Time Trends of Cerebrospinal Fluid Biomarkers of Neurodegeneration in Idiopathic Normal Pressure Hydrocephalus
Abstract
Background:
Longitudinal changes in cerebrospinal fluid (CSF) biomarkers are seldom studied. Furthermore, data on biomarker gradient between lumbar (L-) and ventricular (V-) compartments seems to be discordant.
Objective:
To examine alteration of CSF biomarkers reflecting Alzheimer’s disease (AD)-related amyloid-β (Aβ) aggregation, tau pathology, neurodegeneration, and early synaptic degeneration by CSF shunt surgery in idiopathic normal pressure hydrocephalus (iNPH) in relation to AD-related changes in brain biopsy. In addition, biomarker levels in L- and V-CSF were compared.
Methods:
L-CSF was collected prior to shunt placement and, together with V-CSF, 3–73 months after surgery. Thereafter, additional CSF sampling took place at 3, 6, and 18 months after the baseline sample from 26 iNPH patients with confirmed Aβ plaques in frontal cortical brain biopsy and 13 iNPH patients without Aβ pathology. CSF Amyloid-β42 (Aβ42), total tau (T-tau), phosphorylated tau (P-tau181), neurofilament light (NFL), and neurogranin (NRGN) were analyzed with customized ELISAs.
Results:
All biomarkers but Aβ42 increased notably by 140–810% in L-CSF after CSF diversion and then stabilized. Aβ42 instead showed divergent longitudinal decrease between Aβ-positive and -negative patients in L-CSF, and thereafter increase in Aβ-negative iNPH patients in both L- and V-CSF. All five biomarkers correlated highly between V-CSF and L-CSF (Aβ42 R = 0.87, T-tau R = 0.83, P-tau R = 0.92, NFL R = 0.94, NRGN R = 0.9; all p < 0.0001) but were systematically lower in V-CSF (Aβ42 14 %, T-tau 22%, P-tau 20%, NFL 32%, NRGN 19%). With APOE genotype-grouping, only Aβ42 showed higher concentration in non-carriers of allele ɛ4.
Conclusion:
Longitudinal follow up shows that after an initial post-surgery increase, T-tau, P-tau, and NRGN are stable in iNPH patients regardless of brain biopsy Aβ pathology, while NFL normalized toward its pre-shunt levels. Aβ42 as biomarker seems to be the least affected by the surgical procedure or shunt and may be the best predictor of AD risk in iNPH patients. All biomarker concentrations were lower in V- than L-CSF yet showing strong correlations.
INTRODUCTION
The biochemical composition of cerebrospinal fluid (CSF) is commonly used as a surrogate measure to reflect changes in brain metabolism. CSF is assumed to undergo alterations by the time it arrives in the lumbar region (reviewed in [1]). Idiopathic normal pressure hydrocephalus (iNPH) is a geriatric disorder characterized by impaired gait and balance, urinary incontinence, and cognitive decline with evidence of ventriculomegaly [2, 3]. In iNPH patients, CSF shunting is an effective treatment [4]. Shunt-valve puncture in iNPH patients, often used to test the performance of the shunt, allows for the collection of CSF from the brain ventricles, which is easy, painless, and considered safe. In addition, a right frontal cortical tissue biopsy, collected during shunt placement, allows for analysis of brain pathology. Nearly half of iNPH patients show Alzheimer’s disease (AD)-related amyloid-β (Aβ) pathology in biopsies while 10% show concomitant Aβ and tau pathology [5]. Aβ42 is an amyloid-derived protein that has proven its value to detect Aβ pathology in AD, as well as concomitant Aβ pathology in assisting diagnosis iNPH [6–11].
Total tau (T-tau) and tau phosphorylated at amino acid threonine 181 (P-tau) levels in CSF are key diagnostic biomarkers for AD, but their interpretation as markers of neurodegeneration in the brain is less clear (reviewed in [12]). Secretion of T-tau and P-tau could be induced by Aβ pathology [13, 14], and thus the levels in CSF may at least in part reflect accumulating Aβ pathology in the AD brain, but CSF T-tau also increases in disorders without plaques, e.g., with severe neurodegeneration in Creutzfeldt-Jakob disease [15] and acute brain injury such as stroke [16]. However, there is also a step-wise increase with more severe tau pathology, as determined by positron emission tomography [17].
Neurogranin (NRGN) is a post-synaptic protein that is upregulated in the CSF of AD patients [12, 18–24]. Higher CSF NRGN levels correlate with the rate of cognitive decline [25, 26]. Together these data suggest that NRGN may be a marker of monitor synaptic damage in the brain. High CSF NRGN levels associate with Aβ plaques but not with tau, α-synuclein, or TDP-43 pathology [24], suggesting that, similar to tau, its upregulation in CSF may at least in part reflect a response to accumulating Aβ pathology in the brain.
Neurofilament light chain (NFL) is a scaffolding protein of the neuronal cytoskeleton that is highly expressed in large caliber myelinated axons with a function in axonal structural support, growth, and regulation. CSF NFL is increased in several neurodegenerative conditions and is hypothesized to leak into CSF upon axonal injury and to be a general biomarker of neurodegeneration [27]. NFL associates with disease progression in AD independent of Aβ pathology [28].
Prospective studies on CSF biomarkers are sparse and little is known on the changes after CSF shunt. In this study, we compared Aβ42, T-tau, P-tau, NRGN, and NFL, potential biomarkers of neurodegeneration, in a population of iNPH patients with and without Aβ pathology in their brain biopsy. The objectives of this study were: 1) to determine whether ventricular CSF (V-CSF) would be superior to lumbar CSF (L-CSF) for the analysis of these biomarkers; and 2) to analyze and compare the longitudinal change in Aβ42, T-tau, P-tau, NRGN, and NFL concentrations in L-CSF and V-CSF samples collected repeatedly over 18 months.
METHODS
Study population and sample collection
Altogether, 201 patients with probable iNPH according to Relkin criteria [3] were shunted by right frontal puncture and ventriculoperitoneal CSF shunt (PS Medical Strata II valve) between January 2009 and December 2015 at the Kuopio University Hospital following a previously described protocol [29] (Supplementary Table 1). Brain biopsies of 41 patients were taken during shunt surgery and analyzed according to the established protocol [30]. The biopsy was taken from the right frontal cortex, 3 cm from the midline and anterior to the coronal suture, and the size of the cylinder-shaped sample was 2–5 mm in diameter and 3–7 mm in length. Biopsies were obtained using either biopsy forceps or since 2010 by disposable Temno EvolutionR TT146 biopsy needle (Merit Medical Systems Inc., South Jordan, UT, USA). Out of them, 28 patients with confirmed Aβ plaques in their frontal cortical brain biopsy (5 with concomitant tau pathology) and 13 patients without Aβ pathology (control group) were included in the study (Fig. 1). Two Aβ-positive iNPH patients withdrew in early stage (Fig. 2). All participants had Clinical Dementia Rating (CDR)≤1 and Mini-Mental State Examination (MMSE)≥20. Exclusion criteria were contraindications for lumbar puncture, compromised well-being and serology positive hepatitis B or C, or human immunodeficiency virus.
Fig. 1
Selection of shunted iNPH patients presented as a flow-chart. Eligible patients were sorted to groups based on the histopathological examination of Aβ in frontal cortical brain biopsy. Based on participating Aβ-positive patients, controls were requested with the ratio of 2 : 1, leading eventually to the group sizes of 28 Aβ-positive individuals and 13 Aβ-negative individuals. iNPH, idiopathic normal pressure hydrocephalus; Aβ, amyloid- β; MMSE, Mini-Mental State Examination.
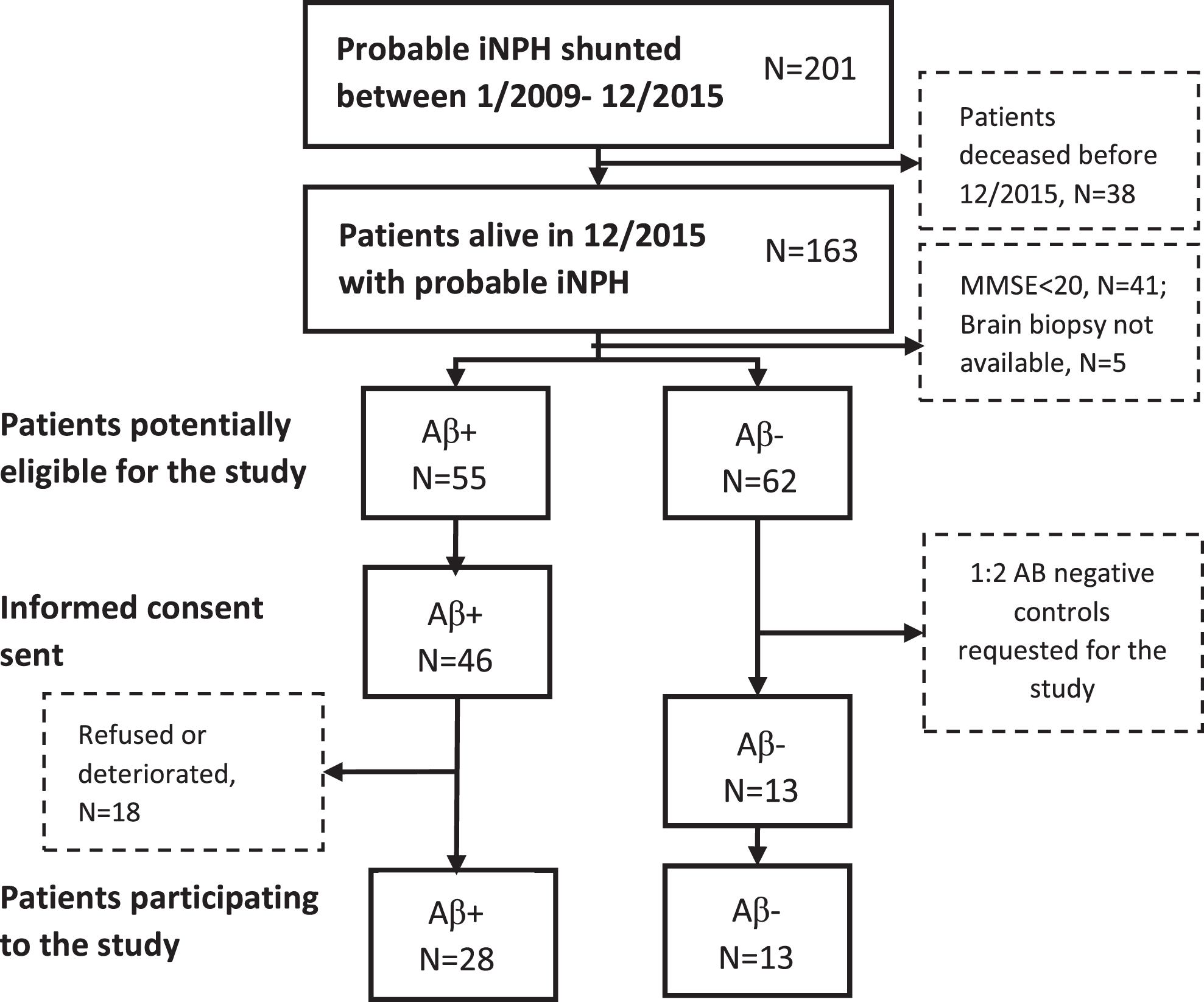
Fig. 2
Sample collection over time with the number of samples collected for V- and L-CSF in the Aβ-positive and -negative groups. Pre-shunt L-CSF was collected prior to the surgery. The B0 sample collection point was at least 3 but up to 73 months (mean 24 months; median, 18 months) after the shunt placement, followed by 3 M, 6 M, and 18 M sample collection points. L-CSF and V-CSF from the same patient were collected on the same day. Drop-outs and deaths between the time points presented as numbers from study population. Cognition was tested at B0, 6 M, and 18M. #7 revision before B1; ##2 revision before B0; *B0 partial samples: 6 in Aβ+ and 3 in Aβ-, 3 M partial samples: 5 in Aβ+ and 5 in Aβ-, 6 M partial samples: 4 in Aβ+ and 4 in Aβ-, 18 M partial samples: 3 in Aβ+ and 5 in Aβ-; **B0: bloody CSF in 2 Aβ+ samples, 3 M: bloody CSF in 1 Aβ+ and 1 Aβ- sample; B1, pre-surgery sample collection time point; B0, baseline visit of the follow-up; 3 M, three-month study visit; 6 M, six-month study visit; 18 M, 18-month study visit; L-CSF, cerebrospinal fluid collected with lumbar puncture; V-CSF, cerebrospinal fluid collected with shunt valve puncture.
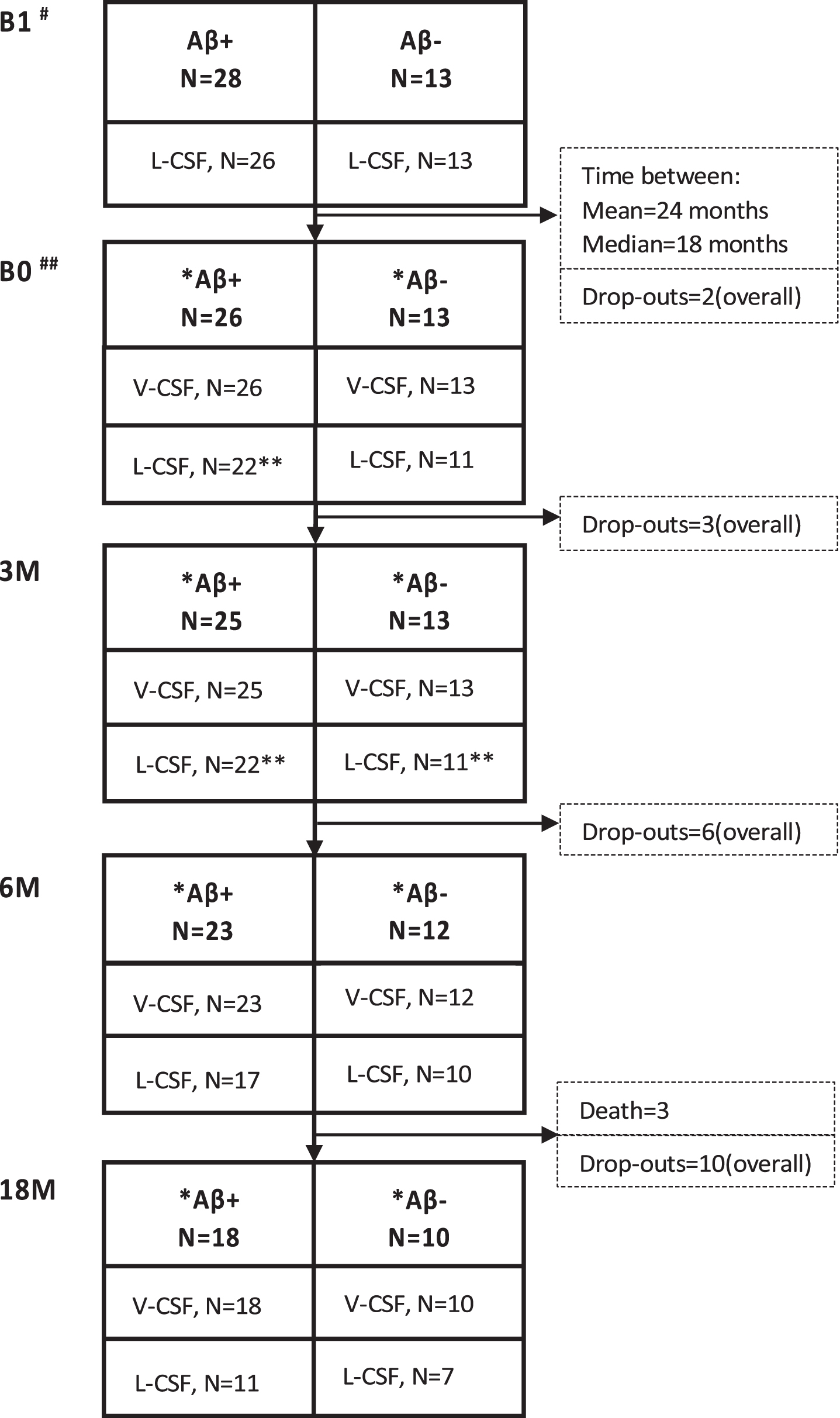
Pre-shunt (B1) L-CSF (n = 39) was obtained during diagnostic CSF diversion, centrifuged and stored at a temperature controlled –80°C freezer. Post-shunting lumbar (L-) and ventricular (V-) CSF were simultaneously collected 3 to 73 months after the shunt placement (median 18 [mean 24] months post-surgery), to allow for putative normalization of the biomarker levels after injury caused by the surgery and thereafter at 3, 6, and 18 months (B0, 3 M, 6 M, and 18 M) sampling points (Fig. 2). All shunt valve punctures (n = 142) to obtain V-CSF were successful without blood contamination, any infections or other procedure-related harm. Lumbar puncture after CSF shunt was successful only in 111 out of 142 attempts (78%) and furthermore 4 samples (3.6%) had blood contamination. In addition, one lumbar puncture led to persisting radicular pain over two months (normal lumbar MRI). Samples were collected in single 10 mL polypropylene tubes to avoid adsorption of proteins to tube walls. CSF samples were mixed to avoid possible gradient effects, centrifuged, aliquoted, frozen and stored at a temperature-controlled –80°C freezer immediately after collection. All samples analyzed in this study had at most one freeze-thaw cycle.
Tissue biopsy results on Aβ and tau pathology were used to divide patients into a control (no AD-type pathology; here referred to as biopsy-negative) group and a group with concomitant AD pathology (Aβ and in five cases also tau; here referred to as biopsy-positive). Patients were APOE genotyped by standard PCR method [31]. DNA was extracted from venous blood using a commercial kit according to the manufacturer’s protocol (Illustra Blood GenomicPrep Mini Spin Kit, GE Healthcare, Little Chalfont, UK). Figure 2 shows the number of L- and V-CSF samples collected at the different sampling points per group indicating dropouts at each time. This study was approved by the Ethics Committee, Hospital District of Northern Savo. All participants gave written, informed consent prior to participation into the study.
Biomarker analysis
All CSF and plasma samples were analyzed at the Clinical Neurochemistry Laboratory, Sahlgrenska University Hospital, Mölndal, Sweden. CSF concentrations of Aβ42, T-tau, and P-tau were measured with INNOTEST® ELISA kits (Fujirebio, Ghent, Belgium). CSF neurofilament light (NFL) concentration was measured with the NF-Light kit (UmanDiagnostics, Umeå, Sweden) [32]. CSF neurogranin (NRGN) concentration was measured using a sandwich ELISA developed at the Clinical Neurochemistry Laboratory [24]. In addition, we measured CSF concentrations of Aβ isoforms Aβ38, Aβ40, and Aβ42 using a multiplexed electrochemiluminescence assay, as described by the kit manufacturer (Meso Scale Discovery, Rockville, MD, USA) [33]. All lumbar and ventricular samples of all sampling time points for each patient were analyzed on the same plate. All biomarker measurements were performed using one batch of reagents by board-certified laboratory technicians blinded to the clinical information.
Statistics
All statistical analysis was performed with IBM SPSS Statistics version 25.00 for IOS. For numerical data, group comparisons and changes over time were analyzed by mixed model multivariate analysis of variance (ANOVA). Pearson correlation coefficients were calculated to evaluate the strength of association between biomarkers in lumbar and ventricular CSF. All significances calculated were two-sided with 5% significance level used.
Data availability statement
All data related but not published within the article is available and will be shared anonymized upon reasonable request to any qualified investigator.
RESULTS
Table 1 summarizes patient demographics, clinical characteristics, and mean biomarker values. Brain biopsy Aβ-positive patients were weighted in 2 : 1 ratio. Brain biopsy Aβ-positive patients were on the average 3.5 years older than biopsy Aβ-negative patients (p = 0.039). The longitudinal progress of gait velocity is presented in Supplementary Figure 1F.
Table 1
Demographic data and biomarker values of the study population with the number of patients
Group | Aβ+, n = 26 | Aβ-, n = 13 | Pooled, n = 39 | |||
Female (%) | 11 (42) | 5 (38) | 16 (41) | |||
Age; mean (min-max) | ||||||
B1 | 76 (63–88) | 72 (64–80) | 75 (63–88) | |||
B0 | 78 (64–89) | 73 (65–81) | 76 (64–89) | |||
MMSE; mean | ||||||
B1 | 24 | 23 | 24 | |||
B0 | 23 | 23 | 23 | |||
6M | 24 | 24 | 24 | |||
18M | 23 | 23 | 23 | |||
APOE genotype (%) | ||||||
34 | 10 (38) | 2 (15) | 12 (31) | |||
33 | 11 (42) | 10 (77) | 21 (54) | |||
24 | 1 (4) | 0 (0) | 1 (3) | |||
23 | 4 (15) | 1 (8) | 5 (13) | |||
Aβ+, Brain biopsy amyloid-Aβ positive, n = 26 | ||||||
Timescale | B1 | B0 | 3 M | 6 M | 18 M | |
Biomarkers (Mean) | Location | |||||
Aβ42 (ng/l) | V-CSF | 481 (440) | 482 (467) | 462 (400) | 587 (519) | |
L-CSF | 704 (724) | 554 (530) | 562 (527) | 538 (522) | 583 (581) | |
T-Tau (ng/l) | V-CSF | 854 (741) | 805 (737) | 824 (757) | 773 (832) | |
L-CSF | 248 (220) | 1,057 (923) | 1,174 (951) | 1,039 (923) | 1,110 (1,054) | |
P-Tau (ng/l) | V-CSF | 99 (100) | 109 (102) | 106 (102) | 114 (123) | |
L-CSF | 41 (42) | 125 (114) | 137 (127) | 130 (125) | 147 (152) | |
NFL (ng/l) | V-CSF | 2,398 (1,404) | 2,215 (1,832) | 1,633 (1,382) | 2,629 (1,987) | |
L-CSF | 1,864 (1,179) | 2,692 (1,884) | 2,669 (2,065) | 2,586 (2,077) | 3,288 (2,135) | |
NRGN (ng/l) | V-CSF | 592 (529) | 651 (532) | 631 (549) | 530 (519) | |
L-CSF | 161(42) | 704 (494) | 825 (619) | 622 (546) | 902 (653) | |
Aβ-, Brain biopsy amyloid-β negative, n = 13 | ||||||
Biomarkers | Location | |||||
Aβ42 (ng/l) | V-CSF | 664 (721) | 705 (711) | 721 (729) | 1,048 (1,057) | |
L-CSF | 786 (771) | 767 (795) | 760 (777) | 825 (810) | 1,052 (1,012) | |
T-Tau (ng/l) | V-CSF | 577 (478) | 574 (492) | 643 (637) | 632 (621) | |
L-CSF | 186 (152) | 617 (506) | 636 (550) | 755 (523) | 845 (751) | |
P-Tau (ng/l) | V-CSF | 74 (69) | 79 (77) | 86 (85) | 110 (115) | |
L-CSF | 34 (27) | 78 (70) | 86 (86) | 98 (85) | 146 (145) | |
NFL (ng/l) | V-CSF | 2,860 (1,796) | 1,629 (1,432) | 1533 (1,257) | 1,757 (1,046) | |
L-CSF | 1,841 (1,060) | 5,136 (4077) | 2,974 (2,651) | 2,198 (1,647) | 3,250 (2,398) | |
NRGN (ng/l) | V-CSF | 462 (325) | 497 (468) | 500 (389) | 498 (432) | |
L-CSF | 70 (40) | 357 (260) | 471 (541) | 405 (303) | 736 (799) |
Patients grouping of Aβ+ and Aβ- is based on the brain biopsy Aβ histopathological examination result. Age of iNPH patients are presented with mean, minimum and maximum, sex as number and percent, MMSE as a mean and APOE ɛ4 carriers as numbers and percent in the timescale of B1, B0, 3 M, 6 M, and 18 M. Biomarker concentration values of Aβ42, T-tau, P-tau, NFL, and NRGN presented as a means and medians with the timescale and location of sample collection. Aβ, AD-related amyloid-β MMSE, Mini-Mental State Examination; APOE ɛ4, apolipoprotein ɛ4 allele; B1, pre-surgery sample collection time point; B0, baseline visit of the follow-up; 3 M, three-month study visit; 6 M, six-month study visit; 18 M, 18-month study visit; Aβ42, Amyloid-β 42 protein; T-tau, total tau; P-tau, tau phosphorylated at threonine 181; NFL, neurofilament light; NRGN, neurogranin; L-CSF, cerebrospinal fluid collected with lumbar puncture; V-CSF, cerebrospinal fluid collected with shunt valve puncture.
Preoperative lumbar CSF Aβ42, T-tau, P-tau, NFL, and NRGN concentrations were similar in brain biopsy Aβ-positive and -negative iNPH patients (Table 1, Fig. 3). After CSF shunt surgery, lumbar CSF Aβ42 decreased (p = 0.043, Fig. 3A), especially in APOE ɛ4 carriers (p = 0.006). Notable increases were seen in T-tau (p < 0.001), P-tau (p < 0.001), and NRGN (p = 0.001), which remained elevated during the later follow-up (Fig. 3C, E, I). The increase was more prominent in Aβ-positive patients regarding P-tau (p = 0.025, Fig. 3E) and tended to be more obvious also in T-tau (p = 0.054, Fig. 3C), but was not significant for NRGN (p = 0.287, Fig. 3I). In NFL based on our modelling, the increase after shunt (p = 0.004) was only temporary and estimated to normalize in 9 months after the surgery (Supplementary Figure 1D). The increase was more obvious in Aβ-negative patients (p = 0.047, Fig. 3G) but was rather related with time delay from surgery to the first follow-up which was significantly shorter in Aβ-negative individuals (average 0.7 versus 2.2 years, p = 0.001). The temporal dynamics of the measured biomarkers in relation to pre-operative values are presented in Supplementary Figure 1A-E.
Fig. 3
Longitudinal analysis of biomarkers of neurodegeneration in lumbar (A, C, E, G, and I) and ventricular (B, D, F, H, and J) CSF for amyloid-β42 (Aβ42; A, B), total tau (T-tau; C, D), tau phosphorylated at threonine 181 (P-tau; E, F), neurofilament light (NFL; G, H), and neurogranin (NRGN; I, J). iNPH patients were grouped into to biopsy positive (dark gray) and biopsy negative (light gray) patients based on the presence or absence of Aβ pathology in their corresponding frontal biopsy. Values expressed as means±standard error. *p < 0.05; **p < 0.01 between biopsy-positive and -negative patients in specific time point. B1, pre-surgery sample collection time point; B0, baseline visit of the follow-up; 3 M, three-month study visit; 6 M, six-month study visit; 18 M, 18-month study visit; L-CSF, cerebrospinal fluid collected with lumbar puncture; V-CSF, cerebrospinal fluid collected with shunt valve puncture.
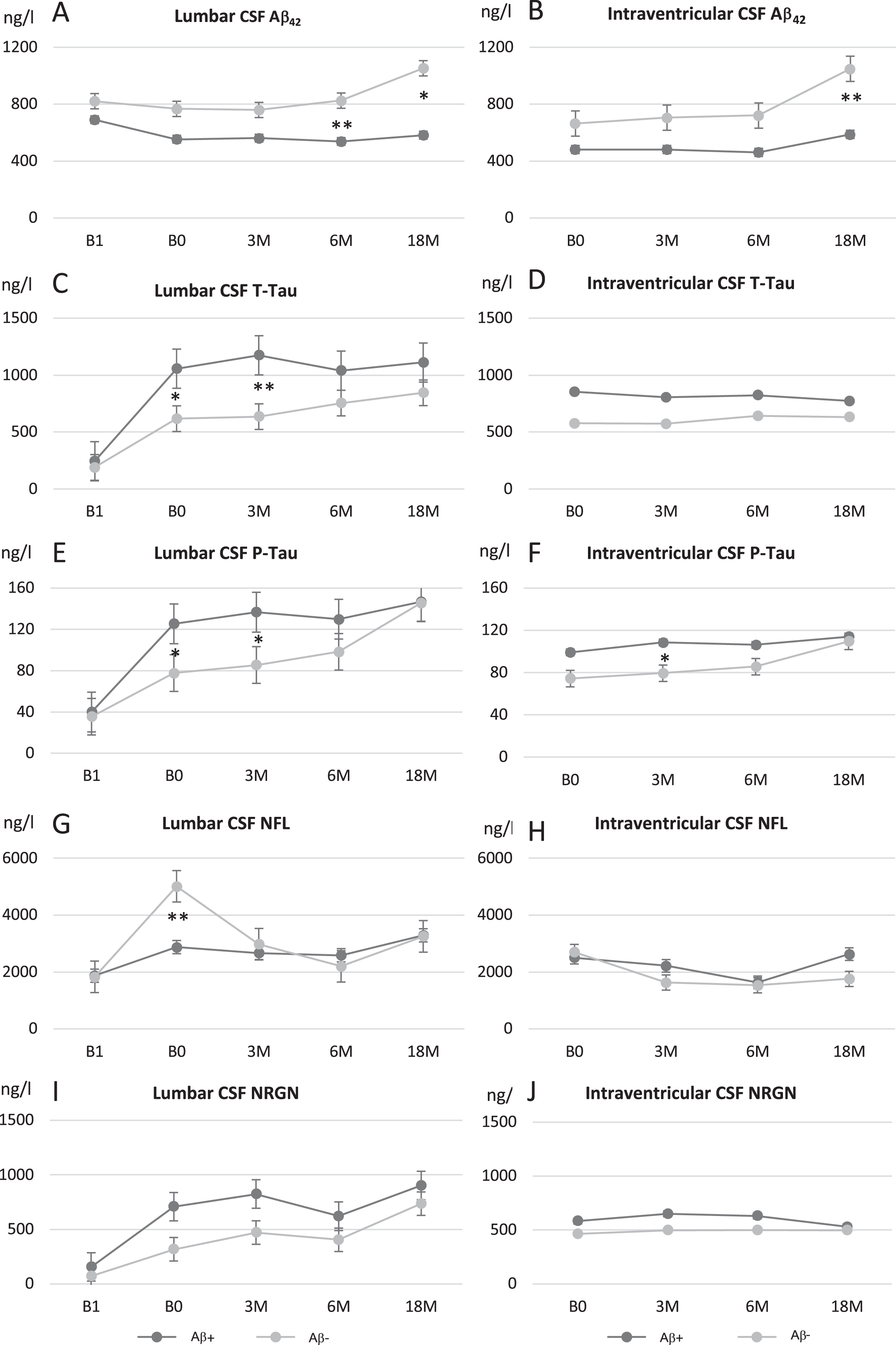
Despite the initial decrease after shunt, Aβ42 concentration from L- and V-CSF showed increase during the entire follow-up (p < 0.0001, Fig. 3). Increase was mostly present in brain biopsy-negative iNPH patients as the positive group remained rather stable after post-surgery decrease. The difference of Aβ42 was significant between the groups throughout the follow-up (p = 0.009). In addition, Aβ42 was lower in APOE ɛ4 carriers and later increased in non-carriers (Fig. 4A, B) (p < 0.0001). With NFL, there was no clear longitudinal change between the groups after the post-surgery fluctuation. Although the increase in T-tau, P-tau, NRGN, and NFL after shunting was somewhat more pronounced in the biopsy-positive iNPH patients through the follow-up, it was not significantly different from the biopsy-negative group (Fig. 3).
Fig. 4
Longitudinal analysis of Aβ42 in lumbar (A) and ventricular (B) CSF in iNPH patients grouped according to APOE ɛ4 genotype carriers (dark gray) and non-carriers (light gray). Values expressed as means±standard error. *p < 0.05*; **p < 0.01 iNPH, idiopathic normal pressure hydrocephalus; APOE ɛ4, apolipoprotein E ɛ4 allele; B1, pre-surgery sample collection timepoint; B0, baseline visit of the follow-up; 3M, three-month study visit; 6 M, six-month study visit; 18 M, 18-month study visit; L-CSF, cerebrospinal fluid collected with lumbar puncture; V-CSF, cerebrospinal fluid collected with shunt valve puncture.
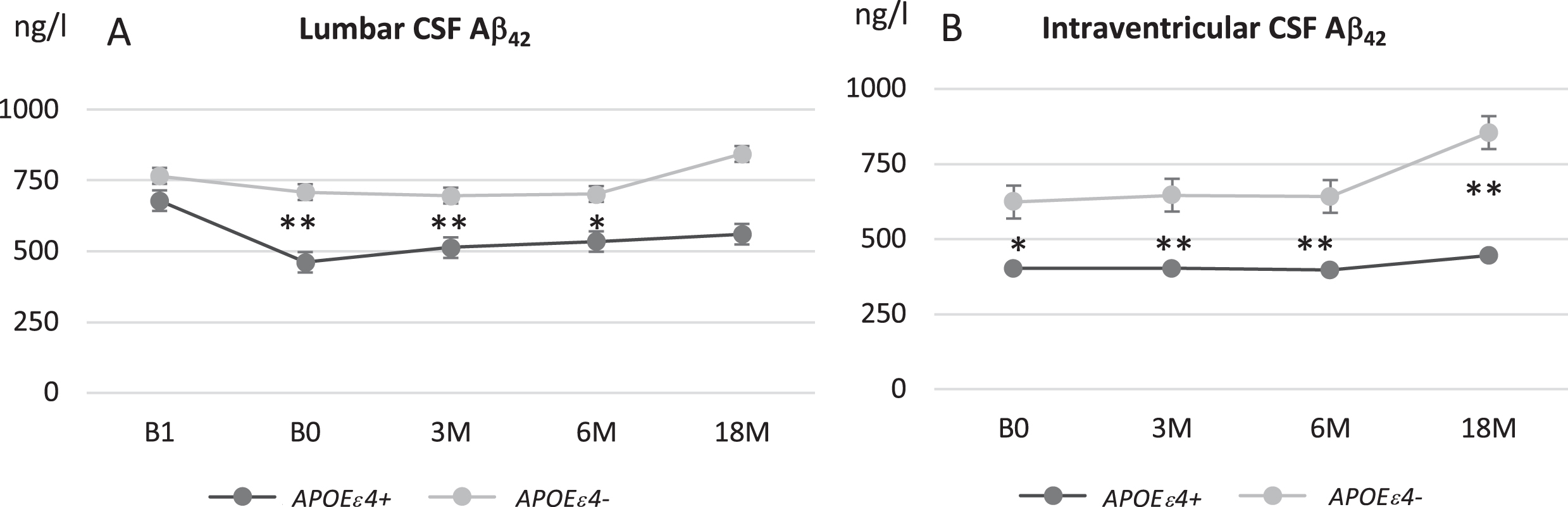
To circumvent inter-individual variation in absolute levels of the biomarkers, we normalized the values of all sampling points as % change towards pre-shunt L-CSF per patient. Again, we observed a significant increase in T-tau, P-tau, and NRGN after shunting in both L- and V-CSF. This increase was 2.5- to 3-fold for T-tau, 2- to 2.5-fold for P-tau, and 6.5- to 8-fold for NRGN and was sustained over time in both L- and V-CSF. Aβ42 showed mild increase of 35% in biopsy Aβ-negative patients both in L- and V-CSF, as the biopsy Aβ-positive patients remained stable. In contrast to Aβ42, T-tau, P-tau, and NRGN, there was no clear increase in NFL in the study population as a whole (Fig. 3G, H), besides the transient increase associated with shunt placement described earlier.
The absolute levels of Aβ42 (14%), T-tau (22%), P-tau (20%), NFL (32%), and NRGN (19%) mea-sured lower in V-CSF compared to L-CSF (Fig. 5). The absolute levels of each biomarker in L- and V-CSF per patient per time point showed a very strong correlation (Aβ42: R = 0.87, p < 0.0001; T-tau: R = 0.83, p < 0.0001; P-tau: R = 0.92, p < 0.0001; NFL: R = 0.94, p < 0.0001; NRGN: R = 0.90, p < 0.0001) (Table 2). No effect of APOE ɛ4 genotype on T-tau, P-tau, NFL, and NRGN levels was observed. The 18M correlation of L- with V-CSF Aβ42/40 ratio was very strong (R = 0.97, p < 0.0001; Fig. 5F).
Table 2
Correlations of Aβ42, T-tau, P-tau, NFL, and NRGN in lumbar- and intraventricular-CSF
Aβ42 | T-tau | P-tau | NFL | NRGN | |
Lumbar CSF | |||||
Aβ42 | 1 | *–0.23 | –0.10 | *–0.21 | *–0.19 |
T-tau | *–0.23 | 1 | ***0.88 | **0.31 | ***0.60 |
P-tau | –0.10 | ***0.88 | 1 | *0.25 | ***0.55 |
NFL | *–0.21 | **0.31 | *0.25 | 1 | *0.17 |
NRGN | *–0.19 | ***0.60 | ***0.55 | *0.17 | 1 |
Intraventricular | |||||
Aβ42 | 1 | 0.01 | *0.19 | –0.05 | 0.07 |
T-tau | 0.01 | 1 | ***0.78 | *0.24 | ***0.43 |
P-tau | *0.19 | ***0.78 | 1 | 0.16 | ***0.44 |
NFL | –0.05 | *0.24 | 0.16 | 1 | 0.06 |
NRGN | 0.07 | ***0.43 | ***0.44 | 0.06 | 1 |
L-CSF &V-CSF | ***0.87 | ***0.83 | ***0.92 | ***0.94 | ***0.90 |
Significances of Pearson-r values presented as *p < 0.05, **p < 0.001, ***p < 0.0001. Aβ42, Amyloid-β 42 protein; T-tau, total tau; P-tau, tau phosphorylated at threonine 181; NFL, neurofilament light; NRGN, neurogranin; L-CSF, cerebrospinal fluid collected with lumbar puncture; V-CSF, cerebrospinal fluid collected with shunt valve puncture.
Fig. 5
Ratios of Aβ42 (A), T-tau (B), P-tau (C), NFL (D), NRGN (E), and Aβ42/40 ratio (F) in V- and L-CSF. Ratios are presented as box and whiskers plot that portrays the median (center line), mean (cross), Q1 (lower edge of box), Q3 (upper edge of box), minimum and maximum (lines) values. Each boxplot presents all results of one sample collection point of the CSF and single dots demonstrate results of a single iNPH patient. The Aβ42/40 result is from 18M time point and presented as correlation matrix. The Aβ42/40 ratios presented showed strong correlation between lumbar and ventricular CSF, expressed as Pearson R2. Linear trend-line adjusted for values to enhance the visibility of correlation. Aβ, amyloid- β; B1, pre-surgery sample collection timepoint; B0, baseline visit of the follow-up; 3M, three-month study visit; 6M, six-month study visit; 18M, 18-month study visit; Aβ42, Amyloid-β 42 protein; T-tau, total tau; P-tau, tau phosphorylated at threonine 181; NFL, neurofilament light; NRGN, neurogranin; L-CSF, cerebrospinal fluid collected with lumbar puncture; V-CSF, cerebrospinal fluid collected with shunt valve puncture; Q1, quartile 1 holding values up to 25 percentile; Q3, quartile 3 holding values up to 75 percentile.
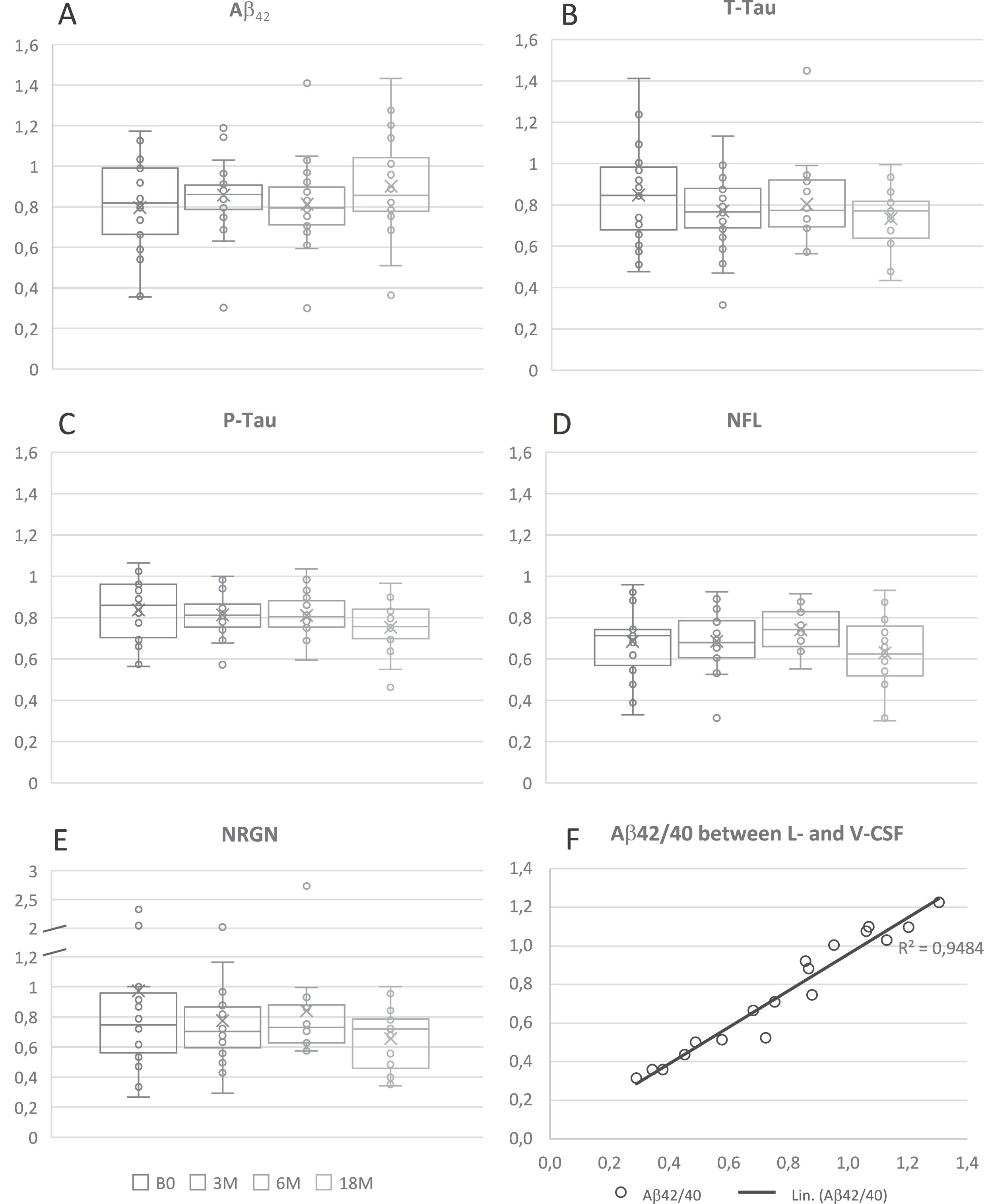
As expected, T-tau showed a very strong correlation with P-tau both in L- and V-CSF (Table 2, Fig. 6). T-tau and P-tau correlated more weakly with NRGN, a correlation of which was somewhat higher in L- versus V-CSF (Table 2, Fig. 6). Aβ42 and NFL, on the other hand, did not correlate well or at all with each other or with T-tau, P-tau, and NRGN (Table 2). Because of a transient increase in NFL in a subset of patients having the B0 sampling point up to 9 months post-surgery, we correlated the NFL values of the pre-shunt, B0 and 3 M sampling points of this group with their corresponding T-tau, P-tau, and NRGN values (data not shown). We found a medium to strong correlation between NFL and T-tau (L-CSF R = 0.76, p < 0.0001; V-CSF R = 0.63, p = 0.009), as well as NFL and P-tau (L-CSF R = 0.64, p < 0.0001; V-CSF R = 0.30, p = 0.263) in both V- and L-CSF. NRGN showed only medium strength correlation in L-CSF (R = 0.56, p = 0.001). These correlations were completely lost in the 6 M and 18 M sampling point values. They were also absent in those iNPH patients that had their 0M sampling point collected over 9 months post-surgery.
Fig. 6
Correlation analysis of T-tau levels versus levels of P-tau (A), T-tau versus NRGN (B), P-tau versus NRGN (C) in lumbar (L-CSF, black triangle) and ventricular (V-CSF, light gray circle) samples. Pearson R2 values and significance level were calculated, and linear graphs adjusted according to the values. All time points of B1, B0, 3 M, 6 M, and 18 M are included in correlation analysis. T-tau, total tau; P-tau, tau phosphorylated at threonine 181; NRGN, neurogranin; B1, pre-surgery sample collection timepoint; B0, baseline visit of the follow-up; 3 M, three-month study visit; 6 M, six-month study visit; 18 M, 18-month study visit; L-CSF, cerebrospinal fluid collected with lumbar puncture; V-CSF, cerebrospinal fluid collected with shunt valve puncture.
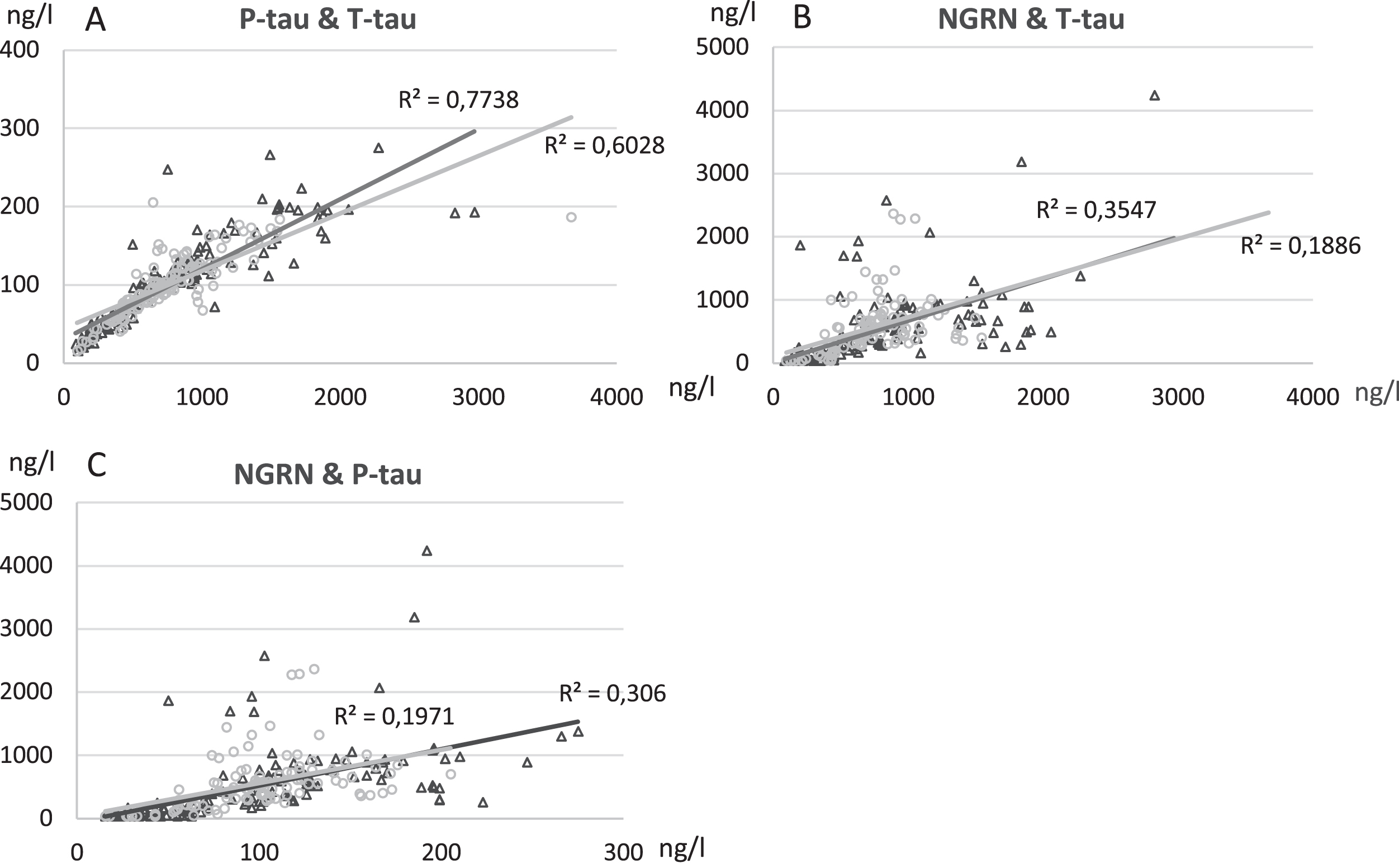
DISCUSSION
In this study, we analyzed longitudinal changes in the concentrations of five potential biomarkers of neurodegeneration (Aβ42, T-tau, P-tau, NRGN, and NFL) in V- and L-CSF of iNPH patients. This study provides the first longitudinal analysis of these biomarkers in simultaneously collected L- and V-CSF. It provides also the first longitudinal comparison of these biomarkers towards pre-operatively obtained L-CSF in iNPH patients who had recovered from the shunt surgery for a minimum of 3 months, after which biomarker levels were assumed to have normalized from acute upregulation resulting from the surgery. In lumbar Aβ42, we found an interesting decrease after shunt surgery, which was most pronounced in brain biopsy-positive patients. However, during the follow-up, the concentrations stabilized in both groups and eventually increased in the brain biopsy-negative group. To our surprise, we observed a sustained longitudinal increase in T-tau, P-tau, and NRGN levels after surgery. Based on our modelling, NFL showed only a transient increase with levels returning to the pre-shunt levels 6 to 9 months post-surgery (Fig. 7). There was also a trend towards somewhat higher biomarker levels in brain biopsy Aβ-positive iNPH patients, apart from Aβ42, and it would be interesting to investigate whether these differences would become significant in larger groups.
Fig. 7
The schematic presentation of the temporal dynamics in biomarkers of neurodegeneration plotted with the time in years (y) from shunt surgery and percentual change from the pre-surgery values (100%). The plots are formed with local polynomial regression and based on the data shown in Supplementary Figure 1A-E. Multipliers added to figure, are highlighting the longitudinal elevation found for biomarkers. Aβ42, Amyloid-β 42; T-tau, total tau; P-tau, tau phosphorylated at threonine 181; NFL, neurofilament light; NRGN, neurogranin.
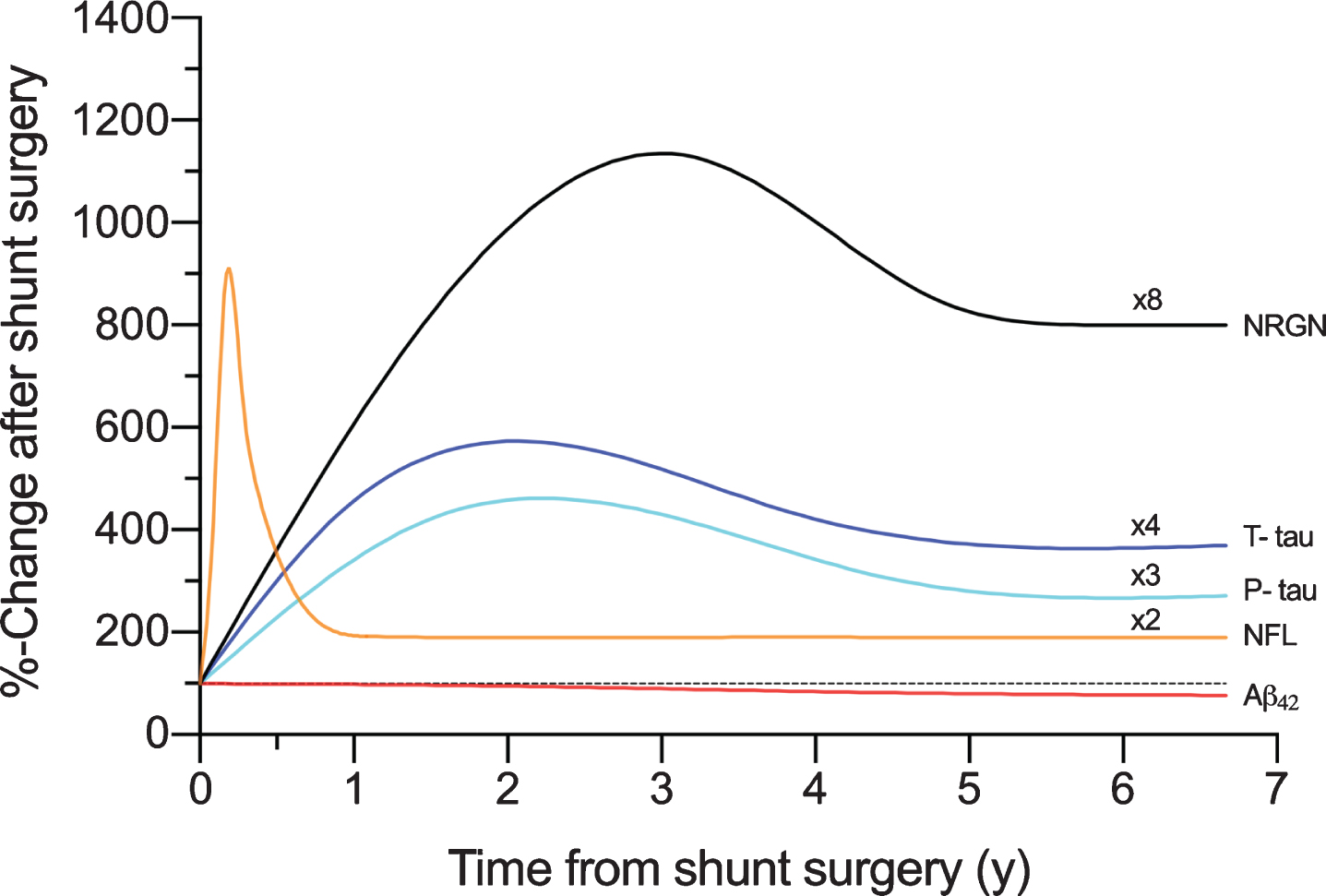
The reason for the sustained increase in T-tau, P-tau, and NRGN after surgery and the NFL decrease toward baseline levels over time remains unclear but may represent the disease process of iNPH or change in CSF flow due to shunt. For AD patients, T-tau and P-tau has been shown to increase over time for 2% per year [34], which may indicate the disease process. In traumatic brain injury (TBI), T-tau levels were shown to already decrease toward baseline levels 20–43 days after the injury [35, 36], while a study in amateur boxers showed that both T-tau and P-tau levels normalized 3 months after brain injury [37]. With NFL, the study from amateur boxers showed that after acute upregulation during the first days after TBI, NFL levels had normalized towards baseline levels in 80% of boxers after 2 weeks [36]. In 20% of boxers, however, NFL levels remained significantly upregulated or were even increased after two weeks compared to the control group and this was postulated to reflect continued sports-related mild TBI [36]. When taking into account the correlation with time delay from surgery to the follow-up CSF sampling, the increase in NFL was probably rather related to timing than brain biopsy Aβ profile. If splitting up the group depending on the time delay prior to the first follow-up sample (early: from 3 to 9 M and late: over 9 M), NFL seems to reflect effects of the shunt surgery, i.e., that the temporary increase after CSF shunt, lasting up to 9 months, may at least partly represent the minor injury related with penetration of the brain in CSF shunt surgery.
The interesting correlation of the early NFL concentrations to T-tau, P-tau, and NRGN evokes question whether the T-tau, P-tau, and NRGN upregulation imply the longer lasting neuronal damage due to or despite of shunt surgery. We found no correlation between MMSE and the measured biomarkers, thus, the informative value of biomarkers about the state of cognitive functions with iNPH patients remains unclear. Whether this is explained by a sustained injury or a change of CSF clearance or flow dynamics because of the shunting, remains to be shown. Since iNPH seems to be somewhat progressive despite shunt treatment in a number of patients [2], biomarkers predicting long-term outcome would be valuable.
CSF T-tau is suggested by the NIA-AA research framework to be a biomarker of neurodegeneration or neuronal injury [38]. We show here that this may need to be combined with NFL or other biomarkers of neurodegeneration to assess treatment effects of disease-modifying therapy as levels of T-tau, P-tau, and NRGN may not decrease over time.
The traditional hypothesis of CSF flow has been challenged [39] and there are evidence that CSF movement is a local mixing and diffusion rather than unidirectional flow of production and absorption. Shunt treatment and the iNPH disease itself can change the CSF flow [40–43] and the composition of the CSF collected in this study.
Simultaneously collected repeated L- and V-CSF samples indicated that in all 5 biomarkers tested, the levels were around 14–32% lower in V- compared with L-CSF. The reason for this remains speculative but the result is in line with a previous report [6] showing lower levels of T-tau, Aβ40 and Aβ42 in V-CSF of iNPH patients. All samples from one individual were run on the same plate, and the absolute values of one individual patient for each particular time point correlated very highly. Thus, we think this cannot be attributed to a technical error in the measurement. A potential reason for the higher concentrations in L-CSF is the dominant diffusion to lumbar CSF due to the gravitation and this gradient effect might be amplified by high molecular weight.
In previous studies [44–46], V-CSF was shown to contain higher levels of T-tau or P-tau compared with L-CSF in iNPH patients, supporting the postulated theory of a concentration gradient of brain-derived proteins with higher levels in V-CSF. However, in these studies the levels were biased by the surgical procedure in V-CSF sampling that seems to have rather long-lasting effect on CSF biomarkers of brain injury [47]. In other studies presenting T-tau or P-tau levels higher in rostral compared with lumbar CSF [46, 48], a rostro-caudal gradient has been suggested. Consequently, the validity of tap test-collected large volume lumbar CSF that may be “contaminated” by V-CSF remains unclear. In addition, a study [49] presenting higher T-tau levels in cisternal CSF compared with L-CSF, had a patient population with trigeminal neuralgia or tension-type headache. In this study, with samples collected up to 3 months post-surgery using the shunt valve puncture, we provide a distinct approach for the gradient comparison but cannot determine the effect of CSF shunt on the gradient.
Current study confirm that shunt valve puncture is considered to be a safe and feasible option to obtain CSF samples from shunted iNPH patients. How-ever, V-CSF requires specific reference limits for diagnostic purpose since the biomarker values are systematically 14–32% lower than in L-CSF. The lumbar puncture success rate (78%) was notably low, which possibly could be explained by potential shrinkage of spinal dura sac [50] due to continuous CSF diversion [51]. The high correlation of biomarker concentrations in V- and L-CSF can be utilized to produce correction factors for specific biomarkers from intraventricular samples. Since the success rate of shunt valve puncture is good and the sampling procedure is easier to repeat, V-CSF analysis of shunted iNPH is a promising tool for biomarker diagnostics in the future.
We are aware that the total number of brain biopsy Aβ-negative iNPH patients is half of the biopsy-positive patients, which may have influence on our results. The other issue to consider is the finite number of iNPH patients in addition to the alternating participation to study visits and the limited success rate of lumbar sample collection. Furthermore, Aβ40 was analyzed only in the first and last time point. We also came by the challenge of variable delay from shunt surgery to the first follow-up sample collection. Especially with the biomarkers related to TBI, e.g., T-tau, P-tau, and NFL, we had to consider all possible explanations for the fluctuation. In addition, the tissue biopsy is rather small, only few cubic mm, and taken from the frontal cortex, thus AD-type pathology present in other areas of the brain could be missed. However, biopsy Aβ correlates well with autopsy [52] and amyloid PET [53].
The examined biomarkers correlated mostly as expected, both between the V-CSF and L-CSF and between other biomarkers. The understanding of the longitudinal behavior of biomarkers of neurodegeneration, including their diffusion between different compartments is important for the correct assessment of advantages and limitations of these biomarkers as biomarkers of disease progression.
In APOE ɛ4 carriers, lumbar Aβ42 was lower and showed a steep decrease after shunt insertion and thereafter a minor tendency to decrease while non–carriers showed milder decrease after shunt and thereafter a significant increase. This result is similar to longitudinal changes previously reported in AD patients [34] and may indicate activation of APOE-related clearance of Aβ by CSF shunt in iNPH patients. Surprisingly, no such APOE-related effect was seen in the increase of CSF P-tau. These intriguing preliminary findings motivate further study.
CONCLUSIONS
Longitudinal follow up shows that after initial upregulation post-surgery, T-tau, P-tau, and NRGN are stable in iNPH patients with or without Aβ pathology in brain biopsy, while NFL normalized towards its pre-shunt levels. Aβ42 instead showed divergent longitudinal decrease between brain biopsy Aβ-positive and -negative patients in L-CSF, and thereafter increase in biopsy-negative iNPH patients in L- and V-CSF. Thus, Aβ42 seems to be the biomarker that is the least affected by the surgical procedure or the presence of shunt and may be the best predictor of AD risk in iNPH patients. The concentration of all biomarkers measured 14–32% lower in V- than L-CSF yet showing strong correlations between the two sample types.
ACKNOWLEDGMENTS
We would like to acknowledge Marita Parviainen, RN, for assistance and cognitive testing. The study was funded by Janssen R&D, a division of Janssen Pharmaceutica NV, Kuopio University Hospital VTR (#5252614) fund and Sigrid Juselius Foundation. HZ is a Wallenberg Scholar supported by grants from the Swedish Research Council (#2018-02532), the European Research Council (#681712), Swedish State Support for Clinical Research (#ALFGBG-720931), the Alzheimer Drug Discovery Foundation (ADDF), USA (#201809-2016862), and the UK Dementia Research Institute at UCL. KB is supported by the Swedish Research Council (#2017-00915), the Alz-heimer Drug Discovery Foundation (ADDF), USA (#RDAPB-201809-2016615), the Swedish Alzhei-mer Foundation (#AF-742881), Hjärnfonden, Sweden (#FO2017-0243), the Swedish state under the agreement between the Swedish government and the County Councils, the ALF-agreement (#ALFGBG-715986), and European Union Joint Program for Neurodegenerative Disorders (JPND2019-466-236).
Authors’ disclosures available online (https://www.j-alz.com/manuscript-disclosures/20-1361r2).
SUPPLEMENTARY MATERIAL
[1] The supplementary material is available in the electronic version of this article: https://dx.doi.org/10.3233/JAD-201361.
REFERENCES
[1] | Shen DD , Artru AA , Adkison KK ((2004) ) Principles and applicability of CSF sampling for the assessment of CNS drug delivery and pharmacodynamics. Adv Drug Deliv Rev 56: , 1825–1857. |
[2] | Koivisto AM , Alafuzoff I , Savolainen S , Sutela A , Rummukainen J , Kurki M , Jääskeläinen JE , Soininen H , Rinne J , Leinonen V ((2013) ) Poor cognitive outcome in shunt-responsive idiopathic normal pressure hydrocephalus. Neurosurgery 72: , 1–8. |
[3] | Relkin N , Marmarou A , Klinge P , Bergsneider M , Black PM ((2005) ) Diagnosing idiopathic normal-pressure hydrocephalus. Neurosurgery 57: (3 Suppl), S4–16; discussion ii-v. |
[4] | Klinge P , Hellström P , Tans J , Wikkelsø C ((2012) ) One-year outcome in the European multicentre study on iNPH. Acta Neurol Scand 126: , 145–153. |
[5] | Leinonen V , Koivisto AM , Savolainen S , Rummukainen J , Tamminen JN , Tillgren T , Vainikka S , Pyykkö OT , Mölsä J , Fraunberg M , Jääskeläinen JE , Soininen H , Rinne J , Alafuzoff I ((2010) ) Amyloid and tau proteins in cortical brain biopsy and Alzheimer’s disease. Ann Neurol 68: , 446–453. |
[6] | Jeppsson A , Zetterberg H , Blennow K , Wikkelsø C ((2013) ) Idiopathic normal-pressure hydrocephalus Pathophysiology and diagnosis by CSF biomarkers. Neurology 80: , 1385–1392. |
[7] | Jingami N , Asada-Utsugi M , Uemura K , Noto R , Takahashi M , Ozaki A , Kihara T , Kageyama T , Takahashi R , Shimohama S , Kinoshita A ((2015) ) Idiopathic normal pressure hydrocephalus has a different cerebrospinal fluid biomarker profile from Alzheimer’s disease. J Alzheimers Dis 45: , 109–115. |
[8] | Seppälä TT , Koivisto AM , Hartikainen P , Helisalmi S , Soininen H , Herukka SK ((2011) ) Longitudinal changes of CSF biomarkers in Alzheimer’s disease. J Alzheimers Dis 25: , 583–594. |
[9] | Blennow K , de Leon MJ , Zetterberg H ((2006) ) Alzheimer’s disease. Lancet 368: , 387–403. |
[10] | Kapaki EN , Paraskevas GP , Tzerakis NG , Sfagos C , Seretis A , Kararizou E , Vassilopoulos D ((2007) ) Cerebrospinal fluid tau, phospho-tau181 and β-amyloid 1-42 in idiopathic normal pressure hydrocephalus: A discrimination from Alzheimer’s disease. Eur J Neurol 14: , 168–173. |
[11] | Olsson B , Lautner R , Andreasson U , Öhrfelt A , Portelius E , Bjerke M , Hölttä M , Rosén C , Olsson C , Strobel G , Wu E , Dakin K , Petzold M , Blennow K , Zetterberg H ((2016) ) CSF and blood biomarkers for the diagnosis of Alzheimer’s disease: A systematic review and meta-analysis. Lancet Neurol 15: , 673–684. |
[12] | Zetterberg H , Blennow K ((2018) ) From cerebrospinal fluid to blood: The third wave of fluid biomarkers for Alzheimer’s disease. J Alzheimers Dis 64: , S271–S279. |
[13] | Maia LF , Kaeser SA , Reichwald J , Hruscha M , Martus P , Staufenbiel M , Jucker M ((2013) ) Changes in amyloid-β and tau in the cerebrospinal fluid of transgenic mice overexpressing amyloid precursor protein. Sci Transl Med 5: , 194re2. |
[14] | Schelle J , Häsler LM , Göpfert JC , Joos TO , Vanderstichele H , Stoops E , Mandelkow EM , Neumann U , Shimshek DR , Staufenbiel M , Jucker M , Kaeser SA ((2017) ) Prevention of tau increase in cerebrospinal fluid of APP transgenic mice suggests downstream effect of BACE1 inhibition. Alzheimers Dement 13: , 701–709. |
[15] | Skillbäck T , Rosén C , Asztely F , Mattsson N , Blennow K , Zetterberg H ((2014) ) Diagnostic performance of cerebrospinal fluid total tau and phosphorylated tau in Creutzfeldt-Jakob disease: Results from the Swedish Mortality Registry. JAMA Neurol 71: , 476–483. |
[16] | Hesse C , Rosengren L , Andreasen N , Davidsson P , Vanderstichele H , Vanmechelen E , Blennow K ((2001) ) Transient increase in total tau but not phospho-tau in human cerebrospinal fluid after acute stroke. Neurosci Lett 297: , 187–190. |
[17] | Janelidze S , Stomrud E , Smith R , Palmqvist S , Mattsson N , Airey DC , Proctor NK , Chai X , Shcherbinin S , Sims JR , Triana-Baltzer G , Theunis C , Slemmon R , Mercken M , Kolb H , Dage JL , Hansson O ((2020) ) Cerebrospinal fluid p-tau217 performs better than p-tau181 as a biomarker of Alzheimer’s disease. Nat Commun 11: , 1683. |
[18] | Remnestål J , Just D , Mitsios N , Fredolini C , Mulder J , Schwenk JM , Uhlén M , Kultima K , Ingelsson M , Kilander L , Lannfelt L , Svenningsson P , Nellgård B , Zetterberg H , Blennow K , Nilsson P , Häggmark-Månberg A ((2016) ) CSF profiling of the human brain enriched proteome reveals associations of neuromodulin and neurogranin to Alzheimer’s disease. Proteomics Clin Appl 10: , 1242–1253. |
[19] | Kvartsberg H , Duits FH , Ingelsson M , Andreasen N , Öhrfelt A , Andersson K , Brinkmalm G , Lannfelt L , Minthon L , Hansson O , Andreasson U , Teunissen CE , Scheltens P , Van Der Flier WM , Zetterberg H , Portelius E , Blennow K ((2015) ) Cerebrospinal fluid levels of the synaptic protein neurogranin correlates with cognitive decline in prodromal Alzheimer’s disease. Alzheimers Dement 11: , 1180–1190. |
[20] | Kvartsberg H , Portelius E , Andreasson U , Brinkmalm G , Hellwig K , Lelental N , Kornhuber J , Hansson O , Minthon L , Spitzer P , Maler JM , Zetterberg H , Blennow K , Lewczuk P ((2015) ) Characterization of the postsynaptic protein neurogranin in paired cerebrospinal fluid and plasma samples from Alzheimer’s disease patients and healthy controls. Alzheimers Res Ther 7: , 40. |
[21] | Kester MI , Teunissen CE , Crimmins DL , Herries EM , Ladenson JKH , Scheltens P , Van Der Flier WM , Morris JC , Holtzman DM , Fagan AM ((2015) ) Neurogranin as a cerebrospinal fluid biomarker for synaptic loss in symptomatic Alzheimer disease. JAMA Neurol 72: , 1275–1280. |
[22] | Wellington H , Paterson RW , Suárez-González A , Poole T , Frost C , Sjöbom U , Slattery CF , Magdalinou NK , Lehmann M , Portelius E , Fox NC , Blennow K , Zetterberg H , Schott JM ((2018) ) CSF neurogranin or tau distinguish typical and atypical Alzheimer disease. Ann Clin Transl Neurol 5: , 162–171. |
[23] | Wellington H , Paterson RW , Portelius E , Törnqvist U , Magdalinou N , Fox NC , Blennow K , Schott JM , Zetterberg H ((2016) ) Increased CSF neurogranin concentration is specific to Alzheimer disease. Neurology 86: , 829–835. |
[24] | Portelius E , Olsson B , Höglund K , Cullen NC , Kvartsberg H , Andreasson U , Zetterberg H , Sandelius Å , Shaw LM , Lee VMY , Irwin DJ , Grossman M , Weintraub D , Chen-Plotkin A , Wolk DA , McCluskey L , Elman L , McBride J , Toledo JB , Trojanowski JQ , Blennow K ((2018) ) Cerebrospinal fluid neurogranin concentration in neurodegeneration: Relation to clinical phenotypes and neuropathology. Acta Neuropathol 136: , 363–376. |
[25] | Casaletto KB , Elahi FM , Bettcher BM , Neuhaus J , Bendlin BB , Asthana S , Johnson SC , Yaffe K , Carlsson C , Blennow K , Zetterberg H , Kramer JH ((2017) ) Neurogranin, a synaptic protein, is associated with memory independent of Alzheimer biomarkers. Neurology 89: , 1782–1788. |
[26] | Portelius E , Zetterberg H , Skillbäck T , Törnqvist U , Andreasson U , Trojanowski JQ , Weiner MW , Shaw LM , Mattsson N , Blennow K ((2015) ) Cerebrospinal fluid neurogranin: Relation to cognition and neurodegeneration in Alzheimer’s disease. Brain 138: , 3373–3385. |
[27] | Bridel C , Van Wieringen WN , Zetterberg H , Tijms BM , Teunissen CE , Alvarez-Cermeño JC , Andreasson U , Axelsson M , Bäckström DC , Bartos A , Bjerke M , Blennow K , Boxer A , Brundin L , Burman J , Christensen T , Fialová L , Forsgren L , Frederiksen JL , Gisslén M , Gray E , Gunnarsson M , Hall S , Hansson O , Herbert MK , Jakobsson J , Jessen-Krut J , Janelidze S , Johannsson G , Jonsson M , Kappos L , Khademi M , Khalil M , Kuhle J , Landén M , Leinonen V , Logroscino G , Lu CH , Lycke J , Magdalinou NK , Malaspina A , Mattsson N , Meeter LH , Mehta SR , Modvig S , Olsson T , Paterson RW , Pérez-Santiago J , Piehl F , Pijnenburg YAL , Pyykkö OT , Ragnarsson O , Rojas JC , Romme Christensen J , Sandberg L , Scherling CS , Schott JM , Sellebjerg FT , Simone IL , Skillbäck T , Stilund M , Sundström P , Svenningsson A , Tortelli R , Tortorella C , Trentini A , Troiano M , Turner MR , Van Swieten JC , Vågberg M , Verbeek MM , Villar LM , Visser PJ , Wallin A , Weiss A , Wikkelsø C , Wild EJ ((2019) ) Diagnostic value of cerebrospinal fluid neurofilament light protein in neurology: A systematic review and meta-analysis. JAMA Neurol 76: , 1035–1048. |
[28] | Mattsson N , Insel PS , Palmqvist S , Portelius E , Zetterberg H ((2016) ) Neurofilament light in Alzheimer’s disease. EMBO Mol Med 8: , 1184–1196. |
[29] | Junkkari A , Luikku AJ , Danner N , Jyrkkänen HK , Rauramaa T , Korhonen VE , Koivisto AM , Nerg O , Kojoukhova M , Huttunen TJ , Jääskeläinen JE , Leinonen V ((2019) ) The Kuopio idiopathic normal pressure hydrocephalus protocol: Initial outcome of 175 patients. Fluids Barriers CNS 16: , 21. |
[30] | Luikku AJ , Hall A , Nerg O , Koivisto AM , Hiltunen M , Helisalmi S , Herukka SK , Junkkari A , Sutela A , Kojoukhova M , Korhonen V , Mattila J , Lötjönen J , Rummukainen J , Alafuzoff I , Jääskeläinen JE , Remes AM , Solomon A , Kivipelto M , Soininen H , Rauramaa T , Leinonen V ((2019) ) Predicting development of Alzheimer’s disease in patients with shunted idiopathic normal pressure hydrocephalus. J Alzheimers Dis 71: , 1233–1243. |
[31] | Pyykkö OT , Helisalmi S , Koivisto AM , Mölsä JAA , Rummukainen J , Nerg O , Alafuzoff I , Savolainen S , Soininen H , Jääskeläinen JE , Rinne J , Leinonen V , Hiltunen M ((2012) ) APOE4 predicts amyloid-β in cortical brain biopsy but not idiopathic normal pressure hydrocephalus. J Neurol Neurosurg Psychiatry 83: , 1119–1124. |
[32] | Gaetani L , Höglund K , Parnetti L , Pujol-Calderon F , Becker B , Eusebi P , Sarchielli P , Calabresi P , Di Filippo M , Zetterberg H , Blennow K ((2018) ) A new enzyme-linked immunosorbent assay for neurofilament light in cerebrospinal fluid: Analytical validation and clinical evaluation. Alzheimers Res Ther 10: , 8. |
[33] | Zetterberg H , Andreasson U , Hansson O , Wu G , Sankaranarayanan S , Andersson ME , Buchhave P , Londos E , Umek RM , Minthon L , Simon AJ , Blennow K ((2008) ) Elevated cerebrospinal fluid BACE1 activity in incipient Alzheimer disease. Arch Neurol 65: , 1102–1107. |
[34] | Lleó A , Alcolea D , Martínez-Lage P , Scheltens P , Parnetti L , Poirier J , Simonsen AH , Verbeek MM , Rosa-Neto P , Slot RER , Tainta M , Izaguirre A , Reijs BLR , Farotti L , Tsolaki M , Vandenbergue R , Freund-Levi Y , Verhey FRJ , Clarimón J , Fortea J , Frolich L , Santana I , Molinuevo JL , Lehmann S , Visser PJ , Teunissen CE , Zetterberg H , Blennow K ((2019) ) Longitudinal cerebrospinal fluid biomarker trajectories along the Alzheimer’s disease continuum in the BIOMARKAPD study. Alzheimers Dement 15: , 742–753. |
[35] | Franz G , Beer R , Kampfl A , Engelhardt K , Schmutzhard E , Ulmer H , Deisenhammer F ((2003) ) Amyloid beta 1-42 and tau in cerebrospinal fluid after severe traumatic brain injury. Neurology 60: , 1457–1461. |
[36] | Neselius S , Brisby H , Theodorsson A , Blennow K , Zetterberg H , Marcusson J ((2012) ) CSF-biomarkers in Olympic boxing: Diagnosis and effects of repetitive head trauma. PLoS One 7: , e33606. |
[37] | Zetterberg H , Hietala MA , Jonsson M , Andreasen N , Styrud E , Karlsson I , Edman Ä , Popa C , Rasulzada A , Wahlund LO , Mehta PD , Rosengren L , Blennow K , Wallin A ((2006) ) Neurochemical aftermath of amateur boxing. Arch Neurol 63: , 1277–1280. |
[38] | Jack CR , Bennett DA , Blennow K , Carrillo MC , Dunn B , Haeberlein SB , Holtzman DM , Jagust W , Jessen F , Karlawish J , Liu E , Molinuevo JL , Montine T , Phelps C , Rankin KP , Rowe CC , Scheltens P , Siemers E , Snyder HM , Sperling R , Elliott C , Masliah E , Ryan L , Silverberg N ((2018) ) NIA-AA Research Framework: Toward a biological definition of Alzheimer’s disease. Alzheimers Dement 14: , 535–562. |
[39] | Miyajima M , Arai H ((2015) ) Evaluation of the production and absorption of cerebrospinal fluid. Neurol Med Chir (Tokyo) 55: , 647–656. |
[40] | Graff-Radford NR ((2014) ) Alzheimer CSF biomarkers may be misleading in normal-pressure hydrocephalus. Neurology 83: , 1573–1575. |
[41] | Eide PK , Valnes LM , Pripp AH , Mardal KA , Ringstad G ((2020) ) Delayed clearance of cerebrospinal fluid tracer from choroid plexus in idiopathic normal pressure hydrocephalus. J Cereb Blood Flow Metab 40: , 1849–1858. |
[42] | Eide PK , Hansson HA ((2020) ) Blood-brain barrier leakage of blood proteins in idiopathic normal pressure hydrocephalus. Brain Res 1727: , 146547. |
[43] | Eide PK , Ringstad G ((2019) ) Delayed clearance of cerebrospinal fluid tracer from entorhinal cortex in idiopathic normal pressure hydrocephalus: A glymphatic magnetic resonance imaging study. J Cereb Blood Flow Metab 39: , 1355–1368. |
[44] | Pyykkö OT , Lumela M , Rummukainen J , Nerg O , Seppälä TT , Herukka SK , Koivisto AM , Alafuzoff I , Puli L , Savolainen S , Soininen H , Jääskeläinen JE , Hiltunen M , Zetterberg H , Leinonen V ((2014) ) Cerebrospinal fluid biomarker and brain biopsy findings in idiopathic normal pressure hydrocephalus. PLoS One 9: , e91974. |
[45] | Brandner S , Thaler C , Lelental N , Buchfelder M , Kleindienst A , Maler JM , Kornhuber J , Lewczuk P ((2014) ) Ventricular and lumbar cerebrospinal fluid concentrations of Alzheimer’s disease biomarkers in patients with normal pressure hydrocephalus and posttraumatic hydrocephalus. J Alzheimers Dis 41: , 1057–1062. |
[46] | Jingami N , Uemura K , Asada-Utsugi M , Kuzuya A , Yamada S , Ishikawa M , Kawahara T , Iwasaki T , Atsuchi M , Takahashi R , Kinoshita A ((2019) ) Two-point dynamic observation of Alzheimer’s disease cerebrospinal fluid biomarkers in idiopathic normal pressure hydrocephalus. J Alzheimers Dis 72: , 271–277. |
[47] | Kruse A , Cesarini KG , Bach FW , Persson L ((1991) ) Increases of Neuron-specific enolase, S-100 Protein, creatine kinase and creatine kinase BB isoenzyme in CSF following intraventricular catheter implantation. Acta Neurochir (Wien) 110: , 106–109. |
[48] | Djukic M , Spreer A , Lange P , Bunkowski S , Wiltfang J , Nau R ((2016) ) Small cisterno-lumbar gradient of phosphorylated Tau protein in geriatric patients with suspected normal pressure hydrocephalus. Fluids Barriers CNS 13: , 15. |
[49] | Tarnaris A , Toma AK , Chapman MD , Petzold A , Keir G , Kitchen ND , Watkins LD ((2011) ) Rostrocaudal dynamics of CSF biomarkers. Neurochem Res 36: , 528–532. |
[50] | Radoš M , Klarica M , Mučić-Pucić B , Nikić I , Raguž M , Galkowski V , Mandić D , Orešković D ((2014) ) Volumetric analysis of cerebrospinal fluid and brain parenchyma in a patient with hydranencephaly and macrocephaly - Case report. Croat Med J 55: , 388–393. |
[51] | Edsbagge M , Andreasson U , Ambarki K , Wikkelso C , Eklund A , Blennow K , Zetterberg H , Tullberg M ((2017) ) Alzheimer’s disease-associated cerebrospinal fluid (CSF) biomarkers do not correlate with CSF volumes or CSF production rate. J Alzheimers Dis 58: , 821–828. |
[52] | Leinonen V , Koivisto AM , Savolainen S , Rummukainen J , Sutela A , Vanninen R , Jääskeläinen J E , Soininen H , Alafuzoff I ((2012) ) Post-mortem findings in 10 patients with presumed normal pressure hydrocephalus and review of the literature. Neuropathol Appl Neurobiol 38: , 72–86. |
[53] | Rinne JO , Wong DF , Wolk DA , Leinonen V , Arnold SE , Buckley C , Smith A , McLain R , Sherwin PF , Farrar G , Kailajärvi M , Grachev ID ((2012) ) Flutemetamol PET imaging and cortical biopsy histopathology for fibrillar amyloid β detection in living subjects with normal pressure hydrocephalus: Pooled analysis of four studies. Acta Neuropathol 124: , 833–845. |