PM2.5 and Dementia in a Low Exposure Setting: The Influence of Odor Identification Ability and APOE
Abstract
Background:
Growing evidence show that long term exposure to air pollution increases the risk of dementia.
Objective:
The aim of this study was to investigate associations between PM2.5 exposure and dementia in a low exposure area, and to investigate the role of olfaction and the APOE ɛ4 allele in these associations.
Methods:
Data were drawn from the Betula project, a longitudinal study on aging, memory, and dementia in Sweden. Odor identification ability was assessed using the Scandinavian Odor Identification Test (SOIT). Annual mean PM2.5 concentrations were obtained from a dispersion-model and matched at the participants’ residential address. Proportional hazard regression was used to calculate hazard ratios.
Results:
Of 1,846 participants, 348 developed dementia during the 21-year follow-up period. The average annual mean PM2.5 exposure at baseline was 6.77μg/m3, which is 1.77μg/m3 above the WHO definition of clean air. In a fully adjusted model (adjusted for age, sex, APOE, SOIT, cardiovascular diseases and risk factors, and education) each 1μg/m3 difference in annual mean PM2.5-concentration was associated with a hazard ratio of 1.23 for dementia (95% CI: 1.01–1.50). Analyses stratified by APOE status (ɛ4 carriers versus non-carriers), and odor identification ability (high versus low), showed associations only for ɛ4 carriers, and for low performance on odor identification ability.
Conclusion:
PM2.5 was associated with an increased risk of dementia in this low pollution setting. The associations between PM2.5 and dementia seemed stronger in APOE carriers and those with below average odor identification ability.
INTRODUCTION
There is a growing body of evidence, showing that long term exposure to air pollution can have a detrimental effect on cognitive abilities [1] and increase the risk of dementia [2]. One estimate suggests that 2% of dementia cases can be attributed to air pollution [3]. Research has shown that particles with a diameter less than 2.5μm (PM2.5), a common component of air pollution, can reach the brain and cause chronic neuroinflammations [4] which can become a driving factor of Alzheimer’s disease (AD) pathology [5]. In addition, air pollution is a known risk factor for cardiovascular diseases (CVD), which in turn can cause vascular dementia (VaD).
The olfactory system is of particular interest when investigating associations between pollution and dementia. Impaired olfactory functions are associated with several neurological disorders [6] and can be an early marker for AD [7]. Olfactory impairments predicted conversion to dementia in a study drawing data from the Betula Project in northern Sweden [8], and a US study [9] demonstrated that low odor identification ability 5 years prior to diagnosis was roughly as good a predictor of dementia as screening for known biomarkers (such as amyloid-β, Aβ) in people already experiencing cognitive decline. In addition, one suggested pathway through which particles can reach the brain goes via the olfactory nerve. Experiments using rodents [10], as well as postmortem examinations of canines [11] and humans [12, 13], suggest that airborne particles bind to olfactory neurons, and reach the olfactory bulb through retrograde transport [14]. Finally, because olfactory receptors in the nasal cavity are directly exposed to inhaled air, olfactory functions could be especially vulnerable to air pollution exposure. A 2016 review [15] concludes that air pollution is associated with decreased olfactory functions. A recent study [16] found associations between air pollution and decline in odor identification ability, in an area with relatively low levels of pollution. However, a previous study also using data from the Betula Project [17] found no associations between air pollution and olfactory functions.
The ɛ4 allele of the APOE gene is also an important factor to consider. APOE ɛ4 is a known risk factor for both AD [18] and VaD [19] and affects many cognitive abilities, including olfactory functions [20]. Studies conducted in Mexico City, a highly polluted area, showed associations between air pollution and olfactory bulb pathology (e.g., presence of Aβ42) even in young age [21], and that APOE ɛ4 accelerated this process [22]. One study, also using data from the Betula project, found associations between APOE ɛ4 and declining odor identification ability, independent of clinical dementia [20]. A few studies have investigated whether APOE ɛ4 carriers are more sensitive to the detrimental effects of air pollution on the brain. These have found stronger associations between air pollution and cognitive outcomes, such as deficits in visuo-spatial functions [23], risk of dementia [24], and rate of cognitive decline [25], in APOE ɛ4 carriers compared to non-carriers. However, a recent British study [26] using a large sample (n = 187,194) drawn from the UK Biobank, found no evidence of a moderating effect of APOE on the relationship between air pollution and dementia. A previous study using data from the Betula Project [27] used NOx as exposure variable and found no modifying effect of APOE.
The aim of this study was to investigate the associations between PM2.5 exposure and dementia, in a low exposure area. Furthermore, we wanted to increase the understanding of the role of olfaction and the APOE ɛ4 allele in the associations between air pollution and dementia.
METHODS
Participants and study sample
The Betula project is a population-based prospective cohort study on memory, health, and aging, that has been described in detail elsewhere [28, 29]. Briefly, the first test wave (T1) took place in 1988-1989 as a first sample (S1) of 1000 participants were randomly selected from the population registry, stratified by age and sex. The participants were recruited from Umeå municipality in northern Sweden and were distributed among 10 age cohorts, five years apart and ranging from 35 to 80 years of age. At each test wave, each participant underwent two test sessions, about one week apart. The first focused on a health examination and the second on cognitive examinations. Every five years new test waves (T2-T6) were conducted with new samples (S2-S6) being introduced, and samples from previous test waves being subject to follow-ups. In the current study we used test wave 3 (T3, 1998-2000) of the Betula project as baseline. Thus, we initially included all participants (n = 2,830) tested at T3. Participants younger than 55 years at baseline were excluded, as were participants with missing PM2.5 estimates. Participants were considered lost to follow-up, and thus not included in the study sample, if any of the following criteria were met at end of follow-up: 1) unknown dementia status; 2) diagnosed with dementia of a type other than AD or VaD (e.g., Lewy body dementia, or Parkinson’s dementia); 3) date of dementia onset predated baseline test date. The final study sample consisted of n = 1,846 participants (see Fig. 1).
Fig. 1
Flow chart from baseline to end of follow up.
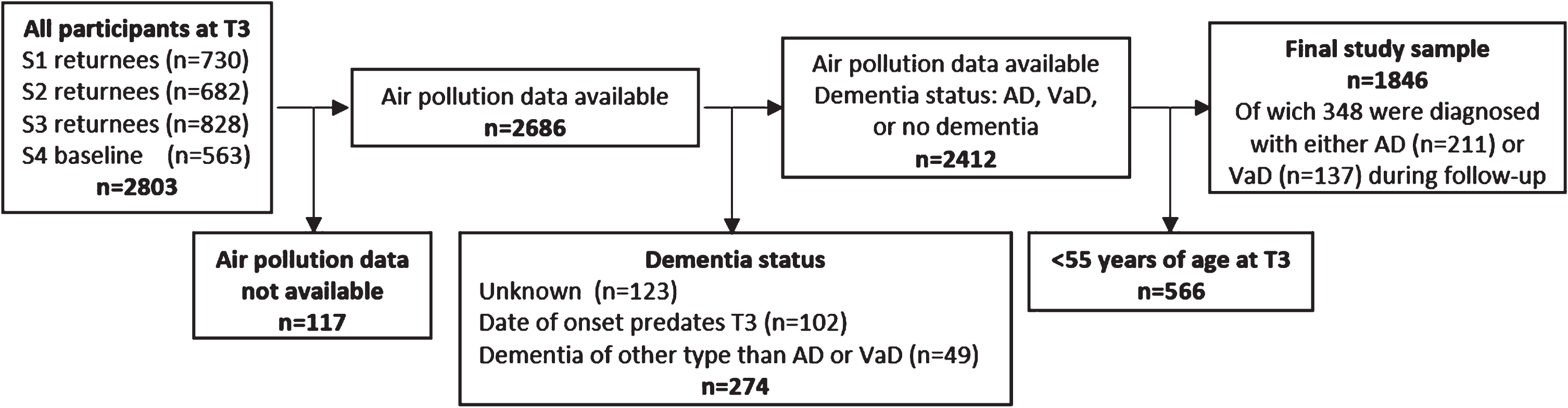
Exposure assessment
Estimates of annual mean levels of PM2.5 at the participant’s residential address at baseline (2000) were obtained from the Swedish Meteorological and Hydrological Institute. The PM2.5 estimates were based on a wind model and a Gaussian air quality dispersion model, the calculations of which have been described elsewhere [30]. Both regional and local emission inventories for the year 2000 were used as input to Gaussian dispersion model simulations of annual mean concentrations of PM2.5. For inner-city streets with buildings on one or both sides, an additional concentration component was simulated with the Danish operational street pollution model [31]. Emission factors for traffic PM-exhaust for different vehicle types, speeds and driving conditions were calculated based on the Handbook on Emission Factors for Road Traffic version 3.1 [32].
The non-exhaust emissions of PM consist mainly of road wear particles, with a minor contribution from brake and tire wear [33, 34]. For small-scale residential heating, emissions were based on a detailed inventory of individual stoves and boilers, in combination with data from chimney sweepers and interviews about the amount of wood burning [30]. Industrial and energy production facilities were included as point sources in the model. Emissions from shipping were also included using a similar method as described elsewhere [35]. Finally, the annual mean long-range contribution of PM2.5 was estimated from the rural background station Vindeln 50 km northwest of Umeå and assumed to be the same for all addresses. Thus, the PM2.5 measure used in the present study mainly depicts within-city contrasts of locally emitted air pollutants, as the long-range contribution only varies across years, not within the study area. The resulting concentrations were lastly added to each study participant’s home addresses. The spatial resolution of the model grids varied from 50 m x 50 m in urban areas, up to 3200 m x 3200 m in the most rural areas.
Dementia diagnoses
The procedure for assessing dementia status has been described in detail elsewhere [29]. In short, data to assess dementia status and disease onset was primarily drawn from clinical information in medical records, supplemented by outcomes from the Betula health- and cognitive testing. The diagnostic protocol did not systematically include cerebrospinal fluid or neuroimaging biomarkers nor postmortem neuropathological findings, but such information was part of the diagnostic decision when available. The information was sufficient for applying clinical criteria-based systems, such as the DSM-IV classification core criteria for dementia [36]. The diagnostic evaluation was performed by the same geropsychiatrist (co-author RA) throughout the study.
APOE genotyping
As part of the Betula project, blood samples were collected, and DNA was extracted to allow for genotyping of selected candidate genes implicated in AD, such as the APOE gene (see [37] for further details). In this study, participant’s APOE status was classified as being either a carrier (homo- or heterozygotic), or non-carrier, of the ɛ4 allele. We were not able to stratify the sample by specific genotypes, as the group sizes would become too small. The frequencies of the different genotypes in our study sample were as follows: ɛ2/ɛ2, n = 6; ɛ2/ɛ3 n = 196; ɛ2/ɛ4, n = 36; ɛ3/ɛ3, n = 904; ɛ3/ɛ4, n = 347; ɛ4/ɛ4, n = 31.
Odor identification
From T3 and onwards, a version [38] of the Scandinavian Odor Identification Test (SOIT) [39] was administered to participants in order to assess odor identification ability. In this version of SOIT, the response alternatives were more similar to the stimuli than in the original version in order to avoid ceiling effects in a large sample. The procedure has been described in detail elsewhere [40]. In short, the test consisted of thirteen odorants, most of them in the form of etheric oils, assumed to be familiar to the study population (e.g., vanilla, orange, bitter almond). The odors were presented one at the time, and after each presentation the participants chose one of four response alternatives. The test score equals the number of correct answers.
Covariates
A number of covariates, all known from previous research to impact cognitive functions and risk of dementia, were included in the analyses. Data on education level for the year 2000 was retrieved through Statistics Sweden and re-coded into three educational levels: compulsory (up to nine years), high school, and post-secondary education. All other covariates: age, sex, and self-reported data on smoking habits and medical history, were drawn from T3 of the Betula project. Smoking status was categorized as “current or former smoker” or “non-smoker”. Participant who reported having had stroke, heart disease, diabetes, or high blood pressure—all of which are risk factors for dementia—within the five-year period prior to T3, were classified as having a history of cardiovascular diseases and risk factors (CVDRF). In one model, we also included results from the Swedish synonym test (SRB-1). In this test, participants were presented to 30 Swedish words on paper. For each of these words five alternatives were given, and the task was to select the alternative that best described the original word. The score was the number of correct answers.
Statistical analyses
Frequencies and percentages were used to describe dementia status, sex, APOE ɛ4 status, level of education, and history of CVDRF. Frequencies, means, and standard deviations were used to describe annual mean PM2.5 exposure, age, and SOIT-score. Cox proportional hazard models were used to calculate hazard ratios (HR) and 95% confidence intervals (CI) for dementia incidence. The time scale in the model was “years”. Censoring occurred when a participant either died, was diagnosed with dementia, was lost to follow-up, moved outside the catchment area of the Betula study, or at the end of the study period. The first model was adjusted for age. The second was additionally adjusted for sex, APOE ɛ4 status, SOIT-score, education level, and history of CVDRF. Individuals with missing data on any confounder were left out of the models listwise. Interaction analyses were performed using Cox proportional hazard models that included the two variables of interest and an interaction term created by multiplying these two variables. When investigating interaction effects, we adjusted our models using the same covariates as in the fully adjusted model, with the exception of CVDRF and education, since these covariates did not show significant associations with risk of dementia (see Table 2). All statistical analyses were conducted using SPSS version 26 software.
Table 2
Hazard ratios (HR) and 95% confidence intervals (CI) for dementia from Cox proportional hazard regressions (*p<0.05, **p<0.01, ***p<0.001)
Age adjusted model | ||
HR | 95% CI | |
PM2.5 a | 1.20 | 1.00–1.45 |
Age | 1.09*** | 1.08–1.11 |
Fully adjusted model | HR | 95% CI |
PM2.5 a | 1.23* | 1.01–1.50 |
Age | 1.11*** | 1.10–1.13 |
Female | 1.64*** | 1.28–2,10 |
APOE ɛ4 carrier b | 2.28*** | 1.81–2.86 |
SOIT-score c | 0.91** | 0.87–0.97 |
History of CVDRF d | 0.85 | 0.68–1.06 |
Current or former smoker | 1.16 | 0.92-1.48 |
Education | ||
Compulsory | ref. | |
High school | 0.81 | 0.63–1.06 |
Post-secondary | 0.98 | 0.73–1.33 |
Notes: a) per 1μg/m3 increase; b) homo- or heterozygotic carrier of the ɛ4 allele; c) score on the Scandinavian Odor Identification Test (0–13); d) had any of the following conditions during the five-year period prior to baseline: heart disease, stroke, hypertension, or diabetes.
RESULTS
The study sample consisted of 1,846 participants. Baseline characteristics for the study population are summarized in Table 1. The time of follow-up ranged between 1-21 years, with an average time of follow-up of 12.2 years (SD: 5.76). During follow up, 211 participants were diagnosed with AD and 137 with VaD. The average annual mean PM2.5 exposure was 6.77μg/m3.
Table 1
Dementia incidence at end of follow-up, and population characteristics at baseline
No dementia | Incident dementia during follow-up | ||
AD | VaD | ||
n=1498 | n=211 | n=137 | |
Years of follow-up, mean (SD) | 13.18 (5.5) | 7.7 (5.0) | 8.15 (4.6) |
PM2.5 exposure, μg/m3, mean (SD) | 6.76 (0.56) | 6.81 (0.57) | 6.83 (0.49) |
Age, mean (SD) | 68.9 (10.4) | 73.7 (7.9) | 75.0 (6.7) |
Sex | |||
Female, n (%) | 799 (53.3) | 152 (72.0) | 86 (62.8) |
Male, n (%) | 699 (46.7) | 59 (28.0) | 51 (37.2) |
APOE ɛ4 carrier, n (%)a | 340 (22.7) | 100 (47.4) | 42 (30.7) |
Odor identification score, mean (SD)b | 6.80 (2.20) | 6.17 (2.35) | 6.13 (2.24) |
Missing, n (%) | 121 (8.1) | 14 (6.6) | 6 (4.4) |
History of CVDRF, n (%)c | 749 (50.0) | 97 (46.0) | 88 (64.2) |
Smoking | |||
Current or former smoker n (%) | 827 (44.8) | 83 (39.3) | 62 (45.3) |
Non-smoker n (%) | 977 (52.9) | 122 (57.8) | 74 (54.0) |
Missing n (%) | 42 (2.3) | 6 (2.8) | 1 (0.7) |
Education, n (%) | |||
Compulsory, n (%) | 523 (34.9) | 101 (47.9) | 68 (49.6) |
High School, n (%) | 554 (37.0) | 68 (32.2) | 38 (27.7) |
Post-secondary, n (%) | 306 (20.4) | 36 (17.1) | 31 (22.6) |
Missing, n (%) | 115 (7.7) | 6 (2.8) | 0 (0) |
SRB, mean (SD) | 21.5 (5.4) | 20.5 (5.3) | 20.3 (5.7) |
Missing, n (%) | 79 (5.3) | 10 (4.7) | 6 (4.4) |
a) homo- or heterozygotic carrier of the ɛ4 allele; b) score on the Scandinavian Odor Identification Test (0–13); c) had any of the following conditions during the five-year period prior to baseline: heart disease, stroke, hypertension, diabetes.
Cox proportional hazard regressions were used to investigate associations between PM2.5 and dementia incidence. As shown in Table 2, a 1μg/m3 difference in annual mean PM2.5-exposure was associated with a hazard ratio of 1.24 in the fully adjusted model. In our study sample, the risk of dementia also increased with high age, presence of at least one APOE ɛ4 allele, female sex, and low SOIT-score. No significant associations were found between dementia incidence and history of CVDRF, or level of education.
When stratified by dementia subtype (AD and VaD), the associations between PM2.5 and dementia in the fully adjusted model were no longer significant (for AD: n = 1709, HR = 1.27, 95% CI: 0.99–1.63, p = 0.065; for VaD: n = 1635, HR = 1.31, 95% CI: 0.95–1.79, p = 0.096).
Because odor identification to some extent is dependent on semantic memory, we re-ran the analysis (using the fully adjusted model) while also adjusting for SRB. This only marginally changed the associations between air pollution and dementia (HR = 1.23, 95% CI: 1.01–1.50).
A significant interaction effect was found between PM2.5 and SOIT-score (HR = 0.90, 95% CI: 0.82–0.99 p = 0.031). Because APOE ɛ4 is associated with both risk of dementia, and olfactory deficits, we removed APOE from the model and re-ran the interaction analysis. This did not have an impact on the interaction between PM and SOIT score. Using the mean value of the SOIT-score (m = 6.68) as cut off, the sample was divided into two groups: high performers (n = 945) and low performers (n = 760). We repeated the analysis for high- and low performers separately. In these analyses we adjusted for all covariates in the fully adjusted model with the exception of SOIT-score. We found a significant association between PM2.5 and dementia incidence for low performers (HR = 1.32, 95% CI:1.02 –1.71), but not for high performers (HR = 1.05, 95% CI:0.78 –1.43). When stratified by dementia subtype, we found a significant association for low performers between PM2.5 and VaD (HR = 1.60, 95% CI:1.09–2.36), but not for high performers (HR = 0.96, 95% CI:057–1.60). No significant associations between PM2.5 and AD were found in either group (for low performers: HR = 1.26, 95% CI:0.90–1.77 for high performers: HR = 1.24, 95% CI:0.85–1.81).
A significant interaction effect between PM2.5 and APOE ɛ4 status was also found (HR = 1.54, 95% CI: 1.02–2.32; p = 0.038). Thereafter, the sample was divided with regards to APOE ɛ4 status, and we repeated the analysis for carriers (n = 482) and non-carriers (n = 1364) separately. In these analyses we adjusted for all covariates in the fully adjusted model with the exception of APOE. We found a significant association between PM2.5 and dementia incidence for APOE ɛ4 carriers (HR = 1.61, 95% CI: 1.15–2.24), but not for non-carriers (HR = 1.07, 95% CI: 0.83–1.38). When stratified by dementia subtype we found a significant association for APOE ɛ4 carriers only, between PM2.5 and AD (HR = 1.65, 95% CI: 1.12–2.43; for non-carriers: HR = 1.06, 95% CI 0.75–1.50), and between PM2.5 and VaD (HR = 2.28, 95% CI: 1.16–4.50; for non-carriers: HR = 0.58, 95% CI 0.77–1.61).
DISCUSSION
The aim of this study was to investigate the associations between PM2.5 exposure and dementia, in an area with low levels of PM2.5 exposure. Furthermore, we wanted to increase the understanding of the role of olfaction and the APOE ɛ4 allele in the associations between air pollution and dementia.
When running separate analyses for APOE ɛ4 carriers and non-carriers, the results showed that the association remained only for APOE ɛ4 carriers. Furthermore, when the analysis was separated into high- versus low performers on odor identification ability, an association between PM2.5 and dementia was found only among the low performing group. Thus, both APOE ɛ4 carriers and those with low odor identification ability may be particularly vulnerable with regards to the associations between PM2.5 exposure and incident dementia. When stratified by dementia subtype, the associations between PM2.5 and dementia in the fully adjusted model were no longer significant. However, the estimates seem very similar for both subtypes, and it is likely the smaller sample size is what causes the p-values to increase.
Contrasting previous research, we did not find significant associations between dementia incidence and history of CVDRF. The narrow time-period and strict criteria of hospitalized/diagnosed CVDRF (self-report on whether the participant, within a five-year period prior to baseline (T3), had seen a doctor or had been hospitalized for any/some of the following: stroke, heart disease, diabetes, high blood pressure) only addresses a part of existing cardiovascular risk factors. Also, by study design, dementia cases with an onset predating T3 were excluded, resulting in a study population with an overall lower dementia and cardiovascular burden, limiting the possibility of finding strong associations. It should however be emphasized that this study did not primarily aim to investigate CVDRF in relation to dementia.
Whereas most studies investigating associations between air pollution and brain health has taken place in heavily polluted urban areas such as Mexico City [11–13] or New York City [25], this current study was conducted in a small town in northern Sweden, where PM2.5 levels are low. Participants’ average PM2.5 exposure at baseline were 6.77μg/m3, which is only 1.77μg/m3 above the WHO definition of clean air [44]. But despite the low exposure, we still found associations between PM2.5 exposure and dementia. We cannot rule out that other unknown, unmeasured, pollutants are causing the observed associations. However, it is becoming more and more clear that health effects from PM2.5 are present at even lower levels than what has been previously known, as illustrated by current WHO Air quality guidelines [41].
Our findings are in line with previous studies that, using the Betula cohort, found associations between nitrogenous oxides (NOx) and increased risk of dementia [42], and later with PM2.5 [43]. In the new air quality guidelines, presented by WHO in September 2021 [44], the air quality guidelines for the annual mean of PM2.5 was lowered from 10μg/m3 to 5μg/m3. In the study area, the yearly annual average of PM2.5 was 6.7, which is thus slightly above the new WHO guidelines, but very low compared to most international settings, and compared the current legally binding air quality standard based on EU recommendations of 25μg/m3 [45].
We observed a HR of 1.23 per 1μg/m3 increase in annual mean PM2.5, which may seem like a very high point estimate compared with most other studies. In a recent meta-analysis, the risk of dementia increased by 3% per 1μg/m3 increase in PM2.5 [46]. The modelled levels of PM2.5 in our study, however, describe contrasts in locally emitted PM2.5, whereas PM from long distance transport is a constant. Interestingly, recent studies have indicated that near source PM may be more hazardous for health than PM from long distance transport, or that effect estimates from studies with high spatial resolution tend to be higher than in studies where the spatial resolution is lower [41]. The local contribution to PM2.5 in this setting is very low, with a narrow distribution in our study sample. However, near-source estimates of air pollution, tend to yield stronger effect estimates compared to regional levels. For example, a Swedish study [41] showed that associations between PM2.5 exposure and mortality can be seen even at small increases of 1μg/m3 or less. We therefore believe that it is relevant to study local contrasts in air pollution in low-exposure areas. It should furthermore be noted that air pollution is a complex mix of particles and gases, and that it is unclear what aspect of air pollution drives the association between annual mean PM2.5 and dementia observed in the present study. Nanoparticles are for example only very crudely captured by annual mean PM2.5 and would be of interest to investigate in further studies on air pollution as a risk factor for neurodegenerative disorders [47].
We know from previous research that declining olfactory functions are early markers of dementia [7, 8], and that air pollution is associated with AD related pathology in the olfactory bulb long before dementia onset [21]. Therefore, it is not surprising that the association between PM2.5 and dementia was stronger for those with lower than average odor identification ability. Some alternative explanations also need mentioning. Previous research has shown that exposure to pollution is associated with olfactory deficits [15]. Thus, one possible explanation to our results is that pollution independently is associated with risk of dementia, and declining olfactory function. However, a previous study using the Betula cohort [17] concluded that air pollution had no detrimental effects on olfactory functions.
Exposure to air pollution has been suggested to be associated with impairments of many cognitive functions [1], and odor identification is partially dependent on both executive functions and semantic memory [48]. Thus, we hypothesized our results may to some extent reflect low cognitive performance in for example semantic memory. However, adjusting our model for SRB, which can be seen as a measure of semantic memory, did not change the associations between air pollution and dementia. In addition, a previous study using the Betula cohort, showed that APOE ɛ4 is associated with poor odor identification [20]. Therefore, we thought it likely that these findings, at least partially, could be confounded by the APOE gene. However, the interaction between PM and odor identification ability remained the same regardless if we adjusted the model for APOE status. These results seem to indicate that APOE did not confound these results.
With regards to APOE, we only found significant associations between pollution and dementia in ɛ4 carriers. These results are in line with a number of previous studies [23–25]. In areas with relatively high levels of pollution, exposure to pollutants is likely to increase the risk for everyone, though the risk will increase more for APOE ɛ4 carriers. The results from this study indicate that in a moderately polluted area, APOE ɛ4 carriers may constitute a sensitive subgroup who may experience an increased risk of dementia at levels of exposure where non-carriers will not. When stratified by sub-diagnosis, the associations persisted for both AD and VaD. APOE ɛ4 is a known risk factor for AD, but some research also suggests that the allele increases the risk of VaD [19], which could be explained by recent findings suggesting that APOE ɛ4 is associated with vascular degradation in the brain [49].
Previous studies using the Beula cohort have found associations between NOx exposure and dementia incidence [42], but with no clear moderating effect of APOE ɛ4 [27]. There are a few differences between the previous studies and this current one. The previous studies used a land use regression (LUR) model to estimate NOx exposure from traffic related sources, whereas this current study used a dispersion model to estimate PM2.5 exposure from all sources. Any or all of the following could help explain the differing results. Firstly: both PM2.5 and NOx have been associated with dementia incidence in epidemiological studies. However, it could be argued that PM2.5 provides more relevant measures given the ample evidence on a cellular level [4, 14] of detrimental effects of PM on the brain. Secondly: the previous studies only considered traffic related pollution, whereas this current study includes PM2.5 estimates from all sources including domestic wood burning. Thirdly: the dispersion model used in this study may provide more accurate estimates than the previously used LUR model. The two methods differ in that the LUR model is based on data from measuring campaigns that were combined with geographic information systems to provide estimates for areas in-between stations, whereas a dispersion model is a mathematical model based on emission data and meteorological conditions [50]. A study comparing the two methods of modelling [51] found that a dispersion model, compared to a LUR model, provided stronger associations between PM2.5 and mortality of various causes. However, a 2014 review [52] concluded that both models are suitable for epidemiological studies. Interestingly, our results are also in contrast with those of a recent large study using the UK Biobank [26], which found no evidence of moderating effect of APOE in the associations between air pollution and dementia. We can only speculate, but the discrepancies could possibly be explained by differences in air pollution concentration models (land use regression versus dispersion models); the assessment of dementia (algorithmically identified versus validated diagnoses in the Betula study); or differences in the length of the follow-up period (mean follow up time of 7 years versus 12 years), between the two studies.
A major strength of this study is the low exposure levels in our study area, allowing us to investigate associations between air pollution and dementia in an area with relatively clean air, compared to larger urban areas. Another strength of this study is the data from the Betula Project, which provided longitudinal data from a large population-based sample. The data on dementia diagnosis is of particular importance. A review from 2021 [2] found that an overreliance on administrative data or medical records can lead to underdiagnosis of dementia, and biased measures of associations between various factors and dementia incidence. Furthermore, the data on dementia incidence lean on a diagnostic protocol that consider both the richness of long-term clinical data in medical records as well as outcomes of the health- and cognitive assessments carried out within the Betula study framework. Thus, the succession of symptoms can be followed individually, and the cognitive level and trajectories of each participant is included in the diagnostic decision. In addition, continuous follow up of medical records allowed for exclusion of participants who developed dementia shortly after baseline assessment and thus may have already started experiencing cognitive impairments. However, a systematic integration of modern neuroradiological diagnostics and cerebrospinal fluid biomarkers would have further supported the accuracy of the clinical diagnosis (as discussed in [29]).
The exposure estimates are another strength of this study. Many studies within the field have used exposure estimates based on, e.g., neighborhood averages, or distance to the nearest road. Our exposure estimates come from a wind model and a Gaussian air quality dispersion model, allowing for a high-resolution grid size of 50 m x 50 m in urban areas. Having estimates of PM2.5 exposure specifically can also be considered a strength, as many studies within this field have used other exposures (e.g., NOx) as a proxy when PM2.5 estimates have not been available. It is important to note that the density of PM2.5 is largely driven by larger particles in that fraction. In future studies in this setting, it would be of interest to study different size fractions within PM2.5, such as ultra-fine particles (i.e., particles with a diameter < 0.1μm), and their associations with dementia, which our exposure data currently does not allow us to do.
A possible limitation of this study, as in any study investigating the effects of air pollution, is the possibility of exposure misclassification. Though it is important to note that exposure assessment in the present study is state-of-the-art within the field, the models only provided estimates of outdoors PM2.5 exposure at participants residential address at baseline. This means we have no information regarding the participants’ movements throughout the day, time spent indoors, exposure at the workplace, etc. Thus, exposure misclassification cannot be ruled out as a possible source of error. If this is the case, we can only speculate on the strength and direction of this bias. Yet another possibility is that it may be harder for people with olfactory dysfunctions to avoid harmful levels of air pollution exposure.
That we only use exposure estimates at baseline is a limitation in our study, and we cannot draw any conclusions as to whether a specific exposure window is of more importance. Though beyond the scope of this study, the question of when, during the lifespan, we are most susceptible to the detrimental effects of air pollution is an important one. A UK study [53] used statistical models to estimate historical exposure levels going back to the 1930 s, in doing so showing associations between pollution exposure in utero and slower increase of IQ from childhood and throughout adulthood. Meanwhile, a Swedish study [54] concluded that pollution could have an impact on dementia risk also in the short-term, as their findings showed “an increased association between exposure to higher levels of air pollution and dementia, with stronger association for the last 5 years of exposure” [49 p.8].
The possibility of residual confounding also needs to be mentioned. Low socioeconomic status (SES) is a well-known risk factor for many ailments, including dementia. As the exposure is assessed at residential address, it may also be an indicator of SES. We control our analysis for education, which is often used as a marker for SES, albeit a crude one. The associations between SES and air pollution are not always very clear [55]. In many parts of the world, low SES also correlates with high exposure to pollutants. However, in Sweden these correlations may not follow the same pattern, as people with high SES are more likely to reside in city centers with relatively high air pollution exposure. Thus, a more accurate measure of SES in a Swedish setting might further strengthen the associations between air pollution and dementia. Another possible limitation is the lack of data on respiratory diseases, such as COPD, which can be caused by air pollution exposure and both increase the risk for dementia [56], and olfactory deficits. Attrition is a limitation inherent in any longitudinal study, especially those concerning the elderly. Participants who continue in study over time may believe to have better health than those who do not [57]. This study has a long follow-up time and is therefore affected by attrition which may limit the generalizability of the results.
Conclusion
Our results show that long term PM2.5 exposure is associated with an increased risk of dementia even in a low exposure setting. The associations between PM2.5 exposure and dementia seem stronger in APOE ɛ4 carriers, and for persons with low odor identification ability. These results are in line with studies conducted in highly polluted areas. Further research is needed to get a better understanding of the link between air pollution, the APOE gene, and incident dementia.
ACKNOWLEDGMENTS
The authors have no acknowledgments to report.
FUNDING
This work was supported by the Swedish Council for Sustainable Development (FORMAS), dnr 942-2015-1099; and the EU’s Horizon 2020 Call: H2020-MG-2018-2019-2020 TUBE: Transport derived Ultrafines and the Brain Effects, Project Number: 814978-2
CONFLICT OF INTEREST
The authors have no conflict of interest to report.
DATA AVAILABLILTY
Data from the Betula project cannot be made publicly available due to ethical and legal restrictions. However, access to these original data is available upon request from the corresponding author (J.A.) and after approval by the Steering Group of the Betula project (https://www.umu.se/en/betula). Access to data by qualified investigators is subject to scientific and ethical review and must comply with the European Union General Data Protection Regulations (GDPR)/all relevant guidelines. The completion of a data transfer agreement (DTA) signed by an institutional official will be required.
REFERENCES
[1] | Clifford A , Lang L , Chen R , Anstey KJ , Seaton A ((2016) ) Exposure to air pollution and cognitive functioning across the life course–A systematic literature review. Environ Res 147: , 383–398. |
[2] | Weuve J , Bennett EE , Ranker L , Gianattasio KZ , Pedde M , Adar SD , Yanosky JD , Power MC ((2021) ) Exposure to air pollution in relation to risk of dementia and related outcomes: An updated systematic review of the epidemiological literature. Environ Health Perspect 129: , 96001. |
[3] | Livingston G , Huntley J , Sommerlad A , Ames D , Ballard C , Banerjee S , Brayne C , Burns A , Cohen-Mansfield J , Cooper C , Costafreda SG , Dias A , Fox N , Gitlin LN , Howard R , Kales HC , Kivimaki M , Larson EB , Ogunniyi A , Orgeta V , Ritchie K , Rockwood K , Sampson EL , Samus Q , Schneider LS , Selbæk G , Teri L , Mukadam N ((2020) ) Dementiaprevention, intervention, and care: 2020 report of the LancetCommission. Lancet 396: , 413–446. |
[4] | Meraz-Rios MA , Toral-Rios D , Franco-Bocanegra D , Villeda-Hernandez J , Campos-Pena V ((2013) ) Inflammatory process in Alzheimer’s disease. Front Integr Neurosci 7: , 59. |
[5] | Heppner FL , Ransohoff RM , Burkhard B ((2015) ) Immune attack: The roleof inflammation in Alzheimer disease. Nat Rev Neurosci 16: , 358–372. |
[6] | Barresi M , Ciurleo R , Giacoppo S , Foti Cuzzola V , Celi D , Bramanti P , Marino S ((2012) ) Evaluation of olfactory dysfunction in neurodegenerative diseases. J Neurol Sci 323: , 16–24. |
[7] | Murphy C ((2019) ) Olfactory and other sensory impairments in Alzheimer disease. Nat Rev Neurol 15: , 11–24. |
[8] | Stanciu I , Larsson M , Nordin S , Adolfsson R , Nilsson LG , Olofsson JK ((2014) ) Olfactory impairment and subjective olfactory complaintsindependently predict conversion to dementia: A longitudinal,population-based study. J Int Neuropsychol Soc 20: , 209–217. |
[9] | Adams D R , Kern DW , Wroblewski KE , McClintock MK , Dale W , Pinto J M ((2018) ) Olfactory dysfunction predicts subsequent dementia in olderU.S. adults. J Am Geriatr Soc 66: , 140–144. |
[10] | Oberdörster G , Sharp Z , Atudorei V , Elder A , Gelein R , Kreyling W , Cox C ((2004) ) Translocation of inhaled ultrafine particles to thebrain. Inhal Toxicol 16: , 437–445. |
[11] | Calderon-Garcidueñas L , Azzarelli B , Acuna H , Garcia R , Gambling TM , Osnaya N , Monroy S , del Rosario Tizapantzi M , Carson JL , Villareal-Calderon A , Rewcastle B ((2002) ) Air pollution and brain damage. Toxicol Pathol 30: , 373–389. |
[12] | Calderon-Garciduenas L , Reed W , Maronpot RR , Henriquez-Roldan C , Delgado-Chavez R , Calderon-Garciduenas A , Dragustinovis I , Franco-Lira M , Aragon-Flores M , Solt AC , Altenburg M , Torres-Jardon R , Swenberg JA ((2004) ) Brain inflammation and Alzheimer’s-like pathology in individuals exposed to severe air pollution. Toxicol Pathol 32: , 650–658. |
[13] | Calderon-Garciduenas L , Mora-Tiscareno A , Ontiveros E , Gomez-Garza G , Barragan-Mejia G , Broadway J , Chapman S , Valencia-Salazar G , Jewells V , Maronpot RR , Henriquez-Roldan C , Perez-Guille B , Torres-Jardon R , Herrit L , Brooks D , Osnaya-Brizuela N , Monroy ME , Gonzalez-Maciel A , Reynoso-Robles R , Villarreal-Calderon R , Solt AC , Engle RW ((2008) ) Air pollution, cognitive deficits and brain abnormalities: A pilot study with children and dogs. Brain Cogn 68: , 117–127. |
[14] | Heusinkveld HJ , Wahle T , Campbell A , Westerink RHS , Tran L , Johnston H , Stone V , Cassee FR , Schins RPF ((2016) ) Neurodegenerative and neurological disorders by small inhaled particles. Neurotoxicology 56: , 94–106. |
[15] | Ajmani GS , Suh HH , Pinto JM ((2016) ) Effects of ambient air pollution exposure on olfaction: A review. Environ Health Perspect 124: , 1683–1693. |
[16] | Ekström IA , Rizzuto D , Grande G , Bellander T , Laukka EJ ((2022) ) Environmental air pollution and olfactory decline in aging. Environ Health Perspect 130: , 27005. |
[17] | Andersson J , Oudin A , Nordin S , Forsberg B , Nordin M ((2022) ) PM2.5exposure and olfactory functions. Int J Environ Health Res 32: , 2484–2495. |
[18] | Farrer LA , Cupples LA , Haines JL , Hyman B , Kukull WA , Mayeux R , Myers RH , Pericak-Vance MA , Risch N , van Dujin CM ((1997) ) Effects of age, sex, and ethnicity on the association between apolipoprotein E genotype and Alzheimer disease. A meta-analysis. J Am Med Assoc 278: , 1349–1356. |
[19] | Rohn TT ((2014) ) Is apolipoprotein E4 an important risk factor for vascular dementia? Int J Clin Exp Pathol 7: , 3504–3511. |
[20] | Olofsson JK , Nordin S , Wiens S , Hedner M , Nilsson LG , Larsson M ((2010) ) Odor identification impairment in carriers of ApoE-varepsilon4 is independent of clinical dementia. Neurobiol Aging 31: , 567–577. |
[21] | Calderon-Garciduenas L , Franco-Lira M , Henriquez-Roldan C , Osnaya N , Gonzalez-Maciel A , Reynoso-Robles R , Villarreal-Calderon R , Herritt L , Brooks D , Keefe S , Palacios-Moreno J , Villarreal-Calderon R , Torres-Jardon R , Medina-Cortina H , Delgado-Chavez R , Aiello-Mora M , Maronpot RR , Doty RL ((2010) ) Urban air pollution: Influences on olfactory function and pathology in exposed children and young adults. Exp Toxicol Pathol 62: , 91–102. |
[22] | Calderon-Garciduenas L , Gonzalez-Maciel A , Reynoso-Robles R , Kulesza RJ , Mukherjee PS , Torres-Jardon R , Ronkko T , Doty RL ((2018) ) Alzheimer’s disease and alpha-synuclein pathology in the olfactorybulbs of infants, children, teens and adults < /=40 years inMetropolitan Mexico City. APOE4 carriers at higher risk of suicideaccelerate their olfactory bulb pathology. Environ Res 166: , 348–362. |
[23] | Schikowski T , Vossoughi M , Vierkotter A , Schulte T , Teichert T , Sugiri D , Fehsel K , Tzivian L , Bae IS , Ranft U , Hoffmann B , Probst-Hensch N , Herder C , Kramer U , Luckhaus C ((2015) ) Association of air pollution with cognitive functions and its modification by APOE gene variants in elderly women. Environ Res 142: , 10–16. |
[24] | Cacciottolo M , Wang X , Driscoll I , Woodward N , Saffari A , Reyes J , Serre ML , Vizuete W , Sioutas C , Morgan TE , Gatz M , Chui HC , Shumaker SA , Resnick SM , Espeland MA , Finch CE , Chen JC ((2017) ) Particulate air pollutants, APOE alleles and their contributions to cognitive impairment in older women and to amyloidogenesis in experimental models. Transl Psychiatry 7: , e1022. |
[25] | Kulick ER , Elkind MSV , Boehme AK , Joyce NR , Schupf N , Kaufman JD , Mayeux R , Manly JJ , Wellenius GA ((2020) ) Long-term exposure to ambient air pollution, APOE-epsilon4 status, and cognitive decline in a cohort of older adults in northern Manhattan. Environ Int 136: , 105440. |
[26] | Parra KL , Alexander GE , Raichlen DA , Klimentidis YC , Furlong MA ((2022) ) Exposure to air pollution and risk of incident dementia in the UK Biobank. Environ Res 209: , 112895. |
[27] | Oudin A , Andersson J , Sundstrom A , Nordin Adolfsson A , Oudin Astrom D , Adolfsson R , Forsberg B , Nordin M ((2019) ) Traffic-related airpollution as a risk factor for dementia: No clear modifying effectsof APOEɛ4 in the Betula Cohort. J Alzheimers Dis 71: , 733–740. |
[28] | Nilsson L-G , Bäckman L , Erngrund K , Nyberg L , Adolfsson R , Bucht G , Karlsson S , Widing M , Winblad B ((1997) ) The betula prospective cohort study: Memory, health, and aging. Aging Neuropsychol Cogn 4: , 1–32. |
[29] | Nyberg L , Boraxbekk CJ , Sorman DE , Hansson P , Herlitz A , Kauppi K , Ljungberg JK , Lovheim H , Lundquist A , Adolfsson AN , Oudin A , Pudas S , Ronnlund M , Stiernstedt M , Sundstrom A , Adolfsson R ((2020) ) Biological and environmental predictors of heterogeneity in neurocognitive ageing: Evidence from Betula and other longitudinal studies. Ageing Res Rev 64: , 101184. |
[30] | Segersson D , Eneroth K , Gidhagen L , Johansson C , Omstedt G , Nylen AE , Forsberg B ((2017) ) Health impact of PM10, PM2.5 and black carbon exposure due to different source sectors in Stockholm, Gothenburg and Umea, Sweden. Int J Environ Res Public Health 14: , 742. |
[31] | Berkowicz A ((2000) ) OSPM A parameterised street pollution model. Environ Monit Assess 65: , 323–331. |
[32] | Hausberger S , Rexeis M , Zallinger M , Luz R (2009) Emission Factors from the Model PHEM for the HBEFA Version 3. Graz University of Technology, Institute for Internal Combustion Engines and Thermodynamics. Report Nr. I-20/2009Haus-Em 33/08/679. |
[33] | Denby BR , Sundvor I , Johansson C , Pirjola L , Ketzel M , Norman M , Kupiainen K , Gustafsson M , Blomqvist G , Omstedt G ((2013) ) A coupledroad dust and surface moisture model to predict non-exhaust roadtraffic induced particle emissions (NORTRIP). Part 1: Road dustloading and suspension modelling. Atmos Environ 77: , 283–300. |
[34] | Omstedt G , Bringfelt B , Johansson C ((2005) ) A model for vehicle-induced non-tailpipe emissions of particles along Swedish roads. Atmos Environ 39: , 6088–6097. |
[35] | Jalkanen JP , Johansson L , Kukkonen J , Brink A , Kalli J , Stipa T ((2012) ) Extension of an assessment model of ship traffic exhaust emissions for particulate matter and carbon monoxide. Atmos Chem Phys 12: , 2641–2659. |
[36] | American Psychiatric Association (2000) Diagnostic and statistical manual of mental disorders. Washington, DC. |
[37] | Sundstrom A , Marklund P , Nilsson LG , Cruts M , Adolfsson R , Van Broeckhoven C , Nyberg L ((2004) ) APOE influences on neuropsychological function after mild head injury - Within-person comparisons. Neurology 62: , 1963–1966. |
[38] | Bende M , Nordin S ((1997) ) Perceptual learning in olfaction. Professional wine tasters versus controls. Physiol Behav 62: , 1065–1070. |
[39] | Nordin S , Brämerson A , Liden E , Bende M ((1998) ) The Scandinavian Odor-Identification Test. Development, reliability, validity and normative data. Acta Otolaryngol 118: , 226–234. |
[40] | Larsson M , Nilsson LG , Olofsson JK , Nordin S ((2004) ) Demographic and cognitive predictors of cued odor identification: Evidence from a population-based study. Chem Senses 29: , 547–554. |
[41] | Segersson D , Johansson C , Forsberg B ((2021) ) Near-source risk functions for particulate matter are critical when assessing the health benefits of local abatement strategies. Int J Environ Res Public Health 18: , 6847. |
[42] | Oudin A , Forsberg B , Adolfsson AN , Lind N , Modig L , Nordin M , Nordin S , Adolfsson R , Nilsson LG ((2016) ) Traffic-related air pollution and dementia incidence in Northern Sweden: A longitudinal study. Environ Health Perspect 124: , 306–312. |
[43] | Åström DO , Adolfsson R , Segersson D , Forsberg B , Oudin A ((2021) ) Local contrasts in concentration of ambient particulate airpollution (PM2.5) and incidence of Alzheimer’s disease and dementia:Results from the Betula Cohort in Northern Sweden. J AlzheimersDis 81: , 83–85. |
[44] | World Health Organization (2020) Ambient (outdoor) air pollution. https://www.who.int/news-room/fact-sheets/detail/ambient-(outdoor)-air-quality-and-health, Last updated September 22, 2021, Accessed on March 22, 2022. |
[45] | European Environment Agency (2021) Air quality standards. https://www.eea.europa.eu/themes/air/air-quality-concentrations/air-quality-standards, Last updated December 3, 2021. Accessed on January 12, 2023. |
[46] | Abolhasani E , Hachinski V , Ghazaleh N , Azarpazhooh MR , Mokhber N , Martin J ((2023) ) Air pollution and incidence of dementia: A systematic review and meta-analysis. Neurology 100: , e242–e254. |
[47] | Calderon-Garciduenas L , Gonzalez-Maciel A , Reynoso-Robles R , Silva-Pereyra HG , Torres-Jardon R , Brito-Aguilar R , Ayala A , Stommel EW , Delgado-Chavez R ((2022) ) Environmentally toxic solid nanoparticles in noradrenergic and dopaminergic nuclei and cerebellum of metropolitan Mexico City children and young adults with neural quadruple misfolded protein pathologies and high exposures to nano particulate matter. Toxics 10: , 164. |
[48] | Hedner M , Larsson M , Arnold N , Zucco GM , Hummel T ((2010) ) Cognitive factors in odor detection, odor discrimination, and odor identification tasks. J Clin Exp Neuropsychol 32: , 1062–1067. |
[49] | Montagne A , Nation DA , Sagare AP , Barisano G , Sweeney MD , Chakhoyan A , Pachicano M , Joe E , Nelson AR , D’Orazio LM , Buennagel DP , Harrington MG , Benzinger TLS , Fagan AM , Ringman JM , Schneider LS , Morris JC , Reiman EM , Caselli RJ , Chui HC , Tcw J , Chen Y , Pa J , Conti PS , Law M , Toga AW , Zlokovic BV ((2020) ) APOE4 leads to blood-brain barrier dysfunction predicting cognitive decline. Nature 581: , 71–76. |
[50] | Beelen R , Voogt M , Duyzer J , Zandveld P , Hoek G ((2010) ) Comparison of the performances of land use regression modelling and dispersion modelling in estimating small-scale variations in long-term air pollution concentrations in a Dutch urban area. Atmos Environ 44: , 4614–4621. |
[51] | Klompmaker JO , Janssen N , Andersen ZJ , Atkinson R , Bauwelinck M , Chen J , de Hoogh K , Houthuijs D , Katsouyanni K , Marra M , Oftedal B , Rodopoulou S , Samoli E , Stafoggia M , Strak M , Swart W , Wesseling J , Vienneau D , Brunekreef B , Hoek G ((2021) ) Comparison of associations between mortality and air pollution exposure estimated with a hybrid, a land-use regression and a dispersion model. Environ Int 146: , 106306. |
[52] | de Hoogh K , Korek M , Vienneau D , Keuken M , Kukkonen J , Nieuwenhuijsen MJ , Badaloni C , Beelen R , Bolignano A , Cesaroni G , Pradas MC , Cyrys J , Douros J , Eeftens M , Forastiere F , Forsberg B , Fuks K , Gehring U , Gryparis A , Gulliver J , Hansell AL , Hoffmann B , Johansson C , Jonkers S , Kangas L , Katsouyanni K , Kunzli N , Lanki T , Memmesheimer M , Moussiopoulos N , Modig L , Pershagen G , Probst-Hensch N , Schindler C , Schikowski T , Sugiri D , Teixido O , Tsai MY , Yli-Tuomi T , Brunekreef B , Hoek G , Bellander T ((2014) ) Comparing land use regression and dispersion modelling to assess residential exposure to ambient air pollution for epidemiological studies. Environ Int 73: , 382–392. |
[53] | Russ TC , Cherrie MPC , Dibben C , Tomlinson S , Reis S , Dragosits U , Vieno M , Beck R , Carnell E , Shortt NK , Muniz-Terrera G , Redmond P , Taylod AM , Clemens T , van Tongeren M , Agius RM , Starr JM , Deary IJ , Pearce JR ((2021) ) Life course air pollution exposure and cognitivedecline: Modelled historical air pollution data and the Lothianbirth cohort 1936. J Alzheimers Dis 79: , 1063–1074. |
[54] | Grande G , Ljungman PLS , Eneroth K , Bellander T , Rizzuto D ((2020) ) Association between cardiovascular disease and long-term exposure toair pollution with the risk of dementia. JAMA Neurol 77: , 801–809. |
[55] | Stroh E , Oudin A , Gustafsson S , Pilesjo P , Harrie L , Stromberg U , Jakobsson K ((2005) ) Are associations between socio-economic characteristics and exposure to air pollution a question of study area size? An example from Scania, Sweden. Int J Health Geogr 4: , 30. |
[56] | Dodd JW ((2015) ) Lung disease as a determinant of cognitive decline and dementia. Alzheimers Res Ther 7: , 32. |
[57] | Jacobsen E , Ran X , Liu A , Chung-Chou HC , Ganguli M ((2021) ) Predictors of attrition in a longitudinal population-based study of aging. Int Psychogeriatr 33: , 767–778. |