Gait Analysis with Wearable Sensors in Isolated REM Sleep Behavior Disorder Associated with Phenoconversion: An Explorative Study
Abstract
Background:
Gait disturbance is a vital characteristic of motor manifestation in α– synucleinopathies, especially Parkinson’s disease. Subtle gait alterations are present in isolated rapid eye movement sleep behavior disorder (iRBD) patients before phenoconversion; it is yet unclear, if gait analysis may predict phenoconversion.
Objective:
To investigate subtle gait alterations and explore whether gait analysis using wearable sensors is associated with phenoconversion of iRBD to α-synucleinopathies.
Methods:
Thirty-one polysomnography-confirmed iRBD patients and 33 healthy controls (HCs) were enrolled at baseline. All participants walked for a minute while wearing 6 inertial sensors on bilateral wrists, ankles, and the trunk (sternal and lumbar region). Three conditions were tested: (i) normal walking, (ii) fast walking, and (iii) dual-task walking.
Results:
Decreased arm range of motion and increased gait variation (stride length, stride time and stride velocity) discriminate converters from HCs at baseline. After an average of 5.40 years of follow-up, 10 patients converted to neurodegenerative diseases (converters). Cox regression analysis showed higher value of stride length asymmetry under normal walking condition to be associated with an early conversion of iRBD to α– synucleinopathies (adjusted HR 4.468, 95% CI 1.088– 18.349, p = 0.038).
Conclusions:
Stride length asymmetry is associated with progression to α– synucleinopathies in patients with iRBD. Gait analysis with wearable sensors may be useful for screening, monitoring, and risk stratification for disease-modifying therapy trials in patients with iRBD.
INTRODUCTION
Rapid-eye-movement (REM) sleep behavior disorder (RBD) has been identified as one of the earliest and most distinct prodromal signs of the α– synucleinopathies [1]. According to longitudinal studies, up to 80% of patients with isolated RBD (iRBD) go on to be diagnosed with Parkinson’s disease (PD), dementia with Lewy bodies (DLB), or multiple system atrophy (MSA) over the course of 5–29 years [2, 3]. Older people are largely affected by these disorders, in particular PD. With the aging of population worldwide, and an absence of absolute cure for these conditions, early identification and preventive approaches appear to be more meaningful [4].
The development of reliable markers for screening iRBD and predicting disease phenoconversion is essential. Such markers will enable us to implement preventive strategies and at the same time would be beneficial for clinical trials to investigate novel disease-modifying therapies. As it is well-known, gait disturbance plays a vital role in the motor manifestation of α– synucleinopathies, especially PD. Gait alterations is considered as an appealing prodromal marker of PD [5]. Emerging instrumental gait analysis technologies, for example, inertial sensors and pressure-sensitive carpets are relatively low-cost tools and sensitive, and allow a continuous assessment of subtle gait abnormalities, thus enabling researchers to efficiently capture minimal gait alterations.
Several cross-sectional studies have demonstrated that subtle gait alterations are present in the prodromal stage before phenoconversion. For example, a previous study using GAITRite gait analysis system indicated that a decreased velocity, cadence, significantly increased double limb support variability, greater stride time variability, and swing time variability in patients with probable RBD [6]. In another recent study, greater foot step variability and asymmetry have been found during dual-task walking in patients with iRBD compared to controls, suggesting an overlap between motor and cognitive domains [7]. In addition, compared with controls and PD non-freezers, poor performance in the coupling of posture and gait initiation has also been shown in patients with iRBD [8]. These alterations negatively correlated with the amount of REM sleep without atonia, reflecting a connection between REM sleep and locomotion domain [8]. Furthermore, decreased gait speed, cadence, and step variability have been reported in patients with iRBD, compared with age-matched controls while performing home-based spontaneous walking tasks [9]. A multi-class model using statistical learning from a probability distribution of gait parameters was highly effective in discriminating patients with iRBD, patients with PD, and healthy controls, with a sensitivity of 100% and specificity of 90%, highlighting the potential of using gait parameters as prognostic and monitoring markers [10].
To our knowledge, the potential of gait analysis to predict progression to α– synucleinopathies in patients with iRBD has not yet been investigated longitudinally. In this study, we examined the longitudinal association of gait alterations using wearable sensors with iRBD phenoconversion to α-synucleinopathies.
METHODS
Participants
Thirty-one iRBD patients, and 33 healthy control subjects (HCs) were recruited in the Department of Neurology at Xuanwu Hospital Capital Medical University between October 2012 and January 2017. The diagnosis of iRBD was confirmed by video-polysomnography (vPSG) performed in the hospital sleep laboratory according to the standard International Classification of Sleep Disorders criteria (ICSD-III) [11]. Clinical evaluation was performed to rule out secondary RBD, including other neurodegenerative diseases, narcolepsy, structural lesions in the brain stem, and drug-induced RBD. HCs with possible RBD symptoms were excluded via clinical evaluation using the RBD Questionnaire Hong Kong (RBDQ-HK, total score≤18) [12]. Participants with severe mobility difficulty due to orthopedic diseases or other neurological disorders were excluded. All participants underwent neurological evaluation for dementia or parkinsonism at enrollment and follow-up. The study was approved by the research ethics board of Xuanwu Hospital, and written informed consent was obtained from all participants.
Baseline clinical assessments and gait analysis
All participants underwent a thorough clinical and neurological examination, using the Unified Parkinson’s Disease Rating Scale (UPDRS), Mini-Mental State Examination (MMSE), and Montreal Cognitive Assessment (MoCA) at baseline status. The body mass index (BMI) was calculated as weight (kg)/height (m2). In our study, only 3 patients (9.7%) were taking clonazepam at baseline status. These patients were asked to discontinue clonazepam 24 h before the gait examination. All participants were asked to walk along a 10-m-long corridor back and forth for 1 minute. Three conditions were tested: (i) normal walking: walking at normal pace, (ii) fast walking: walking at fast pace, and (iii) dual-task walking: walking at a normal pace while continuously subtracting 3 s from 100.
Six wearable sensors (128 Hz; MobilityLab; APDM Inc., Portland, OR, USA) were worn on the bilateral wrists and ankles, and the trunk (sternal and lumbar region) during all walking conditions. Gyroscopes are used to detect temporal gait measures and range of motion. Spatial gait measures are estimated using a biomechanical model [13]. Gait parameters were extracted from both sides to obtain gait measures for each gait cycle of the left and right feet separately. Extracted gait parameters were grouped into independent domains: (i) Pace: stride length, stride velocity, swing variation; (ii) Rhythm: stride time, swing, stance; (iii) Variability: stride time variation, stride velocity variation, stride length variation; (iv) Asymmetry: swing asymmetry, stance asymmetry; (v) Postural control: stride length asymmetry; (vi) Arm: arm range of motion, peak arm velocity, arm range of motion variation, peak arm velocity asymmetry; (vii) Trunk: trunk sagittal range of motion, trunk horizontal range of motion, peak trunk sagittal velocity, peak trunk horizontal velocity. Coefficient of variation was calculated as 100×standard deviation / mean value (average of the left and right sides). Here we list the explanation of each parameter involved in this study as follows:
• Stride length: Distance between a gait cycle. Average of the left and right sides.
• Stride velocity: Walking speed. Average of the left and right sides.
• Stride time: Duration of a complete gait cycle.
• Swing: Average percentage of a gait cycle that either foot is off the ground.
• Stance: Average percentage of a gait cycle that either foot is on the ground.
• Stride length asymmetry: Mean asymmetry of the left and right stride length.
• Stride velocity asymmetry: Mean asymmetry of the left and right stride velocity.
• Swing asymmetry: Mean asymmetry of the left and right swing.
• Stance asymmetry: Mean asymmetry of the left and right stance.
• Peak Arm velocity: Peak angular velocity of arms. Average of the left and right sides.
• Arm range of motion: Range of motion of arms during arm-swing. Average of the left and right sides.
• Peak arm velocity asymmetry: Mean asymmetry of the left and right arm swing velocity.
• Trunk sagittal range of motion: Range of motion of trunk in sagittal planes.
• Trunk horizontal range of motion: Range of motion of trunk in horizontal planes.
• Trunk sagittal peak velocity: Peak angular velocity of trunk in sagittal plane.
• Trunk horizontal peak velocity: Peak angular velocity of trunk in horizontal plane.
Follow-up visits
The iRBD patients in our cohort were prospectively followed via in-person evaluations performed by an experienced movement disorder specialist to monitor disease phenoconversion. All patients with iRBD were followed annually or whenever they reported complaints of cognitive, motor, or autonomic function. Full clinical assessments were performed in each follow-up visit. Phenoconversion was noted upon the occurrence of parkinsonism or dementia. The final diagnosis and the date of the last visit were recorded. Parkinsonism was defined as presence of bradykinesia plus either rigidity or rest tremor. PD diagnosis was made by the Movement Disorder Society (MDS) clinical diagnostic criteria for PD [14]. MSA was diagnosed based on the 2008 criteria for probable MSA [15]. For patients presenting with dementia, a diagnosis of probable DLB were made according to the 2017 fourth consensus report of the DLB Consortium criteria [16]. De novo mild cognitive impairment was not considered as phenoconversion in our study. All follow-up information was used to make the differential diagnosis (i.e., a patient initially diagnosed with parkinsonism probably secondary to PD, but who is later found to have MSA as the cause of their parkinsonism, would be included as MSA with phenoconversion set as the time of initial parkinsonism definition). All iRBD patients who developed PD, MSA, or DLB during follow-up were classified asphenoconverters.
Statistical analysis
Normality and homogeneity of variance were tested. Demographic data were compared between groups using one-way ANOVA or Kruskal Wallis test for continuous variables and the chi-square test or Fisher’s exact test for categorical variables. Differences of gait parameters between groups were assessed using repeated measures analysis of variance (group×walking condition). Post hoc tests were performed on each pair of groups. In order to test the association between baseline gait parameters and phenoconversion to α– synucleinopathies, Cox regression analysis was applied for each gait parameter and UPDRS part III score, with the hazard ratios (HRs) adjusted for age, sex, and BMI. For stratification, we used the 75th percentile value as cut-off point. Spearman’s correlation analyses were conducted to investigate relationships between all gait parameters and the UPDRSIII score. Correlations were determined based on | r| values as follows: 0.8–1.0, very strong; 0.6–0.8, strong; 0.4–0.6, moderate; 0.2–0.4, weak; and 0.0–0.2 very weak or no correlation. A two-sided p-value<0.05 was considered to be significant. All statistical analyses were conducted using SPSS, version 28 (IBM Corp., New York, NY).
RESULTS
Demographic characteristics
The demographic and clinical information for all participants is shown in Table 1. For the cross-sectional analysis at baseline, 31 iRBD patients and 33 HCs were included. The iRBD cohort was prospectively followed with a median of 4.5 years (mean±standard deviation, 5.4±2.8 years). For phenoconverters, the mean interval between the baseline assessment and disease diagnosis was 2.7±1.2 years. Among iRBD patients, 3 lost to follow-up, the remaining 28 (90.3%) patients were prospectively followed. During the follow up, 10 iRBD patients converted to definitive neurodegenerative diseases (converters), whereas the remaining iRBD patients survived to be disease-free (non-converters). Among the converters, 6 converted to PD, 3 to DLB and 1 to MSA. None of the HCs developed neurodegenerative diseases during the follow-up period. The sex distribution differed significantly between overall iRBD and HCs (χ2 = 4.280, p = 0.039). There were no statistically significant differences among age, sex, BMI, MMSE, and MoCA scores across HCs, converters, and non-converters. No differences in age or sex at baseline were found between converters and non-converters. Converters had significantly higher UPDRS III score (z = –2.607, p = 0.09) than non-converters at baseline.
Table 1
Baseline demographics and clinical information for healthy controls (HCs), patients with idiopathic rapid eye movement sleep behavior disorder (iRBD) who converted to definitive neurodegenerative diseases (converters), and remaining iRBD patients survived to be disease-free (non-converters). Data presented as mean±SD. Kruskal-Wallis test and Fisher’s Exact test were used for statistical comparison
HCs | Non-converters | Converters | iRBD | p# | |
N | 33 | 21 | 10 | 31 | |
Age, y | 69.93±5.54 | 67.75±6.59 | 71.43±6.65 | 68.87±6.68 | 0.321 |
Sex, M/F | 20/13 | 18/3 | 8/2 | 26/5 | 0.131 |
BMI | 26.22±5.97 | 24.82±3.31 | 24.24±3.65 | 24.63±3.36 | 0.679 |
MMSE | 27.93±2.51 | 28.10±2.00 | 28.20±1.32 | 28.13±1.78 | 0.844 |
MoCA | 24.19±3.96 | 24.39±3.24 | 24.57±2.64 | 24.44±3.03 | 0.321 |
UPDRS-III | 0.56±1.97 | 1.10±1.41 | 3.20±2.39 | 1.77±2.01 | <0.01* |
RBDQ-HK | 5.37±4.39 | 57.57±16.59 | 58.00±19.78 | 57.71±17.35 | <0.01* |
iRBD, idiopathic rapid eye movement sleep behavior disorder; HCs, healthy controls; M/F, male/female; BMI, body mass index; MMSE, Mini-Mental State Examination; MoCA, Montreal Cognitive Assessment; UPDRSIII, part III of Unified Parkinson’s Disease Rating Scale without action tremor; RBDQ-HK, REM Sleep Behavior Disorder (RBD) Questionnaire-Hong Kong; *p < 0.05. p#, compare between HCs, converters and non-converters.
Gait parameters at baseline under different walking conditions
Significant differences among baseline gait parameters across groups and walking conditions are described below according to independent domains (characteristics described in Supplementary Tables 1–3).
Gait
Significant between-group difference was found in stride length variation (F2,61 = 5.521, p = 0.006). Post-hoc analysis showed that stride length variation was significantly greater in converters compared with HCs under both normal walking (converters, 3.40±3.04; HCs, 1.67±0.71; 95% CI = 0.555–2.900, p = 0.002) and fast walking conditions (converters, 3.83±2.81; HCs, 2.11±1.10; 95% CI = 0.484–2.934, p = 0.003). Furthermore, converters showed a greater stride length variation than non-converters during normal walking (3.40±3.04 vs. 2.09±0.58, 95% CI = 0.064–2.560, p = 0.036) and fast walking condition (3.83±2.81 vs. 1.98±0.60, 95% CI = 0.536–3.153, p = 0.003) (Fig. 1A). Similar results were found in stride time variation (F2,61 = 3.313, p = 0.043) and stride velocity variation (F2,61 = 4.253, p = 0.019). Converters exhibited higher stride time variation than HCs during both normal walking (converters, 3.67±4.77; HCs, 1.56±0.43; 95% CI = 0.424–3.795, p = 0.009) and fast walking conditions (converters, 5.34±7.57; HCs, 1.97±0.86; 95% CI = 0.715–6.037, p = 0.008). Converters demonstrated greater stride time variation than non-converters during fast walking condition (5.34±7.57 vs. 1.99±0.65, 95% CI = 0.526–6.191, p = 0.015) (Fig. 1B). Furthermore, converters showed increased stride velocity variation than HCs under normal walking (converters, 4.80±4.57; HCs, 2.23±0.93; 95% CI = 0.813–4.315, p = 0.002) and fast walking conditions (converters, 5.52±5.07; HCs, 2.83±1.34; 95% CI = 0.724–4.652, p = 0.004). Similarly, converters also exhibited higher stride velocity variation than non-converters during normal walking (4.80±4.57 vs. 2.93±1.03, 95% CI = 0.004–3.732, p = 0.049) and fast walking condition (5.52±5.07 vs. 2.74±0.69, 95% CI = 0.688–4.869, p = 0.005) (Fig. 1C). No significant between-group differences were observed in other gait domains. However, significant difference for gait pace (stride velocity, F2,122 = 117.294, p < 0.001; stride length, F2,122 = 31.018, p < 0.001), gait rhythm (stride time, F2,122 = 80.509, p < 0.001; swing, F2,122 = 75.351, p < 0.001; stance, F2,122 = 75.351, p < 0.001), gait variability (stride length variation, F2,122 = 5.479, p = 0.016; stride time variation, F2,122 = 4.775, p = 0.028; stride velocity variation, F2,122 = 7.439, p = 0.006), gait asymmetry (swing asymmetry, F2,122 = 4.338, p = 0.023), was observed between different walking conditions. All participants walked with slowed speed, shortened step length, larger gait variability and gait asymmetry during dual-task condition.
Fig. 1
The (A) stride length variation, (B) arm range of motion (degrees), (C) stride time variation, and (D) stride velocity variation between iRBD patients, converters, non-converters, and healthy controls under each walking condition. iRBD, idiopathic rapid eye movement sleep behavior disorder; converters, iRBD patiants who were later diagnosed with degenerative disease; non-converters, iRBD patients who remained free from α-synucleinopathies; HCs, healthy controls. Boxplot shows the mean value. Error bars represent the standard deviation of the mean. Asterisks indicate significant differences in post-hoc comparisons (*p < 0.05).
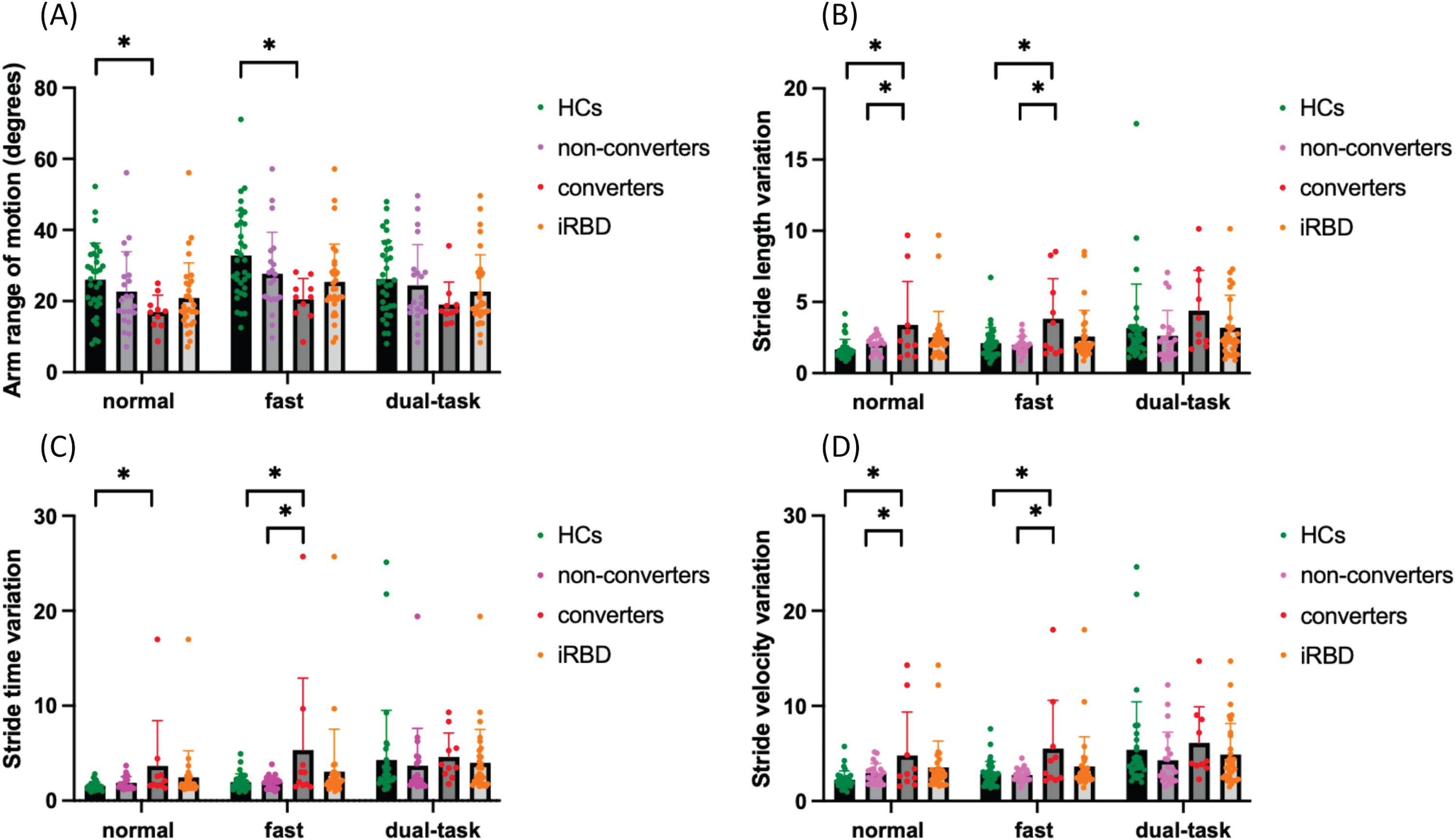
Arm
There was a significant difference between HCs, converters and non-converters in the arm range of motion (F2,61 = 3.695, p = 0.031). Post-hoc analysis showed range of motion was significantly lower in converters in comparison with HCs under normal walking (converters, 16.94±4.73; HCs, 26.02±10.29; 95% CI = 0.194– 17.961, p = 0.044) and fast walking condition (converters, 20.52±5.86; HCs, 32.81±12.68; 95% CI = 2.009– 22.580, p = 0.014) (Fig. 1D). There was a significant main effect of walking condition (arm range of motion, F2,122 = 14.088, p < 0.001; peak arm velocity, F2,122 = 29.987, p < 0.001; peak arm velocity asymmetry, F2,122 = 16.762, p < 0.001) in which all participants walked with a larger arm-swing range of motion, rapid arm swing velocity during fast walking. Larger arm swing velocity asymmetry was seen during dual-task walking for all participants.
Trunk
There was a significant difference between the groups in trunk sagittal range of motion (F2,61 = 3.323, p = 0.043). Post-hoc analysis showed trunk sagittal range of motion was significantly decreased in non-converters in comparison with HCs under fast walking condition (non-converters, 3.81±0.67; HCs, 4.36±0.89; 95% CI = 0.003–1.092, p = 0.048) (Supplementary Table 3). There was a significant main effect of walking condition (trunk sagittal range of motion, F2,122 = 7.915, p < 0.001; trunk horizontal range of motion, F2,122 = 31.545, p < 0.001; peak trunk sagittal velocity, F2,122 = 30.635, p < 0.001; peak trunk horizontal velocity, F2,122 = 13.915, p < 0.001) in which all participants walked with larger trunk sagittal and horizontal range of motion during dual-task condition.
Correlations between gait parameters in iRBD patients
Across all three walking conditions, several gait parameters exhibit strong correlations. These include stride time with stride velocity (r = –0.789, p < 0.001), stride time with swing (r = –0.640, p < 0.001), and stance (r = 0.640, p < 0.001), stride length variation with stride time variation (r = 0.642, p < 0.001), and stride velocity variation (r = 0.811, p < 0.001), and peak horizontal trunk velocity with trunk horizontal range of motion (r = 0.742, p < 0.001). When comparing gait parameters with UPDRS part III score, arm range of motion (r = –0.411, p = 0.022) and peak arm velocity (r = –0.452, p = 0.011) showed moderate correlation during fast walking condition (see Supplementary Tables 4–6).
Baseline gait markers associated with phenoconversion
Based on the results of Cox regression analyses, iRBD patients with higher scores of UPDRS III (adjusted HR = 1.504, 95% CI = 1.141–1.984, p = 0.004) were found to be more likely to convert to α-synucleinopathies. In terms of gait parameters, iRBD patients with increased gait variability (stride length, stride time variability, stride velocity), and increased stride length asymmetry under normal walking condition were also more likely to convert to α-synucleinopathies during a follow-up (stride length variation, adjusted HR = 1.594, 95% CI = 1.132–2.244, p = 0.008; stride time variation, adjusted HR = 1.235, 95% CI = 1.030–1.482, p = 0.023; stride velocity variation, adjusted HR = 1.308, 95% CI = 1.056–1.621, p = 0.014; stride length asymmetry, adjusted HR = 5.659, 95% CI = 1.655–19.357, p = 0.006). Additional predictors were observed for fast walking condition, including decreased arm range of motion, increased arm range of motion variation, increased peak arm velocity asymmetry, increased trunk sagittal range of motion, increased gait variability (stride length, stride time, and stride velocity), increased swing variation, and larger stride length asymmetry (stride length variation, adjusted HR = 1.801, 95% CI = 1.249–2.596, p = 0.002; stride time variation, adjusted HR = 1.169, 95% CI = 1.040–1.313, p = 0.009; stride velocity variation, adjusted HR = 1.324, 95% CI = 1.098–1.597, p = 0.003; stride length asymmetry, adjusted HR = 7.239, 95% CI = 1.785–29.356, p = 0.006; arm range of motion, adjusted HR = 0.889, 95% CI = 0.798–0.989, p = 0.031; arm range of motion variation, adjusted HR = 1.047, 95% CI = 1.009–1.086, p = 0.014; peak arm velocity asymmetry, adjusted HR = 1.059, 95% CI = 1.003–1.118, p = 0.040; trunk sagittal range of motion, adjusted HR = 3.584, 95% CI = 1.118–11.493, p = 0.032). Larger peak arm velocity asymmetry was the only predictor observed under dual-task walking condition (peak arm velocity asymmetry, adjusted HR = 1.060, 95% CI = 1.008–1.115, p = 0.023). Among all the gait predictors, greater stride length asymmetry showed the best single predictable value under both normal and fast walking condition (see in Table 2).
Table 2
COX regression analysis for conversion predictors in iRBD patients, adjusting age, sex and BMI for each variable. Extracted gait analysis parameters were presented into independent domains
Variables | Normal | Fast | Dual-task | ||||||
HR | 95% CI | p | HR | 95% CI | p | HR | 95% CI | p | |
Pace | |||||||||
Stride length (% stature) | 0.932 | 0.819–1.061 | 0.285 | 0.906 | 0.800–1.027 | 0.123 | 0.963 | 0.867–1.069 | 0.479 |
Stride velocity (% stature/s) | 1.003 | 0.912–1.102 | 0.957 | 0.997 | 0.911–1.091 | 0.940 | 0.997 | 0.931–1.067 | 0.928 |
Swing variation | 1.261 | 0.827–1.922 | 0.281 | 1.287 | 1.005–1.648 | 0.046* | 0.760 | 0.428–1.352 | 0.351 |
Rhythm | |||||||||
Stride time (s) | 0.019 | 0–163.773 | 0.391 | 0.009 | 0–79.654 | 0.310 | 0.266 | 0.001–84.316 | 0.652 |
Swing (%) | 1.086 | 0.744–1.585 | 0.669 | 1.148 | 0.783–1.684 | 0.480 | 0.996 | 0.709–1.400 | 0.981 |
Stance (%) | 0.921 | 0.631–1.344 | 0.669 | 0.871 | 0.594–1.277 | 0.480 | 1.004 | 0.714–1.411 | 0.981 |
Variability | |||||||||
Stride length variation | 1.594 | 1.132–2.244 | 0.008* | 1.801 | 1.249–2.596 | 0.002* | 1.238 | 0.971–1.578 | 0.086 |
Stride time variation | 1.235 | 1.030–1.482 | 0.023* | 1.169 | 1.040–1.313 | 0.009* | 1.012 | 0.867–1.181 | 0.879 |
Stride velocity variation | 1.308 | 1.056–1.621 | 0.014* | 1.324 | 1.098–1.597 | 0.003* | 1.088 | 0.921–1.286 | 0.322 |
Asymmetry | |||||||||
Swing asymmetry (%) | 0.978 | 0.775–1.233 | 0.849 | 0.892 | 0.687–1.158 | 0.389 | 0.901 | 0.666–1.219 | 0.500 |
Stance asymmetry (%) | 0.979 | 0.674–1.422 | 0.911 | 0.851 | 0.578–1.253 | 0.413 | 0.845 | 0.512–1.396 | 0.512 |
Postural control | |||||||||
Stride length asymmetry (%) | 5.659 | 1.655–19.357 | 0.006* | 7.239 | 1.785–29.356 | 0.006* | 2.372 | 0.727–7.739 | 0.152 |
Arm– swing | |||||||||
Arm range of motion (degrees) | 0.916 | 0.814–1.030 | 0.142 | 0.889 | 0.798–0.989 | 0.031* | 0.945 | 0.866-1.031 | 0.204 |
Peak arm velocity (degrees/s) | 1.004 | 0.997–1.011 | 0.244 | 1.000 | 0.992–1.009 | 0.932 | 1.003 | 0.993–1.013 | 0.533 |
Arm range of motion variation | 0.999 | 0.955–1.044 | 0.949 | 1.047 | 1.009–1.086 | 0.014* | 1.006 | 0.940–1.077 | 0.861 |
Peak arm velocity asymmetry (%) | 1.051 | 0.998–1.106 | 0.058 | 1.059 | 1.003–1.118 | 0.040* | 1.060 | 1.008–1.115 | 0.023* |
Trunk | |||||||||
Trunk horizontal range of motion (degrees) | 1.270 | 0.905–1.781 | 0.166 | 1.210 | 0.895–1.635 | 0.216 | 1.354 | 0.937–1.957 | 0.106 |
Peak horizontal trunk velocity (degrees/s) | 1.095 | 0.997–1.204 | 0.059 | 1.084 | 0.995–1.182 | 0.065 | 1.061 | 0.964–1.167 | 0.228 |
Trunk sagittal range of motion (degrees) | 1.752 | 0.594–5.169 | 0.310 | 3.584 | 1.118–11.493 | 0.032* | 2.581 | 0.943–7.064 | 0.065 |
Peak sagittal trunk velocity (degrees/s) | 1.011 | 0.859–1.190 | 0.895 | 1.069 | 0.966–1.182 | 0.195 | 1.027 | 0.913–1.156 | 0.656 |
CI, confidence interval; *p < 0.05.
Further stratification of the stride length asymmetry based on the 75th percentile value as cut-off point showed iRBD patients with higher stride length asymmetry (>1.21) under normal walking condition exhibited shorter disease-free survival duration than the remaining patients according to stratification (adjusted HR 4.468, 95% CI 1.088– 18.349, p = 0.038). Similar analysis in fast walking condition showed statically insignificant results.
DISCUSSION
To the best of our knowledge, this is the first study establishing the capability of gait analysis to detect iRBD phenoconversion to α-synucleinopathies. Our results demonstrated that selected gait analysis parameter – stride length asymmetry to be associated with phenoconversion to α-synucleinopathies in patients with iRBD. More specifically, a higher value of stride length asymmetry (>1.21) under normal walking condition was associated with an increased risk of progression to neurodegenerative diseases by 4.5 folds in patients with iRBD. At the baseline status, decreased arm range of motion, and increased variability (stride length, stride time, and stride velocity) discriminate iRBD converters from HCs. Increased variability (stride length, stride time, and stride velocity) also significantly differed between converters and non-converters.
Gait alteration is considered as a cardinal sign of PD. Previous studies have revealed that patients with early-stage PD walked with slower speed and shortened step length than the healthy individuals [17]. When considering more delicate gait alterations during the prodromal phase, gait variability and asymmetry are generally larger than that seen in age-matched healthy people [18]. Cross-sectional studies have shown subtle gait changes in iRBD patients [3]. A study of probable RBD has demonstrated decreased velocity, cadence, and significantly increased double limb support variability, greater stride time variability, and gait swing time variability when compared with health controls [6]. In another study, greater foot step variability and asymmetry have been revealed during dual-task walking in patients with PSG-diagnosed iRBD compared with controls [7]. Although in our study, we did not find significant differences in gait parameters between overall iRBD and HCs, even during the challenging condition (dual-task walking), our results showed that converters had significantly greater variability than HCs under normal and fast walking conditions. Increased gait variability, characterized by stride-to-stride fluctuations when walking, often appears before a reduction in step length in PD [19–21]. Thus, these findings suggest that iRBD patients who have the potential to develop synucleinopathies are prone to be interrupted by gait fluctuations, resembling the earliest alterations seen in PD.
Arm swing originates mainly from passive movements, which are stabilized by active muscle control, maintaining the integrity of human gait along with lower limb movements [22, 23]. Studies have shown that arm range of motion and velocity are reduced in PD compared with the HCs [24, 25]. Similar to lower limb motion, increased arm-swing variability and asymmetry are usually more noticeable during the early and prodromal stage of PD [26, 27]. A previous study developed a classification model using gait parameters that robustly distinguish iRBD from HCs and PD patients with a sensitivity of 100% and specificity of 90% [10]. In this study, limb range of motion and velocity were the main contributors to the model, indicating the importance of these parameters in identifying iRBD [10]. In our study, arm range of motion was significantly lower in converters in comparison with HCs under both normal walking and fast walking conditions. A reduction of arm swing is thought to be related to the dopaminergic depletion in the nigrostriatal circuit and which responds well to levodopa [28]. Thus, reduced arm range of motion may be considered as an early sign of potential phenoconversion iRBD (as a result of dopaminergic depletion).
It has been observed that non-converters not only demonstrated better values compared to converters, such as lower gait variation and larger arm range of motion, but also showed better values than HCs in certain aspects. For instance, non-converters exhibited a slightly larger stride length compared to HCs. It is well-documented that patients with PD present markedly shortened stride length even in the early stages, which can be improved with dopaminergic medications [5]. Therefore, the relatively larger stride length in non-converters may indicate a relatively higher dopamine reservoir or underlying compensation effects.
Notably, a challenging task reveals subtle gait alterations more easily during the prodromal stage of PD [29]. However, we found no significant group differences under dual-task walking. This difference could be mainly due to the differences in dual-task protocols or relatively small sample size which may have weakened the statistical power. Although iRBD groups showed a trend toward worsened gait during dual-task walking, a larger sample size is required to determine whether these changes would have statistical significance.
Identification of markers capable of predicting the phenoconversion from iRBD to clinical α– synucleinopathies are of great importance. Since parkinsonism develops in patients with isolated RBD who are later diagnosed with α-synucleinopathies, quantifying motor assessments represents intriguing markers [2, 30]. As far as we know, this is the first study to demonstrate longitudinal association of gait analysis and the disease conversion from PSG-confirmed iRBD to degenerative α-synucleinopathies. Del Din et al. conducted a longitudinal prospective study that identified selected gait analysis parameters as potentially early diagnostic markers of PD [31]. They recruited 696 healthy controls and longitudinally followed them four times with a 2-year interval. Consistent with Del Din et al. study, our findings showed iRBD patients with greater gait variability (stride length, stride time, stride velocity, and swing) and higher stride length asymmetry were more likely to convert to α-synucleinopathies. Among them, higher stride length asymmetry showed the best longitudinal association to phenoconversion. Greater left-right asymmetry of gait parameters, such as step length asymmetry, has been consistently shown to be associated with the asymmetric nature of bradykinesia and rigidity in patients with PD [18, 32]. Additionally, there is larger stride length asymmetry during walking in iRBD patients than in healthy older people [7]. Our finding supports the use of stride length asymmetry as an important and distinct gait marker in predicting the development of α-synuleinopathies in iRBD patients.
In previous studies, hazard ratios (HRs) of potential biomarkers were reported and could be compared with our findings. Arnaldi et al. followed 344 iRBD patients for 2 years, during which 52 (20%) patients developed synucleinopathy. The highest HR (HR = 4.35) was associated with DAT-SPECT reduction in the putamen of the most affected hemisphere. Additionally, a combination of putamen DAT, constipation, and age showed an HR of 5.71 [33]. In another study, 1,280 iRBD patients were followed up for an average of 4.6 years, with an annual conversion rate of 6.3%. Results revealed that the best HR results were associated with abnormal quantitative motor testing using a combination of alternate-tap test, Purdue pegboard, 3-metre timed-up-and-go, and flamingo balance test (HR = 3.16). Other factors included motor symptoms evaluated by UPDRS part II and part III, olfactory deficit, mild cognitive impairment assessed by neuropsychological examination, and abnormal putamen DAT with HR ranging from 1.98 to 3.03 [30]. In our study, stride length asymmetry showed the best predictive value with an HR of 4.468, similar to the reported putamen DAT in the most affected side, and higher than the UPDRS III score in our study at 1.504. This result suggests that quantitative gait analysis may be a more convenient and cost-effective method with relatively high value in predicting phenoconversion in iRBD patients. However, a large sample size study is required to confirm this finding.
Besides gait parameters, our study found that arm and trunk parameters were also potential predictors of iRBD phenoconversion. These parameters were not examined in Del Din et al. study, including arm range of motion, arm range of motion variation, peak arm velocity asymmetry, and trunk sagittal range of motion. It is important to determine to what extent these predictors may overlap [34], and our results showed that certain parameters indeed exhibited strong correlations. Future evaluations of iRBD gait analysis may only involve selected parameters and call for more precise assessments involving diverse body areas that are commonly affected in α-synuleinopathies.
Limitation
Some limitations of this study should be acknowledged. First, the sample size was small which may weaken the statistical power. After an average of 5.40 years of follow-up, only 10 patients had converted to α-synucleinopathies. The limited number of phenoconverters may restrict the reliability of hazard ratio estimates in this study. Additionally, separate analyses for PD, DLB, and MSA converters were not performed due to the small sample size. Future studies should explore this aspect. Moreover, changes associated with longitudinal disease progression were not analyzed. Future longitudinal studies with larger sample sizes are necessary to confirm our findings and perform prospective analysis to demonstrate dynamic changes. Second, our study did not extract arm range of motion asymmetry–a critical arm-swing measurement for prodromal phase. Future studies should involve the asymmetrical measurement of upper limb movements. Third, RBD symptoms for HCs were clinically excluded via the RBDQ-HK (total score < 18) instead of polysomnography. Thus, we may have had a few RBD patients in our HC group. However, we believe this potential bias is unlikely to be considerable due to the low prevalence of iRBD in the general population [35, 36]. Finally, while it would be important to apply corrections for multiple tests to all the identified p-values, this step was omitted due to the exploratory nature of this study.
Conclusion
We demonstrated that selected gait analysis parameter, in particular stride length asymmetry may serve as a useful gait marker to distinguish iRBD patients at risk of developing neurodegenerative disease. Our findings support the use of the gait analysis with wearable sensors as a promising screening tool to measure gait parameters in prodromal α-synuleinopathies.
ACKNOWLEDGMENTS
We thank all participants’ contributions to this study.
FUNDING
This work was supported by the National Natural Science Foundation of China [No. 82201410, No.82201409], The National Key R& D Program of China [No. 2021YFC2501200, 2018YFC1312001, 2017YFC0840105, 2017YFC1310200], Key Realm R& D Program of Guangdong Province [No. 2018B030337001].
CONFLICT OF INTEREST
The authors have no conflict of interest to report.
DATA AVAILABILITY
The data supporting the findings of this study are available on request from the corresponding author. The data are not publicly available due to privacy or ethical restrictions.
SUPPLEMENTARY MATERIAL
[1] The supplementary material is available in the electronic version of this article: https://dx.doi.org/10.3233/JPD-230397.
REFERENCES
[1] | Postuma RB , Gagnon JF , Vendette M , Fantini ML , Massicotte-Marquez J , Montplaisir J ((2009) ) Quantifying the risk of neurodegenerative disease in idiopathic REM sleep behavior disorder. Neurology 72: , 1296–1300. |
[2] | Miglis MG , Adler CH , Antelmi E , Arnaldi D , Baldelli L , Boeve BF , Cesari M , Dall’Antonia I , Diederich NJ , Doppler K , Dusek P , Ferri R , Gagnon JF , Gan-Or Z , Hermann W , Hogl B , Hu MT , Iranzo A , Janzen A , Kuzkina A , Lee JY , Leenders KL , Lewis SJG , Liguori C , Liu J , Lo C , Ehgoetz Martens KA , Nepozitek J , Plazzi G , Provini F , Puligheddu M , Rolinski M , Rusz J , Stefani A , Summers RLS , Yoo D , Zitser J , Oertel WH ((2021) ) Biomarkers of conversion to alpha-synucleinopathy in isolated rapid-eye-movement sleep behaviour disorder. Lancet Neurol 20: , 671–684. |
[3] | MacKinnon CD , Alibiglou L , Videnovic A ((2019) ) Gait and postural disorders in REM sleep behavior disorder. In Rapid-Eye-Movement Sleep Behavior Disorder, pp. 547–556. |
[4] | Chhetri JK , Mei S , Wang C , Chan P ((2023) ) New horizons in Parkinson’s disease in older populations. Age Ageing 52: . |
[5] | Mirelman A , Bonato P , Camicioli R , Ellis TD , Giladi N , Hamilton JL , Hass CJ , Hausdorff JM , Pelosin E , Almeida QJ ((2019) ) Gait impairments in Parkinson’s disease. Lancet Neurol 18: , 697–708. |
[6] | McDade EM , Boot BP , Christianson TJ , Pankratz VS , Boeve BF , Ferman TJ , Bieniek K , Hollman JH , Roberts RO , Mielke MM , Knopman DS , Petersen RC ((2013) ) Subtle gait changes in patients with REM sleep behavior disorder. Mov Disord 28: , 1847–1853. |
[7] | Ehgoetz Martens KA , Matar E , Hall JM , Phillips J , Szeto JYY , Gouelle A , Grunstein RR , Halliday GM , Lewis SJG ((2019) ) Subtle gait and balance impairments occur in idiopathic rapid eye movement sleep behavior disorder. Mov Disord 34: , 1374–1380. |
[8] | Alibiglou L , Videnovic A , Planetta PJ , Vaillancourt DE , MacKinnon CD ((2016) ) Subliminal gait initiation deficits in rapid eye movement sleep behavior disorder: A harbinger of freezing of gait? Mov Disord 31: , 1711–1719. |
[9] | Del Din S , Yarnall AJ , Barber TR , Lo C , Crabbe M , Rolinski M , Baig F , Hu MT , Rochester L ((2020) ) Continuous real-world gait monitoring in idiopathic REM sleep behavior disorder. J Parkinsons Dis 10: , 283–299. |
[10] | Cochen De Cock V , Dotov D , Lacombe S , Picot MC , Galtier F , Driss V , Giovanni C , Geny C , Abril B , Damm L , Janaqi S ((2022) ) Classifying idiopathic rapid eye movement sleep behavior disorder, controls, and mild Parkinson’s disease using gait parameters. Mov Disord 37: , 842–846. |
[11] | Sateia MJ ((2014) ) International classification of sleep disorders-third edition: Highlights and modifications. Chest 146: , 1387–1394. |
[12] | Li SX , Wing YK , Lam SP , Zhang J , Yu MW , Ho CK , Tsoh J , Mok V ((2010) ) Validation of a new REM sleep behavior disorder questionnaire (RBDQ-HK). Sleep Med 11: , 43–48. |
[13] | Salarian A , Russmann H , Vingerhoets FJ , Dehollain C , Blanc Y , Burkhard PR , Aminian K ((2004) ) Gait assessment in Parkinson’s disease: Toward an ambulatory system for long-term monitoring. IEEE Trans Biomed Eng 51: , 1434–1443. |
[14] | Postuma RB , Berg D , Stern M , Poewe W , Olanow CW , Oertel W , Obeso J , Marek K , Litvan I , Lang AE , Halliday G , Goetz CG , Gasser T , Dubois B , Chan P , Bloem BR , Adler CH , Deuschl G ((2015) ) MDS clinical diagnostic criteria for Parkinson’s disease. Mov Disord 30: , 1591–1601. |
[15] | Gilman S , Wenning GK , Low PA , Brooks DJ , Mathias CJ , Trojanowski JQ , Wood NW , Colosimo C , Durr A , Fowler CJ , Kaufmann H , Klockgether T , Lees A , Poewe W , Quinn N , Revesz T , Robertson D , Sandroni P , Seppi K , Vidailhet M ((2008) ) Second consensus statement on the diagnosis of multiple system atrophy. Neurology 71: , 670–676. |
[16] | McKeith IG , Boeve BF , Dickson DW , Halliday G , Taylor JP , Weintraub D , Aarsland D , Galvin J , Attems J , Ballard CG , Bayston A , Beach TG , Blanc F , Bohnen N , Bonanni L , Bras J , Brundin P , Burn D , Chen-Plotkin A , Duda JE , El-Agnaf O , Feldman H , Ferman TJ , Ffytche D , Fujishiro H , Galasko D , Goldman JG , Gomperts SN , Graff-Radford NR , Honig LS , Iranzo A , Kantarci K , Kaufer D , Kukull W , Lee VMY , Leverenz JB , Lewis S , Lippa C , Lunde A , Masellis M , Masliah E , McLean P , Mollenhauer B , Montine TJ , Moreno E , Mori E , Murray M , O’Brien JT , Orimo S , Postuma RB , Ramaswamy S , Ross OA , Salmon DP , Singleton A , Taylor A , Thomas A , Tiraboschi P , Toledo JB , Trojanowski JQ , Tsuang D , Walker Z , Yamada M , Kosaka K ((2017) ) Diagnosis and management of dementia with Lewy bodies: Fourth consensus report of the DLB Consortium. Neurology 89: , 88–100. |
[17] | Djuric-Jovicic M , Belic M , Stankovic I , Radovanovic S , Kostic VS ((2017) ) Selection of gait parameters for differential diagnostics of patients with de novo Parkinson’s disease. Neurol Res 39: , 853–861. |
[18] | Peterson DS , Horak FB ((2016) ) Neural control of walking in people with parkinsonism. Physiology (Bethesda) 31: , 95–107. |
[19] | Galna B , Lord S , Burn DJ , Rochester L ((2015) ) Progression of gait dysfunction in incident Parkinson’s disease: Impact of medication and phenotype. Mov Disord 30: , 359–367. |
[20] | Hausdorff JM ((2005) ) Gait variability: Methods, modeling and meaning. J Neuroeng Rehabil 2: , 19. |
[21] | Hausdorff JM ((2009) ) Gait dynamics in Parkinson’s disease: common and distinct behavior among stride length, gait variability, and fractal-like scaling. Chaos 19: , 026113. |
[22] | Meyns P , Bruijn SM , Duysens J ((2013) ) The how and why of arm swing during human walking. Gait Posture 38: , 555–562. |
[23] | Navarro-Lopez V , Fernandez-Vazquez D , Molina-Rueda F , Cuesta-Gomez A , Garcia-Prados P , Del-Valle-Gratacos M , Carratala-Tejada M ((2022) ) Arm-swing kinematics in Parkinson’s disease: A systematic review and meta-analysis. Gait Posture 98: , 85–95. |
[24] | Schneider SA , Drude L , Kasten M , Klein C , Hagenah J ((2012) ) A study of subtle motor signs in early Parkinson’s disease. Mov Disord 27: , 1563–1566. |
[25] | Mirelman A , Bernad-Elazari H , Thaler A , Giladi-Yacobi E , Gurevich T , Gana-Weisz M , Saunders-Pullman R , Raymond D , Doan N , Bressman SB , Marder KS , Alcalay RN , Rao AK , Berg D , Brockmann K , Aasly J , Waro BJ , Tolosa E , Vilas D , Pont-Sunyer C , Orr-Urtreger A , Hausdorff JM , Giladi N ((2016) ) Arm swing as a potential new prodromal marker of Parkinson’s disease. Mov Disord 31: , 1527–1534. |
[26] | Huang X , Mahoney JM , Lewis MM , Guangwei D , Piazza SJ , Cusumano JP ((2012) ) Both coordination and symmetry of arm swing are reduced in Parkinson’s disease. Gait Posture 35: , 373–377. |
[27] | Lewek MD , Poole R , Johnson J , Halawa O , Huang X ((2010) ) Arm swing magnitude and asymmetry during gait in the early stages of Parkinson’s disease. Gait Posture 31: , 256–260. |
[28] | Sterling NW , Cusumano JP , Shaham N , Piazza SJ , Liu G , Kong L , Du G , Lewis MM , Huang X ((2015) ) Dopaminergic modulation of arm swing during gait among Parkinson’s disease patients. J Parkinsons Dis 5: , 141–150. |
[29] | Mirelman A , Gurevich T , Giladi N , Bar-Shira A , Orr-Urtreger A , Hausdorff JM ((2011) ) Gait alterations in healthy carriers of the LRRK2 G2019S mutation. Ann Neurol 69: , 193–197. |
[30] | Postuma RB , Iranzo A , Hu M , Hogl B , Boeve BF , Manni R , Oertel WH , Arnulf I , Ferini-Strambi L , Puligheddu M , Antelmi E , Cochen De Cock V , Arnaldi D , Mollenhauer B , Videnovic A , Sonka K , Jung KY , Kunz D , Dauvilliers Y , Provini F , Lewis SJ , Buskova J , Pavlova M , Heidbreder A , Montplaisir JY , Santamaria J , Barber TR , Stefani A , St Louis EK , Terzaghi M , Janzen A , Leu-Semenescu S , Plazzi G , Nobili F , Sixel-Doering F , Dusek P , Bes F , Cortelli P , Ehgoetz Martens K , Gagnon JF , Gaig C , Zucconi M , Trenkwalder C , Gan-Or Z , Lo C , Rolinski M , Mahlknecht P , Holzknecht E , Boeve AR , Teigen LN , Toscano G , Mayer G , Morbelli S , Dawson B , Pelletier A ((2019) ) Risk and predictors of dementia and parkinsonism in idiopathic REM sleep behaviour disorder: A multicentre study. Brain 142: , 744–759. |
[31] | Del Din S , Elshehabi M , Galna B , Hobert MA , Warmerdam E , Suenkel U , Brockmann K , Metzger F , Hansen C , Berg D , Rochester L , Maetzler W ((2019) ) Gait analysis with wearables predicts conversion to parkinson disease. Ann Neurol 86: , 357–367. |
[32] | Fling BW , Curtze C , Horak FB ((2018) ) Gait asymmetry in people with Parkinson’s disease is linked to reduced integrity of callosal sensorimotor regions. Front Neurol 9: , 215. |
[33] | Arnaldi D , Chincarini A , Hu MT , Sonka K , Boeve B , Miyamoto T , Puligheddu M , De Cock VC , Terzaghi M , Plazzi G , Tachibana N , Morbelli S , Rolinski M , Dusek P , Lowe V , Miyamoto M , Figorilli M , Verbizier D , Bossert I , Antelmi E , Meli R , Barber TR , Trnka J , Miyagawa T , Serra A , Pizza F , Bauckneht M , Bradley KM , Zogala D , McGowan DR , Jordan L , Manni R , Nobili F ((2021) ) Dopaminergic imaging and clinical predictors for phenoconversion of REM sleep behaviour disorder. Brain 144: , 278–287. |
[34] | Dommershuijsen LJ , Ikram MK , Darweesh SKL ((2019) ) Quantitative motor functioning in prodromal Parkinson disease. Ann Neurol 86: , 981. |
[35] | Zhang H , Gu Z , Yao C , Cai Y , Li Y , Mao W , Xu E , Postuma RB , Chan P ((2020) ) Risk factors for possible REM sleep behavior disorders: A community-based study in Beijing. Neurology 95: , e2214–e2224. |
[36] | Haba-Rubio J , Frauscher B , Marques-Vidal P , Toriel J , Tobback N , Andries D , Preisig M , Vollenweider P , Postuma R , Heinzer R ((2018) ) Prevalence and determinants of rapid eye movement sleep behavior disorder in the general population. Sleep 41: , zsx197. |