Molecular Variability in Levodopa Absorption and Clinical Implications for the Management of Parkinson’s Disease
Abstract
Levodopa is the most widely used medication for the symptomatic treatment of Parkinson’s disease and, despite being an “old” drug, is still considered the gold standard for offering symptomatic relief. The pharmacokinetic and pharmacodynamics of levodopa have been studied extensively. Our review explores the molecular mechanisms that affect the absorption of this drug, focusing on the large intra- and interindividual variability of absorption that is commonly encountered in daily clinical practice, and on the interaction with other medications. In addition, we will explore the clinical implications of levodopa absorption variability and address current and future strategies for researchers and clinicians.
BACKGROUND
Levodopa was approved by the FDA for medical use in the United States in 1970 and, since then, it has been the gold-standard treatment for persons with Parkinson’s disease (PD).1 It is also used in the treatment of other parkinsonian syndromes (e.g., progressive supranuclear palsy, multiple system atrophy, corticobasal degeneration), but levodopa is generally much less effective for these conditions. Indeed, a reduced response to oral levodopa medication is a red flag indicative of diagnoses other than PD.2 However, people that respond poorly to levodopa may yet still have an underlying diagnosis of PD, if the unsatisfactory response to the medication is explained by inadequate gastrointestinal absorption. Several mechanisms have been studied to find possible factors that impair levodopa absorption. It has been reported that, if administered alone, only 5% of orally administered levodopa is capable of reaching the bloodstream, whereas only 1% can cross the blood-brain barrier.3 Even though different routes to administer levodopa are available, such as inhaled, subcutaneous and intrajejunal levodopa, they have specific indications, and the oral route remains the mainstay for treatment in the majority of people with PD.
Obstacles in the transport of levodopa to the small intestine have a major role in reducing the response to the drug; particularly, secondary to a peripheral metabolization of the drug for which prolonged transit time in the gastrointestinal tract forms an important risk factor.2 Dysphagia, gastroparesis, and constipation can all play roles in explaining a decreased levodopa bioavailability. Moreover, the role of gut microbiota has been extensively studied as a potential factor that may interfere with levodopa absorption. Helicobacter pylori infection has been one of the most common bacteria investigated, but data have yet to demonstrate some consistent results on its negative impact on the pharmacokinetics of levodopa.2 Furthermore, it has been well established that high-protein meals can prevent levodopa absorption, which supports the recommendation that the medication should not be taken concurrently with food (but on an empty stomach, one hour before food intake). Finally, any surgical interventions or medical comorbidities that significantly impair the absorptive function of the gastrointestinal tract can cause a decrease in the drug’s uptake.2
These obstacles alone do not seem sufficient to explain levodopa malabsorption in a significant percentage of patients. A better understanding of these mechanisms is important, both for diagnostic reasons (given the importance of a gratifying response to levodopa for establishing a diagnosis of PD) and obviously for therapeutic reasons, as a better insight into the causes of malabsorption may lead to improved management strategies. Therefore, this narrative review attempts to further explore the mechanisms through which an unsatisfactory response to oral levodopa may occur, focusing mainly on the molecular pathway of the drug from the small intestine to the brain.
MOLECULAR PATHWAYS
rBAT/b(0, +)AT
rBAT: Neutral and Basic amino acid transport protein
b(0, +)AT: b(0, +)-type amino acid transporter. In 2014, Camargo et al. identified the transporter rBAT/b(0, +)AT as the main involved in levodopa absorption in the small intestine, in a study performed in Xenopus laevis oocytes, canine kidney epithelial cells and mice enterocytes. The transporter consists of the heavy chain, rBAT, encoded by gene SLC3A1, whereas the light chain, b(0, +)AT, is encoded by gene SLC7A9.4 Located in the apical membrane, rBAT/b(0, +)AT is a transporter known to play a crucial role in the exchange of neutral and dibasic amino acids across epithelial cells of the renal proximal tubule and small intestine (see Fig. 1). This transporter has several amino acids as substrates, for example: leucine, arginine, cysteine, L-methionine, L-glutamine, L-histidine, L-ornithine, L-phenylalanine and L-tyrosine. Interestingly, the last two L-amino acids have a very similar molecular structure to levodopa.4
Fig. 1
Overview on the molecular pathway of levodopa in the small intestine.
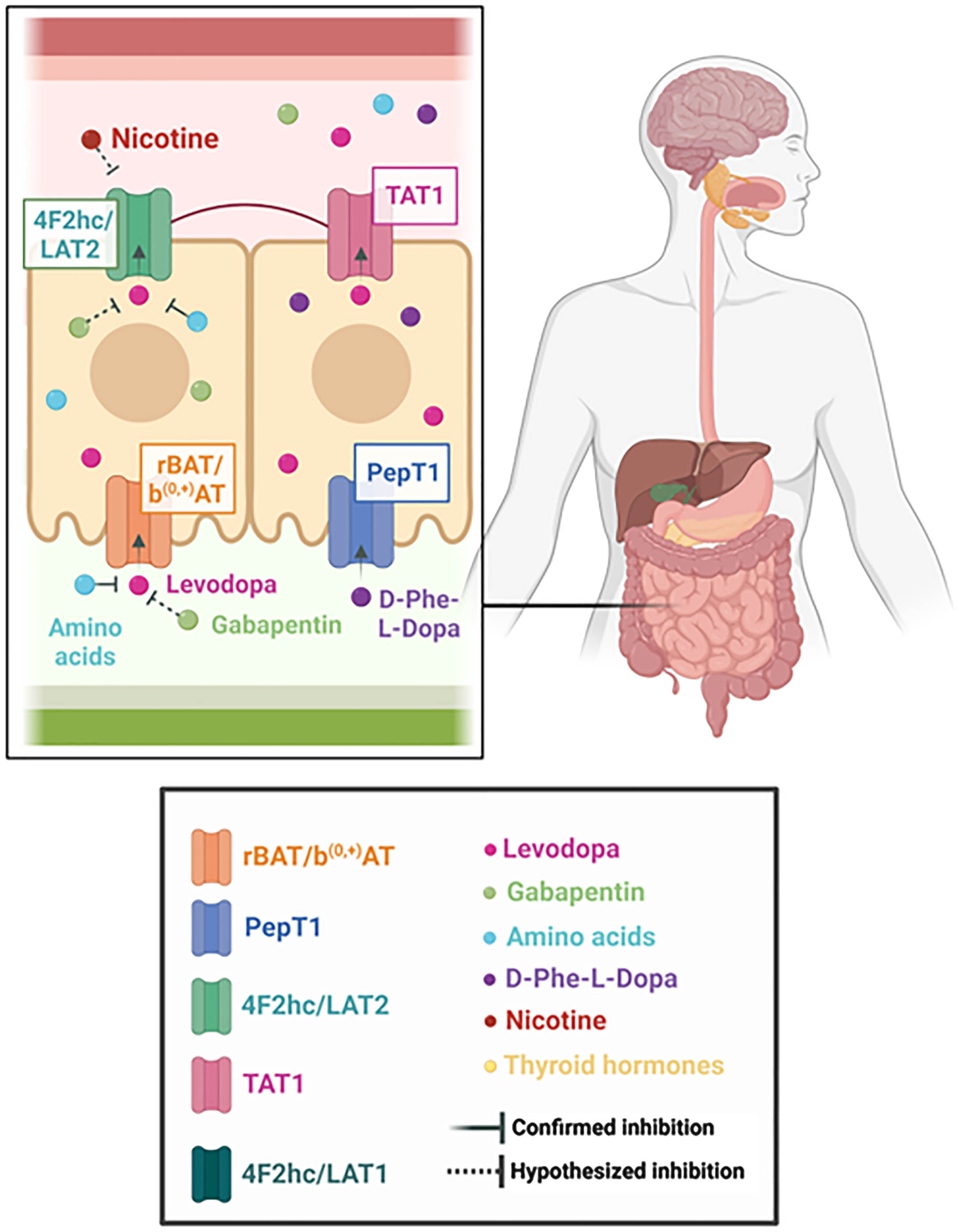
Along with the discovery that levodopa is also a substrate to rBAT/b(0, +)AT, it was also observed in Xenopus laevis oocytes and in intestinal enterocytes that the drug’s uptake was inhibited by the presence of luminal L-arginine and L-leucine, which indicates that neutral and cationic amino acids compete with the dopamine precursor for the same transporter.4 Benserazide and carbidopa, dopa decarboxylase (DDC) inhibitors, did not inhibit rBAT/b(0, +)AT -mediated transport of levodopa, and neither did entacapone.4 Although it is known that protein-rich meals inhibit levodopa absorption, it is noteworthy that amino acids can be found in different types of food. For example, arginine is present in seafood, nuts, seeds, algae, soy protein isolate and watermelon.5 Meanwhile, leucine is an important component of milk, matured cheese, poultry, unprocessed beef, oily and white fish, eggs, legumes, and bread.6 According to the U.S. Department of Agriculture Nutrient Database, for example, 100 grams of dried butternuts contains a 4.86% concentration of arginine, whereas the same quantity of cooked bacon contains a 2.51% concentration. As for leucine, 100 grams of flaxseed contains 1.38% concentration of this nutrient, similar to 100 grams of Grade A egg yolk, which contains 1.35%.7 Therefore, levodopa absorption can be inhibited not only by foods that are commonly perceived as high-protein, but by any significant source of amino acids.
Levodopa is not the only drug that seems to use this transporter in the intestine. The structural analog of GABA, gabapentin, has been suggested to use the same b(0, +)AT system to reach the bloodstream from the intestine.8 Not only the uptake of the drug demonstrates a sodium-independent pattern, but it has also been observed that gabapentin uptake can be cross-inhibited by the presence of both neutral and cationic amino acids. An oligopeptide transporter in the apical membrane, PepT1, has been reported to trans-stimulate the b(0, +)AT system, increasing gabapentin uptake (see below).9 Pregabalin, another gabapentinoid, has also been reported to be transported through b(0, +)AT.8
Cystinuria has been described as a condition derived from mutations in either or both of the genes that encode rBAT/b(0, +)AT (SLC3A1/SLC7A9), causing defective transport of the amino acids in the small intestine and in the renal proximal tubules and, consequently, renal loss of the amino acid.10, 11 The worldwide prevalence of cystinuria has been estimated at 1:7,000, which makes it one of the most common autosomal recessive disorder. In the US, the prevalence appears to be 1:10,000.11 Clinically, because cystine is quite insoluble in urine, it can potentially crystalize in the urinary tract and predispose these individuals to the formation of kidney stones and chronic kidney disease.10 Nonetheless, other than the possibility of incomplete penetrance of the mutation,12 there have also been reports of silent carriers, mostly associated with SLC3A1 mutations, who excrete cystine within the normal range.13 Therefore, it is reasonable to believe that these individuals could escape detection and remain undiagnosed as SLC3A1 mutation carriers. Interestingly, in spite of defective transport via rBAT/b(0, +)AT, even symptomatic patients are unlikely to develop malnutrition, given that the amino acids are often absorbed in the intestine as oligopeptides.14 To our knowledge, there are no studies in the literature investigating the correlation between such mutations and possible levodopa malabsorption. Therefore, it is undetermined whether in individuals carrying such mutations, levodopa could be absorbed similarly to cystine, causing a reducedresponse.
Sex differences may also take part in levodopa absorption. A study observed that SLC3A1 mRNA levels were significantly higher (15-fold) in the duodenum of female mice than in males. Androgen (DHT) and estrogen (E2) replacement further highlighted this difference.15 In parallel, hypophysectomized female mice presented a lower expression of SLC3A1 mRNA, whereas the same was not significant for male mice. Another finding of this study was that SLC3A1 mRNA expression is higher in the duodenum than in the jejunum and ileum.15 One of the limitations when interpreting this study is to infer that mRNA levels have a direct correspondence to protein levels. However, it has been demonstrated previously that women present a superior bioavailability of levodopa than men, which potentially corroborates this finding.16
4F2hc
4F2 heavy chain
Similarly to rBAT, 4F2hc (also known as CD98hc or FRP1; or SLC3A2, due to its homonymous gene) is a heavy chain responsible mainly for stabilizing and trafficking the heteromeric amino acid transporters to the cell membrane.17 Six different light chains (LAT1, LAT2, y+LAT1, y+LAT2, asc-1, and xCT) can bond to 4F2hc and they are the ones that mediate transport function.17, 18 Moreover, 4F2hc is also known to play a crucial role in integrin signaling and mediate cell proliferation.19, 20 It has been extensively studied as a potential target for cancer cell therapy.20 There is limited information in mutations related to the SLC3A2 gene.21 Therefore, in the levodopa molecular pathway, the relevance of 4F2hc regards its role as a chaperone to the light chains LAT1 and LAT2, which will be discussed below.
LAT system
Large Amino Acid Transport system
LAT transporters are Na+-independent antiporters.22, 23 In the discussion of levodopa absorption, two light chains will be highlighted: LAT2, in the small intestine, and LAT1, in the blood-brain barrier (see Fig. 2).20
Fig. 2
Overview on the molecular pathway of levodopa in the brain.
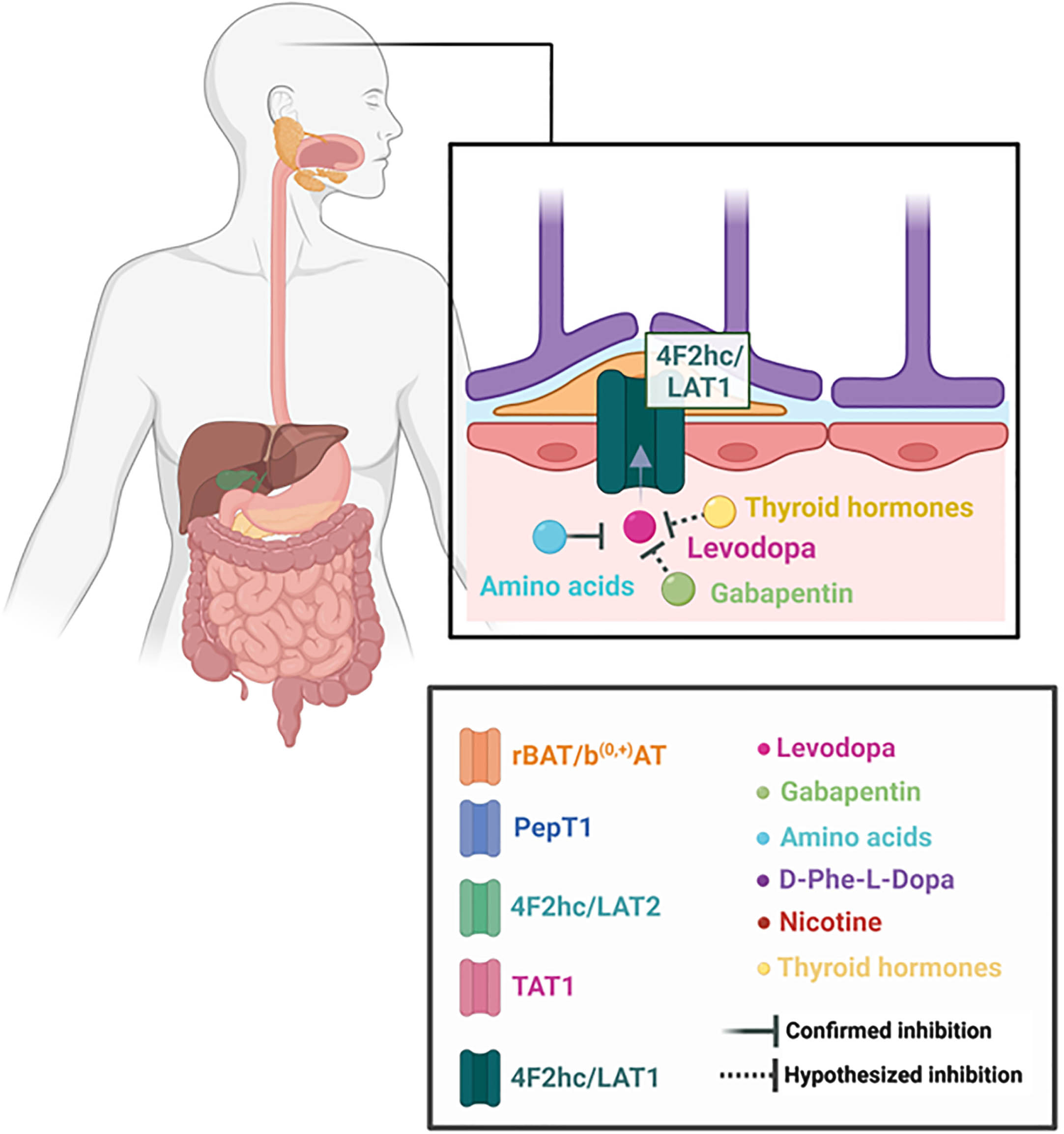
Some studies, particularly older, do not specify which light chain is involved in the transport of some substances and refer to them as LAT system or System L. Regardless, more recent research has determined the heterogeneity of which LAT1 and LAT2 are distributed throughout the human body.
LAT2
Large Amino Acid Transporter 2
LAT2 is a Na+ independent antiporter, encoded by gene SLC7A8, that transports large and small neutral amino acids in epithelial cells, such as the ones in the renal and intestinal tracts.23,24 In comparison with LAT1, LAT2 displays broader substrate selectivity, but lower affinities. It is present in the basolateral membrane of the cells and involved in the efflux phase of the transport of its substrates.23, 24 Braun et al. observed increased urine concentration of glycine, serine, threonine, glutamine, leucine and valine in SLC7A8–/– mice, suggesting that these are some of the amino acids transported through LAT2.25 Moreover, this transporter has been repeatedly associated with levodopa transport from epithelial cells to blood circulation.26–29 Soares-da-Silva et al. observed that SLC7A8 gene silencing significantly reduced the inward and outward flow of levodopa through renal cells, further corroborating its role in the drug transport and providing further evidence of no significant LAT1 activity in levodopa outward transfer in these epithelial cells.26 Additionally, the research was able to supply data on the modulating factors for the transporter, as it had a better response in exporting levodopa in a more acidic extracellular medium, with pH levels of 7.4 to 6.2.26 The study also demonstrated that L-isomers of the small and large amino acids (e.g., alanine, serine, leucine, isoleucine, phenylalanine, methionine, tyrosine, histidine, tryptophan) promoted a marked decrease in levodopa accumulation.26 Interestingly, BCH (2-Amino-2-norbornanecarboxylic acid), a selective inhibitor of the LAT system, not only had a greater rate of levodopa outward transfer, but it was able to block the drug uptake, indicating an inhibitory effect on the accumulation of levodopa inside thecells.26
There are findings that support that gabapentin uses the LAT2 transporter in intestinal cells. In vivo studies in rats demonstrated that the drug is inhibited by the presence of BCH,30 as well as by L-leucine and L-phenylalanine.31 Pregabalin also presents the system L pattern of transport through cells and it even demonstrated mutual inhibition with gabapentin in Caco-2 cells, which have a remarkably similar structure to human small intestinal epithelialcells.32
A number of studies has also indicated involvement of LAT transporters in the uptake of thyroid hormones (T3 and T4). In choriocarcinoma cells, it was observed that T3 and T4 could inhibit the transport activity of LAT transporters [32]. In Caco2 cells, T3 and T4 uptake was Na+-independent, saturable and increased with time. These results evidence that T4 uptake was highly pH dependent, with increased uptake at 5.3 pH, whereas T3 uptake appeared to be pH-independent.33, 34 This information contrasts with the previous description of the increased levodopa outflow in a more acidic medium, given that LAT2 seems to be involved exclusively in the transport of T3; in particular, 3,3’-diiodo-L-thyronine (3,3’-T2).35,36 Nonetheless, the LAT system is far from being the only one involved in the cellular mechanisms of thyroid hormones in intestinal cells, which could explain some confounding factors.36 Indeed, SLC7A8–/– mice did not present a significant difference in the levels of circulating thyroid hormones, which corroborates the relevance of alternative pathways for thyroid hormones to reach circulation.25
Unlike SLC3A1/SLC7A9 mutations, which cause cystinuria, SLC7A8 mutations do not seem to have a very strong correlation with any known disease. Braun et al. described that the phenotype of SLC7A8–/– mice is remarkably mild.25 Nonetheless, some mutations in the SLC7A8 gene have been correlated with age-related hearing loss,37 which could potentially indicate the role of the transporter in the auditory system. Similarly, Knöpfel reported an association of age-related cataract with LAT2 knockout mice.38
Furthermore, in 2013, Kyaw et al. performed in vivo and in vitro studies to investigate levodopa absorption in respect of the presence of nicotine.39 The group explored the levodopa transport through Caco2 cells with and without the presence of nicotine, as well as cotinine, a nicotine metabolite with a longer half-life. After confirming that levodopa transport was mediated by the LAT system with the use of BCH, the authors observed a reduction in the permeability of the drug in the presence of nicotine (25.3%) and cotinine (54.1%).39 To our knowledge, this is the only study that has been published that correlates levodopa absorption and nicotine in the small intestine. Nicotine has been extensively studied as a potential neuroprotective factor in PD and as a possible therapy for levodopa-induced dyskinesia.40–42 Nonetheless, most of the studies focus on nicotine derived from tobacco. It is interesting to observe that nicotine can also be present in a variety of foods, such as potatoes, tomatoes, and peppers, although in a smaller amount.43 The in vivo studies performed by Kyaw et al. consisted of the administration of oral levodopa with and without transdermal nicotine patch application in healthy male volunteers.39 The results demonstrated that the mean plasma concentration of levodopa was significantly decreased in the group with the nicotine patch in comparison with the control group.39 The mechanisms of this inhibition were not explored in the article, but it is interesting to notice that the enteral system was spared due to the application of transdermal patch and yet, a pattern of inhibition was observed. This may suggest alternative pathways unrelated to the intestine through which levodopa absorption could be impacted.
LAT1
Large Amino Acid Transporter 1
The light chain LAT1 is encoded by gene SLC7A5.20 It is abundantly present in blood-brain barrier cells, as well as in the placenta, testis and some types of tumors.22,44,45 It plays an important role in the transport of large neutral amino acids, such as leucine, isoleucine, valine, phenylalanine, tyrosine, tryptophan, methionine and histidine.45 It is a crucial antiporter involved in the transport of several drugs through the blood-brain barrier: not only levodopa, but also 3-O-methyldopa, alpha-methyldopa, baclofen and melphalan.46–49 Gabapentin and pregabalin, previously described in this review, are also known substrates of LAT1.50,51
The structure and function of LAT1 have been increasingly studied due to possible associations of this receptor with several medical conditions, including cancer, insulin resistance and immune diseases.52–54 LAT1 transporter has also been correlated with autism, ALS and PD.54–57 The increased risk of autism in mouse models carrying variants in the SLC7A5 seem to be associated with an imbalance of amino acids exchange and metabolism across the blood-brain barrier (BBB).55,58
Reduced mRNA expression of LAT1 is associated with decreased levodopa transport across the BBB.56 A study demonstrated that the drug’s uptake in mouse brain capillary endothelial cells was almost entirely blocked by the presence of excess histidine, isoleucine, leucine, methionine, phenylalanine, tryptophan, tyrosine, valine and BCH, suggesting a competition for the transporter. The same mechanism was not observed for glutamate, lysine and arginine.46 Another result from this research was the finding for a preference of the 4F2hc/LAT1 complex for levodopa, phenylalanine, tryptophan and tyrosine.46
Tryptophan is an essential amino acid; therefore, it must be obtained from external sources and cannot be endogenously produced.59 Common sources of this substance are oats, bananas, dried prunes, milk, tuna fish, cheese, bread, chicken, turkey, peanuts and chocolate.60 There is a complex interaction between levodopa (and other dopaminergic agents) and tryptophan (and also its products, such as 5-hydroxytryptophan), which is beyond the scope of this review.61 Nonetheless, a competition of these substrates for transporters has been demonstrated.62 Indeed, a study performed in 1978 by Allikmets et al. demonstrated that brain dopamine levels had a lower increase when levodopa was intraperitoneally injected simultaneously with L-tryptophan in male rats.63 Moreover, in 2011, Okuno et al. demonstrated a significant reduction in dopamine release in the striatum of rat models when they were maintained on a high tryptophan diet.64
Interestingly, Taylor et al. indicated that T3 and tryptophan share a similar cellular uptake mechanism and that, indeed, LAT1 is competitively inhibited by T3 in a study involving astrocytes from Xenopus laevis embryos.65 Admittedly, like LAT2 in the small intestine, LAT1 transporters have a major role in thyroid hormones uptake in brain cells.65,66 This antiporter seems to promote the uptake of all iodothyronines, with a predilection for 3,3’-T2 and rT3.66 In this context, it is intriguing to mention that there is scientific data that shows a reduction in TSH concentrations in the first 2 hours after levodopa/carbidopa intake by PD patients, but the same reduction is not observed in patients diagnosed with multiple system atrophy (MSA).67 Indeed, a 2022 systematic review and meta-analysis demonstrated a correlation between PD and thyroid dysfunction.68
In 2002, Uc et al. observed that intraperitoneal injections of beta-adrenergic agonists (isoproterenol and clenbuterol) significantly increased brain extraction of levodopa and L-leucine, without altering CBF. Nadolol, a beta-adrenergic antagonist, was able to block this effect.69 Similar results of an increase in large neutral amino acids transport to the brain with isoprenaline have been reported.70,71 Moreover, a small study in 8 patients published in 1994 demonstrated benefit on Parkinsonian symptoms with the use of albuterol and a more recent pilot study (also with 8 participants) provided some additional tentative evidence for the use of this beta-agonist as an adjuvant therapy for PD patients, as it demonstrated an improved therapeutic response to levodopa.72,73
PEPT1
Peptide Transporter 1
PepT1 (SLC15A1) is present in the apical membrane of small intestinal cells (see Fig. 1).74 However, its main role is not to transfer amino acids, but to act as an oligopeptide transporter.74, 75 It carries a vast array of substrates, which include several drugs, such as bestatin, beta-lactam antibiotics, angiotensin-converting enzyme inhibitors and valacyclovir.76–79 Numerous regulatory mechanisms have been described for this transporter, including hormones, pharmacological agents and diseases.80 Hormonally, it appears to be upregulated with higher plasma concentrations of insulin, treatment with recombinant human growth hormone and short-term exposure to epidermal growth factor (EGF).80–82 Pharmacologically, 5-flourouracil, enrofloxacin, xylanase and pentazocine upregulate this transporter, whereas clonidine amplifies the migration of preformed PepT1 from cytoplasm to the membrane.80, 83, 84, 85
For the molecular understanding of the levodopa pathway through the intestinal system, PepT1 is of great relevance. As an experimental physiological model, in 2017, a group of researchers synthesized the chemical compound D-phenylglycine-L-dopa by bonding a molecule of D-phenylglycine to a levodopa molecule; therefore, creating a dipeptide prodrug of levodopa.86 The study observed a 31.1-fold higher plasma concentration of levodopa when this compound was orally administered than when levodopa was administered alone. Moreover, the compound had a greater anti-Parkinsonian effect than levodopa in 6-OHDA lesioned rats. The brush-border membrane vesicle uptake of the compound was inhibited by dipeptides (L-Gly-L-Pro and L-Gly-L-Phe) and by a known substrate of PepT1, cephradine, supporting the involvement of this transporter in this process.86 These results have not prompted the development of D-phenylglycine-L-dopa as a therapeutic compound for human use, to our knowledge. It is worth mentioning the previously discussed study that observed that trans-stimulation of b(0, +)AT by PepT1 in the apical membrane of enterocytes was capable of increasing gabapentin’s uptake.9 To our knowledge, this trans-stimulation phenomenon has not been yet studied for levodopa.
Interestingly, PepT1 can play a role in the absorption of amino acids. It has been reported that patients with cystinuria may compensate for the loss of SLC3A1 in the intestine by using PepT1 to reabsorb cystine and other amino acids.87 Moreover, it has been suggested that PepT1 has greater relevance in the context of high-protein loads in the intestine, as it is capable of providing additional absorption capacity when amino acid transporters are saturated.88
TAT1
T-type Amino Acid Transporter 1
TAT1 is a Na+ and Cl- independent transporter, present in the basolateral membrane of epithelial cells (see Fig. 1).24, 89 It is encoded by the SLC16A10 gene and it mediates the transport of aromatic amino acids in the intestinal, renal and liver cells, such as tryptophan, tyrosine and phenylalanine.89, 90 Unlike LAT2, it functions as a facilitated diffusion pathway.91 The main role of this uniporter seems to be the property to facilitate the efflux of neutral amino acids through LAT2/4F2hc by providing influx substrates and, therefore, maintaining homeostasis.90,91 It has been demonstrated that levodopa can act as one of its substrates as well and it can even inhibit L-tryptophan and L-phenylalanine uptake by TAT1.92
A study described that defective mice (TAT1–/–) did not display a remarkable phenotype, except for a significant aromatic aminoaciduria and a mild aminoaciduria of neutral amino acids in the context of a high-protein diet.93 However, the 2014 study by Camargo et al. demonstrated that, while TAT1 knockout mice did not differ from wildtype in regards to intracellular levodopa accumulation, they had a significantly reduced transepithelial transport of the drug.5 This suggests an important role of this transporter in levodopa efflux in intestinal enterocytes.
Moreover, a condition that appears to be associated not only with TAT1, but with LAT2 mutations as well, is the rare disease called blue diaper syndrome, caused by tryptophan malabsorption.92
Heat stress
The transporters involved in the molecular pathway of levodopa are also subject to external factors, such as heat stress. A study conducted by Habashy et al. in 2017 compared samples of ileum and muscle tissues amongst two different groups of chickens: one maintained at an environmental temperature of 25°C and another maintained at 35°C. The group of poultry living at a higher temperature, after one day, presented, in both types of tissues, downregulated mRNA levels of b(0, +)AT, TAT1 and LAT1, some of the same transporters discussed here regarding their involvement in levodopa transport. Consequently, they had reduced growth and amino acid consumption and retention.94 Similar findings of inhibition of intestinal digestion and a disturbance of intestinal microbiota were observed in a group of sturgeons exposed to heat stress. The explanation for this phenomenon seems to rely on the epithelial damage and inflammatory response promoted by heat stress.95 In humans, there are limited data on exposure to hyperthermic conditions. Strenuous exercise (i.e., high-intensity aerobic exercise) enhanced intestinal permeability in human subjects in as little as twenty minutes. This could potentially be explained by a state of hypoperfusion, where the circulation is redirected to the periphery, rather than the gut.96 Following prolonged exercises, a rise in core temperature and dehydration could also play a role in this process.96 Taken together, if anything, participation in exercise should enhance the bioavailability of levodopa, and not reduce it. Exercise is also a widely accepted symptomatic management strategy for people with PD, and the idea that enhanced physical activity could promote intestinal permeability and increase the symptomatic response to levodopa only compounds the benefits of exercise. Another endogenous hyperthermic condition may arise during illness. Indeed, there is evidence that fever can induce a delay in gastrointestinal emptying, which, in theory, would indicate a decrease in drug absorption and thus lead to a worsening of Parkinson symptoms. However, the stress associated with the underlying illness made by itself also explain this worsening, and there is insufficient evidence at this point to merely incriminate poor levodopa bioavailability as the reason for clinical deterioration during illness and fever. Fever can also induce tachycardia, which could increase tissue perfusion. Taken together, it is still uncertain how endogenous hyperthermic states could impact levodopa absorption and this area deserves further research.97,98
Interestingly, another aspect to be observed is the seasonal variance of Parkinson’s symptoms and levodopa dosage. This is true for both motor and nonmotor symptoms, and people with PD generally feel better during the warmer months of the year.99 Traveling to warmer climates during the holiday season is also associated with an improvement in symptoms by a proportion of people with PD.100 There are data to suggest that an increase in levodopa equivalent dose (LED) is associated with higher temperatures and warmer months. One possible explanation would be a reduced production of dopamine in the context of seasonal variance.101,102
Tricyclic antidepressants
In 1975, Morgan et al. observed a reduction in the tissue concentration and urinary excretion of levodopa in rat models with a 30 or 3-day use of oral imipramine, suggesting a possible levodopa inhibition by this tricyclic antidepressant.103 In a separate study, performed in human subjects, the urinary excretion of dopamine and its metabolites were reduced in individuals who received pre-treatment with imipramine.104 One of the explanations for this interference, suggested by the authors, was a delay in gastric and intestinal emptying caused by the anticholinergic effect of the antidepressant. If this hypothesis is confirmed, not only imipramine should be a concern for levodopa absorption, but several other drugs that create an anticholinergic response on the organism (e.g., tricyclic antidepressants, dihydropyridines, H2-antagonists, vitamin K antagonists).105, 106
A more recent study observed differences in behavioral effects with the concomitant use in rat models of tricyclic antidepressants (clomipramine, amitriptyline and desipramine) and levodopa, with respect to their varied affinity to serotonin and norepinephrine transporters. Specifically, the results showed an attenuation of levodopa’s effect when in co-administration with any of the three antidepressants in comparison to levodopa administered alone.107 It is crucial to mention that levodopa was administered subcutaneously and that the tricyclic antidepressants were injected intraperitoneally.107 Therefore, the findings of this study suggest a mechanism of inhibition that occurs outside the enteral system, which should encourage more studies exploring possible molecular interference on the levodopa pathway.
In contrast, one study actually observed an improvement in motor function, assessed by the number of contralateral rotations, with the concomitant and chronic use (21 days) of amitriptyline and levodopa in rats.108 Notwithstanding, the rats had a unilateral 6-OHDA lesion and were previously treated with a single dose of apomorphine, a dopamine agonist.108 These conditions could have possibly interfered with the results. It is also worth mentioning that an in vitro study using rat brains demonstrated that imipramine had an inhibitory effect on monoamine oxidase (MAO-A and MAO-B), enzymes that metabolize dopamine.109 It is known that MAO-B inhibitors are vital components of PD therapy for several patients.110
CLINICAL IMPLICATIONS
Screening for genetic mutations
A profound understanding of the molecular pathway involved in the transit of levodopa from the small intestine to the brain opens numerous possibilities for genetic research. Although there are dozens of other genes currently being studied and tested in PD patients, it would be relevant to study whether mutations in the SLC family of transporters, especially SLC3A1, SLC3A2, SLC7A5, and SLC7A9, and mutations in TAT1 and PepT1, could have good validity in predicting levodopa response in PD patients. This approach could further play a role in advancing Precision Medicine for persons with PD.
Concomitant use of levodopa and other drugs
The fact that levodopa shares common pathways with other drugs does not necessarily imply that they can compete for the same transporters. Nonetheless, until there are reliable scientific data to reject this hypothesis, we feel that patients demonstrating an unsatisfactory response to oral levodopa should be advised to avoid the concurrent intake (at the same time as levodopa) of baclofen, alpha-methyldopa, melphalan and tricyclic antidepressants. Any drug with anticholinergic effects should also be taken separately from the antiparkinsonian drug until further data become available. In particular cholinesterase inhibitors are commonly used in PD. Therefore, we recommend to take anticholinesterase inhibitors (donepezil, rivastigmine, etc.) at a separate time during the day and, if possible, to avoid the oral route of administration to mitigate possible interference with absorption of levodopa.
Iron supplementation should also be avoided as it is likely that chemical chelation of levodopa reduces its bioavailability.111 Moreover, given the evidence that both LAT1 and LAT2 are involved in the transport of thyroid hormones, the same recommendation could be applied to thyroid hormone replacement therapy.
Gabapentin and pregabalin, commonly prescribed worldwide for the treatment of neuropathic pain, warrant special consideration. These drugs are transported from the intestine to the cell using some of the same transporters involved in the levodopa pathway. As they are mutually inhibited by the presence of different amino acids, it is possible that they could inhibit each other’s absorption as well. To our knowledge, there is only one published study that analyzed the effect of gabapentin on the motor response to levodopa. It demonstrated that the administration of a maximum of 2400 mg/day of gabapentin, in patients in use of their regular antiparkinsonian drug regimen, had an isolated improvement of the UPDRS III off score in PD patients.112 Further clinical studies will be necessary to accurately determine the effect of the concomitant use of gabapentin (or pregabalin) and levodopa. Interestingly, one study investigated if gabapentin’s absorption was reduced after a high-protein meal, but this did not appear to be the case. Notwithstanding, patients who took gabapentin after a high-protein meal reported reduced adverse effects from the medication, which could reflect a reduced penetration of the drug in the central nervous system.113 A similar study reached similar results: food did not interfere with gabapentin’s concentration in serum, saliva and urine.114
Levodopa and food
The recommendations that levodopa should be taken at least 1 hour before and 2 hours after food intake derives mainly from studies that demonstrated a reduced absorption of the drug when in association with a high-protein meal. The information collected for this review further corroborates this finding. It is crucial to note that even foods that are not usually perceived as protein-rich can contain many of the amino acids that use the same transporters in the levodopa pathway and, potentially, compete with the drug for absorption. Therefore, patients using levodopa should be cautious about food intake when taking their medication.
Levodopa, exercise and heat exposure
We discussed how some transporters are downregulated in the exposure to heat stress. Against this background, and until there are more data to confirm or refute this, PD patients using levodopa, especially the ones with a poor response to the medication, should try to avoid hyperthermic conditions (e.g., hot weather, hot showers or tubs, saunas, or sunbathing) for a prolonged time. In regards to exercise, considering its comprehensive list of benefits (including symptomatic and possibly even disease-modifying effects in PD),115 its impact on levodopa absorption can be further explored. Moreover, different strategies could be scientifically studied to stabilize gut microbiota and reduce cellular oxidative stress, with the aim to mitigate the damage caused by heat stress; these include: microbiota modulation, the use of antioxidants and omega-3 fatty acids and amino acid supplementation.116
Boosters for better levodopa absorption
Caffeine is one of the most consumed stimulants in the world.117 The correlation between caffeine consumption and a lower risk of developing PD is still controversial and beyond the scope of this review.118 However, one crossover, double-blinded and randomized study demonstrated that caffeine has the potential to shorten the latency to the levodopa response, assessed by walking and tapping speed, and to increase the walking magnitude.119 The effect of the stimulant seems to be largely due to its interaction with the adenosine 2A receptor, which appears to be associated with a neuroinflammatory process in PD.118 Non-selective adenosine antagonists, such as theophylline, and selective adenosine 2A antagonists, such as istradefylline have been formulated and approved as adjuvant symptomatic therapy to PD treatment in many countries.118, 120 Caffeine, however, can act not only as a non-selective antagonist to adenosine receptors, A1 and A2, but also as an inverse agonist to adenosine 2A receptors. It has been described that inverse agonists to adenosine receptors have a dual role, both as antagonists, but also, because of higher affinities for the inactive form of the receptors, as blockers to the constitutive receptor activity, which counter the action of the drugs acting through D2 dopamine receptors. Therefore, it has the potential to enhance the action of dopaminergic drugs.120, 121
Ascorbic acid has been studied as a potential preventive and therapeutic drug in the field of neurodegenerative diseases.122 Ascorbic acid has been demonstrated to inhibit levodopa and carbidopa degradation induced by magnesium oxide.123, 124 An in vitro study observed that, when administered with magnesium oxide, levodopa plasma levels were stable, but carbidopa concentration was progressively decreased. The addition of ascorbic acid to the solution was able to prevent the degradation of carbidopa, suggesting that ascorbic acid can reduce levodopa degradation in the periphery and increase its bioavailability.123 Similarly, another study demonstrated that elderly PD patients with poor levodopa availability had an increase in the area under the curve (AUC), peak drug concentration and a reduction in the time to peak drug concentration with the administration of 200 mg ascorbic acid together with levodopa/carbidopa.125 The previously mentioned fact that LAT2 transporter has a better response in more acidic medium may also play a role in these findings. Taken together, these findings indicate the need for more studies about the impact of ascorbic acid on levodopa absorption.
Interestingly, the formulations of levodopa/carbidopa plus ascorbic acid are usually presented as a liquid solution.126 There are different oral formulations of levodopa that aim to optimize the delivery of the drug to the small intestine. Melevodopa (levodopa methyl ester) plus carbidopa is an effervescent tablet, more soluble in water than standard levodopa, that has a more favorable pharmacokinetic profile.127 Studies have observed that melevodopa/carbidopa has a reduced latency to onset of the therapeutic response, without affecting the duration of the “on” time.128 It has even been observed that patients who switched to melevodopa in combination with entacapone had a significant reduction in the “off” period after 6 months.129 Orally disintegrating tablets (ODTs) are also an alternative to standard levodopa. Indeed, in a study with 60 PD patients, 45% preferred the ODTs rather than the conventional treatment.130 Another option, however, would be to take standard levodopa with carbonated water, with the goal to help decrease the latency of the response to the antiparkinsonian medication. The rationale behind this strategy would be to accelerate the disintegration of the tablet and prevent that undigested medication remains in the enteral system;131 also, carbonated water is slightly acidic.
Fibers have also been investigated as a potential candidate to optimize levodopa absorption. A diet rich in insoluble fiber was able to increase plasma levels of levodopa, possibly because of improvement of constipation.132, 133 The greatest effect was observed when fibers were administered early after each dose (30 to 60 minutes).133 Therefore, it would be interesting to investigate whether meals rich in insoluble fiber one hour following levodopa intake could significantly improve the treatment. In addition, any other measures to prevent or minimize constipation should be recommended to people with PD, including regular physical activity, drinking sufficient fluids and use of laxatives if needed.
One study investigated concomitant administration of soybeans with levodopa/carbidopa and observed that this combination increased the self-rated “on” time and reduced dyskinesia symptoms, in comparison to the group which did not receive soybeans.134 This contrasts with the information that soy is rich in amino acids and, therefore, has the potential to inhibit levodopa absorption. More data are necessary to investigate the role of soybeans in levodopa absorption.
As previously mentioned, beta 2-agonists as adjunct therapy to levodopa has been correlated with improvement of Parkinsonian symptoms.71, 72 Nonetheless, large, randomized clinical trials are necessary to further corroborate this association.135 Interfering factors in levodopa absorption are summarized in Fig. 4.
Fig. 3
Elements that have a possible interference with Levodopa absorption.
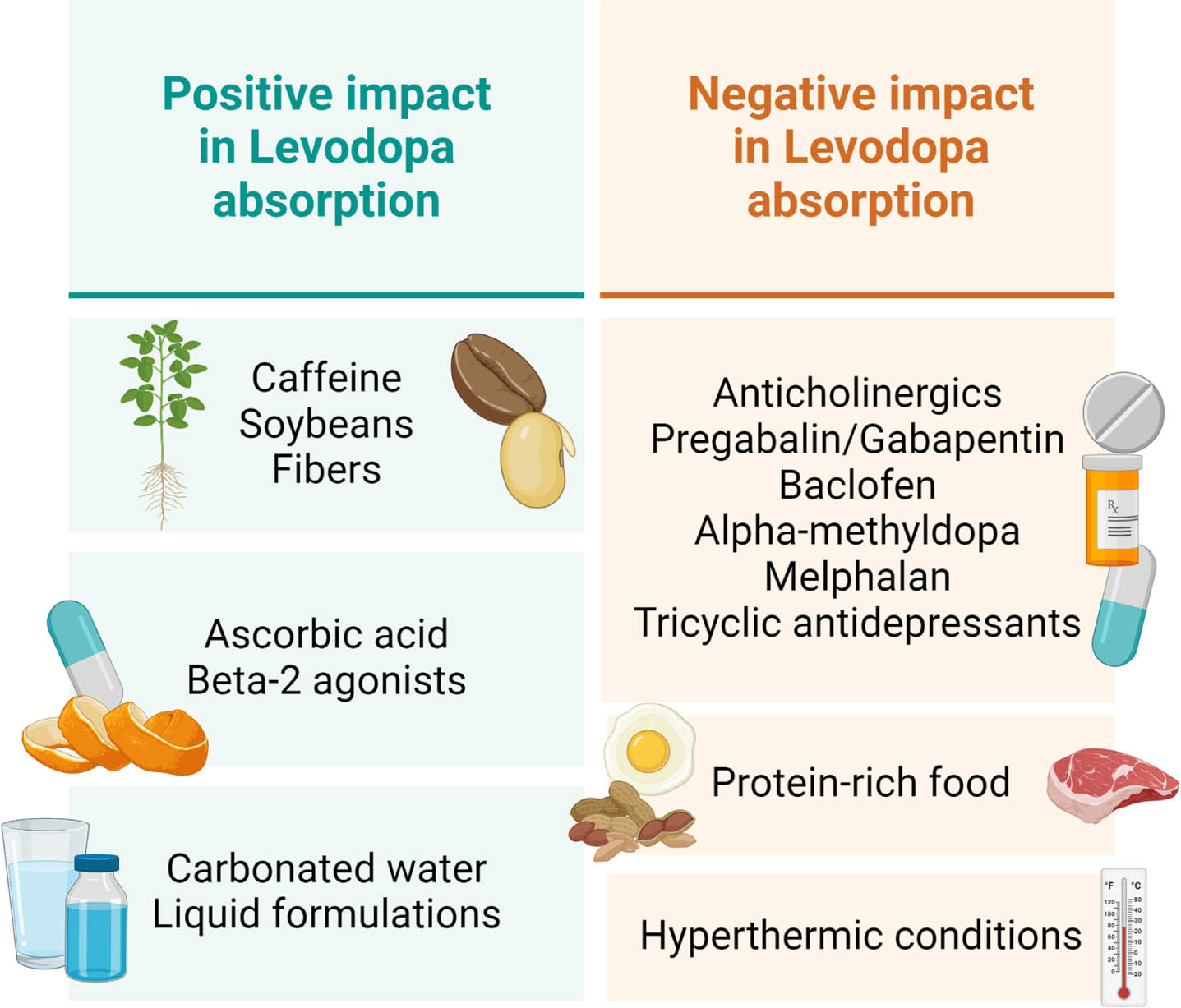
Fig. 4
Main transporters involved in Levodopa absorption and their genetic characteristics.
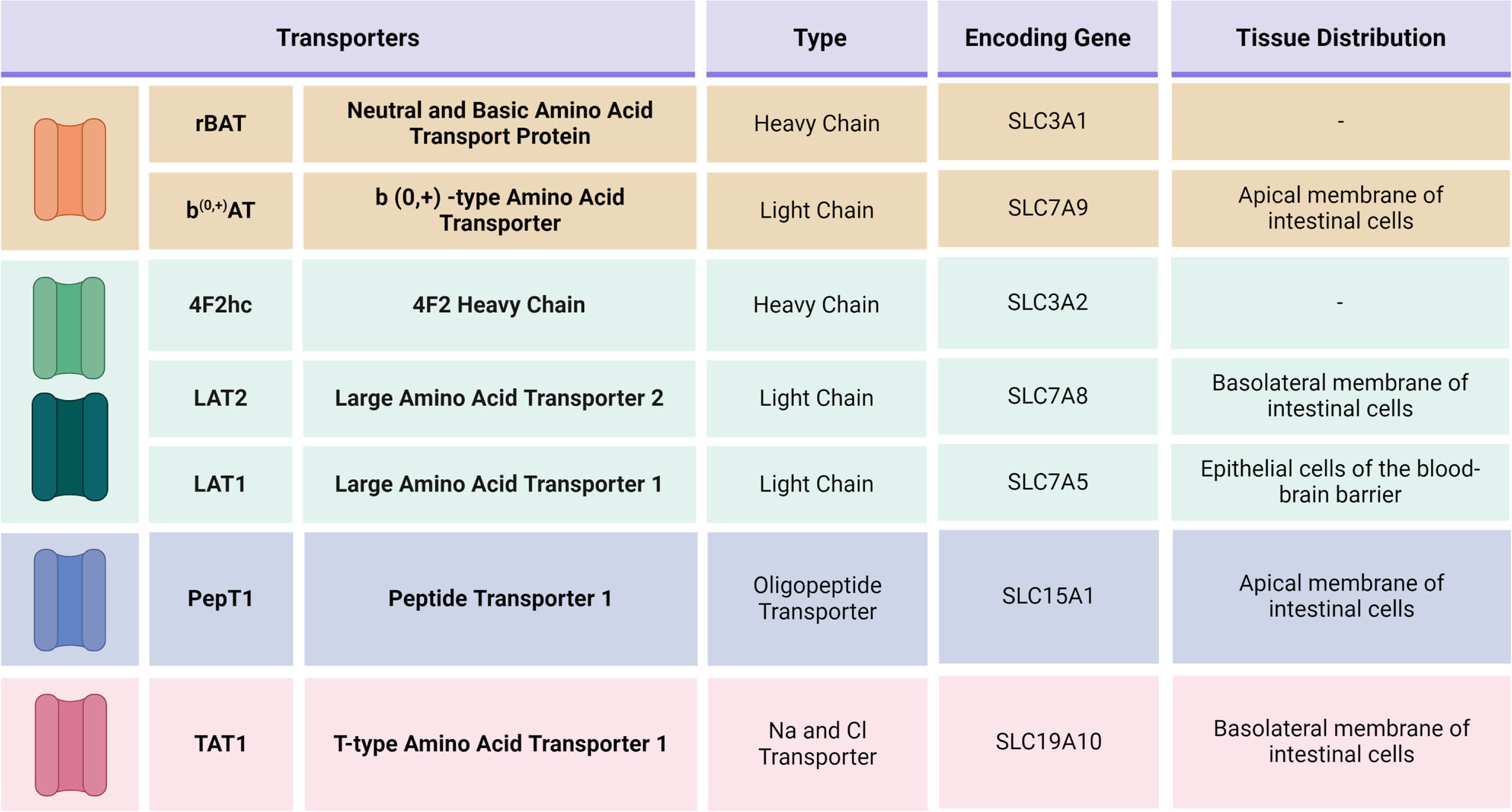
Non-enteral administration of levodopa
Non-oral therapies have been an important topic in the discussion for PD treatment. One such alternative option (which is still dependent on the enteral system) is a pump therapy with intrajejunal levodopa gel, but this route of administration still uses the majority of the same pathways of orally administered levodopa. In addition, there are several routes (i.e. inhaled and subcutaneous levodopa) to bypass the enteral system altogether and, therefore, to avoid transporters such rBAT/b(0, +)AT, LAT2, TAT1, and PEPT1. Some of the dopamine agonists (rotigotine and apomorphine) can also be delivered via a subcutaneous route. These alternate routes of administration are currently still restricted to a minority of patients, in particular those with a fluctuating and erratic response to oral levodopa medication. We will not discuss these alternative routes any further here as the main focus of this review remains to discuss the molecular pathways associated with the oral administration of levodopa. Nonetheless, it is fundamental that the pharmacological non-oral therapies and their molecular features are explored further.136, 137
CONCLUSIONS
Our comprehensive review of the molecular pathways that are associated with the transition of levodopa from the small intestine to the brain has identified a multitude of possibilities through which malabsorption can occur (see Fig. 5). We have also offered several pragmatic recommendations that can be applied in daily clinical practice, while we wait for further scientific evidence to arrive. Our review offers concrete targets for new research studies in this interesting and rapidly developing field.
ACKNOWLEDGMENTS
We would like to thank all authors and reviewers for their contribution in the development and improvement of this article.
FUNDING
This article did not receive any funding.
CONFLICT OF INTEREST
B.R.B. and R.S. are Editorial Board members of this journal, but were not involved in the peer-review process of this article nor they had access to any information regarding its peer review.
REFERENCES
[1] | Abbott A . Levodopa: the story so far. Nature (2010) ; 466: : S6–S7. |
[2] | Leta V , Klingelhoefer L , Longardner K , et al. Gastrointestinal barriers to levodopa transport and absorption in Parkinson’s disease. Eur J Neurol (2023) ; 30: : 1465–1480. |
[3] | Hawkins RA , Mokashi A , Simpson IA. An active transport system in the blood–brain barrier may reduce levodopa availability. Exp Neurol (2005) ; 195: : 267–271. |
[4] | Camargo SMR , Vuille-dit-Bille RN , Mariotta L , et al. The molecular mechanism of intestinal levodopa absorption and its possible implications for the treatment of Parkinson’s disease. J Pharmacol Exp Ther (2014) ; 351: : 114–123. |
[5] | Wu G , Bazer FW , Davis TA , et al. Arginine metabolism and nutrition in growth, health and disease. Amino Acids (2009) ; 37: : 153–168. |
[6] | Vega-Cabello V , Caballero FF , Rodriguez-Artalejo F , et al. Leucine intake and risk of impaired physical function and frailty in older adults. J Gerontol A Biol Sci Med Sci (2023) ; 78: : 241–249. |
[7] | U.S. Department of Agriculture. FoodData Central, https://fdc.nal.usda.gov/index.html ((2024) , accessed 11 January 2024) |
[8] | Piyapolrungroj N , Li C , Bockbrader H , et al. Mucosal uptake of gabapentin (neurontin) vs. pregabalin in the small intestine. Pharm Res (2001) ; 18: : 1126–1130. |
[9] | Nguyen TV , Smith DE , Fleisher D. PEPT1 enhances the uptake of gabapentin via trans-stimulation of b0,+ exchange. Pharm Res (2007) ; 24: : 353–360. |
[10] | Sahota A , Tischfield JA , Goldfarb DS , et al. Cystinuria: genetic aspects, mouse models, and a new approach to therapy. Urolithiasis (2019) ; 47: : 57–66. |
[11] | Wu D , Grund TN , Welsch S , et al. Structural basis for amino acid exchange by a human heteromeric amino acid transporter. Proc Natl Acad Sci U S A (2020) ; 117: : 21281–21287. |
[12] | Kowalczyk NS and Zisman AL. Cystinuria: review of a life-long and frustrating disease. Yale J Biol Med (2021) ; 94: : 681–686. |
[13] | Leclerc D , Boutros M , Suh D , et al. SLC7A9 mutations in all three cystinuria subtypes. Kidney Int (2002) ; 62: : 1550–1559. |
[14] | Hellier MD , Perrett D and Holdsworth CD. Dipeptide absorption in cystinuria. Br Med J (1970) ; 4: : 782–783. |
[15] | Wu KC , Reisman SA and Klaassen CD. Tissue distribution, hormonal regulation, ontogeny, diurnal expression, and induction of mouse cystine transporters Slc3a1 and Slc7a9. Free Radic Res (2020) ; 54: : 525–534. |
[16] | Conti V , Izzo V , Russillo MC , et al. Gender differences in levodopa pharmacokinetics in levodopa-naïve patients with Parkinson’s disease. Front Med (Lausanne) (2022) ; 9: : 909936. |
[17] | Vection S , O’Callaghan D and Keriel A. CD98hc in host-pathogen interactions: roles of the multifunctional host protein during infections. FEMS Microbiol Rev (2022) ; 46: : fuac023. |
[18] | Kantipudi S , Jeckelmann J-M , Ucurum Z , et al. The heavy chain 4F2hc modulates the substrate affinity and specificity of the light chains LAT1 and LAT2. Int J Mol Sci (2020) ; 21: : 7573. |
[19] | Feral CC , Nishiya N , Fenczik CA , et al. CD98hc (SLC3A2) mediates integrin signaling. Proc Natl Acad Sci U S A (2005) ; 102: : 355–360. |
[20] | Kantipudi S and Fotiadis D. Yeast cell-based transport assay for the functional characterization of human 4F2hc-LAT1 and -LAT2, and LAT1 and LAT2 substrates and inhibitors. Front Mol Biosci (2021) ; 8: : 676854. |
[21] | He J , Liu D , Liu M , et al. Characterizing the role of SLC3A2 in the molecular landscape and immune microenvironment across human tumors. Front Mol Biosci (2022) ; 9: : 961410. |
[22] | Puris E , Gynther M , Auriola S , et al. L-Type amino acid transporter 1 as a target for drug delivery. Pharm Res (2020) ; 37: : 88. |
[23] | del Amo EM , Urtti A and Yliperttula M. Pharmacokinetic role of L-type amino acid transporters LAT1 and LAT2. Eur J Pharm Sci (2008) ; 35: : 161–174. |
[24] | Rossier G , Meier C , Bauch C , et al. LAT2, a new basolateral 4F2hc/CD98-associated amino acid transporter of kidney and intestine. J Biol Chem (1999) ; 274: : 34948–34954. |
[25] | Braun D , Wirth EK , Wohlgemuth F , et al. Aminoaciduria, but normal thyroid hormone levels and signalling, in mice lacking the amino acid and thyroid hormone transporter Slc7a8. Biochem J (2011) ; 439: : 249–255. |
[26] | Soares-Da-Silva P , Serrão MP , Pinho MJ , et al. Cloning and gene silencing of LAT2, the L-3,4-dihydroxyphenylalanine (L-DOPA) transporter, in pig renal LLC-PK1 epithelial cells. FASEB J (2004) ; 18: : 1489–1498. |
[27] | Pinho MJ , Serrão MP , Gomes P , et al. Over-expression of renal LAT1 and LAT2 and enhanced L-DOPA uptake in SHR immortalized renal proximal tubular cells. Kidney Int (2004) ; 66: : 216–226. |
[28] | Soares-da-Silva P and Serrão MP. High- and low-affinity transport of L-leucine and L-DOPA by the hetero amino acid exchangers LAT1 and LAT2 in LLC-PK1 renal cells. Am J Physiol Renal Physiol (2004) ; 287: : F252–F261. |
[29] | Fraga S , Serrão MP and Soares-da-Silva P. The L-3,4-dihydroxyphenylalanine transporter in human and rat epithelial intestinal cells is a type 2 hetero amino acid exchanger. Eur J Pharmacol (2002) ; 441: : 127–135. |
[30] | Larsen MS , Frølund S , Nøhr MK , et al. In vivo and in vitro evaluations of intestinal gabapentin absorption: effect of dose and inhibitors on carrier-mediated transport. Pharm Res (2015) ; 32: : 898–909. |
[31] | Stewart BH , Kugler AR , Thompson PR , et al. A saturable transport mechanism in the intestinal absorption of gabapentin is the underlying cause of the lack of proportionality between increasing dose and drug levels in plasma. Pharm Res (1993) ; 10: : 276–281. |
[32] | Su TZ , Feng MR and Weber ML. Mediation of highly concentrative uptake of pregabalin by L-type amino acid transport in Chinese hamster ovary and Caco-2 cells. J Pharmacol Exp Ther (2005) ; 313: : 1406–1415. |
[33] | Prasad PD , Leibach FH , Mahesh VB , et al. Relationship between thyroid hormone transport and neutral amino acid transport in JAR human choriocarcinoma cells. Endocrinology (1994) ; 134: : 574–581. |
[34] | Kelderman-Bolk N . Intestinal absorption of thyroid hormone. Thesis, Erasmus University, Rotterdam, (2015) . |
[35] | Zevenbergen C , Meima ME , Lima de Souza EC , et al. Transport of iodothyronines by human L-type amino acid transporters. Endocrinology (2015) ; 156: : 4345–4355. |
[36] | Hinz KM , Meyer K , Kinne A , et al. Structural insights into thyroid hormone transport mechanisms of the L-type amino acid transporter 2. Mol Endocrinol (2015) ; 29: : 933–942. |
[37] | Espino Guarch M , Font-Llitjós M , Murillo-Cuesta S , et al. Mutations in L-type amino acid transporter-2 support SLC7A8 as a novel gene involved in age-related hearing loss. Elife (2018) ; 7: : e31511. |
[38] | Knöpfel EB , Vilches C , Camargo SMR , et al. Dysfunctional LAT2 amino acid transporter is associated with cataract in mouse and humans. Front Physiol (2019) ; 10: : 688. |
[39] | Kyaw WT , Nagai M , Kaneta M , et al. Effect of nicotine on the pharmacokinetics of levodopa. Clin Neuropharmacol (2013) ; 36: : 46–51. |
[40] | Lu JYD , Su P , Barber JEM , et al. The neuroprotective effect of nicotine in Parkinson’s disease models is associated with inhibiting PARP-1 and caspase-3 cleavage. PeerJ (2017) ; 5: : e3933. |
[41] | Quik M , Perez XA and Bordia T. Nicotine as a potential neuroprotective agent for Parkinson’s disease. Mov Disord (2012) ; 27: : 947–957. |
[42] | Quik M , Mallela A , Ly J , et al. Nicotine reduces established levodopa-induced dyskinesias in a monkey model of Parkinson’s disease. Mov Disord (2013) ; 28: : 1398–1406. |
[43] | Ma C , Liu Y , Neumann S , et al. Nicotine from cigarette smoking and diet and Parkinson disease: a review. Transl Neurodegener (2017) ; 6: : 18. |
[44] | Kanai Y , Segawa H , Miyamoto KI , et al. Expression cloning and characterization of a transporter for large neutral amino acids activated by the heavy chain of 4F2 antigen (CD98). J Biol Chem (1998) ; 273: : 23629–23632. |
[45] | Yanagida O , Kanai Y , Chairoungdua A , et al. Human L-type amino acid transporter 1 (LAT1): characterization of function and expression in tumor cell lines. Biochim Biophys Acta (2001) ; 1514: : 291–302. |
[46] | Kageyama T , Nakamura M , Matsuo A , et al. The 4F2hc/LAT1 complex transports L-DOPA across the blood-brain barrier. Brain Res (2000) ; 879: : 115–121. |
[47] | Uchino H , Kanai Y , Kim DK , et al. Transport of amino acid-related compounds mediated by L-type amino acid transporter 1 (LAT1): insights into the mechanisms of substrate recognition. Mol Pharmacol (2002) ; 61: : 729–737. |
[48] | van Bree JB , Audus KL and Borchardt RT. Carrier-mediated transport of baclofen across monolayers of bovine brain endothelial cells in primary culture. Pharm Res (1988) ; 5: : 369–371. |
[49] | Goldenberg GJ , Lam HY and Begleiter A. Active carrier-mediated transport of melphalan by two separate amino acid transport systems in LPC-1 plasmacytoma cells in vitro. J Biol Chem (1979) ; 254: : 1057–1064. |
[50] | Dickens D , Webb SD , Antonyuk S , et al. Transport of gabapentin by LAT1 (SLC7A5). Biochem Pharmacol (2013) ; 85: : 1672–1683. |
[51] | Takahashi Y , Nishimura T , Higuchi K , et al. Transport of pregabalin via L-type amino acid transporter 1 (SLC7A5) in human brain capillary endothelial cell line. Pharm Res (2018) ; 35: : 246. |
[52] | Kanai Y . Amino acid transporter LAT1 (SLC7A5) as a molecular target for cancer diagnosis and therapeutics. Pharmacol Ther (2022) ; 230: : 107964. |
[53] | Macotela Y , Emanuelli B , Bång AM , et al. Dietary leucine–an environmental modifier of insulin resistance acting on multiple levels of metabolism. PLoS One (2011) ; 6: : e21187. |
[54] | Zhang J , Xu Y , Li D , et al. Review of the correlation of LAT1 with diseases: mechanism and treatment. Front Chem (2020) ; 8: : 564809. |
[55] | Al-Otaish H , Al-Ayadhi L , Bjørklund G , et al. Relationship between absolute and relative ratios of glutamate, glutamine and GABA and severity of autism spectrum disorder. Metab Brain Dis (2018) ; 33: : 843–854. |
[56] | Ohtsuki S , Yamaguchi H , Kang Y-S , et al. Reduction of L-type amino acid transporter 1 mRNA expression in brain capillaries in a mouse model of Parkinson’s disease. Biol Pharm Bull (2010) ; 33: : 1250–1252. |
[57] | Latif S , Kang Y-S . Blood-brain barrier solute carrier transporters and motor neuron disease. Pharmaceutics (2022) ; 14: : 2167. |
[58] | Tărlungeanu DC , Deliu E , Dotter CP , et al. Impaired amino acid transport at the blood brain barrier is a cause of autism spectrum disorder. Cell (2016) ; 167: : 1481–1494.e18. |
[59] | Friedman M . Analysis, nutrition, and health benefits of tryptophan. Int J Tryptophan Res (2018) ; 11: : 1178646918802282. |
[60] | Richard DM , Dawes MA , Mathias CW , et al. L-tryptophan: basic metabolic functions, behavioral research and therapeutic indications. Int J Tryptophan Res (2009) ; 2: : 45–60. |
[61] | Choi Y , Huh E , Lee S , et al. 5-hydroxytryptophan reduces levodopa-induced dyskinesia via regulating AKT/mTOR/S6K and CREB/ΔFosB signals in a mouse model of Parkinson’s disease. Biomol Ther (Seoul) (2023) ; 31: : 402–410. |
[62] | Riederer P . L-dopa competes with tyrosine and tryptophan for human brain uptake. Nutr Metab (1980) ; 24: : 417–423. |
[63] | Allikmets LH and Zharkovsky AM. Biochemical and behavioural studies on the interference between catecholaminergic and serotonergic central systems. Ann Ist Super Sanita (1978) ; 14: : 63–70. |
[64] | Okuno A , Fukuwatari T and Shibata K. High tryptophan diet reduces extracellular dopamine release via kynurenic acid production in rat striatum. J Neurochem (2011) ; 118: : 796–805. |
[65] | Taylor PM and Ritchie JWA. Tissue uptake of thyroid hormone by amino acid transporters. Best Pract Res Clin Endocrinol Metab (2007) ; 21: : 237–251. |
[66] | Friesema EC , Docter R , Moerings EP , et al. Thyroid hormone transport by the heterodimeric human system L amino acid transporter. Endocrinology (2001) ; 142: : 4339–4348. |
[67] | Wingert TD and Hershman JM. Sinemet and thyroid function in Parkinson disease. Neurology (1979) ; 29: : 1073–1074. |
[68] | Charoenngam N , Rittiphairoj T , Ponvilawan B , et al. Thyroid dysfunction and risk of Parkinson’s disease: a systematic review and meta-analysis. Front Endocrinol (Lausanne) (2022) ; 13: : 863281. |
[69] | Uc EY , Dienel GA , Cruz NF , et al. β-Adrenergics enhance brain extraction of levodopa. Mov Disord (2002) ; 17: : 54–59. |
[70] | Eriksson T and Carlsson A. Beta-adrenergic control of brain uptake of large neutral amino acids. Life Sci (1988) ; 42: : 1583–1589. |
[71] | Takao Y , Kamisaki Y and Itoh T. Beta-adrenergic regulation of amine precursor amino acid transport across the blood-brain barrier. Eur J Pharmacol (1992) ; 215: : 245–251. |
[72] | Alexander GM , Schwartzman RJ , Nukes TA , et al. Beta 2-adrenergic agonist as adjunct therapy to levodopa in Parkinson’s disease. Neurology (1994) ; 44: : 1511–1513. |
[73] | Uc EY , Lambert CP , Harik SI , et al. Albuterol improves response to levodopa and increases skeletal muscle mass in patients with fluctuating Parkinson disease. Clin Neuropharmacol (2003) ; 26: : 207–212. |
[74] | Daniel H and Kottra G. The proton oligopeptide cotransporter family SLC15 in physiology and pharmacology. Pflugers Arch (2004) ; 447: : 610–618. |
[75] | Spanier B . Transcriptional and functional regulation of the intestinal peptide transporter PEPT1. J Physiol (2014) ; 592: : 871–879. |
[76] | Tamai I , Nakanishi T , Hayashi K , et al. The predominant contribution of oligopeptide transporter PepT1 to intestinal absorption of beta-lactam antibiotics in the rat small intestine. J Pharm Pharmacol (1997) ; 49: : 796–801. |
[77] | Zhang Y , Sun J , Sun Y , et al. Prodrug design targeting intestinal PepT1 for improved oral absorption: design and performance. Curr Drug Metab (2013) ; 14: : 675–687. |
[78] | Nakanishi T , Tamai I , Takaki A , et al. Cancer cell-targeted drug delivery utilizing oligopeptide transport activity. Int J Cancer (2000) ; 88: : 274–280. |
[79] | Brandsch M . Drug transport via the intestinal peptide transporter PepT1. Curr Opin Pharmacol (2013) ; 13: : 881–887. |
[80] | Wang C-Y , Liu S , Xie X-N , et al. Regulation profile of the intestinal peptide transporter 1 (PepT1). Drug Des Devel Ther (2017) ; 11: : 3511–3517. |
[81] | Thamotharan M , Bawani SZ , Zhou X , et al. Hormonal regulation of oligopeptide transporter pept-1 in a human intestinal cell line. Am J Physiol (1999) ; 276: : C821–C826. |
[82] | Adibi SA . Regulation of expression of the intestinal oligopeptide transporter (Pept-1) in health and disease. Am J Physiol Gastrointest Liver Physiol (2003) ; 285: : G779–G788. |
[83] | Pavlova I , Milanova A , Danova S , et al. Enrofloxacin and probiotic lactobacilli influence PepT1 and LEAP-2 mRNA expression in poultry. Probiotics Antimicrob Proteins (2016) ; 8: : 215–220. |
[84] | Guo S , Liu D , Zhao X , et al. Xylanase supplementation of a wheat-based diet improved nutrient digestion and mRNA expression of intestinal nutrient transporters in broiler chickens infected with Clostridium perfringens. Poult Sci (2014) ; 93: : 94–103. |
[85] | Kouodom MN , Ronconi L , Celegato M , et al. Toward the selective delivery of chemotherapeutics into tumor cells by targeting peptide transporters: tailored gold-based anticancer peptidomimetics. J Med Chem (2012) ; 55: : 2212–2226. |
[86] | Wang C-L , Fan Y-B , Lu H-H , et al. Evidence of D-phenylglycine as delivering tool for improving L-dopa absorption. J Biomed Sci (2010) ; 17: : 71. |
[87] | Woodard LE , Welch RC , Veach RA , et al. Metabolic consequences of cystinuria. BMC Nephrol (2019) ; 20: : 227. |
[88] | Nässl A-M , Rubio-Aliaga I , Fenselau H , et al. Amino acid absorption and homeostasis in mice lacking the intestinal peptide transporter PEPT1. Am J Physiol Gastrointest Liver Physiol (2011) ; 301: : G128–G137. |
[89] | Kim DK , Kanai Y , Matsuo H , et al. The human T-type amino acid transporter-1: characterization, gene organization, and chromosomal location. Genomics (2002) ; 79: : 95–103. |
[90] | Vilches C , Boiadjieva-Knöpfel E , Bodoy S , et al. Cooperation of antiporter LAT2/CD98hc with uniporter TAT1 for renal reabsorption of neutral amino acids. J Am Soc Nephrol (2018) ; 29: : 1624–1635. |
[91] | Ramadan T , Camargo SMR , Herzog B , et al. Recycling of aromatic amino acids via TAT1 allows efflux of neutral amino acids via LAT2-4F2hc exchanger. Pflugers Arch (2007) ; 454: : 507–516. |
[92] | Kim DK , Kanai Y , Chairoungdua A , et al. Expression cloning of a Na+-independent aromatic amino acid transporter with structural similarity to H+/monocarboxylate transporters. J Biol Chem (2001) ; 276: : 17221–17228. |
[93] | Mariotta L , Ramadan T , Singer D , et al. T-type amino acid transporter TAT1 (Slc16a10) is essential for extracellular aromatic amino acid homeostasis control. J Physiol (2012) ; 590: : 6413–6424. |
[94] | Habashy WS , Milfort MC , Adomako K , et al. Effect of heat stress on amino acid digestibility and transporters in meat-type chickens. Poult Sci (2017) ; 96: : 2312–2319. |
[95] | Yang S , Zhang C , Xu W , et al. Heat stress decreases intestinal physiological function and facilitates the proliferation of harmful intestinal microbiota in sturgeons. Front Microbiol (2022) ; 13: : 755369. |
[96] | Dokladny K , Zuhl MN and Moseley PL. Intestinal epithelial barrier function and tight junction proteins with heat and exercise. J Appl Physiol (1985) (2016) ; 120: : 692–701. |
[97] | Sarwari AR and Mackowiak PA. The pharmacologic consequences of fever. Infect Dis Clin North Am (1996) ; 10: : 21–32. |
[98] | Ballard BE. Pharmacokinetics and temperature. J Pharm Sci (1974) ; 63: : 1345–1358. |
[99] | van Wamelen DJ , Podlewska AM , Leta V , et al. Slave to the rhythm: Seasonal differences in non-motor symptoms in Parkinson’s disease. Parkinsonism Relat Disord (2019) ; 63: : 73–76. |
[100] | Janssen Daalen JM , Hubbers J , Sharifi Bonab M , et al. How vacations affect Parkinson’s disease. Mov Disord Clin Pract (2023) ; 10: : 151–153. |
[101] | Rowell D , Nghiem S , Ramagopalan S , et al. Seasonal temperature is associated with Parkinson’s disease prescriptions: an ecological study. Int J Biometeorol (2017) ; 61: : 2205–2211. |
[102] | Bougea A , Papagiannakis N , Simitsi A-M , et al. Ambiental factors in Parkinson’s disease progression: a systematic review. Medicina (Kaunas) (2023) ; 59: : 294. |
[103] | Morgan JP , Rivera-Calimlim L , Messiha F , et al. Imipramine-mediated interference with levodopa absorption from the gastrointestinal tract in man. Neurology (1975) ; 25: : 1029–1034. |
[104] | Messiha FS and Morgan JP. Imipramine-mediated effects on levodopa metabolism in man. Biochem Pharmacol (1974) ; 23: : 1503–1507. |
[105] | Berchou RC . Maximizing the benefit of pharmacotherapy in Parkinson’s disease. Pharmacotherapy (2000) ; 20: : 33S–42S. |
[106] | Bianchine JR and Sunyapridakul L. Interactions between levodopa and other drugs: significance in the treatment of Parkinson’s disease. Drugs (1973) ; 6: : 364–388. |
[107] | Conti MM , Goldenberg AAA , Kuberka A , et al. Effect of tricyclic antidepressants on L-DOPA-induced dyskinesia and motor improvement in hemi-parkinsonian rats. Pharmacol Biochem Behav (2016) ; 142: : 64–71. |
[108] | Kamińska K , Lenda T , Konieczny J , et al. Interactions of the tricyclic antidepressant drug amitriptyline with L-DOPA in the striatum and substantia nigra of unilaterally 6-OHDA-lesioned rats. Relevance to motor dysfunction in Parkinson’s disease. Neurochem Int (2018) ; 121: : 125–139. |
[109] | Nag M . Effect of chlorpromazine, imipramine and lithium on MAO-A and MAO-B activity in rat brain mitochondria. Indian J Exp Biol (2004) ; 42: : 941–944. |
[110] | Tan Y-Y , Jenner P and Chen S-D. Monoamine oxidase-B inhibitors for the treatment of Parkinson’s disease: past, present, and future. J Parkinsons Dis (2022) ; 12: : 477–493. |
[111] | Campbell NR, Hasinoff B. Ferrous sulfate reduces levodopa bioavailability: chelation as a possible mechanism. Clin Pharmacol Ther (1989) ; 45: : 220–225. |
[112] | Van Blercom N , Lasa A , Verger K , et al. Effects of gabapentin on the motor response to levodopa: a double-blind, placebo-controlled, crossover study in patients with complicated Parkinson disease. Clin Neuropharmacol (2004) ; 27: : 124–128. |
[113] | Gidal BE , Maly MM , Budde J , et al. Effect of a high-protein meal on gabapentin pharmacokinetics. Epilepsy Res (1996) ; 23: : 71–76. |
[114] | Benetello P , Furlanut M , Fortunato M , et al. Oral gabapentin disposition in patients with epilepsy after a high-protein meal. Epilepsia (1997) ; 38: : 1140–1142. |
[115] | Johansson ME , Cameron IGM , Van der Kolk NM , et al. Aerobic exercise alters brain function and structure in Parkinson’s disease: a randomized controlled trial. Ann Neurol (2022) ; 91: : 203–216. |
[116] | Lian P , Braber S , Garssen J , et al. Beyond heat stress: intestinal integrity disruption and mechanism-based intervention strategies. Nutrients (2020) ; 12: : 734. |
[117] | Nehlig A , Daval JL and Debry G. Caffeine and the central nervous system: mechanisms of action, biochemical, metabolic and psychostimulant effects. Brain Res Brain Res Rev (1992) ; 17: : 139–170. |
[118] | Negida A , Elfil M , Attia , et al. Caffeine; the forgotten potential for Parkinson’s disease. CNS Neurol Disord Drug Targets (2017) ; 16: : 652–657. |
[119] | Deleu D , Jacob P , Chand P , et al. Effects of caffeine on levodopa pharmacokinetics and pharmacodynamics in Parkinson disease. Neurology (2006) ; 67: : 897–899. |
[120] | Mori A , Chen J-F , Uchida S , et al. The pharmacological potential of adenosine A2A receptor antagonists for treating Parkinson’s disease. Molecules (2022) ; 27: : 2366. |
[121] | Fernández-Dueñas V , Gómez-Soler M , López-Cano M , et al. Uncovering caffeine’s adenosine A2A receptor inverse agonism in experimental parkinsonism. ACS Chem Biol (2014) ; 9: : 2496–2501. |
[122] | Moretti M , Fraga DB and Rodrigues ALS. Preventive and therapeutic potential of ascorbic acid in neurodegenerative diseases. CNS Neurosci Ther (2017) ; 23: : 921–929. |
[123] | Miyaue N , Kubo M and Nagai M. Ascorbic acid can alleviate the degradation of levodopa and carbidopa induced by magnesium oxide. Brain Behav (2022) ; 12: : e2672. |
[124] | Kashihara Y , Terao Y , Yoda K , et al. Effects of magnesium oxide on pharmacokinetics of L-dopa/carbidopa and assessment of pharmacodynamic changes by a model-based simulation. Eur J Clin Pharmacol (2019) ; 75: : 351–361. |
[125] | Nagayama H , Hamamoto M , Ueda M , et al. The effect of ascorbic acid on the pharmacokinetics of levodopa in elderly patients with Parkinson disease. Clin Neuropharmacol (2004) ; 27: : 270–273. |
[126] | Yang H-J , Ehm G , Kim YE , et al. Liquid levodopa-carbidopa in advanced Parkinson’s disease with motor complications. J Neurol Sci (2017) ; 377: : 6–11. |
[127] | Stocchi F , Vacca L , Grassini P , et al. L-dopa pharmacokinetic profile with effervescent melevodopa/carbidopa versus standard-release levodopa/carbidopa tablets in Parkinson’s disease: a randomised study. Parkinsons Dis (2015) ; 2015: : 369465. |
[128] | Fasano A , Bove F , Gabrielli M , et al. . Liquid melevodopa versus standard levodopa in patients with Parkinson disease and small intestinal bacterial overgrowth. Clin Neuropharmacol (2014) ; 37: : 91–95. |
[129] | Zangaglia R , Stocchi F , Sciarretta M , et al. Clinical experiences with levodopa methylester (melevodopa) in patients with Parkinson disease experiencing motor fluctuations: an open-label observational study. Clin Neuropharmacol (2010) ; 33: : 61–66. |
[130] | Nausieda PA , Pfeiffer RF , Tagliati M , et al. A multicenter, open-label, sequential study comparing preferences for carbidopa-levodopa orally disintegrating tablets and conventional tablets in subjects with Parkinson’s disease. Clin Ther (2005) ; 27: : 58–63. |
[131] | Ahlskog JE . The New Parkinson’s Disease Treatment Book: Partnering with Your Doctor to Get the Most from Your Medications. Oxford University Press, (2015) . |
[132] | Astarloa R , Mena MA , Sánchez V , et al. Clinical and pharmacokinetic effects of a diet rich in insoluble fiber on Parkinson disease. Clin Neuropharmacol (1992) ; 15: : 375–380. |
[133] | Boelens Keun JT , Arnoldussen IA , Vriend C , et al. Dietary approaches to improve efficacy and control side effects of levodopa therapy in Parkinson’s disease: a systematic review. Adv Nutr (2021) ; 12: : 2265–2287. |
[134] | Nagashima Y , Kondo T , Sakata M , et al. Effects of soybean ingestion on pharmacokinetics of levodopa and motor symptoms of Parkinson’s disease–In relation to the effects of Mucuna pruriens. J Neurol Sci (2016) ; 361: : 229–234. |
[135] | Magistrelli L and Comi C. Beta2-adrenoceptor agonists in Parkinson’s disease and other synucleinopathies. J Neuroimmune Pharmacol (2020) ; 15: : 74–81. |
[136] | van Laar T and Borgemeester R. The need for non-oral therapy in Parkinson’s disease; a potential role for apomorphine. Parkinsonism Relat Disord (2016) ; 33: (Suppl 1): S22–S27. |
[137] | Olanow CW , Espay AJ , Stocchi F , et al. Continuous subcutaneous levodopa delivery for Parkinson’s disease: a randomized study. J Parkinsons Dis (2021) ; 11: : 177–186. |