Clinical and Imaging Determinants of Neurocognitive Disorders in Post-Acute COVID-19 Patients with Cognitive Complaints
Abstract
Background:
Neurocognitive disorders (NCDs) are a part of the post-acute coronavirus disease (COVID-19) syndrome. No study has specifically evaluated NCDs in post-acute COVID-19 patients with cognitive complaints or their MRI determinants.
Objective:
To characterize NCDs in post-acute COVID-19 patients with cognitive complaints. The secondary objectives were to assess their clinical and MRI determinants.
Methods:
We included 46 patients with a post-acute COVID-19 cognitive complaint referred to the Amiens University Hospital Memory Center. They underwent a neuropsychological assessment and 36 had cerebral MRI. The G3 overall summary score was the sum of the mean z scores for the executive function, language, and action speed domains. Neuropsychological profiles were compared in a general linear model. Clinical determinants were analyzed by stepwise linear regression. White matter hyperintensities (WMH) masks were analyzed using parcel-based WMH symptom mapping to identify the locations of WMHs associated with cognitive performance.
Results:
Repeated ANOVA showed a group effect (p = 0.0001) due to overall lower performance for patients and a domain effect (p = 0.0001) due to a lower (p = 0.007) action speed score. The G3 overall summary score was significantly associated with solely the requirement for oxygen (R2 = 0.319, p = 0.031). WHMs were associated with the G3 overall summary score in the following structures, all right-sided (p < 0.01): superior frontal region, postcentral region, cingulum, cortico-spinal tract, inferior longitudinal fasciculus, internal capsule, and posterior segment of the arcuate fasciculus.
Conclusion:
Post-acute COVID-19 patients with cognitive complaints had NCD, with prominent action slowing, significantly associated with the acute phase oxygen requirement and a right-sided WMH structure pattern.
INTRODUCTION
The acute neurological complications of coronavirus disease (COVID-19) caused by severe acute respiratory syndrome coronavirus 2 (SARS-CoV-2) have been largely described: encephalopathy, encephalitis, acute disseminated encephalomyelitis and myelitis, cerebrovascular complications, acute hemorrhagic necrotizing encephalopathy, seizures, and peripheral nervous system diseases [1–6]. The prevalence has been estimated to be 13.5%, with age, sex (male), diabetes, hypertension, intubation, and higher sequential organ failure assessment scores associated with neurological complications. Neurological disorders have also been shown to be a risk factor of a bad outcome [6]. Multiple mechanisms underlie such neurological manifestations [4], with inflammation considered to be prominent, supported by neuropathological and cerebrospinal fluid (CSF) findings [1, 4, 7, 8]. The most common brain magnetic resonance imaging (MRI) findings are hypersignals in the temporal lobes and non-confluent white matter multifocal abnormalities, variably associated with contrast enhancement and/or hemorrhagic lesions [9, 10].
Apart from the acute phase, patient follow-up has led to the identification of post-acute COVID-19 syndrome, in which neurological disorders are frequent, particularly neurocognitive disorders (NCDs) [11]. NCDs are defined by evidence of significant cognitive decline from a previous level of performance in one or more cognitive domains [12]. Post-acute COVID-19 syndrome is defined by persistent symptoms and/or delayed long-term complications beyond four weeks from the onset of symptoms [11, 13] and concerns many other organ systems: pulmonary, hematological, cardiovascular, renal, endocrine, hapatogastrointestinal, and dermatological [11]. The most common neurological symptom is cognitive complaints [14]. Post-COVID-19 NCD was assessed with a neuropsychological battery within a mean time interval of less than four weeks from the onset of symptoms [15]. The authors reported prominent impairment of verbal memory, executive function, action speed, language, and visuoconstructive abilities in 35 COVID-19 patients [15]. Low cognitive performance was associated with headache and clinical hypoxia during the acute phase. The few studies to evaluate post-acute COVID-19 NCD with a neuropsychological battery have reported prominent impairment of verbal memory, executive function, and action speed in series of 15 to 50 patients [16–20]. Verbal memory impairment has been shown to be associated with acute respiratory distress syndrome [16] and cognitive impairment with the severity of respiratory symptoms [19]. No study has yet specifically evaluated the relationship between NCD in post-acute COVID-19 patients and cognitive complaints. Such cognitive complaints refer to a self-perceived decline in an individual’s memory and/or other cognitive abilities over time.
A single study [18] reported an association between the Montreal Cognitive Assessment and frontoparietal hypometabolism. MRI corelates of post-acute COVID-19 NCD are still completely unexplored. Associating clinical and imaging determinants of NCD in post-acute COVID-19 patients with cognitive complaints would contribute to a better understanding of the mechanism of the disease and help in deciding the most appropriate management and therapeutics.
The main objective of our study was to characterize the NCD pattern (with a neuropsychological battery) in post-acute COVID-19 patients referred for cognitive complaints. The secondary objectives were to assess their prevalence and clinical and imaging determinants (automated analysis of white matter hyperintensities (WMHs) on cerebral MRI).
METHODS
Population
We included French-speaking patients with a post-acute COVID-19 cognitive complaint referred (mostly by a pneumologist, a general practitioner, or another specialist) to the memory center of the Amiens University Medical Center (Amiens, France) following a COVID-19 infection diagnosed as certain (positive nasopharyngeal swab for SARS CoV-2 by reverse transcriptase polymerase chain reaction assay and/or positive antibodies against SARS CoV-2) or probable (typical clinical symptoms during the COVID-19 epidemic confirmed by an infectious disease specialist) (one patient). The exclusion criteria were: 1) illiteracy, 2) alcoholism or severe comorbidities (severe cardiac, respiratory, or renal disease), 3) concurrent neurological and/or psychiatric disorders (except for depression or anxiety), and 4) a history of major or minor NCD [12]. All patients underwent a clinical examination and were tested using a standardized neuropsychological battery. Cerebral MRI with 3D fluid-attenuated inversion recovery (FLAIR) sequences was performed for all patients but was available at the time of analysis for 36. Clinical data were collected, including age, gender, the history of acute COVID-19 (oxygen requirement, intubation, intensive care unit, fever, delirium), medical history (cardiovascular risk factors, depressive syndrome), neurological examination, and drugs used during the neuropsychological assessment (anxiolytics, antipsychotics). Ethical approval was obtained from the local institutional review board (CNIL: N° PI2020_843_0059).
Neuropsychological assessment
Patients were assessed using a standardized neuropsychological battery [21] that covered 1) general cognitive efficiency, with the GRECO version of the Mini-Mental Test Examination [22], 2) language, with the Boston Naming Test [23], 3) visuoconstructive abilities, with the Rey-Osterrieth Complex Figure Copy Test [24], 4) episodic memory, with the French adaptation of the Free and Cued Selective Reminding Test [25] and the Doors and People Test [26], 5) action speed, with the Digit Symbol-Coding Test [27], 6) executive function, with the GREFEX versions [28] of verbal fluency (letter and category) tests [29], the Trail Making Test (TMT) [30] and the Stroop test [31], and 7) behavioral executive disorders, with the Behavioral Dysexecutive Syndrome Inventory [32]. Behavioral dysexecutive disorders (such as apathy) were considered only if behavioral changes were not accounted for by psychiatric conditions, such as depression [28]. Depressive symptoms were evaluated using the Montgomery-Åsberg Depression Rating Scale [33]. The State-Trait Anxiety Inventory [34] was used to measure the presence and severity of symptoms of anxiety via self-reporting. Fatigue was assessed using the fatigue severity scale [35]. Activities of daily living were assessed using the modified Instrumental Activities of Daily Living scale [36].
MRI
Image acquisition
MRI (sequences: 3D FLAIR, gadolinium 3D T1, T2-weighted gradient echo, diffusion) was performed using two magnetic resonance scanners: 3.0 T (PhilipsR with head coil) and 1.5 T (General ElectricR, optima MR450W). The acquisition characteristics of the 3D FLAIR for the 3T scanner were repetition time: 8000 ms echo time: 320.3 ms, inversion time: 1650 ms, field of view: 25×25 cm, matrix: 224×224, thickness: 1.5 mm, and one excitation and those for the 1.5 T scanner were repetition time: 7602 ms, echo time: 137 ms, inversion time: 2042 ms, field of view: 22×26 cm, matrix: 192×192, thickness: 1.6 mm, and one excitation. The acquisition characteristics of gadolinium 3D T1 for the 3T scanner were repetition time: 7.9 ms echo time: 5.5 ms, field of view: 25×25 cm, matrix: 250×312, thickness: 1 mm and those for the 1.5 T scanner were repetition time: 8 ms, echo time: 3.2 ms, field of view: 26×23.4 cm, matrix: 312×312, thickness: 1 mm. The acquisition characteristics of T2-weighted gradient echo for the 3T scanner were repetition time: 6150 ms echo time: 13.8 ms, field of view: 24×24 cm, matrix: 320×320, thickness: 5 mm and those for the 1.5 T scanner were repetition time: 750 ms, echo time: 23 ms, field of view: 24×24 cm, matrix: 320×324, thickness: 5 mm. The acquisition characteristics of diffusion (B0 and B1000) for the 3T scanner were repetition time: 2900 ms echo time: 78 ms, field of view: 23×23 cm, matrix: 152×106, thickness: 5 mm and those for the 1.5 T scanner were repetition time: 9444 ms, echo time: 72 ms, field of view: 26×26 cm, matrix: 130×140, thickness: 4 mm.
Visual analysis
Neurologists blinded to the clinical data used a validated method [37] to assess the presence of focal lesions, including infarcts and hemorrhages, of WMHs [38], defined according to recent diagnostic criteria [38–40]. WMH microbleeds (not including hemosiderosis) were rated according to the Standards for Reporting Vascular Changes on Neuroimaging [38].
Segmentation and normalization of WMHs
3D FLAIR images were normalized in the MNI space (MNI152 atlas) using SPM12 (http://www.fil.ion.ucl.ac.uk/spm/software/spm12/). The quality of the brain normalizations was visually validated. WMHs were automatically extracted using the Lesion Prediction Algorithm from the LST toolbox [41, 42] running on SPM12. It has been found to be among the top ranked methods for the analysis of both research and clinical datasets [43]. WMH masks were used to compute their volume using a threshold of 0.5 [41, 42].
Parcel-based WMH Symptom Mapping and multivariate analysis
Lesional determinants were analyzed by assessing the relationship between cognitive performance and WMHs, both in terms of volume and location, according to a previously validated method [44]. The locations of WMHs associated with cognitive performance were identified by analyzing WMH masks using parcel-based WMH Symptom Mapping (PWMHSM) with NiiStatV9 (https://www.nitrc.org/projects/niistat/), running with Matlab R2018b (https://in.mathworks.com/products/matlab.html) and SPM12 (http://www.fil.ion.ucl.ac.uk/spm/). Statistical power was increased by parceling the brain into various regions using the Automatic Anatomical Labelling (AAL) [45] and NatbrainLab (CAT) [46] atlases. These atlases are combined in NiiStat and include 150 grey-matter structures (AAL) and tracts (CAT). The AAL atlas was also used because visual examination showed that certain WMHs were located near grey-matter structures that are not covered by the CAT atlas. Analyses were performed in regions with WMHs observed in at least four patients. The significance threshold was set to a p-value of 0.01, corrected for multiple comparisons using the false discovery rate (FDR).
The bivariate step was followed by multivariate analysis performed using stepwise linear regression analysis [44]. The dependent variable was the global cognitive score and the independent variables submitted to regression the global WMH volume and the volume of WMHs in each region found to be significant in PWMHSM analysis.
Statistics
Test cut-offs
The analysis was based on a validated framework for the interpretation of cognitive data [47] using data from a large group of healthy controls (HCs) (n = 1,003, males: 35.9%, mean age±SD: 62±11.3, mean years of full-time education±SD: 11.4±3.2) [48]) and consisted of four main steps: 1) transformation of component scores, 2) adjustment for significant demographic factors, 3) combination of component scores into five domain scores (action speed, executive functions, episodic memory, and language and visuoconstructive abilities), and 4) combination of domain scores into an overall summary score. Component scores were combined according to the cognitive domain; if several component z scores assessed the same domain, they were averaged to yield a domain score. The executive function score was the mean value of the z scores for category fluency and the error rate in the TMT Part B minus Part A. The memory score was the mean value of the z scores for the third free recall and the delayed free recall in the Free and Cued Selective Reminding Test. The visuoconstructive function score was the z score for the Rey-Osterrieth Complex Figure Copy Test. The language score was the mean value of the z scores for the Boston Naming Test. The action speed score was the mean value for the completion time in the TMT Part B and the Digit Symbol Coding Test. After checking the homogeneity of the score distribution across the domains, the five domain scores were combined in two different ways. This resulted in two overall summary scores: 1) the overall cognitive summary score, corresponding to the average of the five domain scores, and 2) the shortened cognitive summary score, corresponding to the mean of the most frequently impaired domains. Finally, the scores were categorized (i.e., normal or impaired) using the 5th percentile. Missing data were only interpreted as corresponding to an impairment when the neuropsychologist indicated that the patient was unable to perform the task. In other cases, the data were not modified and were considered to be missing.
Analysis of neuropsychological and clinical results
Neuropsychological profiles (z scores) of patients were determined by comparison of their performance with those of HCs using analysis of variance (ANOVA) with repeated measures on the domain score (language, visuoconstructive abilities, memory, executive function, and action speed) and the group (patients with post-acute COVID-19 cognitive complaints versus HCs) as a between-subject factor. A post hoc test was performed using deviation contrast analysis.
As previously shown [49, 50], the optimal overall summary score is formed by combining scores that are the most sensitive to the study condition. The selection of sensitive domain z scores was performed by stepwise logistic regression analysis with the group (patients, controls) as the dependent variable and the following scores submitted as independent variables: each of the five domain scores (language, visuoconstructive abilities, memory, executive function, and action speed), the global 5 cognitive score, corresponding to the mean of the z scores of the five cognitive domains), the global 4 cognitive score, corresponding to the mean of the z scores for the four most impaired domains in the ANOVA analysis, and the global 3 (G3) cognitive score (G3 overall summary score), corresponding to the mean of the z scores for the three most impaired domains in the ANOVA analysis [50]. The prevalence of cognitive impairment was computed after dichotomization using the 5th percentile; the 95% CI for the prevalence of impairment was computed using the equation p±zx√ (p×(1 – p)/n).
Examination of the clinical determinants of post-acute COVID-19 NCD was carried out in two steps. First, we computed Pearson correlation coefficients between the optimal overall summary score (i.e., G3, see below) and clinical indices (oxygen requirement, intubation, intensive care unit, non-invasive ventilation, fever, delirium, medical history of stroke, medical history of depressive syndrome, cardiovascular risk factors, depressive syndrome, anxiety, fatigue, neurological examination [focal deficit, tendon reflex, Babinski sign, cerebellar syndrome], and anxiolytic and anti-depressant use). Second, clinical indices with a correlation of p < 0.2 were subjected to stepwise linear regression. Demographic factors were not included in the regression analysis, as cognitive z-scores were already adjusted for demographic factors.
The threshold for statistical significance was set to p = 0.05. Statistical analyses were performed using SPSS software (version 15, SPSS Inc., Chicago, IL, USA).
RESULTS
Population
The demographic breakdown (Table 1) was characterized by a mean age of 50.9 years, a predominance of females (74%), and a high level of education: 60.9% of patients had a tertiary level of education (more than 11 years of schooling), 30.4% a secondary level (between 9 and 11 years of schooling), and only 8.7% a primary level (less than 9 years of schooling). No patients had observable neurological complications during the acute phase. The fatigue score indicated impairment for 80.4% of patients. Only 13% of patients were intubated for COVID-19. No patient had impaired autonomy. Thirty-seven patients underwent cerebral MRI, of whom 36 had FLAIR sequences (which were used for the MRI analysis). One patient had a stroke sequela (with a medical history before developing COVID-19 symptoms); she had a neuropsychological assessment after her stroke and before becoming ill with COVID-19, which was normal. The time interval from COVID-19 (date of symptoms onset) to neuropsychological assessment was 254 (±90) days, from COVID-19 to MRI 202 (±80) days, and between the neuropsychological assessment and MRI 54 (±61) days.
Table 1
Demographic and MRI characteristics of the post-acute COVID-19 cognitive complaint population
N = 46 | |
Age (y) | 50.9 (±14) |
Gender, male | 11 (23.9%) |
Education level | |
primary | 4 (8.7%) |
secondary | 14 (30.4%) |
tertiary | 28 (60.9%) |
Right-handed | 42 (91.3%) |
Time between COVID-19 and Neuropsychological assessment (days) | 254 (±90) |
Time between COVID-19 and MRI (days) | 202 (±80) |
Time between MRI and Neuropsychological assessment (days) | 54 (±61) |
During COVID-19 | |
Intensive care unit | 11 (23.9%) |
Intubation | 6 (13%) |
Noninvasive ventilation | 8 (17.3%) |
Oxygen requirement | 17 (36.9%) |
Fever | 30 (65.2%) |
Delirium | 5 (10.8%) |
Anosmia/Ageusia | 23 (50%) |
Medical history | |
Depression | 10 (21.7%) |
Stroke | 3 (6.5%) |
Cardiovascular risk factors (at least one) | 25 (54.3%) |
During neurological consultation | |
Anxiolytic use | 9 (19.5%) |
Anti-depressant use | 12 (26.1%) |
Sleeping disorders | 10 (21.7%) |
Motor deficit | 3 (6.5%) |
Sensitive deficit | 3 (6.5%) |
Vivid deep tendon reflexes | 9 (19.6%) |
Abolition of deep tendon reflexes | 6 (13%) |
Cerebellar syndrome | 2 (4.3%) |
Depression | 15 (32.6%) |
Anxiety (moderate or elevated) | 19 (41.3%) |
Fatigue | 37 (80.4%) |
MMSE | 27.9 (±1.5) |
Cerebral MRI | 37 (80.4%) |
3D FLAIR | 36 (78.3%) |
White matter abnormalities | 19 (51.3%) |
Focal lesion | 1 (2.7%) |
Leptomeningeal enhancement | 3 (5.4%) |
Microbleeds | 3 (8.1%) |
MMSE, Mini-Mental State Examination; FLAIR, fluid-attenuated inversion recovery.
Cognitive deficit and profile
Repeated ANOVA showed a group effect (p = 0.0001), due to overall lower performance in patients (patients: mean z score = –0.514±0.089, HCs: mean z score = 0.017±0.021), and a domain effect (p = 0.0001), due to overall lower (p = 0.007) action speed score (mean z score = –0.817±0.142), followed by language (mean z score = –0.685±0.152), executive functions (mean z score = –0.627±0.139) and memory (mean z score = –0.486±0.151) scores (visuoconstructive ability mean z score = 0.044±0.149) according to contrast analysis. Finally, the group×domain interaction was significant (p = 0.001) due to lower performance in the action speed, executive function, and language domains (Fig. 1) in patients.
Fig. 1
Cognitive profile (z-scores) of post-acute COVID-19 cognitive complaint. Blue: z-scores of each cognitive domain for the healthy controls. Red: z-scores of each cognitive domain for the COVOD-19 patients with a cognitive complaint. The group×domain interaction was significant (p = 0.001) due to lower performance in the action speed, executive function, and language domains in patients.
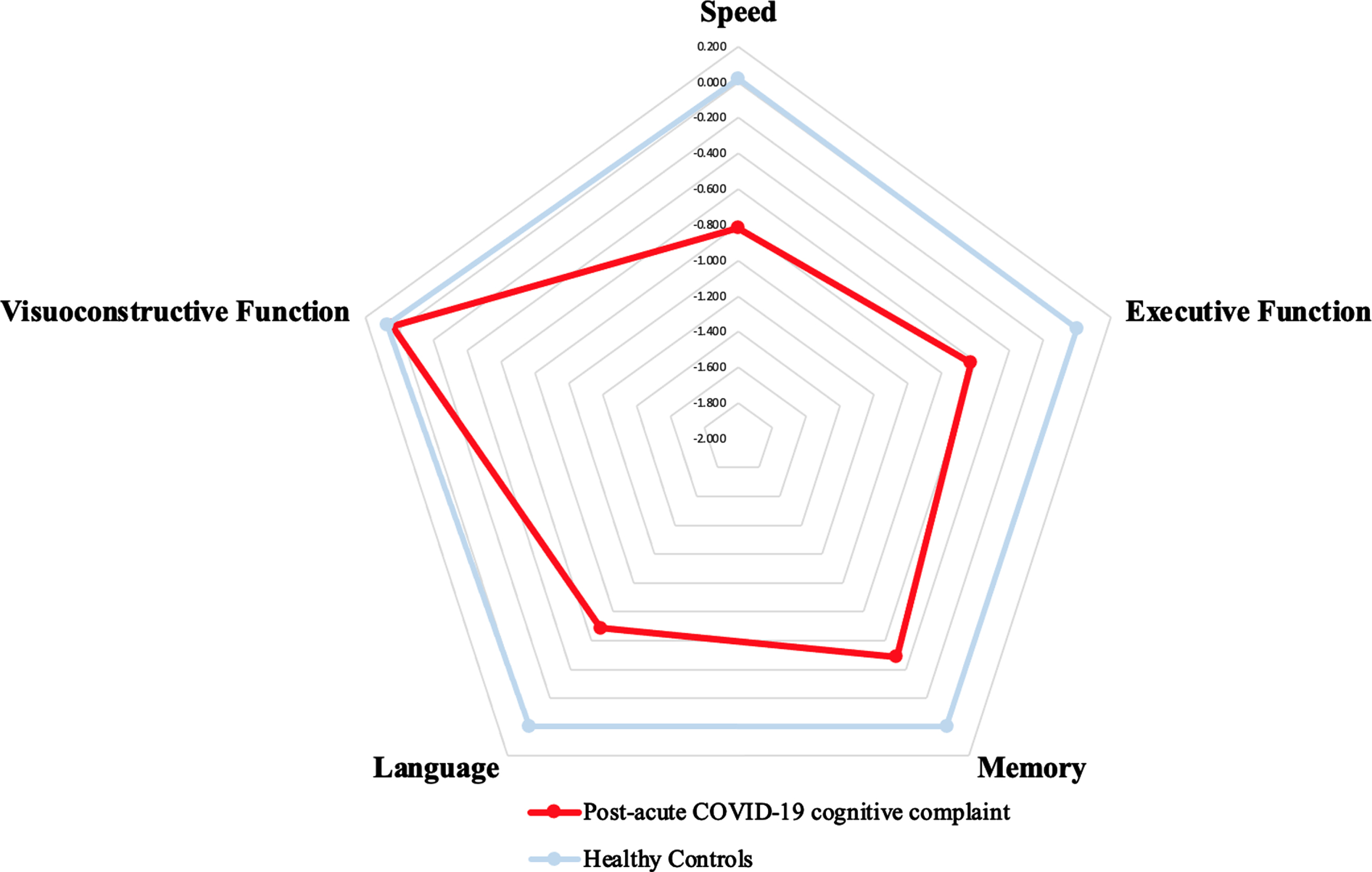
Based on these results, the four-factor overall summary score was computed using the z scores of action speed, executive functions, language, and memory and the three-factor (G3) overall summary score, using the z scores of action speed, executive functions, and language.
Definition of overall summary score and prevalence of CI‘
Stepwise logistic regression selected the G3 overall summary score as the most discriminative cognitive index associated with post-acute COVID-19 cognitive complaints versus HC (odds ratio (OR) = 5.0033, 95% CI [3.185–7.951], p = 0.001). The prevalence of impairment based on the G3 overall summary score was 19.6%, 95% CI [8.1%–31.0%].
Clinical determinants
The results of bivariate analysis are presented in Table 2. In the stepwise linear regression model, the G3 overall summary score was significantly associated with solely the requirement for oxygen (R2 = 0.319, p = 0.031).
Table 2
Pearson correlation of G3 overall summary score and clinical factors
R | p | |
During COVID-19 | ||
Intensive care unit | –0.181 | 0.129 |
Intubation | –0.247 | 0.06 |
Noninvasive ventilation | –0.231 | 0.073 |
Oxygen requirement | –0.251 | 0.057 |
Fever | –0.034 | 0.415 |
Delirium | –0.08 | 0.31 |
Anosmia/Ageusia | 0.001 | 0.994 |
Medical history | ||
Depression | –0.033 | 0.418 |
Stroke | –0.18 | 0.13 |
Cardiovascular risk factors (at least one) | –0.112 | 0.243 |
Anxiolytic use | –0.111 | 0.245 |
Anti-depressant use | –0.098 | 0.272 |
Sleeping disorders | 0.138 | 0.194 |
Motor deficit | 0.117 | 0.233 |
Sensitive deficit | 0.052 | 0.373 |
Vivid deep tendon reflexes | 0 | 0.998 |
Abolition of deep tendon reflexes | 0.202 | 0.178 |
Cerebellar syndrome | –0.162 | 0.282 |
Depression | –0.014 | 0.466 |
Anxiety | –0.15 | 0.174 |
Fatigue | 0.157 | 0.164 |
Imaging determinants
The frequency of WMHs is presented in Fig. 2. In PWMHSM analysis, WHMs were associated with the G3 overall summary score in the following structures, all localized to the right hemisphere: superior frontal region (mean z = –3.74), postcentral region (mean z = –3.71), cingulum (mean z = –3.56), cortico-spinal tract (mean z = –3.57), inferior longitudinal fasciculus (mean z = –3.62), internal capsule (mean z = –3.39), and posterior segment of the arcuate fasciculus (mean z = –3.41) (Fig. 3 and Supplementary Table 1). The data for these six regions were subjected to linear regression analysis.
Fig. 2
Frequency map of white matter hyperintensities in each structure. Color bar: frequency (higher number corresponds to higher frequency) of white matter hyperintensities in each structure of the Automatic Anatomical Labelling (AAL) and NatbrainLab (CAT) atlases. (e.g., tracts in white color correspond to the presence of WMH in 25% of patients). R, right; L, left.
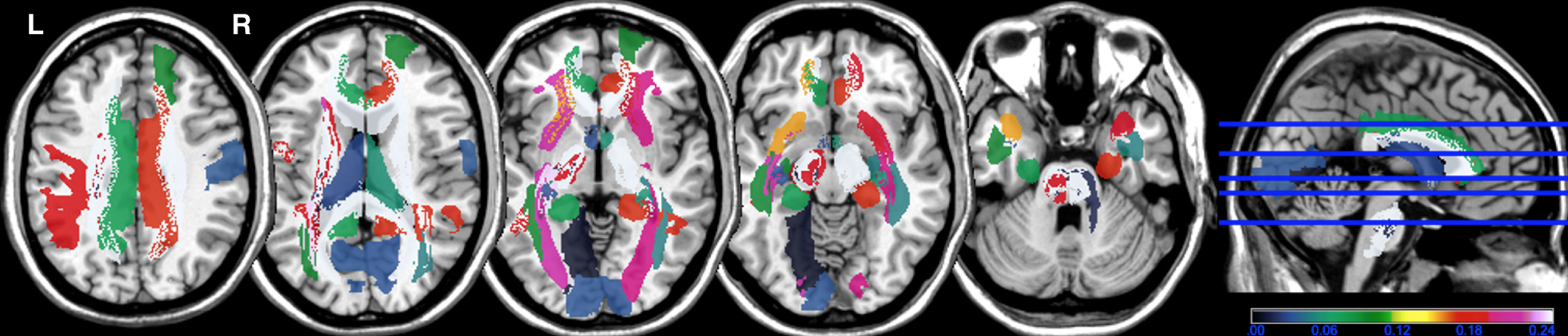
Fig. 3
Parcel-based white matter hyperintensities symptom mapping (PWMHSM) analysis of structures associated with the G3 overall summary score. Color bar: z-score analysis of the strength of the association between white matter hyperintensities and the G3 overall summary score in structures of the Automatic Anatomical Labelling (AAL) and NatbrainLab (CAT) atlases (superior frontal region (mean z = –3.74), postcentral region (mean z = –3.71), cingulum (mean z = –3.56), cortico-spinal tract (mean z = –3.57), inferior longitudinal fasciculus (mean z = –3.62), internal capsule (mean z = –3.39), and posterior segment of the arcuate fasciculus (mean z = –3.41)). R, right; L, left.
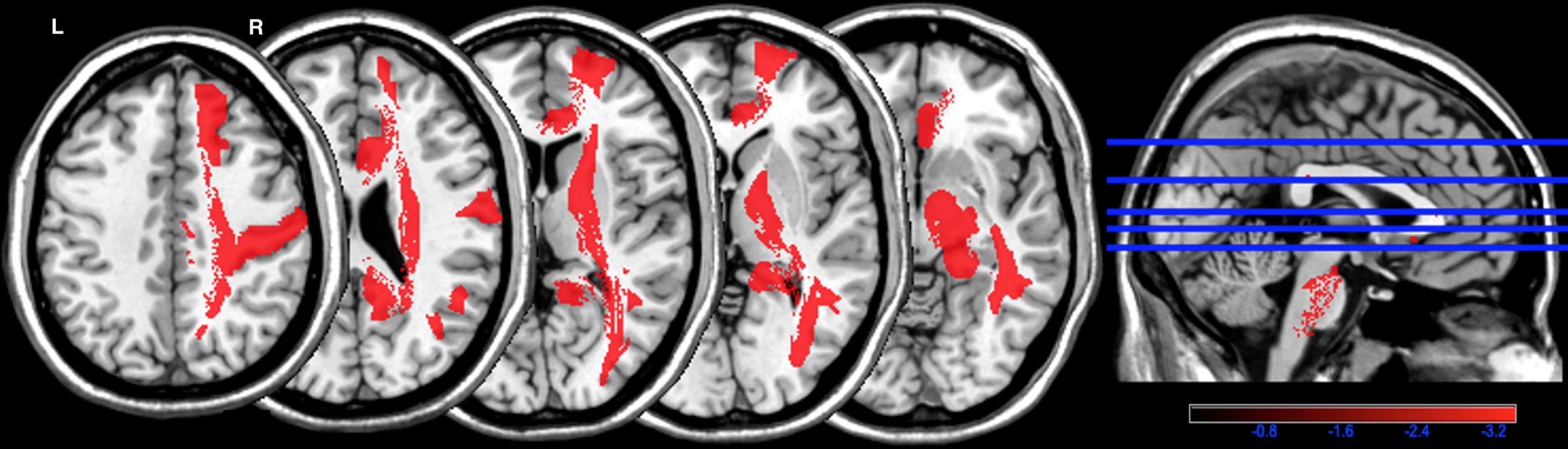
The presence of microbleeds (R = 0.15, p = 0.28), leptomeningeal enhancement (R = 0.12, p = 0.27, and the presence of focal lesions (R = 0.198, p = 0.15) did not correlate with the G3 overall summary score. However, the global volume of WMHs (7.4±20.9 ml) significantly correlated with the G3 overall summary score (R = –0.646; p = 0.0001).
The global WMH volume and the six regions found by PWMHSM were used for stepwise linear regression analysis, which selected WMHs in the right superior frontal region (R2 = 0.44, p = 0.0001).
DISCUSSION
In the present study, we demonstrate the presence of NCD in post-acute COVID-19 patients with cognitive complaints. The cognitive domains most highly affected were action speed, executive function, and language (naming) and the most pertinent cognitive index was the G3 overall summary score (the mean of the z scores for the cognitive domains of action speed, executive function, and language). The cognitive deficit was slight (mean z = –0.514). The prevalence of NCD (expressed by the G3 overall summary score) was 19.6%, (95% CI [8.1%–31.0%])), with the only clinical determinant being the requirement for oxygen. Importantly, we show a pattern of WMHs associated with post-acute COVID-19 NCD with cognitive complaints (expressed by the G3 overall summary score), all right sided and consisting of WMHs in the superior frontal region, postcentral region, right cingulum, cortico-spinal tract, inferior longitudinal fasciculus, internal capsule, and posterior segment of the arcuate fasciculus. Only the right superior frontal region was significantly associated with the G3 overall summary score when considering the global WMH volume.
Our results for the post-acute COVID-19 NCD cognitive profile, showing a predominance of slowing and executive dysfunction, are in accordance with those of already published studies [16–20]. In terms of language, Alemeria et al. [15] showed impairment based on reduced performance on the Boston Naming Test, performed from 10 to 35 days after hospital discharge, for 35 patients. We are the first to describe the cognitive profile and relatively high prevalence of NCD (19.6%) in an already preselected population: post-acute COVID-19 with cognitive complaints. We confirmed the significant prevalence of NCD in post-acute COVID-19 syndrome [11] and the importance of clinical follow-up of COVID-19 patients [13]. A cognitive complaint during follow-up should be seriously considered and the patient referred to a memory center for an exhaustive evaluation and follow-up, in particular if they required oxygen during the acute phase.
Furthermore, we found NCD in our population to be significantly associated with the requirement for oxygen. A similar association between cognitive impairment and the severity of respiratory symptoms has been reported [19]. The mechanism of post-acute COVID-19 NCD was hypothesized to involve multiple factors [51–53]: preexisting comorbidities, the course of hospitalization (delirium), pulmonary damage (hypoxia), vascular damage, and neuronal injury. Depression, anxiety, and fatigue, which are very common post-acute COVID-19 symptoms [11, 14, 54], were not associated with NCD, as in the previous study [15]. The presence of one or more of these symptoms should not be considered to indicate the presence of a cognitive complaint and although these frequent symptoms need to be managed by a specialist, a memory consultation is also necessary. In addition, there was no association with cardiovascular risk factors or delirium during the acute phase. The association we found with the requirement for oxygen does not appear to be related to hypoxic severity, as we found no association with intubation or noninvasive ventilation and systematic cognitive evaluation is not recommended for other types of hypoxic pneumonia. The presence of cognitive complaints is better explained by acute inflammation [55, 56], which may also involve the nervous system during the acute and immediately post-acute phase of the disease [4].
Finely, this study is the first to highlight an association between a pattern of WMHs in NCD (expressed by the G3 overall summary score: the mean of the z scores for the cognitive domains of action speed, executive function, and language) in post-acute COVID-19 patients and cognitive complaints. The pattern was right sided, showing WMHs in the superior frontal region, postcentral region, right cingulum, cortico-spinal tract, inferior longitudinal fasciculus, internal capsule, and posterior segment of the arcuate fasciculus. The global WMH volume is known to be associated with action speed and executive function [57]. In our study, only the right superior frontal region was associated with the G3 overall summary score when we took into account the global WMH volume. The prominent impairment concerned action speed. In a previous study on healthy subjects, Périn et al. [58] localized the region for the phasic alertness of action speed (evaluated by simple reaction time correlates in a brain activation functional MRI study) to the right frontal superior region, right inferior parietal region, and right anterior cingulate region. A study evaluating action speed in patients with focal lesions by the TMT highlighted an association with the right superior frontal region [59]. Additionally the right cingulum is known to control action speed [60]. The right arcuate fasciculus was also shown to control action speed in multiple sclerosis patients [61]. Such action speed has a perceptive and motor component [62], consisting of the post-central region (primary sensorial somatosensory region) [63] and the internal capsule and cortico-spinal tract (motor pathways) [64, 65]. In addition, the right superior frontal region [66] and the right cingulum [67] are also known to control executive function. The neural basis of language is left sided [68, 69]. Our patients were mostly right-handed (91.3%). Nevertheless, the bilateral inferior longitudinal fasciculus sustains the lexico-semantic process [70] and visual recognition in naming [69].
Our study had several limitations, including the small monocentric sample. In addition, we did not compare our data to that from a group of patients with COVID-19 without cognitive complaints. However, the study design included COVID-19 patients with cognitive complaints but who did not all have NCD, which made it possible to search for the source of inter-subject cognitive variability in WMH, and this design was validated. In addition, concerning the neuropsychological data, the HCs did not experience COVID-19-related mental health issues. Furthermore, collecting the neuropsychological data from the 1,009 HCs during the COVID-19 pandemic would have been impossible. Another limitation was the lack of cerebral MRI for a number of patients at the date of the analysis, as one of the most important questions was the specificity of WMHs in COVID-19. WMHs without enhancement have already been described in COVID-19 [10]. In a separate study, they were assumed to be related to demyelination resulting from inflammation and showed no specific pattern of distribution [71]. Our population had no history of evolving neurological disease. The WMHs that we observed may have partially been of vascular origin [41]. However, the cases of NCD were not associated with vascular risk factors and the patients had no history of NCD or cognitive complaints. A previous study reported a pattern of cortical hypometabolism in post-acute COVID-19 that was associated with the Montreal Cognitive Assessment [18]. However, we did not analyze the grey matter that could have contributed to the NCD in our group of patients. Future studies with larger samples combining the analysis of WMHs and grey matter with clinical and MRI follow-up are required. Overall, our clinical and imaging results support a role for inflammation in the cognitive complaints in post-acute COVID-19 NCD in our population.
Our study had several strengths, including standardized neuropsychological assessment and validated methods [47, 48] and the automated PWMHSM analysis of WMHs.
In conclusion, post-acute COVID-19 patients with cognitive complaints showed NCD, with prominent action slowing, significantly associated with the requirement for oxygen during the acute phase and a right-sided pattern of WMHs.
DISCLOSURE STATEMENT
Authors’ disclosures available online (https://www.j-alz.com/manuscript-disclosures/21-5506r2).
SUPPLEMENTARY MATERIAL
[1] The supplementary material is available in the electronic version of this article: https://dx.doi.org/10.3233/JAD-215506.
REFERENCES
[1] | Ellul MA , Benjamin L , Singh B , Lant S , Michael BD , Easton A , Kneen R , Defres S , Sejvar J , Solomon T ((2020) ) Neurological associations of COVID-19. Lancet Neurol 19: , 767–783. |
[2] | Helms J , Kremer S , Merdji H , Clere-Jehl R , Schenck M , Kummerlen C , Collange O , Boulay C , Fafi-Kremer S , Ohana M , Anheim M , Meziani F ((2020) ) Neurologic features in severe SARS-CoV-2 infection. N Engl J Med 382: , 2268–2270. |
[3] | Hernández-Fernández F , Sandoval Valencia H , Barbella-Aponte RA , Collado-Jiménez R , Ayo-Martín Ó , Barrena C , Molina-Nuevo JD , García-García J , Lozano-Setién E , Alcahut-Rodriguez C , Martínez-Martín Á , Sánchez-López A , Segura T ((2020) ) Cerebrovascular disease in patients with COVID-19: Neuroimaging, histological and clinical description. Brain 143: , 3089–3103. |
[4] | Keyhanian K , Umeton RP , Mohit B , Davoudi V , Hajighasemi F , Ghasemi M ((2020) ) SARS-CoV-2 and nervous system: From pathogenesis to clinical manifestation. J Neuroimmunol 350: , 577436. |
[5] | Paterson RW , Brown RL , Benjamin L , Nortley R , Wiethoff S , Bharucha T , Jayaseelan DL , Kumar G , Raftopoulos RE , Zambreanu L , Vivekanandam V , Khoo A , Geraldes R , Chinthapalli K , Boyd E , Tuzlali H , Price G , Christofi G , Morrow J , McNamara P , McLoughlin B , Lim ST , Mehta PR , Levee V , Keddie S , Yong W , Trip SA , Foulkes AJM , Hotton G , Miller TD , Everitt AD , Carswell C , Davies NWS , Yoong M , Attwell D , Sreedharan J , Silber E , Schott JM , Chandratheva A , Perry RJ , Simister R , Checkley A , Longley N , Farmer SF , Carletti F , Houlihan C , Thom M , Lunn MP , Spillane J , Howard R , Vincent A , Werring DJ , Hoskote C , Jäger HR , Manji H , Zandi MS ((2020) ) The emerging spectrum of COVID-19 neurology: Clinical, radiological and laboratory findings. Brain 143: , 3104–3120. |
[6] | Frontera JA , Sabadia S , Lalchan R , Fang T , Flusty B , Millar-Vernetti P , Snyder T , Berger S , Yang D , Granger A , Morgan N , Patel P , Gutman J , Melmed K , Agarwal S , Bokhari M , Andino A , Valdes E , Omari M , Kvernland A , Lillemoe K , Chou SH-Y , McNett M , Helbok R , Mainali S , Fink EL , Robertson C , Schober M , Suarez JI , Ziai W , Menon D , Friedman D , Friedman D , Holmes M , Huang J , Thawani S , Howard J , Abou-Fayssal N , Krieger P , Lewis A , Lord AS , Zhou T , Kahn DE , Czeisler BM , Torres J , Yaghi S , Ishida K , Scher E , de Havenon A , Placantonakis D , Liu M , Wisniewski T , Troxel AB , Balcer L , Galetta S ((2021) ) A prospective study of neurologic disorders in hospitalized patients with COVID-19 in New York City. Neurology 96: , e575–e586. |
[7] | Matschke J , Lütgehetmann M , Hagel C , Sperhake JP , Schröder AS , Edler C , Mushumba H , Fitzek A , Allweiss L , Dandri M , Dottermusch M , Heinemann A , Pfefferle S , Schwabenland M , Sumner Magruder D , Bonn S , Prinz M , Gerloff C , Püschel K , Krasemann S , Aepfelbacher M , Glatzel M ((2020) ) Neuropathology of patients with COVID-19 in Germany: A post-mortem case series. Lancet Neurol 19: , 919–929. |
[8] | Edén A , Kanberg N , Gostner J , Fuchs D , Hagberg L , Andersson L-M , Lindh M , Price RW , Zetterberg H , Gisslén M ((2021) ) CSF biomarkers in patients with COVID-19 and neurologic symptoms: A case series. Neurology 96: , e294–e300. |
[9] | Kremer S , Lersy F , Anheim M , Merdji H , Schenck M , Oesterlé H , Bolognini F , Messie J , Khalil A , Gaudemer A , Carré S , Alleg M , Lecocq C , Schmitt E , Anxionnat R , Zhu F , Jager L , Nesser P , Mba YT , Hmeydia G , Benzakoun J , Oppenheim C , Ferré J-C , Maamar A , Carsin-Nicol B , Comby P-O , Ricolfi F , Thouant P , Boutet C , Fabre X , Forestier G , de Beaurepaire I , Bornet G , Desal H , Boulouis G , Berge J , Kazémi A , Pyatigorskaya N , Lecler A , Saleme S , Edjlali-Goujon M , Kerleroux B , Constans J-M , Zorn P-E , Mathieu M , Baloglu S , Ardellier F-D , Willaume T , Brisset J-C , Caillard S , Collange O , Mertes PM , Schneider F , Fafi-Kremer S , Ohana M , Meziani F , Meyer N , Helms J , Cotton F ((2020) ) Neurologic and neuroimaging findings in patients with COVID-19: A retrospective multicenter study. Neurology 95: , e1868–e1882. |
[10] | Kremer S , Lersy F , de Sèze J , Ferré J-C , Maamar A , Carsin-Nicol B , Collange O , Bonneville F , Adam G , Martin-Blondel G , Rafiq M , Geeraerts T , Delamarre L , Grand S , Krainik A , Caillard S , Constans JM , Metanbou S , Heintz A , Helms J , Schenck M , Lefèbvre N , Boutet C , Fabre X , Forestier G , de Beaurepaire I , Bornet G , Lacalm A , Oesterlé H , Bolognini F , Messié J , Hmeydia G , Benzakoun J , Oppenheim C , Bapst B , Megdiche I , Henry Feugeas M-C , Khalil A , Gaudemer A , Jager L , Nesser P , Talla Mba Y , Hemmert C , Feuerstein P , Sebag N , Carré S , Alleg M , Lecocq C , Schmitt E , Anxionnat R , Zhu F , Comby P-O , Ricolfi F , Thouant P , Desal H , Boulouis G , Berge J , Kazémi A , Pyatigorskaya N , Lecler A , Saleme S , Edjlali-Goujon M , Kerleroux B , Zorn P-E , Matthieu M , Baloglu S , Ardellier F-D , Willaume T , Brisset JC , Boulay C , Mutschler V , Hansmann Y , Mertes P-M , Schneider F , Fafi-Kremer S , Ohana M , Meziani F , David J-S , Meyer N , Anheim M , Cotton F ((2020) ) Brain MRI findings in severe COVID-19: A retrospective observational study. Radiology 297: , E242–E251. |
[11] | Nalbandian A , Sehgal K , Gupta A , Madhavan MV , McGroder C , Stevens JS , Cook JR , Nordvig AS , Shalev D , Sehrawat TS , Ahluwalia N , Bikdeli B , Dietz D , Der-Nigoghossian C , Liyanage-Don N , Rosner GF , Bernstein EJ , Mohan S , Beckley AA , Seres DS , Choueiri TK , Uriel N , Ausiello JC , Accili D , Freedberg DE , Baldwin M , Schwartz A , Brodie D , Garcia CK , Elkind MSV , Connors JM , Bilezikian JP , Landry DW , Wan EY ((2021) ) Post-acute COVID-19 syndrome. Nat Med 27: , 601–615. |
[12] | Association American Psychiatric ((2013) ) Diagnostic and Statistical Manual of Mental Disorders: DSM-5, American Psychiatric Publishing, Washington, DC. |
[13] | Fernández-de-Las-Peñas C , Palacios-Ceña D , Gómez-Mayordomo V , Cuadrado ML , Florencio LL ((2021) ) Defining Post-COVID symptoms (post-acute COVID, long COVID, persistent post-COVID): An integrative classification. Int J Environ Res Public Health 18: , 2621. |
[14] | Al-Ramadan A , Rabab’h O , Shah J , Gharaibeh A ((2021) ) Acute and post-acute neurological complications of COVID-19. Neurol Int 13: , 102–119. |
[15] | Almeria M , Cejudo JC , Sotoca J , Deus J , Krupinski J ((2020) ) Cognitive profile following COVID-19 infection: Clinical predictors leading to neuropsychological impairment. Brain Behav Immun Health 9: , 100163. |
[16] | Ferrucci R , Dini M , Groppo E , Rosci C , Reitano MR , Bai F , Poletti B , Brugnera A , Silani V , D’Arminio Monforte A , Priori A ((2021) ) Long-lasting cognitive abnormalities after COVID-19. Brain Sci 11: , 235. |
[17] | Graham EL , Clark JR , Orban ZS , Lim PH , Szymanski AL , Taylor C , DiBiase RM , Jia DT , Balabanov R , Ho SU , Batra A , Liotta EM , Koralnik IJ ((2021) ) Persistent neurologic symptoms and cognitive dysfunction in non-hospitalized Covid-19 “long haulers.”. Ann Clin Transl Neurol 8: , 1073–1085. |
[18] | Hosp JA , Dressing A , Blazhenets G , Bormann T , Rau A , Schwabenland M , Thurow J , Wagner D , Waller C , Niesen WD , Frings L , Urbach H , Prinz M , Weiller C , Schroeter N , Meyer PT ((2021) ) Cognitive impairment and altered cerebral glucose metabolism in the subacute stage of COVID-19. Brain 144: , 1263–1276. |
[19] | Miskowiak K , Johnsen S , Sattler S , Nielsen S , Kunalan K , Rungby J , Lapperre T , Porsberg C ((2021) ) Cognitive impairments four months after COVID-19 hospital discharge: Pattern, severity and association with illness variables. Eur Neuropsychopharmacol 46: , 39–48. |
[20] | Ortelli P , Ferrazzoli D , Sebastianelli L , Engl M , Romanello R , Nardone R , Bonini I , Koch G , Saltuari L , Quartarone A , Oliviero A , Kofler M , Versace V ((2021) ) Neuropsychological and neurophysiological correlates of fatigue in post-acute patients with neurological manifestations of COVID-19: Insights into a challenging symptom. J Neurol Sci 420: , 117271. |
[21] | Godefroy O , Leclercq C , Roussel M , Moroni C , Quaglino V , Beaunieux H , Tallia H , Nédélec-Ciceri C , Bonnin C , Thomas-Anterion C , Varvat J , Aboulafia-Brakha T , Assal F , GRECOG-VASC Neuropsychological Committee ((2012) ) French adaptation of the vascular cognitive impairment harmonization standards: The GRECOG-VASC study. Int J Stroke 7: , 362–363. |
[22] | Kalafat M , Hugonot-Diener L , Poitrenaud J ((2003) ) Standardisation et étalonnage français du Mini Mental State (MMS) version GRÉCO. Rev Neuropsychol 13: , 209–236. |
[23] | Thuillard Colombo F , Assal G ((1992) ) Adaptation française du test de dénomination de Boston. Versions abrégées. Eur Rev Appl Psychol 42: , 67–73. |
[24] | Rey A ((1959) ), Test de copie d’une figure complexe: Manuel., Paris. |
[25] | Van der Linden M , Coyette F , Poitrenaud J , et les membres du GREMEM ((2004) ) L’épreuve de rappel libre/rappel indicé à 16 items (RL/RI-16)“L’évaluation des troubles de la mémoire. Présentation de quatre tests de mémoire épisodique”, Solal, Marseille. |
[26] | Baddeley A , Emslie H , Nimmo-Smith I ((1994) ), Doors and people: A test of visual and verbal recall and recognition. |
[27] | Weschler D ((1955) ), The Weschler Adult Intelligence Scale (Manual). |
[28] | Godefroy O , Azouvi P , Robert P , Roussel M , LeGall D , Meulemans T , Groupe de Réflexion sur l’Evaluation des Fonctions Exécutives Study Group ((2010) ) Dysexecutive syndrome:Diagnostic criteria and validation study. Ann Neurol 68: , 855–864. |
[29] | Cardebat D , Doyon B , Puel M , Goulet P , Joanette Y ((1990) ) [Formal and semantic lexical evocation in normal subjects. Performance and dynamics of production as a function of sex, age and educational level]. Acta Neurol Belg 90: , 207–217. |
[30] | Reitan R ((1958) ), Validity of trail making tests as an indicator of organic brain damage. |
[31] | Stroop J ((1935) ) Studies of interference in serial verbal reactions. J Exp Psychol 18: , 643–662. |
[32] | Godefroy O , Grefex (Groupe de Réflexion pour l’évaluation des Fonctions EXécutives) ((2008) ) Fonctions exécutives et pathologies neurologiques et psychiatriques: évaluation en pratique clinique, Marseille. |
[33] | Montgomery SA , Asberg M ((1979) ) A new depression scale designed to be sensitive to change. Br J Psychiatry J Ment Sci 134: , 382–389. |
[34] | Spielberger C , Gorsuch R , Lushene R , Vagg P , Jacobs G ((1983) ), Manual for the State-Trait Anxiety Inventory (Form Y1 - Y2). |
[35] | Krupp LB ((1989) ) The Fatigue Severity Scale: Application to patients with multiple sclerosis and systemic lupus erythematosus. Arch Neurol 46: , 1121. |
[36] | Lawton MP , Brody EM ((1969) ) Assessment of older people: Self-maintaining and instrumental activities of daily living. Gerontologist 9: , 179–186. |
[37] | Godefroy O , Duhamel A , Leclerc X , Saint Michel T , Hénon H , Leys D ((1998) ) Brain-behaviour relationships. Some models and related statistical procedures for the study of brain-damaged patients. Brain 121: (Pt 8), 1545–1556. |
[38] | Wardlaw JM , Smith EE , Biessels GJ , Cordonnier C , Fazekas F , Frayne R , Lindley RI , O’Brien JT , Barkhof F , Benavente OR , Black SE , Brayne C , Breteler M , Chabriat H , Decarli C , de Leeuw F-E , Doubal F , Duering M , Fox NC , Greenberg S , Hachinski V , Kilimann I , Mok V , Oostenbrugge R van , Pantoni L , Speck O , Stephan BCM , Teipel S , Viswanathan A , Werring D , Chen C , Smith C , van Buchem M , Norrving B , Gorelick PB , Dichgans M , STandards for ReportIng Vascular changes on nEuroimaging (STRIVE v1) ((2013) ) Neuroimaging standards for research into small vessel disease and its contribution to ageing and neurodegeneration. Lancet Neurol 12: , 822–838. |
[39] | Hachinski V , Iadecola C , Petersen RC , Breteler MM , Nyenhuis DL , Black SE , Powers WJ , DeCarli C , Merino JG , Kalaria RN , Vinters HV , Holtzman DM , Rosenberg GA , Wallin A , Dichgans M , Marler JR , Leblanc GG ((2006) ) National Institute of Neurological Disorders and Stroke-Canadian Stroke Network vascular cognitive impairment harmonization standards. Stroke 37: , 2220–2241. |
[40] | Cordonnier C , Potter GM , Jackson CA , Doubal F , Keir S , Sudlow CLM , Wardlaw JM , Al-Shahi Salman R ((2009) ) improving interrater agreement about brain microbleeds: Development of the Brain Observer MicroBleed Scale (BOMBS). Stroke 40: , 94–99. |
[41] | Schmidt P , Gaser C , Arsic M , Buck D , Förschler A , Berthele A , Hoshi M , Ilg R , Schmid VJ , Zimmer C , Hemmer B , Mühlau M ((2012) ) An automated tool for detection of FLAIR-hyperintense white-matter lesions in Multiple Sclerosis. Neuroimage 59: , 3774–3783. |
[42] | Schmidt P ((2017) ) Bayesian inference for structured additive regression models for large-scale problems with applications to medical imaging. Dissertation, LMU München: Faculty of Mathematics, Computer Science and Statistics. |
[43] | Vanderbecq Q , Xu E , Ströer S , Couvy-Duchesne B , Diaz Melo M , Dormont D , Colliot O , Alzheimer’s Disease Neuroimaging Initiative ((2020) ) Comparison and validation of seven white matter hyperintensities segmentation software in elderly patients. Neuroimage Clin 27: , 102357. |
[44] | Arnoux A , Toba MN , Duering M , Diouf M , Daouk J , Constans J-M , Puy L , Barbay M , Godefroy O ((2018) ) Is VLSM a valid tool for determining the functional anatomy of the brain? Usefulness of additional Bayesian network analysis. Neuropsychologia 121: , 69–78. |
[45] | Tzourio-Mazoyer N , Landeau B , Papathanassiou D , Crivello F , Etard O , Delcroix N , Mazoyer B , Joliot M ((2002) ) Automated anatomical labeling of activations in SPM using a macroscopic anatomical parcellation of the MNI MRI single-subject brain. Neuroimage 15: , 273–289. |
[46] | Catani M , Thiebaut de Schotten M ((2008) ) A diffusion tensor imaging tractography atlas for virtual in vivo dissections. Cortex 44: , 1105–1132. |
[47] | Godefroy O , Gibbons L , Diouf M , Nyenhuis D , Roussel M , Black S , Bugnicourt JM , GREFEX study group ((2014) ) Validation of an integrated method for determining cognitive ability: Implications for routine assessments and clinical trials. Cortex 54: , 51–62. |
[48] | Roussel M , Godefroy O ((2016) ) La batterie GRECOGVASC: Evaluation et diagnostic des troubles neurocognitifs vasculaires avec ou sans contexte d’accident vasculaire cérébral, De Boeck Universite. |
[49] | Boone M , Roussel M , Chauffert B , Le Gars D , Godefroy O ((2016) ) Prevalence and profile of cognitive impairment in adult glioma: A sensitivity analysis. J Neurooncol 129: , 123–130. |
[50] | Barbay M , Taillia H , Nédélec-Ciceri C , Bompaire F , Bonnin C , Varvat J , Grangette F , Diouf M , Wiener E , Mas J-L , Roussel M , Godefroy O , GRECOG-VASC Study Group ((2018) ) Prevalence of poststroke neurocognitive disorders using National Institute of Neurological Disorders and Stroke-Canadian Stroke Network, VASCOG Criteria (Vascular Behavioral and Cognitive Disorders), and Optimized Criteria of Cognitive Deficit. Stroke 49: , 1141–1147. |
[51] | Alonso-Lana S , Marquié M , Ruiz A , Boada M ((2020) ) Cognitive and neuropsychiatric manifestations of COVID-19 and effects on elderly individuals with dementia. Front Aging Neurosci 12: , 588872. |
[52] | Miners S , Kehoe PG , Love S ((2020) ) Cognitive impact of COVID-19: Looking beyond the short term. Alzheimers Res Ther 12: , 170. |
[53] | Baker HA , Safavynia SA , Evered LA ((2021) ) The “third wave”: Impending cognitive and functional decline in COVID-19 survivors. Br J Anaesth 126: , 44–47. |
[54] | Oronsky B , Larson C , Hammond TC , Oronsky A , Kesari S , Lybeck M , Reid TR ((2021) ) A review of persistent post-COVID syndrome (PPCS). Clin Rev Allergy Immunol, doi: 10.1007/s12016-021-08848-3 |
[55] | Sahu U , Biswas D , Singh AK , Khare P ((2021) ) Mechanism involved in the pathogenesis and immune response against SARS-CoV-2 infection. Virusdisease 32: , 211–219. |
[56] | Yongzhi X ((2021) ) COVID-19-associated cytokine storm syndrome and diagnostic principles: An old and new Issue. Emerg Microbes Infect 10: , 266–276. |
[57] | Debette S , Markus HS ((2010) ) The clinical importance of white matter hyperintensities on brain magnetic resonance imaging: Systematic review and meta-analysis. BMJ 341: , c3666. |
[58] | Périn B , Godefroy O , Fall S , de Marco G ((2010) ) Alertness in young healthy subjects: An fMRI study of brain region interactivity enhanced by a warning signal. Brain Cogn 72: , 271–281. |
[59] | Kopp B , Rösser N , Tabeling S , Stürenburg HJ , de Haan B , Karnath H-O , Wessel K ((2015) ) Errors on the Trail Making Test are associated with right hemispheric frontal lobe damage in stroke patients. Behav Neurol 2015: , 309235. |
[60] | Bubb EJ , Metzler-Baddeley C , Aggleton JP ((2018) ) The cingulum bundle: Anatomy, function, and dysfunction. Neurosci Biobehav Rev 92: , 104–127. |
[61] | Matias-Guiu JA , Cortés-Martínez A , Montero P , Pytel V , Moreno-Ramos T , Jorquera M , Yus M , Arrazola J , Matías-Guiu J ((2018) ) Structural MRI correlates of PASAT performance in multiple sclerosis. BMC Neurol 18: , 214. |
[62] | Godefroy O , Roussel M , Despretz P , Quaglino V , Boucart M ((2010) ) Age-related slowing: Perceptuomotor, decision, or attention decline? Exp Aging Res 36: , 169–189. |
[63] | Raju H , Tadi P ((2021) ) Neuroanatomy, somatosensory cortex. In StatPearls,, StatPearls Publishing, Treasure Island (FL). |
[64] | AbuHasan Q , Munakomi S ((2021) ) Neuroanatomy, pyramidal tract. In StatPearls, StatPearls Publishing, Treasure Island (FL). |
[65] | Emos MC , Agarwal S ((2021) ) Neuroanatomy, internal capsule. In StatPearls, StatPearls Publishing, Treasure Island (FL). |
[66] | Di X , Rypma B , Biswal BB ((2014) ) Correspondence of executive function related functional and anatomical alterations in aging brain. Prog Neuropsychopharmacol Biol Psychiatry 48: , 41–50. |
[67] | Maldonado IL , Parente de Matos V , Castro Cuesta TA , Herbet G , Destrieux C ((2020) ) The human cingulum: From the limbic tract to the connectionist paradigm. Neuropsychologia 144: , 107487. |
[68] | Fujii M , Maesawa S , Ishiai S , Iwami K , Futamura M , Saito K ((2016) ) Neural basis of language: An overview of an evolving model. Neurol Med Chir (Tokyo) 56: , 379–386. |
[69] | Mandonnet E ((2017) ) A surgical approach to the anatomo-functional structure of language. Neurochirurgie 63: , 122–128. |
[70] | Herbet G , Zemmoura I , Duffau H ((2018) ) Functional anatomy of the inferior longitudinal fasciculus: From historical reports to current hypotheses. Front Neuroanat 12: , 77. |
[71] | Ladopoulos T , Zand R , Shahjouei S , Chang JJ , Motte J , Charles James J , Katsanos AH , Kerro A , Farahmand G , Vaghefi Far A , Rahimian N , Ebrahimzadeh SA , Abedi V , Papathanasiou M , Labedi A , Schneider R , Lukas C , Tsiodras S , Tsivgoulis G , Krogias C ((2021) ) COVID-19: Neuroimaging features of a pandemic. J Neuroimaging 31: , 228–243. |