Effects of aerobic and combined aerobic-resistance exercise on motor function in sedentary older adults: A randomized clinical trial
Abstract
BACKGROUND:
Sedentary behavior is widespread among older adults and accelerates the decline of motor function. Nevertheless, there is insufficient evidence concerning the effectiveness of regular exercise in enhancing the same in sedentary older adults.
OBJECTIVE:
To compare the effects of 24 weeks of aerobic and combined aerobic-resistance exercise on the motor function of sedentary older adults.
METHODS:
Sixty healthy sedentary older (65–80 years) were randomly enrolled. Participants were randomly divided into 3 groups (1:1:1): aerobic exercise group (AEG), combined aerobic-resistance exercise group (CEG), and health education group (HEG). The training group underwent a five-day-a-week regimen, with each session lasting for 40 minutes (including 10 min warm-up and cool-down). HEG received only monthly health lectures. We assessed lower limb muscle strength (30-second sit-to-stand ability), single-dual task gait, static and dynamic balance functions at baseline and after 24 weeks of intervention using per-protocol analysis.
RESULTS:
Among 60 elderly healthy who were randomized (mean age 70.59
CONCLUSION:
Both the aerobic exercise and combined aerobic-resistance exercise programs are effective in enhancing lower limb muscle strength, dynamic balance, and static balance while standing with eyes closed in sedentary older adults. Furthermore, the combined aerobic-resistance exercise program is more effective in improving dual-task gait speed as well as medial and lateral dynamic balance.
1.Introduction
Physical inactivity and sedentary lifestyles are rampant worldwide, resulting in an upsurge of health problems. Sedentary behavior has various harmful effects on the body, activating mechanisms such as metabolic disorders and endocrine dysregulation, which increase the risk of chronic diseases, systemic dysfunction, and all-cause mortality. Among all age groups, the elderly individuals exhibit the highest levels of sedentary behavior. Given the increased vulnerability of the elderly to chronic diseases, it is critical to provide greater attention to the sedentary elderly population and implement appropriate prevention and intervention measures [1, 2, 3, 4].
The aging process is usually accompanied by an attenuation of physiological functions, which not only involves the onset of age-related chronic diseases but also encompasses functional decline. Motor functions, include muscle strength, balance control, and walking stability; they deteriorate with age, leading to an increased risk of falls among the elderly [5]. Among these motor functions, muscle strength is particularly vulnerable, with older adults experiencing an approximate annual loss of 3% in muscle strength and 1–2% in muscle mass [6]. Significantly, sedentary behavior is identified as an independent risk factor for declining motor function in older adults, irrespective of aging itself [7]. Existing studies have indicated that extended sedentary behavior in older adults leads to a reduction in lean body mass percentage, along with decreases in muscle mass and strength, and impaired balance control and walking performance. This type of evidence underscores the elevated risk of falls and sarcopenia among sedentary older adults, which arise due to both structural and overall motor function changes [8, 9, 10, 11].
Regular physical activity has a strong correlation with a decreased risk of falls in older adults, which can be attributed to its positive effects on gait performance, balance control, and muscle strength [5, 10, 11]. Consequently, physical activity guidelines recommend that older adults engage in a minimum of 150 minutes of exercise per week to reap the benefits of good health. However, sedentary older adults often fail to meet the recommended guidelines [12, 13, 14]. For enhancing physical function and reducing the risk of falls in older adults, aerobic exercise and resistance training are both recommended as primary forms of exercise. Aerobic exercise enhances cardiovascular adaptation and induces changes in skeletal muscle metabolism, whereas resistance training improves muscle strength and mass [15, 16, 17].
Research indicates that even when performed below the recommended duration in the guidelines, aerobic exercise and combined aerobic-resistance exercise can still improve physical function in older adults. Additionally, combined aerobic-resistance exercise appears to be more effective than aerobic exercise alone in reducing the risk of falls among older adults [18, 19, 20]. However, it must be noted that given the detrimental effects of extended sedentary behavior on physical function in older adults, sedentary individuals may require more extended intervention periods of exercise to achieve equivalent health benefits to those of non-sedentary individuals. Nevertheless, the optimal exercise duration for enhancing physical function in sedentary older adults remains ambiguous.
Considering the insufficient exercise motivation and poor physical fitness factors regularly observed among sedentary older adults, the purpose of this study is to examine the intervention effects of 24 weeks of aerobic exercise and combined aerobic-resistance exercise on the physical function of this population, while adhering to the recommended duration specified in physical activity guidelines. We hypothesize that both aerobic exercise and combined aerobic-resistance exercise will result in varying degrees of improvement in the physical function of sedentary older adults, with combined exercise demonstrating better outcomes than aerobic exercise alone.
2.Material and methods
2.1Participants and recruitment
Elder healthy (65–80 years old) were recruited in communities from June to July, 2020 in Shenyang, Liaoning Province, China. To determine whether the participants met the criteria for sedentary behavior (energy expenditure
Figure 1.
Study flow chart. AEG, aerobic exercise group; CEG, combined aerobic-resistance group; HEG, health education group.
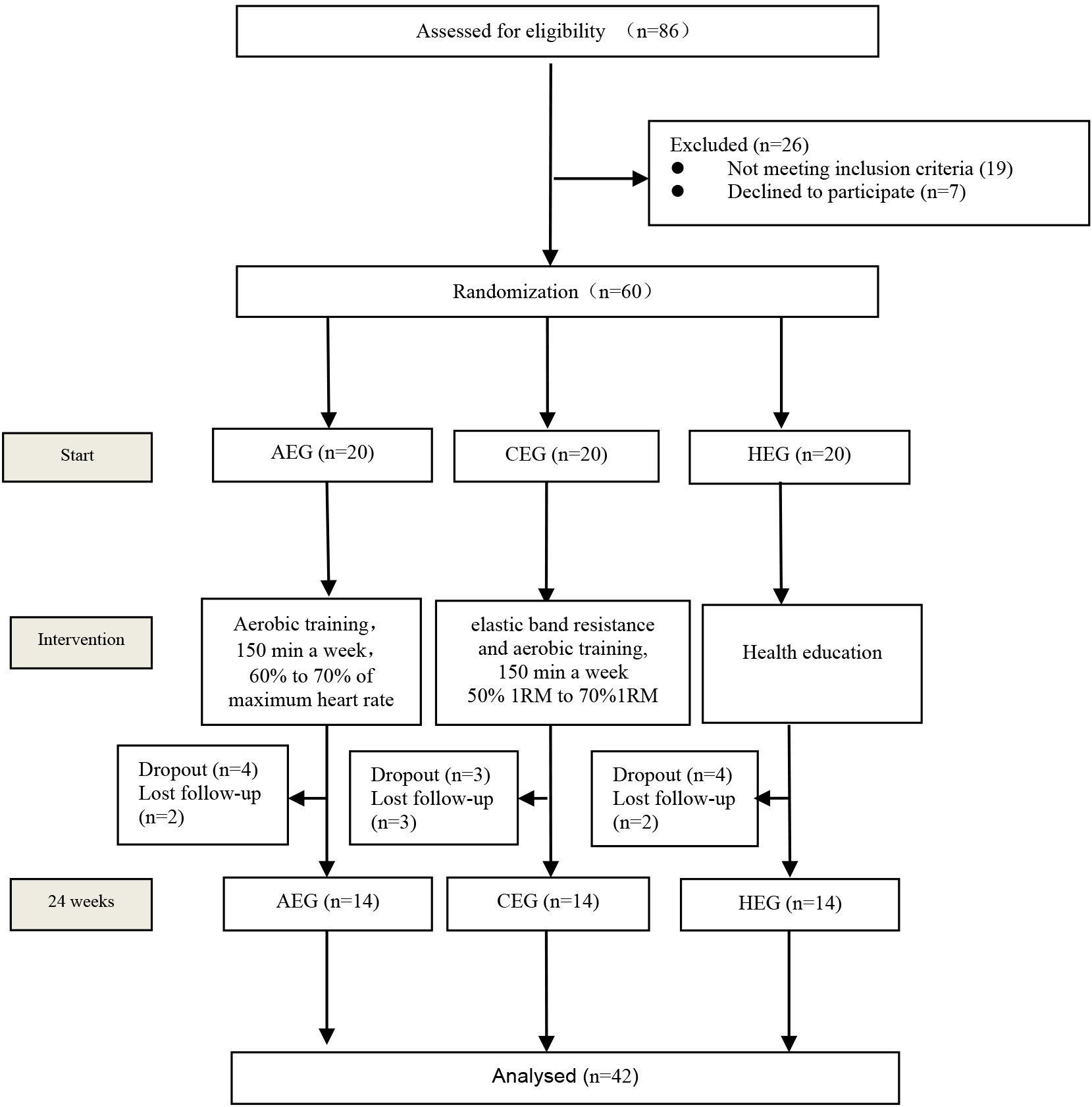
2.2Study design
This 24-week, single-center, single-blind, three-arm, controlled trial was conducted in accordance with the Declaration of Helsinki and approved by the Ethics Committee of Shenyang Sport University (code: 2020[3]). A total of 86 volunteers were initially assessed for eligibility, of which 60 were randomized in a 1:1:1 fashion (computer generated randomization) to three groups: aerobic exercise group (AEG,
2.2.1Inclusion criteria
(1) Aged 65–80 years; (2) energy expenditure
2.2.2Exclusion criteria
(1) Suffering from mental or cognitive disorders causing unable to cooperate; (2) Cardiovascular diseases that do not limit exercise, such as arrhythmia, history of angina pectoris, hypertension, coronary artery disease, myocardial infarction and heart valve disease; (3) Suffering from endocrine system diseases such as diabetes, gout, osteoporosis and obesity; (4) Suffering from benign or malignant neoplastic diseases; (5) Any neurological and musculoskeletal disorders that unable to complete the planned exercise program are excluded.
2.2.3Intervention
The exercise groups underwent a 24-week uninterrupted exercise regimen supervised by a clinical exercise physiologist (CEP). The regimen was designed according to the physical activity recommendations for older adults issued by the published guidelines of the World Health Organization guidelines [12] and the American College of Sports Medicine [13]. These guidelines dictate that individuals aged 60 and over should engage in at least 150 minutes of moderate-intensity exercise every week. Prior to the intervention, all participants received training and were made aware of the exercise intervention program.
2.2.4Aerobic exercise group
The aerobic exercise protocol consisted of a 5-min warm-up (at 50–60% of maximum heart rate) and 30 min of power cycling aerobics training (at 60–70% maximum heart rate), and a final 5-minute cooldown [22]. To monitor participant exercise intensity during training, a heart rate monitor (Polar Electro, Kempele, Finland) was utilized. The resistance of the power bicycle was adjusted to maintain the prescribed exercise intensity level and allow participants to successfully complete their aerobic training.
2.2.5Combined exercise group
Combined exercise training consisted of aerobic and resistance exercise with the same duration as the aerobic group. The combined exercise group consisted of approximately 10 minutes of elastic band resistance exercise and 20 minutes of power cycling aerobic exercise (60–70% of maximum heart rate), each training beginning with a 5-minute warm-up and ending with a 5-minute cool-down. Resistance training includes band resistance exercises for the triceps, quadriceps, glutes and hamstrings of the calf, including four resistance movements: heel lifts, lunges, kicks and knee flexion. Two sets of training were completed for each of these four movements, with 8–12 repetitions per set. The intensity of resistance training was gradually increased from 50% to 70% of the maximum muscle strength (1RM) measured before the start of the intervention program. The participants’ 1-RM was assessed every 4 weeks, and the resistance training intensity was adjusted accordingly during the training period. Participants were continuously monitored for heart rate and received supervision from clinicians throughout their training.
2.2.6Health education group
The health education group attended a 30-minute health education lecture every month for 24 weeks, delivered by the exercise physiologist via PowerPoint. The lecture topics included sedentary behavior, exercise for the health of older adults, and other relevant information. Additionally, it was emphasized that older adults should engage in regular physical activity and minimize sedentary time.
2.3Measurements
The participants’ basic data (including age, height, weight, and blood pressure) were assessed by a dedicated research assistant. The remaining motor function tests and data collection were conducted by scientific staff who were blinded to the participants’ study protocol assignment, both before the start of the trial and at the end of the 24-week intervention.
2.3.1Sedentary behavior measurements
The inclusion criteria for sedentary people defined sedentary behavior as “any waking behavior characterized by energy expenditure
2.3.230-s chair stand test
The chair stand test is a reliable and convenient method of measuring lower limb muscle strength in older adults and is useful for predicting the risk of falls in this population [24]. Lower limb muscle strength was assessed by measuring the number of repetitions of standing-sitting within 30 seconds. A higher number of repetitions indicates better lower limb muscle strength [25].
2.3.3Single and dual task gait test
During the single-dual task, gait parameters were recorded using the Footscan gait analysis system(RsScan International, Olen, Belgium), following the same test procedures as in our previous study [26]. Participants were given three practice sessions on the force plate prior to the formal test to familiarize themselves with the experimental environment and ensure compliance with the experimental standards. During the test, participants walked barefoot on the platform at a customary speed for three trials. For the dual-task test, participants simultaneously performed a numeric arithmetic task while walking, as directed by the tester. The gait parameters collected included gait speed, step length, and step width in both single and dual gait tasks, and the results of the experiment were averaged over the three experiments for analysis.
2.3.4Static and dynamic balance
Static balance was tested with a Good Balance system (Metitur Oy, Jyvaskyla, Finland). During the static balance test, participants completed four different modes of standing, including standing on the left leg with eyes open, standing on the right leg with eyes open, standing on the left leg with eyes closed, and standing on the right leg with eyes closed. The test was performed with the participants standing barefoot, arms resting on both sides of the body, body stationary, and eyes looking directly at the screen. The participants stood on one leg with the supporting leg in the center of the dynamometer and the other leg bent at 90
As the Good Balance system is unable to perform dynamic balance tests, dynamic balance was tested using the Techno-body Prokin (Technobody Inc, Italy), where the participants stood on an unstable platform. To avoid potential injury due to the high demands placed on older adults during closed-eye dynamic balance testing, only open-eye bipedal stance was assessed. The testing requirements were the same as those for static balance. The parameters of the dynamic balance test included the Overall Stability Index (OSI), the Anterior-Posterior Stability Index (APSI), and the Medial-Lateral Stability Index (MLSI). A higher stability index indicates poorer stability for the participants.
Table 1
Baseline characteristics of participants
Variables | Aerobic exercise group ( | Combined group ( | Health education group ( | |
Age (years) | 70.20 | 70.03 | 71.21 | 0.568 |
Height(meters) | 1.63 | 1.64 | 1.62 | 0.814 |
Weight (kg) | 67.54 | 65.91 | 65.85 | 0.659 |
SPB (mmHg) | 129.71 | 126.21 | 128.71 | 0.263 |
DBP (mmHg) | 80.43 | 81.14 | 83.07 | 0.473 |
HR (bpm) | 81.71 | 81.36 | 76.29 | 0.317 |
MET | 1.26 | 1.27 | 1.28 | 0.560 |
30-s chair stand test (rps) | 10.64 | 10.64 | 10.50 | 0.978 |
Single task gait | ||||
Gait speed (m/s) | 1.49 | 1.48 | 1.49 | 0.971 |
Gait length (cm) | 64.57 | 64.80 | 64.42 | 0.969 |
Gait width (cm) | 9.53 | 8.45 | 8.05 | 0.236 |
Dual task gait | ||||
Gait speed (m/s) | 1.30 | 1.31 | 1.29 | 0.976 |
Gait length (cm) | 62.90 | 62.50 | 61.00 | 0.218 |
Gait width (cm) | 8.86 | 8.65 | 9.40 | 0.106 |
Static balance | ||||
Left single leg stance | ||||
OE, Cop-X (mm) | 577.33 | 532.43 | 530.36 | 0.990 |
OE, Cop-Y (mm) | 566.87 | 588.61 | 565.47 | 0.871 |
CE, Cop-X (mm) | 1285.81 | 1290.78 | 1304.59 | 0.976 |
CE, Cop-Y (mm) | 1357.05 | 1378.09 | 1333.04 | 0.906 |
Right single leg stance | ||||
OE, Cop-X (mm) | 539.70 | 526.27 | 542.38 | 0.916 |
OE, Cop-Y (mm) | 548.36 | 541.77 | 540.16 | 0.981 |
CE, Cop-X (mm) | 1350.99 | 1329.15 | 1362.55 | 0.939 |
CE, Cop-Y (mm) | 1363.69 | 1374.31 | 1382.29 | 0.900 |
Dynamic balance | ||||
OSI | 4.64 (4.25, 6.26) | 4.59 (4.40, 5.71) | 4.89 (4.55, 4.46) | 0.660 |
APSI | 2.87 (2.41, 3.91) | 3.13 (2.89, 3.32) | 3.51 (3.06, 3.67) | 0.228 |
MLSI | 3.78 (3.24, 4.12) | 3.39 (3.17, 4.80) | 3.46 (3.19, 4.12) | 0.689 |
SBP, systolic blood pressure; DBP, diastolic blood pressure; HR, Heart rate; bpm, beats per minute; OE, open eyes; CE, close eyes; COP-X, average center of pressure X; COP-Y, average center of pressure Y; OSI, Overall Stability Index; APSI, Anterior-Posterior Stability Index; MLSI, Medial-Lateral Stability Index.
2.4Statistical analysis
Outcome analyses were conducted using a per protocol set (PPS) analysis of participants who completed the full 24-week study and were compliant with the protocol. Data were analyzed using SPSS version 25.0, and the normality of the data was assessed using the Shapiro-Wilk test. If the data were found to be non-normally distributed, they were inverted and transformed to meet the requirements of a normal distribution. Baseline characteristics of the groups were compared using a one-way ANOVA, and the effects of the different interventions were assessed using a 3 (group)
3.Results
3.1Participants’ characteristics
The flow of participants throughout the study is shown in Fig. 1. Among 60 elderly healthy who were randomized (mean age 70.59
Table 2
30-s chair stand and single-dual task gait outcomes
Variables | Aerobic exercise group | Combined group | Health education group | Group | Time | Group | ||||||
---|---|---|---|---|---|---|---|---|---|---|---|---|
Baseline | 24 weeks | Baseline | 24 weeks | Baseline | 24 weeks | F | P | F | P | F | P | |
30-s chair stand test (rps) | 10.64 | 12.07 | 10.64 | 13.57 | 10.50 | 10.29 | 2.65 | 0.083 | 94.87 | 37.80 | ||
Single task gait | ||||||||||||
Gait speed (m/s) | 1.49 | 1.50 | 1.48 | 1.49 | 1.49 | 1.49 | 0.01 | 0.985 | 3.35 | 0.075 | 0.18 | 0.837 |
Gait length (cm) | 64.57 | 64.52 | 64.80 | 66.62 | 64.42 | 64.86 | 0.48 | 0.623 | 1.11 | 0.298 | 0.646 | 0.530 |
Gait width (cm) | 9.53 | 9.98 | 8.45 | 8.11 | 8.05 | 7.99 | 2.55 | 0.091 | 0.01 | 0.962 | 0.48 | 0.620 |
Dual task gait | ||||||||||||
Gait speed (m/s) | 1.30 | 1.32 | 1.31 | 1.41 | 1.29 | 1.28 | 1.47 | 0.241 | 12.81 | 0.010 | 10.43 | |
Gait length (cm) | 62.90 | 63.90 | 62.50 | 64.90 | 61.00 | 62.00 | 0.63 | 0.534 | 1.89 | 0.177 | 0.72 | 0.494 |
Gait width (cm) | 8.86 | 8.76 | 8.65 | 8.81 | 9.40 | 8.61 | 0.95 | 0.397 | 2.65 | 0.110 | 2.00 | 0.109 |
Table 3
Static balance outcomes
Variables | Aerobic exercise group | Combined group | Health education group | Group | Time | Group | ||||||
---|---|---|---|---|---|---|---|---|---|---|---|---|
Baseline | 24 weeks | Baseline | 24 weeks | Baseline | 24 weeks | F | P | F | P | F | P | |
Left single leg stance | ||||||||||||
OE,Cop-X(mm) | 577.33 | 530.77 | 532.43 | 504.04 | 530.36 | 527.54 | 0.06 | 0.939 | 2.33 | 0.135 | 0.94 | 0.401 |
OE,Cop-Y(mm) | 566.87 | 556.97 | 588.61 | 524.10 | 565.47 | 567.88 | 0.02 | 0.982 | 6.53 | 0.015 | 4.79 | 0.014 |
CE,Cop-X(mm) | 1285.81 | 1183.78 | 1290.78 | 1108.71 | 1304.59 | 1242.11 | 0.51 | 0.604 | 46.36 | 4.19 | 0.022 | |
CE,Cop-Y(mm) | 1357.05 | 1263.32 | 1378.09 | 1128.51 | 1333.04 | 1250.67 | 0.35 | 0.708 | 61.09 | 8.41 | 0.001 | |
Right single leg stance | ||||||||||||
OE,Cop-X(mm) | 539.70 | 528.63 | 526.27 | 484.71 | 542.38 | 544.35 | 0.59 | 0.544 | 2.25 | 0.142 | 1.31 | 0.282 |
OE,Cop-Y(mm) | 548.36 | 541.19 | 541.77 | 519.54 | 540.16 | 549.50 | 0.09 | 0.911 | 0.30 | 0.587 | 0.56 | 0.580 |
CE,Cop-X(mm) | 1350.99 | 1196.43 | 1329.15 | 1136.01 | 1362.55 | 1272.06 | 0.52 | 0.596 | 70.94 | 2.98 | 0.062 | |
CE,Cop-Y(mm) | 1363.69 | 1229.96 | 1374.31 | 1134.68 | 1382.29 | 1289.33 | 0.65 | 0.527 | 87.14 | 0.89 | 0.003 |
Table 4
Dynamic balance outcomes
Variables | Aerobic exercise group | Combined group | Health education group | Group | Time | Group | ||||||
---|---|---|---|---|---|---|---|---|---|---|---|---|
Baseline | 24 weeks | Baseline | 24 weeks | Baseline | 24 weeks | F | P | F | P | F | P | |
OSI | 4.64 (4.25, 6.26) | 4.19 (3.99,4.84) | 4.59 (4.40, 5.71) | 3.78 (3.46, 4.90) | 4.89 (4.55, 4.46) | 4.92 (4.36, 5.34) | 2.09 | 0.137 | 27.78 | 8.34 | 0.001 | |
APSI | 2.87 (2.41, 3.91) | 2.49 (1.98, 2.90) | 3.13 (2.89, 3.32) | 2.57 (2.36, 3.31) | 3.51 (3.06, 3.67) | 3.31 (3.05, 3.63) | 5.06 | 0.011 | 20.55 | 4.13 | 0.024 | |
MLSI | 3.78 (3.24, 4.12) | 3.43 (2.89, 3.77) | 3.39 (3.17, 4.80) | 2.83 (2.39, 3.66) | 3.46 (3.19, 4.12) | 3.46 (3.06, 3.89) | 1.96 | 0.155 | 12.29 | 7.43 | 0.002 |
3.2Outcome of 30-s chair stand
Significant main effects over time and time x group interaction effects were observed for the 30-second chair stand (
3.3Outcome of single-double task gait
There were no significant effects of time, group, or time
3.4Outcome of static balance
A significant time main effect and time x group interaction effects were observed for the COP-Y in the open-eye left leg standing mode (
3.5Outcome of dynamic balance
Significant main effects of time (
4.Discussion
The objective of this study was to examine the impact of two exercise programs that adhered to physical activity guidelines, as well as health education, on lower limb muscle strength, single-dual task gait parameters, and static and dynamic balance function in sedentary older adults who were in good health. The main finding of the study was that after 24 weeks of performing both aerobic and combined aerobic-resistance exercise, lower limb muscle strength, static balance with the eyes closed, and dynamic balance were improved among sedentary older adults. Combined exercise was more effective in improving dual-task gait speed, and medial and lateral dynamic balance.
The sedentary lifestyle can worsen the decline in muscle mass and strength that occurs naturally in older adults, thus exacerbating the progression of age-related sarcopenia [27, 28]. Reduced muscle strength has been identified as a risk factor for higher rates of falls and all-cause mortality in older adults [29, 30]. However, there are distinct regional features of muscle decay in older people, with studies confirming that lower body muscle strength and muscle power decline more rapidly during aging compared to upper body muscle strength [31], suggesting the importance of targeted lower limb training to maintain muscle strength in old age.
Previous research has established that changes in muscle strength resulting from a sedentary lifestyle can be reliably measured using the chair stand test [32], therefore the 30-second chair stand test was chosen to assess changes in muscle strength in sedentary older adults in this study. Our study confirmed that both aerobic and combined aerobic-resistance exercise significantly increased 30-second chair stand performance, suggesting that both exercise programs can improve lower limb muscle strength in sedentary older adults [25]. Sousa et al. confirmed these findings in a study that showed 32 weeks of moderate to vigorous intensity aerobic exercise and combined aerobic-resistance exercise were effective in improving 30-second chair stand performance in older men. However, their results suggested that the combined exercise was more effective in improving the strength of the lower limb muscles of the same group [19]. In a similar vein, Timmons et al. conducted a study to measure lower limb muscle strength quantitatively before and after 12 weeks of aerobic exercise and combined aerobic-resistance exercise in older adults. Their results showed that combined aerobic-resistance exercise was more effective than aerobic exercise alone in improving lower limb strength [20]. Resistance exercise is a well-established strategy for preventing and improving muscle weakness in older individuals [33]. The exercise promotes muscle hypertrophy by augmenting muscle protein synthesis and increasing the cross-sectional area of fast muscle fibers, leading to an enhancement in muscle strength [34, 35]. On the other hand, aerobic exercise enhances mitochondrial ATP production within skeletal muscles, as well as increasing muscle protein synthesis. Moreover, it improves exercise function in general by enhancing aerobic metabolic capacity and increasing both cardiovascular and respiratory endurance [36, 37]. The current study supports that aerobic exercise can induce similar muscle strength gains as combined aerobic-resistance exercise, presumably due to the physiological mechanisms by which it enhances skeletal muscle biology and overall exercise function. However, the reasons for the variation in findings can be multifactorial. One possible cause is the difference in outcomes resulting from gender ratio variations among participants. Our study included older adults of both genders, while Sousa et al.’s study involved a homogeneous group of older males. While both males and females experience age-related declines in muscle mass and strength, the extent of these changes varies based on gender, with females showing a significantly greater proportion of muscle mass and strength loss [38]. Additionally, resistance exercise’s impact on muscle strength is heavily influenced by its intensity and gender; a study by Beneka et al. revealed that men experience more significant strength gains than women with low to moderate-intensity resistance exercise [39]. The decision to mix genders in our study could have contributed to the similar results found between combined aerobic-resistance exercise and aerobic exercise alone. Additionally, it is worth noting that while Timmons et al. utilized an objective instrument to measure lower limb muscle strength, Sousa et al. and our study assessed it through chair stand performance. This indicates that increases in strength and functional abilities among older adults may not necessarily be linear and that strength developments might not entirely translate to functional outcomes. Thus, differences in various factors such as training intensities, participant gender, and measurement methods may have contributed to the differences observed. Overall, our study findings suggest that for sedentary older adults meeting the physical activity guidelines, both aerobic exercise and combined aerobic-resistance exercise offer equivalent enhancements to muscle strength.
In older adults, aging and sedentary lifestyles may lead to the decline of walking function characterized by changes in various gait parameters, such as stride length, stride width, and gait speed. These alterations significantly elevate the risk of falls in this population [40, 41]. Wang et al. reported significant improvements in gait speed and stride length among older adults following a 12-week multi-component exercise program incorporating resistance, endurance, and balance training [42]. Conversely, our study discovered that after 24 weeks of moderate-intensity aerobic and combined aerobic-resistance exercise, there were no significant improvements observed in stride length, stride width, and gait speed among sedentary older adults. These conflicting outcomes could be the result of the variances in the type and intensity of the exercise programs among the studies. Furthermore, as our participants were entirely sedentary and lacked habitual exercise in their daily activities, improving their walking function may require extra time.
Nonetheless, our results validate that combined aerobic-resistance exercise has a significant positive impact on gait speed during dual-task walking patterns among sedentary older adults, while the changes in stride length and width are not noteworthy. In actuality, older adults often walk and simultaneously perform various tasks to meet their daily needs. Studies indicate that older adults with reduced gait speed and increased variability of stride during dual-task walking conditions face an elevated risk of falling in the future [43, 44]. A reduction in dual-task walking performance among older adults is closely linked with a decline in walking-related system function, resulting in an increased reliance of the sensory control system on cognition [45]. Compared to active older adults, sedentary older adults may be at greater risk of experiencing falls due to cognitive and physical functional decline that can occur [46]. Other studies have corroborated these findings, indicating that exercise can enhance dual-task walking ability in older adults by primarily improving gait speed under dual-task conditions. These studies suggest that combined aerobic and resistance exercise confers greater benefits for improving dual-task gait speed [47].
Age-related walking stability is closely associated with the maintenance of balance, which is reliant on inputs from the vestibular, visual, and proprioceptive systems, as well as the integration of these inputs with the central nervous system [48, 49]. Reduced balance function is strongly linked to an increased risk of falls amongst the elderly population [50]. There is strong evidence to suggest that physical exercise can enhance both static and dynamic balance and decrease older adults’ risk of falls [51]. In our study, we examined the impact of two exercise modalities on the static and dynamic balance of sedentary older adults. Specifically, we compared the effects of aerobic exercise and combined aerobic-resistance exercise on participants’ balance while standing with eyes closed and open. Our study found that both types of exercises improved static balance function when performed with eyes closed, but had little impact on static balance with eyes open. This may be due to the fact that our participants were healthy individuals who did not present challenges to their postural control while standing with open eyes, thereby potentially rendering them less sensitive to changes caused by exercise interventions. Conversely, this difference is more pronounced in the eyes-closed standing position, where visual cues are not available to assist postural control and balance. Messier et al. reported similar findings, where they observed differences in postural sway only amongst older adults exhibiting different movement types in the eyes-closed position [52]. The consistent evidence available supports that postural control difficulty among older adults leads to greater dependence on visual input for maintaining body balance. This finding further strengthens the conclusions of our study [53, 54]. However, it is important to note that our study also observed an improvement in static balance in the standing position with eyes closed among participants who received health education, which suggests a potential positive impact of the health education intervention on balance performance. Regarding the effects of the two exercise regimens on dynamic balance, our results demonstrated that both aerobic and resistance training improved dynamic balance function to varying degrees. Additionally, the combined exercise was observed to have a more significant effect in enhancing medial and lateral dynamic balance. The observed overall improvement in dynamic balance following exercise interventions may be attributed to an increase in lower limb muscle strength. Prior studies attest that maintenance of lower limb muscle strength in older adults contributes to the maintenance of effective postural stability during aging [55]. Our results showed that aerobic exercise and combined aerobic resistance exercise could better improve static balance and dynamic balance in sedentary elderly standing with eyes closed.
Overall, our results suggest that aerobic exercise meeting physical activity guidelines and combined aerobic-resistance exercise improve lower limb muscle strength, dynamic balance, and static balance in sedentary elderly standing with eyes closed. Combined aerobic-resistance exercise has an advantage in improving dual-task gait speed in sedentary older adults. Given that sedentary older adults lack motivation to exercise, to ensure that sedentary older adults would benefit maximally at the minimum time threshold for meeting guideline exercise. We recommend that simultaneous aerobic and resistance exercise in sedentary elderly is beneficial in improving motor function and reducing the risk of falls in sedentary elderly in many aspects.
4.1Limitations
Present study has some limitations. Firstly, our sample size was limited. Secondly, all participants were drawn from Asian populations, which may limit the generalizability of our findings to other regions and ethnic groups. Additionally, our intervention method was relatively singular, and future studies may benefit from exploring the use of multi-component exercise programs that include endurance and balance training among sedentary elderly individuals to improve overall motor function.
5.Conclusion
Aerobic and combined aerobic-resistance exercise are beneficial in improving lower limb muscle strength, dynamic balance, and static balance in standing with eyes closed in sedentary older adults. Our study findings suggest that aerobic combined with resistance exercise has a more pronounced positive effect on dual-task gait speed, as well as medial and lateral dynamic balance, when compared to aerobic exercise alone. We suggest that simultaneous aerobic and resistance exercise is beneficial for improving motor function in many aspects and reducing the risk of falls in sedentary elderly.
Funding
Science and Technology Projects in Guangzhou: 202102010007.
Ethics statement
The study was conducted in accordance with the Declaration of Helsinki and approved by the Ethics Committee of Shenyang Sport University (code: 2020[3]). All participants signed an informed consent form.
Author contributions
ZY and XL: Conceptualization, Methodology, Funding acquisition. WZ and HL: Writing-Original draft preparation and Reviewing. XZ, TS: Designed the figures, Visualization and Investigation and Polished the manuscript. All authors read and approved the final version of the manuscript and agree with the order of presentation of the authors.
Acknowledgments
We acknowledge the help of ChatGPT for polishing part of the manuscript.
Conflict of interest
The authors declare that they have no competing interests.
References
[1] | Park JH, Moon JH, Kim HJ, et al. Sedentary Lifestyle: Overview of Updated Evidence of Potential Health Risks. Korean J Fam Med. (2020) ; 41: (6): 365-373. |
[2] | Matthews CE, George SM, Moore SC, et al. Amount of time spent in sedentary behaviors and cause-specific mortality in US adults. Am J Clin Nutr. (2012) ; 95: (2): 437-445. |
[3] | Davis MG, Fox KR, Stathi A, et al. Objectively measured sedentary time and its association with physical function in older adults. Journal of Aging and Physical Activity, (2014) ; 22: (4). |
[4] | Katzmarzyk PT, Powell KE, Jakicic JM, et al. Sedentary Behavior and Health: Update from the 2018 Physical Activity Guidelines Advisory Committee. Med Sci Sports Exerc. (2019) ; 51: (6): 1227-1241. |
[5] | Thomas E, Battaglia G, Patti A, et al. Physical activity programs for balance and fall prevention in elderly: A systematic review. Medicine (Baltimore). (2019) ; 98: (27): e16218. |
[6] | Reid KF, Pasha E, Doros G, et al. Longitudinal decline of lower extremity muscle power in healthy and mobility-limited older adults: influence of muscle mass, strength, composition, neuromuscular activation and single fiber contractile properties. Eur J Appl Physiol. (2014) ; 114: (1): 29-39. |
[7] | Thyfault JP, Du Mengmeng, Kraus WE, et al. Physiology of sedentary behavior and its relationship to health outcomes. Medicine and Science in Sports and Exercise. (2015) ; 47: (6). |
[8] | Reid N, Healy GN, Gianoudis J, et al. Association of sitting time and breaks in sitting with muscle mass, strength, function, and inflammation in community-dwelling older adults. Osteoporos Int. (2018) ; 29: (6): 1341-1350. |
[9] | Gianoudis J, Bailey CA, Daly RM. Associations between sedentary behaviour and body composition, muscle function and sarcopenia in community-dwelling older adults. Osteoporos Int. (2015) ; 26: (2): 571-579. |
[10] | Rosenberg DE, Bellettiere J, Gardiner PA, et al. Independent Associations Between Sedentary Behaviors and Mental, Cognitive, Physical, and Functional Health Among Older Adults in Retirement Communities. The Journals of Gerontology. Series A, Biological Sciences and Medical Sciences, (2016) ; 71: (1). |
[11] | Graafmans WC, Ooms ME, Hofstee HM, et al. Falls in the elderly: a prospective study of risk factors and risk profiles. Am J Epidemiol, (1996) ; 143: (11): 1129-1136. |
[12] | Global Recommendations on Physical Activity for Health Geneva: World Health Organization, (2010) . |
[13] | Chodzko-Zajko WJ, Proctor DN, Fiatarone SM, et al. American College of Sports Medicine position stand. Exercise and physical activity for older adults. Med Sci Sports Exerc. (2009) ; 41: (7): 1510-1530. |
[14] | Bull FC, Al-Ansari SS, Biddle S, et al. World Health Organization 2020 guidelines on physical activity and sedentary behaviour. Br J Sports Med. (2020) ; 54: (24): 1451-1462. |
[15] | Gries KJ, Raue U, Perkins RK, et al. Cardiovascular and skeletal muscle health with lifelong exercise. J Appl Physiol (1985). (2018) ; 125: (5): 1636-1645. |
[16] | Daussin FN, Zoll J, Dufour SP, et al. Effect of interval versus continuous training on cardiorespiratory and mitochondrial functions: relationship to aerobic performance improvements in sedentary subjects. Am J Physiol Regul Integr Comp Physiol. (2008) ; 295: (1): R264-R272. |
[17] | Caruso FR, Arena R, Phillips SA, et al. Resistance exercise training improves heart rate variability and muscle performance: a randomized controlled trial in coronary artery disease patients. Eur J Phys Rehabil Med. (2015) ; 51: (3): 281-289. |
[18] | Sousa N, Mendes R, Abrantes C, et al. Effectiveness of combined exercise training to improve functional fitness in older adults: A randomized controlled trial. Geriatr Gerontol Int. (2014) ; 14: (4): 892-898. |
[19] | Sousa N, Mendes R, Silva A, et al. Combined exercise is more effective than aerobic exercise in the improvement of fall risk factors: a randomized controlled trial in community-dwelling older men. Clin Rehabil. (2017) ; 31: (4): 478-486. |
[20] | Timmons JF, Minnock D, Hone M, et al. Comparison of time-matched aerobic, resistance or concurrent exercise training in older adults. Scandinavian Journal of Medicine & Science in Sports. (2018) ; 28: (11). |
[21] | Letter to the editor: standardized use of the terms “sedentary” and “sedentary behaviours”. Appl Physiol Nutr Metab. (2012) ; 37: (3): 540-542. |
[22] | Akbari KA, Shams A, Shamsipour DP, et al. The effect of low and moderate intensity aerobic exercises on sleep quality in men older adults. Pak J Med Sci. (2014) ; 30: (2): 417-421. |
[23] | Santos-Lozano A, Marin PJ, Torres-Luque G, et al. Technical variability of the GT3X accelerometer. Med Eng Phys. (2012) ; 34: (6): 787-790. |
[24] | Macfarlane DJ, Chou KL, Cheng YH, et al. Validity and normative data for thirty-second chair stand test in elderly community-dwelling Hong Kong Chinese. Am J Hum Biol. (2006) ; 18: (3): 418-421. |
[25] | Jones CJ, Rikli RE, Beam WC. A 30-s chair-stand test as a measure of lower body strength in community-residing older adults. Res Q Exerc Sport. (1999) ; 70: (2): 113-119. |
[26] | Yan ZW, Yang Z, Yang J, et al. Tai Chi for spatiotemporal gait features and dynamic balancing capacity in elderly female patients with non-specific low back pain: A six-week randomized controlled trial. J Back Musculoskelet Rehabil, (2022) . |
[27] | Smith L, Tully M, Jacob L, et al. The Association Between Sedentary Behavior and Sarcopenia Among Adults Aged ⩾ 65 Years in Low- and Middle-Income Countries. Int J Environ Res Public Health, (2020) ; 17: (5). |
[28] | Shad BJ, Wallis G, van Loon LJ, et al. Exercise prescription for the older population: The interactions between physical activity, sedentary time, and adequate nutrition in maintaining musculoskeletal health. Maturitas. (2016) ; 93: : 78-82. |
[29] | Moreland JD, Richardson JA, Goldsmith CH, et al. Muscle weakness and falls in older adults: a systematic review and meta-analysis. J Am Geriatr Soc. (2004) ; 52: (7): 1121-1129. |
[30] | Li R, Xia J, Zhang XI, et al. Associations of Muscle Mass and Strength with All-Cause Mortality among US Older Adults. Med Sci Sports Exerc. (2018) ; 50: (3): 458-467. |
[31] | Hughes VA, Frontera WR, Wood M, et al. Longitudinal muscle strength changes in older adults: influence of muscle mass, physical activity, and health. J Gerontol A Biol Sci Med Sci. (2001) ; 56: (5): B209-B217. |
[32] | Ramsey KA, Rojer A, D’Andrea L, et al. The association of objectively measured physical activity and sedentary behavior with skeletal muscle strength and muscle power in older adults: A systematic review and meta-analysis. Ageing Res Rev. (2021) ; 67: : 101266. |
[33] | Peterson MD, Rhea MR, Sen A, et al. Resistance exercise for muscular strength in older adults: a meta-analysis. Ageing Res Rev. (2010) ; 9: (3): 226-237. |
[34] | Johnston AP, De Lisio M, Parise G. Resistance training, sarcopenia, and the mitochondrial theory of aging. Appl Physiol Nutr Metab. (2008) ; 33: (1): 191-199. |
[35] | Jun WH, Mi HN, Dong HM, et al. Aging-induced Sarcopenia and Exercise. The Official Journal of the Korean Academy of Kinesiology. (2017) ; 19: (2). |
[36] | Konopka AR, Douglass MD, Kaminsky LA, et al. Molecular adaptations to aerobic exercise training in skeletal muscle of older women. J Gerontol A Biol Sci Med Sci. (2010) ; 65: (11): 1201-1207. |
[37] | Erlich AT, Tryon LD, Crilly MJ, et al. Function of specialized regulatory proteins and signaling pathways in exercise-induced muscle mitochondrial biogenesis. Integr Med Res. (2016) ; 5: (3): 187-197. |
[38] | A. A, G. G, M. H, et al. Muscle morphology, enzyme activity and muscle strength in elderly men and women. Clinical Physiology. (1981) ; 1: (1). |
[39] | Beneka A, Malliou P, Fatouros I, et al. Resistance training effects on muscular strength of elderly are related to intensity and gender. J Sci Med Sport. (2005) ; 8: (3): 274-283. |
[40] | Hausdorff JM, Rios DA, Edelberg HK. Gait variability and fall risk in community-living older adults: a 1-year prospective study. Arch Phys Med Rehabil. (2001) ; 82: (8): 1050-1056. |
[41] | Koblinsky ND, Atwi S, Cohen E, et al. Lower Thalamic Blood Flow Is Associated With Slower Stride Velocity in Older Adults. Front Aging Neurosci. (2020) ; 12: : 571074. |
[42] | Wang RY, Wang YL, Cheng FY, et al. Effects of combined exercise on gait variability in community-dwelling older adults. Age (Dordr). (2015) ; 37: (3): 9780. |
[43] | Priest AW, Salamon KB, Hollman JH. Age-related differences in dual task walking: a cross sectional study. J Neuroeng Rehabil. (2008) ; 5: : 29. |
[44] | Hollman JH, Kovash FM, Kubik JJ, et al. Age-related differences in spatiotemporal markers of gait stability during dual task walking. Gait Posture. (2007) ; 26: (1): 113-119. |
[45] | Nordin E, Moe-Nilssen R, Ramnemark A, et al. Changes in step-width during dual-task walking predicts falls. Gait Posture. (2010) ; 32: (1): 92-97. |
[46] | Marie T, Frédéric B, Caroline T, et al. Impact of physical activity and sedentary behaviour on fall risks in older people: a systematic review and meta-analysis of observational studies. European Review of Aging and Physical Activity. (2012) ; 9: (1). |
[47] | Plummer P, Zukowski LA, Giuliani C, et al. Effects of Physical Exercise Interventions on Gait-Related Dual-Task Interference in Older Adults: A Systematic Review and Meta-Analysis. Gerontology: International Journal of Experimental and Clinical Gerontology. (2016) ; 62: (1). |
[48] | Cromwell RL, Newton RA. Relationship between balance and gait stability in healthy older adults. J Aging Phys Act. (2004) ; 12: (1): 90-100. |
[49] | Peterka RJ. Sensorimotor integration in human postural control. J Neurophysiol. (2002) ; 88: (3): 1097-1118. |
[50] | Salzman B. Gait and balance disorders in older adults. American family physician. (2010) ; 82: (1). |
[51] | Giuseppe FP, Rocco P, Lorenzo ADB, et al. The Effects of Physical Exercise on Balance and Prevention of Falls in Older People: A Systematic Review and Meta-Analysis. Journal of Clinical Medicine. (2020) ; 9: (8). |
[52] | Messier SP, Royer TD, Craven TE, et al. Long-term exercise and its effect on balance in older, osteoarthritic adults: results from the Fitness, Arthritis, and Seniors Trial (FAST). J Am Geriatr Soc. (2000) ; 48: (2): 131-138. |
[53] | Pyykkö I, Jäntti P, Aalto H. Postural control in elderly subjects. Age and ageing. (1990) ; 19: (3). |
[54] | Hay L, Bard C, Fleury M, et al. Availability of visual and proprioceptive afferent messages and postural control in elderly adults. Exp Brain Res. (1996) ; 108: (1): 129-139. |
[55] | Pizzigalli L, Filippini A, Ahmaidi S, et al. Prevention of falling risk in elderly people: the relevance of muscular strength and symmetry of lower limbs in postural stability. J Strength Cond Res. (2011) ; 25: (2): 567-574. |