Betaine Mitigates Amyloid-β-Associated Neuroinflammation by Suppressing the NLRP3 and NF-κB Signaling Pathways in Microglial Cells
Abstract
Background:
Microglia-driven neuroinflammation has been shown to be involved in the entire process of Alzheimer’s disease (AD). Betaine is a natural product that exhibits anti-inflammatory activity; however, the exact underlying molecular mechanisms are poorly understood.
Objective:
Our study focused on determining the effect of betaine against amyloid-β42 oligomer (AβO)-induced inflammation in microglial BV2 cells and investigating the underlying mechanism.
Methods:
AβO was used to establish an in vitro AD model using BV2 cells. A 3-(4,5-dimethylthiazol-2-yl)-2,5-diphenyl-2H-tetrazolium bromide assay was used to measure BV2 cell viability with different concentrations of AβO and betaine. Reverse transcription–polymerase chain reaction and enzyme-linked immunosorbent assays were used to determine the expression levels of inflammatory factors, such as interleukin-1β (IL-1β), interleukin-18 (IL-18), and tumor necrosis factor α (TNF-α). Western blotting was used to evaluate the activation of the NOD-like receptor pyrin domain containing-3 (NLRP3) inflammasome and nuclear transcription factor-κB p65 (NF-κB p65). Moreover, we used phorbol 12-myristate 13-acetate (PMA) to activate NF-κB in order to validate that betaine exerted anti-neuroinflammatory effects through regulation of the NF-κB/NLRP3 signaling pathway.
Results:
We used 2 mM betaine to treat 5μM AβO-induced microglial inflammation. The administration of betaine effectively decreased the levels of IL-1β, IL-18, and TNF-α without affecting cell viability in BV2 microglial cells.
Conclusion:
Betaine inhibited AβO-induced neuroinflammation in microglia by inhibiting the activation of the NLRP3 inflammasome and NF-κB, which supports further evaluation of betaine as a potential effective modulator for AD.
INTRODUCTION
With the gradual increase in the age of the population, the annual incidence of Alzheimer’s disease (AD) has reached 6.48% [1]. AD is the leading cause of dementia and places a heavy burden on both families and society. However, AD pathogenesis is multifactorial and remains poorly understood. Many studies have suggested that chronic neuroinflammation, especially hyperactivated microglia and excessive production of inflammatory factors, is strongly related to the pathogenesis of AD [2–5]. Microglia express pattern recognition receptors (PRRs) that can interact with amyloid-β (Aβ), the latter of which is the main toxic protein during the progression of AD [6]. The interaction of PRRs with Aβ induces a phenotypic switch from resting to activated microglia with a chronic inflammatory state [7, 8]. In this state, the transcription factor-κB (NF-κB) signaling axis is activated, and nuclear entry of NF-κB drives classical signal transduction pathways [9]. Studies have shown that upregulation of NOD-like receptor pyrin domain containing-3 (NLRP3) brings NLRP3, apoptosis-associated speck-like protein (ASC), and pro-caspase 1 proteins together to form the NLRP3 inflammasome [10]. The activation of NLRP3 leads to the cleavage of active caspase-1, which further produces mature inflammatory factors such as interleukin-1β (IL-1β), IL-18, and tumor necrosis factor-α (TNF-α), thereby resulting in neuronal damage [11].
Previous studies have revealed that targeting microglia-driven molecular pathways can mitigate neuroinflammation and provide reliable therapeutic intervention for AD progression [12]. Currently, a variety of natural products and components derived from herbs, such as lignans, flavonoids, and tannins, inhibit the activation of microglia to suppress harmful inflammation and could be promising for postponing the progression of AD [13]. Betaine is commonly found in natural plants and animals, including seafood, especially marine invertebrates, wheat germ or bran, and spinach. Betaine is suggested to be added to the diet as a supplement, which is rapidly absorbed and utilized as an osmolyte and source of methyl groups and thereby helps to maintain liver, heart, and kidney health [14]. Besides, betaine has anti-inflammatory effects. Its use has been suggested for the treatment of liver inflammation [15, 16] and acute severe ulcerative colitis [17]. Additionally, a previous study has shown that betaine markedly inhibits neuroinflammation in LPS-activated N9 microglia [18]. However, whether betaine can effectively alleviate the inflammatory response in an AD microglial model and the exact molecular mechanism are unclear. Therefore, our research aimed to reveal the effect of betaine on neuroinflammatory responses in an amyloid-β42 oligomer (AβO)-stimulated BV2 microglial cell model and to evaluate the underlying anti-inflammatory mechanisms in AD.
MATERIALS AND METHODS
Reagents and antibodies
Betaine (molecular formula: C5H11NO2, CAS No.: 107-43-7, purity >98%) was procured from Shanghai Yuanye Bio-Technology Co., Ltd, China. AβO was purchased from China, Peptides Co. 3-(4,5-Dimethylthiazol-2-yl)-2,5-diphenyl-2H-tetrazolium bromide (MTT) was obtained from Sigma-Aldrich, USA. The NF-κB activator phorbol 12-myristate 13-acetate [19] (PMA, a protein kinase C activator that can induce NF-κB activation in BV2 cells; this concentration was confirmed in previous studies; molecular formula: C36H56O8, CAS No.: 16561-29-8, purity >98%) was procured from MedChemExpress, China. A protein quantification (BCA assay) kit was provided by Beyotime Institute of Biotechnology, China. Antibodies against NF-κB p65, NLRP3, ASC, and Caspase-1 were provided by Cell Signaling Technology (USA). Enzyme-linked immunosorbent assay (ELISA) kits were procured from CUSABIO Technology, China (IL-1β ELISA kit: detection range: 31.25 pg/mL-2000 pg/mL; IL-18 ELISA kit: detection range: 1.56 pg/mL-100 pg/mL; TNF-α ELISA kit: detection range: 15.6 pg/mL-1000 pg/mL. Kit intra-assay precision: CV% <8%, interassay precision: CV% <10%).
Cell culture
Microglial BV2 cells (National Infrastructure of Cell Line Resource, China) were cultured in Dulbecco’s modified Eagle’s medium (DMEM) (Grand Island Biological Company, USA) containing 10% fetal bovine serum (FBS, Sigma-Aldrich, USA) and 1% penicillin-streptomycin (Grand Island Biological Company, USA). The microglia-containing medium was incubated in an incubator at 37°C and 5% CO2. We passaged the cells once when they covered 70–80% of the culture dish. The medium was changed once a day.
Preparation of AβO solution
Briefly, 1 mg of AβO powder was dissolved in DMSO to obtain a 1 mM stock solution, which was further diluted with DMEM to a final concentration of 5μM. The soluble fraction was stored at –80°C.
Cell treatment
For quantitative real-time PCR (qRT-PCR) and ELISA, BV2 cells were randomly divided into four groups: 1) the control group, in which cells were starved with DMEM containing 2% FBS and 1% penicillin–streptomycin (reduced serum medium); 2) the AβO-induced neuroinflammatory AD cell model group, in which cells were treated with reduced-serum medium containing 5μM AβO; 3) the AβO-induced neuroinflammatory AD cell model group treated with betaine, in which cells were pretreated with 2 mM betaine for 2 h, and then the medium was replaced with reduced serum medium containing 5μM AβO and 2 mM betaine for the next 6 or 24 h; and 4) a group in which cells were treated with reduced-serum medium containing 2 mM betaine. For western blotting, we set another group to validate the production and release of inflammatory factors through NF-κB/NLRP3 signaling pathway regulation; in this group, the cells were treated with the NF-κB activator 10μM PMA, 5μM AβO, and 2 mM betaine.
MTT cell viability assay
We used the MTT assay to detect BV2 cell viability after treatment with AβO and betaine. BV2 cells in good growth conditions (5×103 cells per well) were collected and plated in 96-well plates. Subsequently, BV2 cells were treated with different concentrations of AβO (0, 0.2, 0.5, 1, 2, 5, 10μM) and betaine (0, 0.1, 0.2, 0.5, 1, 2, 5, 10 mM) for 24 h. After incubation, we removed the culture medium and added 10μL of 5 mg/mL MTT solution to each well for 4 h of culture at 37°C. Then, the supernatant was discarded, 100μL of dimethyl sulfoxide (DMSO; Sigma-Aldrich, USA) was added to each well, and the crystals were fully dissolved by shaking for 5 min. We used a spectrophotometer (Thermo Fisher, Vantaa, Finland) to measure the absorbance at a wavelength of 490 nm to calculate BV2 viability. The concentrations of AβO and betaine that had no toxic effect on BV2 cells were used to establish the model. All experiments were repeated three times.
Quantitative real-time PCR (qRT-PCR)
BV2 cells were plated in 6-well plates (5×105 cells per well), incubated with 2 mM betaine for 2 h and then stimulated with 5μM AβO and 2 mM betaine for 6 h. Total RNA was extracted by an RNAprep Pure Cell/Bacteria Kit (TIANGEN Biotech Co., Ltd, China). A PrimeScript™ RT Reagent Kit with gDNA Eraser (Beijing Solarbio Science & Technology Co., Ltd, China) was used for reverse transcription to cDNA. cDNA was amplified with TB Green® Premix Ex Taq™ II (Beijing Solarbio Science & Technology Co., Ltd, China) in a final volume of 20μL. Quantitative PCR was performed using the Step One Plus Real-time PCR System (Applied Biosystems, Foster City, USA): preincubation at 95°C for 30 s, 40 denaturation cycles at 95°C for 5 s, followed by annealing at 60°C for 30 s and 95°C for 15 s. After the reaction, the results were quantified by SDS software (Applied Biosystems, USA) and converted into Ct values expressed as fold changes. All experiments were repeated three times. The primer sequences (Sangon Biotechnology, China) used for amplification of cDNA from each group are shown in Table 1.
Table 1
PCR primer sequences used
Gene target | Forwards sequence (5’-3’) | Reverse sequence (5’-3’) |
TNF-α | TGCACCACCATCAAGGACTCAA | GACAGAGGCAACCTGACCACTC |
IL-1β | TTTCCTCCTTGCCTCTGATGGG | CCACACGTTGACAGCTAGGTTC |
IL-18 | ACGTGTTCCAGGACACAACA | GGCGCATGTGTGCTAATCAT |
GAPDH | GAAGGGCATCTTGGGCTACAC | GTTGTCATTGAGAGCAATGCCA |
Western blotting
We used a western blotting assay to qualitatively evaluate pathway protein expression. BV2 cells in a good growth state were inoculated into 6-well plates (5×105 cells per well). When the cells were 80% confluent, the medium was replaced with medium containing 2 mM betaine with or without 10 nM PMA. After 2 h, we added 5μM AβO to stimulate the cells for 24 h. Radioimmunoprecipitation assay (RIPA) lysis buffer, phosphatase inhibitors, and protease inhibitors were used to lyse the cells. Specifically, NF-κB was extracted with a Nuclear and Cytoplasmic Protein Extraction Kit, an extraction kit purchased from Beyotime Institute of Biotechnology (China). The concentrations of the proteins were determined using BCA. Proteins were separated and transferred to polyvinylidene fluoride (PVDF) membranes using sodium dodecyl sulfate-polyacrylamide gel electrophoresis (SDS-PAGE) with different concentrations corresponding to the target protein. In the presence of 5% nonfat milk at room temperature for two hours, the membranes were blocked and then incubated with primary antibodies against NLRP3 (1:1000), caspase-1 (1:1000), ASC (1:800), NF-κB p65 (1:1000), GAPDH (1:5000, Abcam), and PCNA (1:3000, Proteintech), which were diluted at the concentrations recommended in the instructions, at 4°C overnight. After full washing with Tris-HCl buffer and 0.1% Tween 20, the membranes were incubated with secondary antibodies at room temperature for 2 h. Finally, the washed film was placed on the densitometric scan, and ECL western blotting reagents were added to visualize the protein signals. GAPDH was used as an internal reference. Protein band densities were quantified using ImageJ (ImageJ version 1.51e, National Institutes of Health, USA). We performed three independent replicates for each target protein.
ELISA
The expression levels of the inflammatory factors IL-1β, IL-18, and TNF-α were quantitatively determined by ELISA. BV2 cells in a good growth state were inoculated into 6-well plates (5×105 per well). At 80% confluence, after pretreatment with 2 mM betaine for 2 h, the cells were cultured in DMEM containing 5μM AβO and 2 mM betaine for the next 24 h. After centrifugation for 5 min at 20,000 rpm, 100μL samples were prepared with reagents and standards as instructed. Then, 100μL of standard or sample was added to each well, and the plate was incubated at 37°C for 2 h. After removing the liquid from each well without washing, 100μL of biotin-conjugated antibody was added to each well, and the plate was incubated at 37°C for 1 h. The liquid was aspirated, and each well was washed 3 times. Then, 100μL HRP-avidin was added to each well, and the plate was incubated at 37°C for 1 h. After aspirating and washing the plates 5 times, 90μL of TMB substrate was added to each well, and the plate was incubated for 15–30 min at 37°C in the dark. The reaction was stopped with stop solution. A spectrophotometer was used to measure the absorbance at a wavelength of 450 nm. Based on the standard curve, the sample values were calculated. Every sample was assayed in triplicate.
Statistical analysis
Three independent replicate experiments were conducted to determine the mean±standard deviation (SD). The normality of data distribution was assessed using the Shapiro–Wilk test. The statistical significance of differences among groups was determined by one-way analysis of variance (one-way ANOVA) and Tukey’s post hoc test for normally distributed data. Bonferroni correction was used to analyze nonnormally distributed data. We used GraphPad Prism version 8.0.1 (GraphPad Prism Software, Inc., USA) for all statistical analyses. Differences with p < 0.05 were considered statistically significant.
RESULTS
Effects of betaine and AβO on the activation of BV2 cells
To establish an AD cell model to simulate the mechanism of neuroinflammation, the MTT assay was used to determine the most suitable concentrations of AβO and betaine. To avoid drug cytotoxicity and to maximize the pharmaceutical effect, we chose the maximum concentrations of AβO and betaine that had no marked influence on the activation of BV2 cells. The results are shown in Fig. 1. Concentrations of betaine below 5 mM did not significantly reduce BV2 cell viability; thus, 2 mM was selected as the therapeutic concentration of betaine for subsequent experiments (Fig. 1A). To establish a more stable and suitable inflammatory AD cell model and to avoid causing cell death, we selected a concentration of 5μM AβO to stimulate BV2 cells (Fig. 1B).
Fig. 1
Effects of betaine and AβO on the activity of BV2 cells. After 24 h of treatment with (A) betaine or (B) AβO, MTT assays confirmed BV2 cell viability. The data were collected from three independent experiments (n = 3). ***p < 0.001, ****p < 0.0001 versus the control group.
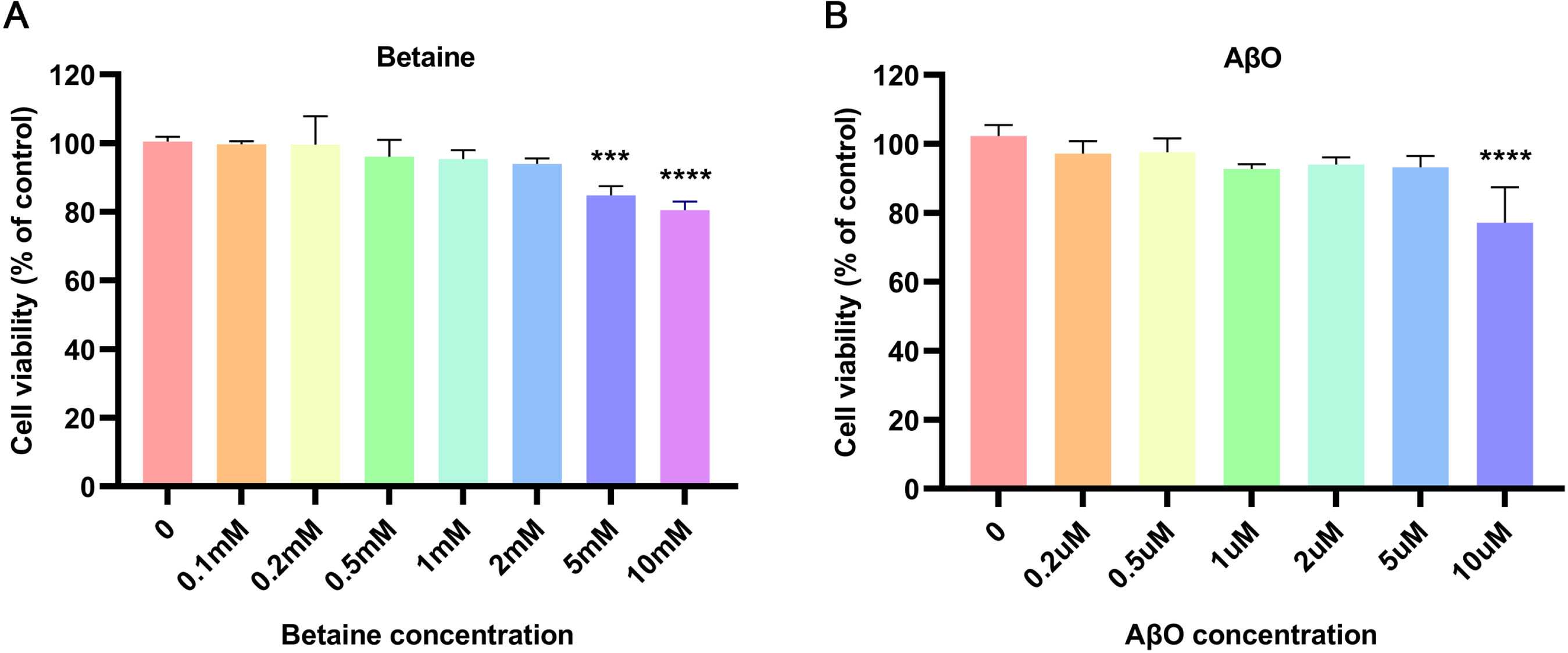
Betaine inhibited the AβO-induced production of inflammatory factors in BV2 cells
We used qRT-PCR to detect the mRNA levels of the inflammatory factors IL-1β, IL-18, and TNF-α in each group, and the results are shown in Fig. 2. We found that after 5μM AβO treatment, the mRNA levels of IL-1β, IL-18, and TNF-α were significantly increased to 1.68 (Fig. 2A, p = 0.0006), 2.08 (Fig. 2B, p = 0.0017), and 1.50 times (Fig. 2C, p = 0.0029) compared with those in the control group and were significantly reduced to 67.24% (Fig. 2A, p = 0.0025), 54.54% (Fig. 2B, p = 0.0038), and 68.54% (Fig. 2C, p = 0.0040) in the betaine-pretreated group, respectively.
Fig. 2
Effect of betaine on AβO-induced inflammatory cytokine production in BV2 cells. A–C) The mRNA levels of IL-1β, IL-18, and TNF-α were analyzed by qRT-PCR. The data were collected from three independent experiments (n = 3). **p < 0.01, ***p < 0.001 versus the control group. # #p < 0.01 versus the AβO group.
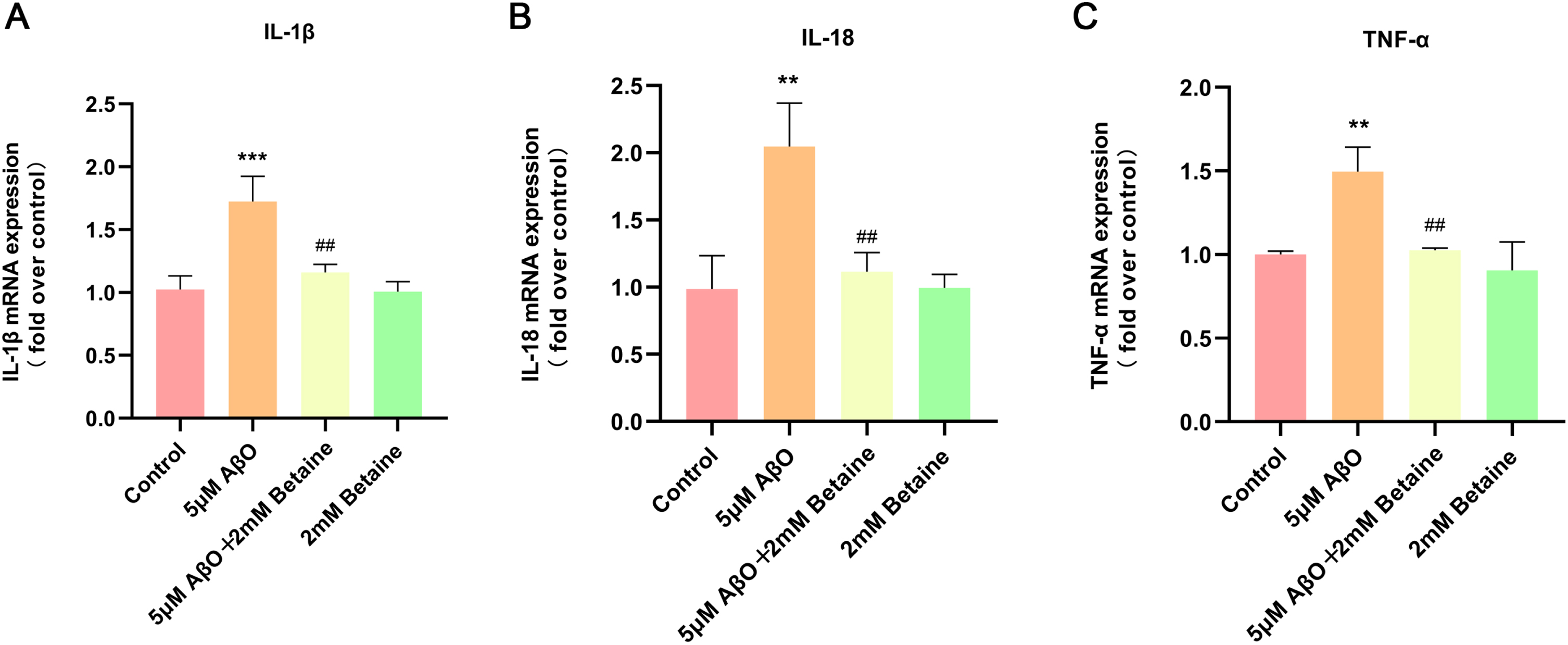
Then, we further used ELISA to quantify the changes in these inflammatory factors (Fig. 3). We found that the release of IL-1β, IL-18 and TNF-α was dramatically elevated to 1.96, (Fig. 3A, p = 0.0004), 3.77 (Fig. 3B, p = 0.0002), and 2.68 (Fig. 3C, p < 0.0001) with treatment of AβO, respectively, compared with that in the control group; however, the administration of betaine significantly decreased the release of these cytokines to 70.47% (Fig. 3A, p = 0.0104), 60.03% (Fig. 3B, p = 0.0119), and 50.86% (Fig. 3C, p < 0.0001) in the betaine-pretreated group. Both qRT-PCR and ELISA results showed that the betaine-treated group and the control group were not significantly different.
Fig. 3
The levels of IL-1β, IL-18, and TNF-α in the supernatant were determined by ELISA. The data were collected from three independent experiments (n = 3). ***p < 0.001, ****p < 0.0001 versus the control group. #p < 0.05, # # # #p < 0.0001 versus the AβO group.
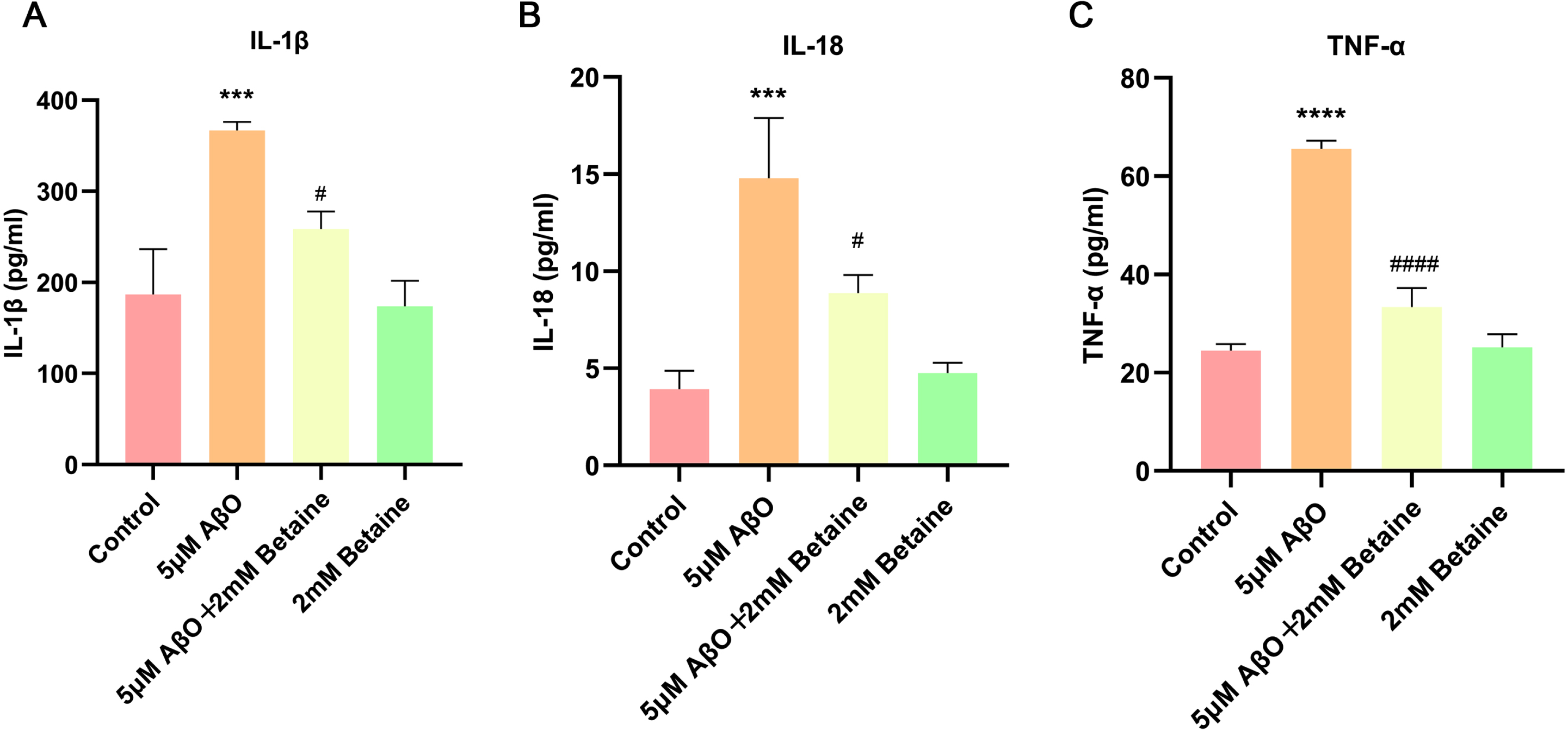
Betaine can attenuate the activation of the NLRP3 inflammasome in AβO-treated BV2 cells
It is well known that the NLRP3 inflammasome is a key protein in proinflammatory cytokine transcriptional regulation. To further reveal the mechanism of the neuroprotective effect of betaine, we used western blotting to verify the protein levels of the NLRP3/caspase-1-related pathway (Fig. 4). Compared with the control group, AβO promoted the expression of NLRP3 (Fig. 4A), caspase-1 (Fig. 4B), and ASC (Fig. 4C) in microglia, while betaine pretreatment inhibited the promotion effect of AβO on the expression of NLRP3 (Fig. 4A), caspase-1 (Fig. 4B), and ASC (Fig. 4C). In addition, NLRP3, caspase-1 and ASC protein expression in BV2 cells treated with 10 nM PMA [20] was significantly increased compared to that in the betaine-pretreated AβO group (Fig. 4). Our results demonstrated that the levels of NLRP3, ASC and caspase-1 were increased during AβO-induced microglial inflammation, which further suggested that the increased level of the inflammatory complex leads to the activation of caspase-1 and the subsequent cleavage of the inflammatory cytokines IL-18 and IL-1β.
Fig. 4
Betaine attenuates activation of the NLRP3 inflammasome in AβO-treated BV2 cells. A–C) Determination of NLRP3, caspase-1 and ASC protein levels. The data were collected from three independent experiments (n = 3). *p < 0.05, **p < 0.01 versus the control group. #p < 0.05 versus the AβO group. &p < 0.05 versus the AβO+betaine group.
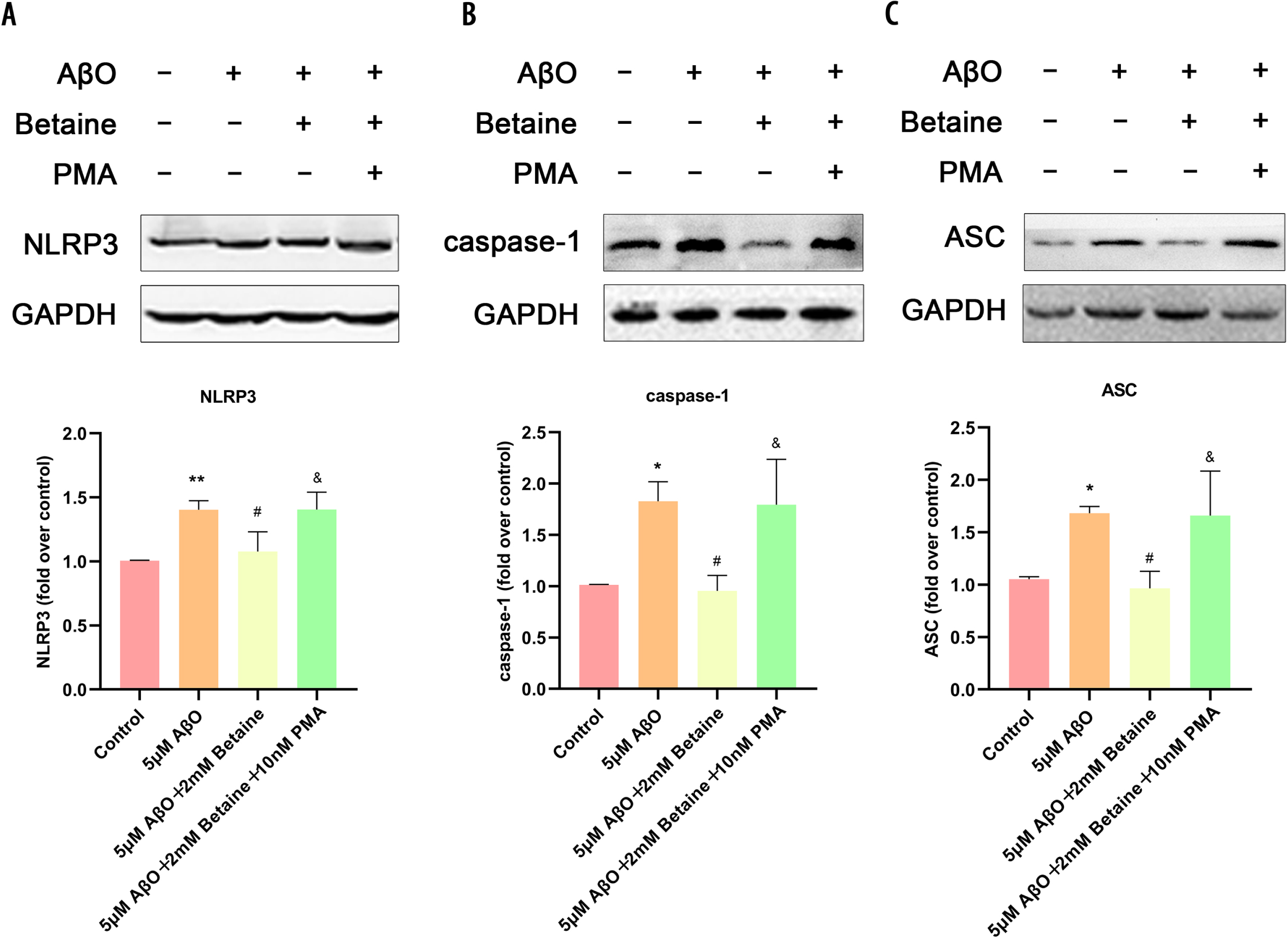
Betaine attenuated the activation of the NF-κB pathway in AβO-treated BV2 cells
We investigated whether betaine could regulate NF-κB pathway activation in BV2 cells (Fig. 5), as the NF-κB pathway is involved in regulating NLRP3 inflammasome activation. Figure 5 show that after treatment with AβO, the expression of NF-κB p65 in the cytoplasm of microglia was lower than that in the control group, which suggested that AβO significantly promoted NF-κB p65 nuclear translocation; however, this response was suppressed by betaine. The above data indicated that betaine inhibited the activation of the NLRP3 inflammasome as well as the NF-κB pathway when used to suppress inflammation, which is the upstream signaling pathway of the NLRP3 inflammasome and can regulate NLRP3 inflammasome activation, in AβO-treated BV2 cells.
Fig. 5
Betaine attenuates the activation of the NLRP3 inflammasome and the NF-κB pathway in AβO-treated BV2 cells. Cytoplasmic (A) and nuclear NF-κB (p65) (B) levels were analyzed by western blotting. The data were collected from independent experiments (n = 3). **p < 0.01, ***p < 0.001 versus the control group. # # #p < 0.001 versus the AβO group. &p < 0.05, &&p < 0.01 versus the AβO+betaine group.
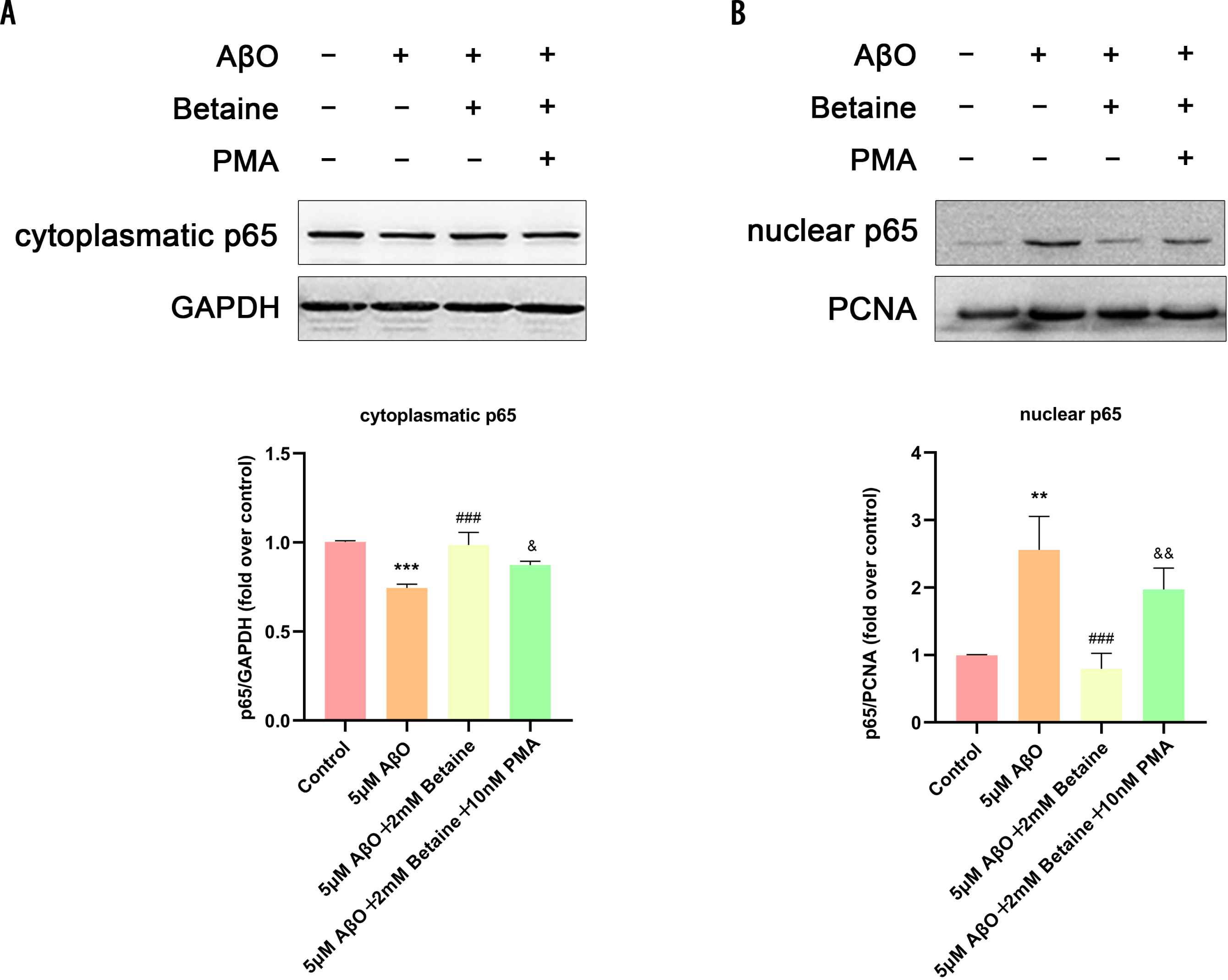
DISCUSSION
In our study, betaine suppressed NLRP3 inflammasomes and NF-κB to reduce the levels of IL-1β, IL-18, and TNF-α. Betaine mitigated AβO-associated neuroinflammation in microglial cells. This is the study to identify the anti-neuroinflammatory effect and the exact molecular mechanism of betaine in a microglial model of AD. This effect resulted from the inhibition of inflammation mediated through the NF-κB/NLRP3 pathway. As a result of these studies, new therapeutic candidates and targets for AD can be identified.
Fig. 6
Schematic diagram of the anti-neuroinflammatory mechanisms of betaine. AβO can bind to PRRs on the surface of microglia, promote NF-κB translocation into the nucleus, and trigger NLRP3, TNF-α, pro-IL-1β, and pro-IL-18 transcription and expression, which promotes NLRP3 inflammasome activation. The NLRP3 inflammasome then interacts with ASC to induce caspase-1 cleavage and maturation. Betaine simultaneously inhibits NF-κB and NLRP3 to exert anti-neuroinflammatory effects and reduce the production of IL-1β and IL-18 in microglial cells.
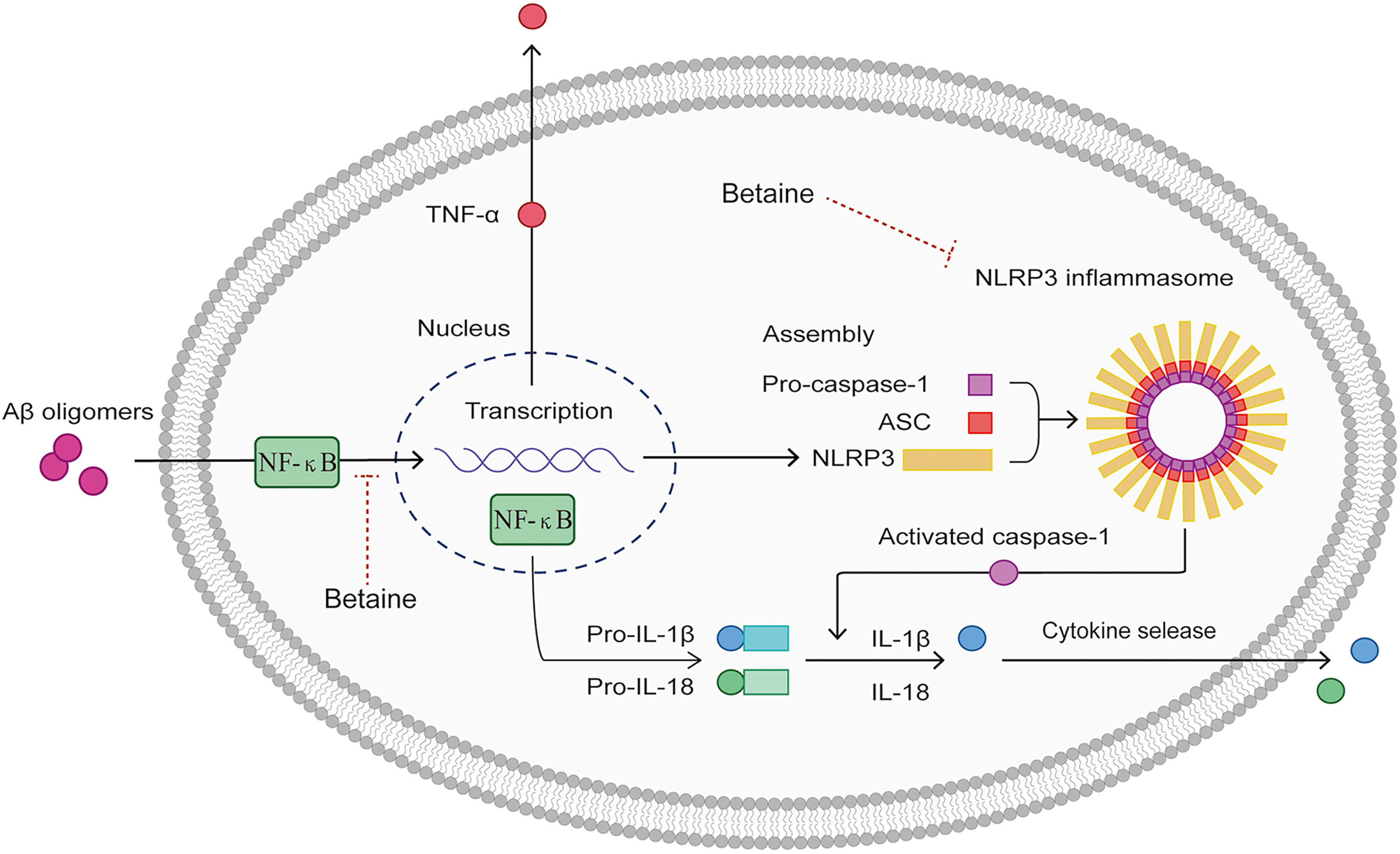
The characteristics of AD-related neuroinflammation include reactive microglia around Aβ plaques [21]. Aβ plaques bind to different PRRs to induce microglial activation and inflammatory responses [22, 23]. Microglial overactivation may result in elevated levels of receptors on cell surfaces, as well as increased expression of cytokines and chemokines, which induce inflammation. Numerous studies have confirmed that proinflammatory mediators produced by the activation of microglia have neurotoxic effects and disrupt synaptic activity, consequently leading to cognitive deficits and neurodegeneration [24–27]. Thus, we selected the BV2 cell line treated with AβO, which is a known inducer of neuroinflammation, as an in vitro model of AD for simulating microglial activation. Our results showed that treatment with 5μM AβO effectively activated microglia and induced inflammatory responses without microglial cell death, consistent with previous studies [28, 29].
What is the fundamental process by which betaine has anti-neuroinflammatory effects? The NF-κB p50 and p65 subunits make up the homodimeric or heterodimeric protein complex known as the NF-B complex. The NF-κB dimers p50 and p65 are kept dormant in the cytoplasm and in complexes with IκB family inhibitor proteins under physiological circumstances [30]. The phosphorylation and degradation of IκB in AD is mediated by long-term activation of PRRs, which also permits the NF-κB p65 subunit to enter the nucleus and bind to a particular DNA consensus sequence, increasing the inflammatory response to Aβ [31]. Our research demonstrated that AβO enhanced the nuclear levels of NF-κB (p65) in BV2 cells but that NF-κB (p65) expression was lowered in the cytoplasm, leading to nuclear translocation of NF-κB (p65). Betaine treatment at a 2 mM concentration reduced the nuclear translocation of NF-κB. Additionally, the inhibitory effect of betaine on the NF-κB pathway was greatly reduced by treatment with PMA (10 nM), an activator of this system. This further validated that betaine inhibited neuroinflammation by suppressing NF-κB activation in BV2 cells exposed to AβO.
A sizable cytosolic multiprotein complex called the NLRP3 inflammasome is composed of caspase-1, NLRP3, and ASC [32]. Studies have shown that microglia express NLRP3 at a higher level than neurons and astrocytes and that AβO can effectively cause inflammasome activation [33]. When PRRs bind to Aβ during AD, the NLRP3 inflammasome assembles and is activated by NF-κB activation in microglia, which results in the activation of caspase-1-mediated inflammatory responses [34], including the cleavage and noncanonical secretion of the proinflammatory cytokines IL-1β and IL-18 [35] and initiation of an inflammatory form of cell death known as pyroptosis [36]. Recent research revealed that in both severe and mild AD patients, the mRNA levels of the inflammasome components NLRP3 and caspase-1, as well as the downstream effectors IL-1 and IL-18, were upregulated [37]. Genetic research using Nlrp3 or Casp1 knockout mice has also shown that NLRP3 inflammasome deficits prevent APP/PS1 mice from cognitive decline and amyloid deposition [38]. An earlier study showed that the activation of NLRP3 inflammasome genes was reduced by betaine in the livers of diabetic mice [39]. Mechanistically, the authors suggested that betaine indirectly activated PKB/Akt, which resulted in forkhead box O1 (FOXO1) inactivation via phosphorylation of activated FOXO1 to induce its transfer from the nucleus into the cytoplasm [40]; decreased expression of thioredoxin interacting protein, which functions as an endogenous inhibitor of ROS-scavenging proteins [41]; and decreased ROS, leading to inhibition of the NLRP3 inflammasome. Hence, experts speculate that betaine inhibits inflammation factor processing by blocking NLRP3 inflammasome activation directly or through the IRS-1/PKB/Akt/FOXO1 signaling pathway to prevent activation of NLRP3 indirectly. The pathway of the NF-κB and NLRP3 inflammasome controls genes encoding proinflammatory cytokines [14]. In an early study on aged kidneys, researchers found that betaine can suppress NF-κB activity and the expression of inflammatory factor genes, such as TNF-α and IL-1 [42], and increasing numbers of in vitro and in vivo studies have demonstrated that betaine inhibits inflammatory cytokine production by dampening NF-κB activation [43]. Moreover, activated caspase-1 in the canonical inflammasome complex is the most extensively identified mechanism for IL-1β and IL-18 processing, and there are currently no lines of evidence (direct or indirect) regarding the effects of betaine on inflammasome-independent sources of proinflammatory cytokines [43]. The above results are similar to ours. Our research demonstrated that AβO increased the expression of NLRP3, caspase-1, and ASC in microglia, and betaine intervention greatly decreased the expression of these proteins, hence lowering the release of mature IL-18 and IL-1β in the following steps. This finding further demonstrated that betaine plays an anti-neuroinflammatory role by inhibiting AβO-induced NLRP3 inflammasome activation in microglia, thereby inhibiting the production of downstream inflammatory factors. In addition, PMA reduced the inhibitory effect of betaine on the NLRP3 inflammasome, indicating that NF-κB inhibits microglial activation by meditating the downstream NLRP3 signaling pathway (Fig. 6).
A previous study showed that betaine reduced AβO-induced degeneration in an AD model of the nematode Caenorhabditis elegans by lowering homocysteine levels [44]. Recently, Ibi et al. found that betaine intake prevented the development of cognitive impairment in 3xTg mice and suppressed the expression of genes involved in synapses and antioxidant activity in the hippocampi of 3xTg mice [45], prompting a further investigation into the possible mechanisms involved. The NLRP3 inflammasome and several of its upstream signaling pathways, such as the NF-κB signaling pathway, provide promising pharmacological targets for AD. Many traditional Chinese medicine monomers, such as baicalin [46], mangiferin [47], and stigmasterol [48], have been explored in in vivo and in vitro AD models. It has been proven that many traditional Chinese medicine monomers with anti-inflammatory properties can suppress the activation of the NLRP3 inflammasome by suppressing the NF-κB signaling pathway and then mitigate chronic neuroinflammation. Our results are consistent with the few available studies that have demonstrated that betaine suppresses Aβ-induced neuroinflammation [49]. We first proposed and verified the inhibitory effect of betaine on NF-κB and the NLRP3 inflammasome in an AD inflammatory model in vitro.
The main limitation of our study is that it was carried out in a cell model of neuroinflammation in microglia. Whether betaine has a therapeutic effect on cognitive impairment in vivo and a neuroprotective effect in neurons needs further verification. Previous studies have confirmed that persistent activation of the NLRP3 inflammasome triggers microglial dysfunction and reduces autophagy and mitophagy, which forms a vicious feedback loop of Aβ deposition, tau accumulation, and microglial activation [50]. Mueed et al. also reported that betaine supplementation induces autophagy, thereby inhibiting the accumulation of Aβ [51]. However, whether betaine can affect tau and autophagy by inhibiting NLRP3 inflammasome activation in the AD model will be explored in the future.
Conclusion
In conclusion, our research shows that betaine can reduce the transcription and expression of inflammatory factors produced by AβO-induced microglial activation. Furthermore, betaine inhibits the NLRP3 inflammasome by downregulating NF-κB. Therefore, betaine can hinder the chronic neuroinflammatory response induced by AβO, suggesting that betaine could be used to prevent and treat AD.
ACKNOWLEDGMENTS
The authors have no acknowledgments to report.
FUNDING
This study was supported by the Key Project of the National Natural Science Foundation of China (U20A20354); Beijing Brain Initiative from Beijing Municipal Science & Technology Commission (Z201100005520016, Z201100005520017); National major R&D projects of China-Scientific technological innovation 2030 (2021ZD0201802); the National Key Scientific Instrument and Equipment Development Project (31627803); the Key Project of the National Natural Science Foundation of China (81530036).
CONFLICT OF INTEREST
Jianping Jia is Editorial Board Members of this journal but was not involved in the peer-review process nor had access to any information regarding its peer-review.
The remaining author has no conflict of interest to report.
DATA AVAILABILITY
The data supporting the findings of this study are available within the article and its supplementary material.
REFERENCES
[1] | Leng F , Edison P ((2021) ) Neuroinflammation and microglial activation in Alzheimer disease: Where do we go from here? Nat Rev Neurol 17: , 157–172. |
[2] | Fillit H , Ding WH , Buee L , Kalman J , Altstiel L , Lawlor B , Wolf-Klein G ((1991) ) Elevated circulating tumor necrosis factor levels in Alzheimer’s disease. Neurosci Lett 129: , 318–320. |
[3] | Strauss S , Bauer J , Ganter U , Jonas U , Berger M , Volk B ((1992) ) Detection of interleukin-6 and alpha 2-macroglobulinimmunoreactivity in cortex and hippocampus of Alzheimer’s diseasepatients. Lab Invest 66: , 223–230. |
[4] | Calsolaro V , Edison P ((2016) ) Neuroinflammation in Alzheimer’s disease: Current evidence and future directions. Alzheimers Dement 12: , 719–732. |
[5] | Navarro V , Sanchez-Mejias E , Jimenez S , Munoz-Castro C , Sanchez-Varo R , Davila JC , Vizuete M , Gutierrez A , Vitorica J ((2018) ) Microglia in Alzheimer’s disease: Activated, dysfunctional or degenerative. Front Aging Neurosci 10: , 140. |
[6] | Sarlus H , Heneka MT ((2017) ) Microglia in Alzheimer’s disease. J Clin Invest 127: , 3240–3249. |
[7] | Cai Z , Hussain MD , Yan LJ ((2014) ) Microglia, neuroinflammation, and beta-amyloid protein in Alzheimer’s disease. Int J Neurosci 124: , 307–321. |
[8] | Kurt-Jones EA , Popova L , Kwinn L , Haynes LM , Jones LP , Tripp RA , Walsh EE , Freeman MW , Golenbock DT , Anderson LJ , Finberg RW ((2000) ) Pattern recognition receptors TLR4 and CD14 mediate response to respiratory syncytial virus. Nat Immunol 1: , 398–401. |
[9] | Vaure C , Liu Y ((2014) ) A comparative review of toll-like receptor 4 expression and functionality in different animal species. Front Immunol 5: , 316. |
[10] | Nizami S , Hall-Roberts H , Warrier S , Cowley SA , Di Daniel E ((2019) ) Microglial inflammation and phagocytosis in Alzheimer’s disease: Potential therapeutic targets. Br J Pharmacol 176: , 3515–3532. |
[11] | Kaur D , Sharma V , Deshmukh R ((2019) ) Activation of microglia and astrocytes: A roadway to neuroinflammation and Alzheimer’s disease. Inflammopharmacology 27: , 663–677. |
[12] | Singh D ((2022) ) Astrocytic and microglial cells as the modulators of neuroinflammation in Alzheimer’s disease. J Neuroinflammation 19: , 206. |
[13] | Pei H , Ma L , Cao Y , Wang F , Li Z , Liu N , Liu M , Wei Y , Li H ((2020) ) Traditional Chinese medicine for Alzheimer’s disease and other cognitive impairment: A review. Am J Chin Med 48: , 487–511. |
[14] | Zhao G , He F , Wu C , Li P , Li N , Deng J , Zhu G , Ren W , Peng Y ((2018) ) Betaine in inflammation: Mechanistic aspects and applications. Front Immunol 9: , 1070. |
[15] | Wang C , Ma C , Gong L , Dai S , Li Y ((2021) ) Preventive and therapeutic role of betaine in liver disease: A review on molecular mechanisms. Eur J Pharmacol 912: , 174604. |
[16] | Hagar H , Husain S , Fadda LM , Attia NM , Attia MMA , Ali HM ((2019) ) Inhibition of NF-kappaB and the oxidative stress -dependent caspase-3 apoptotic pathway by betaine supplementation attenuates hepatic injury mediated by cisplatin in rats. Pharmacol Rep 71: , 1025–1033. |
[17] | Chen L , Liu D , Mao M , Liu W , Wang Y , Liang Y , Cao W , Zhong X ((2022) ) Betaine ameliorates acute sever ulcerative colitis by inhibiting oxidative stress induced inflammatory pyroptosis. Mol Nutr Food Res 66: , e2200341. |
[18] | Shi H , Wang XL , Quan HF , Yan L , Pei XY , Wang R , Peng XD ((2019) ) Effects of betaine on LPS-stimulated activation of microglial M1/M2 phenotypes by suppressing TLR4/NF-kappaB pathways in N9 cells. Molecules 24: , 367. |
[19] | Listwak SJ , Rathore P , Herkenham M ((2013) ) Minimal NF-kappaB activity in neurons. Neuroscience 250: , 282–299. |
[20] | Han IO , Kim HS , Kim HC , Joe EH , Kim WK ((2003) ) Synergistic expression of inducible nitric oxide synthase by phorbol ester and interferon-gamma is mediated through NF-kappaB and ERK in microglial cells. J Neurosci Res 73: , 659–669. |
[21] | Zhang Y , Zhao Y , Zhang J , Yang G ((2020) ) Mechanisms of NLRP3 inflammasome activation: Its role in the treatment of Alzheimer’s disease. Neurochem Res 45: , 2560–2572. |
[22] | Venegas C , Heneka MT ((2017) ) Danger-associated molecular patterns in Alzheimer’s disease. J Leukoc Biol 101: , 87–98. |
[23] | Althafar ZM ((2022) ) Targeting microglia in Alzheimer’s disease: From molecular mechanisms to potential therapeutic targets for small molecules. Molecules 27: , 4124. |
[24] | Wang WY , Tan MS , Yu JT , Tan L ((2015) ) Role of pro-inflammatory cytokines released from microglia in Alzheimer’s disease. Ann Transl Med 3: , 136. |
[25] | von Bernhardi R , Eugenin-von Bernhardi L , Eugenin J ((2015) ) Microglial cell dysregulation in brain aging and neurodegeneration. Front Aging Neurosci 7: , 124. |
[26] | Cagnin A , Brooks DJ , Kennedy AM , Gunn RN , Myers R , Turkheimer FE , Jones T , Banati RB ((2001) ) In-vivo measurement of activated microglia in dementia. Lancet 358: , 461–467. |
[27] | Di Filippo M , Tozzi A , Costa C , Belcastro V , Tantucci M , Picconi B , Calabresi P ((2008) ) Plasticity and repair in the post-ischemic brain. Neuropharmacology 55: , 353–362. |
[28] | Chen S , Jia J ((2020) ) Tenuifolin attenuates amyloid-beta42-induced neuroinflammation in microglia through the NF-kappaB signaling pathway. J Alzheimers Dis 76: , 195–205. |
[29] | Jiao H , Jia J ((2022) ) Ginsenoside compound K acts via LRP1 to alleviate Amyloid beta(42)-induced neuroinflammation in microglia by suppressing NF-kappaB. Biochem Biophys Res Commun 590: , 14–19. |
[30] | Lawrence T ((2009) ) The nuclear factor NF-kappaB pathway in inflammation. Cold Spring Harb Perspect Biol 1: , a001651. |
[31] | Yu H , Lin L , Zhang Z , Zhang H , Hu H ((2020) ) Targeting NF-kappaB pathway for the therapy of diseases: Mechanism and clinical study. Signal Transduct Target Ther 5: , 209. |
[32] | Ozaki E , Campbell M , Doyle SL ((2015) ) Targeting the NLRP3 inflammasome in chronic inflammatory diseases: Current perspectives. J Inflamm Res 8: , 15–27. |
[33] | Anderson FL , Biggs KE , Rankin BE , Havrda MC ((2023) ) NLRP3 inflammasome in neurodegenerative disease. Transl Res 252: , 21–33. |
[34] | Martinon F , Burns K , Tschopp J ((2002) ) The inflammasome: A molecular platform triggering activation of inflammatory caspases and processing of proIL-beta. Mol Cell 10: , 417–426. |
[35] | Freeman LC , Ting JP ((2016) ) The pathogenic role of the inflammasome in neurodegenerative diseases. J Neurochem 136: (Suppl 1), 29–38. |
[36] | Martinon F , Mayor A , Tschopp J ((2009) ) The inflammasomes: Guardians of the body. Annu Rev Immunol 27: , 229–265. |
[37] | Saresella M , La Rosa F , Piancone F , Zoppis M , Marventano I , Calabrese E , Rainone V , Nemni R , Mancuso R , Clerici M ((2016) ) The NLRP3 and NLRP1 inflammasomes are activated in Alzheimer’s disease. Mol Neurodegener 11: , 23. |
[38] | Heneka MT , Kummer MP , Stutz A , Delekate A , Schwartz S , Vieira-Saecker A , Griep A , Axt D , Remus A , Tzeng TC , Gelpi E , Halle A , Korte M , Latz E , Golenbock DT ((2013) ) NLRP3 is activated inAlzheimer’s disease and contributes to pathology in APP/PS1 mice. Nature 493: , 674–678. |
[39] | Kim DH , Kim SM , Lee B , Lee EK , Chung KW , Moon KM , An HJ , Kim KM , Yu BP , Chung HY ((2017) ) Effect of betaine on hepatic insulin resistance through FOXO1-induced NLRP3 inflammasome. J Nutr Biochem 45: , 104–114. |
[40] | Wang Y , Zhou Y , Graves DT ((2014) ) FOXO transcription factors: Their clinical significance and regulation. Biomed Res Int 2014: , 925350. |
[41] | Li X , Rong Y , Zhang M , Wang XL , LeMaire SA , Coselli JS , Zhang Y , Shen YH ((2009) ) Up-regulation of thioredoxin interacting protein (Txnip) by p38 MAPK and FOXO1 contributes to the impaired thioredoxin activity and increased ROS in glucose-treated endothelial cells. Biochem Biophys Res Commun 381: , 660–665. |
[42] | Go EK , Jung KJ , Kim JY , Yu BP , Chung HY ((2005) ) Betaine suppresses proinflammatory signaling during aging: The involvement of nuclear factor-kappaB via nuclear factor-inducing kinase/IkappaB kinase and mitogen-activated protein kinases. J Gerontol A Biol Sci Med Sci 60: , 1252–1264. |
[43] | Xia Y , Chen S , Zhu G , Huang R , Yin Y , Ren W ((2018) ) Betaine inhibits interleukin-1beta production and release: Potential mechanisms. Front Immunol 9: , 2670. |
[44] | Leiteritz A , Dilberger B , Wenzel U , Fitzenberger E ((2018) ) Betaine reduces beta-amyloid-induced paralysis through activation of cystathionine-beta-synthase in an Alzheimer model of Caenorhabditis elegans. Genes Nutr 13: , 21. |
[45] | Ibi D , Hirashima K , Kojima Y , Sumiya K , Kondo S , Yamamoto M , Ando T , Hiramatsu M ((2021) ) Preventive effects of continuous betaine intake on cognitive impairment and aberrant gene expression in hippocampus of 3xTg mouse model of Alzheimer’s disease. J Alzheimers Dis 79: , 639–652. |
[46] | Jin X , Liu MY , Zhang DF , Zhong X , Du K , Qian P , Yao WF , Gao H , Wei MJ ((2019) ) Baicalin mitigates cognitive impairment and protects neurons from microglia-mediated neuroinflammation via suppressing NLRP3 inflammasomes and TLR4/NF-kappaB signaling pathway. CNS Neurosci Ther 25: , 575–590. |
[47] | Lei LY , Wang RC , Pan YL , Yue ZG , Zhou R , Xie P , Tang ZS ((2021) ) Mangiferin inhibited neuroinflammation through regulating microglial polarization and suppressing NF-kappaB, NLRP3 pathway. Chin J Nat Med 19: , 112–119. |
[48] | Jie F , Yang X , Yang B , Liu Y , Wu L , Lu B ((2022) ) Stigmasterol attenuates inflammatory response of microglia via NF-kappaB and NLRP3 signaling by AMPK activation. Biomed Pharmacother 153: , 113317. |
[49] | Sun J , Wen S , Zhou J , Ding S ((2017) ) Association between malnutrition and hyperhomocysteine in Alzheimer’s disease patients and diet intervention of betaine. J Clin Lab Anal 31: , e22090. |
[50] | Liang T , Zhang Y , Wu S , Chen Q , Wang L ((2022) ) The role of NLRP3 inflammasome in Alzheimer’s disease and potential therapeutic targets. Front Pharmacol 13: , 845185. |
[51] | Mueed Z , Mehta D , Rai PK , Kamal MA , Poddar NK ((2020) ) Cross-interplay between osmolytes and mTOR in Alzheimer’s disease pathogenesis. Curr Pharm Des 26: , 4699–4711. |