102 worms were shorter than GRU101 worms across all the time points, except day 2 (P < 0.05). (B) Cocoa supplementation significantly increased the body length of GRU 102 worms to reach similar levels to GRU101 worms starting from day 4. (C) GRU102 worms were thinner than GRU101 worms across all the time points (P < 0.05). (D) Cocoa supplementation increased the thickness of GRU102 worms to reach similar levels to GRU101 worms at day 8.
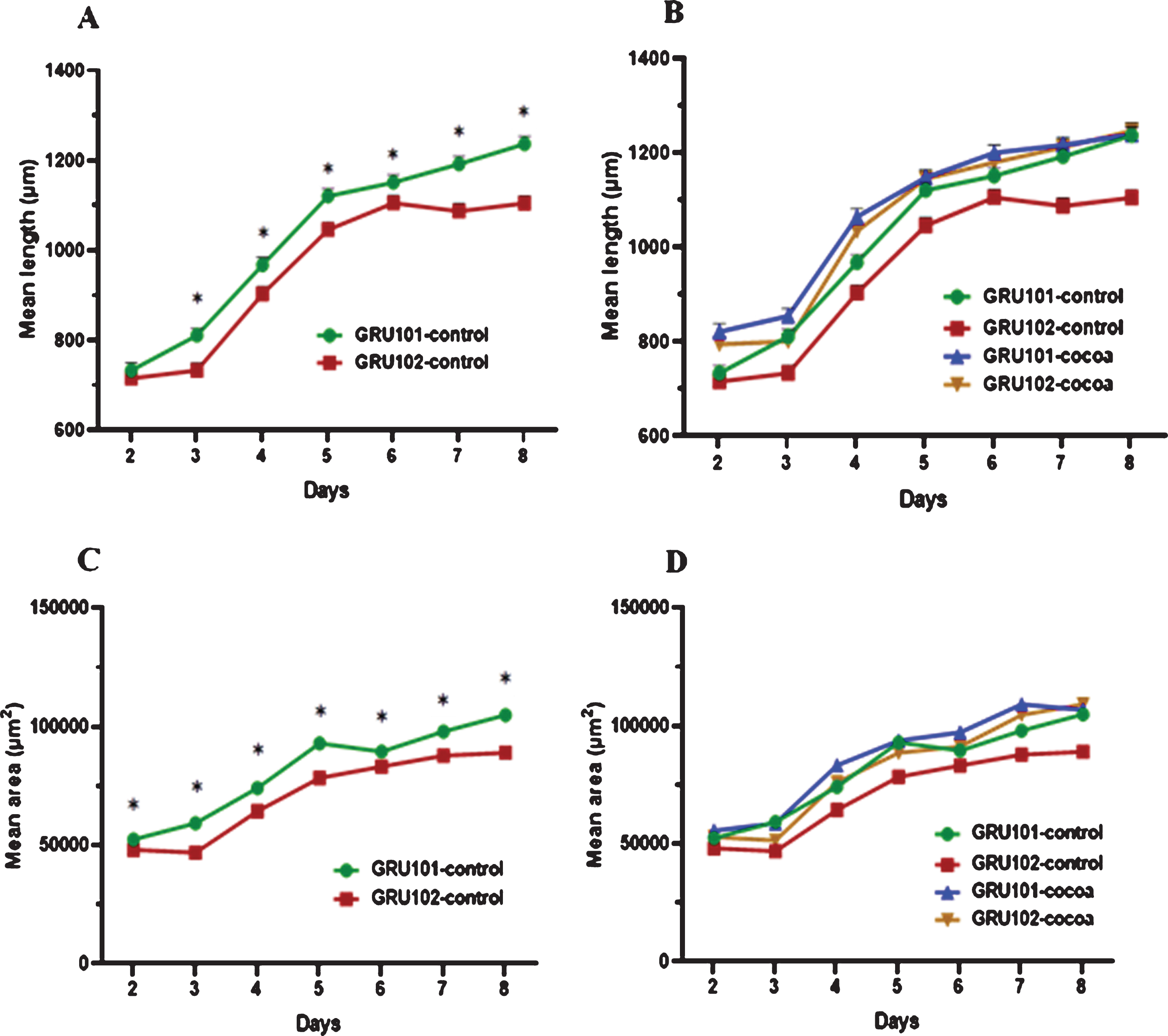
3.2Pan-neuronal Aβ1–42 expressing worms showed a reduced maximum speed at old age which was reversed by cocoa supplementation
Mean speed (μm/s), maximum speed (μm/s) and maximum amplitude (μm) were measured as indicators of worm locomotion across the lifespan starting from day 2 to day 12. There was no significant difference for mean speed between the strains across all the time points, except day 8 (Fig. 3A) However, cocoa supplementation significantly increased the mean speed of both strains across most of the time points (Fig. 3B). Maximum speed of Aβ1–42 expressing worms (GRU102) showed a reduction at later stage of life starting from day 10 upto day 12 compared to control (GRU101) worms (P < 0.05, Fig. 3C). Cocoa supplementation reversed this reduction in GRU 102 strain to reach similar levels to GRU101 (Fig. 3D). Maximum amplitude of GRU102 worms was significantly lower across most of the time points compared to GRU101 worms (P < 0.05, Fig. 3E). However, cocoa supplementation was not able to reverse this reduction in GRU102 strain at most of the time points (Fig. 3F).
Fig. 3
Locomotion parameters of control and cocoa supplemented GRU102 (Aβ1–42) and GRU101 (control) worms. Mean speed (μm/s), maximum speed (μm/s) and maximum amplitude (μm) were measured from day 2 to day 12. Experiments were performed in triplicate. Values are expressed in mean±SEM (n≥42 per group). *P < 0.05 for the indicated comparison (calculated using multivariate test, general linear model). (A) There was no significant difference between 2 worm strains for mean speed across all the time points, except day 8. (B) Overall, cocoa-supplementation increased the mean speed of both strains from day 4. (C) GRU102 worms showed a reduction in maximum speed at old age (day 10–12) which was significantly low at day 11 and 12 compared to GRU101 worms. (D) Overall, cocoa improved the maximum speed in both strains across most of the time points and reversed the reduction (P > 0.05) of maximum speed in GRU102 strain at old age to reach similar levels to GRU101. (E) The maximum amplitude of GRU102 worms was less across most of the time points (especially at the middle age) compared to GRU101 worms. (F) Even though cocoa improved the maximum amplitude of GRU102 worms at some of the time points, there was no significant effect at most of the time points.
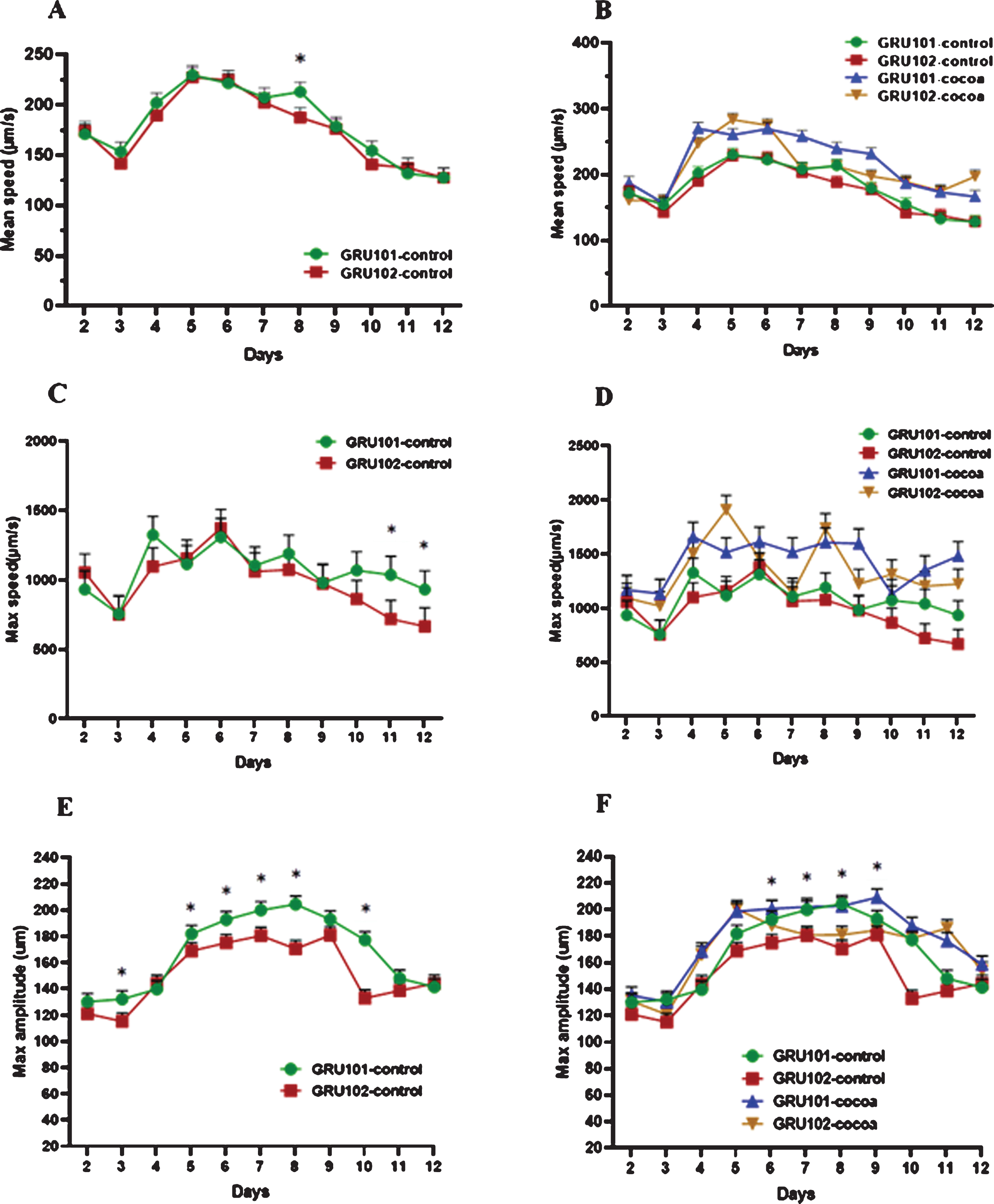
3.3Cocoa supplementation maintained a similar motility reduction rate in pan-neuronal Aβ1–42 expressing worms at old age as their control counterparts
Worms were further studied for their motility at old age by grouping them at day 12 into either class A, B or C and re-scoring them for their class every day until they die. Class “A” represented the highly motile group. Class “B” was less motile compared to class “A” and class “C” was the least motile group. Worms showed no significant difference for their class at day 12 (Fig. 4A). However, cocoa-fed GRU101 (control) worms took a significantly reduced number of days to reach class B/C from A (P < 0.05, Fig. 4B).
Fig. 4
Locomotion of control and cocoa supplemented GRU102 (Aβ1–42) and GRU101 (control) worms at old age. Worms were categorized into either class A, B or C at day 12 depending on their motility. They were re-scored for class every day until they die. For each group, average number of days to reach class B/C from A was calculated. Experiments were performed in triplicate. Values are expressed in mean±SEM (n≥24). (A) There was no significant difference between the groups for class “A” and “B+C” at day 12 (*P < 0.05 for the indicated comparison calculated using general linear model, univariate analysis). (B) The number of days taken by cocoa-supplemented GRU101 worms to reach class “B/C” from “A” was significantly lower (*P < 0.05 for the indicated comparison calculated using t-Test, two-sample assuming equal variances).
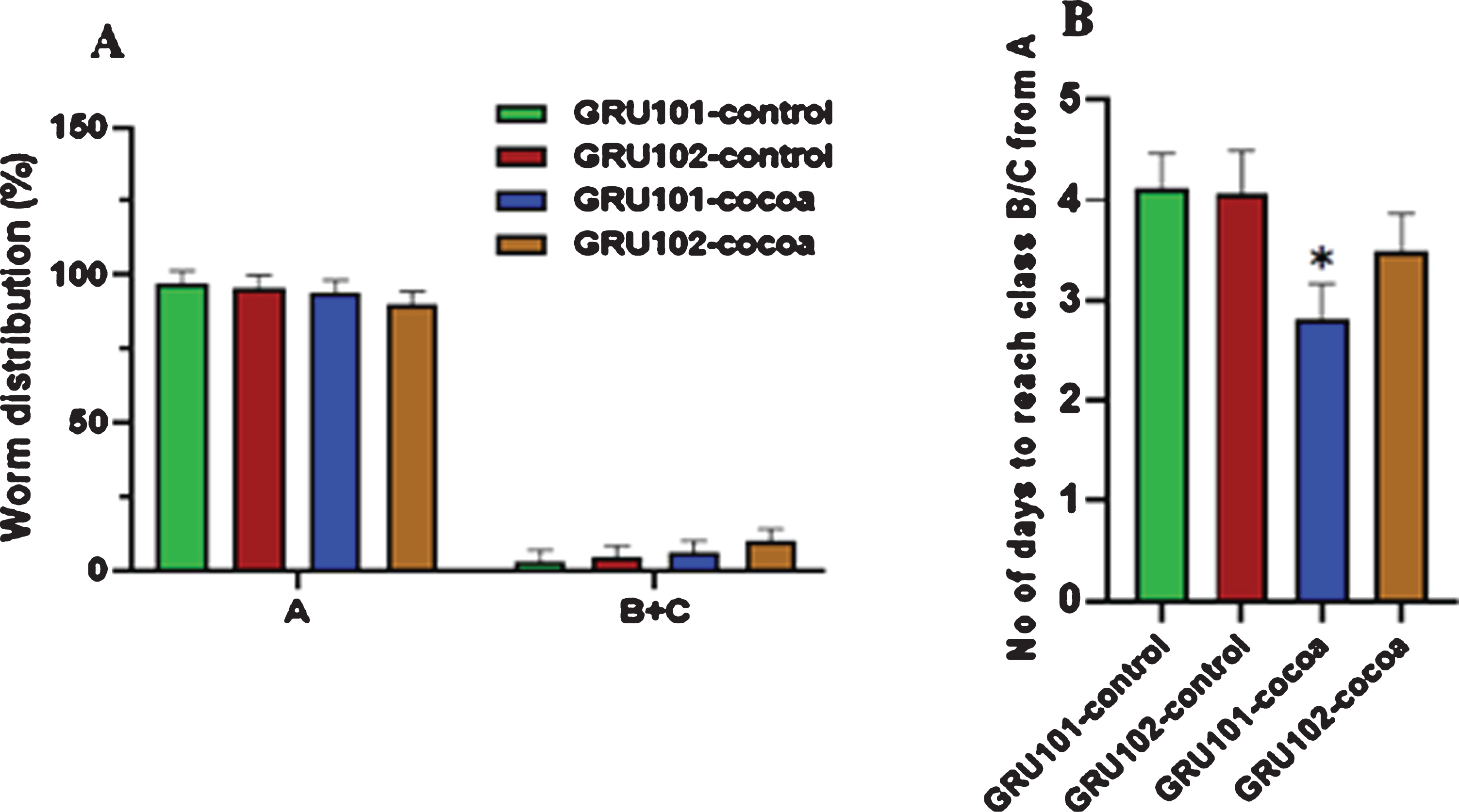
3.4Cocoa supplementation improved the short-term memory loss in pan-neuronal AB1–42 expressing worms at old age
As worms showed a rapid reduction in chemotaxis and it was not detectable at day 12 (old age), we performed learning and short-term associative memory assay only at day 4 and 8 to represent young and middle age of worms respectively. There was no significant difference between GRU101 (control) and GRU102 (Aβ1–42) worms for the naive chemotaxis at either day 4 or day 8 (Fig. 5A). However, cocoa supplementation improved the naive chemotaxis index in both strains at day 8 compared to their own controls (P < 0.05, Fig. 5B). Similar to naive chemotaxis index, there was no significant difference between the strains for learning index at both time points (Fig. 5C). Cocoa supplementation improved learning index in GRU102 worms at day 4 compared to its own control (P < 0.05, Fig. 5D). Interestingly, GRU102 worms showed a significant short-term memory loss (dementia index) compared to GRU101 worms at both 1 hr and 2 hr time points after conditioning at day 8 (P < 0.05, Fig. 5E). GRU102 worms showed a 113.3%increase in short-term memory loss at 1 hr time point and 46.8%increase at 2 hr time point compared to GRU101 worms. Cocoa supplementation reversed this short-term memory loss in GRU102 at both 1 hr and 2 hr time points to reach similar levels to GRU101 (Fig. 5F). The reduction in short-term memory loss in cocoa-supplemented GRU101 worms at 2 hr time point at day 8 (46.8%) was significant compared to its control counterparts (P < 0.05). At day 8, cocoa-supplemented GRU102 worms showed a 73.4%and 63.0%reduction in short-term memory loss at 1 hr and 2 hr time points respectively compared to GRU102 control worms.
Fig. 5
Naive chemotaxis index, learning index and dementia index (short-term memory loss) of control and cocoa-supplemented GRU101 (control) and GRU102 (Aβ1–42) worms at day 4 (1st day of adulthood) and day 8 (5th day of adulthood) using C. elegans positive butanone learning and short-term memory assay. Chemotaxis assay was performed for well-fed, synchronized worms and naive chemotaxis index was calculated, chemotaxis index (CI) = [(number of worms at butanone) –(number of worms at ethanol)]/ [total number of worms on the plate]. Well-fed, synchronized worms were starved for 1 hour, then fed in the presence of 10%butanone for 1 hour. Worms were tested immediately after 1 hr from food-butanone association for their learning, learning Index (LI) = chemotaxis indext –chemotaxis indexNaive. Dementia index (short-term associative memory loss) was measured after 1 hr and 2 hrs from the food-butanone association without exposing to butanone again (Dementia indext = Learning index0-Learning indext). Four independent experiments were performed (n≥50 worms per group). Values are expressed in mean±SEM. *P < 0.05 for the indicated comparison (calculated using multivariate test, general linear model). (A) There was no significant difference between 2 strains at both day 4 and 8 for the naive chemotaxis index. (B) Cocoa significantly increased the naive chemotaxis in both strains at day 8 (P < 0.05). (C) There was no significant difference between 2 strain at both day 4 and 8 for the learning index. (D) Cocoa significantly increased the learning in GRU102 worms at day 4 (P < 0.05). (E) GRU102 worms showed a higher dementia index (short-term memory loss) at both 1 hr and 2 hr time points from conditioning at day 8 (P < 0.05). (F) Cocoa significantly reduced the dementia index in GRU102 worms at both time points (t = 1 and t = 2) at day 8 to reach similar levels to GRU101 worms.
![Naive chemotaxis index, learning index and dementia index (short-term memory loss) of control and cocoa-supplemented GRU101 (control) and GRU102 (Aβ1–42) worms at day 4 (1st day of adulthood) and day 8 (5th day of adulthood) using C. elegans positive butanone learning and short-term memory assay. Chemotaxis assay was performed for well-fed, synchronized worms and naive chemotaxis index was calculated, chemotaxis index (CI) = [(number of worms at butanone) –(number of worms at ethanol)]/ [total number of worms on the plate]. Well-fed, synchronized worms were starved for 1 hour, then fed in the presence of 10%butanone for 1 hour. Worms were tested immediately after 1 hr from food-butanone association for their learning, learning Index (LI) = chemotaxis indext –chemotaxis indexNaive. Dementia index (short-term associative memory loss) was measured after 1 hr and 2 hrs from the food-butanone association without exposing to butanone again (Dementia indext = Learning index0-Learning indext). Four independent experiments were performed (n≥50 worms per group). Values are expressed in mean±SEM. *P < 0.05 for the indicated comparison (calculated using multivariate test, general linear model). (A) There was no significant difference between 2 strains at both day 4 and 8 for the naive chemotaxis index. (B) Cocoa significantly increased the naive chemotaxis in both strains at day 8 (P < 0.05). (C) There was no significant difference between 2 strain at both day 4 and 8 for the learning index. (D) Cocoa significantly increased the learning in GRU102 worms at day 4 (P < 0.05). (E) GRU102 worms showed a higher dementia index (short-term memory loss) at both 1 hr and 2 hr time points from conditioning at day 8 (P < 0.05). (F) Cocoa significantly reduced the dementia index in GRU102 worms at both time points (t = 1 and t = 2) at day 8 to reach similar levels to GRU101 worms.](https://ip.ios.semcs.net:443/media/nha/2021/6-2/nha-6-2-nha200114/nha-6-nha200114-g005.jpg)
3.5Cocoa supplementation reduced Aβ fibril levels in pan-neuronal Aβ1–42 expressing worms at both young and middle age
A significantly high fluorescence was detected in GRU102 (Aβ1–42) worms compared to GRU101 (control) worms with ThT fluorescent dye at both day 4 and day 8 (P < 0.05, Fig. 6A). With cocoa supplementation, GRU102 worms showed a significantly reduced Aβ fibril levels at both day 4 and day 8 compared to untreated GRU102 worms (P < 0.05, Fig. 6B).
Fig. 6
Aβ fibril levels in control and cocoa supplemented GRU101 (control) and GRU102 (Aβ1–42) worms at day 4 and day 8. Values are expressed in mean±SEM of three independent experiments with at least n = 100 worms per experiment. *P < 0.05 for the indicated comparison (calculated using univariate test, general linear model). (A) Th T fluorescence for the control and cocoa-supplemented GRU101 and GRU102 worms; untreated GRU102 (AB1–42) worms showed a higher Th T fluorescence compared to GRU101 (control) worms at both day 4 and day 8 (P < 0.05). Cocoa supplementation significantly reduced the Th T fluorescence in GRU102 (AB1–42) worms at both day 4 and day 8. (B) Graph corrected for the background fluorescence of GRU101 worms; cocoa supplementation significantly reduced the Aβ fibril levels in GRU102 worms at both day 4 and day 8 (P < 0.05).
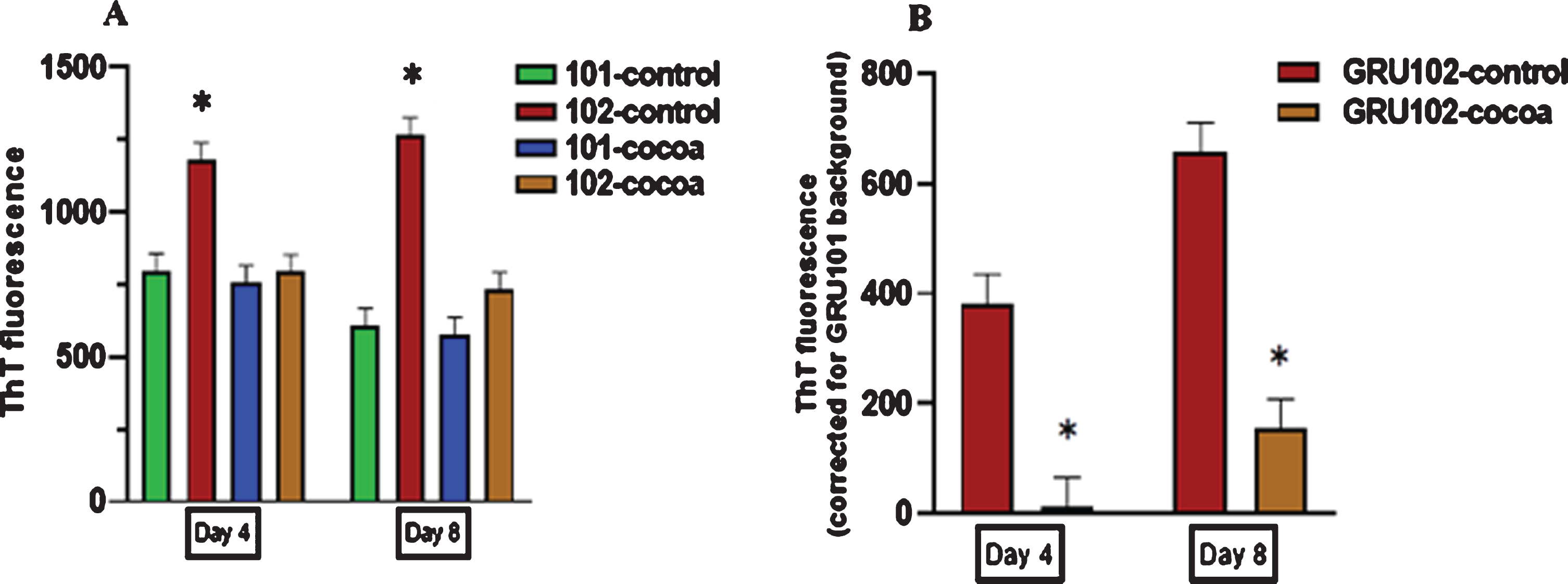
3.6Cocoa supplementation at a dose of 3 mg reversed the mean lifespan reduction in pan-neuronal Aβ1–42 expressing worms
Worm diet was supplemented with cocoa at 1 mg, 2 mg, 3 mg, 4 mg and 5 mg doses. We found GRU101 (control) worms to have a mean lifespan of 15.8 days and GRU102 (Aβ1–42) to have a mean lifespan of 14.9 days at our laboratory conditions at 20 °C (Fig. 7, Table 1). The mean lifespan of GRU102 worms was significantly lower compared to GRU101 worms (P < 0.05). The median lifespan of GRU101 and GRU102 worms were 15 and 14 days respectively and was not significantly different. Cocoa supplementation at a dose of 3 mg significantly extended the mean lifespan of GRU102 worms (8.5%) to reach similar levels to GRU101 worms. Moreover, these GRU102 worms showed a significantly increased median lifespan (P < 0.05, 14.3%) compared to their own control. In addition, 2 mg (7.1%) and 4 mg (7.1%) cocoa doses extended the median lifespan of GRU102 worms compared to their own control. Supplementing worm diet with cocoa at a dose of 5 mg significantly decreased the mean lifespan of both stains (P < 0.05, 14.4%for GRU101 and 7.0%for GRU102) while significantly reducing the median lifespan of GRU101 worms (P < 0.05, 13.3%). Besides, cocoa at 1 mg dose also decreased both mean and median lifespan of GRU102 worms compared to GRU101 worms (P < 0.05, 6.5%mean and 7.1%median). However, none of the cocoa doses extended the maximum lifespan of GRU102 worms.
Fig. 7
Survival curves for control and cocoa-supplemented GRU101 and GRU102 (Aβ1–42) worms. Experiments were performed in triplicate (100 < n>200 for all groups). Differences between groups for mean and median lifespans were calculated using log rank (Mantel-Cox) test. (A) GRU102 worms showed a significantly lower (P < 0.05) mean lifespan compared to GRU101 (control strain). (B) Cocoa at a dose of 1 mg decreased the mean and median lifespan of GRU102 worms compared to GRU101 worms (P < 0.05, 6.5%mean and 7.1%median). (C) Cocoa supplementation at a dose of 2 mg increased the median lifespan of GRU102 worms compared to their own control (P < 0.05, 7.1%). (D) Cocoa at a dose of 3 mg significantly increased the mean (P < 0.05, 8.5%) lifespan of GRU102 worms to reach similar levels to GRU101 worms. Median lifespan of GRU102 worms was significantly extended compared to their own control (P < 0.05, 14.3%). (E) Cocoa at a dose of 4 mg increased the median lifespan of GRU102 worms compared to their own control (P < 0.05, 7.1%). (F) Cocoa at 5 mg/ml dose decreased the mean lifespan of both worm strains (P < 0.05, 14.4%for GRU101 and 7.0%for GRU102).
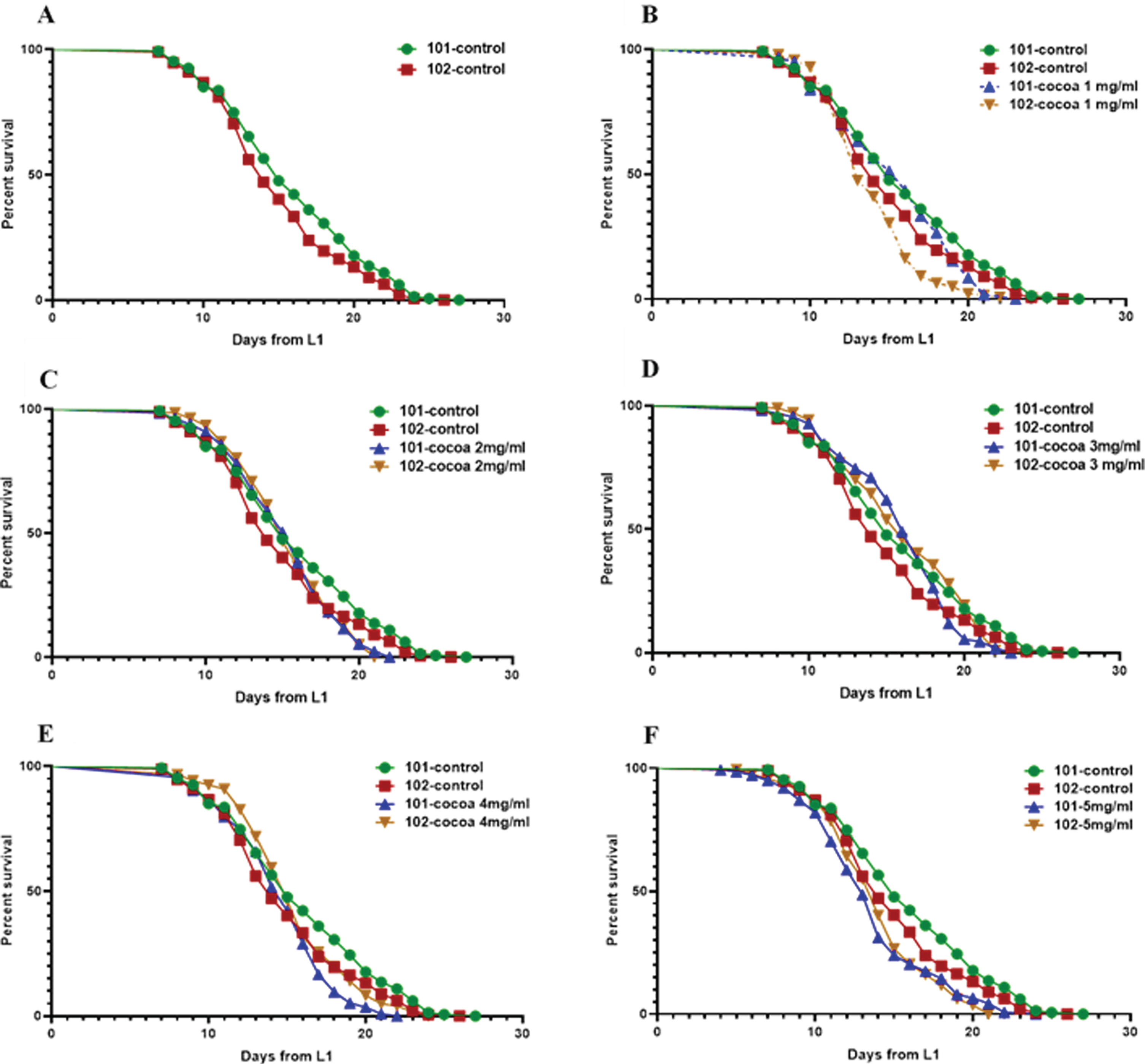
Table 1
Mean, median and maximum lifespan of control and cocoa supplemented GRU101 and GRU102 worms
Treatment | Mean lifespan | % Extension compared to control | Median lifespan | % Extension compared to control | Maximum lifespan |
GRU101-control | 15.8±0.38 | 15 | 20.6 | ||
GRU102-control | 14.9±0.30a | 14 | 20.2 | ||
GRU101-cocoa, 1 mg | 15.3±0.36 | –3.5 | 16 | 6.7 | 18.0a |
GRU102-cocoa, 1 mg | 13.9±0.24a,b | –6.5 | 13a | –7.1 | 17.2a,b |
GRU101-cocoa, 2 mg | 15.2±0.30 | –4.1 | 16 | 6.7 | 19.0 |
GRU102-cocoa, 2 mg | 15.4±0.27 | 3.0 | 15b | 7.1 | 18.9 |
GRU101-cocoa, 3 mg | 15.9±0.34 | 0.5 | 16 | 6.7 | 18.8 |
GRU102-cocoa, 3 mg | 16.2±0.39b | 8.5 | 16b | 14.3 | 18.8 |
GRU101-cocoa, 4 mg | 14.5±0.31a | –8.4 | 15 | 0 | 17.9a |
GRU102-cocoa, 4 mg | 15.4±0.32 | 3.6 | 15b | 7.1 | 19.0 |
GRU101-cocoa, 5 mg | 13.6±0.33a | –14.4 | 13a | –13.3 | 17.9a |
GRU102-cocoa, 5 mg | 13.9±0.27a,b | –7.0 | 14 | 0 | 17.9a |
aStatistically significant (P < 0.05) compared to GRU101-control group. bStatistically significant (P < 0.05) compared to GRU102-control group.
4Discussion
A transgenic C. elegans strain (GRU102) which expresses pan-neuronal Aβ1–42 was used to study the effects of long-term cocoa supplementation on Aβ1–42 induced toxicity, particularly on the short-term memory loss observed in human AD. In our study, we administered whole cocoa powder to C. elegans by spreading it over the E. coli lawn. However, it is possible for E. coli to modify cocoa powder and produce different metabolites. Studies have demonstrated that polyphenols in cocoa are poorly absorbed in the intestine in their natural form and therefore, the degradation of these compounds in the colon by microbes is one of the most effective ways to produce secondary metabolites that increases their bioavailability [23, 24]. Therefore, we assume that even if E. coli modifies cocoa, it will not have any adverse impact on the beneficial effects of cocoa polyphenols.
Cocoa contains a number of polyphenolic compounds, in particular it is rich in flavanols. The major flavanol compounds found in cocoa are the monomeric forms, epicatechin, catechin and their oligomeric and polymeric forms, procyanidins. In addition to polyphenols, cocoa contains methylxanthines, predominantly theobromine about 2–3%of by weight [25]. Almost all the studies attribute the health-promoting effects of cocoa to cocoa polyphenols and the role of methylxanthines on these beneficial effects of cocoa polyphenols remains unanswered [26]. Processing with alkali is known to reduce the beneficial polyphenol content in natural cocoa powder [27–29]. However, the compositional analysis showed that the commercially processed cocoa powder we used in this study was not devoid in flavonoids and other polyphenols. Our cocoa powder contained 27.01 mg GAE/g total phenolics which is about 56%compared to natural cocoa powder. The total flavonoids were 10.13 mg CE/g which is about 40%of the total flavonoids in natural cocoa powder [30].
Polyphenols, in general are known to have low bioaccessibility and bioavailability, and most of them cannot be absorbed in their natural forms. Monomers and dimeric or trimeric proanthocyanidins are quite stable in the gastric environment and therefore, are travelled to the small intestine with almost no changes [31]. Monomers are able to cross the gut barrier and around 20%are rapidly absorbed, undergo rapid and extensive metabolization and circulate in the blood stream [23]. Epicatechin shows a higher bioavailability compared to the other monomers. Dimeric and trimeric procyanidins are known to poorly absorb in the gastrointestinal tract. The acidic environment in the stomach degrades some dimers to epicatechin and undergo interflavan bond conversion [31]. Intake of cocoa with large amount of flavanol monomers and dimers has been resulted in the occurrence of B2 and B5 dimers in human plasma in modest concentrations [32]. Oligomeric procyanidins (the degree of polymerization above three) and polymeric flavanols which are not absorbed in their naive form reach the colon and are being metabolized by the local microbiota to form secondary metabolites, increasing their bioavailability [32, 33]. The role of flavonoids in the modulation of neurodegeneration is often related to their antioxidant capacity, but other mechanisms are also known to be involved. These mechanisms depend on the ability of these compounds to cross the blood-brain barrier (BBB) [34]. Several studies have demonstrated that catechin and epicatechin are able to cross the BBB therefore, indicating them as prospective compounds to exert protective effects in neurodegeneration [34, 35].
In this study, we categorized worms as young (day 4), middle (day 8) and old (day 12) to see the effects at different stages of life. Worm body length and area were measured as indicators of worm growth and development. GRU102 (Aβ1–42) worms showed a significantly reduced body length and area compared to GRU101 (control) worms. However, cocoa supplementation reversed this reduction in both length and area in GRU102 worms to reach similar levels to GRU101 worms. We measured well-characterized locomotion parameters of C. elegans including mean speed and maximum speed to see how neuronal expression of Aβ1–42 influences worm movements. In addition, we used maximum amplitude which is known as a marker of C. elegans muscle strength [36]. Even though the mean speed of worms was not affected by Aβ toxicity, maximum speed was significantly affected at old age. Cocoa supplementation reversed this reduction at old age to reach similar levels to GRU101 worms. GRU102 worms showed a reduced maximum amplitude across most of the time points compared to GRU101 worms. However, cocoa supplementation was not effectively reversed this reduction in maximum amplitude, particularly in mid life. We used another method to identify the changes in locomotion at further old age starting from day 12 by classifying worms into three different classes. Results showed that there was no significant difference between the two strains in terms of the number of days that they took to reach the low motile category from high motile category. When the worms were fed with cocoa, GRU101 worms took significantly lower number of days to reach the low motile group. In our lifespan experiment, we observed a significant reduction in lifespan in both strains with 5 mg/ml cocoa dose and a greater reduction (about two times) in GRU101 strain compared to GRU102. This greater reduction in lifespan in GRU101 strain may be the reason for these worms to take a lesser number of days to reach to the low motile group.
C. elegans chemotaxis behaviour, a neuronally-controlled process was used to study the Aβ induced deficits in neuronal function and the effects of cocoa-supplementation. Naive chemotaxis which is the natural response of C. elegans for butanone, learning index which is an indicator of the extent that worms learn food-butanone association and the short-term memory loss (dementia index) were measured. Control and the Aβ expressing strain showed no difference in both naive chemotaxis index and learning index at both young age and middle age. However, cocoa supplementation significantly increased the learning index in GRU102 worms at young age. A recently published study suggest that Aβ is associated more strongly with a deficit in learning than any aspect of memory dysfunction [37]. Therefore, our results suggest that cocoa might be useful in improving the ability of learning in AD. Loss of memory is among the first symptoms reported by AD patients and therefore, one of the key characteristics of AD [38]. Pan-neuronal expression of Aβ seemed to induce a short-term memory loss in C. elegans at their middle age. This short-term memory loss has not been described anywhere else before according to our knowledge and we report it here as one of the novel findings of our study. Thus, this model closely resembles human AD and therefore, more competent in drug screening studies compared to other available C. elegans models of AD. Interestingly, Aβ induced memory loss was reversed by cocoa supplementation.
Fong, Teo [16] report that they detected Aβ protein in GRU102 worms in aggregated form at day 12 by using anti-Aβ antibodies. We detected Aβ fibrils at both young and middle age of worms using ThT which is a commonly used probe to monitor in vitro Aβ fibril formation. Upon binding to Aβ fibrils, ThT gives a strong fluorescence signal at approximately 482 nm when excited at 440 nm [39]. According to our results, cocoa supplementation effectively reduced the Aβ fibril levels in GRU102 worms at both young and middle age.
Our study was consistent with the previous study which reports that Aβ expressing worms to have a significantly reduced lifespan compared to control worms (16). One cocoa doses we tested reversed the mean lifespan reduction in Aβ expressing worms. However, the lowest and the highest doses of cocoa further reduced the lifespan in Aβ expressing worms. Lifespan reduction was observed in the control strain as well with the highest cocoa dose used. As it has been shown that caloric restriction can extend the lifespan in C. elegans (40), we assume that cocoa powder as a rich source of carbohydrates (more than 50%of the weight) may reduce the lifespan of C. elegans when worms were supplemented a high dose. The lifespan reduction with the lowest dose may be due to the availability of potential beneficial bioactive components in lesser amounts.
Aβ, an amphiphilic and partly folded molecule is prone to self-aggregate and produce intermediate soluble oligomers, protofibrils and finally insoluble fibrils [41]. The neurotoxicity of Aβ is known to depend on their aggregated state, in which oligomers and protofibrils are more toxic than monomers and mature fibrils [42]. A previous study found a cocoa peptide reduced the Aβ induced toxicity in a transgenic C. elegans (CL4176) which expresses Aβ1–42 in body wall muscles by upshifting the temperature from 16 °C to 25 °C [11]. Aβ expression results in a concomitant progressive paralysis phenotype in this strain. They further report that this beneficial effect was achieved through the reduction of toxic Aβ levels (as determined by western blot) by reducing the Aβ oligomerization. Another study supports these beneficial effects of cocoa against Aβ toxicity by showing that cocoa polyphenols reduced the Aβ oligomerization in vitro and rescued the synaptic deficits induced by Aβ oligomers in a mouse model suggesting that cocoa has multiple disease-modifying properties in AD (9). Another study found cocoa polyphenols triggered neuroprotection by activating brain-derived neurotrophic factor (BDNF) signalling pathway on both Aβ plaques and Aβ oligomers treated cells, resulting in the counteraction of neurite dystrophy [10]. Epicatechin, catechin and their mixture were able to protect PC12 cells from Aβ induced neurotoxicity in another study showing more evidence for the neuroprotective effects of cocoa polyphenols [12]. In another study, epicatechin and catechin inhibited the formation of Aβ fibrils from fresh Aβ1–40 and Aβ1–42 and destabilized preformed Aβ fibrils in a dose-dependent manner [43]. Diaz, Treviño [44] reported that epicatechin reduced the deterioration of spatial memory induced by the Aβ25–35, in addition to reducing oxidative stress and inflammation in the hippocampus of the animals treated with epicatechin+Aβ25–35. These previous findings support our study suggesting that cocoa can alleviate Aβ induced deficits in worm locomotion, cognition and lifespan may be through reducing the toxic Aβ levels and rescuing the neuronal damage through the action of polyphenols and peptide components.
5Conclusion
Our study demonstrated that cocoa-supplementation can protective against Aβ induced toxiciticy in C. elegans. We showed that cocoa can alleviate some Aβ induced deficits in worm locomotion, short-term associative memory and lifespan while reducing Aβ fibril levels in worms. However, further studies are required to identify the active components and mechanisms of action.
Acknowledgments and funding
This study was supported by La Trobe University Postgraduate Research Scholarship (LTUPRS) and La Trobe University Full Fee Research Scholarship (LTUFFRS).
Conflict of interest
There are no conflicts to declare.
References
[1] | Bekris LM , Yu C-E , Bird TD , Tsuang DW . Genetics of Alzheimer disease. J Geriatr Psychiatry Neurol. (2010) ;23: (4):213–27. |
[2] | Di Resta C , Ferrari M . New molecular approaches to Alzheimer’s disease. Clin Biochem. (2019) ;72: :81–6. |
[3] | Xu W , Tan L , Wang H-F , Jiang T , Tan M-S , Tan L , et al. Meta-analysis of modifiable risk factors for Alzheimer’s disease. (2015) ;86: (12):1299–306. |
[4] | Féart C , Samieri C , Barberger-Gateau P . Mediterranean diet and cognitive function in older adults. (2010) ;13: (1):14–8. |
[5] | Morris MC , Tangney CC , Wang Y , Sacks FM , Bennett DA , Aggarwal NT . MIND diet associated with reduced incidence of Alzheimer’s disease. Alzheimer’s & Dementia. (2015) ;11: (9):1007–14. |
[6] | Sathya S , Pandima Devi K . Chapter 15 - The Use of Polyphenols for the Treatment of Alzheimer’s Disease. In: Farooqui T, Farooqui AA, editors. Role of the Mediterranean Diet in the Brain and Neurodegenerative Diseases: Academic Press; (2018) . pp. 239–52. |
[7] | Vinson JA , Proch J , Bose P , Muchler S , Taffera P , Shuta D , et al. Chocolate is a powerful ex vivo and in vivo antioxidant, an antiatherosclerotic agent in an animal model, and a significant contributor to antioxidants in the European and American Diets. J Agric Food Chem. (2006) ;54: (21):8071–6. |
[8] | Lee KW , Kim YJ , Lee HJ , Lee CY . Cocoa Has More Phenolic Phytochemicals and a Higher Antioxidant Capacity than Teas and Red Wine. Journal of Agricultural and Food Chemistry. (2003) ;51: (25):7292–5. |
[9] | Wang J , Varghese M , Ono K , Yamada M , Levine S , Tzavaras N , et al. Cocoa extracts reduce oligomerization of amyloid-β: implications for cognitive improvement in Alzheimer’s disease. J Alzheimers Dis. (2014) ;41: (2):643–50. |
[10] | Cimini A , Gentile R , D’Angelo B , Benedetti E , Cristiano L , Avantaggiati ML , et al. Cocoa powder triggers neuroprotective and preventive effects in a human Alzheimer’s disease model by modulating BDNF signaling pathway. J Cell Biochem. (2013) ;114: (10):2209–20. |
[11] | Martorell P , Bataller E , Llopis S , Gonzalez N , Álvarez B , Montón F , et al. A Cocoa Peptide Protects Caenorhabditis elegans from Oxidative Stress and β-Amyloid Peptide Toxicity. PLOS ONE. (2013) ;8: (5):e63283. |
[12] | Heo HJ , Lee CY . Epicatechin and catechin in cocoa inhibit amyloid beta protein induced apoptosis. J Agric Food Chem. (2005) ;53: (5):1445–8. |
[13] | Link CD . Expression of human beta-amyloid peptide in transgenic Caenorhabditis elegans. Proceedings of the National Academy of Sciences. (1995) ;92: (20):9368–72. |
[14] | Link CD , Taft A , Kapulkin V , Duke K , Kim S , Fei Q , et al. Gene expression analysis in a transgenic Caenorhabditis elegans Alzheimer’s disease model. Neurobiol Aging. (2003) ;24: (3):397–413. |
[15] | McColl G , Roberts BR , Gunn AP , Perez KA , Tew DJ , Masters CL , et al. The Caenorhabditis elegans A beta 1-42 model of Alzheimer disease predominantly expresses A beta 3-42. J Biol Chem. (2009) ;284: (34):22697–702. |
[16] | Fong S , Teo E , Ng LF , Chen C-B , Lakshmanan LN , Tsoi SY , et al. Energy crisis precedes global metabolic failure in a novel Caenorhabditis elegans Alzheimer Disease model. Scientific Reports. (2016) ;6: (1):33781. |
[17] | Wu Y , Wu Z , Butko P , Christen Y , Lambert MP , Klein WL , et al. Amyloid-beta-induced pathological behaviors are suppressed by Ginkgo biloba extract EGb 761 and ginkgolides in transgenic Caenorhabditis elegans. J Neurosci.. (2006) ;26: (50):13102–13. |
[18] | Alexander AG , Marfil V , Li C . Use of Caenorhabditis elegans as a model to study Alzheimer’s disease and other neurodegenerative diseases. Front Genet. (2014) ;5: :279. |
[19] | Herndon LA , Schmeissner PJ , Dudaronek JM , Brown PA , Listner KM , Sakano Y , et al. Stochastic and genetic factors influence tissue-specific decline in ageing C. elegans. Nature. (2002) ;419: (6909):808–14. |
[20] | Kauffman A , Parsons L , Stein G , Wills A , Kaletsky R , Murphy C . C. elegans positive butanone learning, short-term, and long-term associative memory assays. J Vis Ex. (2011) (49): |
[21] | Sutphin GL , Kaeberlein M . Measuring Caenorhabditis elegans life span on solid media. Journal of visualized experiments: JoVE. (2009) (27):1152. |
[22] | Xin L , Yamujala R , Wang Y , Wang H , Wu WH , Lawton MA , et al. Acetylcholineestarase-inhibiting alkaloids from Lycoris radiata delay paralysis of amyloid beta-expressing transgenic C. elegans CLP4176. PLoS One. (2013) ;8: (5):e63874. |
[23] | Sorrenti V , Ali S , Mancin L , Davinelli S , Paoli A , Scapagnini G . Cocoa Polyphenols and Gut Microbiota Interplay: Bioavailability, Prebiotic Effect, and Impact on Human Health. Nutrients. (2020) ;12: (7):1908. |
[24] | Rechner AR , Smith MA , Kuhnle G , Gibson GR , Debnam ES , Srai SK , et al. Colonic metabolism of dietary polyphenols: influence of structure on microbial fermentation products. Free Radic Biol Med. (2004) ;36: (2):212–25. |
[25] | Katz DL , Doughty K , Ali A . Cocoa and chocolate in human health and disease. Antioxid Redox Signal. (2011) ;15: (10):2779–811. |
[26] | Jalil AMM , Ismail A . Polyphenols in cocoa and cocoa products: is there a link between antioxidant properties and health? Molecules. (2008) ;13: (9):2190–219. |
[27] | Robinson T , Ranalli AW , Phillips AW . Cocoa Polyphenols, Changes in Cocoa Tannins during Processing. Journal of Agricultural and Food Chemistry. (1961) ;9: (4):295–8. |
[28] | Kealey Kirk S , Snyder Rodney M , Romanczyk Leo J Jr , Hammerstone John F Jr , Buck Margaret M , Cipolla Giovanni G , inventors; MARS INC, assignee. Method for producing fat and/or solids from cocoa beans1996 1996/09/06/Application date. |
[29] | Gu L , House SE , Wu X , Ou B , Prior RL . Procyanidin and catechin contents and antioxidant capacity of cocoa and chocolate products. J Agric Food Chem.. (2006) ;54: (11):4057–61. |
[30] | Munasinghe M , Almotayri A , Thomas J , Heydarian D , Weerasinghe M , Jois M . Cocoa improves age-associated health and extends lifespan in C. elegans. Nutrition and Healthy Aging. (2021) ;6: :73–86. |
[31] | Aprotosoaie AC , Miron A , Trifan A , Luca VS , Costache I-I . The Cardiovascular Effects of Cocoa Polyphenols-An Overview. Diseases. (2016) ;4: (4):39. |
[32] | Keen CL , Holt RR , Polagruto JA , Wang JF , Schmitz HH . Cocoa flavanols and cardiovascular health. Phytochemistry Reviews. (2002) ;1: (2):231–40. |
[33] | Khan N , Monagas M , Andres-Lacueva C , Casas R , Urpí-Sardà M , Lamuela-Raventós RM , et al. Regular consumption of cocoa powder with milk increases HDL cholesterol and reduces oxidized LDL levels in subjects at high-risk of cardiovascular disease. Nutr Metab Cardiovasc Dis. (2012) ;22: (12):1046–53. |
[34] | Faria A , Pestana D , Teixeira D , Couraud PO , Romero I , Weksler B , et al. Insights into the putative catechin and epicatechin transport across blood-brain barrier. Food Funct. (2011) ;2: (1):39–44. |
[35] | Abd El Mohsen MM , Kuhnle G , Rechner AR , Schroeter H , Rose S , Jenner P , et al. Uptake and metabolism of epicatechin and its access to the brain after oral ingestion. Free Radic Biol Med. (2002) ;33: (12):1693–702. |
[36] | Nahabedian JF , Qadota H , Stirman JN , Lu H , Benian GM . Bending amplitude - a new quantitative assay of C. elegans locomotion: identification of phenotypes for mutants in genes encoding muscle focal adhesion components. Methods. (2012) ;56: (1):95–102. |
[37] | Lim YY , Baker JE , Bruns L , Mills A , Fowler C , Fripp J , et al. Association of deficits in short-term learning and Aβ and hippoampal volume in cognitively normal adults. Neurology.2020:10.1212/WNL.0000000000010728. |
[38] | Jahn H . Memory loss in Alzheimer’s disease. Dialogues Clin Neurosci. (2013) ;15: (4):445–54. |
[39] | Xue C , Lin TY , Chang D , Guo Z . Thioflavin T as an amyloid dye: fibril quantification, optimal concentration and effect on aggregation. Royal Society Open Science. (2017) ;4: (1):160696. |
[40] | Lee GD , Wilson MA , Zhu M , Wolkow CA , de Cabo R , Ingram DK , et al. Dietary deprivation extends lifespan in Caenorhabditis elegans. Aging cell. (2006) ;5: (6):515–24. |
[41] | Serpell LC . Alzheimer’s amyloid fibrils: structure and assembly. Biochim Biophys Acta. (2000) ;1502: (1):16–30. |
[42] | Phan HTT , Samarat K , Takamura Y , Azo-Oussou AF , Nakazono Y , Vestergaard MdC . Polyphenols Modulate Alzheimer’s Amyloid Beta Aggregation in a Structure-Dependent Manner. Nutrients. (2019) ;11: (4):756. |
[43] | Ono K , Yoshiike Y , Takashima A , Hasegawa K , Naiki H , Yamada M . Potent anti-amyloidogenic and fibril-destabilizing effects of polyphenols in vitro: implications for the prevention and therapeutics of Alzheimer’s disease. J Neurochem. (2003) ;87: (1):172–81. |
[44] | Diaz A , Treviño S , Pulido-Fernandez G , Martínez-Muñoz E , Cervantes N , Espinosa B , et al. Epicatechin Reduces Spatial Memory Deficit Caused by Amyloid-β25–35 Toxicity Modifying the Heat Shock Proteins in the CA1 Region in the Hippocampus of Rats. Antioxidants. (2019) ;8: (5):113. |