Unlocking the Potential of Repetitive Transcranial Magnetic Stimulation in Alzheimer’s Disease: A Meta-Analysis of Randomized Clinical Trials to Optimize Intervention Strategies
Abstract
Background:
Repetitive transcranial magnetic stimulation (rTMS) is an advanced and noninvasive technology that uses pulse stimulation to treat cognitive impairment. However, its specific effects have always been mixed with those of cognitive training, and the optimal parameter for Alzheimer’s disease (AD) intervention is still ambiguous.
Objective:
This study aimed to summarize the therapeutic effects of pure rTMS on AD, excluding the influence of cognitive training, and to develop a preliminary rTMS treatment plan.
Methods:
Between 1 January 2010 and 28 February 2023, we screened randomized controlled clinical trials from five databases (PubMed, Web of Science, Embase, Cochrane, and ClinicalTrials. gov). We conducted a meta-analysis and systematic review of treatment outcomes and rTMS treatment parameters.
Result:
A total of 4,606 articles were retrieved. After applying the inclusion and exclusion criteria, 16 articles, comprising 655 participants (308 males and 337 females), were included in the final analysis. The findings revealed that rTMS significantly enhances both global cognitive ability (p = 0.0002, SMD = 0.43, 95% CI = 0.20–0.66) and memory (p = 0.009, SMD = 0.37, 95% CI = 0.09–0.65). Based on follow-up periods of at least 6 weeks, the following stimulation protocols have demonstrated efficacy for AD: stimulation sites (single or multiple targets), frequency (20 Hz), stimulation time (1–2 s), interval (20–30 s), single pulses (≤2500), total pulses (>20000), duration (≥3 weeks), and sessions (≥20).
Conclusions:
This study suggests that rTMS may be an effective treatment option for patients with AD, and its potential therapeutic capabilities should be further developed in the future.
INTRODUCTION
Alzheimer’s disease (AD) is a neurodegenerative disease characterized by a gradual decline in cognitive ability and deterioration of daily life skills. It is the most common type of dementia, accounting for 50–70% of cases, and is primarily characterized by severe memory impairment relative to age-matched peers [1]. The global incidence of AD and its continuum, encompassing prodromal AD and preclinical AD, is estimated to affect 32, 69, and 315 million individuals, respectively. Collectively, this accounts for a significant population of 416 million individuals within the AD spectrum, representing approximately 22% of the global population aged≥50 years [2]. In the geriatric cohort, AD accounts for 4.9% of the total mortality and poses an additional 40% heightened risk of mortality, specifically among female individuals [3]. With advancing age, the susceptibility to AD increases, accompanied by a parallel rise in disease burden [4] and mortality risks [2, 5]. Consequently, there is an imperative demand for an efficacious therapeutic approach to combat AD.
However, the underlying mechanisms of AD are not fully understood. Currently, drug therapies for AD primarily focus on the inhibition of acetylcholinesterase activity within the brain [6]. Pharmacological approaches primarily focus on vascular prevention and symptomatic treatment utilizing cholinesterase inhibitors and N-methyl-D-aspartic acid antagonists [7, 8]. Although more than 20 compounds have completed large-scale phase III double-blind randomized controlled trials in patients with various stages of AD by 2019, none have demonstrated efficacy in slowing cognitive decline or improving overall functioning [9].
Repetitive transcranial magnetic stimulation (rTMS) can be administered as a repetitive stimulation sequence at different frequencies, with stimuli delivered to the same or different brain regions at varying intervals. A single pulse can depolarize neurons and elicit measurable effects, whereas rTMS can modulate the excitability of the cortex at stimulated sites and in remote areas through functional anatomical connections [10]. A pulsed magnetic field can be employed to generate an electric current in the brain, which can temporarily stimulate or inhibit specific areas[11]. The mechanisms underlying the after-effects of rTMS resemble the phenomena of long-term potentiation (LTP) and long-term depression observed in animals [12]. LTP is induced when a presynaptic neuron is stimulated before a postsynaptic neuron (pre-post) within a time interval of tens of milliseconds. Conversely, stimulation in the reverse order (post–pre) led to long-term depression. No alterations in synaptic strength were observed when the inter-stimulus interval exceeded 100 ms [13]. In conclusion, TMS is a potent tool for shaping cortical networks and enhancing the performance of healthy individuals [14]. Several studies have documented substantial improvements in speed and accuracy across tasks related to perception, motion, and executive processing following TMS. This improvement primarily stems from the direct modulation of cortical regions or networks, facilitating more efficient processing [14]. Additionally, this approach involves addition and subtraction, which disrupt competitive or distracting processes that may otherwise impede task performance [14].
Therefore, rTMS has been widely used to treat AD [15]. Research indicates that after rTMS treatment, motor cortex excitability increases in AD groups, while cholinergic function decreases, and both GABAergic and glutamatergic functions in AD patients diminish [16]. Furthermore, the majority of studies suggest a general reduction in the resting motor threshold in individuals with AD compared to healthy age-matched subjects. This reduction is often interpreted as a marker of heightened motor cortex excitability [17–20]. Moreover, increased motor excitability and cortical reorganization in AD may explain the frontomedial shift in motor areas, which is interpreted as a compensatory mechanism that preserves motor programming despite AD progression [21]. Most studies have shown that rTMS can effectively enhance the cognition [22, 23], emotion [24], and language [25–27] in patients with AD. However, other studies have provided different perspectives. While improvements in cognition can be observed using the Alzheimer’s Disease Assessment Scale-Cognition Subscale (ADAS-cog), significant enhancement in global cognition is not evident when assessed by the Min-Mental State Examination (MMSE) [28, 29]. One study revealed a strong correlation between the MMSE total score and ADAS-Cog, but a weaker correlation between the change scores of MMSE and ADAS-Cog [30]. Additionally, another investigation indicated fluctuations in ADAS-Cog scores corresponding to specific MMSE scores, suggesting that ADAS-Cog is more accurate than MMSE in assessing the extent of cognitive impairment [31]. While some studies have shown no significant changes in memory [32], emotion, or executive function [28, 33], others have demonstrated improvements in these cognitive abilities [34–36]. Consequently, there is considerable controversy regarding the efficacy of rTMS for the treatment of AD. Therefore, the present study was essential to reassess the therapeutic efficacy of rTMS.
Distinguishing the therapeutic effect of rTMS in patients with AD from those of cognitive training (CT) presents a challenge. To date, CT has been demonstrated to independently enhance cognitive abilities [37], slow cognitive decline for up to five years [38], and yield moderate effects with significant overall improvements in the behavioral and functional aspects of mild-to-moderate AD [39, 40]. However, most meta-analyses of the effects of rTMS on AD have not excluded articles on rTMS combined with CT [22, 23, 41]. Consequently, the potential benefits of CT have not been excluded from these meta-analyses. This inclusion complicates the differentiation between the effects of rTMS and CT, making it challenging to isolate the individual effects of rTMS alone. Determining whether improvements in cognitive ability arise from rTMS or CT becomes challenging. Therefore, this study aimed to investigate the specific role of rTMS in patients with AD, excluding the influence of CT, to gain a clearer understanding of the individual impact of rTMS.
Additionally, the therapeutic efficacy of rTMS is influenced by various factors such as the location of stimulation, frequency, and pulse count. For instance, some studies have suggested that high-frequency rTMS is more effective compared to low-frequency rTMS in enhancing the cognitive abilities of patients with AD [42, 43]. The use of multiple targets can result in a more pronounced improvement than using a single target [24, 44]. Low-frequency rTMS has also been suggested to improve memory function in patients with AD [45]. Therefore, further studies are required to clarify rTMS parameters. In addition, most studies determined the rTMS stimulation plan based on guidelines [46–48] and their expertise. Thus, establishing an optimal TMS stimulation plan in practice remains challenging for most studies and treatments. The third objective was to comprehensively summarize the parameters employed in rTMS intervention based on existing randomized controlled trials (RCT), meta-analyze the impact of these parameters on final intervention outcomes, and ultimately derive the optimal parameters for rTMS intervention in cognitive impairment.
MATERIALS AND METHODS
The study was designed in accordance with the Preferred Reporting Items for Systematic Reviews and Meta-Analyses (PRISMA) guidelines outlined by Liberati [49]. Moreover, the study was prospectively registered at PROSPERO (ID: CRD42023421243) to ensure transparency and adherence to rigorous research standards.
Searching strategy for literature
The systematic literature search was conducted rigorously using five major databases (PubMed, Web of Science, Embase, Cochrane, and Clinical Trails.gov) and was independently performed by two experienced researchers (LXY and LYM). The search period spanned from 1 January 2010 to 28 February 2023 and specific keywords were used to ensure the inclusion of only relevant articles: (“Cognitive Dysfunction” OR “Cognitive Impairment” OR “AD” OR “Alzheimer”) AND (“Transcranial Magnetic Stimulation” OR “TMS” OR “Repetitive Transcranial Magnetic Stimulation” OR “rTMS”) AND (“Randomized Controlled Trial” OR “Randomized” OR “Placebo”). The keywords for the randomized controlled trials were obtained from the McMaster Health Knowledge Refinery (HKR, https://hiruweb.mcmaster.ca/hkr/hedges/medline), a trusted source that provides a balance between sensitivity and specificity. In cases of discrepancies between the two researchers, a senior investigator was consulted to determine the inclusion or exclusion of articles based on pre-defined criteria, thereby ensuring that the final list of articles was thoroughly screened and reviewed.
Inclusion and exclusion criteria
Specific inclusion and exclusion criteria were formulated to ensure the homogeneity of studies included in this review. The specific inclusion criteria were formulated as follows: 1) participants must be human; 2) studies must be randomized controlled trials focused on AD; 3) research must solely involve rTMS treatment without combing it with other interventions; 4) the mode of stimulation was rTMS; 5) studies must include an intervention group receiving real rTMS treatment and a control group that received sham rTMS treatment; 6) sufficient pre- and post- original data (including available mean and standard deviation); 7) outcomes should be measured using neuropsychological scales, E-Prime trails, or other objective measures.
Conversely, studies were excluded if they: 1) focused on cognitive impairment resulting from other diseases (such as Parkinson’s disease, cerebral trauma, stroke, etc.); 2) utilized stimulation modes other than rTMS (such as intermittent theta burst stimulation or other TMS technologies); 3) were based on animal research; 4) did not evaluate cognition or only included EEG, MRI, or other physiological indices; 5) were published in languages other than English; 6) were in the form of case reports, comments, letters, or review.
Information of included studies
A total of 4,606 articles were identified from five databases (Web of Science, 704; Cochrane, 3713; PubMed, 57; Embase, 129; and CT.gov, 3). A total of 293 duplicate records were excluded. Subsequently, 4,270 articles were excluded for various reasons, including not meeting the inclusion and exclusion criteria, involving alternative treatment methods, focusing solely only on healthy individuals, etc. This left only 43 articles on the topic. Upon full-text review, 13 were excluded due to the absence of a sham group, seven were excluded for not employing a single rTMS method, six were excluded for presenting incomplete data, and one was excluded for using the intermittent theta burst stimulation method. Finally, 16 studies were included in this meta-analysis. The filtering process is illustrated in Fig. 1.
Fig. 1
Flow chart depicting the inclusion and exclusion process for the meta-analysis.
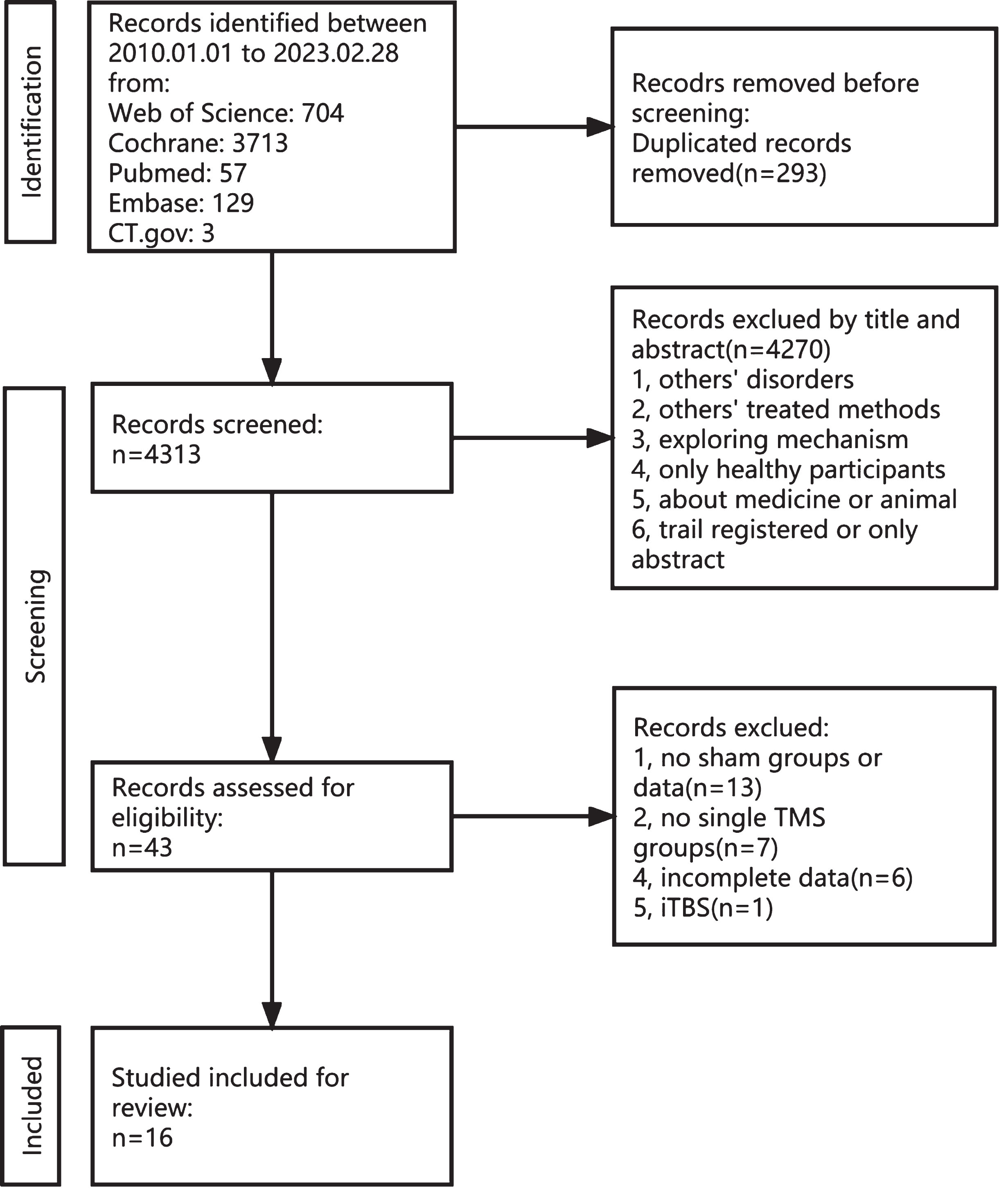
Data extraction
Data extraction from each included study was conducted independently by two researchers who met the predefined inclusion and exclusion criteria. The extracted information was comprehensive and covered a variety of aspects, such as the study’s basic characteristics, including the first author, publication year, group size, sex ratio, age, education level, diagnostic criteria, baseline cognitive scores, and dropout rate. Additionally, the study design, duration, pre- and post-outcome measures (mean [M] and standard deviation [SD]), adverse events, and treatment interventions were meticulously recorded, including the mode of TMS, type of coil, stimulus intensity, stimulating position and number, frequency, duration per trial, interval time per trial, total time per treatment, single pulses per treatment, total pulses for all treatments, treatment frequency, treatment period, sessions, and the sham stimulation method.
During cross-design studies, data were consolidated when the participants received identical stimulations during the same stage. Conversely, in parallel studies, the data were aggregated across different groups during both the pre-and post-stages. If data from the same study were available in multiple databases, the database with the most extensive information and the highest number of participants was selected. In instances where outcomes were reported at distinct time points, data were promptly obtained following the selection of the interventions. In cases where the studies lacked adequate or ambiguous information, the corresponding authors were approached for clarification. If the information was still not clear, the WebPlot Digitiser tool (https://apps.automeris.io/wpd/) was used to measure the graph or article. Based on the original axis, line chart, or bar chart, this tool can measure approximate raw data for subsequent analysis. Any disparities in the data retrieved by the two researchers were resolved through active discussions with a third professional researcher to ensure consensus.
Study quality
The quality of the included studies was assessed using the evaluation criteria of the Cochrane risk of bias tool, which is widely accepted in the field [50]. The evaluations were conducted independently by two researchers, and any discrepancies were resolved by inviting a third researcher to review the studies. Quality assessment focused on seven key aspects: random sequence generation, allocation concealment, blinding of participants and implementers, blinding of outcome assessment, integrity of results, selective reporting, and other biases. For each aspect, the risk of bias was categorized into three levels: low, high, and unclear. This approach ensured a comprehensive and rigorous evaluation of the quality of included studies.
Data analysis
The Cochrane Review Manager (RevMan) 5.4 statistical software (Cochrane Collaboration Network Review Manager, Oxford, UK) was employed for data analysis. The effect size was calculated using the Standard Mean Difference (SMD), and 95% confidence intervals (CIs) were assessed using the Z-test. The heterogeneity of a study was calculated using the I2 test: If the I2 < 50%, indicating low heterogeneity, a fixed-effect model was employed; conversely, if I2 ≥50%, suggesting high heterogeneity, a random-effects model was used to analyze the treatment effect size. According to the Cochrane Manual for Systematic Evaluation of Inventions, STATA software (version 17.0; STATA Corp., College Station, TX, USA) was used to perform Begg’s and Egger’s tests to create funnel plots and analyze the size of the publication bias of each article.
The change in treatment was determined as the difference between the baseline mean value before treatment and that immediately after treatment. The maintenance effect of treatment is reflected by the difference between the baseline mean value and the follow-up. The formula used in this study is as follows:
(1)
(2)
In all analyses, p < 0.05 was considered statistically significant.
RESULTS
Basic characteristics
This meta-analysis included 16 studies involving 655 patients with AD. Of the participants, 308 were male and 337 were female, with a mean age of 71.53 years. The average disease duration was 3.57 years, and the average length of education was 8.29 years. Most studies used diagnostic criteria such as the National Institute of Neurological and Communicative Disease and Stroke and Alzheimer’s Disease and Related Disorders Association (NINCDS-ADRDA), the National Institute on Aging and Alzheimer Association (NIA-AA), and the Diagnostic and Statistical Manual of Mental Disorders (DSM). The details are presented in Table 1.
Table 1
Basic characteristics of included studies
Study | Design | Group | Participants (total) | Gender (M/F) | Age (y) | Disease Duration | Education (y) | Diagnosis Criteria |
Ahmed (2012) [43] | parallel | rTMS-20 HZ rTMS -1 HZ Sham | 45 | 5/10 6/9 5/10 | 65.9 (5.9) 68.6 (6.7) 68.3 (4.9) | 3.9 (2.3) 4.1 (2.3) 4.4 (2.5) | NA | NINCDS-ADRDA |
Cotelli (2010) [25] | parallel | rTMS Sham | 10 | NA | 71.2 (6.1) 74.4 (3.8) | NA | 6.4 (1.3) 4.8 (0.4) | NINCDS-ADRDA |
Hu (2022) [59] | parallel | rTMS Sham | 42 | 8/13 10/11 | 76.86 (6.07) 75.33 (5.73) | 3.52 (1.67) 3.88 (2.01) | 13.05 (3.67) 11.24 (4.39) | NIA-AA |
Jia (2021) [58] | parallel | rTMS Sham | 69 | 10/25 11/23 | 71.41 (8.85) 73.41 (7.73) | NA | 7.70 (5.26) 7.50 (5.19) | DSM-V |
Koch (2022) [60] | parallel | rTMS Sham | 50 | 11/14 13/12 | 75.0 (5.6) 72.3 (7.2) | 1.4 (0.33) 1.2 (0.33) | 10.2 (4.4) 8.6 (4.1) | IWG-2 |
Li (2021) [51] | parallel | rTMS Sham | 75 | 20/17 24/14 | 65.97 (8.47) 64.58 (7.88) | 3.7 (1.75) 3.97 (1.62) | 5.65 (3.21) 6.75 (4.51) | DSM-V |
Lu (2022) [52] | parallel | rTMS Sham | 55 | 19/8 19/9 | 69.2 (7.1) 73.7 (7.2) | NA | 7.3 (4.4) 7.5 (5.2) | DSM-V |
Padala (2020) [53] | parallel | rTMS Sham | 20 | 8/1 10/1 | 74.3 (5.7) 79.6 (7.7) | NA | NA | UBACC > 15, age > 55, AES-C > 30, MMSE > 18 |
Rutherford (2015) [54] | cross-over | rTMS/Sham | 10 | 4/6 | 57–87 | NA | NA | Clinical/neuropsychological criteria for AD |
Saitoh (2022) [55] | parallel | rTMS-120% RMT rTMS-90% RMT Sham | 40 | 8/7 3/10 4/8 | 76.2 77.2 75.8 | 3.5 4.8 4.6 | 13.7 12.2 13.2 | NINCDS-ADRDA |
Wei (2022) [36] | parallel | rTMS Sham | 56 | 9/20 7/20 | 70.00 (8.63) 71.67 (7.16) | NA | 7.34 (5.54) 6.63 (4.99) | DSM-V |
Wu (2015) [56] | parallel | rTMS Sham | 52 | 16/10 15/11 | 71.4 (4.9) 71.9 (4.8) | 5.1 (1.5) 5.1 (1.5) | 11.4 (2.7) 11.5 (2.1) | NINCDS-ADRDA |
Yao (2022) [61] | parallel | rTMS Sham | 27 | 8/7 6/6 | 63.87 (6.85) 67.60 (7.88) | 1.97 (1.29) 2.02 (1.26) | 10.53 (3.80) 9.40 (3.63) | NIA-AA |
Zhao (2017) [34] | parallel | rTMS Sham | 30 | 7/10 6/7 | 69.3 (5.8) 71.4 (5.2) | NA | 4.8 (1.9) 4.9 (3.5) | DSM-VI |
Leocani (2021) [62] | parallel | rTMS Sham | 28 | 9/7 6/6 | 69.6(7.9) 72.6 (8.3) | 4.2 (2.0) 4.2 (1.1) | 9.2 (4.5) 7.8 (3.4) | NINCDS-ADRDA |
Tao (2022) [57] | parallel | rTMS Sham | 46 | 11/12 10/13 | 67.8(5.4) 68.9(4.9) | NA | 4.7 (2.1) 4.6 (3.4) | DSM-IV, NINCDS-ADRDA |
M, male; F, female; AD, Alzheimer’s Disease; NINCDS-ADRDA, National Institute of Neurological and Communicative Disease and Stroke and Alzheimer’s Disease and Related Disorders Association; NIA-AA, National Institute on Aging and Alzheimer’s Association; DSM, Diagnostic and Statistical Manual of Mental Disorders; IWG, International Working Group; UBACC, UCSD Brief Assessment of Capacity to Consent; AES-C, Apathy Evaluation Scale-clinician version; MMSE, Mini-Mental State Examination.
In this study, the treatment methods were comprehensively analyzed, and all relevant details potentially influencing outcomes were extracted from each study. The intensities in most studies were 90%, 100%, and 120% of the motor threshold. Among the various stimulation sites explored, the dorsolateral prefrontal cortex (DLPFC) emerged as a frequently targeted region across multiple studies [25, 43, 51–57]. Additionally, other targets included the hippocampus [36, 58], angular gyrus [59], precuneus [60], cerebellum [61], and regions adjacent to the temporal lobe [34, 62]. While the majority of studies utilized one or two stimulation sites with treatment frequency of 10 Hz and 20 Hz, two studies utilized low-frequency stimulation at 1 Hz [43] and 5 Hz [61]. Most of the included studies utilized a stimulation time of 2–5 s, interval of 20–30 s, stimulation duration of 20–30 min, single pulses of 1000–1500, and total pulses of 20000–40000. The treatment frequency was typically five times per week, with a total treatment duration of 2–4 weeks and 30 sessions. Global cognition was measured in almost every study using tools such as the MMSE, Montreal Cognitive Assessment (MoCA), and ADAS-Cog, with the exception of four studies. Further details are provided in Table 2.
Table 2
Treatment methods of included studies
Study | Intensity (% MT) | Stimulation sites | Site numbers | Frequency | Stimulation time | Interval | Stimulation duration | Single pulses | Total pulses | Treatment frequency | Duration | Sessions | Main results |
Ahmed (2012) [43] | 90 | B-DLPFC | 2 | 20 HZ | 5 s | 25 s | 20 min | 4,000 | 20,000 | 5/per week | 5 days | 5 | MMSE, GDS, IADL |
100 | 1 HZ | 5 s | 30 s | 33 min | |||||||||
Cotelli (2010) [25] | 100 | L-DLPFC | 1 | 20 HZ | 2 s | 28 s | 25 min | 2,000 | 20,000 | 5/per week | 2 weeks | 10 | MMSE, ADL, IADL |
Hu (2022) [59] | 90 | B-AG | 2 | 40 HZ | 2 s | 58 s | 30 min | 2,400 | 28,800 | 3/per week | 4 weeks | 12 | MMSE, ADAS-cog |
Jia (2021) [58] | 100-110 | Hippocampus1 | 1 | 10 HZ | 2 s | 28 s | 20 min | 800 | 8,000 | 5/per week | 2 weeks | 10 | MMSE, CDR, PVLT |
Koch (2022) [60] | 100 | Precuneus | 1 | 20 HZ | 2 s | 28 s | 20 min | 1,600 | 51,200 | first 2 weeks, 5/per week; last 22 weeks, 1/per week | 24 weeks | 32 | MMSE, CDR, ADAS-cog, ADCS-ADL, FAB, NPI |
Li (2021) [51] | 100 | L-DLPFC | 1 | 20 HZ | 1 s | 10 s | 20 min | 2,000 | 60,000 | 5/per week | 6 weeks | 30 | MMSE, ADAS-cog |
Lu (2022) [52] | 120 | L-DLPFC | 1 | 10 HZ | 5 s | 25 s | 15 min | 1,500 | 22,500 | 5/per week | 3 weeks | 15 | MoCA, CSDD, CES-D |
Padala (2020) [53] | 120 | L-DLPFC | 1 | 10 HZ | 4 s | 26 s | 37.5 min | 3,000 | 60,000 | 5/per week | 4 weeks | 20 | MMSE, AES, IADL, ADL, 3MS, TMT-A, TMT-B, CGI-S, ZBS |
Rutherford (2015) [54] | 90–100 | B-DLPFC | 2 | 20 HZ | 2 s | 5 s | 5.83 min | 2,000 | 26,000 | first 2 weeks: 5/per week; last 2 weeks: total 3 times | 4 weeks | 13 | ADAS-cog, RMBC, Associative memory, Word-image Associate |
Saitoh (2022) [55] | 120 90 | B-DLPFC | 2 | 10 HZ | 4 s | 26 s | 20 min | 1,200 | ≥6,000 | first 2 weeks:≥8 times; last 2 weeks:≥1 time /per week | 4 weeks | ≥10 | MMSE, ADAS-cog, MOCA, NPI, CDR, EQ-5D-5L |
Wei (2022) [36] | 100–110 | Parietal-hippocampal2 | 1 | 10 HZ | 2 s | 28 s | 20 min | 800 | 8,000 | 5/per week | 2 weeks | 10 | MMSE, PVLT, CDR, ADL, PHQ-9 |
Wu (2015) [56] | 80 | L-DLPFC | 1 | 20 HZ | NA | NA | NA | 1,200 | 24,000 | 5/per week | 4 weeks | 20 | ADAS-cog, BEHAVE-AD |
Yao (2022) [61] | 90 | Bilateral cerebellum | 2 | 5 HZ | NA | NA | 20 min | 2,000 | 40,000 | 5/per week | 4 weeks | 20 | MMSE, MOCA, ADAS-cog, RAVLT, CDT, BNT, VFT, TMT, SDMT, DST |
Zhao (2017) [34] | NA | Parietal P3/P4, posterior temporal T5/T6 | 4 (3/per day) | 20 HZ | 10 s | 20 s | 30 min | 12,000 | 360,000 | 5/per week | 6 weeks | 30 | ADAS-cog, MMSE, MOCA, WHO-UCLA AVLT |
Leocani (2021) [62] | 120 | Bilateral frontal parietal-temporal regions | NA | 10 HZ | NA | 22 s | NA | 840 | 13,440 | first 4 weeks: 3/per week; last 4 weeks: 1/per week | 8 weeks | 16 | ADAS-cog |
Tao (2022) [57] | 100 | L-DLPFC | 1 | 20 HZ | 2 s | 25 s | 20 min | 1,760 | 52,800 | 5/per week | 6 weeks | 30 | MMSE, ADAS-cog, MoCA, MBI |
1Actually, the hippocampus was taken as the seed, and the left lateral parietal showed high functional connectivity with the left hippocampus seed was chosen as the stimulation site. 2Actually, the hippocampus was taken as the seed, and the left lateral parietal showed high functional connectivity with the left hippocampus seed was chosen as the stimulation site. B-DLPFC, Bilateral Dorsolateral Prefrontal Cortex; L-DLPFC, Left Dorsolateral Prefrontal Cortex; R-DLPFC, Right Dorsolateral Prefrontal Cortex; B-AG, Bilateral Angular Gyrus; MMSE, Mini Mental State Examination; IADL, Instrumental Daily Living Activity; GDS, Geriatric Depression Scale; DST, Digit Span Test; WMS, Wechsler Memory Scale; VMPT, Oktem Verbal Memory Process Test; BNT, Boston Naming Test; BFRT, Benton Facial Recognition Test; Stroop; CDT, Clock Drawing Test; NPI, Neuropsychiatric Inventory Questionnaire; FBI, Frontal Behavioral Inventory; BBT, Berg Balance Test; TUG, Timed Up and Go Test; QoL-AD, Quality of Life in Alzheimer’s Disease; ADL, Activities of Daily Living; MoCA, Montreal Cognitive Assessment; ROCF, Rey-Osterrieth Complex Figure task; ST-TIME, Stroop time; DIG-DET, Digit Detection; IWI, Interview With Informant; ADAS-cog, Alzheimer’s Disease Assessment Scale-cognitive subscale; CDR, Clinical Dementia Rating; PVLT, 12-Word Philadelphia Verbal Learning Test; RAVLT, Ray Auditory Verbal Learning Test; DSST, Digit Symbol Substitution Test; FAB, Frontal Assessment Battery; ADCS-ADL, Alzheimer’s Disease Cooperative Study–Activities of Daily Living; CES-D, Centre for Epidemiologic Studies Depression Scale; CSDD, Cornell Scale for Depression in Dementia; 3MS, Modified Mini Mental State Exam; AES, Apathy Evaluation Scale; TMT-A, Trails Making Test A; TMT-B, Trails Making Test B; CGI-S, Cognitive Global Impression –Severity; ZBS, Zarit Burden Scale; EXIT-25, Executive Function –25; RMBC, Revised Memory and Behavior Checklist; EQ-5D-5 L, EuroQOL 5 dimensions 5-level; PHQ-9, Patient Health Questionnaire-9; BEHAVE-AD, Behavioral Pathology in Alzheimer’s Disease Rating Scale; VFT, Verbal Fluency Test; SDMT, Symbol Digit Modalities Test; WHO-UCLA AVLT, The Chinese version of World Health Organization University of California-Los Angeles, Auditory Verbal Learning Test; RMBT, Rivermead Behavioural Memory Test; MBI, Modified Barthel Index.
Quality of included studies
The quality of the included studies was evaluated independently by two researchers using the RevMan 5.4. As illustrated in Figs. 2 and 3, with the exception of one study [54], most included studies were randomized controlled. A few studies did not report their allocation concealment methods or outcome indicators. Overall, the quality of all the included studies was relatively good.
Fig. 2
Risk of bias based on Cochrane’s Handbook.
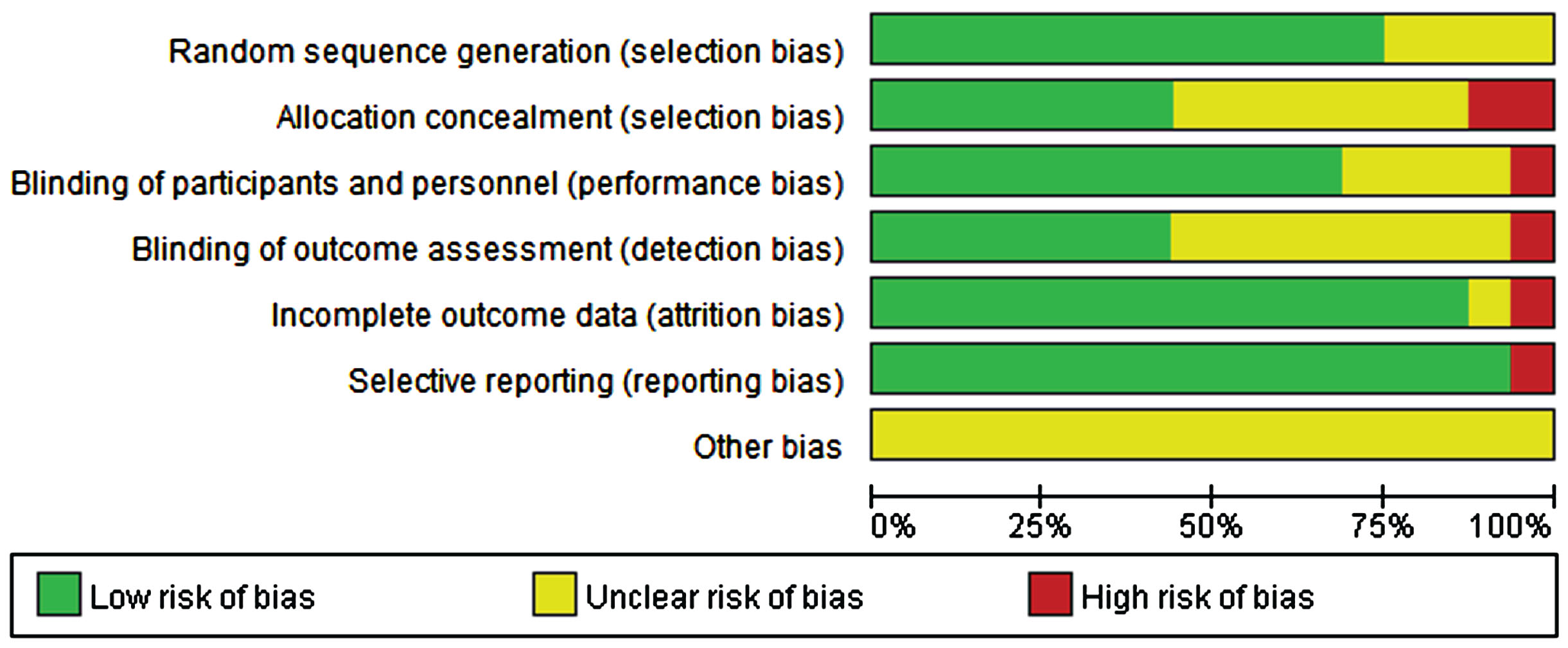
Fig. 3
Risk of bias in every study evaluated by researchers.
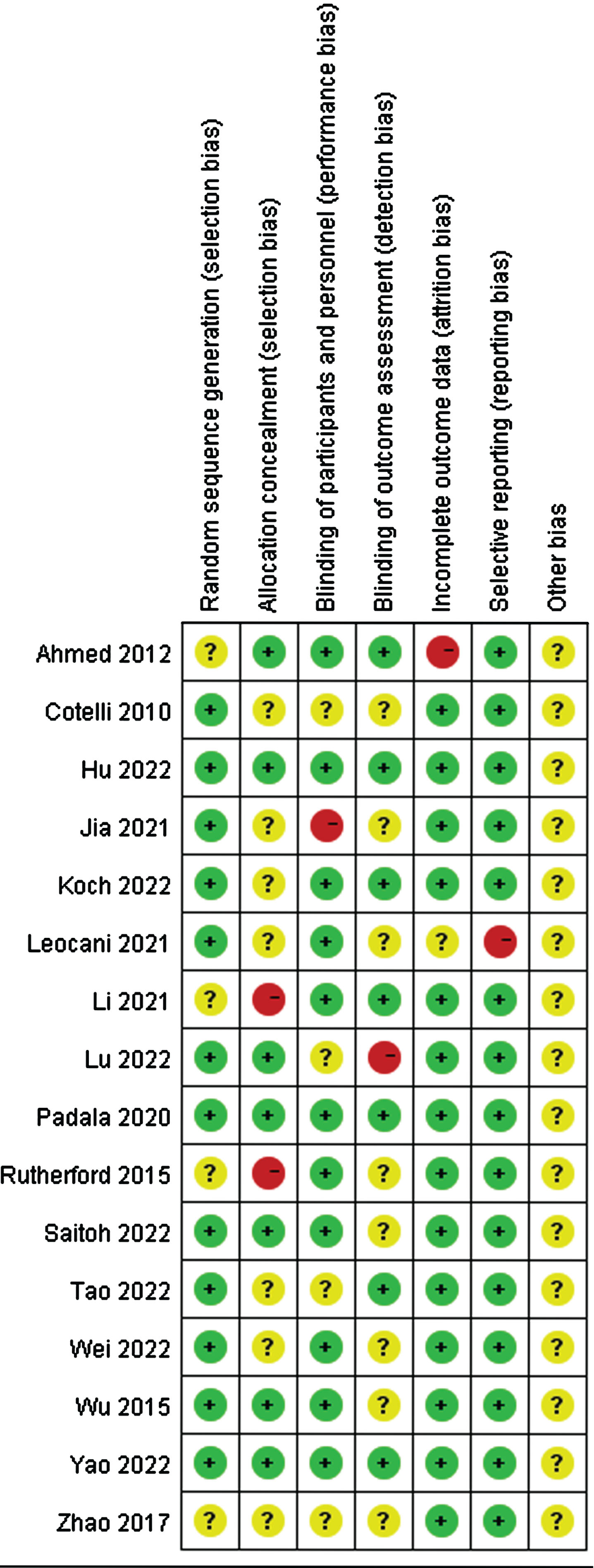
Effects of RTMS on global cognition
All scales across the studies that assessed global cognition, primarily the MMSE, MoCA, and ADAS-cog, were combined. Given an I2 value of 50%, indicating moderate heterogeneity, a random-effects model was employed. A total of 350 experimental and 310 sham participants were included in the study. Results indicated that participants with cognitive impairment exhibited a significant improvement in cognition with rTMS treatment compared to sham stimulation (p = 0.0002, SMD = 0.43, 95% CI = 0.20–0.66) (Fig. 4).
Fig. 4
Investigating the global cognitive effects of rTMS.
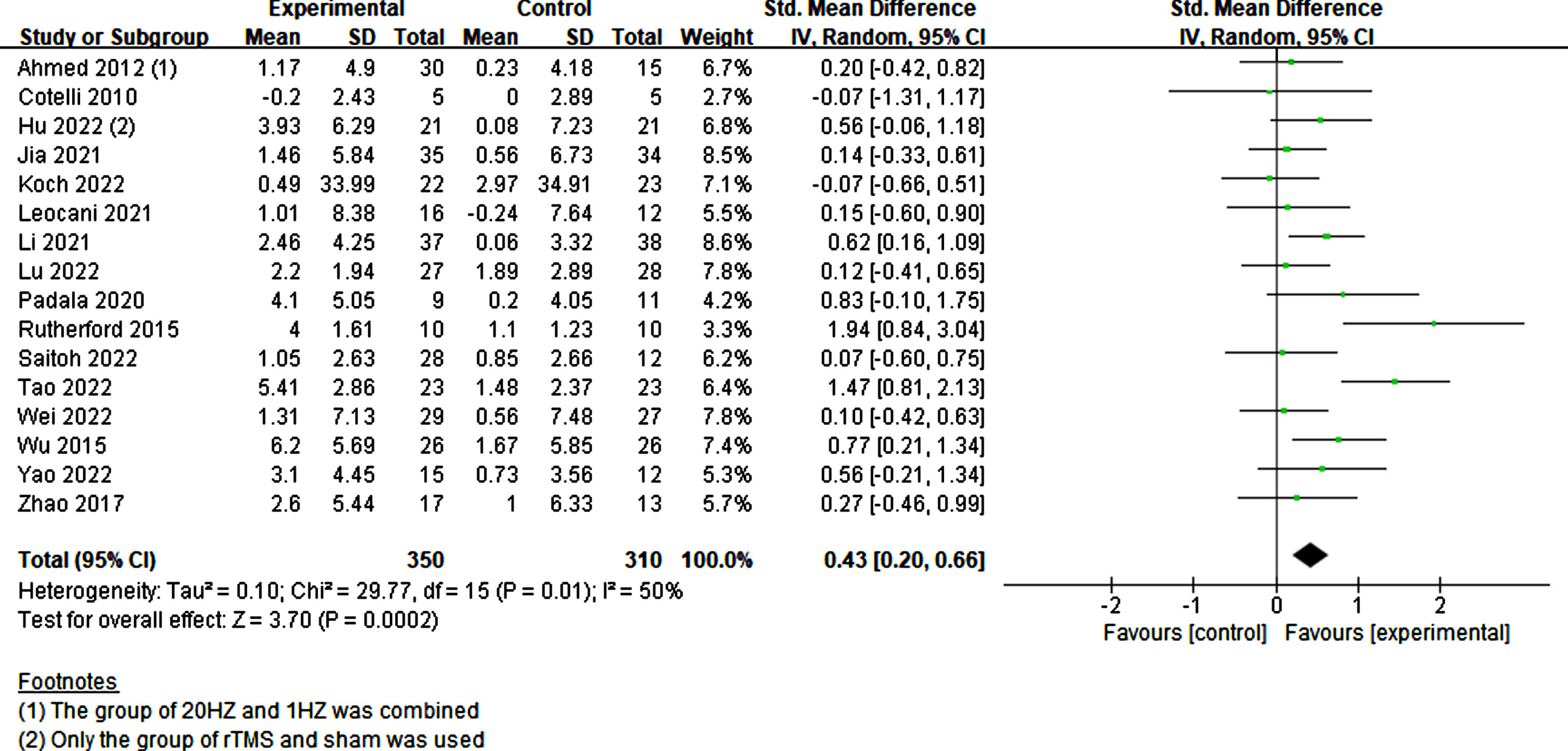
Subgroup analysis of RTMS on global cognition
To evaluate the effects of various rTMS treatment protocols on cognitive impairment, a comprehensive assessment of various parameters was conducted. These parameters included participant characteristics, stimulation position and number, frequency, trial duration, trial interval, total treatment time, single pulses administered per treatment, total pulses across all treatments, treatment frequency, treatment period, sessions, and sham stimulation method employed. Interestingly, both single-target (p = 0.008, SMD = 0.43, 95% CI = 0.12–0.75) and multi-target (p = 0.02, SMD = 0.43, 95% CI = 0.08–0.78) stimulation exhibited improvements in cognitive ability (see Fig. 5). Furthermore, owing to limited studies on the frequencies of 1 Hz and 5 Hz, the analysis focused on 10 Hz and 20 Hz. Notably, higher frequency (20 Hz) rTMS demonstrated a significant enhancement in cognition (p = 0.002, SMD = 0.65, 95% CI = 0.23–0.1.06), whereas lower frequency (10 Hz) rTMS did not yield significant improvements (p = 0.18, SMD = 0.17, 95% CI = –0.08–0.41) (see Fig. 6).
Fig. 5
Differential treatment effects based on the number of stimulation sites in rTMS.
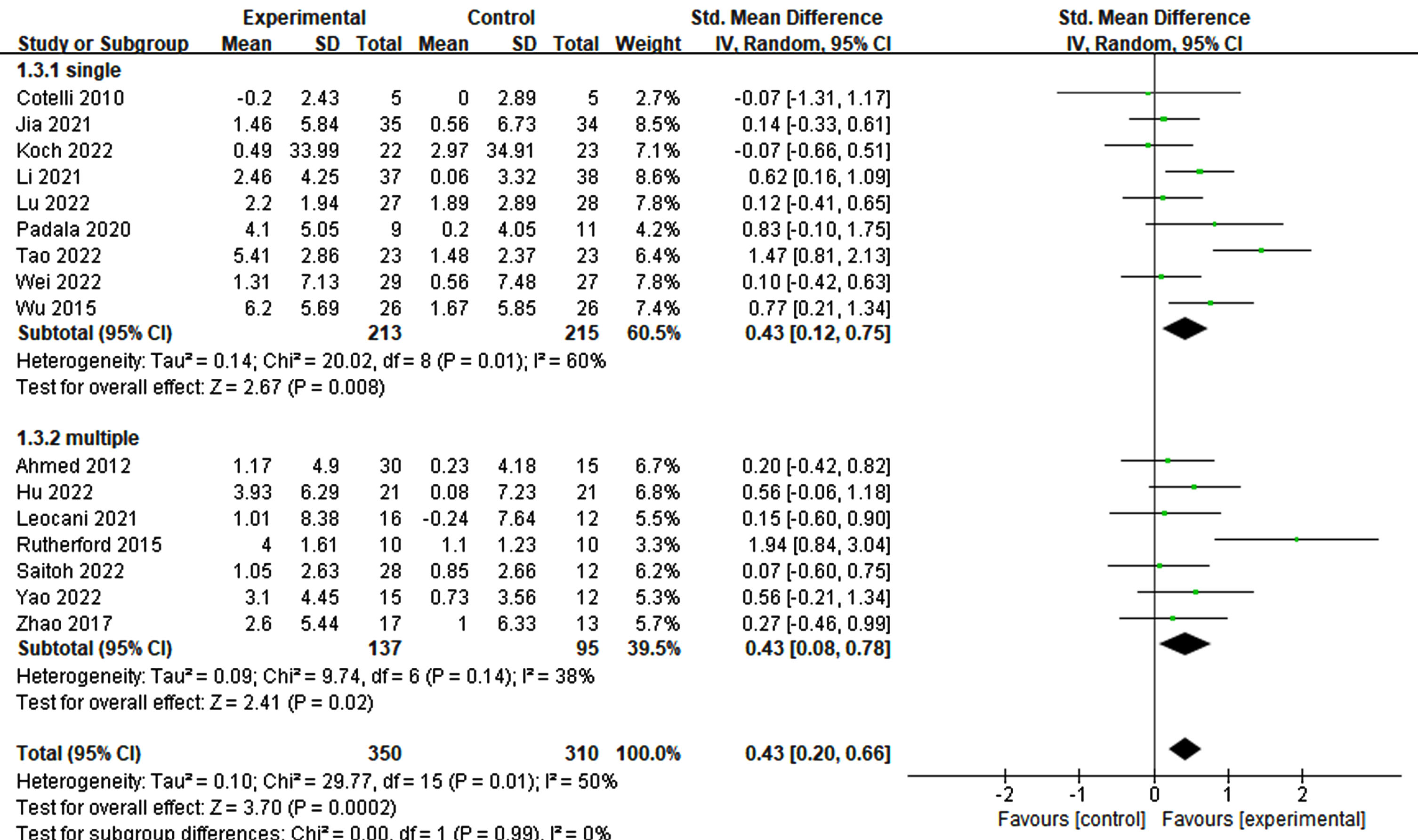
Fig. 6
Treatment effects across various frequencies in rTMS.
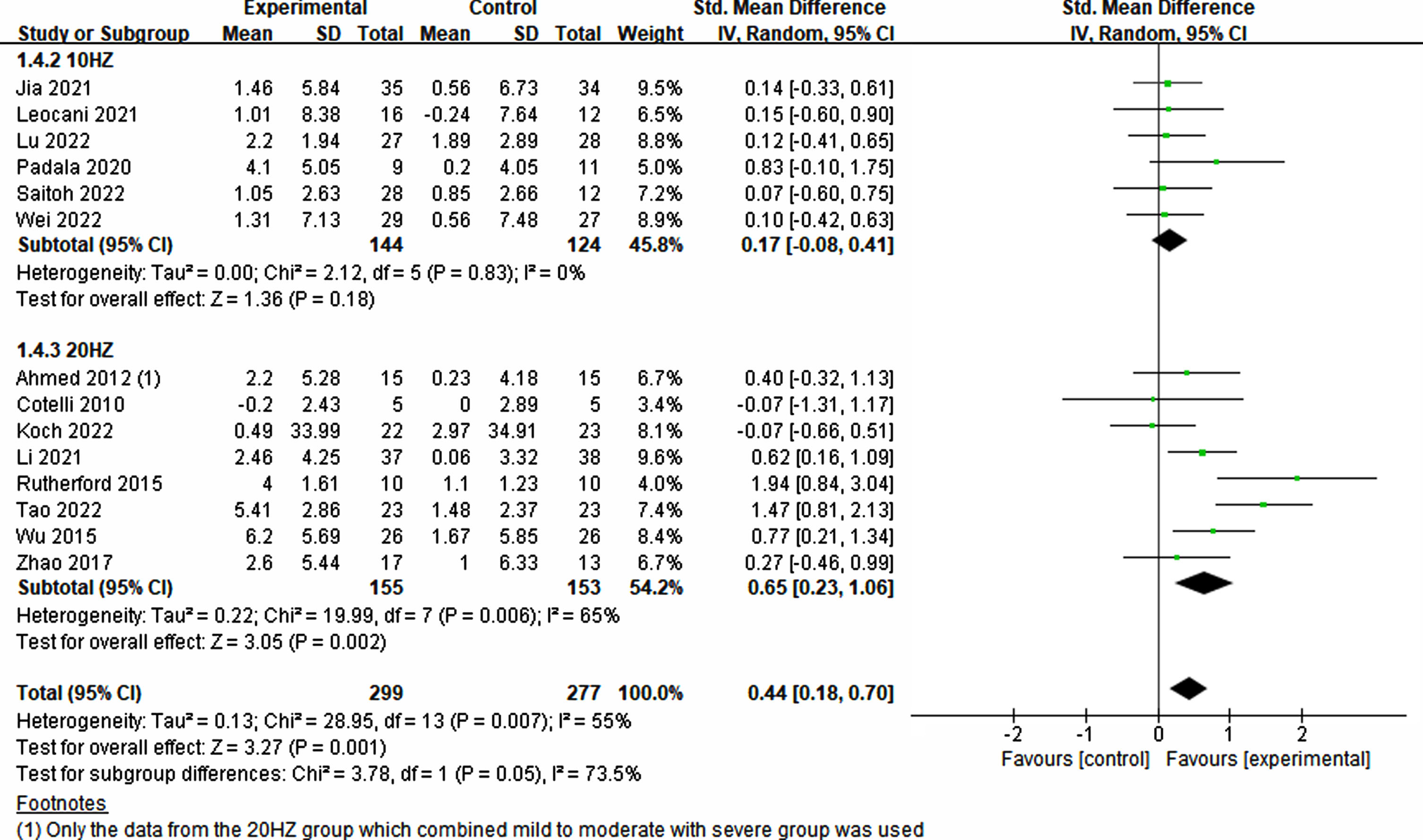
Of the 12 studies reviewed, those with a stimulation duration of 1–2 s had a statistically significant effect (p = 0.01, SMD = 0.54, 95% CI = 0.12–0.95) compared to those with a 4–5 s duration (p = 0.19, SMD = 0.22, 95% CI = –0.11–0.54) (Fig. 7). Regarding interval times reported in a trial, a statistical effect was observed. Specifically, only intervals ranging from 20–30 s (p = 0.04, SMD = 0.28, 95% CI = 0.02–0.54) exhibited significant cognitive improvement, whereas intervals of≤10 s did not (p = 0.07, SMD = 1.18, 95% CI = –0.09-2.46) (refer to Fig. 8).
Fig. 7
Impact of stimulated time on treatment effects in rTMS.
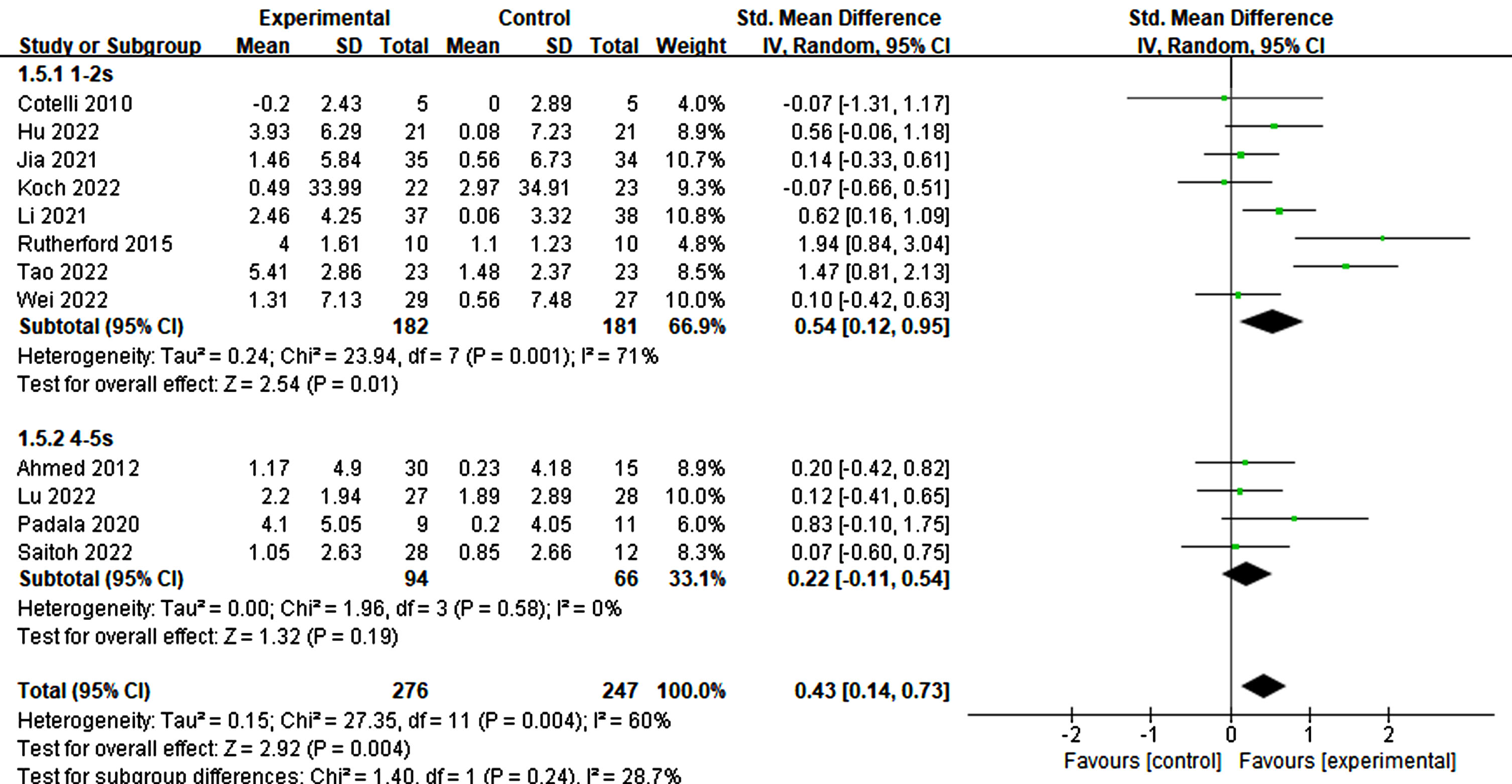
Fig. 8
Differential treatment effects based on interval time in rTMS.
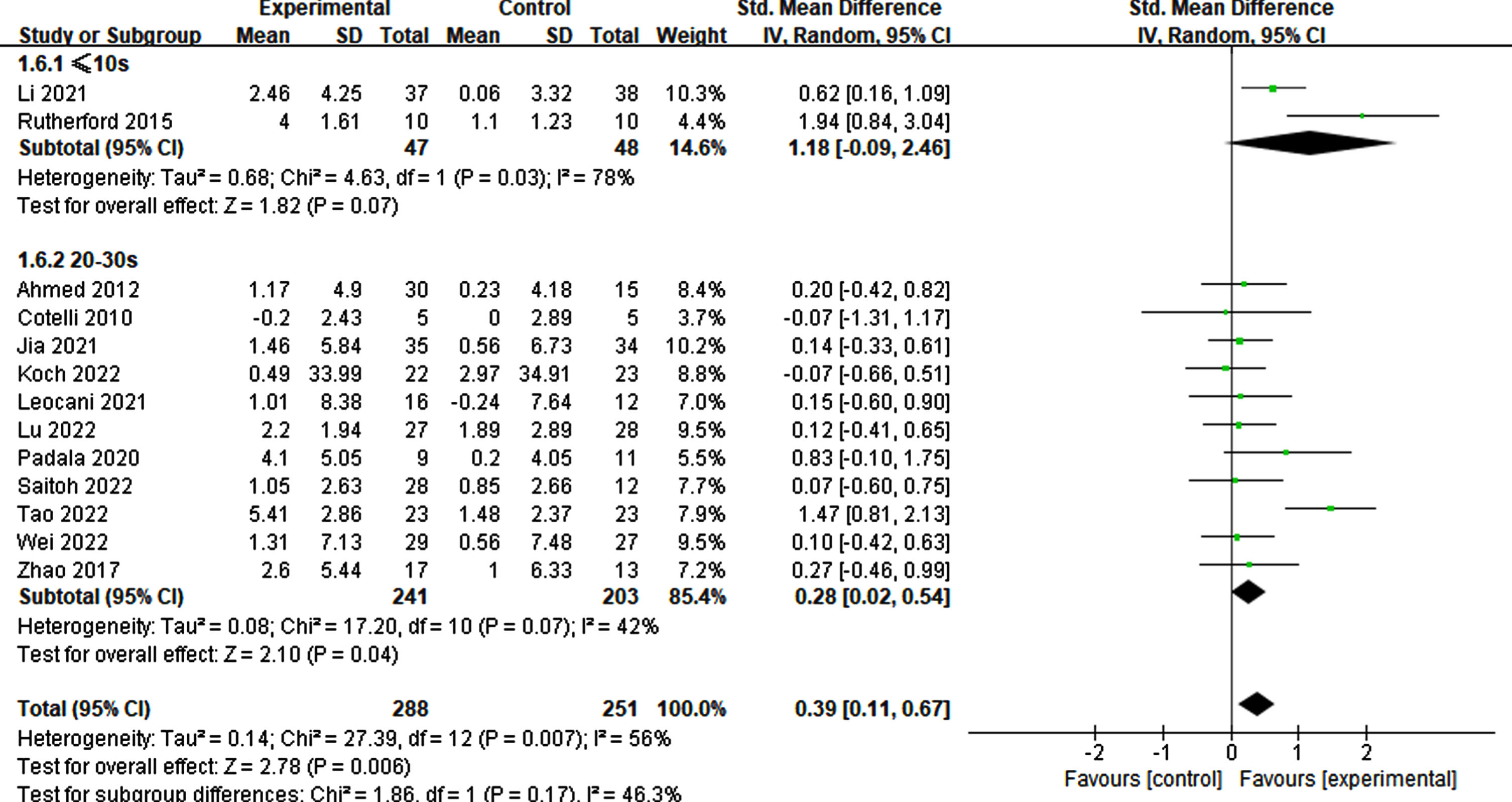
The pulse plays a crucial role in the success of rTMS treatment in improving cognition. Significant differences were observed among different groups of single pulses (≤1500: p = 0.05, SMD = 0.23, 95% CI = –0.00–0.46; 1500 < n≤2500: p = 0.004, SMD = 0.69, 95% CI = 0.22–1.16), while no significant difference was noted in the group with >2500 pulses (p = 0.10, SMD = 0.35, 95% CI = –0.07–0.77) (refer to Fig. 9). Furthermore, the total number of pulses emerged as a critical factor. The findings indicated that a pulse count exceeding 20000 was essential for significant improvement (20000–30000: p = 0.02, SMD = 0.71, 95% CI = 0.14–1.29; ≥40000: p = 0.009, SMD = 0.52, 95% CI = 0.13–0.92). Conversely, counts below 20000 did not demonstrate a significant impact (≤10000: p = 0.49, SMD = 0.12, 95% CI = –0.23–0.47; 10000–20000: p = 0.52, SMD = 0.15, 95% CI = –0.30–0.59) (refer to Fig. 10).
Fig. 9
Analyzing the impact of single pulses.
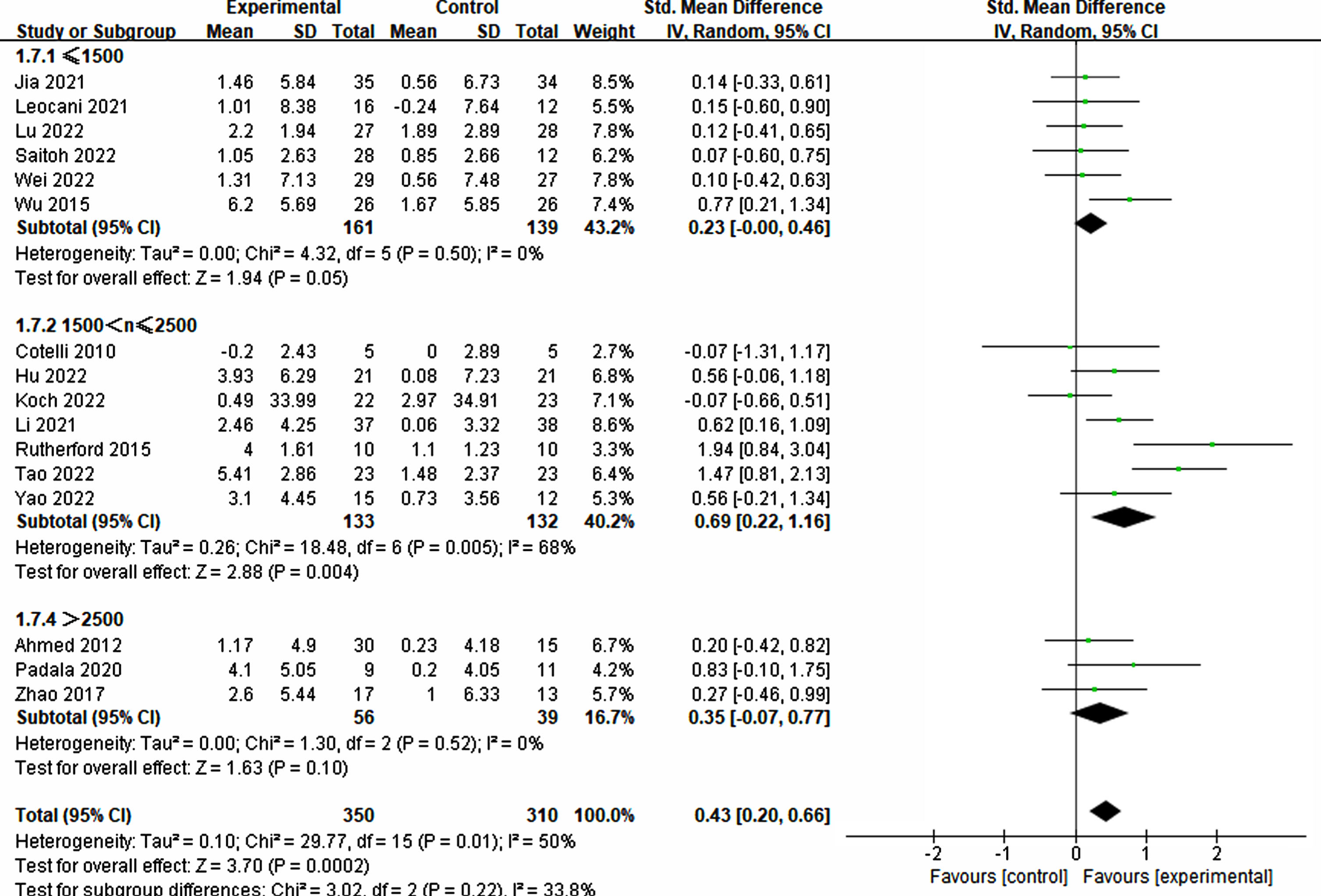
Fig. 10
Investigating the influence of total pulses.
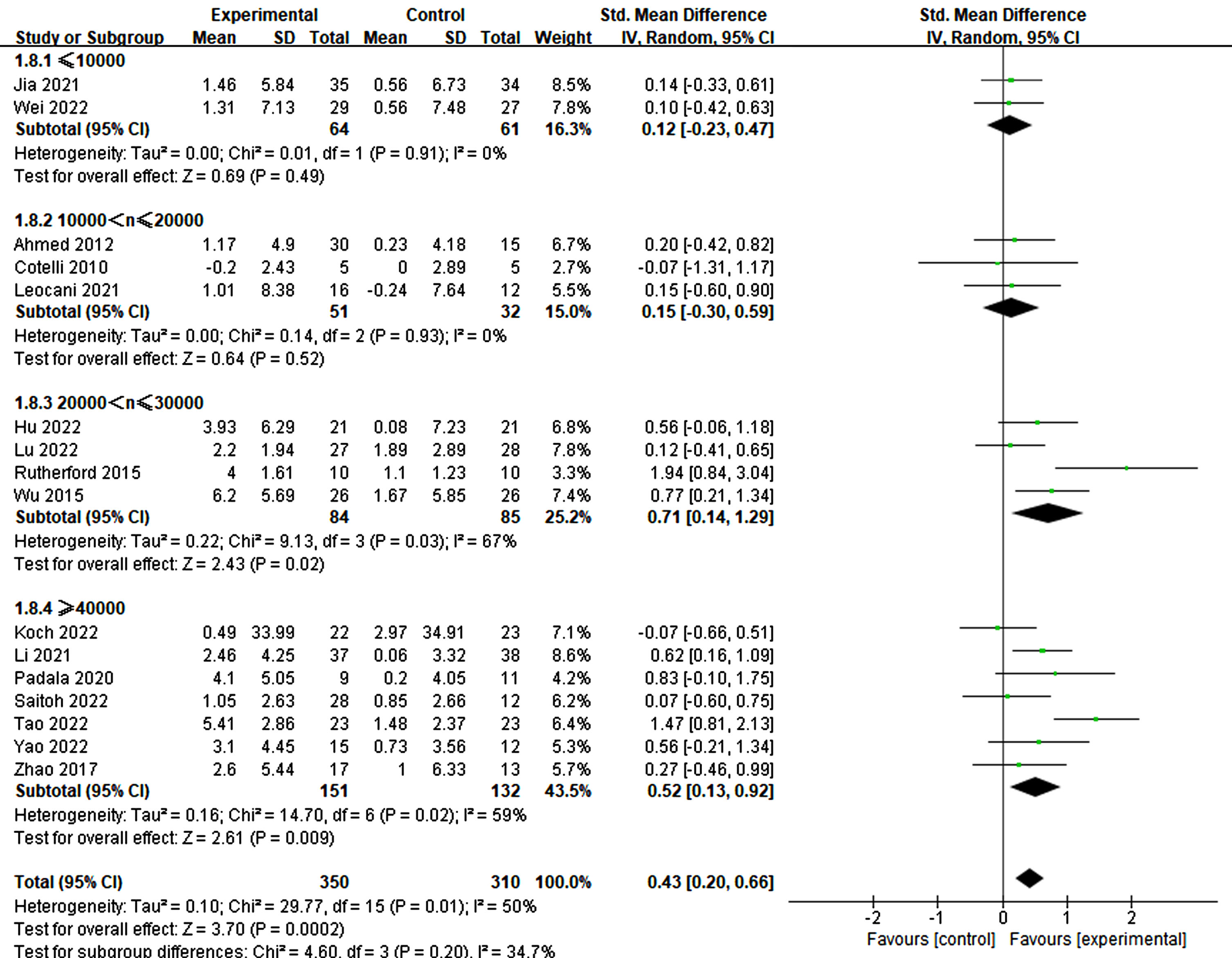
Furthermore, the treatment duration was also significant in producing therapeutic effects. Specifically, treatments lasting more than three weeks demonstrated significant improvements in cognitive ability (3–4 weeks: p = 0.007, SMD = 0.56, 95% CI = 0.15–0.97; ≥6 weeks: p = 0.02, SMD = 0.65, 95% CI = 0.10–1.19) (refer to Fig. 11). Similarly, more than 20 treatment sessions were found to be necessary for significant improvement (≥20 sessions: p = 0.003, SMD = 0.60, 95% CI = 0.20–1.01) (refer to Fig. 12).
Fig. 11
Differential treatment effects of treatment duration in rTMS.
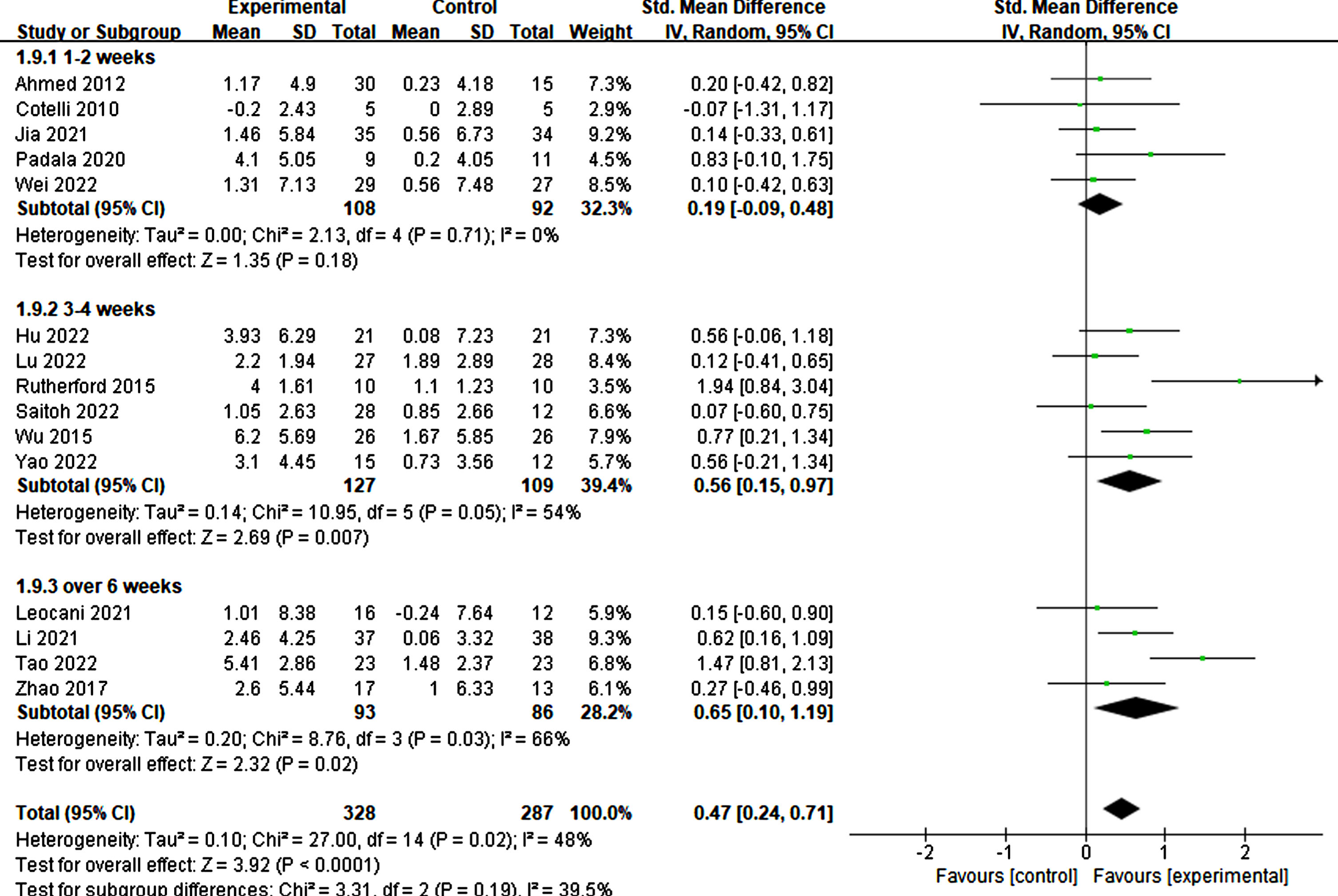
Fig. 12
Different treatment effects in sessions.
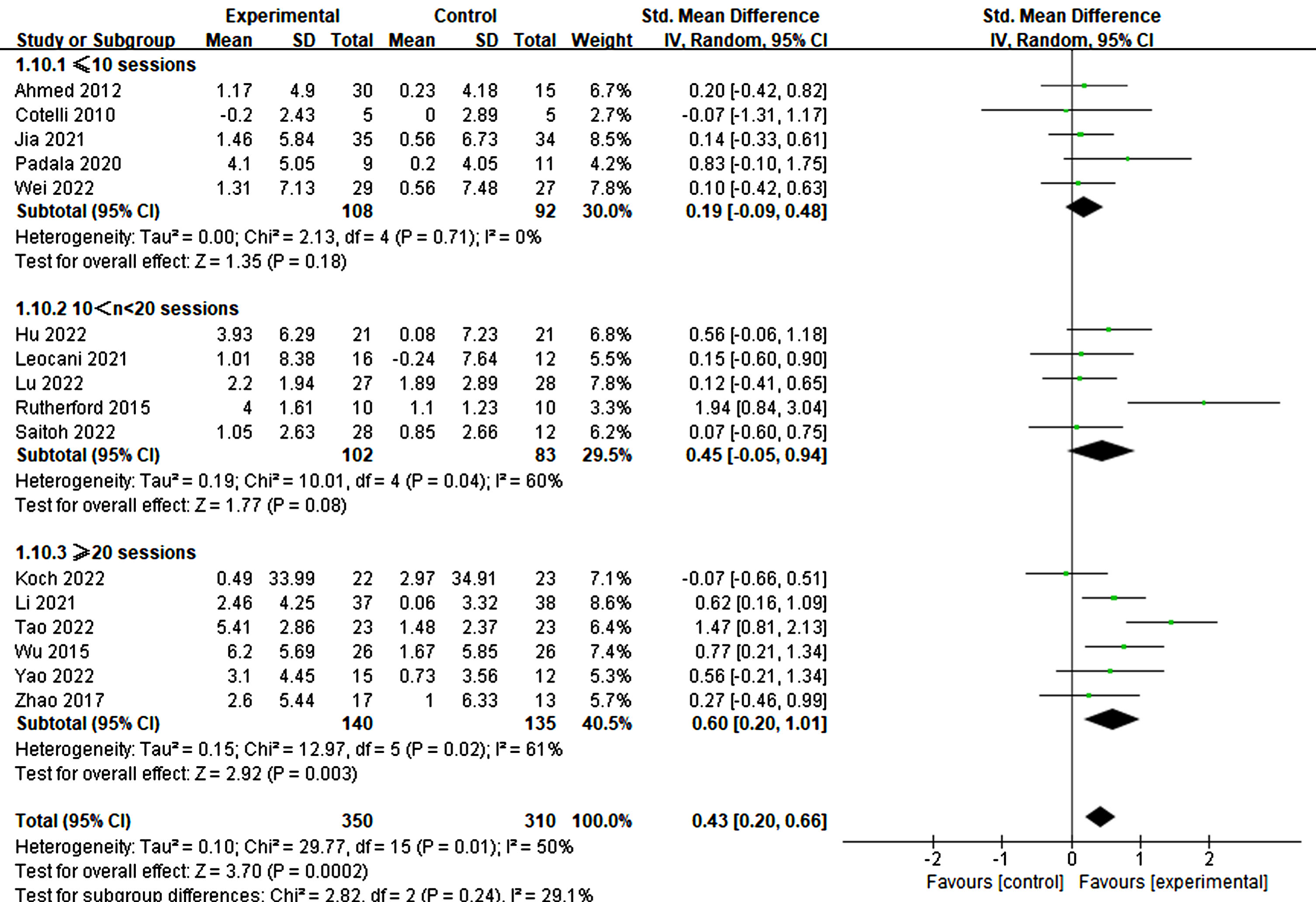
Determining whether rTMS therapy should be continued is challenging. Addressing this issue can assist in determining effective treatment plans, including the duration and frequency of consolidation. Therefore, we conducted further analysis of the follow-up effect of rTMS on cognitive impairment. Our findings indicated that the effect lasted for a minimum of 6 weeks (p = 0.009, SMD = 0.29, 95% CI = 0.07–0.50) (see Fig. 13).
Fig. 13
Follow-up effect of rTMS on cognitive impairment.
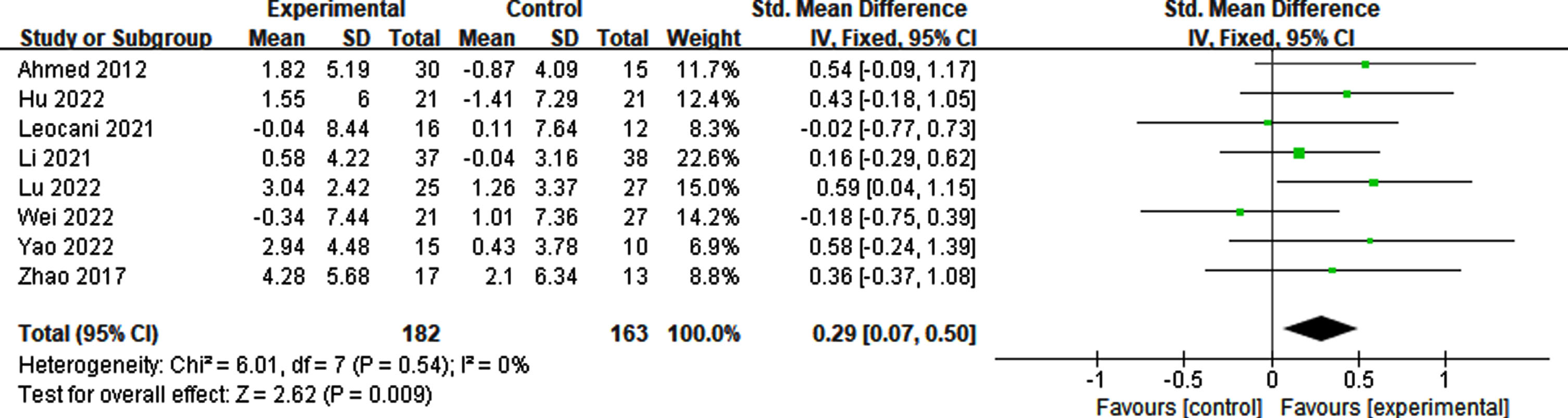
Effects of RTMS on memory
Memory impairment serves as a key indicator of AD. Four studies analyzed the effects of rTMS on memory by combining all the scales that measure memory. A slight heterogeneity was observed in this meta-analysis (p = 0.30, I2 = 18%). The results indicate that the application of rTMS led to statistically significant improvements compared to sham stimulation (p = 0.009, SMD = 0.37, 95% CI = 0.09–0.65) (see Fig. 14).
Fig. 14
Forest plot revealing the impact of rTMS on memory.
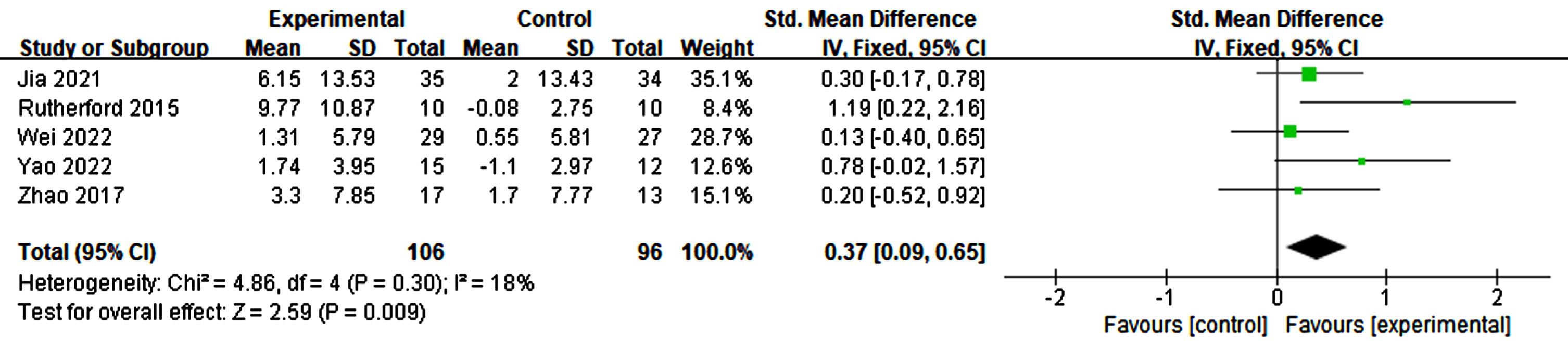
Effects of RTMS on executive function
Three studies reported the outcomes of executive function. Scales measuring executive function include the Trail Making Test, Clock Drawing Test, and Frontal Assessment Battery. The results show that no significant change was found due to rTMS (p = 0.46, SMD = 0.15, 95% CI = –0.26–0.57) (see Fig. 15).
Fig. 15
Forest plot demonstrating the effect of rTMS on executive function.

Effects of RTMS on emotion
Four studies included in the analysis reported results on emotional outcomes using scales such as the Geriatric Depression Scale, Patient Health Questionnaire-9, and Cornell Scale for Depression in Dementia [36, 43, 52]. The findings suggest that there was no statistically significant effect on emotion compared to sham stimulation (p = 0.78, SMD = 0.07, 95% CI = –0.40–0.53) (see Fig. 16).
Fig. 16
Forest plot demonstrating the impact of rTMS on emotion.

Effects of rTMS on daily living
Six studies considered daily living as effective for participants in basic living abilities, such as living alone, dressing correctly, and eating timely, and complex living abilities, such as shopping, taking medicine, and taking part in social activities. The result suggested that rTMS had no significant effect on the activities of daily living (p = 0.23, SMD = 0.40, 95% CI = –0.25–1.04) (see Fig. 17).
Fig. 17
Forest plot displaying the effect of rTMS on daily living.
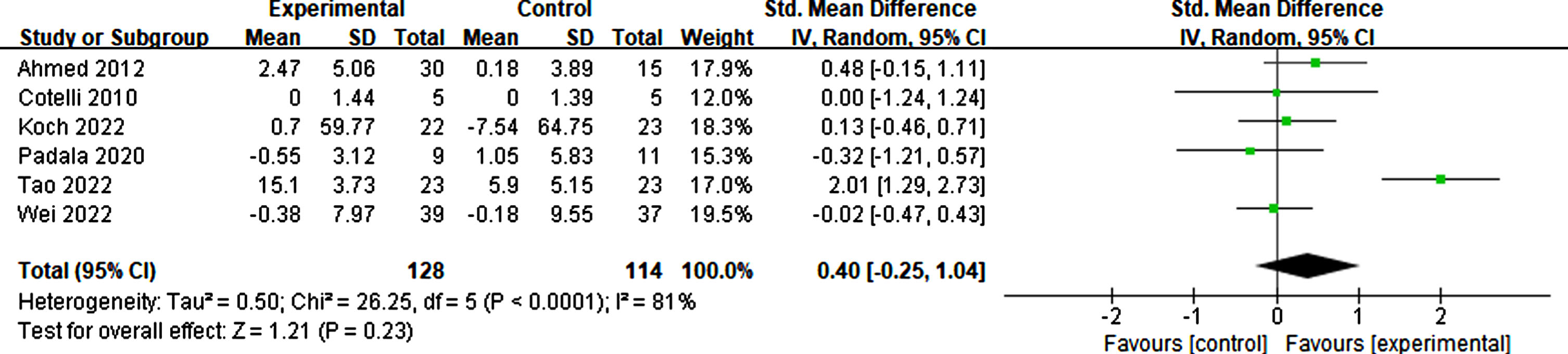
Effects of RTMS on neuropsychiatric symptoms
Koch et al. [60], Saitoh et al. [55], and Wu et al. [56] investigated changes in neuropsychiatric using the Neuropsychiatric Inventory Questionnaire. The outcomes showed moderate heterogeneity (I2 = 53%). Thus, the random effect mode was adopted, and there was no significant difference in neuropsychiatric symptoms between rTMS treatment and sham groups (p = 0.40, SMD = 0.21, 95% CI = –0.29–0.72) (see Fig. 18).
Fig. 18
Forest plot demonstrating the effect of rTMS on neuropsychiatric symptoms.

Side effect of RTMS
Eleven studies reported the side effects of rTMS. The proportion of adverse effects during rTMS treatment was 21.43% . Compared to the sham group, individuals receiving true rTMS stimulation experienced significantly more adverse effects (p = 0.009, SMD = 2.29, 95% CI = 1.23–4.27). These symptoms include headache, scalp tingling, neck pain or stiffness, insomnia, and fatigue. However, most adverse effects disappeared following rTMS treatment (Fig. 19).
Fig. 19
Side effects caused by rTMS.
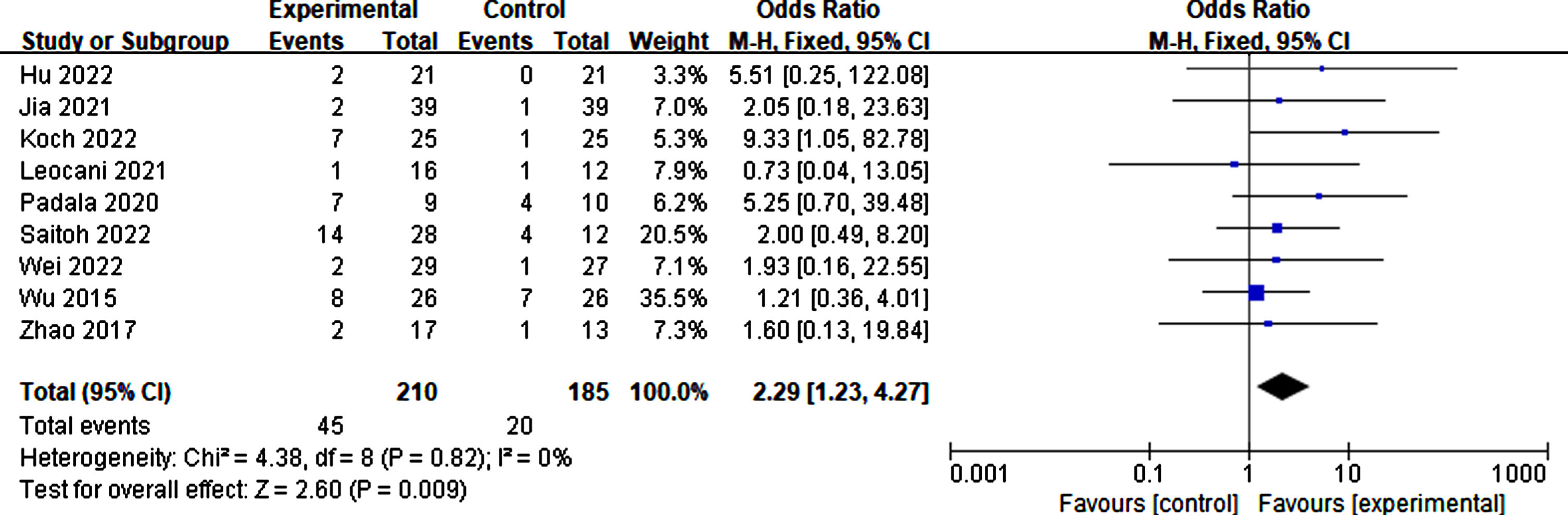
Publication bias analysis
A funnel plot was employed to evaluate publication bias and sensitivity, given that more than 10 studies were included in this meta-analysis. The result indicates that the funnel plot exhibits complete symmetry (Fig. 20). In addition, quantitative analysis was conducted using the Begg and Egger tests, and the results showed no significant publication bias (Begg: p = 0.2604; Egger: p = 0.2578).
Fig. 20
Funnel plot of publication bias.
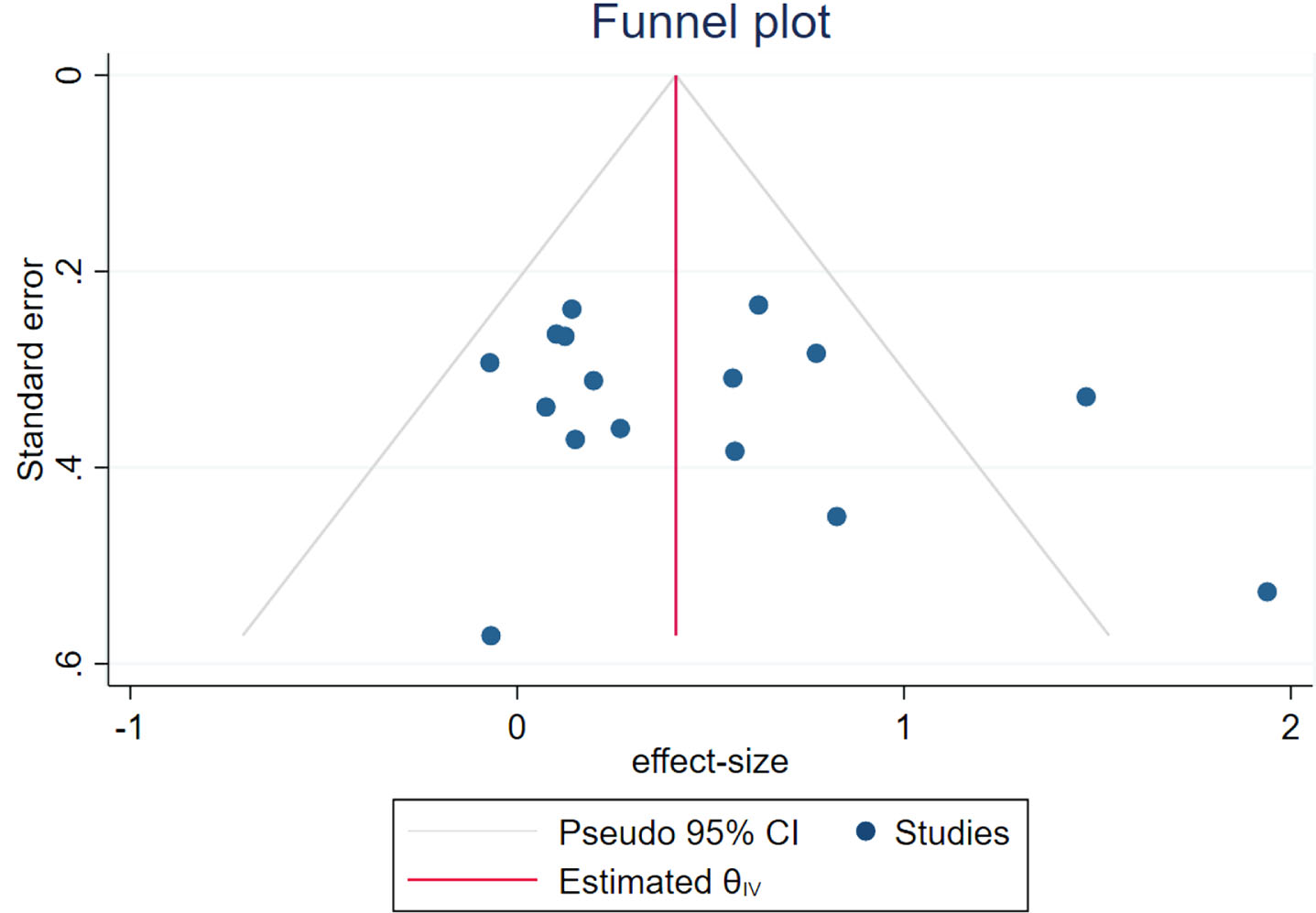
DISCUSSION
This meta-analysis included 16 randomized controlled trial articles published between 1 January 2010 and 28 February 2023 encompassing a cohort of 655 participants. The findings of our study demonstrate that rTMS yields a significant and moderate effect on improving global cognitive function, with an effect size of 0.43; the therapeutic benefits were sustained for at least 6 weeks. Interestingly, the results indicate that stimulation sites (single target and multiple targets), frequency (20 Hz), stimulation time (1–2 s), interval (20–30 s), single pulses (≤2500), total pulses (>20000), duration (≥3 weeks), and sessions (≥20) effectively enhance the overall cognitive ability of individuals with AD.
Our findings are consistent with and reinforce the results of contemporary rigorously controlled trials investigating the efficacy of rTMS in individuals diagnosed with AD [16, 28, 63]. These studies indicate that rTMS can effectively enhance the overall cognitive ability of patients with AD, potentially benefiting approximately 80% [64]. However, this meta-analysis excluded RCTs assessing the influence of CT in improving global cognition and memory in patients with AD. Some studies have reported no significant differences when comparing rTMS combined with medication or CT [22], whereas other studies have suggested that rTMS combined with CT is more effective compared to sham treatment with CT [65, 66]. A meta-analysis by Menardi et al. [67] compared the effects of cognitive training on the cognitive ability of patients with AD and found that only rTMS + CT produced significant results, while standalone rTMS showed no effectiveness. Although the latter result contradicts our current conclusions, at the same time, it also highlights the influence of the literature containing CT on the results. While our study primarily focused on the effects of rTMS alone, it is important to note that we did not directly compare the cognitive improvements induced by rTMS and CT alone. Therefore, caution should be exercised when making definitive statements regarding the superiority of rTMS over CT. CT is an important factor that affects cognitive ability and should not be included when considering the impact of rTMS alone. However, this finding contradicts several studies suggesting that significant enhancements in cognitive abilities are observed only when rTMS is combined with CT, as rTMS alone does not yield substantial improvements [68–71]. Based on this understanding, it is crucial to emphasize that a single CT scan can indeed have a positive effect on cognitive ability. In the future, a more detailed evaluation of the effects of rTMS alone, considering the influence of CT, is warranted.
Additionally, based on the current literature, most studies in this meta-analysis targeted the DLPFC. However, we did not compare the treatment effects at different rTMS stimulation sites. Post-TMS treatment, there was an observed increase in neural activity within the precuneus, accompanied by changes in effective DLPFC connectivity. Moreover, an inverse correlation between prefrontal-to-parietal connectivity and cognitive impairment was observed [72]. TMS stimulation led to pronounced excitability in the DLPFC [73], a crucial element in cortical plasticity and cognitive function [74]. The findings suggest that rTMS holds promise as an efficacious intervention for AD by augmenting cortical excitability [19, 75–77] and enduring neuroplasticity alterations [51, 78, 79]. Furthermore, some studies indicated that post-TMS treatment, neural activity in the anterior cingulate cortex was enhanced, brain oscillations in the beta band intensified, and functional connections between the anterior cingulate cortex and medial frontal lobe region of the default mode network (DMN) were transformed [80]. Hence, the dynamic modulation of intra- and inter-DMN connectivity at the baseline level holds promise as a prospective indicator for predicting a positive treatment response to rTMS in individuals with cognitive impairment [81]. Stimulating the DLPFC is a viable option to effectively boost cortical excitability in individuals with AD.
Furthermore, the therapeutic effects of rTMS are largely influenced by different treatment parameters. To determine the optimal parameters for the rTMS treatment of cognitive impairment, researchers conducted a subgroup analysis of rTMS treatment on patients with cognitive impairment. The results showed that stimulation sites (single target and multiple targets), frequency (20 Hz), stimulation time (1–2 s), interval (20–30 s), single pulses(≤2500), total pulses (>20000), duration (≥3 weeks), and sessions (≥20) can effectively improve the overall cognitive ability of patients with AD. Similar to the results of this study, numerous studies have highlighted that high-frequency rTMS can effectively enhance patients’ cognitive abilities [41, 42, 68, 80]. High-frequency rTMS is postulated to be involved in modifications akin to LTP of synaptic efficacy, the impairment of which is widely regarded as the fundamental pathophysiological correlate underlying cognitive deterioration in AD [82]. The therapeutic parameters of TMS are important parameters that affect its therapeutic effects. Currently, when a parameter subgroup analysis is conducted, the classification criteria may be unilateral. Different criteria may have led to different conclusions. For example, Menardi et al. [67] pointed out that there was no significant difference between 5 HZ and 10 HZ in the treatment of cognitive ability in AD. However, Zhang et al. [83] pointed out that compared to 1 HZ, 10 HZ can effectively improve cognitive performance. Therefore, when comparing the parameters, the partitioning criteria are very important. Moving forward, further studies need to explore the parameters of TMS for treating various neurological conditions.
In the analysis of the therapeutic effect of rTMS on memory in this study, it was found that rTMS could effectively improve the memory ability of patients with AD (SMD = 0.37). Sandrini et al. [84] also reported that rTMS could effectively improve long-term episodic memory function of patients with AD. Regarding the effect of rTMS on other cognitive behaviors, we determined that it had no significant therapeutic effect on executive function, daily life ability, or neuropsychiatric symptoms. Consistent with previous studies, Xie et al. [85] examined the effects of rTMS on memory and executive function and found no substantial disparities compared with the control group. Similarly, Wei et al. [23] discovered that while rTMS did not effectively ameliorate patients’ memory, executive function, or emotions, it was capable of enhancing their daily life abilities. Iimori et al. [86] emphasized that, when compared to patients with depression, there was no evidence supporting the effectiveness of rTMS in improving the executive function of patients with AD and schizophrenia. Most meta-analyses have shown that there is no significant improvement in executive function, language, etc.; the results should be explained more prudently because of the limited samples and different measurement tools with high heterogeneity. Moreover, it is crucial not to overlook the advancements in executive function [87], daily living [88], and neuropsychiatric symptoms [56] as reported in some RCTs. Further research must be undertaken to explore the multifarious therapeutic effects of rTMS in individuals with AD.
TMS employs pulsed magnetic fields to stimulate the central nervous system, causing changes in brain cell activity and inducing physiological changes. Consequently, it is necessary to further investigate whether these pulsed magnetic fields have negative effects. Our meta-analysis revealed that the rTMS group experienced significantly more negative events compared to the sham group, with a rate of approximately 21.43% . Adverse reactions to TMS therapy vary with age and disease status. Maizey et al. [89] pointed out that mild adverse reactions to TMS in healthy populations are around 5% but may be exacerbated by initial expectations or anxiety of the participants. The incidence of adverse reactions among elderly patients with depression was 12.4% [90]. This indicates that it is necessary to further monitor adverse reactions produced during TMS therapy, understand their origins, and explore interventions to potentially mitigate their incidence.
This study had several limitations. First, the number of included studies was relatively small, falling within the scope of small-sample studies and exhibiting a moderate degree of heterogeneity, including both randomized controlled trials and crossover designs. Second, owing to the wide heterogeneity of the stimulation sites used in each study, it was challenging to effectively compare the therapeutic effects produced by the different stimulation sites. Third, the sub-analysis of stimulation parameters was limited by the small number of studies reporting specific conditions and parameters, leading to the cautious adoption of TMS treatment plans. Finally, relatively few samples were available for sub-analysis of the effects on other cognitive and behavioral functions, leading to high heterogeneity and controversial results. Therefore, a more rational view of the therapeutic effects of TMS on attention, language, behavior, and other domains should be considered, and further research is needed to explore these effects more comprehensively.
Overall, this meta-analysis provides evidence supporting the significant and moderate effects of rTMS in improving global cognitive function in individuals with AD. This study suggests that rTMS may be an efficacious intervention that augments cortical excitability, induces enduring neuroplastic alterations, and potentially modulates cholinergic neurons, synaptic dysfunction, and BDNF expression. However, it is important to consider the limitations of this study and the need for further research to explore the optimal parameters, potential negative effects, and effects on other cognitive and behavioral functions.
Conclusion
rTMS has been shown to improve global cognitive function and memory significantly and effectively in patients with AD. However, there were no significant differences between the rTMS and sham groups in terms of executive function, emotions, daily living skills, or neuropsychiatric symptoms. Additionally, for AD, such a stimulation treatment plan may be an effective choice to improve cognitive ability: stimulation sites (single target or multiple targets), frequency (20 Hz), stimulation time (1–2 s), interval (20–30 s), single pulses (≤2500), total pulses (>20000), duration (≥3 weeks), and sessions (≥20). Finally, rTMS was associated with significantly milder adverse events than the sham treatment, and its effect lasted for at least 6 weeks. In the future, it will be important to pay more attention to the selection of rTMS parameters.
AUTHOR CONTRIBUTIONS
Sha Li (Conceptualization; Formal analysis; Investigation; Methodology; Writing – original draft); Xiaoyong Lan (Data curation; Formal analysis); Yumei Liu (Data curation; Formal analysis); Junhong Zhou (Supervision; Writing – review & editing); Zian Pei (Investigation; Software); Xiaolin Su (Investigation; Validation); Yi Guo (Funding acquisition; Supervision; Writing – review & editing).
ACKNOWLEDGMENTS
The authors have no acknowledgements to report.
FUNDING
This study was funded by the Shenzhen Science and Technology Innovation Commission Project ((Shenzhen, Hong Kong, and Macao Class C), SGDX20210823103805042)) and the Shenzhen Science and Technology Innovation Commission Sustainable Development Project (KCXFZ20201221173400001).
CONFLICT OF INTEREST
The authors have no conflict of interest to report.
DATA AVAILABILITY
The data presented in this study are available in the inserted articles.
REFERENCES
[1] | Zhang XX , Tian Y , Wang. ZT , Ma. YH , Tan L , Yu JT ((2021) ) The epidemiology of Alzheimer’s disease modifiable risk factors and prevention. J Prev Alzheimers Dis 8: , 313–321. |
[2] | Gustavsson A , Norton N , Fast T , Frolich L , Georges J , Holzapfel D , Kirabali T , Krolak-Salmon P , Rossini PM , Ferretti MT , Lanman L , Chadha AS , van der Flier WM ((2023) ) Global estimates on the number of persons across the Alzheimer’s disease continuum. Alzheimers Dement 19: , 658–670. |
[3] | Ganguli M , Dodge HH , Shen C , Pandav RS , DeKosky ST ((2005) ) Alzheimer disease and mortality. Arch Neurol 62: , 779–784. |
[4] | Chan KY , Wang W , Wu JJ , Liu L , Theodoratou E , Car J , Middleton L , Russ TC , Deary IJ , Campbell H , Wang W , Rudan I , Global Health Epidemiology Reference Group (GHERG) ((2013) ) Epidemiology of Alzheimer’s disease and other forms of dementia in China, 1990-2010: A systematic review and analysis. Lancet 381: , 2016–2023. |
[5] | Reitz C , Brayne C , Mayeux R ((2011) ) Epidemiology of Alzheimer disease. Nat Rev Neurol 7: , 137–152. |
[6] | Singhal A , Bangar O , Naithani V ((2012) ) Medicinal plants with a potential to treat Alzheimer and associated symptoms. Int J Nutr Pharmacol Neurol Dis 2: , 84–92. |
[7] | Massoud F , Léger GC ((2011) ) Pharmacological treatment of Alzheimer disease. Can J Psychiatry 56: , 579–588. |
[8] | Tariot PN , Federoff HJ ((2003) ) Current treatment for Alzheimer disease and future prospects. Alzheimer Dis Assoc Disord 17: , S105–S113. |
[9] | Long JM , Holtzman DM ((2019) ) Alzheimer disease: An update on pathobiology and treatment strategies. Cell 179: , 312–339. |
[10] | Kobayashi M , Pascual-Leone A ((2003) ) Transcranial magnetic stimulation in neurology. Lancet Neurol 2: , 145–156. |
[11] | Hallett M ((2000) ) Transcranial magnetic stimulation and the human brain. Nature 406: , 147–150. |
[12] | Klomjai W , Katz R , Lackmy-Vallee A ((2015) ) Basic principles of transcranial magnetic stimulation (TMS) and repetitive TMS (rTMS). Ann Phys Rehabil Med 58: , 208–213. |
[13] | Levy WB , Steward O ((1983) ) Temporal contiguity requirements for long-term associative potentiation/depression in the hippocampus. Neuroscience 8: , 791–797. |
[14] | Luber B , Lisanby SH ((2014) ) Enhancement of human cognitive performance using transcranial magnetic stimulation (TMS). Neuroimage 85: , 961–970. |
[15] | Ridding M , Rothwell J ((2007) ) Is there a future for therapeutic use of transcranial magnetic stimulation? Nat Rev Neurosci 8: , 559–567. |
[16] | Mimura Y , Nishida H , Nakajima S , Tsugawa S , Morita S , Yoshida K , Tarumi R , Ogyu K , Wada M , Kurose S , Miyazaki T , Blumberger DM , Daskalakis ZJ , Chen R , Mimura M , Noda Y ((2021) ) Neurophysiological biomarkers using transcranial magnetic stimulation in Alzheimer’s disease and mild cognitive impairment: A systematic review and meta-analysis. Neurosci Biobehav Rev 121: , 47–59. |
[17] | Martorana A , Mori F , Esposito Z , Kusayanagi H , Monteleone F , Codeca C , Sancesario G , Bernardi G , Koch G ((2009) ) Dopamine modulates cholinergic cortical excitability in Alzheimer’s disease patients. Neuropsychopharmacology 34: , 2323–2328. |
[18] | Di Lazzaro V , Pilato F , Dileone M , Saturno E , Oliviero A , Marra C , Daniele A , Ranieri F , Gainotti G , Tonali PA ((2006) ) In vivo cholinergic circuit evaluation in frontotemporal and Alzheimer dementias. Neurology 66: , 1111–1113. |
[19] | Inghilleri M , Conte A , Frasca V , Scaldaferri N , Gilio F , Santini M , Fabbrini G , Prencipe M , Berardelli A ((2006) ) Altered response to rTMS in patients with Alzheimer’s disease. Clin Neurophysiol 117: , 103–109. |
[20] | Pennisi G , Alagona G , Ferri R , Greco S , Santonocito D , Pappalardo A , Bella R ((2002) ) Motor cortex excitability in Alzheimer disease: One year follow-up study. Neurosci Lett 329: , 293–296. |
[21] | Pennisi G , Ferri R , Lanza G , Cantone M , Pennisi M , Puglisi V , Malaguarnera G , Bella R ((2011) ) Transcranial magnetic stimulation in Alzheimer’s disease: A neurophysiological marker of cortical hyperexcitability. J Neural Transm (Vienna) 118: , 587–598. |
[22] | Lin Y , Jiang WJ , Shan PY , Lu M , Wang T , Li RH , Zhang N , Ma L ((2019) ) The role of repetitive transcranial magnetic stimulation (rTMS) in the treatment of cognitive impairment in patients with Alzheimer’s disease: A systematic review and meta-analysis. J Neurol Sci 398: , 184–191. |
[23] | Wei Z , Fu J , Liang H , Liu M , Ye X , Zhong P ((2022) ) The therapeutic efficacy of transcranial magnetic stimulation in managing Alzheimer’s disease: A systemic review and meta-analysis. Front Aging Neurosci 14: , 980998. |
[24] | Moreno AS , Nguyen JP , Calmelet A , Le Saout E , Damier P , de Decker L , Lefaucheur JP ((2022) ) Multi-site rTMS with cognitive training improves apathy in the long term in Alzheimer’s disease: A 4-year chart review. Clin Neurophysiol 137: , 75–83. |
[25] | Cotelli M , Calabria M , Manenti R , Rosini S , Zanetti O , Cappa SF , Miniussi C ((2011) ) Improved language performance in Alzheimer disease following brain stimulation. J Neurol Neurosurg Psychiatry 82: , 794–797. |
[26] | Boggio PS , Valasek CA , Campanha C , Giglio AC , Baptista NI , Lapenta OM , Fregni F ((2011) ) Non-invasive brain stimulation to assess and modulate neuroplasticity in Alzheimer’s disease. Neuropsychol Rehabil 21: , 703–716. |
[27] | Cotelli M , Manenti R , Cappa SF , Geroldi C , Zanetti O , Rossini PM , Miniussi C ((2006) ) Effect of transcranial magnetic stimulation on action naming in patients with Alzheimer disease. Arch Neurol 63: , 1602–1604. |
[28] | Dong X , Yan L , Huang L , Guan X , Dong C , Tao H , Wang T , Qin X , Wan Q ((2018) ) Repetitive transcranial magnetic stimulation for the treatment of Alzheimer’s disease: A systematic review and meta-analysis of randomized controlled trials. PLoS One 13: , e0205704. |
[29] | Bentwich J , Dobronevsky E , Aichenbaum S , Shorer R , Peretz R , Khaigrekht M , Marton RG , Rabey JM ((2011) ) Beneficial effect of repetitive transcranial magnetic stimulation combined with cognitive training for the treatment of Alzheimer’s disease: A proof of concept study. J Neural Transm 118: , 463–471. |
[30] | Levine SZ , Yoshida K , Goldberg Y , Samara M , Cipriani A , Efthimiou O , Iwatsubo T , Leucht S , Furukawa TA ((2021) ) Linking the Mini-Mental State Examination, the Alzheimer’s Disease Assessment Scale-Cognitive Subscale and the Severe Impairment Battery: Evidence from individual participant data from five randomised clinical trials of donepezil. Evid Based Ment Health 24: , 56–61. |
[31] | Wouters H , van Gool WA , Schmand B , Zwinderman AH , Lindeboom R ((2010) ) Three sides of the same coin: Measuring global cognitive impairment with the MMSE, ADAS-cog and CAMCOG. Int J Geriatr Psychiatry 25: , 770–779. |
[32] | Kumar S , Zomorrodi R , Ghazala Z , Goodman MS , Blumberger DM , Daskalakis ZJ , Fischer CE , Mulsant BH , Pollock BG , Rajji TK ((2023) ) Effects of repetitive paired associative stimulation on brain plasticity and working memory in Alzheimer’s disease: A pilot randomized double-blind-controlled trial. Int Psychogeriatr 35: , 143–155. |
[33] | Traikapi A , Kalli I , Kyriakou A , Stylianou E , Symeou RT , Kardama A , Christou YP , Phylactou P , Konstantinou N ((2022) ) Episodic memory effects of gamma frequency precuneus transcranial magnetic stimulation in Alzheimer’s disease: A randomized multiple baseline study. J Neuropsychol 17: , 279–301. |
[34] | Zhao J , Li Z , Cong Y , Zhang J , Tan M , Zhang H , Geng N , Li M , Yu W , Shan P ((2017) ) Repetitive transcranial magnetic stimulation improves cognitive function of Alzheimer’s disease patients. Oncotarget 8: , 33864–33871. |
[35] | Roque Roque GY , Reyes-Lopez JV , Ricardo Garcell J , LoH M. , Aguilar Fabre L , Trejo Cruz G , Canizares Gomez S , Calderon Moctezuma AR , Ortega Cruz F , Ortíz Baron A , Arias García NA , Espino Cortes M , Hernandez Montiel H , Gonzalez Olvera J ((2021) ) Effect of transcranial magnetic stimulation as an enhancer of cognitive stimulation sessions on mild cognitive impairment: Preliminary results. Psychiatry Res 304: , 114151. |
[36] | Wei L , Zhang Y , Wang J , Xu L , Yang K , Lv X , Zhu Z , Gong Q , Hu W , Li X , Qian M , Shen Y , Chen W ((2022) ) Parietal-hippocampal rTMS improves cognitive function in Alzheimer’s disease and increases dynamic functional connectivity of default mode network. Psychiatry Res 315: , 114721. |
[37] | Jaeggi SM , Buschkuehl M , Jonides J , Shah P ((2011) ) Short- and long-term benefits of cognitive training. Proc Natl Acad Sci U S A 108: , 10081–10086. |
[38] | Belleville S ((2008) ) Cognitive training for persons with mild cognitive impairment. Int Psychogeriatr 20: , 57–66. |
[39] | Sitzer DI , Twamley EW , Jeste DV ((2006) ) Cognitive training in Alzheimer’s disease: A meta-analysis of the literature. Acta Psychiatr Scand 114: , 75–90. |
[40] | Farina E , Mantovani F , Fioravanti R , Pignatti R , Chiavari L , Imbornone E , Olivotto F , Alberoni M , Mariani C , Nemni R ((2006) ) Evaluating two group programmes of cognitive training in mild-to-moderate AD: Is there any difference between a ‘global’ stimulation and a ‘cognitive-specific’ one? Aging Ment Health 10: , 211–218. |
[41] | Liao X , Li G , Wang A , Liu T , Feng S , Guo Z , Tang Q , Jin Y , Xing G , McClure MA , Chen H , He B , Liu H , Mu Q ((2015) ) Repetitive transcranial magnetic stimulation as an alternative therapy for cognitive impairment in Alzheimer’s disease: A meta-analysis. J Alzheimers Dis 48: , 463–472. |
[42] | Jiang W , Wu Z , Wen L , Sun L , Zhou M , Jiang X , Gui Y ((2022) ) The efficacy of high- or low-frequency transcranial magnetic stimulation in Alzheimer’s disease patients with behavioral and psychological symptoms of dementia. Adv Ther 39: , 286–295. |
[43] | Ahmed MA , Darwish ES , Khedr EM , El Serogy YM , Ali AM ((2012) ) Effects of low versus high frequencies of repetitive transcranial magnetic stimulation on cognitive function and cortical excitability in Alzheimer’s dementia. J Neurol 259: , 83–92. |
[44] | Alcalá-Lozano R , Morelos-Santana E , Cortés-Sotres JF , Garza-Villarreal EA , Sosa-Ortiz AL , González-Olvera JJ ((2018) ) Similar clinical improvement and maintenance after rTMS at 5Hz using a simple vs. complex protocol in Alzheimer’s disease. Brain Stimul 11: , 625–627. |
[45] | Turriziani P , Smirni D , Mangano GR , Zappala G , Giustiniani A , Cipolotti L , Oliveri M ((2019) ) Low-frequency repetitive transcranial magnetic stimulation of the right dorsolateral prefrontal cortex enhances recognition memory in Alzheimer’s disease. J Alzheimers Dis 72: , 613–622. |
[46] | Wassermann EM ((1998) ) Risk and safety of repetitive transcranial magnetic stimulation: Report and suggested guidelines from the International Workshop on the Safety of Repetitive Transcranial Magnetic Stimulation, June 5–7, 1996. Electroencephalography Clin Neurophysiol 108: , 1–16. |
[47] | Lefaucheur JP , Andre-Obadia N , Antal A , Ayache SS , Baeken C , Benninger DH , Cantello RM , Cincotta M , de Carvalho M , De Ridder D , Devanne H , Di Lazzaro V , Filipovic SR , Hummel FC , Jaaskelainen SK , Kimiskidis VK , Koch G , Langguth B , Nyffeler T , Oliviero A , Padberg F , Poulet E , Rossi S , Rossini PM , Rothwell JC , Schonfeldt-Lecuona C , Siebner HR , Slotema CW , Stagg CJ , Valls-Sole J , Ziemann U , Paulus W , Garcia-Larrea L ((2014) ) Evidence-based guidelines on the therapeutic use of repetitive transcranial magnetic stimulation (rTMS). Clin Neurophysiol 125: , 2150–2206. |
[48] | Rossi S , Hallett M , Rossini PM , Pascual-Leone A , Safety of TMSCG ((2009) ) Safety, ethical considerations, and application guidelines for the use of transcranial magnetic stimulation in clinical practice and research. Clin Neurophysiol 120: , 2008–2039. |
[49] | Liberati A , Altman DG , Tetzlaff J , Mulrow C , Gotzsche PC , Ioannidis JP , Clarke M , Devereaux PJ , Kleijnen J , Moher D ((2009) ) The PRISMA statement for reporting systematic reviews and meta-analyses of studies that evaluate health care interventions: Explanation and elaboration. PLoS Med 6: , e1000100. |
[50] | Sterne JAC , Savovic J , Page MJ , Elbers RG , Blencowe NS , Boutron I , Cates CJ , Cheng HY , Corbett MS , Eldridge SM , Emberson JR , Hernan MA , Hopewell S , Hrobjartsson A , Junqueira DR , Juni P , Kirkham JJ , Lasserson T , Li T , McAleenan A , Reeves BC , Shepperd S , Shrier I , Stewart LA , Tilling K , White IR , Whiting PF , Higgins JPT ((2019) ) RoB 2: A revised tool for assessing risk of bias in randomised trials.l. BMJ 366: , 4898. |
[51] | Li X , Qi G , Yu C , Lian G , Zheng H , Wu S , Yuan TF , Zhou D ((2021) ) Cortical plasticity is correlated with cognitive improvement in Alzheimer’s disease patients after rTMS treatment. Brain Stimul 14: , 503–510. |
[52] | Lu H , Chan SSM , Ma S , Lin C , Mok VCT , Shi L , Wang D , Mak AD , Lam LCW ((2022) ) Clinical and radiomic features for predicting the treatment response of repetitive transcranial magnetic stimulation in major neurocognitive disorder: Results from a randomized controlled trial. Hum Brain Mapp 43: , 5579–5592. |
[53] | Padala PR , Boozer EM , Lensing SY , Parkes CM , Hunter CR , Dennis RA , Caceda R , Padala KP ((2020) ) Neuromodulation for apathy in Alzheimer’s disease: A double-blind, randomized, sham-controlled pilot study. J Alzheimers Dis 77: , 1483–1493. |
[54] | Rutherford G , Lithgow B , Moussavi Z ((2015) ) Short and long-term effects of rTMS treatment on Alzheimer’s disease at different stages: A pilot study. J Exp Neurosci 2015: , 43–51. |
[55] | Saitoh Y , Hosomi K , Mano T , Takeya Y , Tagami S , Mori N , Matsugi A , Jono Y , Harada H , Yamada T , Miyake A ((2022) ) Randomized, sham-controlled, clinical trial of repetitive transcranial magnetic stimulation for patients with Alzheimer’s dementia in Japan. Front Aging Neurosci 14: , 993306. |
[56] | Wu Y , Xu W , Liu X , Xu Q , Tang L , Wu S ((2015) ) Adjunctive treatment with high frequency repetitive transcranial magnetic stimulation for the behavioral and psychological symptoms of patients with Alzheimer’s disease: A randomized, double-blind, sham-controlled study. Shanghai Arch Psychiatry 27: , 280–288. |
[57] | Tao Y , Lei B , Zhu Y , Fang X , Liao L , Chen D , Gao C ((2022) ) Repetitive transcranial magnetic stimulation decreases serum amyloid-beta and increases ectodomain of p75 neurotrophin receptor in patients with Alzheimer’s disease. J Integr Neurosci 21: , 140. |
[58] | Jia Y , Xu L , Yang K , Zhang Y , Lv X , Zhu Z , Chen Z , Zhu Y , Wei L , Li X , Qian M , Shen Y , Hu W , Chen W ((2021) ) Precision repetitive transcranial magnetic stimulation over the left parietal cortex improves memory in Alzheimer’s disease: A randomized, double-blind, sham-controlled study. Front Aging Neurosci 13: , 693611. |
[59] | Hu Y , Jia Y , Sun Y , Ding Y , Huang Z , Liu C , Wang Y ((2022) ) Efficacy and safety of simultaneous rTMS–tDCS over bilateral angular gyrus on neuropsychiatric symptoms in patients with moderate Alzheimer’s disease: A prospective, randomized, sham-controlled pilot study. Brain Stimul 15: , 1530–1537. |
[60] | Koch G , Casula EP , Bonnì S , Borghi I , Assogna M , Minei M , Pellicciari MC , Motta C , D’Acunto A , Porrazzini F , Maiella M , Ferrari C , Caltagirone C , Santarnecchi E , Bozzali M , Martorana A ((2022) ) Precuneus magnetic stimulation for Alzheimer’s disease: A randomized, sham-controlled trial. Brain 145: , 3776–3786. |
[61] | Yao Q , Tang F , Wang Y , Yan Y , Dong L , Wang T , Zhu D , Tian M , Lin X , Shi J ((2022) ) Effect of cerebellum stimulation on cognitive recovery in patients with Alzheimer disease: A randomized clinical trial. Brain Stimul 15: , 910–920. |
[62] | Leocani L , Dalla Costa G , Coppi E , Santangelo R , Pisa M , Ferrari L , Bernasconi MP , Falautano M , Zangen A , Magnani G , Comi G ((2021) ) Repetitive transcranial magnetic stimulation with H-coil in Alzheimer’s disease: A double-blind, placebo-controlled pilot study. Front Neurol 11: , 614351. |
[63] | Chou YH , Ton That V , Sundman M ((2020) ) A systematic review and meta-analysis of rTMS effects on cognitive enhancement in mild cognitive impairment and Alzheimer’s disease. Neurobiol Aging 86: , 1–10. |
[64] | Rabey JM , Dobronevsky E ((2016) ) Repetitive transcranial magnetic stimulation (rTMS) combined with cognitive training is a safe and effective modality for the treatment of Alzheimer’s disease: Clinical experience. J Neural Transm (Vienna) 123: , 1449–1455. |
[65] | Bagattini C , Zanni M , Barocco F , Caffarra P , Brignani D , Miniussi C , Defanti CA ((2020) ) Enhancing cognitive training effects in Alzheimer’s disease: rTMS as an add-on treatment. Brain Stimul 13: , 1655–1664. |
[66] | Qin Y , Ba L , Zhang F , Jian S , Zhang M , Zhu W ((2023) ) Cerebral blood flow changes induced by high-frequency repetitive transcranial magnetic stimulation combined with cognitive training in Alzheimer’s disease. Front Neurol 14: , 1037864. |
[67] | Menardi A , Dotti L , Ambrosini E , Vallesi A ((2022) ) Transcranial magnetic stimulation treatment in Alzheimer’s disease: A meta-analysis of its efficacy as a function of protocol characteristics and degree of personalization. J Neurol 269: , 5283–5301. |
[68] | Cheng CPW , Wong CSM , Lee KK , Chan APK , Yeung JWF , Chan WC ((2018) ) Effects of repetitive transcranial magnetic stimulation on improvement of cognition in elderly patients with cognitive impairment: A systematic review and meta-analysis.. Int J Geriatr Psychiatry 33: , e1–e13. |
[69] | Brem AK , Schilberg L , Freitas C , Atkinson N , Seligson E , Pascual-Leone A ((2013) ) P3–293: Effects of cognitive training and rTMS in Alzheimer’s disease. Alzheimers Dement 9: , 664. |
[70] | Rabey JM , Dobronevsky E , Aichenbaum S , Gonen O , Marton RG , Khaigrekht M ((2013) ) Repetitive transcranial magnetic stimulation combined with cognitive training is a safe and effective modality for the treatment of Alzheimer’s disease: A randomized, double-blind study. J Neural Transm (Vienna) 120: , 813–819. |
[71] | Lee J , Choi BH , Oh E , Sohn EH , Lee AY ((2016) ) Treatment of Alzheimer’s disease with repetitive transcranial magnetic stimulation combined with cognitive training: A prospective, randomized, double-blind, placebo-controlled study. J Clin Neurol 12: , 57–64. |
[72] | Nardone R , Sebastianelli L , Versace V , Ferrazzoli D , Saltuari L , Trinka E ((2021) ) TMS-EEG co-registration in patients with mild cognitive impairment, Alzheimer’s disease and other dementias: A systematic review. Brain Sci 11: , 303. |
[73] | Joseph S , Knezevic D , Zomorrodi R , Blumberger DM , Daskalakis ZJ , Mulsant BH , Pollock BG , Voineskos A , Wang W , Rajji TK , Kumar S ((2021) ) Dorsolateral prefrontal cortex excitability abnormalities in Alzheimer’s Dementia: Findings from transcranial magnetic stimulation and electroencephalography study. Int J Psychophysiol 169: , 55–62. |
[74] | Chu CS , Li CT , Brunoni AR , Yang FC , Tseng PT , Tu YK , Stubbs B , Carvalho AF , Thompson T , Rajji TK , Yeh TC , Tsai CK , Chen TY , Li DJ , Hsu CW , Wu YC , Yu CL , Liang CS ((2021) ) Cognitive effects and acceptability of non-invasive brain stimulation on Alzheimer’s disease and mild cognitive impairment: A component network meta-analysis. J Neurol Neurosurg Psychiatry 92: , 195–203. |
[75] | Guerra A , Assenza F , Bressi F , Scrascia F , Del Duca M , Ursini F , Vollaro S , Trotta L , Tombini M , Chisari C , Ferreri F ((2011) ) Transcranial magnetic stimulation studies in Alzheimer’s disease. Int J Alzheimers Dis 2011: , 263817. |
[76] | Casula EP , Borghi I , Maiella M , Pellicciari MC , Bonni S , Mencarelli L , Assogna M , D’Acunto A , Di Lorenzo F , Spampinato DA , Santarnecchi E , Martorana A , Koch G ((2023) ) Regional precuneus cortical hyperexcitability in Alzheimer’s disease patients. Ann Neurol 93: , 371–383. |
[77] | Chou YH , Sundman M , Ton That V , Green J , Trapani C ((2022) ) Cortical excitability and plasticity in Alzheimer’s disease and mild cognitive impairment: A systematic review and meta-analysis of transcranial magnetic stimulation studies. Ageing Res Rev 79: , 101660. |
[78] | Nardone R , Tezzon F , Holler Y , Golaszewski S , Trinka E , Brigo F ((2014) ) Transcranial magnetic stimulation (TMS)/repetitive TMS in mild cognitive impairment and Alzheimer’s disease. Acta Neurol Scand 129: , 351–366. |
[79] | Julkunen P , Jauhiainen AM , Westeren-Punnonen S , Pirinen E , Soininen H , Kononen M , Paakkonen A , Maatta S , Karhu J ((2008) ) Navigated TMS combined with EEG in mild cognitive impairment and Alzheimer’s disease: A pilot study. J Neurosci Methods 172: , 270–276. |
[80] | Koch G , Bonnì S , Pellicciari MC , Casula EP , Mancini M , Esposito R , Ponzo V , Picazio S , Di Lorenzo F , Serra L , Motta C , Maiella M , Marra C , Cercignani M , Martorana A , Caltagirone C , Bozzali M ((2018) ) Transcranial magnetic stimulation of the precuneus enhances memory and neural activity in prodromal Alzheimer’s disease. Neuroimage 169: , 302–311. |
[81] | Chen HF , Sheng XN , Yang ZY , Shao PF , Xu HH , Qin RM , Zhao H , Bai F ((2023) ) Multi-networks connectivity at baseline predicts the clinical efficacy of left angular gyrus-navigated rTMS in the spectrum of Alzheimer’s disease: A sham-controlled study. CNS Neurosci Ther 29: , 2267–2280. |
[82] | Terry RD , Masliah E , Salmon DP , Butters N , DeTeresa R , Hill R , Katzman R ((1991) ) Physical basis of cognitive alterations in Alzheimer’s disease: Synapse loss is the major correlate of cognitive impairment. Ann Neurol 30: , 572–580. |
[83] | Zhang. XQ , Lan. XY , Chen. CJ , Ren. HX , Guo. Y ((2021) ) Effects of repetitive transcranial magnetic stimulation in patients with mild cognitive impairment: A meta-analysis of randomized controlled trials. Front Hum Neurosci 15: , 723715. |
[84] | Sandrini M , Cappa SF , Rossi S , Rossini PM , Miniussi C ((2003) ) The role of prefrontal cortex in verbal episodic memory: rTMS evidence. J Cogn Neurosci 15: , 855–861. |
[85] | Xie Y , Li Y , Nie L , Zhang W , Ke Z , Ku Y ((2021) ) Cognitive enhancement of repetitive transcranial magnetic stimulation in patients with mild cognitive impairment and early Alzheimer’s disease: A systematic review and meta-analysis. Front Cell Dev Biol 9: , 734046. |
[86] | Iimori T , Nakajima S , Miyazaki T , Tarumi R , Ogyu K , Wada M , Tsugawa S , Masuda F , Daskalakis ZJ , Blumberger DM , Mimura M , Noda Y ((2019) ) Effectiveness of the prefrontal repetitive transcranial magnetic stimulation on cognitive profiles in depression, schizophrenia, and Alzheimer’s disease: A systematic review. Prog Neuropsychopharmacol Biol Psychiatry 88: , 31–40. |
[87] | Budak M , Bayraktaroglu Z , Hanoglu L ((2022) ) The effects of repetitive transcranial magnetic stimulation and aerobic exercise on cognition, balance and functional brain networks in patients with Alzheimer’s disease. Cogn Neurodyn 17: , 39–61. |
[88] | Teti Mayer J , Masse C , Chopard G , Nicolier M , Bereau M , Magnin E , Monnin J , Tio G , Haffen E , Vandel P , Bennabi D ((2021) ) Repetitive transcranial magnetic stimulation as an add-on treatment for cognitive impairment in Alzheimer’s disease and its impact on self-rated quality of life and caregiver’s burden. Brain Sci 11: , 740. |
[89] | Maizey L , Allen CP , Dervinis M , Verbruggen F , Varnava A , Kozlov M , Adams RC , Stokes M , Klemen J , Bungert A , Hounsell CA , Chambers CD ((2013) ) Comparative incidence rates of mild adverse effects to transcranial magnetic stimulation. Clin Neurophysiol 124: , 536–544. |
[90] | Overvliet GM , Jansen RAC , van Balkom A , van Campen DC , Oudega ML , van der Werf YD , van Exel E , van den Heuvel OA , Dols A ((2021) ) Adverse events of repetitive transcranial magnetic stimulation in older adults with depression, a systematic review of the literature. Int J Geriatr Psychiatry 36: , 383–392. |