Multiple System Atrophy: An Oligodendroglioneural Synucleinopathy1
Abstract
Multiple system atrophy (MSA) is an orphan, fatal, adult-onset neurodegenerative disorder of uncertain etiology that is clinically characterized by various combinations of parkinsonism, cerebellar, autonomic, and motor dysfunction. MSA is an α-synucleinopathy with specific glioneuronal degeneration involving striatonigral, olivopontocerebellar, and autonomic nervous systems but also other parts of the central and peripheral nervous systems. The major clinical variants correlate with the morphologic phenotypes of striatonigral degeneration (MSA-P) and olivopontocerebellar atrophy (MSA-C). While our knowledge of the molecular pathogenesis of this devastating disease is still incomplete, updated consensus criteria and combined fluid and imaging biomarkers have increased its diagnostic accuracy. The neuropathologic hallmark of this unique proteinopathy is the deposition of aberrant α-synuclein in both glia (mainly oligodendroglia) and neurons forming glial and neuronal cytoplasmic inclusions that cause cell dysfunction and demise. In addition, there is widespread demyelination, the pathogenesis of which is not fully understood. The pathogenesis of MSA is characterized by propagation of misfolded α-synuclein from neurons to oligodendroglia and cell-to-cell spreading in a “prion-like” manner, oxidative stress, proteasomal and mitochondrial dysfunction, dysregulation of myelin lipids, decreased neurotrophic factors, neuroinflammation, and energy failure. The combination of these mechanisms finally results in a system-specific pattern of neurodegeneration and a multisystem involvement that are specific for MSA. Despite several pharmacological approaches in MSA models, addressing these pathogenic mechanisms, no effective neuroprotective nor disease-modifying therapeutic strategies are currently available. Multidisciplinary research to elucidate the genetic and molecular background of the deleterious cycle of noxious processes, to develop reliable biomarkers and targets for effective treatment of this hitherto incurable disorder is urgently needed.
INTRODUCTION
Multiple system atrophy (MSA) is a rare, rapidly progressing, fatal neurodegenerative disorder of uncertain etiology that is clinically characterized by a variable combination of parkinsonism, cerebellar impairment, autonomic and motor dysfunctions [1]. The term MSA was coined 1969 to pool previously described neurological entities: olivopontocerebellar atrophy (OPCA), the Shy-Drager syndrome, and striatonigral degeneration (SND) [2]. Together with Parkinson’s disease (PD) and dementia with Lewy bodies (DLB), MSA belongs to the group of α-synucleinopathies, which are morphologically characterized by abnormal accumulation of fibrillary α-synuclein (αS) [3–6]. αS inclusions in oligodendroglia are the recognized neuropathologic hallmarks of MSA [7] and may even represent a primary pathologic event [8]. Degeneration of multiple neuronal pathways over the course of the disease causes a multifaceted clinical picture. Depending on the predominant clinical phenotype, the disease is sub-classified into a parkinsonian variant (MSA-P) associated with SND, a cerebellar (MSA-C) variant with OPCA with predominant cerebellar features, and a combination of both forms, referred to as “mixed” MSA [9, 10]. The underlying molecular mechanisms are poorly understood, but converging evidence suggests a “prion-like” spreading of misfolded αS to represent a key event in the pathogenic cascade leading to systemic neurodegeneration of this oligodendroglioneuronal proteinopathy [8, 11–16]. Due to overlapping clinical presentations, the distinction between early stage MSA, PD, and atypical parkinsonian disorders (pure autonomic failure or adult-onset cerebellar ataxia) may be difficult [17–22].
Although symptomatic therapies are available for parkinsonism and autonomic failures, their response is often poor if not absent, and no effective disease-modifying therapies are currently available. However, due to remarkable progress in our understanding of the etiopathogenesis of MSA, novel therapeutic targets have emerged from preclinical studies and interventional trials [23]. The focus of the present review is the current knowledge on the neuropathology and etiopathogenesis of MSA.
EPIDEMIOLOGY AND NATURAL HISTORY
MSA is an orphan disease with an estimated incidence of 0.6–0.7 cases per 100,000 person-years, with a range of 0.1 to 3.0 cases per 100,000 person-years [24], whereas studies from Russia and Northern Sweden reported incidences of 0.1 and 2.4 per 100,000 person-years, respectively [25, 26]. Prevalence estimates range from 1.9 to 4.9 [27, 28] and may reach up to 7.8 after the age of 40 [29]. The incidence increases with age up to 12/100,000 above 70 years [30]. In the Western hemisphere, MSA-P involves about 70 to 80% [31], whereas MSA-C is more frequent in Asian populations accounting for about 67–84%, mixed phenotypes being more common [32–35], probably due to genetic or environmental factors [1, 6]. The motor symptom onset is 56±9 years, with both sexes equally affected [36]. However, like PD, 20 to 75% of MSA cases have a prodromal/preclinical phase with non-motor symptoms including cardiovascular autonomic failure, urogenital and sexual dysfunction, orthostatic hypotension, REM sleep behavior disorder, and respiratory disorders, which may precede the motor presentation by months to years [9, 37, 38]. Average age of onset is earlier in MSA-C compared to MSA-P [39, 40], the latter showing a trend to reach more disability [41]. The duration after clinical diagnosis is usually 6 to 10 (mean 9.5) years [23, 31, 42, 43], with few patients surviving more than 15 years [44, 45]. Mean survival is usually similar for both phenotypes [39, 45–47]. Others reported a mean survival time of 7.9 years for MSA-P, with a 5-year survival of 78% [48] or a 43% death rate during 3 years of follow-up [49]. A Pan-American multicenter study revealed that 68% of the participants presented with MSA-P, with an age at onset of 61.5 years and the others with MSA-C at 57.4 years [50]. A prospective cohort study in the US reported a median survival from symptom onset of 9.8 (95% CI 8.8–10.7) years [51]. A Japanese group found that patients with initial cerebellar ataxia had a better prognosis than those with initial parkinsonism or autonomic failure [40]. Early development of severe autonomic failure more than tripled the risk of shorter survival [52, 53]. A recent meta-analysis identified the following variables as unfavorable predictors of survival: severe dysautonomia, early development of combined autonomic and motor features, and early falls; conversely, MSA phenotypes and sex did not predict survival [54]. Among clinically diagnosed synucleinopathies with parkinsonism, MSA-P had the highest risk of death compared with reference patients [55].
ETIOLOGY AND GENETICS
The causes of MSA are unknown; however, as for other neurodegenerative diseases, a complex interaction of genetic and environmental mechanisms seems likely [56]. MSA is a predominantly sporadic disease and a family history of parkinsonism or ataxia is defined as a non-supporting feature in the current diagnostic criteria [9]. However, familial aggregation of parkinsonism has been observed in MSA [57, 58], and autopsy-proven familial cases have been reported. In a few pedigrees, the disease has been transmitted in an autosomal dominant or recessive inheritance pattern [44, 59–62]. Unlike PD, no single gene mutation linked to familial forms and no definite risk factors have been identified. A loss-of-function mutation in the COQ2 gene, encoding the coenzyme Q10 (COQ10)-synthesizing enzyme (4-hydroxybenzoate-polyprenyl transferase), was reported in Japanese familial and sporadic cases [63–66], the association being particularly strong for MSA-C [67–69]. A case of familial MSA was associated with compound heterocygous nonsense (R387X) and missense (V393A) mutations in COQ2 [70]. However, the link between COQ2 gene and MSA risk was not confirmed in other patient populations [64, 66, 71–74]. The COQ2 V393A polymorphism is associated with MSA in other Asian populations than Japanese [68, 75]. This implies that COQ2 polymorphisms are region-specific and do not represent common genetic factors for MSA. Decreased levels of COQ10 in plasma and cerebellum of MSA patients regardless of the COQ2 phenotype indicate impaired COQ biosynthesis which may contribute to the pathogenesis of MSA [76–79] through decreased electron transport in mitochondria and increased vulnerability to oxidative stress [65]. Changes of sequence TMEM230 gene have been reported in MSA patients in southwest China [80], while no differences were found in the genotype distribution and allele frequency of polymorphisms in VMAT2 and TMEM106B between MSA and controls [81]. A discordant loss of copy numbers of SHC 2 was found in monozygotic twins and Japanese patients with sporadic MSA, but not in the US [71, 82]. SNCA polymorphism (encoding αS and other loci), suggested to be associated with increased risks for MSA [83–85], was not confirmed in different patient cohorts [73, 83, 86–89]. Other studies showed that some SNCA polymorphisms are not likely a common cause of MSA in the Chinese population [90]. Two single-nucleotide polymorphisms of the SNCA locus showed a significant association with MSA in European patients [84], while no SNCA multiplications were seen in a series of 58 pathologically confirmed MSA cases [91]. A recent genome-wide association study did not detect any genome-wide significant association between tagged single nucleotide polymorphisms and MSA risk [87]. αS mRNA levels were comparable between MSA and controls, suggesting that αS expression is not the fundamental cause of MSA. A G51D SNCA mutation in British families was associated with autosomal dominant parkinsonism and neuropathologic findings comparable to both PD and MSA [92, 93], and a similar pathology was reported in a Finnish family with a novel αS mutation A53E [94]. The occurrence of glial cytoplasmic inclusion (GCI)-like oligodendroglial inclusions in familial PD due to SNCA mutations also suggests that both disorders form a continuum of αS pathology with related etiologies [95]. The same risk variants of the SNCA gene are also associated with risks for PD [96, 97], which indicates shared pathogenic mechanisms between these two synucleinopathies. On the other hand, there is little genetic evidence linking SNCA to MSA [98]. No SNCA mutations have been identified in true sporadic MSA, and no protein-changing Mendelian gene mutations have been identified in rare families [99]. Screening for PD causal genes (MAPT, PDYN, Parkin, PINK1, LRRK2) did not reveal any association with MSA [100–103], although MAPT H1 variation has been suggested to be associated with risk of MSA [104]. Contribution of LRRK2 exonic variants to susceptibility are under discussion [105, 106]. Gaucher-disease-associated glucocerebrosidase (GBA) variants were associated with MSA [107], but whether the GBA gene L444P mutation modifies the risk for MSA deserves further studies [108]. Among 108 autopsy-confirmed British MSA cases, one heterozygous GBA mutation (0.92%) versus 1.17% in controls was observed [109]. No association between other GBA mutations [110] nor with C9ORF expansions have been found [111, 112]. Mutations in the gene encoding the F-box only protein 7 (FBXO7) that immunochemically were detected in large proportions of αS positive inclusions (Lewy bodies (LBs), GCIs) were suggested to play a role in the pathogenesis of synucleinopathies [113], but no pathogenic mutations in FBXO7 among PD and MSA patients of Japanese or other ethnicities were observed [114]. Tumor necrosis factor (TNF-1031C) gene polymorphism was increased significantly in Japanese MSA patients compared with controls [115]. Other nucleotide polymorphisms (FBXO47, ELOVL7, and EDN1) were suggested as gene products, but none of the single polymorphisms reached genome-wide significance [116]. While rs75932628 triggering TREM2 was shown to increase the risk for AD, no association with the risk for MSA was observed [117]. A meta-analysis suggested that polymorphisms of the LINGO1 and LINGO2 (Nogo receptor-interacting protein-1 and – 2) decrease the risk of PD but not of MSA [118].
Other genes coding for apolipoprotein E, dopamine β-hydroxylase, ubiquitin, and leucine-rich kinase 2 showed no association with MSA [119], whereas polymorphism of several genes involved in inflammatory processes have been associated with elevated MSA risk, e.g., there was a fivefold risk to develop MSA with homozygosity for interleukin-1A allele, and the α-1-antichymotrypsin AA genotype (ACT-AA) is associated with an earlier onset and faster disease progression [120]. Another study showed association of MSA with polymorphisms of genes involved in oxidative stress [121]. A recent genome-wide study of a large MSA cohort and population-matched controls found an estimated heritability at 2.09–6.65%, which could be due to the presence of misdiagnosed cases in the cohorts and questions a common genetic background of MSA [87].
RNA analyses of MSA brain tissue have revealed alterations in a number of genes including α- and β-immunoglobuline [122], dysregulation of micro-RNAs (miRNA) resulting in downregulation of the carrier family SLC1A1 and SLC6, and dysregulation of miR-202 and miR-96 [123, 124]. miRNAs are small non-coding RNAs that regulate gene expression. miRNA expression profiles from formalin-fixed paraffin-embedded tissue revealed downregulation of miR-129-2-3p and miR-129-5p in the pons and cerebellum that was confirmed in frozen tissue from MSA patients [125]. Circulating miRNAs were differentially expressed [126]. Strand-specific RNA-sequencing analysis of MSA brain transcriptome showed disruption of long intervening non-coding RNAs (incRNAs) in the frontal cortex along with protein coding genes related to iron metabolism and immune response regulation indicating another level of complexity in transcriptional pathology of MSA [127, 128]. Analysis of the prion protein (PRNP) gene in MSA showed that the homozygous state of position 129 is not a risk factor for MSA and no other variants of the PRNP gene were associated with increased risk for MSA [100]. PRNP M129V homozygosity in MSA [129] was obviously not confirmed. However, other recent studies with MSA-derived αS aggregates have shown that they have a similar ability to undergo template-directed propagation, like PrP prions, and evidence is now emerging that αS aggregates can have different protein conformations, referred as strains, similar to what has been shown in prion disease [130]. These data suggest that αS becomes a prion in MSA, which supports its recent classification as a prion disease [13, 98], although there are challenges to the hypothesis that MSA is a prion disease.
A few epidemiological studies support the notion that epigenetic factors or environmental toxins may be associated with the risk of developing MSA [131, 132]. Due to limitations of environmental studies, there are no convincing data to correlate increased risk of MSA with occupational and daily habits such as exposure to solvents, pesticides, or other toxins like mercury or cyanide [133, 134]. An occupational story of farming related to higher MSA risk [134] could not be replicated [135–137]. Herbal medications have been shown to constitute an MSA risk factor for the Korean population [136]. A history of smoking was less frequent in MSA patients [138]. The association of alcohol consumption with MSA is under discussion [137]. Overall, no single occupational or environmental factor was shown to modify the disease risk.
In conclusion, all studies on the etiology of MSA suffer from limited numbers of cases as the disease is rare and frequently underdiagnosed, and differentiation from other parkinsonian syndromes is difficult, particularly in early disease stages. The definite diagnosis of MSA can only be made at postmortem examination, which, however, is missing in the majority of cases involved in genetic or epidemiological studies, contributing to their inconclusive results.
NEUROPATHOLOGY
Macroscopy
Naked eye inspection of the MSA brain may show mild diffuse cortical atrophy in the frontal lobes and significant atrophy of the cerebellum and pontine base. A few cases with severe frontal or temporal atrophy [139–141], and one case with asymmetrical temporal atrophy were reported [142]. Slicing the brain reveals atrophy and dark brownish discoloration of the posterolateral putamen due to deposition of lipofuscin, neuromelanin, and increased iron content in this area [143]. Pallor of substantia nigra (SN) and locus ceruleus (LC) are common, but without midbrain atrophy. MSA-C presents with various degrees of paleo- and neocerebellar atrophy, narrowing of the cerebellar folia, decrease and brown discoloration of the cerebellar white matter, the degeneration of which is more severe than of the cerebellar cortices [144]. The superior cerebellar peduncle and deep cerebellar nuclei are preserved. Severe atrophy of the pontine basis and middle cerebellar peduncle may be associated with reduction in size of the inferior olivary nucleus. The macroscopic changes in MSA-C cases may occasionally be difficult to distinguish from some spinocerebellar atrophias (SCA), especially SCA1 [145, 146].
Histopathology
The histological core features of MSA encompass four major types of different severity: (1) Specific αS immunoreactive inclusion pathology with four types of inclusions, i.e., GCIs within oligodendrocytes, also referred to as Papp-Lantos bodies [147], the presence of which is required for the postmortem diagnosis of definite MSA [7]. Less frequent are glial nuclear (GNI), neuronal cyotoplasmic (NCI), and neuronal nuclear inclusions (NNI), astroglial cytoplasmic inclusions and neuronal threads, also composed of αS [148]; (2) selective neuronal loss and axonal degeneration involving multiple regions of the nervous system with brunt on the striatonigral and OPC systems; (3) myelin degeneration with pallor and reduction in myelin basic protein (MBP), with accompanying astrogliosis; and (4) microglial activation [149]. GCIs and the resulting neurodegeneration occur in typical multisystemic distribution involving not only the striatonigral and OPC systems, but also autonomic nuclei of the brainstem (LC, nucleus raphe, dorsal vagal nuclei, etc.), spinal cord, sacral visceral pathways [132, 150, 151], and the peripheral nervous system [49, 152, 153], characterizing MSA as a multi-system/-organ disorder [86, 154, 155].
The degree of neuronal loss and cellular inclusions in different brain areas is related to the MSA motor subtype of SND and OPCA [156, 157]. Quantitative analyses of neuronal loss and GCI density showed a positive correlation between both lesions and an increase with disease duration [156, 158–160]. Region-specific astrogliosis is positively correlated with αS pathology in MSA in contrast to PD [161]. In general, the degree of astrogliosis parallels the severity of neurodegeneration [156]. Microglial activation in degenerating regions accompanies GCI pathology and is more abundant in white matter areas with mild to moderate demyelination [162]. In MSA-C, the cerebellar subcortical white matter and cerebellar brainstem projections are the earliest foci of αS pathology, followed by an involvement of other central nervous system (CNS) regions. The severity of GCIs correlated with demyelination, and loss of Purkinje cells increased with disease duration [163].
Inclusion pathology
The ultrastructure and biochemical composition of GCIs and other inclusions have been reviewed [4, 147, 149, 164–166]. Ultrastructurally, the GCIs are non-membrane bound cytoplasmic aggregates composed of loosely packed and coated or straight filaments 15–40 nm in diameter consisting of polymerized αS and filaments associated with granulated material related to cytoplasmic organelles such as mitochondria and secretory vesicles [167, 168]. NCIs consist of a meshwork of randomly arranged loosely packed granule-associated 18–28 nm filaments, similar to GCIs [167], while NNIs are composed of densely packed fibrillary structures forming bundles. Immuno-electron microscopy showed αS labelling of both granular and filamentous structures [169]. GCIs are characterized by aggregation of phosphorylated (Ser129) αS similar to LBs, forming the central core of the inclusion [147, 170]. In addition, they contain ubiquitin, and a number of other proteins such as tau, tubulin, heat shock, aggresomal and microtubule-associated proteins [171], synphilin, p25α (tubulin polymerization-promoting protein/TPPP, an OLG-specific phosphoprotein), oligodendroglial markers, p62 kinases, AMBRA1 (autophagy/beclin 1 regulator 1), MT-III metallothionein, etc. (see Table 1a,b). GCIs of MSA are both Campbell-Switzer- and Gallyas-Braak-positive, whereas LBs are negative for Gallyas-Braak stain [204]. Purification of αS containing inclusions revealed that GCIs consist of 11.9% αS, 2.8% α-β-crystallin, and 1.7% 14-3-3 protein compared to 8.5, 2.0 and 1.5% in LBs [205]. In early disease stages, diffuse αS staining in neuronal nuclei and cytoplasm occurs in many gray matter areas, suggesting that primary aggregation of nonfibrillary αS occurs in neurons [141]. In surviving striatal neurons as well as in GCI-containing oligodendrocytes of MSA patients, IRS-1pS312 staining was significantly increased, indicating their insulin resistance [206].
Table 1a
List of protein constituents and their major functions identified in glial
Protein identified by routine immunohistochemistry or mass spectrometry (MS+) | Main function / Cellular process | Reference |
α-Synuclein (MS+) (Syn 202, 205, 215 > SNL-4 > LB509 > Syn 208), (S129-P, S87-P) | Presynaptic vesicle release | [173, 174] |
β-Tubulin (MS+), α-Tubulin (MS+)b | Microtubule nucleation | [171] |
HDAC6 (histone deacetylase 6)b | Tubulin degradation | |
20S proteasome subunitsb | ||
p62/SQSTM1 (26 kDa protein/sequestosome 1)b | Autophagy | |
14-3-3 protein (in subset of GCIs) | Signal transduction | [175] |
Elk1 | Transcription factor | [176] |
Bcl-2 (MS+) | Apoptosis | |
P39 | CDK5-activator | [177] |
Carbonic anhydrase isoenzyme IIa (MS+) | ||
cdk-5 (cyclin-dependent kinase 5) (MS+) | Cell cycle regulation | [178] |
Midkinea | Neurotrophic factor | [179] |
τ2 (reversible on exposure to detergent) | Microtubule-associated | [180, 181] |
Isoform of 4-repeat tau protein (hypo-phosphorylated) (MS+) | Microtubule-associated | |
DARPP32 | Regulation of signal transduction | [182] |
Dorfin | Protein degradation | [183] |
Heat shock proteins Hsc70, Hsp70b Hsp90b (MS+) | Protein folding | |
DJ-1 | ||
LRRK2 | [184] | |
Rab5, Rabaptin-5 | Endocytosis regulation | [185] |
Parkin | [184] | |
Mitogen-activated protein kinase (MAPK) | Signal transduction | [178] |
NEDD-8 (MS+) | Protein degradation | |
Other microtubule-associated proteins (MAPs): MAP-1A and -1B; MAP-2 isoform 1, and isoform 4 (all MS+) | ||
Phosphoinositide 3-kinase (P13K) (MS+) | ||
p25α/TPPP (MS+) (tubulin polymerization-promoting protein) | ||
Septin-2, –3, –5, –6 and –9 | ||
Synphilin-1 | αS interaction protein (SNCAIP) | [186] |
Transferrina | ||
HtrA2/Omi | Apoptosis | [187] |
Ubiquitin (MS+) SUMO-1 (small ubiquitin modifier 1) | Protein degradation | |
Leu-7a | [188] | |
p62-co-localization with α-Syn (inconsistent) | [189] | |
AMBRA1 | Autophagy regulation | |
NBR1 - autophagic adapter protein | Autophagy | [190] |
Metallothionein-III (MT-III) | Metal binding | [191] |
α-β-Crystallin | Protein folding | [192] |
NUB-1 (negative regulator of ubiquitin-like protein 1) | Negative regulation of NEDD8 | [193, 194] |
Parkin co-regulated gene (PACRG) | Regulation of cell death | [195] |
Protein disulfide isomerase (PDI) | Protein folding | [196, 197] |
F-box only protein 7 (FBXO7) | Ubiquitination | [198] |
XIAP (x-linked inhibitor of apoptosis protein) | regulation of apoptosis | [199] |
Table 1b
Candidate proteins that have so far eluded detection by routine immunohistochemistry
Actin, γ-1, and γ-2 propeptides (MS+) |
Amyloid-β precursor protein (MS+) |
β-Synuclein (MS+) |
Cytokeratin |
Desmin |
Glial fibrillary acidic protein (GFAP) (MS+) |
Myelin basic protein (MBP)-3, –4, –5 (MS+) |
Myelin oligodendrocyte glycoprotein (MOG), α- and β-isoforms (MS+) |
Myosin (9 distinct isoforms) (MS+) |
Neurofilaments (NF-3, NF-HC, NF-LC) (MS+) |
Vimentin |
Distribution of lesions
GCIs occur in an anatomically selective manner and are widely distributed in cerebral gray and white matter, with highest densities in deeper laminae of motor cortex, dorsolateral putamen, globus pallidus, subthalamus, SNpc, pontine basal nuclei, motor nuclei of V, VII, and XII cranial nerves, pontomedullary reticular nuclei, cerebellum, intermediolateral column of the spinal cord, and preganglionic autonomic nerve structures [147]. In white matter, they are most numerous beneath the motor cortex, in external and internal capsule, corpus callosum, corticospinal tracts, and cerebellar white matter [207–209]. Accumulation of phosphorylated αS also occurs in subpial and periventricular astrocytes after long disease duration [210]. However, since αS-positive astrocytes in these regions also occur in LB disease, they may not be a specific feature of MSA [211]. Positive correlation between neuronal loss and the density of GCIs highlights their pivotal role for neuronal death [119, 166], while in the SN severe neuronal loss is associated with relatively low density of GCIs, indicating that certain areas are affected earlier in the disease course and have been burnt out [86]. Much less frequent are GNIs showing a similar distribution as GCIs, while the density of NCIs and NNIs is unrelated to that of GCIs [212]. NCIs seem to be more widespread than previously assumed and show a hierarchical pattern related with the duration of the disease, but independent of the pattern of neuronal destruction, suggesting that other factors may induce the subtype-dependent neuronal loss related to cognitive dysfunction [154].
Based on semiquantitative assessment of GCI density, neuronal loss, and gliosis, the striatonigral and OPC lesions were graded into four degrees of severity indicated by an SND+ OPCA score and related to both clinical key features and disease duration [213] (see Fig. 1). While this grading scale revealed a low correlation between both systems and the natural history of the disorder, a similar system showed an overlap between them [158]. Stereological studies of the basal ganglia in MSA revealed a substantial loss of neurons in SN, putamen, and globus pallidus (p < 0.01) and to a lesser extent in caudate nucleus (p < 0.03). A lower number of oligodendrocytes was only observed in putamen (p < 0.04) and globus pallidus (p < 0.01), whereas the number of astrocytes was higher in putamen (p < 0.04) and caudate nucleus (p < 0.01). Higher numbers of microglia were found in all examined regions with greatest difference in the otherwise unaffected red nucleus (p < 0.01). These data support the region-specific patterns of pathological changes in MSA [214]. Another neuropathological study showed that the striatonigral region was most severely affected in 34%, the OPC in 17%, while in almost half the cases both regions were equally affected [156]. These data differ from Japanese studies, where OPC pathology was greatest in up to 40% and 18% showed predominant striatonigral damage, reflecting the different phenotypical presentations among populations [32, 215]. In view of the frequent overlap and mixed forms, the value of grading systems to determine the evolution of MSA is under discussion [86, 96].
Fig.1
Schematic distribution of various combination types of SND and OPCA in 42 autopsy-proven cases of MSA (22 MS-P, 20 MS-C), showing different severity of morphological lesions (from [213]).
![Schematic distribution of various combination types of SND and OPCA in 42 autopsy-proven cases of MSA (22 MS-P, 20 MS-C), showing different severity of morphological lesions (from [213]).](https://ip.ios.semcs.net:443/media/jad/2018/62-3/jad-62-3-jad170397/jad-62-jad170397-g001.jpg)
Neurodegeneration with cell loss and gliosis in MSA not only involves the striatonigral and OPC systems, but affects many other parts of the central, autonomic, and peripheral nervous system underpinning the multisystem character of the disease [4, 86, 149, 154]. Consistently and severely affected areas are putamen, caudate nuclei, SN, pontine and medullary tegmental nuclei, inferior olive, and cerebellar white matter; moderately affected are motor cortex and globus pallidus; and mild lesions occur in cingulate cortex, hypothalamus, nucleus basalis Meynert, thalamus, subthalamus, and pontine tegmentum [216]. The posterior putamen is involved in early disease stages [217].
Although cortical involvement in MSA was considered rare in earlier studies, more robust methods showed around 20% reduction of neurons in motor and supplementary motor cortex [218]. Early degeneration of the basal ganglia drives late onset cortical atrophy [219]. Betz cell loss and astrocytosis in cerebral cortex have been described in proven MSA cases [220–223], degeneration of frontal and temporal neocortices affects more lower laminae than upper ones [224]. Stereological studies found significantly fewer neurons in frontal and parietal cortex of MSA brains compared with controls and significantly more astrocytes and microglia in frontal, parietal, and temporal cortex, whereas no change in the total number of oligodendrocytes was seen in any of the neocortical regions [225]. This indicates that the involvement of the neocortex is more widespread than previously thought. Neocortical neuronal loss was significantly more severe in MSA patients with impaired executive function and cognitive impairment [225–228], while recent MRI studies, although showing widespread cortical, subcortical, and white matter alterations, suggested only a marginal contribution of cortical pathology to cognitive deficits but more impact of focal fronto-striatal degeneration [229]. The presence of LB-like inclusions in neocortex [154], of frequent globular NCIs in the medial temporal region [230, 381] and in the perirhinal cortex without hippocampal involvement [231] were associated with cognitive or behavioral impairment, while others found no pathological differences between MSA cases with and without cognitive changes [232]. Although volumetric MRI analysis suggested hippocampal atrophy in MSA, little information on neuronal loss in hippocampus is available. Recent studies suggested that a greater burden of NCIs in the limbic regions is associated with CI in MSA [233].
Reduced neuronal numbers in the anterior olfactory nucleus and intrabulbar part of the primary olfactory (pyriform) cortex may underlay olfactory dysfunction in MSA [234], although this is less pronounced than in PD. Progressive retinal ganglion cell loss has been observed in MSA, both in vivo and by neuropathologic assessment [235–237]. More relative preservation of the temporal sector of the retinal nerve fiber layer and less severe atrophy of the macular ganglion cell layer complex, due to damaged large myelinated optic nerve fibers, differ from that in PD [238]. Retinal thinning worsens with disease progression and severity [235].
Demyelination and gliosis
Demyelination with variable intensity is frequent in MSA and mainly involves the striatonigral and OPC region, the external capsule and cerebellar white matter [162], in MSA-C the frontal and occipital white matter [239]. These changes are detected during lifetime by diffusion tensor imaging (DTI) MRI [240, 241], specifically in putamen and middle cerebellar peduncle [242–245], but no portion of the nervous system appears to be spared [144]. Demyelination is associated with reduction in myelin proteins including sphingomyelin, sulfatide, and galactoceramide by about 50% [246]. Whether myelin loss is a secondary event attributable to neuronal loss or a primary lesion, which in turn leads to neuronal and axonal loss, is unknown, but it also may be related to oligodendroglial dysfunction. Loss of oligodendroglial TPPP/p25α immunoreactivity correlated significantly with the degree of microglial reaction and loss of MBP density as a marker of tract degeneration [15]. White matter degeneration causes destruction of neuronal loops, leading to dysfunction of the whole-brain network [247], and may be related to disorders of cerebral autoregulation [248].
Gliosis is invariably described in the degenerating areas of MSA brain [156, 249]. In general, the degree of region-specific astrogliosis parallels the severity of neurodegeneration and correlates positively with αS pathology in MSA [162, 250] in contrast to PD [251]. Significantly increased monoaminoxidase B (MAO-B), a biomarker of astrogliosis, in degenerating putamen (+83%), was associated with astrogliosis, and positive correlation with αS accumulation, while less severe increase of MAO-B in SN (+10%) was positively related with that of membrane-bound αS. MAO-A decreased moderately only in atrophic MSA putamen (–27%) and was not changed in SN in PD, thus distinguishing astrocyte behavior in these disorders [252].
Microglial activation, accompanying αS pathology and phagocytosing degenerating myelin, is prominent in degenerating regions (putamen, pallidum, SN, pons, and prefrontal cortex) [253], in particular in white matter tracts that provide input to the cerebellum and extrapyramidal system [162, 254]. It can be visualized both histologically [254] and by in vivo PET imaging [255]. Activation of TRL4 and myeloperoxidase, a key enzyme for the production of reactive oxygen species in phagocytic cells, has been reported in activated MSA microglia [256–258]. A trend of increased M1 compared to M2 activation, identified by co-localization of TSPO with CD68 immunoreactivity [259], suggests that microglial activation is at least in part determined by oligodendroglial GCIs in affected areas. Stereological studies in the white matter revealed a significant increase of microglia (∼100%) without concomitant astrogliosis and absence of significant oligodendroglial degeneration [260]. In summary, there is evidence that microglia cells play an important role in the initiation of progression of MSA like in other neurodegenerative diseases [261, 262]. This is supported by transgenic mouse models indicating an active contribution of microglial activation to pathogenesis of the disease [263] by triggering neuroinflammatory responses in the MSA brain [264].
Lesions of the autonomic and peripheral neuronal system
Degenerative involvement of preganglionic autonomic neurons of the brainstem and spinal cord underlies the multidomain autonomic failure in MSA [49, 151, 265, 266]. The supraspinal lesion sites include the cholinergic neurons of the ventrolateral nucleus ambiguus [267, 268], pedunculo-pontine/laterodorsal tegmental nuclei [269], ventral periaqueductal DAergic neurons, which may contribute to excessive daytime sleepiness [270], the medullary arcuate nucleus [271], the noradrenergic LC [157], the serotonergic medullary groups (nucleus raphe magnus, obscurus, and pallidus) and ventrolateral medulla [53, 272], ventromedullary neurokinin-1 (NK-1) receptor immunoreactive neurons [273], the caudal raphe neurons with sparing of the rostral part [274, 275], the catecholaminergic neurons of the rostral ventrolateral medulla (C1 group), and noradrenergic neurons of the caudal ventrolateral medulla (A1 group) [267, 276]. Loss of A5 noradrenergic neurons was comparable to that in LC and pontine tegmentum [277]. The medullary catecholaminergic and serotonergic systems are involved even in the early stages of MSA, and dysfunction of the medullary serotonergic system could be responsible for sudden death [278]. Further involved areas are the dorsal vagal nucleus [267], the periaqueductal gray [150, 279], the Edinger-Westphal nucleus and posterior hypothalamus [280] including the histaminergic tuberomamillary neurons [281], the tuberomammillary nucleus [280, 282], and suprachiasmatic nucleus [267]. Affected is also the ponto-medullary reticular formation [160, 283], while the branchimotor neurons of the nucleus ambiguus are preserved [284]. Adrenergic neurons are more susceptible than serotonergic neurons. The density of αS did not correlate with neuronal loss (ranging from 47 to 70%) in any of these medullary areas and there was no correlation between αS burden and disease duration for any regions of interest, indicating that loss of monoaminergic neurons may progress independently from αS accumulation [285]. Mild degeneration of cardiac sympathetic nerves can occur in MSA, which accounts for mild to moderate decrease in the numbers of tyrosine hydroxylase but not of neurofilament-immunoreactive nerve fibers in the epicardium and for the slight decrease in cardiac uptake of 123Imetaiodobenzylguanidine (123IMIBG) by SPECT assessing postganglionic presynaptic nerve endings. However, depletion of cardiac sympathetic nerve is closely related to the presence of αS pathology in the sympathetic ganglia of the CNS [208, 286]. At lower levels of the autonomous nervous system lesions involve sympathetic preganglionic neurons in the intermediolateral cell column of the thoracolumbar spinal cord [157, 287], sympathetic ganglia, and Schwann cells in autonomic nerves [211]. Spinal cord pathology is further characterized by neuronal loss in the Onuf’s nucleus in the lumbosacral region [157, 288], and minor or rarely severe loss of upper and lower motor neurons [289, 290], while the involvement of anterior horn cells is under discussion [157, 291].
Involvement of the peripheral nervous system in MSA includes αS aggregates in sympathetic ganglia, skin nerve fibers [292, 293], and Schwann cells [293a], contrasting with axonal predominance of αS pathology in DLB [293b] which have also been described in MSA models but without functional deficits [153]. However, others showed lack of phosphorylated αS immunoreactivity in dermal fibers in contrast to PD [293, 294]. Filamentous aggregations of αS were found in the cytoplasm of Schwann cells in cranial, spinal, and autonomic nerves in MSA [49, 211, 295], and reduced sudomotor nerve density suggests pre- and postganglionic denervation [296, 296a].
αS pathology has been reported in the enteric nervous system [297].
Iron in MSA
Iron depositions in the putamen of MSA patients are a hallmark of the disease [298]. Unfortunately, there is unsatisfactory data about alterations of iron metabolism in MSA and the relevance of iron in the pathogenesis of this disorder. However, there is recent evidence of iron deficiency due to iron dysregulation in MSA indicating a deficit in bioavailable iron in MSA [299], whereas iron accumulation in MSA-P may be an epiphenomenon of the degenerative process [300]. To the best of our knowledge, no proteomic study of light chain ferritin levels have been performed in MSA, as in corticobasal degeneration and progressive supranuclear palsy (PSP) [301]. Iron can convert native αS into a β-sheet conformation and promotes/accelerates its aggregation either directly or via increasing levels of oxidative stress. Interestingly, αS has been identified to have a ferrireductase activity and an iron-regulated element on mRNA level, implying a direct interaction between iron and αS [302].
Postmortem analyses have revealed increased iron content and associated neuronal loss particularly in the putamen of MSA, while others observed it also in the SN, globus pallidus, and caudate nucleus of MSA patients [298, 303–306]. Although severe neuronal loss in LC was described, alterations in iron concentration have not been documented there [307]. There is evidence that iron content (Fe3 +) in globus pallidus and SN is more pronounced in MSA than in PD, DLB, and controls, being similar to the levels found in PSP [257, 307].
However, reduction in bioavailable iron was shown recently in MSA by a detailed postmortem analyses of human brain tissue (MSA, PD, and control) assessing iron, ferritin, transferrin receptor (TfR) and ferroportin distribution in pons, putamen, and SN [299]. In MSA, there are increased ferritin levels in pons (2.5-fold) and putamen (4.5-fold), an increase in iron stored within ferritin (2-fold) in pons and activated microglial cells with intense ferritin staining in SN and pons. Interestingly, the transporter ferroportin was decreased in MSA pons and putamen (0.75) in contrast to upregulation of ferroportin in SN in PD. Co-localization analyses of pons revealed selective expression of ferroportin in neurons and the Golgi apparatus which was observed in a filiform manner around the nucleus in PD and controls, while in MSA pons the ferroportin signal was weaker and more diffusely distributed—a pattern which is also seen in SN of PD patients. TfR expression was unchanged in pons and SN in PD, MSA, and controls, while putamen showed decreased TfR expression in PD compared to controls and MSA. The results of this study suggest that neurodegeneration is accompanied by region-specific differences in iron dysregulation which might be regarded as disease specific patterns in MSA, where limited iron export coupled with an increase in ferritin iron load results in decreased bioavailability of iron in MSA pons [302]. A dysregulation of iron export coupled with an increase in ferritin iron was detected to a lesser extent also in putamen. Although it is still unclear whether iron accumulation is rather a consequence and secondary event in the cascade of neuronal degeneration than a primary cause. Correlating the severity of putamen atrophy and iron accumulation suggests that iron accumulation is a secondary effect of neurodegeneration as significantly increased iron in the putamen is associated with advanced atrophy compared to moderate iron accumulation in globus pallidus along with less severe atrophy [302].
Subtypes of MSA
Pathological and clinical studies have shown that MSA has a wider range of presentations than previously thought, which expands the list of differential diagnoses. Several subtypes of MSA do not fit into the current classification [308]:
“Minimal change” MSA-P is a rare aggressive form with GCIs and neurodegeneration almost restricted to SN and putamen, thus representing “pure” SND [309–312], suggesting that GCI formation is an early event and may be responsible for some of the clinical symptoms of MSA. One patient with “minimal” MSA-C showed widespread GCIs with NCIs and NNIs restricted to pontine basis, cerebellar vermis and inferior olivary nuclei, associated with neuronal loss indicating a common link between both lesions in early stages of the disease [313]. Co-existence of “minimal changes” MSA with sporadic Creutzfeldt-Jakob disease was reported in a 64-year-old Spanish woman [314]. Postmortem detection of MSA pathology in neurologically normal individuals (prodromal/preclinical MSA) with GCIs limited to the pons and inferior olivary nuclei and mild neuronal loss restricted to SN is extremely rare [315, 316], suggesting that this region may be afflicted first in MSA-P. The presence of GCIs may represent an age-related phenomenon not necessarily processing to overt clinical disease, classifying these cases as “incidental MSA” similar to incidental Lewy body disease [317]. The other extreme are “benign” MSA cases with prolonged survival up to 15 years or more in 2-3% of MSA patients [43, 318]. Most of them showed a slowly progressing parkinsonism resembling PD in the first 10 years of disease with subsequent rapid deterioration after development of autonomic failure, before which correct diagnosis was difficult. Late onset of both cardiovascular autonomic and urinary voiding disorders were suggested to be responsible for prolonged survival in MSA irrespective of its subtype [319]. Many of these patients developed motor fluctuations and levodopa-induced choreiform dyskinesias, which would have indicated deep brain stimulation, not recommended for MSA patients [320, 321]. These rare long surviving patients with MSA-P were considered “benign” forms [43], whereas other cases with clinical course of 18 years revealed extensive distribution of GCIs in CNS [322]. A non-motor variant of pathologically confirmed MSA showed neither parkinsonism nor cerebellar symptoms [323]. Overlapping and distinguishing features of MSA and PD are summarized in Fig. 2.
Fig.2
Overlapping and distinguishing features of MSA and PD at the pathogenic, neuropathologic and clinical level (modified from [22]).
![Overlapping and distinguishing features of MSA and PD at the pathogenic, neuropathologic and clinical level (modified from [22]).](https://ip.ios.semcs.net:443/media/jad/2018/62-3/jad-62-3-jad170397/jad-62-jad170397-g002.jpg)
An atypical form of MSA with abundant αS inclusions was identified as frontotemporal lobe degeneration with αS (FTLD-synuclein) in the presence of SND and variable OPC degeneration, but in the absence of autonomic dysfunction [324, 325]. Another case of an MSA-P phenotype due to FTLD-TDP type A with severe striatal degeneration and mild cerebellar involvement was described recently [326]. Rare cases with pathologic hexanucleotide repeat expansion in C9ORF72, a gene linked to amyotrophic lateral sclerosis and FTLD, demonstrated clinical and neuroimaging features indistinguishable from MSA [327]. A rare cerebello-brainstem-dominant form of x-linked adrenoleukodystrophy should also be considered in the differential diagnosis of MSA [328]. These and other subtypes should be considered in establishing a correct diagnosis early in the course of MSA, which has implications for prognosis, selection of treatment and counseling of patients and their families.
Concomitant pathologies
Like other neurodegenerative disorders, many of which occurring in advanced age, various diseases are occasionally associated with MSA. The presence of LBs, the hallmark of PD and DLB, in MSA ranged from 10 to 23% [86, 329], whereas in Japanese MSA cases no concomitant Lewy pathology was found [215]. Extremely rare association of early stage MSA (striatonigral degeneration) with widespread LBs, referred to as “transitional variant” of PD and MSA [330], is of unknown significance. A synucleinopathy with features of both MSA and DLB was described [331]. Concomitant Alzheimer-like lesions in MSA are less frequent than in age-matched controls, mainly observed in old age [329], although recently MSA was reported in a male aged 69 years with pre-existing AD [332]. Studies of 139 MSA cases revealed chronic traumatic encephalopathy (CTE) pathology in 6% and aging-related tau astrogliopathy pathology [332a] in 8%. Since seven of 8 MSA/CTE cases had low Braak NFT stages (≤ III), age-related tau pathology would not be expected to make CTE pathology, and a small subset of these individuals had no history of contact sports or head trauma [333]. TDP-43 pathology, frequently observed in old brains, AD, certain forms of FTLD, and motor neuron disease [158], is generally rare in MSA, predominantly located in medio-temporal lobe and subcortical brain areas, suggested to represent an age-related “incidental” phenomenon [6], and no fused in sarcoma lesions were found in MSA brain [334]. An infantile MSA case linked to neuronal intranuclear myelin inclusion disease [335] appears questionable. Co-occurrence of MSA and PSP is very rare, with only four cases being reported in the literature [336]. The frequency of argyrophilic grains, a 4-repeat tauopathy, of approximately 20% in a Japanese MSA series [337], was similar to that reported in PSP [338]. The presence of unusual tau-positive cytoplasmic inclusions in astroglia of a few MSA brains, not co-localized with αS positive GCIs, suggested that tau may be related to a degenerative pathway different from that induced by αS [339]. The relevance of tau-positive (more 4-R than 3-R tau) inclusions in the astroglia in a single MSA brain [340] is unknown, although an interaction between different pathological proteins in neurodegenerative disorders suggests shared common pathogenic mechanisms [341–346].
There is still an open debate whether multiple sclerosis can cause parkinsonian symptoms or the co-existence of both diseases is accidental [347]. So far, only 45 cases of co-occurring parkinsonism and MSA have been reported, but CSF αS data are lacking [347, 348]. The association of MSA and multiple sclerosis has been reported only in two cases based on clinico-radiological and/or CSF findings [349, 350]. Despite essential differences in the neuropathology and etiopathogenesis of MSA and multiple sclerosis, and their rare co-occurrence, there is multiple pathogenic overlap between both disorders with similar basic mechanisms, resulting in chronic degeneration [155].
Clinical presentation
Parkinsonism, with rigidity, slowness of movements, postural instability, gait disability, and tendency to fall, characterizes the poorly levodopa-responsive motor presentation of MSA-P [23]. The motor findings are rarely asymmetrical [351]. Rest tremor is rare, whereas irregular postural and action tremor may occur [352, 353]. Cerebellar ataxia, wide-based gait, uncoordinated limb movements, action tremor, downbeat nystagmus, and hypometric saccades predominate in MSA-C [354]. Hyperreflexia and a Babinski sign may occur in 30–50% of patients, while abnormal postures, such as bent spine, antecollis, and hand or foot dystonia are rare [354]. Dysphonia, repeated falls, drooling, dysphagia, dystonia, and pain occur in advanced stages of the disease [355]. Spinal myoclonus in a MSA-C patient was caused by αS deposition in spinal cord [356]. Among non-motor symptoms, observed in 75–95% of patients [357], autonomic failure, in particular urogenital (urinary incontinence, impaired M. detrusor contractibility) and cardiovascular disorders, are frequent early features of MSA, but are not specific for this disease [353, 358, 359]. On the other hand, autonomic dysfunction may be the only presenting feature in some MSA patients [360]. Severe orthostatic hypotension is the main symptom of cardiovascular autonomic failure, often manifested as recurrent syncope, dizziness, nausea, headache, and weakness, but it is also seen in many other conditions [361]. Cardiovascular autonomic failure associated with degeneration of the nucleus ambiguus has been reproduced in the MSA mouse model [268]. Orthostatic hypotension usually occurs after the onset of genitourinary symptoms. Other non-motor features include constipation (in one third of the patients), vasomotor failure with diminished sweating (hypohydrosis) [362, 363], pupillomotor abnormalities and oculomotor dysfunctions [364]. Excessive daytime sleepiness shows a frequency similar to that encountered in PD [365, 366], and a similar frequency in Caucasian and Japanese MSA patients [367]. Gender differences were apparent for depression (women > men) and early autonomic failure (men > women) [357]. The prevalence of REM sleep behavior disorder, often preceding the onset of the motor disorders, is 88% or more [368, 369]. Restless legs syndrome is more prevalent in MSA as compared to the general population [370]. Respiratory disturbances including diurnal or nocturnal inspiratory stridor and sleep apnea are frequent and may occur together [371, 372], the latter representing a major cause of death in MSA [373, 374]. Reduced orexin (associated with sleep apnea syndrome) immunoreactivity has been observed in the nucleus basalis of Meynert in MSA [375].
Dementia and visual hallucinations, characteristic for DLB, are rare symptoms of MSA [9], although mild cognitive impairment [376, 377] and frontal-lobe dysfunction with attention and execution deficits or emotional incontinence driven by focal striatofrontal degeneration [229] do occur. Emotional and behavioral changes, including depression, anxiety, and panic attacks affect about one-third of MSA patients [354, 378, 379]. Applying the Movement Disorder Society diagnostic criteria for Parkinson disease-dementia, 11.7% of MSA patients were demented on level-2 examinations, executive dysfunction was seen in 52%, memory impairment in 15%, and language and visuospatial dysfunctions in 14% and 13%, respectively [380]. MSA-P and MSA-C were suggested to have different cognitive and mood profiles [381]. Cognitive deficits correlate with frontal atrophy and disease duration [382]. MSA is featured by a relentless worsening of the motor and non-motor symptoms, with more rapid progress at the onset [31]. The causes of death usually include (aspiration) bronchopneumonia, suffocation, or sudden death [31]. Older age at onset and early severe autonomic failure are negative prognostic factors, whereas a cerebellar phenotype and later onset of autonomic failure predict slower disease progression [1, 46, 52]. Because of its protean manifestations, MSA can be misdiagnosed, or disorders with other etiologies and pathologies can mimic MSA, especially at disease onset, as shown by a retrospective autopsy study in a cohort of patients with the clinical diagnosis of MSA, where only 62% were confirmed at autopsy [19]. Autonomic failure may be indistinguishable from pure autonomic failure or parkinsonism with autonomic failure. MSA-C patients presenting with late-onset cerebellar ataxia and additional autonomic failure can mimic genetic, toxic, or immune-mediated ataxias, spinocerebellar ataxia, or late-onset Friedreich’s ataxia [247]. MSA-C can be misdiagnosed as sporadic adult-onset cerebellar ataxia [383]. Conversely, patients with sporadic adult-onset cerebellar ataxia can be misdiagnosed as having MSA, when they develop urinary dysfunction or orthostatic hypotension [17]. SCAs, a group of autosomal dominant genetic disorders, is characterized by progressive degeneration of the cerebellum and its efferent and afferent connections, but a significant proportion of these patients have apparently sporadic cerebellar ataxia [384–386]. Some SCAs (2, 3, 6, and 17) may develop parkinsonism with nigrostriatal degeneration [387, 388], sometimes even without cerebellar dysfunction. They can be misdiagnosed as MSA-P when accompanied by autonomic failure, while others may mimic MSA-C [389]. A family with SCA1 triplet repeat expression and MSA-C-like clinical presentation [146] at postmortem showed multilocal neurodegeneration and sparse argyrophilic inclusions positive for tau and ubiquitin; but since αS immunochemistry was unavailable at that time, a definite diagnosis of MSA could not be made. SCA3 gene variants may also act as susceptibility factors for the development of MSA-C [390], whereas SCA6 is not commonly associated with MSA [120]. A prospective evaluation of 1,500 patients with progressive cerebellar ataxia identified 11% MSA-C cases [391]. Therefore, genetic testing for SCAs should be included in the diagnostic workup for MSA [392]. Other genetic disorders which can clinically mimic MSA include fragile X-associated ataxia syndrome, Perry syndrome, and other autosomal recessive cerebellar ataxias [18, 393, 394]. However, fragile X-associated tremor/ataxia syndrome is rare in MSA [395]. The accuracy of the clinical diagnosis of MSA is still unsatisfactory with a positive predictive value even in the later stages ranging from 60 to 90% [19, 396, 397], but the true rate of over- or under-diagnosis of MSA is not known. Various disorders which may mimic an MSA phenotype have been revised recently [23].
CLINICAL DIAGNOSTIC CRITERIA
Revised consensus guidelines define 3 degrees of certainty of the clinical diagnosis of MSA: definite, probable, and possible [9] (Fig. 3).
Fig.3
Diagnostic scheme for MSA according to the current consensus diagnostic criteria.
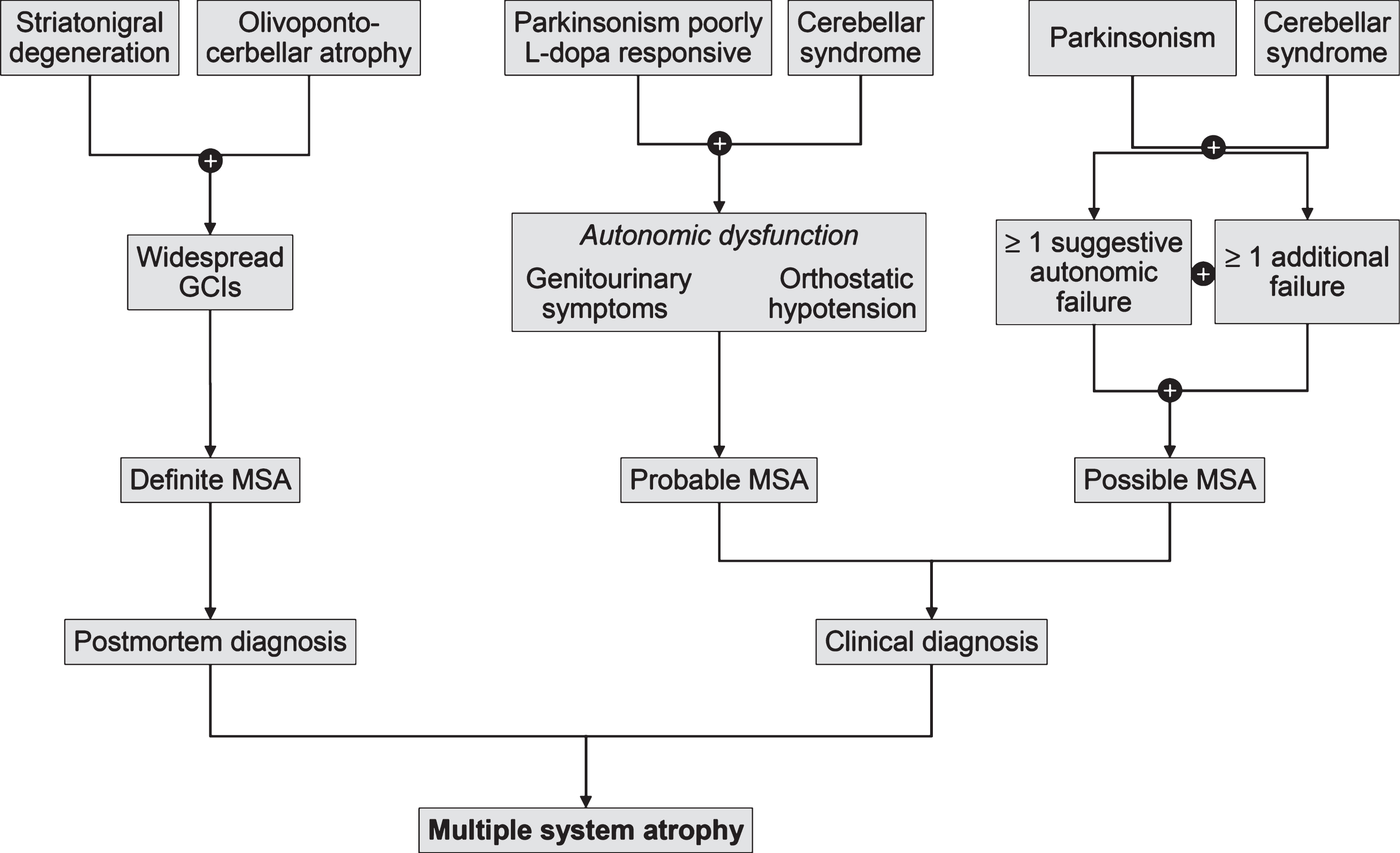
Definite MSA requires postmortem evidence of widespread αS-positive inclusions with concomitant SND or OPCA [7].
Probable MSA is defined as a sporadic, progressive disorder in adults, clinically characterized by severe autonomic failure, involve urinary dysfunction and poorly levodopa-responsive parkinsonism or cerebellar ataxia.
Possible MSA can be diagnosed, when a sporadic, progressive adult-onset disorder with parkinsonism or cerebellar ataxia is accompanied by at least one of the additional features suggesting autonomic or urogenital dysfunction plus one other clinical or neuroimaging abnormality. Recognition of patients with early or possible MSA may be supported by including one or more “red flags” (warning signs); two or more out of six red flags had a specificity of 98.3% and a sensitivity of 84.2% [353, 354]. Recent studies confirmed the validity and reliability of an eight-item pilot scale for the assessment of early MSA symptoms [398].
The revised consensus criteria regard dementia as a non-supportive feature of MSA [9]. However, recent evidence suggests that dementia occurs in up to 31% of MSA patients [399–401], indicating that the diagnosis of MSA cannot be excluded by the presence of dementia, although the molecular and structural correlates of cognitive decline are still unclear [232, 233, 400].
Biomarkers
No reliable diagnostic and prognostic fluid biomarkers are currently available, although many studies suggest that a combination of CSF biomarkers, such as DJ-1, phospho-tau, light chain neurofilament protein, and Aβ42 may be helpful in the differential diagnosis between MSA and other parkinsonian disorders [6, 402]. Oxidized DJ-1 protein levels in erythrocytes can be used as a marker for the differential diagnosis of PD and MSA [403]. The results of proteomics for biomarker discovery and miRNA expression need further evaluation [400a]. As cardiac sympathetic postganglionic denervation distinguishes PD from MSA patients showing intact innervation, 123IMIBG scan can help differentiation of the two disorders with a pooled specificity of 77% (95%, CI: 68–84%) [404]. Despite some overlap with PD (reduced 123IMIBG uptake), the presence of normal or only mildly reduced tracer uptake, supports the diagnosis of MSA-P [405, 406]. In patients with isolated autonomic failure, 123IMIBG myocardial scintigraphy may be a valuable predictor of conversion to MSA [407]. However, several interactions limit the value of this method [408]. Odor identification tests showing severe loss of smell may exclude MSA [409], separating PD from MSA with a sensitivity of 76.7% and a specificity of 95.7% [410].
MRI abnormalities including the “hot-cross bun” sign, a cruciform hyperintensity in the pons [411] and the “putaminal rim sign” that marks hyperintensity bordering the dorsolateral margin of the putamen in T2-weighted MRI reflecting degeneration and iron (Fe3 +) deposition may differentiate MSA-P from PD [412–417]. They are, however, non-specific signs and, therefore, not included in the recent consensus criteria [9], while putaminal atrophy shows 92.3% specificity but low sensitivity (44.4%) for distinguishing MSA-P from PD [418]. The combination of “swallow-tail” sign and putaminal hypointensity can increase the accuracy of discrimination between MSA and idiopathic PD [419]. Others showed significantly increased putaminal MD (mean diffusivity) volumes in the small anterior region of interest in MSA-P versus PD [420]. Another key distinguishing feature is the extensive and widespread volume loss across the entire brain in MSA-P, which is not seen in PD [421]. In quantitative MRI studies, the bilateral R2* increase in putamen best separated MSA-P patients from PD [422], consistent with susceptibility weighted imaging results demonstrating higher iron deposition in putamen versus PD [423]. DTI allows differentiation between PD and MSA-P, the latter showing higher values of the apparent diffusion coefficient in the inner capsule, corona radiata, and lateral periputaminal white matter [424] and other pathological differences between MSA-P and PD [425]. Combined use of diffusion ratios, magnetic susceptibility values/quantitative susceptibility mapping allowed differentiation of MSA-P and MSA-C from other parkinsonian syndromes, with sensitivities and specificities of 81–100% [425a]. The relevance of non-specific MRI features in MSA has been critically reviewed recently [426]. Abnormalities in left anterior thalamic radiation and bilateral corticospinal tract are specific for MSA in relation to PD and controls [427]. Elevated putaminal apparent diffusion coefficient and 123IMIBG tests are also useful for differentiation between MSA and PD [428]. FDG-PET can distinguish MSA-P from PD by showing different patterns of decreased glucose metabolism with a specific positive predicting value of 97% [429]. For autonomic function testing, symptomatic causes have to be excluded before attributing them to MCA. Imaging of presymptomatic DAergic functions using 123Iβ-CIT SPECT may not satisfactorily separate MSA from PD, whereas DAD2 receptor ligands that target postsynaptic DAergic functions differentiate PD (normal or increased signal) from MSA (reduced signal) [240]. Dopamine transporter (DaT) imaging showed more prominent and earlier DaT loss in anterior caudate and ventral putamen in MSA than in PD [430, 431], although normal DaT imaging does not exclude MSA [432]. In autopsy-confirmed cases a greater asymmetry of striatal binding was seen in MSA than in PD [433], but it is highly correlated with postmortem SN cell loss [434]. 18F-dopa PET showed more widespread basal ganglia dysfunction in MSA than in PD without evidence of early compensatory increase in Dopa uptake [435]. Ancillary investigations for MSA have been summarized recently [1, 23, 436, 437]. The diagnostic validity of skin punch biopsies for the demonstration of αS deposits in Schwann or other cells in peripheral nerves in MSA patients is under discussion and needs further evaluation in pathologically confirmed cases [210, 292, 293].
PATHOGENESIS
General mechanisms
Although our understanding of MSA remains incomplete, evidence from animal models and human postmortem studies indicate that the accumulation of misfolded αS, particularly in oligodendroglia, plays an essential role in the disease process [155, 164, 443]. MSA is currently considered a synucleinopathy with specific (oligodendro-) glioneuronal degeneration, associated with early myelin dysfunction and neuronal degeneration related to retrograde axonal disease [8, 444, 445]. Although it is tempting to speculate that primary neuronal pathology leads to secondary oligodendroglial degeneration as suggested by the finding that NCIs are more widespread than previously assumed and exist in areas lacking GCIs [154], the robust observation that distribution and severity of neurodegeneration reflect subregional GCI densities supports the assumption that MSA is a primary oligodendrogliopathy [15, 164, 166]. The causative role of GCI pathology in introduction of the neurodegenerative process was confirmed experimentally in transgenic mice overexpressing human αS in oligodendrocytes [446–449].
The selectivity of the neurodegeneration in MSA is determined by the concerted interaction of multiple noxious factors, among them ectopic αS accumulation in oligodendrocytes, “prion-like” propagation of misfolded αS, proteasomal and mitochondrial dysfunction [8, 124], dysregulation of myelin lipids [246, 450], genetic polymorphism [118, 121], microglial activation [257], neuroinflammation [261, 451], proteolytic disturbance, autophagy [191]. and other factors contributing to oxidative stress, which is suggested to be a major pathogenic factor in MSA and related diseases [452, 453]. This suggests a multi-mechanistic hypothesis of the etiopathogenesis of MSA [454].
α-Synuclein and prions
αS, a heat stable cytosolic protein, primarily located in presynaptic nerve terminals, when present in oligodendrocytes in human MSA and transgenic models, has undergone post-translational modification (oxidation, phosphorylation, nitration, etc.) enhanced by oxidative stress [455, 456]. αS in GCIs is phosphorylated at residue Ser-129 and ubiquitinated like in LBs in PD and DLB [457–459].
Biochemical studies revealed a significant accumulation of membrane-associated αS in affected regions of MSA brains containing both neuronal and glial inclusions [251], but most of the soluble αS was also present in areas with few GCIs, suggesting that altered solubility precedes the formation of GCIs and that an increase in soluble monomeric αS could result in a conversion into abnormal insoluble, filamentous aggregations. Various levels of αS isoforms were seen, 140 and 112 isoforms as well as aggregation-prone synphilin-A and parkin isoforms being significantly increased, whereas αS 126 was decreased [460]. These changes of isoform expression profiles suggest alterations in regulation of transcriptions and in protein-protein interactions that may be important in protein aggregation processes being key pathways in the pathogenesis of MSA [460]. The toxicity of αS in its different forms is still undecided, with some reporting a cytoprotective function of αS aggregation in insoluble deposits [461, 462], while others suggest that oligomeric αS is the most toxic form of the protein [463–465].
The source of αS in GCIs and the role of many protein components are enigmatic, although GCIs express αS mitochondrial RNA (mRNA) [466]. Widespread mRNA dysregulation in MSA has been recapitulated in murine models [124]. A number of studies indicates that αS oligomers are released by neurons and taken up by surrounding oligodendrocytes to form GCIs [467]. Incubation of recombinant αS with an oligodendrocyte cell line demonstrated its ability to take up and to accumulate αS into GCI-like structures [459]. SNCA transcripts identified in oligodendrocyte lienage cells may not be the origin of αS in GCIs and MSA [6]. Accumulation of αS in oligodendrocytes induces their dysfunction resulting in reduced trophic support and demyelination shown in the MBP-h/αS mouse model [468, 469]. The leading role of GCI pathology is supported by the “minimal change” (MC-MSA) forms, where severe GCI burden is associated with less severe neuronal loss but shorter disease duration [312].
Changes in MBP levels in MSA brain suggest myelin lipid dysfunction [246, 469, 470], which together with aberration in protein distribution may lead to myelin deficit [471], a crucial pathomechanism in MSA [172]. Elevated matrix metalloproteinase activity may also contribute to the disease process by promoting blood-brain barrier dysfunction and myelin degeneration [472]. Oligodendroglial dysfunction supports the notion that neurodegeneration may occur secondary to demyelination and lack of trophic support by GCI-bearing oligodendroglia [232], but the causative mechanisms of demyelination are not yet fully understood. The temporal evolution of αS pathology in PD [473] and postmortem demonstration of αS inclusions within grafted fetal neurons transplanted into PD brains [474–476] suggested that spreading/propagation of pathological αS species is a mechanism underlying disease progression in α-synucleinopathies [459, 477]. Evidence from human studies, cell culture and animal models has strengthened the concept that pathology arising from neurodegeneration-related proteins such as αS, amyloid-β, and tau, may propagate in a “prion-like” fashion [478, 479]. On the other hand, the prion hypothesis of selective neuronal vulnerability may be another important factor contributing to specific patterns of neurodegeneration [480]. Increasing evidence supports the notion that αS, which is primarily generated by neurons, can be toxic once released to the extracellular environment [481, 482]. It can then propagate to other neurons or glia and to other functionally connected networks in a “prion-like” fashion [16, 483–486]. Propagation and accumulation of αS in glial cells could lead to activation of these cells and subsequent neuroinflammation [261, 487, 488]. Progressive neurodegeneration in MSA results from αS protein misfolding into a self-templating prion confirmation that spreads throughout the brain [491]. Neuroinflammation may also favor the formation of intracellular αS aggregates as a consequence of cytokine release and the shift to a non-inflammatory environment [441]. Pathogenic mechanisms leading to elevated αS levels in neurons underlie neuronal secretion and subsequent uptake of αS by oligodendrocytes [489]. Homogenates containing αS derived from brain biopsy samples from MSA patients triggered aggregates of phosphorylated αS and a neurodegenerative cascade in mice that was compatible with human MSA pathology [130, 490]. Current data indicating that MSA prions are remarkably stable and resistent to inactivation strongly suggest caution when working with materials that might contain αS prions [491]. In vitro studies and animal models have also confirmed the seeding ability of αS [459, 492–494], and that MSA is caused by a unique strain of αS assemblies [130, 495–497]. The existing data suggest that neuron-derived αS with conformational changes contributes to the formation of GCIs, that primary oligodendroglia dysfunction may cause accumulation of αS fibrils in their cytoplasm, and that cell-to-cell spreading of αS may initiate new aggregate formation as the disease propagates [14, 498, 499]. Viral-induced oligodendroglial expression of αS allows replicating some of the key features of MSA, e.g., how αS accumulation in selected oligodendroglial populations contribute to the pathophysiology of the disease [500, 501].
Current view on the pathogenic pathways of MSA (see Fig. 4)
Although major advances have been achieved in understanding the pathophysiological structure of αS [495, 496, 503, 504], the first hit that triggers the neurodegenerative cascade remains to be elucidated. In the light of in vitro studies, suggesting that the oligodendroglial-specific phosphoprotein-25α (p25α) relocation from the myelin sheath to the oligodendroglial cytoplasm followed by cytoplasmic accumulation of p25α is an intriguing early finding in MSA [505, 506]. It functions in the stabilization of microtubules and the differentiation of oligodendrocytes [507] and is associated with myelin dysfunction, reduction in full-length MBP, demyelination of small-caliber axons, and an increase in oligodendroglial soma size, preceding αS aggregation [505]. The interaction between p25α, a potent stimulator of αS aggregation [505, 508, 509], and αS promotes its phosphorylation and aggregation into insoluble oligomers with later formation of GCIs. These changes and decrease in p25α in oligodendrocytes containing αS positive GCIs imply that mitochondrial dysfunction may lead to secondary p25α relocation [510]. The aberrant αS undergoing fibrillation then aggregates to form GCIs, enhanced by misplaced p25α, which is being incorporated into inclusions before αS [511]. The role of the microtubule-associated TPPP/p25 in PD and related diseases has recently been reviewed. Aggregation of αS interferes with oligodendrocytes, preventing the formation of mature oligodendroglial cells [512, 513]. Transgenic expression of human αS indicated that accumulation of αS in oligodendroglia induces subsequent degeneration of both oligodendroglia and neurons [468, 514]. Enhanced FAS (Fas cell surface death receptor) gene expression is an early hallmark of oligodendroglial pathology in MSA that may be related to αS dependent degeneration [262, 515]. Differential involvement of the cystein protease inhibitor cystein C that is associated with increased risk of neurodegeneration in MSA phenotypes supports its role in MSA pathogenesis [516]. Formation of GCIs interferes with oligodendroglial and neuronal trophic transport leading to death of these cells and to initiation of neuroinflammation by activation of quiescent microglia [451]. The association of GCI burden and activated microglial cells [254] suggests that αS triggers neuroinflammatory responses. This was corroborated by experimental studies both in vitro and in vivo [261, 263, 517]. Microglia activation may contribute to the neurodegenerative process in MSA via increased levels of reactive oxygen species in degenerating areas [258]. However, the mechanisms inducing αS dependent microglia activation remain to be elucidated [249]. It can be speculated, that the paradoxical protein expression in pons of MSA (with increased tissue iron, increased ferritin, and decreased ferroportin indicating reduction of bioavailable iron) is associated with neuroinflammation as hepcidin is induced by cytokines and may result in chronic diseases like anemia with elevation of total body iron with reduced bioavailable iron [518]. Changes in T-cell-associated cytokines may shed light on immune mechanisms that contribute to MSA [519]. Increased mRNA levels of GSK3β that is involved in neuroinflammatory pathways, MHC class II+ and CD45+ positive cells in prefrontal cortex of MSA suggest widespread neuroinflammatory reactions in MSA pathogenesis [520].
Finally, the cell death mechanisms are poorly understood. Increased iron levels in degenerating brain areas suggested that oxidative stress may play a significant role in the selected neuronal death in MSA, and microglial activation may contribute to increased levels of reactive oxygen species in the degenerating areas [258]. Loss of phosphoprotein DARPP-32 and calbindin-D 28k in areas of less prominent or absent neuronal loss indicates that calcium toxicity and disturbance of the phosphorylated state of proteins are early events in MSA pathogenesis [520]. The apoptosis-modulating proteins Bax and Bcl-X are increased, which may lead to initiation of apoptosis in the affected areas [521]. Pathological and biochemical analyses revealed that autophagy/beclin1 regulator 1 (AMBRA1) is a component of the pathological hallmark and upstream autophagy proteins are impaired in the MSA brain. AMBRA1 is a novel hub binding protein of αS and plays a central role through the degradative dynamics of αS [191]. Mechanisms possibly related to cell death in MSA include X-linked inhibitor of apoptosis protein (XIAP) which is upregulated in GCI- and NCI-bearing cells [200], proteasomal or autophagosomal dysfunction [522], supported by experimental studies [469, 523]. αS accumulation in cells may induce metabolic imbalance, which may promote cell death. Phosphoinositide 3-kinase upregulation in neurons and oligodendrocytes suggests a possible response to apoptotic signals in these cells [524]. Further mechanisms include proteasomal [525] or autophagosomal dysfunctions [522, 526], supported by experimental studies [258, 469, 523], or an altered communication between neurons and oligodendrocytes due to perturbation of their neurotrophic transport [527].
CURRENT THERAPIES
So far there are no causative or disease-modifying treatments available and symptomatic therapies are limited [1, 49]. Levodopa responsiveness has been reported initially in 83% of MSA-P patients [353], but the effect is usually transient, and only 31% showed a response for a period of 3.5 years [31]. In some patients, motor fluctuations with wearing-off phenomena or off-bound dystonia were observed [438]. Deep brain stimulation could not be recommended for MSA [321], while active immunization against αS and combination with anti-inflammatory treatment may be promising therapeutic strategies [16, 439–442]. New strategies targeting αS are in progress [23, 436, 443], based on completed or ongoing interventional trials by the MSA Coalition [23]. Therefore, there is a strong need to clarify the pathogenic mechanisms in MSA in order to develop new therapeutic strategies options.
CONCLUSIONS AND FURTHER OUTLOOK
Current evidence supports the hypothesis that misfolded αS contributes to oxidative stress through a pathway that induces microglial activation and antioxidant response requiring an additional protein structure [528], but oxidative stress appears unlikely to represent the sole mechanism for αS aggregation. Region specific increased accumulation of intracellular iron in pons and putamen in MSA may indicate its local dysregulation due to a deficit of bioavailable iron [299]. While experimental studies support the involvement of the proteasome and autophagosome dysfunction in oligodendroglial α-synucleinopathy [258, 523], excitotoxic cell death was not aggravated by GCI pathology [529]. The burden of neuronal pathology appears to increase multifocally as an effect of disease duration associated with increasing overall αS burden, the underlying mechanisms of which as well as of those leading to widespread demyelination need further elucidation.
In conclusion, the cascade of events that underlies the pathogenesis of MSA is currently not completely understood. Recent studies using animal models that only partly replicate the human pathology and the molecular dynamics of the neurodegenerative process have provided some progress in our understanding of MSA pathogenesis. Relocation of p25α from the myelin sheaths to the oligodendroglial soma (due to mitochondrial dysfunction), with formation of cytoplasmic p25α inclusions seems to precede aggregation of transformed αS assemblies in oligodendrocytes. This is associated with disruption of myelin homeostasis. The source of αS in oligodendrogliosis is unclear, but it contains αS mRNA expression and αS may be secreted by neurons and taken up by oligodendrocytes to form GCIs. Secondary events in the oligodendroglial inclusion pathway include reduced trophic support to axons and neurons by reduced glial cell line-derived neurotrophic factor. Neuroinflammation, oxidative stress, proteolytic dysbalance, and energy failure are further essential factors in the cascade leading to neurodegeneration in MSA. The disease is currently viewed as a primary synucleinopathy with specific (oligodendro)glial-neuronal degeneration developing secondarily via the oligo-myelin-axon-neuron complex [155], and also has been listed among the predominant oligodendroglial proteinopathies [15]. Strong evidence against a primary neuronal pathology with formation of GCIs resulting from secondary accumulation of pathological αS that may be of neuronal origin [527], is the fact that GCIs are the hallmark of MSA and not of PD, a disease with similar lesion patterns of αS immunoreactive inclusions (LBs) but no or few GCIs, which differentiates the two disorders [451]. Although the source of αS in both disorders is under discussion, “prion-like” spreading of the misfolded protein, oxidative damage, mitochondrial dysfunction, proteolytic dysbalance, dysregulation of myelin lipids, neuroinflammation, and energy failure are the essential noxious factors in the cascade leading to the pathogenesis of systemic neurodegeneration in this unique proteinopathy. Multidisciplinary research to further elucidate the pathologic mechanisms of neurodegeneration in MSA in order to develop reliable biomarkers for early diagnosis and disease-modifying therapies of this hitherto incurable disorder is strongly needed.
ACKNOWLEDGMENTS
The author thanks Mr. E. Mitter-Ferstl, PhD, for secretarial and graphical work. The study was partially funded by the Society for the Promotion of Research in Experimental Neurology, Vienna, Austria.
The author’s disclosure is available online (http://j-alz.com/manuscript-disclosures/17-0397r1).
REFERENCES
[1] | Fanciulli A , Wenning GK ((2015) ) Multiple-system atrophy. N Engl J Med 372: , 249–263. |
[2] | Graham JG , Oppenheimer DR ((1969) ) Orthostatic hypotension and nicotine sensitivity in a case of multiple system atrophy. J Neurol Neurosurg Psychiatry 32: , 28–34. |
[3] | Spillantini MG , Goedert M ((2016) ) Synucleinopathies: Past, present and future. Neuropathol Appl Neurobiol 42: , 3–5. |
[4] | Jellinger KA ((2014) ) Neuropathology. In Multiple System Atrophy, Wenning GK, Fanciulli A, eds. Springer-Verlag Vienna, pp. 17–55. |
[5] | Goedert M , Jakes R , Spillantini MG ((2017) ) The synucleinopathies: Twenty years on. J Parkinsons Dis 7: , S53–S71. |
[6] | Koga S , Dickson DW ((2017) ) Recent advances in neuropathology, biomarkers and therapeutic approach of multiple system atrophy. J Neurol Neurosurg Psychiatry, doi 10.1136/jnnp-2017-315813 |
[7] | Trojanowski JQ , Revesz T ((2007) ) Proposed neuropathological criteria for the post mortem diagnosis of multiple system atrophy. Neuropathol Appl Neurobiol 33: , 615–620. |
[8] | Jellinger KA , Wenning GK ((2016) ) Multiple system atrophy: Pathogenic mechanisms and biomarkers. J Neural Transm (Vienna) 123: , 555–572. |
[9] | Gilman S , Wenning GK , Low PA , Brooks DJ , Mathias CJ , Trojanowski JQ , Wood NW , Colosimo C , Durr A , Fowler CJ , Kaufmann H , Klockgether T , Lees A , Poewe W , Quinn N , Revesz T , Robertson D , Sandroni P , Seppi K , Vidailhet M ((2008) ) Second consensus statement on the diagnosis of multiple system atrophy. Neurology 71: , 670–676. |
[10] | Lyoo CH , Jeong Y , Ryu YH , Lee SY , Song TJ , Lee JH , Rinne JO , Lee MS ((2008) ) Effects of disease duration on the clinical features and brain glucose metabolism in patients with mixed type multiple system atrophy. Brain 131: , 438–446. |
[11] | Dehay B , Vila M , Bezard E , Brundin P , Kordower JH ((2016) ) Alpha-synuclein propagation: New insights from animal models. Mov Disord 31: , 161–168. |
[12] | McCann H , Cartwright H , Halliday GM ((2016) ) Neuropathology of alpha-synuclein propagation and Braak hypothesis. Mov Disord 31: , 152–160. |
[13] | Woerman AL , Watts JC , Aoyagi A , Giles K , Middleton LT , Prusiner SB ((2017) ) alpha-Synuclein: Multile system atrophy prions. Cold Spring Harb Perspect Med. doi: 10.1101/cshperspect.a024588 |
[14] | Goedert M , Masuda-Suzukake M , Falcon B ((2017) ) Like prions: The propagation of aggregated tau and alpha-synuclein in neurodegeneration. Brain 140: , 266–278. |
[15] | Rohan Z , Milenkovic I , Lutz MI , Matej R , Kovacs GG ((2016) ) Shared and distinct patterns of oligodendroglial response in alpha-synucleinopathies and tauopathies. J Neuropathol Exp Neurol 75: , 1100–1109. |
[16] | Valera E , Monzio Compagnoni G , Masliah E ((2016) ) Review: Novel treatment strategies targeting alpha-synuclein in multiple system atrophy as a model of synucleinopathy. Neuropathol Appl Neurobiol 42: , 95–106. |
[17] | Kim HJ , Jeon BS , Jellinger KA ((2015) ) Diagnosis and differential diagnosis of MSA: Boundary issues. J Neurol 262: , 1801–1813. |
[18] | Kim HJ , Stamelou M , Jeon B ((2016) ) Multiple system atrophy-mimicking conditions: Diagnostic challenges. Parkinsonism Relat Disord 22: (Suppl 1), S12–S15. |
[19] | Koga S , Aoki N , Uitti RJ , van Gerpen JA , Cheshire WP , Josephs KA , Wszolek ZK , Langston JW , Dickson DW ((2015) ) When DLB, PD, and PSP masquerade as MSA: An autopsy study of 134 patients. Neurology 85: , 404–412. |
[20] | Mestre TA , Gupta A , Lang AE ((2016) ) MRI signs of multiple system atrophy preceding the clinical diagnosis: The case for an imaging-supported probable MSA diagnostic category. J Neurol Neurosurg Psychiatry 87: , 443–444. |
[21] | Rizzo G , Copetti M , Arcuti S , Martino D , Fontana A , Logroscino G ((2016) ) Accuracy of clinical diagnosis of Parkinson disease: A systematic review and meta-analysis. Neurology 86: , 566–576. |
[22] | Krismer F , Jellinger KA , Scholz SW , Seppi K , Stefanova N , Antonini A , Poewe W , Wenning GK ((2014) ) Multiple system atrophy as emerging template for accelerated drug discovery in alpha-synucleinopathies. Parkinsonism Relat Disord 20: , 793–799. |
[23] | Krismer F , Wenning GK ((2017) ) Multiple system atrophy: Insights into a rare and debilitating movement disorder. Nat Rev Neurol 13: , 232–243. |
[24] | Bower JH , Maraganore DM , McDonnell SK , Rocca WA ((1997) ) Incidence of progressive supranuclear palsy and multiple system atrophy in Olmsted County, Minnesota, 1976 to 1990. Neurology 49: , 1284–1288. |
[25] | Linder J , Stenlund H , Forsgren L ((2010) ) Incidence of Parkinson’s disease and parkinsonism in northern Sweden: A population-based study. Mov Disord 25: , 341–348. |
[26] | Winter Y , Bezdolnyy Y , Katunina E , Avakjan G , Reese JP , Klotsche J , Oertel WH , Dodel R , Gusev E ((2010) ) Incidence of Parkinson’s disease and atypical parkinsonism: Russian population-based study. Mov Disord 25: , 349–356. |
[27] | Chio A , Magnani C , Schiffer D ((1998) ) Prevalence of Parkinson’s disease in Northwestern Italy: Comparison of tracer methodology and clinical ascertainment of cases. Mov Disord 13: , 400–405. |
[28] | Tison F , Yekhlef F , Chrysostome V , Sourgen C ((2000) ) Prevalence of multiple system atrophy. Lancet 355: , 495–496. |
[29] | Schrag A , Ben-Shlomo Y , Quinn NP ((1999) ) Prevalence of progressive supranuclear palsy and multiple system atrophy: A cross-sectional study. Lancet 354: , 1771–1775. |
[30] | Chrysostome V , Tison F , Yekhlef F , Sourgen C , Baldi I , Dartigues JF ((2004) ) Epidemiology of multiple system atrophy: A prevalence and pilot risk factor study in Aquitaine, France. Neuroepidemiology 23: , 201–208. |
[31] | Wenning GK , Geser F , Krismer F , Seppi K , Duerr S , Boesch S , Kollensperger M , Goebel G , Pfeiffer KP , Barone P , Pellecchia MT , Quinn NP , Koukouni V , Fowler CJ , Schrag A , Mathias CJ , Giladi N , Gurevich T , Dupont E , Ostergaard K , Nilsson CF , Widner H , Oertel W , Eggert KM , Albanese A , del Sorbo F , Tolosa E , Cardozo A , Deuschl G , Hellriegel H , Klockgether T , Dodel R , Sampaio C , Coelho M , Djaldetti R , Melamed E , Gasser T , Kamm C , Meco G , Colosimo C , Rascol O , Meissner WG , Tison F , Poewe W ((2013) ) The natural history of multiple system atrophy: A prospective European cohort study. Lancet Neurol 12: , 264–274. |
[32] | Yabe I , Soma H , Takei A , Fujiki N , Yanagihara T , Sasaki H ((2006) ) MSA-C is the predominant clinical phenotype of MSA in Japan: Analysis of 142 patients with probable MSA. J Neurol Sci 249: , 115–121. |
[33] | Watanabe H , Saito Y , Terao S , Ando T , Kachi T , Mukai E , Aiba I , Abe Y , Tamakoshi A , Doyu M , Hirayama M , Sobue G ((2002) ) Progression and prognosis in multiple system atrophy: An analysis of 230 Japanese patients. Brain 125: , 1070–1083. |
[34] | Kim HJ , Jeon BS , Lee JY , Yun JY ((2011) ) Survival of Korean patients with multiple system atrophy. Mov Disord 26: , 909–912. |
[35] | Ozawa T , Onodera O ((2017) ) Multiple system atrophy: Clinicopathological characteristics in Japanese patients. Proc Jpn Acad Ser B Phys Biol Sci 93: , 251–258. |
[36] | Quinn N ((1989) ) Multiple system atrophy–the nature of the beast. J Neurol Neurosurg Psychiatry Suppl: , 78–89. |
[37] | Jecmenica-Lukic M , Poewe W , Tolosa E , Wenning GK ((2012) ) Premotor signs and symptoms of multiple system atrophy. Lancet Neurol 11: , 361–368. |
[38] | Xie T , Kang UJ , Kuo S-H , Poulopoulos M , Greene P , Fahn S ((2015) ) Comparison of clinical features in pathologically confirmed PSP and MSA patients followed at a tertiary center. NPJ Parkinson’s Dis 1: , 15007. |
[39] | Coon EA , Sletten DM , Suarez MD , Mandrekar JN , Ahlskog JE , Bower JH , Matsumoto JY , Silber MH , Benarroch EE , Fealey RD , Sandroni P , Low PA , Singer W ((2015) ) Clinical features and autonomic testing predict survival in multiple system atrophy. Brain 138: , 3623–3631. |
[40] | Sakushima K , Nishimoto N , Nojima M , Matsushima M , Yabe I , Sato N , Mori M , Sasaki H ((2015) ) Epidemiology of multiple system atrophy in Hokkaido, the northernmost island of Japan. Cerebellum 14: , 682–687. |
[41] | Lee SW , Koh SB ((2012) ) Clinical features and disability milestones in multiple system atrophy and progressive supranuclear palsy. J Mov Disord 5: , 42–47. |
[42] | Klockgether T , Ludtke R , Kramer B , Abele M , Burk K , Schols L , Riess O , Laccone F , Boesch S , Lopes-Cendes I , Brice A , Inzelberg R , Zilber N , Dichgans J ((1998) ) The natural history of degenerative ataxia: A retrospective study in 466 patients. Brain 121: (Pt 4), 589–600. |
[43] | Petrovic IN , Ling H , Asi Y , Ahmed Z , Kukkle PL , Hazrati LN , Lang AE , Revesz T , Holton JL , Lees AJ ((2012) ) Multiple system atrophy-parkinsonism with slow progression and prolonged survival: A diagnostic catch. Mov Disord 27: , 1186–1190. |
[44] | Itoh K , Kasai T , Tsuji Y , Saito K , Mizuta I , Harada Y , Sudoh S , Mizuno T , Nakagawa M , Fushiki S ((2014) ) Definite familial multiple system atrophy with unknown genetics. Neuropathology 34: , 309–313. |
[45] | Schrag A , Wenning GK , Quinn N , Ben-Shlomo Y ((2008) ) Survival in multiple system atrophy. Mov Disord 23: , 294–296. |
[46] | Roncevic D , Palma JA , Martinez J , Goulding N , Norcliffe-Kaufmann L , Kaufmann H ((2014) ) Cerebellar and parkinsonian phenotypes in multiple system atrophy: Similarities, differences and survival. J Neural Transm 121: , 507–512. |
[47] | Starhof C , Korbo L , Funch Lassen C , Winge K , Friis S ((2016) ) Clinical features in a danish population-based cohort of probable multiple system atrophy patients. Neuroepidemiology 46: , 261–267. |
[48] | Jecmenica-Lukic M , Petrovic IN , Pekmezovic T , Kostic VS ((2014) ) Clinical outcomes of two main variants of progressive supranuclear palsy and multiple system atrophy: A prospective natural history study. J Neurol 261: , 1575–1583. |
[49] | Bensimon G , Ludolph A , Agid Y , Vidailhet M , Payan C , Leigh PN ((2009) ) Riluzole treatment, survival and diagnostic criteria in Parkinson plus disorders: The NNIPPS study. Brain 132: , 156–171. |
[50] | Gatto E , Rodriguez-Violante M , Consentino C , Chana-Cuevas P , Miranda M , Gallin E , Etcheverry JL , Nunez Y , Parisi V , Persi G , Vecchi C , Sanguinetti A , Alleva A , Aparcana J , Torres L , Litvan I ((2014) ) Pan-American Consortium of Multiple System Atrophy (PANMSA). A Pan-American multicentre cohort study of Multiple System Atrophy. J Parkinsons Dis 4: , 693–698. |
[51] | Low PA , Reich SG , Jankovic J , Shults CW , Stern MB , Novak P , Tanner CM , Gilman S , Marshall FJ , Wooten F , Racette B , Chelimsky T , Singer W , Sletten DM , Sandroni P , Mandrekar J ((2015) ) Natural history of multiple system atrophy in the USA: A prospective cohort study. Lancet Neurol 14: , 710–719. |
[52] | Figueroa JJ , Singer W , Parsaik A , Benarroch EE , Ahlskog JE , Fealey RD , Parisi JE , Sandroni P , Mandrekar J , Iodice V , Low PA , Bower JH ((2014) ) Multiple system atrophy: Prognostic indicators of survival. Mov Disord 29: , 1151–1157. |
[53] | Tada M , Onodera O , Ozawa T , Piao YS , Kakita A , Takahashi H , Nishizawa M ((2007) ) Early development of autonomic dysfunction may predict poor prognosis in patients with multiple system atrophy. Arch Neurol 64: , 256–260. |
[54] | Glasmacher SA , Leigh PN , Saha RA ((2017) ) Predictors of survival in progressive supranuclear palsy and multiple system atrophy: A systematic review and meta-analysis. J Neurol Neurosurg Psychiatry 88: , 402–411. |
[55] | Savica R , Grossardt BR , Bower JH , Ahlskog JE , Boeve BF , Graff-Radford J , Rocca WA , Mielke MM ((2017) ) Survival and causes of death among people with clinically diagnosed synucleinopathies with parkinsonism: A population-based study. JAMA Neurol 74: , 839–846. |
[56] | Brown RC , Lockwood AH , Sonawane BR ((2005) ) Neurodegenerative diseases: An overview of environmental risk factors. Environ Health Perspect 113: , 1250–1256. |
[57] | Fujioka S , Ogaki K , Tacik PM , Uitti RJ , Ross OA , Wszolek ZK ((2014) ) Update on novel familial forms of Parkinson’s disease and multiple system atrophy. Parkinsonism Relat Disord 20: (Suppl 1), S29–S34. |
[58] | Vidal JS , Vidailhet M , Derkinderen P , Tzourio C , Alperovitch A ((2010) ) Familial aggregation in atypical Parkinson’s disease: A case control study in multiple system atrophy and progressive supranuclear palsy. J Neurol 257: , 1388–1393. |
[59] | Hohler AD , Singh VJ ((2012) ) Probable hereditary multiple system atrophy-autonomic (MSA-A) in a family in the United States. J Clin Neurosci 19: , 479–480. |
[60] | Wullner U , Schmitt I , Kammal M , Kretzschmar HA , Neumann M ((2009) ) Definite multiple system atrophy in a German family. J Neurol Neurosurg Psychiatry 80: , 449–450. |
[61] | Hara K , Momose Y , Tokiguchi S , Shimohata M , Terajima K , Onodera O , Kakita A , Yamada M , Takahashi H , Hirasawa M , Mizuno Y , Ogata K , Goto J , Kanazawa I , Nishizawa M , Tsuji S ((2007) ) Multiplex families with multiple system atrophy. Arch Neurol 64: , 545–551. |
[62] | Soma H , Yabe I , Takei A , Fujiki N , Yanagihara T , Sasaki H ((2006) ) Heredity in multiple system atrophy. J Neurol Sci 240: , 107–110. |
[63] | Multiple-System Atrophy Research Collaboration ((2013) ) Mutations in COQ2 in familial and sporadic multiple-system atrophy. N Engl J Med 371: , 233–244. Erratum in N Engl J Med 371, 94, 2014. |
[64] | Chen YP , Zhao B , Cao B , Song W , Guo X , Wei QQ , Yang Y , Yuan LX , Shang HF ((2015) ) Mutation scanning of the COQ2 gene in ethnic Chinese patients with multiple-system atrophy. Neurobiol Aging 36: , 1222 e1227–1211. |
[65] | Tsuji S ((2014) ) Susceptibility gene in multiple system atrophy (MSA). Rinsho Shinkeigaku 54: , 969–971. |
[66] | Ogaki K , Fujioka S , Heckman MG , Rayaprolu S , Soto-Ortolaza AI , Labbe C , Walton RL , Lorenzo-Betancor O , Wang X , Asmann Y , Rademakers R , Graff-Radford N , Uitti R , Cheshire WP , Wszolek ZK , Dickson DW , Ross OA ((2014) ) Analysis of COQ2 gene in multiple system atrophy. Mol Neurodegener 9: , 44. |
[67] | Wen XD , Li HF , Wang HX , Ni W , Dong Y , Wu ZY ((2015) ) Mutation analysis of COQ2 in Chinese patients with cerebellar subtype of multiple system atrophy. CNS Neurosci Ther 21: , 626–630. |
[68] | Zhao Q , Yang X , Tian S , An R , Zheng J , Xu Y ((2016) ) Association of the COQ2 V393A variant with risk of multiple system atrophy in East Asians: A case-control study and meta-analysis of the literature. Neurol Sci 37: , 423–430. |
[69] | Sun Z , Ohta Y , Yamashita T , Sato K , Takemoto M , Hishikawa N , Abe K ((2016) ) New susceptible variant of COQ2 gene in Japanese patients with sporadic multiple system atrophy. Neurol Genet 2: , e54. |
[70] | Mitsui J , Koguchi K , Momose T , Takahashi M , Matsukawa T , Yasuda T , Tokushige SI , Ishiura H , Goto J , Nakazaki S , Kondo T , Ito H , Yamamoto Y , Tsuji S ((2017) ) Three-year follow-up of high-dose ubiquinol supplementation in a case of familial multiple system atrophy with compound heterozygous COQ2 mutations. Cerebellum 16: , 664–672. |
[71] | Ferguson MC , Garland EM , Hedges L , Womack-Nunley B , Hamid R , Phillips JA 3rd , Shibao CA , Raj SR , Biaggioni I , Robertson D ((2014) ) SHC2 gene copy number in multiple system atrophy (MSA). Clin Auton Res 24: , 25–30. |
[72] | Ronchi D , Di Biase E , Franco G , Melzi V , Del Sorbo F , Elia A , Barzaghi C , Garavaglia B , Bergamini C , Fato R , Mora G , Del Bo R , Fortunato F , Borellini L , Trezzi I , Compagnoni GM , Monfrini E , Frattini E , Bonato S , Cogiamanian F , Ardolino G , Priori A , Bresolin N , Corti S , Comi GP , Di Fonzo A ((2016) ) Mutational analysis of COQ2 in patients with MSA in Italy. Neurobiol Aging 45: , 213 e211–212. |
[73] | Sharma M , Wenning G , Krüger R ((2014) ) Mutant COQ2 in multiple-system atrophy. N Engl J Med 371: , 80–83. |
[74] | Jeon BS , Farrer MJ , Bortnick SF , Korean Canadian Alliance on Parkinson’s Disease and Related Disorders ((2014) ) Mutant COQ2 in multiple-system atrophy. N Engl J Med 371: , 80. |
[75] | Lin CH , Tan EK , Yang CC , Yi Z , Wu RM ((2015) ) COQ2 gene variants associate with cerebellar subtype of multiple system atrophy in Chinese. Mov Disord 30: , 436–437. |
[76] | Barca E , Kleiner G , Tang G , Ziosi M , Tadesse S , Masliah E , Louis ED , Faust P , Kang UJ , Torres J , Cortes EP , Vonsattel JP , Kuo SH , Quinzii CM ((2016) ) Decreased coenzyme Q10 levels in multiple system atrophy cerebellum. J Neuropathol Exp Neurol 75: , 663–672. |
[77] | Mitsui J , Matsukawa T , Yasuda T , Ishiura H , Tsuji S ((2016) ) Plasma coenzyme Q10 levels in patients with multiple system atrophy. JAMA Neurol 73: , 977–980. |
[78] | Schottlaender LV , Bettencourt C , Kiely AP , Chalasani A , Neergheen V , Holton JL , Hargreaves I , Houlden H ((2016) ) Coenzyme Q10 levels are decreased in the cerebellum of multiple-system atrophy patients. PLoS One 11: , e0149557. |
[79] | Salviati L, Trevisson E, Doimo M, Navas P ((2017) ) Primary coenzyme Q10 deficiency. In GeneReviews®, Pagon RA, Adam MP, Ardinger HH, Wallace SE, Amemiya A, Bean LJH, Bird TD, Ledbetter N, Mefford HC, Smith RJH, Stephens K eds. 2017/01/27 Edition. University of Washington, Seattle, pp. 1993–2017. |
[80] | Yang X , An R , Xi J , Zhen J , Chen Y , Huang H , Tian S , Zhao Q , Ning P , Xu Y ((2017) ) Sequence TMEM230 gene in patients with multiple system atrophy in a southwest Chinese population: A pilot study. J Neurol Sci 375: , 264–265. |
[81] | Hu T , Chen Y , Ou R , Wei Q , Cao B , Zhao B , Wu Y , Song W , Chen X , Shang HF ((2017) ) Association analysis of polymorphisms in VMAT2 and TMEM106B genes for Parkinson’s disease, amyotrophic lateral sclerosis and multiple system atrophy. J Neurol Sci 377: , 65–71. |
[82] | Sasaki H , Emi M , Iijima H , Ito N , Sato H , Yabe I , Kato T , Utsumi J , Matsubara K ((2011) ) Copy number loss of (src homology 2 domain containing)-transforming protein 2 (SHC2) gene: Discordant loss in monozygotic twins and frequent loss in patients with multiple system atrophy. Mol Brain 4: , 24. |
[83] | Ross OA , Vilarino-Guell C , Wszolek ZK , Farrer MJ , Dickson DW ((2010) ) Reply to: SNCA variants are associated with increased risk of multiple system atrophy. Ann Neurol 67: , 414–415. |
[84] | Scholz SW , Houlden H , Schulte C , Sharma M , Li A , Berg D , Melchers A , Paudel R , Gibbs JR , Simon-Sanchez J , Paisan-Ruiz C , Bras J , Ding J , Chen H , Traynor BJ , Arepalli S , Zonozi RR , Revesz T , Holton J , Wood N , Lees A , Oertel W , Wullner U , Goldwurm S , Pellecchia MT , Illig T , Riess O , Fernandez HH , Rodriguez RL , Okun MS , Poewe W , Wenning GK , Hardy JA , Singleton AB , Del Sorbo F , Schneider S , Bhatia KP , Gasser T ((2009) ) SNCA variants are associated with increased risk for multiple system atrophy. Ann Neurol 65: , 610–614. |
[85] | Al-Chalabi A , Durr A , Wood NW , Parkinson MH , Camuzat A , Hulot JS , Morrison KE , Renton A , Sussmuth SD , Landwehrmeyer BG , Ludolph A , Agid Y , Brice A , Leigh PN , Bensimon G ((2009) ) Genetic variants of the alpha-synuclein gene SNCA are associated with multiple system atrophy. PLoS One 4: , e7114. |
[86] | Ahmed Z , Asi YT , Sailer A , Lees AJ , Houlden H , Revesz T , Holton JL ((2012) ) The neuropathology, pathophysiology and genetics of multiple system atrophy. Neuropathol Appl Neurobiol 38: , 4–24. |
[87] | Federoff M , Price TR , Sailer A , Scholz S , Hernandez D , Nicolas A , Singleton AB , Nalls M , Houlden H ((2016) ) Genome-wide estimate of the heritability of multiple system atrophy. Parkinsonism Relat Disord 22: , 35–41. |
[88] | Sun Z , Xiang X , Tang B , Chen Z , Peng H , Xia K , Jiang H ((2015) ) SNP rs11931074 of the SNCA gene may not be associated with multiple system atrophy in Chinese population. Int J Neurosci 125: , 612–615. |
[89] | Yun JY , Lee WW , Lee JY , Kim HJ , Park SS , Jeon BS ((2010) ) SNCA variants and multiple system atrophy. Ann Neurol 67: , 554–555. |
[90] | Chen Y , Wei QQ , Ou R , Cao B , Chen X , Zhao B , Guo X , Yang Y , Chen K , Wu Y , Song W , Shang HF ((2015) ) Genetic variants of SNCA are associated with susceptibility to Parkinson’s disease but not amyotrophic lateral sclerosis or multiple system atrophy in a Chinese population. PLoS One 10: , e0133776. |
[91] | Lincoln SJ , Ross OA , Milkovic NM , Dickson DW , Rajput A , Robinson CA , Papapetropoulos S , Mash DC , Farrer MJ ((2007) ) Quantitative PCR-based screening of alpha-synuclein multiplication in multiple system atrophy. Parkinsonism Relat Disord 13: , 340–342. |
[92] | Kiely AP , Asi YT , Kara E , Limousin P , Ling H , Lewis P , Proukakis C , Quinn N , Lees AJ , Hardy J , Revesz T , Houlden H , Holton JL ((2013) ) alpha-Synucleinopathy associated with G51D SNCA mutation: A link between Parkinson’s disease and multiple system atrophy? Acta Neuropathol 125: , 753–769. |
[93] | Kiely AP , Ling H , Asi YT , Kara E , Proukakis C , Schapira AH , Morris HR , Roberts HC , Lubbe S , Limousin P , Lewis PA , Lees AJ , Quinn N , Hardy J , Love S , Revesz T , Houlden H , Holton JL ((2015) ) Distinct clinical and neuropathological features of G51D SNCA mutation cases compared with SNCA duplication and H50Q mutation. Mol Neurodegener 10: , 41. |
[94] | Pasanen P , Myllykangas L , Siitonen M , Raunio A , Kaakkola S , Lyytinen J , Tienari PJ , Poyhonen M , Paetau A ((2014) ) A novel alpha-synuclein mutation A53E associated with atypical multiple system atrophy and Parkinson’s disease-type pathology. Neurobiol Aging 35: , 2180 e2181–2185. |
[95] | Fujishiro H , Imamura AY , Lin WL , Uchikado H , Mark MH , Golbe LI , Markopoulou K , Wszolek ZK , Dickson DW ((2013) ) Diversity of pathological features other than Lewy bodies in familial Parkinson’s disease due to SNCA mutations. Am J Neurodegener Dis 2: , 266–275. |
[96] | Satake W , Nakabayashi Y , Mizuta I , Hirota Y , Ito C , Kubo M , Kawaguchi T , Tsunoda T , Watanabe M , Takeda A , Tomiyama H , Nakashima K , Hasegawa K , Obata F , Yoshikawa T , Kawakami H , Sakoda S , Yamamoto M , Hattori N , Murata M , Nakamura Y , Toda T ((2009) ) Genome-wide association study identifies common variants at four loci as genetic risk factors for Parkinson’s disease. Nat Genet 41: , 1303–1307. |
[97] | Simon-Sanchez J , Schulte C , Bras JM , Sharma M , Gibbs JR , Berg D , Paisan-Ruiz C , Lichtner P , Scholz SW , Hernandez DG , Kruger R , Federoff M , Klein C , Goate A , Perlmutter J , Bonin M , Nalls MA , Illig T , Gieger C , Houlden H , Steffens M , Okun MS , Racette BA , Cookson MR , Foote KD , Fernandez HH , Traynor BJ , Schreiber S , Arepalli S , Zonozi R , Gwinn K , van der Brug M , Lopez G , Chanock SJ , Schatzkin A , Park Y , Hollenbeck A , Gao J , Huang X , Wood NW , Lorenz D , Deuschl G , Chen H , Riess O , Hardy JA , Singleton AB , Gasser T ((2009) ) Genome-wide association study reveals genetic risk underlying Parkinson’s disease. Nat Genet 41: , 1308–1312. |
[98] | Nussbaum RL ((2017) ) Genetics of synucleinopathies. Cold Spring Harb Perspect Med. doi 10.1101/cshperspect.a024109 |
[99] | Stemberger S , Scholz SW , Singleton AB , Wenning GK ((2011) ) Genetic players in multiple system atrophy: Unfolding the nature of the beast. Neurobiol Aging 32: , 1924 e1925–1914. |
[100] | Chelban V , Bettencourt C , Houlden H ((2016) ) Updates on potential therapeutic targets in MSA. ACNR 15: , 8–11. |
[101] | Brooks JA , Houlden H , Melchers A , Islam AJ , Ding J , Li A , Paudel R , Revesz T , Holton JL , Wood N , Lees A , Singleton AB , Scholz SW ((2011) ) Mutational analysis of parkin and PINK1 in multiple system atrophy. Neurobiol Aging 32: , 548 e545–547. |
[102] | Fogel BL , Baker C , Curnow A , Perlman SL , Geschwind DH , Coppola G ((2013) ) Mutations in PDYN are not responsible for multiple system atrophy. J Neurol 260: , 927–928. |
[103] | Yuan X , Chen Y , Cao B , Zhao B , Wei Q , Guo X , Yang Y , Yuan L , Shang H ((2015) ) An association analysis of the R1628P and G2385R polymorphisms of the LRRK2 gene in multiple system atrophy in a Chinese population. Parkinsonism Relat Disord 21: , 147–149. |
[104] | Labbe C , Heckman MG , Lorenzo-Betancor O , Murray ME , Ogaki K , Soto-Ortolaza AI , Walton RL , Fujioka S , Koga S , Uitti RJ , van Gerpen JA , Petersen RC , Graff-Radford NR , Younkin SG , Boeve BF , Cheshire WP Jr , Low PA , Sandroni P , Coon EA , Singer W , Wszolek ZK , Dickson DW , Ross OA ((2016) ) MAPT haplotype diversity in multiple system atrophy. Parkinsonism Relat Disord 30: , 40–45. |
[105] | Heckman MG , Schottlaender L , Soto-Ortolaza AI , Diehl NN , Rayaprolu S , Ogaki K , Fujioka S , Murray ME , Cheshire WP , Uitti RJ , Wszolek ZK , Farrer MJ , Sailer A , Singleton AB , Chinnery PF , Keogh MJ , Gentleman SM , Holton JL , Aoife K , Mann DM , Al-Sarraj S , Troakes C , Dickson DW , Houlden H , Ross OA ((2014) ) LRRK2 exonic variants and risk of multiple system atrophy. Neurology 83: , 2256–2261. |
[106] | Scholz SW , Majounie E , Revesz T , Holton JL , Okun MS , Houlden H , Singleton AB ((2015) ) Multiple system atrophy is not caused by C9orf72 hexanucleotide repeat expansions. Neurobiol Aging 36: , 1223 e1221–1222. |
[107] | Mitsui J , Matsukawa T , Sasaki H , Yabe I , Matsushima M , Durr A , Brice A , Takashima H , Kikuchi A , Aoki M , Ishiura H , Yasuda T , Date H , Ahsan B , Iwata A , Goto J , Ichikawa Y , Nakahara Y , Momose Y , Takahashi Y , Hara K , Kakita A , Yamada M , Takahashi H , Onodera O , Nishizawa M , Watanabe H , Ito M , Sobue G , Ishikawa K , Mizusawa H , Kanai K , Hattori T , Kuwabara S , Arai K , Koyano S , Kuroiwa Y , Hasegawa K , Yuasa T , Yasui K , Nakashima K , Ito H , Izumi Y , Kaji R , Kato T , Kusunoki S , Osaki Y , Horiuchi M , Kondo T , Murayama S , Hattori N , Yamamoto M , Murata M , Satake W , Toda T , Filla A , Klockgether T , Wullner U , Nicholson G , Gilman S , Tanner CM , Kukull WA , Stern MB , Lee VM , Trojanowski JQ , Masliah E , Low PA , Sandroni P , Ozelius LJ , Foroud T , Tsuji S ((2015) ) Variants associated with Gaucher disease in multiple system atrophy. Ann Clin Transl Neurol 2: , 417–426. |
[108] | Sun QY , Guo JF , Han WW , Zuo X , Wang L , Yao LY , Pan Q , Xia K , Yan XX , Tang BS ((2013) ) Genetic association study of glucocerebrosidase gene L444P mutation in essential tremor and multiple system atrophy in mainland China. J Clin Neurosci 20: , 217–219. |
[109] | Segarane B , Li A , Paudel R , Scholz S , Neumann J , Lees A , Revesz T , Hardy J , Mathias CJ , Wood NW , Holton J , Houlden H ((2009) ) Glucocerebrosidase mutations in 108 neuropathologically confirmed cases of multiple system atrophy. Neurology 72: , 1185–1186. |
[110] | Srulijes K , Hauser AK , Guella I , Asselta R , Brockmann K , Schulte C , Solda G , Cilia R , Maetzler W , Schols L , Wenning GK , Poewe W , Barone P , Wullner U , Oertel W , Berg D , Goldwurm S , Gasser T ((2013) ) No association of GBA mutations and multiple system atrophy. Eur J Neurol 20: , e61–e62. |
[111] | Schottlaender LV , Houlden H ((2014) ) Mutant COQ2 in multiple-system atrophy. N Engl J Med 371: , 81. |
[112] | Chen X , Chen Y , Wei Q , Ou R , Cao B , Zhao B , Shang HF ((2016) ) C9ORF72 repeat expansions in Chinese patients with Parkinson’s disease and multiple system atrophy. J Neural Transm (Vienna) 123: , 1341–1345. |
[113] | Zhao T , Severijnen LA , van der Weiden M , Zheng PP , Oostra BA , Hukema RK , Willemsen R , Kros JM , Bonifati V ((2013) ) FBXO7 immunoreactivity in alpha-synuclein-containing inclusions in Parkinson disease and multiple system atrophy. J Neuropathol Exp Neurol 72: , 482–488. |
[114] | Conedera S , Apaydin H , Li Y , Yoshino H , Ikeda A , Matsushima T , Funayama M , Nishioka K , Hattori N ((2016) ) FBXO7 mutations in Parkinson’s disease and multiple system atrophy. Neurobiol Aging 40: , 192 e191–195. |
[115] | Nishimura M , Kuno S , Kaji R , Kawakami H ((2005) ) Influence of a tumor necrosis factor gene polymorphism in Japanese patients with multiple system atrophy. Neurosci Lett 374: , 218–221. |
[116] | Sailer A , Scholz SW , Nalls MA , Schulte C , Federoff M , Price TR , Lees A , Ross OA , Dickson DW , Mok K , Mencacci NE , Schottlaender L , Chelban V , Ling H , O’Sullivan SS , Wood NW , Traynor BJ , Ferrucci L , Federoff HJ , Mhyre TR , Morris HR , Deuschl G , Quinn N , Widner H , Albanese A , Infante J , Bhatia KP , Poewe W , Oertel W , Hoglinger GU , Wullner U , Goldwurm S , Pellecchia MT , Ferreira J , Tolosa E , Bloem BR , Rascol O , Meissner WG , Hardy JA , Revesz T , Holton JL , Gasser T , Wenning GK , Singleton AB , Houlden H ((2016) ) A genome-wide association study in multiple system atrophy. Neurology 87: , 1591–1598. |
[117] | Chen Y , Chen X , Guo X , Song W , Cao B , Wei Q , Ou R , Zhao B , Shang HF ((2015) ) Assessment of TREM2 rs75932628 association with Parkinson’s disease and multiple system atrophy in a Chinese population. Neurol Sci 36: , 1903–1906. |
[118] | Chen Y , Cao B , Yang J , Wei Q , Ou RW , Zhao B , Song W , Guo X , Shang H ((2015) ) Analysis and meta-analysis of five polymorphisms of the LINGO1 and LINGO2 genes in Parkinson’s disease and multiple system atrophy in a Chinese population. J Neurol 262: , 2478–2483. |
[119] | Ozawa T ((2006) ) Pathology and genetics of multiple system atrophy: An approach to determining genetic susceptibility spectrum. Acta Neuropathol 112: , 531–538. |
[120] | Furiya Y , Hirano M , Kurumatani N , Nakamuro T , Matsumura R , Futamura N , Ueno S ((2005) ) Alpha-1-antichymotrypsin gene polymorphism and susceptibility to multiple system atrophy (MSA). Brain Res Mol Brain Res 138: , 178–181. |
[121] | Soma H , Yabe I , Takei A , Fujiki N , Yanagihara T , Sasaki H ((2008) ) Associations between multiple system atrophy and polymorphisms of SLC1A4, SQSTM1, and EIF4EBP1 genes. Mov Disord 23: , 1161–1167. |
[122] | Mills JD , Kim WS , Halliday GM , Janitz M ((2015) ) Transcriptome analysis of grey and white matter cortical tissue in multiple system atrophy. Neurogenetics 16: , 107–122. |
[123] | Lee ST , Chu K , Jung KH , Ban JJ , Im WS , Jo HY , Park JH , Lim JY , Shin JW , Moon J , Lee SK , Kim M , Roh JK ((2015) ) Altered expression of miR-202 in cerebellum of multiple-system atrophy. Mol Neurobiol 51: , 180–186. |
[124] | Ubhi K , Rockenstein E , Kragh C , Inglis C , Spencer B , Michael S , Mante M , Adame A , Galasko D , Masliah E ((2014) ) Widespread microRNA dysregulation in multiple system atrophy - disease-related alteration in miR-96. Eur J Neurosci 39: , 1026–1041. |
[125] | Wakabayashi K , Mori F , Kakita A , Takahashi H , Tanaka S , Utsumi J , Sasaki H ((2016) ) MicroRNA expression profiles of multiple system atrophy from formalin-fixed paraffin-embedded samples. Neurosci Lett 635: , 117–122. |
[126] | Vallelunga A , Ragusa M , Di Mauro S , Iannitti T , Pilleri M , Biundo R , Weis L , Di Pietro C , De Iuliis A , Nicoletti A , Zappia M , Purrello M , Antonini A ((2014) ) Identification of circulating microRNAs for the differential diagnosis of Parkinson’s disease and Multiple System Atrophy. Front Cell Neurosci 8: , 156. |
[127] | Mills JD , Ward M , Kim WS , Halliday GM , Janitz M ((2016) ) Strand-specific RNA-sequencing analysis of multiple system atrophy brain transcriptome. Neuroscience 322: , 234–250. |
[128] | Wan P , Su W , Zhuo Y ((2017) ) The role of long noncoding RNAs in neurodegenerative diseases. Mol Neurobiol 54: , 2012–2021. |
[129] | Shibao C , Garland EM , Gamboa A , Vnencak-Jones CL , Van Woeltz M , Haines JL , Yu C , Biaggioni I ((2008) ) PRNP M129V homozygosity in multiple system atrophy vs. Parkinson’s disease. Clin Auton Res 18: , 13–19. |
[130] | Prusiner SB , Woerman AL , Mordes DA , Watts JC , Rampersaud R , Berry DB , Patel S , Oehler A , Lowe JK , Kravitz SN , Geschwind DH , Glidden DV , Halliday GM , Middleton LT , Gentleman SM , Grinberg LT , Giles K ((2015) ) Evidence for alpha-synuclein prions causing multiple system atrophy in humans with parkinsonism. Proc Natl Acad Sci U S A 112: , E5308–E5317. |
[131] | Sturm E , Stefanova N ((2014) ) Multiple system atrophy: Genetic or epigenetic? Exp Neurobiol 23: , 277–291. |
[132] | VanderHorst VG , Samardzic T , Saper CB , Anderson MP , Nag S , Schneider JA , Bennett DA , Buchman AS ((2015) ) alpha-Synuclein pathology accumulates in sacral spinal visceral sensory pathways. Ann Neurol 78: , 142–149. |
[133] | Hanna PA , Jankovic J , Kirkpatrick JB ((1999) ) Multiple system atrophy: The putative causative role of environmental toxins. Arch Neurol 56: , 90–94. |
[134] | Vanacore N ((2005) ) Epidemiological evidence on multiple system atrophy. J Neural Transm (Vienna) 112: , 1605–1612. |
[135] | Cho JW , Jeon BS , Jeong D , Choi YJ , Lee JY , Lee HS , Hong SY ((2008) ) Association between parkinsonism and participation in agriculture in Korea. J Clin Neurol 4: , 23–28. |
[136] | Seo JH , Yong SW , Song SK , Lee JE , Sohn YH , Lee PH ((2010) ) A case-control study of multiple system atrophy in Korean patients. Mov Disord 25: , 1953–1959. |
[137] | Vidal JS , Vidailhet M , Elbaz A , Derkinderen P , Tzourio C , Alperovitch A ((2008) ) Risk factors of multiple system atrophy: A case-control study in French patients. Mov Disord 23: , 797–803. |
[138] | Vanacore N , Bonifati V , Fabbrini G , Colosimo C , De Michele G , Marconi R , Stocchi F , Nicholl D , Bonuccelli U , De Mari M , Vieregge P , Meco G ((2005) ) Case-control study of multiple system atrophy. Mov Disord 20: , 158–163. |
[139] | Brown RG , Lacomblez L , Landwehrmeyer BG , Bak T , Uttner I , Dubois B , Agid Y , Ludolph A , Bensimon G , Payan C , Leigh NP ((2010) ) Cognitive impairment in patients with multiple system atrophy and progressive supranuclear palsy. Brain 133: , 2382–2393. |
[140] | Chung EJ , Lee WY , Yoon WT , Kim BJ , Lee GH ((2009) ) MIBG scintigraphy for differentiating Parkinson’s disease with autonomic dysfunction from Parkinsonism-predominant multiple system atrophy. Mov Disord 24: , 1650–1655. |
[141] | Yoshida M ((2007) ) Multiple system atrophy: Alpha-synuclein and neuronal degeneration. Neuropathology 27: , 484–493. |
[142] | Shibuya K , Nagatomo H , Iwabuchi K , Inoue M , Yagishita S , Itoh Y ((2000) ) Asymmetrical temporal lobe atrophy with massive neuronal inclusions in multiple system atrophy. J Neurol Sci 179: , 50–58. |
[143] | Dexter DT , Jenner P , Schapira AH , Marsden CD ((1992) ) Alterations in levels of iron, ferritin, and other trace metals in neurodegenerative diseases affecting the basal ganglia. The Royal Kings and Queens Parkinson’s Disease Research Group. Ann Neurol 32: (Suppl), S94–S100. |
[144] | Matsusue E , Fujii S , Kanasaki Y , Kaminou T , Ohama E , Ogawa T ((2009) ) Cerebellar lesions in multiple system atrophy: Postmortem MR imaging-pathologic correlations. AJNR Am J Neuroradiol 30: , 1725–1730. |
[145] | Bower JH , Dickson DW , Taylor L , Maraganore DM , Rocca WA ((2002) ) Clinical correlates of the pathology underlying parkinsonism: A population perspective. Mov Disord 17: , 910–916. |
[146] | Gilman S , Sima AA , Junck L , Kluin KJ , Koeppe RA , Lohman ME , Little R ((1996) ) Spinocerebellar ataxia type 1 with multiple system degeneration and glial cytoplasmic inclusions. Ann Neurol 39: , 241–255. |
[147] | Jellinger KA , Lantos PL ((2010) ) Papp-Lantos inclusions and the pathogenesis of multiple system atrophy: An update. Acta Neuropathol 119: , 657–667. |
[148] | Wenning GK , Jellinger KA ((2005) ) The role of alpha-synuclein in the pathogenesis of multiple system atrophy. Acta Neuropathol 109: , 129–140. |
[149] | Jellinger KA ((2014) ) Neuropathology of multiple system atrophy: New thoughts about pathogenesis. Mov Disord 29: , 1720–1741. |
[150] | Benarroch EE , Schmeichel AM , Low PA , Parisi JE ((2010) ) Differential involvement of the periaqueductal gray in multiple system atrophy. Auton Neurosci 158: , 111–117. |
[151] | Ozawa T ((2007) ) Morphological substrate of autonomic failure and neurohormonal dysfunction in multiple system atrophy: Impact on determining phenotype spectrum. Acta Neuropathol 114: , 201–211. |
[152] | Wakabayashi K , Mori F , Tanji K , Orimo S , Takahashi H ((2010) ) Involvement of the peripheral nervous system in synucleinopathies, tauopathies and other neurodegenerative proteinopathies of the brain. Acta Neuropathol 120: , 1–12. |
[153] | Kuzdas-Wood D , Irschick R , Theurl M , Malsch P , Mair N , Mantinger C , Wanschitz J , Klimaschewski L , Poewe W , Stefanova N , Wenning GK ((2015) ) Involvement of peripheral nerves in the transgenic PLP-alpha-syn model of multiple system atrophy: Extending the phenotype. PLoS One 10: , e0136575. |
[154] | Cykowski MD , Coon EA , Powell SZ , Jenkins SM , Benarroch EE , Low PA , Schmeichel AM , Parisi JE ((2015) ) Expanding the spectrum of neuronal pathology in multiple system atrophy. Brain 138: , 2293–2309. |
[155] | Jellinger KA , Wenning GK ((2016) ) Overlaps between multiple system atrophy and multiple sclerosis: A novel perspective. Mov Disord 31: , 1767–1771. |
[156] | Ozawa T , Paviour D , Quinn NP , Josephs KA , Sangha H , Kilford L , Healy DG , Wood NW , Lees AJ , Holton JL , Revesz T ((2004) ) The spectrum of pathological involvement of the striatonigral and olivopontocerebellar systems in multiple system atrophy: Clinicopathological correlations. Brain 127: , 2657–2671. |
[157] | Wenning GK , Tison F , Ben Shlomo Y , Daniel SE , Quinn NP ((1997) ) Multiple system atrophy: A review of 203 pathologically proven cases. Mov Disord 12: , 133–147. |
[158] | Halliday GM , Holton JL , Revesz T , Dickson DW ((2011) ) Neuropathology underlying clinical variability in patients with synucleinopathies. Acta Neuropathol 122: , 187–204. |
[159] | Inoue M , Yagishita S , Ryo M , Hasegawa K , Amano N , Matsushita M ((1997) ) The distribution and dynamic density of oligodendroglial cytoplasmic inclusions (GCIs) in multiple system atrophy: A correlation between the density of GCIs and the degree of involvement of striatonigral and olivopontocerebellar systems. Acta Neuropathol 93: , 585–591. |
[160] | Papp MI , Lantos PL ((1994) ) The distribution of oligodendroglial inclusions in multiple system atrophy and its relevance to clinical symptomatology. Brain 117: , 235–243. |
[161] | Tong J , Ang LC , Williams B , Furukawa Y , Fitzmaurice P , Guttman M , Boileau I , Hornykiewicz O , Kish SJ ((2015) ) Low levels of astroglial markers in Parkinson’s disease: Relationship to alpha-synuclein accumulation. Neurobiol Dis 82: , 243–253. |
[162] | Ishizawa K , Komori T , Arai N , Mizutani T , Hirose T ((2008) ) Glial cytoplasmic inclusions and tissue injury in multiple system atrophy: A quantitative study in white matter (olivopontocerebellar system) and gray matter (nigrostriatal system). Neuropathology 28: , 249–257. |
[163] | Brettschneider J , Irwin DJ , Boluda S , Byrne MD , Fang L , Lee EB , Robinson JL , Suh E , Van Deerlin VM , Toledo JB , Grossman M , Hurtig H , Dengler R , Petri S , Lee VM , Trojanowski JQ ((2017) ) Progression of alpha-synuclein pathology in multiple system atrophy of the cerebellar type. Neuropathol Appl Neurobiol 43: , 315–329. |
[164] | Bleasel JM , Halliday GM , Kim WS ((2016) ) Animal modeling an oligodendrogliopathy - multiple system atrophy. Acta Neuropathol Commun 4: , 12. |
[165] | Giasson BI , Mabon ME , Duda JE , Montine TJ , Robertson D , Hurtig HI , Lee VM , Trojanowski JQ ((2003) ) Tau and 14-3-3 in glial cytoplasmic inclusions of multiple system atrophy. Acta Neuropathol 106: , 243–250. |
[166] | Wenning GK , Stefanova N , Jellinger KA , Poewe W , Schlossmacher MG ((2008) ) Multiple system atrophy: A primary oligodendrogliopathy. Ann Neurol 64: , 239–246. |
[167] | Arima K , Murayama S , Mukoyama M , Inose T ((1992) ) Immunocytochemical and ultrastructural studies of neuronal and oligodendroglial cytoplasmic inclusions in multiple system atrophy. 1. Neuronal cytoplasmic inclusions. Acta Neuropathol 83: , 453–460. |
[168] | Burn DJ , Jaros E ((2001) ) Multiple system atrophy: Cellular and molecular pathology. Mol Pathol 54: , 419–426. |
[169] | Nishie M , Mori F , Yoshimoto M , Takahashi H , Wakabayashi K ((2004) ) A quantitative investigation of neuronal cytoplasmic and intranuclear inclusions in the pontine and inferior olivary nuclei in multiple system atrophy. Neuropathol Appl Neurobiol 30: , 546–554. |
[170] | Gai WP , Pountney DL , Power JH , Li QX , Culvenor JG , McLean CA , Jensen PH , Blumbergs PC ((2003) ) alpha-Synuclein fibrils constitute the central core of oligodendroglial inclusion filaments in multiple system atrophy. Exp Neurol 181: , 68–78. |
[171] | Chiba Y , Takei S , Kawamura N , Kawaguchi Y , Sasaki K , Hasegawa-Ishii S , Furukawa A , Hosokawa M , Shimada A ((2012) ) Immunohistochemical localization of aggresomal proteins in glial cytoplasmic inclusions in multiple system atrophy. Neuropathol Appl Neurobiol 38: , 559–571. |
[172] | Ettle B , Schlachetzki JC , Winkler J ((2016) ) Oligodendroglia and myelin in neurodegenerative diseases: More than just bystanders? Mol Neurobiol 53: , 3046–3062. |
[173] | Duda JE , Giasson BI , Gur TL , Montine TJ , Robertson D , Biaggioni I , Hurtig HI , Stern MB , Gollomp SM , Grossman M , Lee VM , Trojanowski JQ ((2000) ) Immunohistochemical and biochemical studies demonstrate a distinct profile of alpha-synuclein permutations in multiple system atrophy. J Neuropathol Exp Neurol 59: , 830–841. |
[174] | Paleologou KE , Oueslati A , Shakked G , Rospigliosi CC , Kim HY , Lamberto GR , Fernandez CO , Schmid A , Chegini F , Gai WP , Chiappe D , Moniatte M , Schneider BL , Aebischer P , Eliezer D , Zweckstetter M , Masliah E , Lashuel HA ((2010) ) Phosphorylation at S87 is enhanced in synucleinopathies, inhibits alpha-synuclein oligomerization, and influences synuclein-membrane interactions. J Neurosci 30: , 3184–3198. |
[175] | Kawamoto Y , Akiguchi I , Nakamura S , Budka H ((2002) ) Accumulation of 14-3-3 proteins in glial cytoplasmic inclusions in multiple system atrophy. Ann Neurol 52: , 722–731. |
[176] | Iwata A , Miura S , Kanazawa I , Sawada M , Nukina N ((2001) ) alpha-Synuclein forms a complex with transcription factor Elk-1. J Neurochem 77: , 239–252. |
[177] | Honjyo Y , Kawamoto Y , Nakamura S , Nakano S , Akiguchi I ((2001) ) P39 immunoreactivity in glial cytoplasmic inclusions in brains with multiple system atrophy. Acta Neuropathol 101: , 190–194. |
[178] | Nakamura S , Kawamoto Y , Nakano S , Akiguchi I , Kimura J ((1998) ) Cyclin-dependent kinase 5 and mitogen-activated protein kinase in glial cytoplasmic inclusions in multiple system atrophy. J Neuropathol Exp Neurol 57: , 690–698. |
[179] | Kato S , Shinozawa T , Takikawa M , Kato M , Hirano A , Awaya A , Ohama E ((2000) ) Midkine, a new neurotrophic factor, is present in glial cytoplasmic inclusions of multiple system atrophy brains. Acta Neuropathol 100: , 481–489. |
[180] | Shibuya K , Uchihara T , Nakamura A , Ishiyama M , Yamaoka K , Yagishita S , Iwabuchi K , Kosaka K ((2003) ) Reversible conformational change of tau2 epitope on exposure to detergent in glial cytoplasmic inclusions of multiple system atrophy. Acta Neuropathol (Berl) 105: , 508–514. |
[181] | Piao YS , Hayashi S , Hasegawa M , Wakabayashi K , Yamada M , Yoshimoto M , Ishikawa A , Iwatsubo T , Takahashi H ((2001) ) Co-localization of alpha-synuclein and phosphorylated tau in neuronal and glial cytoplasmic inclusions in a patient with multiple system atrophy of long duration. Acta Neuropathol 101: , 285–293. |
[182] | Honjo Y , Shirakashi Y , Kawamoto Y , Akiguchi I ((2008) ) Anti-DARPP32 antibody-immunopositive inclusions in the brain of patients with multiple system atrophy. Clin Neuropathol 27: , 309–316. |
[183] | Hishikawa N , Niwa J , Doyu M , Ito T , Ishigaki S , Hashizume Y , Sobue G ((2003) ) Dorfin localizes to the ubiquitylated inclusions in Parkinson’s disease, dementia with Lewy bodies, multiple system atrophy, and amyotrophic lateral sclerosis. Am J Pathol 163: , 609–619. |
[184] | Huang Y , Song YJ , Murphy K , Holton JL , Lashley T , Revesz T , Gai WP , Halliday GM ((2008) ) LRRK2 and parkin immunoreactivity in multiple system atrophy inclusions. Acta Neuropathol 116: , 639–646. |
[185] | Nakamura S , Kawamoto Y , Nakano S , Akiguchi I ((2000) ) Expression of the endocytosis regulatory proteins Rab5 and Rabaptin-5 in glial cytoplasmic inclusions from brains with multiple system atrophy. Clin Neuropathol 19: , 51–56. |
[186] | Oláh J , Bertrand P , Ovadi J ((2017) ) Role of the microtubule-associated TPPP/p25 in Parkinson’s and related diseases and its therapeutic potential. Expert Rev Proteomics 14: , 301–309. |
[187] | Wakabayashi K , Engelender S , Tanaka Y , Yoshimoto M , Mori F , Tsuji S , Ross CA , Takahashi H ((2002) ) Immunocytochemical localization of synphilin-1, an alpha-synuclein-associated protein, in neurodegenerative disorders. Acta Neuropathol 103: , 209–214. |
[188] | Kawamoto Y , Kobayashi Y , Suzuki Y , Inoue H , Tomimoto H , Akiguchi I , Budka H , Martins LM , Downward J , Takahashi R ((2008) ) Accumulation of HtrA2/Omi in neuronal and glial inclusions in brains with alpha-synucleinopathies. J Neuropathol Exp Neurol 67: , 984–993. |
[189] | Pountney DL , Chegini F , Shen X , Blumbergs PC , Gai WP ((2005) ) SUMO-1 marks subdomains within glial cytoplasmic inclusions of multiple system atrophy. Neurosci Lett 381: , 74–79. |
[190] | Terni B , Rey MJ , Boluda S , Torrejon-Escribano B , Sabate MP , Calopa M , van Leeuwen FW , Ferrer I ((2007) ) Mutant ubiquitin and p62 immunoreactivity in cases of combined multiple system atrophy and Alzheimer’s disease. Acta Neuropathol 113: , 403–416. |
[191] | Miki Y , Tanji K , Mori F , Tatara Y , Utsumi J , Sasaki H , Kakita A , Takahashi H , Fimia GM , Wakabayashi K ((2017) ) AMBRA1, a novel alpha-synuclein-binding protein, is implicated in the pathogenesis of multiple system atrophy. Brain Pathol. doi 10.1111/bpa.12461 |
[192] | Odagiri S , Tanji K , Mori F , Kakita A , Takahashi H , Wakabayashi K ((2012) ) Autophagic adapter protein NBR1 is localized in Lewy bodies and glial cytoplasmic inclusions and is involved in aggregate formation in alpha-synucleinopathy. Acta Neuropathol 124: , 173–186. |
[193] | Pountney DL , Dickson TC , Power JH , Vickers JC , West AJ , Gai WP ((2011) ) Association of metallothionein-III with oligodendroglial cytoplasmic inclusions in multiple system atrophy. Neurotox Res 19: , 115–122. |
[194] | Okita Y , Rcom-H’cheo-Gauthier AN , Goulding M , Chung RS , Faller P , Pountney DL ((2017) ) Metallothionein, copper and alpha-synuclein in alpha-synucleinopathies. Front Neurosci 11: , 114. |
[195] | Pountney DL , Treweek TM , Chataway T , Huang Y , Chegini F , Blumbergs PC , Raftery MJ , Gai WP ((2005) ) Alpha B-crystallin is a major component of glial cytoplasmic inclusions in multiple system atrophy. Neurotox Res 7: , 77–85. |
[196] | Tanji K , Tanaka T , Mori F , Kito K , Takahashi H , Wakabayashi K , Kamitani T ((2006) ) NUB1 suppresses the formation of Lewy body-like inclusions by proteasomal degradation of synphilin-1. Am J Pathol 169: , 553–565. |
[197] | Tanji K , Mori F , Kakita A , Zhang H , Kito K , Kamitani T , Takahashi H , Wakabayashi K ((2007) ) Immunohistochemical localization of NUB1, a synphilin-1-binding protein, in neurodegenerative disorders. Acta Neuropathol 114: , 365–371. |
[198] | Taylor JM , Song YJ , Huang Y , Farrer MJ , Delatycki MB , Halliday GM , Lockhart PJ ((2007) ) Parkin Co-Regulated Gene (PACRG) is regulated by the ubiquitin-proteasomal system and is present in the pathological features of Parkinsonian diseases. Neurobiol Dis 27: , 238–247. |
[199] | Honjo Y , Ito H , Horibe T , Takahashi R , Kawakami K ((2011) ) Protein disulfide isomerase immunopositive glial cytoplasmic inclusions in patients with multiple system atrophy. Int J Neurosci 121: , 543–550. |
[200] | Kawamoto Y , Ito H , Ihara M , Takahashi R ((2014) ) XIAP immunoreactivity in glial and neuronal cytoplasmic inclusions in multiple system atrophy. Clin Neuropathol 33: , 76–83. |
[201] | Schlossmacher MG , Frosch MP , Gai WP , Medina M , Sharma N , Forno L , Ochiishi T , Shimura H , Sharon R , Hattori N , Langston JW , Mizuno Y , Hyman BT , Selkoe DJ , Kosik KS ((2002) ) Parkin localizes to the Lewy bodies of Parkinson disease and dementia with Lewy bodies. Am J Pathol 160: , 1655–1667. |
[202] | Mollenhauer B , Cullen V , Kahn I , Krastins B , Outeiro TF , Pepivani I , Ng J , Schulz-Schaeffer W , Kretzschmar HA , McLean PJ , Trenkwalder C , Sarracino DA , Vonsattel JP , Locascio JJ , El-Agnaf OM , Schlossmacher MG ((2008) ) Direct quantification of CSF alpha-synuclein by ELISA and first cross-sectional study in patients with neurodegeneration. Exp Neurol 213: , 315–325. |
[203] | Gai WP , Power JH , Blumbergs PC , Culvenor JG , Jensen PH ((1999) ) Alpha-synuclein immunoisolation of glial inclusions from multiple system atrophy brain tissue reveals multiprotein components. J Neurochem 73: , 2093–2100. |
[204] | Uchihara T , Nakamura A , Mochizuki Y , Hayashi M , Orimo S , Isozaki E , Mizutani T ((2005) ) Silver stainings distinguish Lewy bodies and glial cytoplasmic inclusions: Comparison between Gallyas-Braak and Campbell-Switzer methods. Acta Neuropathol 110: , 255–260. |
[205] | McCormack A , Chegeni N , Chegini F , Colella A , Power J , Keating D , Chataway T ((2016) ) Purification of alpha-synuclein containing inclusions from human post mortem brain tissue. J Neurosci Methods 266: , 141–150. |
[206] | Bassil F , Canron MH , Vital A , Bezard E , Li Y , Greig NH , Gulyani S , Kapogiannis D , Fernagut PO , Meissner WG ((2017) ) Insulin resistance and exendin-4 treatment for multiple system atrophy. Brain 140: , 1420–1436. |
[207] | Nishie M , Mori F , Fujiwara H , Hasegawa M , Yoshimoto M , Iwatsubo T , Takahashi H , Wakabayashi K ((2004) ) Accumulation of phosphorylated alpha-synuclein in the brain and peripheral ganglia of patients with multiple system atrophy. Acta Neuropathol 107: , 292–298. |
[208] | Sone M , Yoshida M , Hashizume Y , Hishikawa N , Sobue G ((2005) ) alpha-Synuclein-immunoreactive structure formation is enhanced in sympathetic ganglia of patients with multiple system atrophy. Acta Neuropathol 110: , 19–26. |
[209] | Holton JL , Lees AL , Revesz T ((2011) ) Multiple system atrophy. In Neurodegeneration: The Molecular Pathology of Dementia and Movement Disorders, 2nd Edition, Dickson DW, Weller RO, eds. Blackwell Publishing Ltd., Oxford, pp. 242–252. |
[210] | Nakamura K , Mori F , Kon T , Tanji K , Miki Y , Tomiyama M , Kurotaki H , Toyoshima Y , Kakita A , Takahashi H , Yamada M , Wakabayashi K ((2015) ) Accumulation of phosphorylated alpha-synuclein in subpial and periventricular astrocytes in multiple system atrophy of long duration. Neuropathology 36: , 157–167. |
[211] | Koga S , Kasanuki K , Dickson DW ((2017) ) Alpha-synuclein astrogliopathy: A possible specific feature in alpha-synucleinopathy. Neuropathol 37: , 379–381. |
[212] | Armstrong RA , Cairns NJ , Lantos PL ((2004) ) A quantitative study of the pathological changes in ten patients with multiple system atrophy (MSA). J Neural Transm (Vienna) 111: , 485–495. |
[213] | Jellinger KA , Seppi K , Wenning GK ((2005) ) Grading of neuropathology in multiple system atrophy: Proposal for a novel scale. Mov Disord 20: (Suppl 12), S29–S36. |
[214] | Salvesen L , Ullerup BH , Sunay FB , Brudek T , Lokkegaard A , Agander TK , Winge K , Pakkenberg B ((2015) ) Changes in total cell numbers of the basal ganglia in patients with multiple system atrophy - A stereological study. Neurobiol Dis 74: , 104–113. |
[215] | Ozawa T , Tada M , Kakita A , Onodera O , Ishihara T , Morita T , Shimohata T , Wakabayashi K , Takahashi H , Nishizawa M ((2010) ) The phenotype spectrum of Japanese multiple system atrophy. J Neurol Neurosurg Psychiatry 81: , 1253–1255. |
[216] | Dickson DW ((2012) ) Parkinson’s disease and parkinsonism: Neuropathology. Cold Spring Harb Perspect Med 2: , a009258. |
[217] | Seppi K , Schocke MF , Mair KJ , Esterhammer R , Scherfler C , Geser F , Kremser C , Boesch S , Jaschke W , Poewe W , Wenning GK ((2006) ) Progression of putaminal degeneration in multiple system atrophy: A serial diffusion MR study. Neuroimage 31: , 240–245. |
[218] | Tsuchiya K , Ozawa E , Haga C , Watabiki S , Ikeda M , Sano M , Ooe K , Taki K , Ikeda K ((2000) ) Constant involvement of the Betz cells and pyramidal tract in multiple system atrophy: A clinicopathological study of seven autopsy cases. Acta Neuropathol 99: , 628–636. |
[219] | Brenneis C , Egger K , Scherfler C , Seppi K , Schocke M , Poewe W , Wenning GK ((2007) ) Progression of brain atrophy in multiple system atrophy. A longitudinal VBM study. J Neurol 254: , 191–196. |
[220] | Fujita T, Doi M, Ogata T, Kanazawa I, Mizusawa H ((1993) ) Cerebral cortical pathology of sporadic olivopontocerebellar atrophy. J Neurol Sci 116: , 41–46. |
[221] | Konagaya M , Sakai M , Matsuoka Y , Konagaya Y , Hashizume Y ((1999) ) Multiple system atrophy with remarkable frontal lobe atrophy. Acta Neuropathol 97: , 423–428. |
[222] | Konagaya M , Konagaya Y , Sakai M , Matsuoka Y , Hashizume Y ((2002) ) Progressive cerebral atrophy in multiple system atrophy. J Neurol Sci 195: , 123–127. |
[223] | Wakabayashi K , Hayashi S , Kakita A , Yamada M , Toyoshima Y , Yoshimoto M , Takahashi H ((1998) ) Accumulation of alpha-synuclein/NACP is a cytopathological feature common to Lewy body disease and multiple system atrophy. Acta Neuropathol 96: , 445–452. |
[224] | Armstrong RA , Lantos PL , Cairns NJ ((2005) ) Multiple system atrophy: Laminar distribution of the pathological changes in frontal and temporal neocortex–a study in ten patients. Clin Neuropathol 24: , 230–235. |
[225] | Salvesen L , Winge K , Brudek T , Agander TK , Lokkegaard A , Pakkenberg B ((2017) ) Neocortical neuronal loss in patients with multiple system atrophy: A stereological study. Cereb Cortex 27: , 400–410. |
[226] | Kim HJ , Jeon BS , Kim YE , Kim JY , Kim YK , Sohn CH , Yun JY , Jeon S , Lee JM , Lee JY ((2013) ) Clinical and imaging characteristics of dementia in multiple system atrophy. Parkinsonism Relat Disord 19: , 617–621. |
[227] | Cao B , Zhao B , Wei QQ , Chen K , Yang J , Ou R , Wu Y , Shang HF ((2015) ) The global cognition, frontal lobe dysfunction and behavior changes in Chinese patients with multiple system atrophy. PLoS One 10: , e0139773. |
[228] | Kim JS , Yang JJ , Lee DK , Lee JM , Youn J , Cho JW ((2015) ) Cognitive impairment and its structural correlates in the parkinsonian subtype of multiple system atrophy. Neurodegener Dis 15: , 294–300. |
[229] | Fiorenzato E , Weis L , Seppi K , Onofrj M , Cortelli P , Zanigni S , Tonon C , Kaufmann H , Shepherd TM , Poewe W , Krismer F , Wenning G , Antonini A , Biundo R ((2017) ) Brain structural profile of multiple system atrophy patients with cognitive impairment. J Neural Transm (Vienna) 124: , 293–302. |
[230] | Homma T , Mochizuki Y , Komori T , Isozaki E ((2016) ) Frequent globular neuronal cytoplasmic inclusions in the medial temporal region as a possible characteristic feature in multiple system atrophy with dementia. Neuropathology 36: , 421–431. |
[231] | Saito M , Hara M , Ebashi M , Morita A , Okada K , Homma T , Sugitani M , Endo K , Uchihara T , Kamei S ((2017) ) Perirhinal accumulation of neuronal alpha-synuclein in a multiple system atrophy patient with dementia. Neuropathology. doi 10.1111/neup.12381 |
[232] | Asi YT , Ling H , Ahmed Z , Lees AJ , Revesz T , Holton JL ((2014) ) Neuropathological features of multiple system atrophy with cognitive impairment. Mov Disord 29: , 884–888. |
[233] | Koga S , Parks A , Uitti RJ , van Gerpen JA , Cheshire WP , Wszolek ZK , Dickson DW ((2017) ) Profile of cognitive impairment and underlying pathology in multiple system atrophy. Mov Disord 32: , 405–413. |
[234] | Kovacs T , Papp MI , Cairns NJ , Khan MN , Lantos PL ((2003) ) Olfactory bulb in multiple system atrophy. Mov Disord 18: , 938–942. |
[235] | Mendoza-Santiesteban CE , Palma JA , Martinez J , Norcliffe-Kaufmann L , Hedges TR 3rd , Kaufmann H ((2015) ) Progressive retinal structure abnormalities in multiple system atrophy. Mov Disord 30: , 1944–1953. |
[236] | Mendoza-Santiesteban CE , Palma JA , Ortuno-Lizaran I , Cuenca N , Kaufmann H ((2017) ) Pathologic confirmation of retinal ganglion cell loss in multiple system atrophy. Neurology 88: , 2233–2235. |
[237] | Fischer MD , Synofzik M , Kernstock C , Dietzsch J , Heidlauf R , Schicks J , Srulijes K , Wiethoff S , Menn O , Berg D , Schols L , Schiefer U ((2013) ) Decreased retinal sensitivity and loss of retinal nerve fibers in multiple system atrophy. Graefes Arch Clin Exp Ophthalmol 251: , 235–241. |
[238] | Mendoza-Santiesteban CE , Gabilondo I , Palma JA , Norcliffe-Kaufmann L , Kaufmann H ((2017) ) The retina in multiple system atrophy: Systematic review and meta-analysis. Front Neurol 8: , 206. |
[239] | Wang PS , Yeh CL , Lu CF , Wu HM , Soong BW , Wu YT ((2017) ) The involvement of supratentorial white matter in multiple system atrophy: A diffusion tensor imaging tractography study. Acta Neurol Belg 117: , 213–220. |
[240] | Brooks DJ , Seppi K ((2009) ) Proposed neuroimaging criteria for the diagnosis of multiple system atrophy. Mov Disord 24: , 949–964. |
[241] | Prodoehl J , Li H , Planetta PJ , Goetz CG , Shannon KM , Tangonan R , Comella CL , Simuni T , Zhou XJ , Leurgans S , Corcos DM , Vaillancourt DE ((2013) ) Diffusion tensor imaging of Parkinson’s disease, atypical parkinsonism, and essential tremor. Mov Disord 28: , 1816–1822. |
[242] | Blain CR , Barker GJ , Jarosz JM , Coyle NA , Landau S , Brown RG , Chaudhuri KR , Simmons A , Jones DK , Williams SC , Leigh PN ((2006) ) Measuring brain stem and cerebellar damage in parkinsonian syndromes using diffusion tensor MRI. Neurology 67: , 2199–2205. |
[243] | Schocke MF , Seppi K , Esterhammer R , Kremser C , Jaschke W , Poewe W , Wenning GK ((2002) ) Diffusion-weighted MRI differentiates the Parkinson variant of multiple system atrophy from PD. Neurology 58: , 575–580. |
[244] | Shiga K , Yamada K , Yoshikawa K , Mizuno T , Nishimura T , Nakagawa M ((2005) ) Local tissue anisotropy decreases in cerebellopetal fibers and pyramidal tract in multiple system atrophy. J Neurol 252: , 589–596. |
[245] | Paviour DC , Thornton JS , Lees AJ , Jager HR ((2007) ) Diffusion-weighted magnetic resonance imaging differentiates Parkinsonian variant of multiple-system atrophy from progressive supranuclear palsy. Mov Disord 22: , 68–74. |
[246] | Don AS , Hsiao JH , Bleasel JM , Couttas TA , Halliday GM , Kim W ((2014) ) Altered lipid levels provide evidence for myelin dysfunction in multiple system atrophy. Acta Neuropathol Commun 2: , 150. |
[247] | Lin DJ , Hermann KL , Schmahmann JD ((2014) ) Multiple system atrophy of the cerebellar type: Clinical state of the art. Mov Disord 29: , 294–304. |
[248] | Indelicato E , Fanciulli A , Poewe W , Antonini A , Pontieri FE , Wenning GK ((2015) ) Cerebral autoregulation and white matter lesions in Parkinson’s disease and multiple system atrophy. Parkinsonism Relat Disord 21: , 1393–1397. |
[249] | Bruck D , Wenning GK , Stefanova N , Fellner L ((2016) ) Glia and alpha-synuclein in neurodegeneration: A complex interaction. Neurobiol Dis 85: , 262–274. |
[250] | Song YJ , Halliday GM , Holton JL , Lashley T , O’Sullivan SS , McCann H , Lees AJ , Ozawa T , Williams DR , Lockhart PJ , Revesz TR ((2009) ) Degeneration in different parkinsonian syndromes relates to astrocyte type and astrocyte protein expression. J Neuropathol Exp Neurol 68: , 1073–1083. |
[251] | Tong J , Wong H , Guttman M , Ang LC , Forno LS , Shimadzu M , Rajput AH , Muenter MD , Kish SJ , Hornykiewicz O , Furukawa Y ((2010) ) Brain alpha-synuclein accumulation in multiple system atrophy, Parkinson’s disease and progressive supranuclear palsy: A comparative investigation. Brain 133: , 172–188. |
[252] | Tong J , Rathitharan G , Meyer JH , Furukawa Y , Ang L-C , Boileau I , Guttman M , Hornykiewicz O , Kish SJ ((2017) ) Brain monoamine oxidase B and A in human parkinsonian dopamine deficiency disorders. Brain 140: , 2460–2474. |
[253] | Gerhard A , Pavese N , Hotton G , Turkheimer F , Es M , Hammers A , Eggert K , Oertel W , Banati RB , Brooks DJ ((2006) ) In vivo imaging of microglial activation with [11C](R)-PK11195 PET in idiopathic Parkinson’s disease. Neurobiol Dis 21: , 404–412. |
[254] | Ishizawa K , Komori T , Sasaki S , Arai N , Mizutani T , Hirose T ((2004) ) Microglial activation parallels system degeneration in multiple system atrophy. J Neuropathol Exp Neurol 63: , 43–52. |
[255] | Gerhard A , Banati RB , Goerres GB , Cagnin A , Myers R , Gunn RN , Turkheimer F , Good CD , Mathias CJ , Quinn N , Schwarz J , Brooks DJ ((2003) ) [11C](R)-PK11195 PET imaging of microglial activation in multiple system atrophy. Neurology 61: , 686–689. |
[256] | Hoffmann A , Ettle B , Bruno A , Kulinich A , Hoffmann AC , von Wittgenstein J , Winkler J , Xiang W , Schlachetzki JC ((2016) ) Alpha-synuclein activates BV2 microglia dependent on its aggregation state. Biochem Biophys Res Commun 479: , 881–886. |
[257] | Stefanova N , Reindl M , Neumann M , Kahle PJ , Poewe W , Wenning GK ((2007) ) Microglial activation mediates neurodegeneration related to oligodendroglial alpha-synucleinopathy: Implications for multiple system atrophy. Mov Disord 22: , 2196–2203. |
[258] | Stefanova N , Kaufmann WA , Humpel C , Poewe W , Wenning GK ((2012) ) Systemic proteasome inhibition triggers neurodegeneration in a transgenic mouse model expressing human alpha-synuclein under oligodendrocyte promoter: Implications for multiple system atrophy. Acta Neuropathol 124: , 51–65. |
[259] | Kiely AP , Asi YT , Ahmed Z , Revesz T , Holton JL ((2014) ) Microglial activation in multiple system atrophy (abs.). Neuropathol Appl Neurobiol 40: (Suppl 1), 37. |
[260] | Nykjaer C , Brudek T , Salvesen L , Pakkenberg B ((2017) ) Changes in the cell population in brain white matter in multiple system atrophy. Mov Disord 32: , 1074–1082. |
[261] | Fellner L , Stefanova N ((2013) ) The role of glia in alpha-synucleinopathies. Mol Neurobiol 47: , 575–586. |
[262] | von Bernhardi R , Eugenin-von Bernhardi L , Eugenin J ((2015) ) Microglial cell dysregulation in brain aging and neurodegeneration. Front Aging Neurosci 7: , 124. |
[263] | Stefanova N , Fellner L , Reindl M , Masliah E , Poewe W , Wenning GK ((2011) ) Toll-like receptor 4 promotes alpha-synuclein clearance and survival of nigral dopaminergic neurons. Am J Pathol 179: , 954–963. |
[264] | Fellner L , Irschick R , Schanda K , Reindl M , Klimaschewski L , Poewe W , Wenning GK , Stefanova N ((2013) ) Toll-like receptor 4 is required for alpha-synuclein dependent activation of microglia and astroglia. Glia 61: , 349–360. |
[265] | Iodice V , Lipp A , Ahlskog JE , Sandroni P , Fealey RD , Parisi JE , Matsumoto JY , Benarroch EE , Kimpinski K , Singer W , Gehrking TL , Gehrking JA , Sletten DM , Schmeichel AM , Bower JH , Gilman S , Figueroa J , Low PA ((2012) ) Autopsy confirmed multiple system atrophy cases: Mayo experience and role of autonomic function tests. J Neurol Neurosurg Psychiatry 83: , 453–459. |
[266] | Coon E , Cutsforth-Gregory J , Benarroch E ((2017) ) Neuropathology of autonomic dysfunction in synucleinopathies. Mov Disord 33: , 349–358. |
[267] | Benarroch EE , Schmeichel AM , Sandroni P , Low PA , Parisi JE ((2006) ) Involvement of vagal autonomic nuclei in multiple system atrophy and Lewy body disease. Neurology 66: , 378–383. |
[268] | Kuzdas D , Stemberger S , Gaburro S , Stefanova N , Singewald N , Wenning GK ((2013) ) Oligodendroglial alpha-synucleinopathy and MSA-like cardiovascular autonomic failure: Experimental evidence. Exp Neurol 247: , 531–536. |
[269] | Schmeichel AM , Buchhalter LC , Low PA , Parisi JE , Boeve BW , Sandroni P , Benarroch EE ((2008) ) Mesopontine cholinergic neuron involvement in Lewy body dementia and multiple system atrophy. Neurology 70: , 368–373. |
[270] | Benarroch EE , Schmeichel AM , Dugger BN , Sandroni P , Parisi JE , Low PA ((2009) ) Dopamine cell loss in the periaqueductal gray in multiple system atrophy and Lewy body dementia. Neurology 73: , 106–112. |
[271] | Noda K , Katayama S , Watanabe C , Yamamura Y , Nakamura S ((1997) ) Decrease of neurons in the medullary arcuate nucleus of multiple system atrophy: Quantitative comparison with Parkinson’s disease and amyotrophic lateral sclerosis. J Neurol Sci 151: , 89–91. |
[272] | Benarroch EE , Schmeichel AM , Low PA , Parisi JE ((2004) ) Involvement of medullary serotonergic groups in multiple system atrophy. Ann Neurol 55: , 418–422. |
[273] | Benarroch EE , Schmeichel AM , Low PA , Parisi JE ((2003) ) Depletion of ventromedullary NK-1 receptor-immunoreactive neurons in multiple system atrophy. Brain 126: , 2183–2190. |
[274] | Benarroch EE , Schmeichel AM , Low PA , Parisi JE ((2007) ) Depletion of putative chemosensitive respiratory neurons in the ventral medullary surface in multiple system atrophy. Brain 130: , 469–475. |
[275] | Benarroch EE , Schmeichel AM , Parisi JE ((2002) ) Depletion of mesopontine cholinergic and sparing of raphe neurons in multiple system atrophy. Neurology 59: , 944–946. |
[276] | Benarroch EE , Schmeichel AM , Parisi JE , Low PA ((2015) ) Putative neuropathological interactions in MSA: Focus in the rostral ventrolateral medulla. Clin Auton Res 25: , 77–80. |
[277] | Benarroch EE , Schmeichel AM , Low PA , Sandroni P , Parisi JE ((2008) ) Loss of A5 noradrenergic neurons in multiple system atrophy. Acta Neuropathol 115: , 629–634. |
[278] | Tada M , Kakita A , Toyoshima Y , Onodera O , Ozawa T , Morita T , Nishizawa M , Takahashi H ((2009) ) Depletion of medullary serotonergic neurons in patients with multiple system atrophy who succumbed to sudden death. Brain 132: , 1810–1819. |
[279] | Benarroch EE ((2010) ) Neural control of the bladder: Recent advances and neurologic implications. Neurology 75: , 1839–1846. |
[280] | Shy G , Drager GA ((1960) ) A neurological syndrome associated with orthostatic hypotension. A clinicopathological study. Arch Neurol 2: , 511–527. |
[281] | Benarroch EE , Schmeichel AM , Parisi JE , Low PA ((2015) ) Histaminergic tuberomammillary neuron loss in multiple system atrophy and dementia with Lewy bodies. Mov Disord 30: , 1133–1139. |
[282] | Nakamura S , Ohnishi K , Nishimura M , Suenaga T , Akiguchi I , Kimura J , Kimura T ((1996) ) Large neurons in the tuberomammillary nucleus in patients with Parkinson’s disease and multiple system atrophy. Neurology 46: , 1693–1696. |
[283] | Wenning GK , Tison F , Elliott L , Quinn NP , Daniel SE ((1996) ) Olivopontocerebellar pathology in multiple system atrophy. Mov Disord 11: , 157–162. |
[284] | Benarroch EE , Schmeichel AM , Parisi JE ((2003) ) Preservation of branchimotor neurons of the nucleus ambiguus in multiple system atrophy. Neurology 60: , 115–117. |
[285] | Coon EA , Schmeichel AM , Parisi JE , Cykowski MD , Low PA , Benarroch EE ((2016) ) Medullary neuronal loss is not associated with alpha-synuclein burden in multiple system atrophy. Mov Disord 31: , 1802–1809. |
[286] | Orimo S , Kanazawa T , Nakamura A , Uchihara T , Mori F , Kakita A , Wakabayashi K , Takahashi H ((2007) ) Degeneration of cardiac sympathetic nerve can occur in multiple system atrophy. Acta Neuropathol 113: , 81–86. |
[287] | Gray F , Vincent D , Hauw JJ ((1988) ) Quantitative study of lateral horn cells in 15 cases of multiple system atrophy. Acta Neuropathol 75: , 513–518. |
[288] | Yamamoto T , Sakakibara R , Uchiyama T , Liu Z , Ito T , Awa Y , Yamamoto K , Kinou M , Yamanishi T , Hattori T ((2005) ) When is Onuf’s nucleus involved in multiple system atrophy? A sphincter electromyography study. J Neurol Neurosurg Psychiatry 76: , 1645–1648. |
[289] | Konno H , Yamamoto T , Iwasaki Y , Iizuka H ((1986) ) Shy-Drager syndrome and amyotrophic lateral sclerosis. Cytoarchitectonic and morphometric studies of sacral autonomic neurons. J Neurol Sci 73: , 193–204. |
[290] | Sima AA , Caplan M , D’Amato CJ , Pevzner M , Furlong JW ((1993) ) Fulminant multiple system atrophy in a young adult presenting as motor neuron disease. Neurology 43: , 2031–2035. |
[291] | Su M , Yoshida Y , Hirata Y , Watahiki Y , Nagata K ((2001) ) Primary involvement of the motor area in association with the nigrostriatal pathway in multiple system atrophy: Neuropathological and morphometric evaluations. Acta Neuropathol 101: , 57–64. |
[292] | Doppler K , Weis J , Karl K , Ebert S , Ebentheuer J , Trenkwalder C , Klebe S , Volkmann J , Sommer C ((2015) ) Distinctive distribution of phospho-alpha-synuclein in dermal nerves in multiple system atrophy. Mov Disord 30: , 1688–1692. |
[293] | Zange L , Noack C , Hahn K , Stenzel W , Lipp A ((2015) ) Phosphorylated alpha-synuclein in skin nerve fibres differentiates Parkinson’s disease from multiple system atrophy. Brain 138: , 2310–2321. |
{ label needed for ref[@id='ref293a'] } | Nakamura K , Mori F , Kon T , Tanji K , Miki Y , Tomiyama M , Kurotaki H , Toyoshima Y , Kakita A , Takahashi H , Yamada M , Wakabayashi K ((2015) ) Filamentous aggregations of phosphorylated alpha-synuclein in Schwann cells (Schwann cell cytoplasmic inclusions) in multiple system atrophy. Acta Neuropathol Commun 3: , 29. |
{ label needed for ref[@id='ref293b'] } | Donadio V , Incensi A , Rizzo G , Capellari S , Pantieri R , Stanzani Maserati M , Devigili G , Eleopra R , Defazio G , Montini F , Baruzzi A , Liguori R ((2017) ) A new potential biomarker for dementia with Lewy bodies: Skin nerve alpha-synuclein deposits. Neurol 89: , 318–326. |
[294] | Haga R , Sugimoto K , Nishijima H , Miki Y , Suzuki C , Wakabayashi K , Baba M , Yagihashi S , Tomiyama M ((2015) ) Clinical utility of skin biopsy in differentiating between Parkinson’s disease and multiple system atrophy. Parkinsons Dis 2015: , 167038. |
[295] | Mori F , Inenaga C , Yoshimoto M , Umezu H , Tanaka R , Takahashi H , Wakabayashi K ((2002) ) Alpha-synuclein immunoreactivity in normal and neoplastic Schwann cells. Acta Neuropathol 103: , 145–151. |
[296] | Provitera V , Nolano M , Caporaso G , Stancanelli A , Manganelli F , Iodice R , Selim MM , De Rosa A , Lanzillo B , Pellecchia MT , De Michele G , Santoro L ((2014) ) Postganglionic sudomotor denervation in patients with multiple system atrophy. Neurology 82: , 2223–2229. |
{ label needed for ref[@id='ref296a'] } | Coon EA , Fealey RD , Sletten DM , Mandrekar JN , Benarroch EE , Sandroni P , Low PA , Singer W ((2017) ) Anhidrosis in multiple system atrophy involves pre- and postganglionic sudomotor dysfunction. Mov Disord 32: , 397–404. |
[297] | Pouclet H , Lebouvier T , Coron E , Rouaud T , Flamant M , Toulgoat F , Roy M , Vavasseur F , Bruley des Varannes S , Neunlist M , Derkinderen P ((2012) ) Analysis of colonic alpha-synuclein pathology in multiple system atrophy. Parkinsonism Relat Disord 18: , 893–895. |
[298] | Fearnley JM , Lees AJ ((1990) ) Striatonigral degeneration. A clinicopathological study. Brain 113: (Pt 6), 1823–1842. |
[299] | Visanji NP , Collingwood JF , Finnegan ME , Tandon A , House E , Hazrati LN ((2013) ) Iron deficiency in parkinsonism: Region-specific iron dysregulation in Parkinson’s disease and multiple system atrophy. J Parkinsons Dis 3: , 523–537. |
[300] | Lee JH , Han YH , Kang BM , Mun CW , Lee SJ , Baik SK ((2013) ) Quantitative assessment of subcortical atrophy and iron content in progressive supranuclear palsy and parkinsonian variant of multiple system atrophy. J Neurol 260: , 2094–2101. |
[301] | Ebrahim AS , Kulathingal J , Murray ME , Casey-Castanedes M , Dickson DW , Yen SH , Sevlever D ((2011) ) A proteomic study identifies different levels of light chain ferritin in corticobasal degeneration and progressive supranuclear palsy. Acta Neuropathol 122: , 727–736. |
[302] | Kaindlstorfer C , Jellinger KA , Eschlböck S , Stefanova N , Weiss G , Wenning GK ((2018) ) The relevance of iron in the pathogenesis of multiple system atrophy: A viewpoint. J Alzheimers Dis 61: , 1253–1273. |
[303] | Schwarz J , Weis S , Kraft E , Tatsch K , Bandmann O , Mehraein P , Vogl T , Oertel WH ((1996) ) Signal changes on MRI and increases in reactive microgliosis, astrogliosis, and iron in the putamen of two patients with multiple system atrophy. J Neurol Neurosurg Psychiatry 60: , 98–101. |
[304] | Lang AE , Curran T , Provias J , Bergeron C ((1994) ) Striatonigral degeneration: Iron deposition in putamen correlates with the slit-like void signal of magnetic resonance imaging. Can J Neurol Sci 21: , 311–318. |
[305] | Dexter DT , Carayon A , Javoy-Agid F , Agid Y , Wells FR , Daniel SE , Lees AJ , Jenner P , Marsden CD ((1991) ) Alterations in the levels of iron, ferritin and other trace metals in Parkinson’s disease and other neurodegenerative diseases affecting the basal ganglia. Brain 114: (Pt 4), 1953–1975. |
[306] | Watanabe H , Fukatsu H , Hishikawa N , Hashizume Y , Sobue G ((2004) ) Field strengths and sequences influence putaminal MRI findings in multiple system atrophy. Neurology 62: , 671. |
[307] | Jellinger KA ((2003) ) Neuropathological spectrum of synucleinopathies.. Mov Disord 18: (Suppl 6), S2–12. |
[308] | Watanabe H , Riku Y , Nakamura T , Hara K , Ito M , Hirayama M , Yoshida M , Katsuno M , Sobue G ((2016) ) Expanding concept of clinical conditions and symptoms in multiple system atrophy. Rinsho Shinkeigaku 56: , 457–464. |
[309] | Berciano J , Valldeoriola F , Ferrer I , Rumia J , Pascual J , Marin C , Rey MJ , Tolosa E ((2002) ) Presynaptic parkinsonism in multiple system atrophy mimicking Parkinson’s disease: A clinicopathological case study. Mov Disord 17: , 812–816. |
[310] | Kon T , Mori F , Tanji K , Miki Y , Wakabayashi K ((2013) ) An autopsy case of preclinical multiple system atrophy (MSA-C). Neuropathology 33: , 667–672. |
[311] | Wenning GK , Ben Shlomo Y , Magalhaes M , Daniel SE , Quinn NP ((1994) ) Clinical features and natural history of multiple system atrophy. An analysis of 100 cases. Brain 117: , 835–845. |
[312] | Ling H , Asi YT , Petrovic IN , Ahmed Z , Prashanth LK , Hazrati LN , Nishizawa M , Ozawa T , Lang A , Lees AJ , Revesz T , Holton JL ((2015) ) Minimal change multiple system atrophy: An aggressive variant? Mov Disord 30: , 960–967. |
[313] | Wakabayashi K , Mori F , Nishie M , Oyama Y , Kurihara A , Yoshimoto M , Kuroda N ((2005) ) An autopsy case of early (“minimal change”) olivopontocerebellar atrophy (multiple system atrophy-cerebellar). Acta Neuropathol 110: , 185–190. |
[314] | Rodriguez-Diehl R , Rey MJ , Gironell A , Martinez-Saez E , Ferrer I , Sanchez-Valle R , Jague J , Nos C , Gelpi E ((2012) ) “Preclinical” MSA in definite Creutzfeldt-Jakob disease. Neuropathology 32: , 158–163. |
[315] | Fujishiro H , Ahn TB , Frigerio R , DelleDonne A , Josephs KA , Parisi JE , Eric Ahlskog J , Dickson DW ((2008) ) Glial cytoplasmic inclusions in neurologically normal elderly: Prodromal multiple system atrophy? Acta Neuropathol 116: , 269–275. |
[316] | Parkkinen L , Hartikainen P , Alafuzoff I ((2007) ) Abundant glial alpha-synuclein pathology in a case without overt clinical symptoms. Clin Neuropathol 26: , 276–283. |
[317] | DelleDonne A , Klos KJ , Fujishiro H , Ahmed Z , Parisi JE , Josephs KA , Frigerio R , Burnett M , Wszolek ZK , Uitti RJ , Ahlskog JE , Dickson DW ((2008) ) Incidental Lewy body disease and preclinical Parkinson disease. Arch Neurol 65: , 1074–1080. |
[318] | Kim HJ , Jeon BS ((2012) ) Multiple system atrophy with prolonged survival. Mov Disord 27: , 1834. |
[319] | Calandra-Buonaura G , Guaraldi P , Sambati L , Lopane G , Cecere A , Barletta G , Provini F , Contin M , Martinelli P , Cortelli P ((2013) ) Multiple system atrophy with prolonged survival: Is late onset of dysautonomia the clue? Neurol Sci 34: , 1875–1878. |
[320] | Kim HJ , Jeon BS , Lee JY , Yun JY , Kim YE , Paek SH ((2012) ) Young-onset multiple system atrophy. J Neurol Sci 319: , 168–170. |
[321] | Meissner WG , Laurencin C , Tranchant C , Witjas T , Viallet F , Guehl D , Damier P , Houeto JL , Tison F , Eusebio A , Vital A , Streichenberger N , Lannes B , Maues de Paula A , Thobois S ((2016) ) Outcome of deep brain stimulation in slowly progressive multiple system atrophy: A clinico-pathological series and review of the literature. Parkinsonism Relat Disord 24: , 69–75. |
[322] | Masui K , Nakata Y , Fujii N , Iwaki T ((2012) ) Extensive distribution of glial cytoplasmic inclusions in an autopsied case of multiple system atrophy with a prolonged 18-year clinical course. Neuropathology 32: , 69–76. |
[323] | Gaig C , Iranzo A , Tolosa E , Vilaseca I , Rey MJ , Santamaria J ((2008) ) Pathological description of a non-motor variant of multiple system atrophy. J Neurol Neurosurg Psychiatry 79: , 1399–1400. |
[324] | Aoki N , Boyer PJ , Lund C , Lin WL , Koga S , Ross OA , Weiner M , Lipton A , Powers JM , White CL 3rd , Dickson DW ((2015) ) Atypical multiple system atrophy is a new subtype of frontotemporal lobar degeneration: Frontotemporal lobar degeneration associated with alpha-synuclein. Acta Neuropathol 130: , 93–105. |
[325] | Rohan Z , Rahimi J , Weis S , Kapas I , Auff E , Mitrovic N , Liberski PP , Sikorska B , Matej R , Kovacs GG ((2015) ) Screening for alpha-synuclein immunoreactive neuronal inclusions in the hippocampus allows identification of atypical MSA (FTLD-synuclein). Acta Neuropathol 130: , 299–301. |
[326] | Sousa AL , Taipa R , Quinn N , Revesz T , Melo Pires M , Magalhaes M ((2017) ) Frontotemporal lobar degeneration-TDP with ‘multiple system atrophy phenocopy syndrome’. Neuropathol Appl Neurobiol. doi 10.1111/nan.12391 |
[327] | Goldman JS , Quinzii C , Dunning-Broadbent J , Waters C , Mitsumoto H , Brannagan TH 3rd , Cosentino S , Huey ED , Nagy P , Kuo SH ((2014) ) Multiple system atrophy and amyotrophic lateral sclerosis in a family with hexanucleotide repeat expansions in C9orf72. JAMA Neurol 71: , 771–774. |
[328] | Ogaki K , Koga S , Aoki N , Lin W , Suzuki K , Ross OA , Dickson DW ((2016) ) Adult-onset cerebello-brainstem dominant form of X-linked adrenoleukodystrophy presenting as multiple system atrophy: Case report and literature review. Neuropathology 36: , 64–76. |
[329] | Jellinger KA ((2007) ) More frequent Lewy bodies but less frequent Alzheimer-type lesions in multiple system atrophy as compared to age-matched control brains. Acta Neuropathol 114: , 299–303. |
[330] | Mochizuki A , Komatsuzaki Y , Shoji S ((2002) ) Association of Lewy bodies and glial cytoplasmic inclusions in the brain of Parkinson’s disease. Acta Neuropathol 104: , 534–537. |
[331] | Sikorska B , Papierz W , Preusser M , Liberski PP , Budka H ((2007) ) Synucleinopathy with features of both multiple system atrophy and dementia with Lewy bodies. Neuropathol Appl Neurobiol 33: , 126–129. |
[332] | Lin CW , Tseng CY , Lo CP , Tu MC ((2016) ) A case of multiple system atrophy with preexisting Alzheimer’s disease and predating the hot cross bun sign. Acta Neurol Taiwan 25: , 152–159. |
{ label needed for ref[@id='ref332a'] } | Kovacs GG , Ferrer I , Grinberg LT , Alafuzoff I , Attems J , Budka H , Cairns NJ , Crary JF , Duyckaerts C , Ghetti B , Halliday GM , Ironside JW , Love S , Mackenzie IR , Munoz DG , Murray ME , Nelson PT , Takahashi H , Trojanowski JQ , Ansorge O , Arzberger T , Baborie A , Beach TG , Bieniek KF , Bigio EH , Bodi I , Dugger BN , Feany M , Gelpi E , Gentleman SM , Giaccone G , Hatanpaa KJ , Heale R , Hof PR , Hofer M , Hortobagyi T , Jellinger K , Jicha GA , Ince P , Kofler J , Kovari E , Kril JJ , Mann DM , Matej R , McKee AC , McLean C , Milenkovic I , Montine TJ , Murayama S , Lee EB , Rahimi J , Rodriguez RD , Rozemuller A , Schneider JA , Schultz C , Seeley W , Seilhean D , Smith C , Tagliavini F , Takao M , Thal DR , Toledo JB , Tolnay M , Troncoso JC , Vinters HV , Weis S , Wharton SB , White CL 3rd , Wisniewski T , Woulfe JM , Yamada M , Dickson DW ((2016) ) Aging-related tau astrogliopathy (ARTAG): Harmonized evaluation strategy. Acta Neuropathol 131: , 87–102. |
[333] | Koga S , Dickson DW , Bieniek KF ((2016) ) Chronic traumatic encephalopathy pathology in multiple system atrophy. J Neuropathol Exp Neurol 75: , 963–970. |
[334] | Geser F , Malunda JA , Hurtig HI , Duda JE , Wenning GK , Gilman S , Low PA , Lee VM-Y , Trojanowski JQ ((2011) ) TDP-43 pathology occurs infrequently in multiple system atrophy. Neuropathol Appl Neurobiol 37: , 358–365. |
[335] | Bergmann M , Kuchelmeister K , Kryne-Kubat B , Burwinkel F , Harms K , Gullotta F ((1994) ) Infantile multiple system atrophy with cytoplasmic and intranuclear glioneuronal inclusions. Acta Neuropathol 87: , 642–647. |
[336] | Silveira-Moriyama L , Gonzalez AM , O’Sullivan SS , Williams DR , Massey L , Parkkinen L , Ahmed Z , de Silva R , Chacon JR , Revesz T , Lees AJ , Holton JL ((2009) ) Concomitant progressive supranuclear palsy and multiple system atrophy: More than a simple twist of fate? Neurosci Lett 467: , 208–211. |
[337] | Wakabayashi K , Kawachi I , Toyoshima Y , Takahashi H ((1999) ) Occurrence of argyrophilic grains in multiple system atrophy: Histopathological examination of 26 autopsy cases. No To Shinkei 51: , 433–437. |
[338] | Togo T , Sahara N , Yen SH , Cookson N , Ishizawa T , Hutton M , de Silva R , Lees A , Dickson DW ((2002) ) Argyrophilic grain disease is a sporadic 4-repeat tauopathy. J Neuropathol Exp Neurol 61: , 547–556. |
[339] | Nagaishi M , Yokoo H , Nakazato Y ((2011) ) Tau-positive glial cytoplasmic granules in multiple system atrophy. Neuropathology 31: , 299–305. |
[340] | Jellinger K ((2012) ) Unusual tau in MSA. Neuropathology 32: , 110–111. |
[341] | Jellinger KA ((2011) ) Interaction between alpha-synuclein and other proteins in neurodegenerative disorders. ScientificWorldJournal 11: , 1893–1907. |
[342] | Jellinger KA ((2012) ) Interaction between pathogenic proteins in neurodegenerative disorders. J Cell Mol Med 16: , 1166–1183. |
[343] | Wenning GK , Jellinger KA ((2005) ) The role of alpha-synuclein and tau in neurodegenerative movement disorders. Curr Opin Neurol 18: , 357–362. |
[344] | Colom-Cadena M , Grau-Rivera O , Planellas L , Cerquera C , Morenas E , Helgueta S , Muñoz L , Kulisevsky J , Martí MJ , Tolosa E , Clarimon J , Lleó A , Gelpi E ((2017) ) Regional overlap of pathologies in Lewy body disorders. J Neuropathol Exp Neurol 76: , 216–224. |
[345] | Wong YC , Krainc D ((2017) ) alpha-synuclein toxicity in neurodegeneration: Mechanism and therapeutic strategies. Nat Med 23: , 1–13. |
[346] | Spires-Jones TL , Attems J , Thal DR ((2017) ) Interactions of pathological proteins in neurodegenerative diseases. Acta Neuropathol 134: , 187–205. |
[347] | Barun B , Brinar VV , Zadro I , Lusic I , Radovic D , Habek M ((2008) ) Parkinsonism and multiple sclerosis–is there association? Clin Neurol Neurosurg 110: , 958–961. |
[348] | Bougea A , Kapaki E , Paraskevas GP , Kilidireas K , Andreadou E ((2015) ) Multiple sclerosis and Parkinson’s disease: The two faces of neurodegeneration. Report of the first Greek case and review of the literature. Neurol Sci 36: , 2281–2285. |
[349] | Chuang C , Kocen RS , Quinn NP , Daniel SE ((2001) ) Case with both multiple system atrophy and primary progressive multiple sclerosis with discussion of the difficulty in their differential diagnosis. Mov Disord 16: , 355–358. |
[350] | Finke C , Siebert E , Plotkin M , Wenning GK , Reuter U ((2010) ) Multiple system atrophy masking multiple sclerosis. Clin Neurol Neurosurg 112: , 59–61. |
[351] | Batla A , Stamelou M , Mensikova K , Kaiserova M , Tuckova L , Kanovsky P , Quinn N , Bhatia KP ((2013) ) Markedly asymmetric presentation in multiple system atrophy. Parkinsonism Relat Disord 19: , 901–905. |
[352] | Kaindlstorfer C , Granata R , Wenning GK ((2013) ) Tremor in multiple system atrophy – a review. Tremor Other Hyperkinet Mov (N Y) 3: , pii: tre-03-165-4252-4251, doi: 4210.7916/D4258NV4259GZ4259. |
[353] | Köllensperger M , Geser F , Ndayisaba JP , Boesch S , Seppi K , Ostergaard K , Dupont E , Cardozo A , Tolosa E , Abele M , Klockgether T , Yekhlef F , Tison F , Daniels C , Deuschl G , Coelho M , Sampaio C , Bozi M , Quinn N , Schrag A , Mathias CJ , Fowler C , Nilsson CF , Widner H , Schimke N , Oertel W , Del Sorbo F , Albanese A , Pellecchia MT , Barone P , Djaldetti R , Colosimo C , Meco G , Gonzalez-Mandly A , Berciano J , Gurevich T , Giladi N , Galitzky M , Rascol O , Kamm C , Gasser T , Siebert U , Poewe W , Wenning GK ((2010) ) Presentation, diagnosis, and management of multiple system atrophy in Europe: Final analysis of the European multiple system atrophy registry. Mov Disord 25: , 2604–2612. |
[354] | Kollensperger M , Geser F , Seppi K , Stampfer-Kountchev M , Sawires M , Scherfler C , Boesch S , Mueller J , Koukouni V , Quinn N , Pellecchia MT , Barone P , Schimke N , Dodel R , Oertel W , Dupont E , Ostergaard K , Daniels C , Deuschl G , Gurevich T , Giladi N , Coelho M , Sampaio C , Nilsson C , Widner H , Sorbo FD , Albanese A , Cardozo A , Tolosa E , Abele M , Klockgether T , Kamm C , Gasser T , Djaldetti R , Colosimo C , Meco G , Schrag A , Poewe W , Wenning GK ((2008) ) Red flags for multiple system atrophy. Mov Disord 23: , 1093–1099. |
[355] | Wenning GK , Ben-Shlomo Y , Hughes A , Daniel SE , Lees A , Quinn NP ((2000) ) What clinical features are most useful to distinguish definite multiple system atrophy from Parkinson’s disease? J Neurol Neurosurg Psychiatry 68: , 434–440. |
[356] | Bank A , Oakley D , Frosch M , Schmahmann J ((2016) ) Spinal cord alpha synuclein deposition associated with spinal myoclonus in a patient with multiple system atrophy of the cerebellar type (MSA-C) (Poster). Neurology 86: (Suppl 16), P4.310. |
[357] | Eschlböck S , Benke T , Boesch S , Delazer M , Djamshidian-Tehrani A , Fanciulli A , Granata R , Högl B , Kaindlstorfer C , Kiss G , Krismer F , Mair K , Nocker M , Raccagni C , Scher?er C , Seppi K , Stefani A , Poewe W , Wenning GK ((2017) ) Non-motor symptoms and gender differences in multiple system atrophy (abstr.). NeuroLogisch Supp 1: , 8. |
[358] | Colosimo C ((2011) ) Nonmotor presentations of multiple system atrophy. Nat Rev Neurol 7: , 295–298. |
[359] | Papatsoris AG , Papapetropoulos S , Singer C , Deliveliotis C ((2008) ) Urinary and erectile dysfunction in multiple system atrophy (MSA). Neurourol Urodyn 27: , 22–27. |
[360] | Tandon R , Pradhan S ((2015) ) Autonomic predominant multiple system atrophy in the context of Parkinsonian and cerebellar variants. Clin Neurol Neurosurg 130: , 110–113. |
[361] | Pavy-Le Traon A , Piedvache A , Perez-Lloret S , Calandra-Buonaura G , Cochen-De Cock V , Colosimo C , Cortelli P , Debs R , Duerr S , Fanciulli A , Foubert-Samier A , Gerdelat A , Gurevich T , Krismer F , Poewe W , Tison F , Tranchant C , Wenning G , Rascol O , Meissner WG ((2016) ) New insights into orthostatic hypotension in multiple system atrophy: A European multicentre cohort study. J Neurol Neurosurg Psychiatry 87: , 554–561. |
[362] | Lipp A , Sandroni P , Ahlskog JE , Fealey RD , Kimpinski K , Iodice V , Gehrking TL , Weigand SD , Sletten DM , Gehrking JA , Nickander KK , Singer W , Maraganore DM , Gilman S , Wenning GK , Shults CW , Low PA ((2009) ) Prospective differentiation of multiple system atrophy from Parkinson disease, with and without autonomic failure. Arch Neurol 66: , 742–750. |
[363] | Shindo K , Tsuchiya M , Ichinose Y , Koh K , Hata T , Yamashiro N , Kobayashi F , Nagasaka T , Takiyama Y ((2017) ) Vasomotor regulation in patients with multiple system atrophy. J Neural Transm (Vienna) 124: , 477–481. |
[364] | Anderson T , Luxon L , Quinn N , Daniel S , Marsden CD , Bronstein A ((2008) ) Oculomotor function in multiple system atrophy: Clinical and laboratory features in 30 patients. Mov Disord 23: , 977–984. |
[365] | Videnovic A ((2017) ) Management of sleep disorders in Parkinson’s disease and multiple system atrophy. Mov Disord 32: , 659–668. |
[366] | Moreno-Lopez C , Santamaria J , Salamero M , Del Sorbo F , Albanese A , Pellecchia MT , Barone P , Overeem S , Bloem B , Aarden W , Canesi M , Antonini A , Duerr S , Wenning GK , Poewe W , Rubino A , Meco G , Schneider SA , Bhatia KP , Djaldetti R , Coelho M , Sampaio C , Cochen V , Hellriegel H , Deuschl G , Colosimo C , Marsili L , Gasser T , Tolosa E ((2011) ) Excessive daytime sleepiness in multiple system atrophy (SLEEMSA study). Arch Neurol 68: , 223–230. |
[367] | Shimohata T , Nakayama H , Tomita M , Ozawa T , Nishizawa M ((2012) ) Daytime sleepiness in Japanese patients with multiple system atrophy: Prevalence and determinants. BMC Neuro 12: , 130. |
[368] | Palma JA , Fernandez-Cordon C , Coon EA , Low PA , Miglis MG , Jaradeh S , Bhaumik AK , Dayalu P , Urrestarazu E , Iriarte J , Biaggioni I , Kaufmann H ((2015) ) Prevalence of REM sleep behavior disorder in multiple system atrophy: A multicenter study and meta-analysis. Clin Auton Res 25: , 69–75. |
[369] | Iranzo A , Santamaria J , Rye DB , Valldeoriola F , Marti MJ , Munoz E , Vilaseca I , Tolosa E ((2005) ) Characteristics of idiopathic REM sleep behavior disorder and that associated with MSA and PD. Neurology 65: , 247–252. |
[370] | Ghorayeb I , Dupouy S , Tison F , Meissner WG ((2014) ) Restless legs syndrome in multiple system atrophy. J Neural Transm (Vienna) 121: , 1523–1527. |
[371] | Ghorayeb I , Bioulac B , Tison F ((2004) ) Relationship between stridor and sleep apnoea syndrome: Is it as simple as that? J Neurol Neurosurg Psychiatry 75: , 512–513; author reply 513. |
[372] | Ohshima Y , Nakayama H , Matsuyama N , Hokari S , Sakagami T , Sato T , Koya T , Takahashi T , Kikuchi T , Nishizawa M , Shimohata T ((2017) ) Natural course and potential prognostic factors for sleep-disordered breathing in multiple system atrophy. Sleep Med 34: , 13–17. |
[373] | Wenning GK , Stefanova N ((2009) ) Recent developments in multiple system atrophy. J Neurol 256: , 1791–1808. |
[374] | Shimohata T , Aizawa N , Nakayama H , Taniguchi H , Ohshima Y , Okumura H , Takahashi T , Yokoseki A , Inoue M , Nishizawa M ((2016) ) Mechanisms and prevention of sudden death in multiple system atrophy. Parkinsonism Relat Disord 30: , 1–6. |
[375] | Mishima T , Kasanuki K , Koga S , Castanedes-Casey M , Wszolek ZK , Tsuboi Y , Dickson DW ((2017) ) Reduced orexin immunoreactivity in Perry syndrome and multiple system atrophy. Parkinsonism Relat Disord. doi: 10.1016/j.parkreldis.2017.06.003 |
[376] | Bak TH , Caine D , Hearn VC , Hodges JR ((2006) ) Visuospatial functions in atypical parkinsonian syndromes. J Neurol Neurosurg Psychiatry 77: , 454–456. |
[377] | Burk K , Daum I , Rub U ((2006) ) Cognitive function in multiple system atrophy of the cerebellar type. Mov Disord 21: , 772–776. |
[378] | Schrag A , Sheikh S , Quinn NP , Lees AJ , Selai C , Mathias C , Litvan I , Lang AE , Bower JH , Burn DJ , Low P , Jahanshahi M ((2010) ) A comparison of depression, anxiety, and health status in patients with progressive supranuclear palsy and multiple system atrophy. Mov Disord 25: , 1077–1081. |
[379] | Kao AW , Racine CA , Quitania LC , Kramer JH , Christine CW , Miller BL ((2009) ) Cognitive and neuropsychiatric profile of the synucleinopathies: Parkinson disease, dementia with Lewy bodies, and multiple system atrophy. Alzheimer Dis Assoc Disord 23: , 365–370. |
[380] | Auzou N , Dujardin K , Biundo R , Foubert-Samier A , Barth C , Duval F , Tison F , Defebvre L , Antonini A , Meissner WG ((2015) ) Diagnosing dementia in multiple system atrophy by applying Movement Disorder Society diagnostic criteria for Parkinson’s disease dementia. Parkinsonism Relat Disord 21: , 1273–1277. |
[381] | Koga S , Parks A , Uitti RJ , van Gerpen JA , Cheshire WP , Wszolek ZK , Dickson DW ((2017) ) Profile of cognitive impairment and underlying pathology in multiple system atrophy. Mov Disord 32: , 405–413. |
[382] | Chang CC , Chang YY , Chang WN , Lee YC , Wang YL , Lui CC , Huang CW , Liu WL ((2009) ) Cognitive deficits in multiple system atrophy correlate with frontal atrophy and disease duration. Eur J Neurol 16: , 1144–1150. |
[383] | Gilman S , Little R , Johanns J , Heumann M , Kluin KJ , Junck L , Koeppe RA , An H ((2000) ) Evolution of sporadic olivopontocerebellar atrophy into multiple system atrophy. Neurology 55: , 527–532. |
[384] | Abele M , Burk K , Schols L , Schwartz S , Besenthal I , Dichgans J , Zuhlke C , Riess O , Klockgether T ((2002) ) The aetiology of sporadic adult-onset ataxia. Brain 125: , 961–968. |
[385] | Futamura N , Matsumura R , Fujimoto Y , Horikawa H , Suzumura A , Takayanagi T ((1998) ) CAG repeat expansions in patients with sporadic cerebellar ataxia. Acta Neurol Scand 98: , 55–59. |
[386] | Schols L , Szymanski S , Peters S , Przuntek H , Epplen JT , Hardt C , Riess O ((2000) ) Genetic background of apparently idiopathic sporadic cerebellar ataxia. Hum Genet 107: , 132–137. |
[387] | Kim JM , Hong S , Kim GP , Choi YJ , Kim YK , Park SS , Kim SE , Jeon BS ((2007) ) Importance of low-range CAG expansion and CAA interruption in SCA2 Parkinsonism. Arch Neurol 64: , 1510–1518. |
[388] | Kim JY , Kim SY , Kim JM , Kim YK , Yoon KY , Lee BC , Kim JS , Paek SH , Park SS , Kim SE , Jeon BS ((2009) ) Spinocerebellar ataxia type 17 mutation as a causative and susceptibility gene in parkinsonism. Neurology 72: , 1385–1389. |
[389] | Doherty KM , De Pablo-Fernandez E , Houlden H , Polke JM , Lees AJ , Warner TT , Holton JL ((2016) ) MSA-C or SCA 17? A clinicopathological case update. Mov Disord 31: , 1582–1584. |
[390] | Nirenberg MJ , Libien J , Vonsattel JP , Fahn S ((2007) ) Multiple system atrophy in a patient with the spinocerebellar ataxia 3 gene mutation. Mov Disord 22: , 251–254. |
[391] | Hadjivassiliou M , Martindale J , Shanmugarajah P , Grunewald RA , Sarrigiannis PG , Beauchamp N , Garrard K , Warburton R , Sanders DS , Friend D , Duty S , Taylor J , Hoggard N ((2016) ) Causes of progressive cerebellar ataxia: Prospective evaluation of 1500 patients. J Neurol Neurosurg Psychiatry 88: , 301–309. |
[392] | Kim HJ , Jeon BS , Shin J , Lee WW , Park H , Jung YJ , Ehm G ((2014) ) Should genetic testing for SCAs be included in the diagnostic workup for MSA? Neurology 83: , 1733–1738. |
[393] | Mehta AR , Fox SH , Tarnopolsky M , Yoon G ((2011) ) Mitochondrial mimicry of multiple system atrophy of the cerebellar subtype. Mov Disord 26: , 753–755. |
[394] | Stamelou M , Quinn NP , Bhatia KP ((2013) ) “Atypical” atypical parkinsonism: New genetic conditions presenting with features of progressive supranuclear palsy, corticobasal degeneration, or multiple system atrophy-a diagnostic guide. Mov Disord 28: , 1184–1199. |
[395] | Biancalana V , Toft M , Le Ber I , Tison F , Scherrer E , Thibodeau S , Mandel JL , Brice A , Farrer MJ , Durr A ((2005) ) FMR1 premutations associated with fragile X-associated tremor/ataxia syndrome in multiple system atrophy. Arch Neurol 62: , 962–966. |
[396] | Joutsa J , Gardberg M , Roytta M , Kaasinen V ((2014) ) Diagnostic accuracy of parkinsonism syndromes by general neurologists. Parkinsonism Relat Disord 20: , 840–844. |
[397] | Osaki Y , Ben-Shlomo Y , Lees AJ , Wenning GK , Quinn NP ((2009) ) A validation exercise on the new consensus criteria for multiple system atrophy. Mov Disord 24: , 2272–2276. |
[398] | Matsushima M , Yabe I , Takahashi I , Hirotani M , Kano T , Horiuchi K , Houzen H , Sasaki H ((2017) ) Validity and reliability of a pilot scale for assessment of multiple system atrophy symptoms. Cerebellum Ataxias 4: , 11. |
[399] | Kawai Y , Suenaga M , Takeda A , Ito M , Watanabe H , Tanaka F , Kato K , Fukatsu H , Naganawa S , Kato T , Ito K , Sobue G ((2008) ) Cognitive impairments in multiple system atrophy: MSA-C vs MSA-P. Neurology 70: , 1390–1396. |
[400] | Stankovic I , Krismer F , Jesic A , Antonini A , Benke T , Brown RG , Burn DJ , Holton JL , Kaufmann H , Kostic VS , Ling H , Meissner WG , Poewe W , Semnic M , Seppi K , Takeda A , Weintraub D , Wenning GK ((2014) ) Cognitive impairment in multiple system atrophy: A position statement by the neuropsychology task force of the MDS multiple system atrophy (MODIMSA) study group. Mov Disord 29: , 857–867. |
{ label needed for ref[@id='ref400a'] } | Magdalinou NK , Noyce AJ , Pinto R , Lindstrom E , Holmen-Larsson J , Holtta M , Blennow K , Morris HR , Skillback T , Warner TT , Lees AJ , Pike I , Ward M , Zetterberg H , Gobom J ((2017) ) Identification of candidate cerebrospinal fluid biomarkers in parkinsonism using quantitative proteomics. Parkinsonism Relat Disord 37: , 65–71. |
[401] | Kitayama M , Wada-Isoe K , Irizawa Y , Nakashima K ((2009) ) Assessment of dementia in patients with multiple system atrophy. Eur J Neurol 16: , 589–594. |
[402] | Laurens B , Constantinescu R , Freeman R , Gerhard A , Jellinger K , Jeromin A , Krismer F , Mollenhauer B , Schlossmacher MG , Shaw LM , Verbeek MM , Wenning GK , Winge K , Zhang J , Meissner WG ((2015) ) Fluid biomarkers in multiple system atrophy: A review of the MSA Biomarker Initiative. Neurobiol Dis 80: , 29–41. |
[403] | Yamagishi Y , Saigoh K , Saito Y , Ogawa I , Mitsui Y , Hamada Y , Samukawa M , Suzuki H , Kuwahara M , Hirano M , Noguchi N , Kusunoki S ((2017) ) Diagnosis of Parkinson’s disease and the level of oxidized DJ-1 protein. Neurosci Res. doi: 10.1016/j.neures.2017.06.008 |
[404] | Treglia G , Stefanelli A , Cason E , Cocciolillo F , Di Giuda D , Giordano A ((2011) ) Diagnostic performance of iodine-123-metaiodobenzylguanidine scintigraphy in differential diagnosis between Parkinson’s disease and multiple-system atrophy: A systematic review and a meta-analysis. Clin Neurol Neurosurg 113: , 823–829. |
[405] | Kaindlstorfer C , Krismer F , Fanciulli A , Eschlböck S , Nocker M , Bösch S , Mair K , Scherfler C , Djamshidian-Tehrani A , Uprimny C , Donnemiller E , Virgolini I , Seppi K , Poewe W , Wenning G ((2016) ) Diagnostic value of cardiac 123I-MIBG SPECT and CT co-registration in PD and MSA-P (Poster). Neurologisch Supp 1: , 38. |
[406] | Nagayama H , Ueda M , Yamazaki M , Nishiyama Y , Hamamoto M , Katayama Y ((2010) ) Abnormal cardiac [(123)I]-meta-iodobenzylguanidine uptake in multiple system atrophy. Mov Disord 25: , 1744–1747. |
[407] | Baschieri F , Calandra-Buonaura G , Cecere A , Barletta G , Contin M , Parchi P , Cortelli P ((2017) ) Iodine-123-meta-iodobenzylguanidine myocardial scintigraphy in isolated autonomic failure: Potential red flag for future multiple system atrophy. Front Neurol 8: , 225. |
[408] | Rascol O , Schelosky L ((2009) ) 123I-metaiodobenzylguanidine scintigraphy in Parkinson’s disease and related disorders. Mov Disord 24: (Suppl 2), S732–S741. |
[409] | Krismer F , Pinter B , Mueller C , Mahlknecht P , Nocker M , Reiter E , Djamshidian-Tehrani A , Boesch SM , Wenning GK , Scherfler C , Poewe W , Seppi K ((2017) ) Sniffing the diagnosis: Olfactory testing in neurodegenerative parkinsonism. Parkinsonism Relat Disord 35: , 36–41. |
[410] | Abele M , Riet A , Hummel T , Klockgether T , Wullner U ((2003) ) Olfactory dysfunction in cerebellar ataxia and multiple system atrophy. J Neurol 250: , 1453–1455. |
[411] | Schrag A , Good CD , Miszkiel K , Morris HR , Mathias CJ , Lees AJ , Quinn NP ((2000) ) Differentiation of atypical parkinsonian syndromes with routine MRI. Neurology 54: , 697–702. |
[412] | Matsusue E , Fujii S , Kanasaki Y , Sugihara S , Miyata H , Ohama E , Ogawa T ((2008) ) Putaminal lesion in multiple system atrophy: Postmortem MR-pathological correlations. Neuroradiology 50: , 559–567. |
[413] | Lee JH , Kim TH , Mun CW , Han YH ((2015) ) Progression of subcortical atrophy and iron deposition in multiple system atrophy: A comparison between clinical subtypes. J Neurol 262: , 1876–1882. |
[414] | Hwang I , Sohn CH , Kang KM , Jeon BS , Kim HJ , Choi SH , Yun TJ , Kim JH ((2015) ) Differentiation of parkinsonism-predominant multiple system atrophy from idiopathic Parkinson disease using 3T susceptibility-weighted MR imaging, focusing on putaminal change and lesion asymmetry. AJNR Am J Neuroradiol 36: , 2227–2234. |
[415] | Ramli N , Nair SR , Ramli NM , Lim SY ((2015) ) Differentiating multiple-system atrophy from Parkinson’s disease. Clin Radiol 70: , 555–564. |
[416] | Sugiyama A , Ito S , Suichi T , Sakurai T , Mukai H , Yokota H , Yonezu T , Kuwabara S ((2015) ) Putaminal hypointensity on T2*-weighted MR imaging is the most practically useful sign in diagnosing multiple system atrophy: A preliminary study. J Neurol Sci 349: , 174–178. |
[417] | Deguchi K , Ikeda K , Kume K , Takata T , Kokudo Y , Kamada M , Touge T , Honjo N , Masaki T ((2015) ) Significance of the hot-cross bun sign on T2*-weighted MRI for the diagnosis of multiple system atrophy. J Neurol 262: , 1433–1439. |
[418] | Feng JY , Huang B , Yang WQ , Zhang YH , Wang LM , Wang LJ , Zhong XL ((2015) ) The putaminal abnormalities on 3.0T magnetic resonance imaging: Can they separate parkinsonism-predominant multiple system atrophy from Parkinson’s disease? Acta Radiol 56: , 322–328. |
[419] | Wang N , Yang H , Li C , Fan G , Luo X ((2017) ) Using ‘swallow-tail’ sign and putaminal hypointensity as biomarkers to distinguish multiple system atrophy from idiopathic Parkinson’s disease: A susceptibility-weighted imaging study. Eur Radiol 27: , 3174–3180. |
[420] | De Marzi R , Bajaj S , Krismer F , Larcher B , Ringler S , Wenning GK , Schocke M , Poewe W , Seppi K ((2017) ) Putaminal Diffusion imaging for the differential diagnosis of the parkinsoniao variant of multiple system atrophy from Parkinson’s disease: Impact of segmentation accuracy. NeuroLogisch Suppl 1: , 8–9. |
[421] | Planetta PJ , Kurani AS , Shukla P , Prodoehl J , Corcos DM , Comella CL , McFarland NR , Okun MS , Vaillancourt DE ((2015) ) Distinct functional and macrostructural brain changes in Parkinson’s disease and multiple system atrophy. Hum Brain Mapp 36: , 1165–1179. |
[422] | Focke NK , Helms G , Pantel PM , Scheewe S , Knauth M , Bachmann CG , Ebentheuer J , Dechent P , Paulus W , Trenkwalder C ((2011) ) Differentiation of typical and atypical Parkinson syndromes by quantitative MR imaging. AJNR Am J Neuroradiol 32: , 2087–2092. |
[423] | Wang Y , Butros SR , Shuai X , Dai Y , Chen C , Liu M , Haacke EM , Hu J , Xu H ((2012) ) Different iron-deposition patterns of multiple system atrophy with predominant parkinsonism and idiopathetic Parkinson diseases demonstrated by phase-corrected susceptibility-weighted imaging. AJNR Am J Neuroradiol 33: , 266–273. |
[424] | Cnyrim CD , Kupsch A , Ebersbach G , Hoffmann KT ((2014) ) Diffusion tensor imaging in idiopathic Parkinson’s disease and multisystem atrophy (Parkinsonian type). Neurodegener Dis 13: , 1–8. |
[425] | Chen B , Fan G , Sun W , Shang X , Shi S , Wang S , Lv G , Wu C ((2017) ) Usefulness of diffusion-tensor MRI in the diagnosis of Parkinson variant of multiple system atrophy and Parkinson’s disease: A valuable tool to differentiate between them?610 e619-610 e. Clin Radiol 72: , 615. |
{ label needed for ref[@id='ref425a'] } | Ito K , Ohtsuka C , Yoshioka K , Kameda H , Yokosawa S , Sato R , Terayama Y , Sasaki M ((2017) ) Differential diagnosis of parkinsonism by a combined use of diffusion kurtosis imaging and quantitative susceptibility mapping. Neuroradiol 59: , 759–769. |
[426] | Pradhan S , Tandon R ((2017) ) Relevance of non-specific MRI features in multiple system atrophy. Clin Neurol Neurosurg 159: , 29–33. |
[427] | Ji L , Wang Y , Zhu D , Liu W , Shi J ((2015) ) White matter differences between multiple system atrophy (parkinsonian type) and Parkinson’s disease: A diffusion tensor image study. Neuroscience 305: , 109–116. |
[428] | Umemura A , Oeda T , Hayashi R , Tomita S , Kohsaka M , Yamamoto K , Sawada H ((2013) ) Diagnostic accuracy of apparent diffusion coefficient and 123I-metaiodobenzylguanidine for differentiation of multiple system atrophy and Parkinson’s disease. PLoS One 8: , e61066. |
[429] | Tang CC , Eidelberg D ((2010) ) Abnormal metabolic brain networks in Parkinson’s disease from blackboard to bedside. Prog Brain Res 184: , 161–176. |
[430] | Oh M , Kim JS , Kim JY , Shin KH , Park SH , Kim HO , Moon DH , Oh SJ , Chung SJ , Lee CS ((2012) ) Subregional patterns of preferential striatal dopamine transporter loss differ in Parkinson disease, progressive supranuclear palsy, and multiple-system atrophy. J Nucl Med 53: , 399–406. |
[431] | Nocker M , Seppi K , Donnemiller E , Virgolini I , Wenning GK , Poewe W , Scherfler C ((2012) ) Progression of dopamine transporter decline in patients with the Parkinson variant of multiple system atrophy: A voxel-based analysis of [123I]beta-CIT SPECT. Eur J Nucl Med Mol Imaging 39: , 1012–1020. |
[432] | McKinley J , O’Connell M , Farrell M , Lynch T ((2014) ) Normal dopamine transporter imaging does not exclude multiple system atrophy. Parkinsonism Relat Disord 20: , 933–934. |
[433] | Perju-Dumbrava LD , Kovacs GG , Pirker S , Jellinger K , Hoffmann M , Asenbaum S , Pirker W ((2012) ) Dopamine transporter imaging in autopsy-confirmed Parkinson’s disease and multiple system atrophy. Mov Disord 27: , 65–71. |
[434] | Kraemmer J , Kovacs GG , Perju-Dumbrava L , Pirker S , Traub-Weidinger T , Pirker W ((2014) ) Correlation of striatal dopamine transporter imaging with post mortem substantia nigra cell counts. Mov Disord 29: , 1767–1773. |
[435] | Lewis SJ , Pavese N , Rivero-Bosch M , Eggert K , Oertel W , Mathias CJ , Brooks DJ , Gerhard A ((2012) ) Brain monoamine systems in multiple system atrophy: A positron emission tomography study. Neurobiol Dis 46: , 130–136. |
[436] | Levin J , Maass S , Schuberth M , Höglinger G ((2017) ) Multiple system atrophy. In Movement Disorders Curricula, Falup-Pecurariu C, Ferreira J, Martinez-Martin P, Chaudhuri KR, eds. Springer Wien, pp. 183–192. |
[437] | Saeed U , Compagnone J , Aviv RI , Strafella AP , Black SE , Lang AE , Masellis M ((2017) ) Imaging biomarkers in Parkinson’s disease and Parkinsonian syndromes: Current and emerging concepts. Transl Neurodegener 6: , 8. |
[438] | Boesch SM , Wenning GK , Ransmayr G , Poewe W ((2002) ) Dystonia in multiple system atrophy. J Neurol Neurosurg Psychiatry 72: , 300–303. |
[439] | Mandler M , Valera E , Rockenstein E , Mante M , Weninger H , Patrick C , Adame A , Schmidhuber S , Santic R , Schneeberger A , Schmidt W , Mattner F , Masliah E ((2015) ) Active immunization against alpha-synuclein ameliorates the degenerative pathology and prevents demyelination in a model of multiple system atrophy. Mol Neurodegener 10: , 10. |
[440] | Valera E , Spencer B , Fields JA , Trinh I , Adame A , Mante M , Rockenstein E , Desplats P , Masliah E ((2017) ) Combination of alpha-synuclein immunotherapy with anti-inflammatory treatment in a transgenic mouse model of multiple system atrophy. Acta Neuropathol Commun 5: , 2. |
[441] | Vieira BD , Radford RA , Chung RS , Guillemin GJ , Pountney DL ((2015) ) Neuroinflammation in multiple system atrophy: Response to and cause of alpha-synuclein aggregation. Front Cell Neurosci 9: , 437. |
[442] | Tweedie D , Sambamurti K , Greig NH ((2007) ) TNF-alpha inhibition as a treatment strategy for neurodegenerative disorders: New drug candidates and targets. Curr Alzheimer Res 4: , 378–385. |
[443] | Schenk DB , Koller M , Ness DK , Griffith SG , Grundman M , Zago W , Soto J , Atiee G , Ostrowitzki S , Kinney GG ((2017) ) First-in-human assessment of PRX002, an anti-alpha-synuclein monoclonal antibody, in healthy volunteers. Mov Disord 32: , 211–218. |
[444] | Jellinger KA ((2015) ) Multiple system atrophy – a synucleinopathy with specific glioneuronal degeneration. Austin J Clin Neuro 2: , 1071. |
[445] | Jellinger KA ((2015) ) What’s new in multiple system atrophy. Curr Neurobio 6: , 11–14. |
[446] | Ettle B , Kerman BE , Valera E , Gillmann C , Schlachetzki JC , Reiprich S , Buttner C , Ekici AB , Reis A , Wegner M , Bauerle T , Riemenschneider MJ , Masliah E , Gage FH , Winkler J ((2016) ) alpha-Synuclein-induced myelination deficit defines a novel interventional target for multiple system atrophy. Acta Neuropathol 132: , 59–75. |
[447] | Kahle PJ , Neumann M , Ozmen L , Muller V , Jacobsen H , Spooren W , Fuss B , Mallon B , Macklin WB , Fujiwara H , Hasegawa M , Iwatsubo T , Kretzschmar HA , Haass C ((2002) ) Hyperphosphorylation and insolubility of alpha-synuclein in transgenic mouse oligodendrocytes. EMBO Rep 3: , 583–588. |
[448] | Stefanova N , Reindl M , Neumann M , Haass C , Poewe W , Kahle PJ , Wenning GK ((2005) ) Oxidative stress in transgenic mice with oligodendroglial alpha-synuclein overexpression replicates the characteristic neuropathology of multiple system atrophy. Am J Pathol 166: , 869–876. |
[449] | Stemberger S , Poewe W , Wenning GK , Stefanova N ((2010) ) Targeted overexpression of human alpha-synuclein in oligodendroglia induces lesions linked to MSA-like progressive autonomic failure. Exp Neurol 224: , 459–464. |
[450] | Bleasel JM , Wong JH , Halliday GM , Kim WS ((2014) ) Lipid dysfunction and pathogenesis of multiple system atrophy. Acta Neuropathol Commun 2: , 15. |
[451] | Halliday GM ((2015) ) Re-evaluating the glio-centric view of multiple system atrophy by highlighting the neuronal involvement. Brain 138: , 2116–2119. |
[452] | Jellinger KA , Krismer F ((2014) ) Aetiopathogenesis. In Multiple System Atrophy, Wenning GK, Fanciulli A, eds. Springer-Verlag, Vienna, pp. 57–81. |
[453] | Ubhi K , Lee PH , Adame A , Inglis C , Mante M , Rockenstein E , Stefanova N , Wenning GK , Masliah E ((2009) ) Mitochondrial inhibitor 3-nitroproprionic acid enhances oxidative modification of alpha-synuclein in a transgenic mouse model of multiple system atrophy. J Neurosci Res 87: , 2728–2739. |
[454] | Federoff M ((2016) ) Multiple system atrophy: Moving towards a multi-mechanistic hypothesis. Int J Neurol Neurothe 3: , 046. |
[455] | Beyer K , Ariza A ((2007) ) Protein aggregation mechanisms in synucleinopathies: Commonalities and differences. J Neuropathol Exp Neurol 66: , 965–974. |
[456] | Chavarria C , Souza JM ((2013) ) Oxidation and nitration of alpha-synuclein and their implications in neurodegenerative diseases. Arch Biochem Biophys 533: , 25–32. |
[457] | Fujiwara H , Hasegawa M , Dohmae N , Kawashima A , Masliah E , Goldberg MS , Shen J , Takio K , Iwatsubo T ((2002) ) alpha-Synuclein is phosphorylated in synucleinopathy lesions. Nat Cell Biol 4: , 160–164. |
[458] | Hasegawa M , Fujiwara H , Nonaka T , Wakabayashi K , Takahashi H , Lee VM , Trojanowski JQ , Mann D , Iwatsubo T ((2002) ) Phosphorylated alpha-synuclein is ubiquitinated in alpha-synucleinopathy lesions. J Biol Chem 277: , 49071–49076. |
[459] | Rey NL , George S , Brundin P ((2016) ) Review: Spreading the word: Precise animal models and validated methods are vital when evaluating prion-like behaviour of alpha-synuclein. Neuropathol Appl Neurobiol 42: , 51–76. |
[460] | Brudek T , Winge K , Rasmussen NB , Bahl JM , Tanassi J , Agander TK , Hyde TM , Pakkenberg B ((2016) ) Altered alpha-synuclein, parkin, and synphilin isoform levels in multiple system atrophy brains. J Neurochem 136: , 172–185. |
[461] | Tanaka M , Kim YM , Lee G , Junn E , Iwatsubo T , Mouradian MM ((2004) ) Aggresomes formed by alpha-synuclein and synphilin-1 are cytoprotective. J Biol Chem 279: , 4625–4631. |
[462] | Jellinger KA ((2013) ) Synuclein and Parkinson’s disease: An update. In Emerging Drugs and Targets for Parkinson’s disease, Martinez A, Gil C, eds. The Royal Society of Chemistry London, UK, pp. 175–214. |
[463] | Andreasen M , Lorenzen N , Otzen D ((2015) ) Interactions between misfolded protein oligomers and membranes: A central topic in neurodegenerative diseases? Biochim Biophys Acta 1848: , 1897–1907. |
[464] | Lindstrom V , Fagerqvist T , Nordstrom E , Eriksson F , Lord A , Tucker S , Andersson J , Johannesson M , Schell H , Kahle PJ , Moller C , Gellerfors P , Bergstrom J , Lannfelt L , Ingelsson M ((2014) ) Immunotherapy targeting alpha-synuclein protofibrils reduced pathology in (Thy-1)-h[A30P] alpha-synuclein mice. Neurobiol Dis 69: , 134–143. |
[465] | Roberts HL , Brown DR ((2015) ) Seeking a mechanism for the toxicity of oligomeric alpha-synuclein. Biomolecules 5: , 282–305. |
[466] | Asi YT , Simpson JE , Heath PR , Wharton SB , Lees AJ , Revesz T , Houlden H , Holton JL ((2014) ) Alpha-synuclein mRNA expression in oligodendrocytes in MSA. Glia 62: , 964–970. |
[467] | Reyes JF , Rey NL , Bousset L , Melki R , Brundin P , Angot E ((2014) ) Alpha-synuclein transfers from neurons to oligodendrocytes. Glia 62: , 387–398. |
[468] | Shults CW , Rockenstein E , Crews L , Adame A , Mante M , Larrea G , Hashimoto M , Song D , Iwatsubo T , Tsuboi K , Masliah E ((2005) ) Neurological and neurodegenerative alterations in a transgenic mouse model expressing human alpha-synuclein under oligodendrocyte promoter: Implications for multiple system atrophy. J Neurosci 25: , 10689–10699. |
[469] | Ubhi K , Rockenstein E , Mante M , Inglis C , Adame A , Patrick C , Whitney K , Masliah E ((2010) ) Neurodegeneration in a transgenic mouse model of multiple system atrophy is associated with altered expression of oligodendroglial-derived neurotrophic factors. J Neurosci 30: , 6236–6246. |
[470] | Grigoletto J , Pukass K , Gamliel A , Davidi D , Katz-Brull R , Richter-Landsberg C , Sharon R ((2017) ) Higher levels of myelin phospholipids in brains of neuronal alpha-Synuclein transgenic mice precede myelin loss. Acta Neuropathol Commun 5: , 37. |
[471] | Wong JH , Halliday GM , Kim WS ((2014) ) Exploring myelin dysfunction in multiple system atrophy. Exp Neurobiol 23: , 337–344. |
[472] | Bassil F , Monvoisin A , Canron MH , Vital A , Meissner WG , Tison F , Fernagut PO ((2015) ) Region-specific alterations of matrix metalloproteinase activity in multiple system atrophy. Mov Disord 30: , 1802–1812. |
[473] | Braak H , Del Tredici K , Rub U , de Vos RA , Jansen Steur EN , Braak E ((2003) ) Staging of brain pathology related to sporadic Parkinson’s disease. Neurobiol Aging 24: , 197–211. |
[474] | Kordower JH , Chu Y , Hauser RA , Olanow CW , Freeman TB ((2008) ) Transplanted dopaminergic neurons develop PD pathologic changes: A second case report. Mov Disord 23: , 2303–2306. |
[475] | Kordower JH , Dodiya HB , Kordower AM , Terpstra B , Paumier K , Madhavan L , Sortwell C , Steece-Collier K , Collier TJ ((2011) ) Transfer of host-derived alpha synuclein to grafted dopaminergic neurons in rat. Neurobiol Dis 43: , 552–557. |
[476] | Li W , Englund E , Widner H , Mattsson B , van Westen D , Latt J , Rehncrona S , Brundin P , Bjorklund A , Lindvall O , Li JY ((2016) ) Extensive graft-derived dopaminergic innervation is maintained 24 years after transplantation in the degenerating parkinsonian brain. Proc Natl Acad Sci U S A 113: , 6544–6549. |
[477] | Masuda-Suzukake M , Nonaka T , Hosokawa M , Oikawa T , Arai T , Akiyama H , Mann DM , Hasegawa M ((2013) ) Prion-like spreading of pathological alpha-synuclein in brain. Brain 136: , 1128–1138. |
[478] | Goedert M ((2015) ) Alzheimer’s and Parkinson’s diseases: The prion concept in relation to assembled Abeta, tau, and alpha-synuclein. Science 349: , 1255555. |
[479] | Brettschneider J , Del Tredici K , Lee VM , Trojanowski JQ ((2015) ) Spreading of pathology in neurodegenerative diseases: A focus on human studies. Nat Rev Neurosci 16: , 109–120. |
[480] | Walsh DM , Selkoe DJ ((2016) ) A critical appraisal of the pathogenic protein spread hypothesis of neurodegeneration. Nat Rev Neurosci 17: , 251–260. |
[481] | Bendor JT , Logan TP , Edwards RH ((2013) ) The function of alpha-synuclein. Neuron 79: , 1044–1066. |
[482] | Guo JL , Lee VM ((2014) ) Cell-to-cell transmission of pathogenic proteins in neurodegenerative diseases. Nat Med 20: , 130–138. |
[483] | Desplats P , Lee HJ , Bae EJ , Patrick C , Rockenstein E , Crews L , Spencer B , Masliah E , Lee SJ ((2009) ) Inclusion formation and neuronal cell death through neuron-to-neuron transmission of alpha-synuclein. Proc Natl Acad Sci U S A 106: , 13010–13015. |
[484] | Luk KC , Kehm V , Carroll J , Zhang B , O’Brien P , Trojanowski JQ , Lee VM ((2012) ) Pathological alpha-synuclein transmission initiates Parkinson-like neurodegeneration in nontransgenic mice. Science 338: , 949–953. |
[485] | Luk KC , Kehm VM , Zhang B , O’Brien P , Trojanowski JQ , Lee VM ((2012) ) Intracerebral inoculation of pathological alpha-synuclein initiates a rapidly progressive neurodegenerative alpha-synucleinopathy in mice. J Exp Med 209: , 975–986. |
[486] | Valdinocci D , Radford RA , Siow SM , Chung RS , Pountney DL ((2017) ) Potential modes of intercellular alpha-synuclein transmission. Int J Mol Sci 18: , 469. |
[487] | Lee EJ , Woo MS , Moon PG , Baek MC , Choi IY , Kim WK , Junn E , Kim HS ((2010) ) Alpha-synuclein activates microglia by inducing the expressions of matrix metalloproteinases and the subsequent activation of protease-activated receptor-1. J Immunol 185: , 615–623. |
[488] | Lee HJ , Suk JE , Patrick C , Bae EJ , Cho JH , Rho S , Hwang D , Masliah E , Lee SJ ((2010) ) Direct transfer of alpha-synuclein from neuron to astroglia causes inflammatory responses in synucleinopathies. J Biol Chem 285: , 9262–9272. |
[489] | Kisos H , Pukass K , Ben-Hur T , Richter-Landsberg C , Sharon R ((2012) ) Increased neuronal alpha-synuclein pathology associates with its accumulation in oligodendrocytes in mice modeling alpha-synucleinopathies. PLoS One 7: , e46817. |
[490] | Bernis ME , Babila JT , Breid S , Wusten KA , Wullner U , Tamguney G ((2015) ) Prion-like propagation of human brain-derived alpha-synuclein in transgenic mice expressing human wild-type alpha-synuclein. Acta Neuropathol Commun 3: , 75. |
[491] | Woerman AL , Kazmi SA , Patel S , Freyman Y , Oehler A , Aoyagi A , Mordes DA , Halliday GM , Middleton LT , Gentleman SM , Olson SH , Prusiner SB ((2018) ) MSA prions exhibit remarkable stability and resistance to inactivation. Acta Neuropathol 135: , 49–63. |
[492] | Shimozawa A , Ono M , Takahara D , Tarutani A , Imura S , Masuda-Suzukake M , Higuchi M , Yanai K , Hisanaga SI , Hasegawa M ((2017) ) Propagation of pathological alpha-synuclein in marmoset brain. Acta Neuropathol Commun 5: , 12. |
[493] | Danzer KM , Krebs SK , Wolff M , Birk G , Hengerer B ((2009) ) Seeding induced by alpha-synuclein oligomers provides evidence for spreading of alpha-synuclein pathology. J Neurochem 111: , 192–203. |
[494] | Luk KC , Song C , O’Brien P , Stieber A , Branch JR , Brunden KR , Trojanowski JQ , Lee VM ((2009) ) Exogenous alpha-synuclein fibrils seed the formation of Lewy body-like intracellular inclusions in cultured cells. Proc Natl Acad Sci U S A 106: , 20051–20056. |
[495] | Peelaerts W , Bousset L , Van der Perren A , Moskalyuk A , Pulizzi R , Giugliano M , Van den Haute C , Melki R , Baekelandt V ((2015) ) alpha-Synuclein strains cause distinct synucleinopathies after local and systemic administration. Nature 522: , 340–344. |
[496] | Melki R ((2015) ) Role of different alpha-synuclein strains in synucleinopathies, similarities with other neurodegenerative diseases. J Parkinsons Dis 5: , 217–227. |
[497] | Peelaerts W , Baekelandt V ((2016) ) a-Synuclein strains and the variable pathologies of synucleinopathies. J Neurochem Suppl 1: , 256–274. |
[498] | Tofaris GK , Goedert M , Spillantini MG ((2016) ) The transcellular propagation and intracellular trafficking of alpha-synuclein. Cold Spring Harb Perspect Med 7: , a024380. |
[499] | Hasegawa M , Nonaka T , Masuda-Suzukake M ((2016) ) alpha-Synuclein: Exerimental pathology, Cold Spring Harb Perspect Med 6: , pii: a024273. |
[500] | Bassil F , Guerin PA , Dutheil N , Li Q , Klugmann M , Meissner WG , Bezard E , Fernagut PO ((2017) ) Viral-mediated oligodendroglial alpha-synuclein expression models multiple system atrophy. Mov Disord 32: , 1230–1239. |
[501] | Mandel RJ , Marmion DJ , Kirik D , Chu Y , Heindel C , McCown T , Gray SJ , Kordower JH ((2017) ) Novel oligodendroglial alpha synuclein viral vector models of multiple system atrophy: Studies in rodents and nonhuman primates. Acta Neuropathol Commun 5: , 47. |
[502] | Jellinger KA ((2016) ) Recent advances in multiple system atrophy. J Neurol Neuromed 1: , 6–17. |
[503] | Binolfi A , Limatola A , Verzini S , Kosten J , Theillet FX , Rose HM , Bekei B , Stuiver M , van Rossum M , Selenko P ((2016) ) Intracellular repair of oxidation-damaged alpha-synuclein fails to target C-terminal modification sites. Nat Commun 7: , 10251. |
[504] | Theillet FX , Binolfi A , Bekei B , Martorana A , Rose HM , Stuiver M , Verzini S , Lorenz D , van Rossum M , Goldfarb D , Selenko P ((2016) ) Structural disorder of monomeric alpha-synuclein persists in mammalian cells. Nature 530: , 45–50. |
[505] | Song YJ , Lundvig DM , Huang Y , Gai WP , Blumbergs PC , Hojrup P , Otzen D , Halliday GM , Jensen PH ((2007) ) p25alpha relocalizes in oligodendroglia from myelin to cytoplasmic inclusions in multiple system atrophy. Am J Pathol 171: , 1291–1303. |
[506] | Kovacs GG , Laszlo L , Kovacs J , Jensen PH , Lindersson E , Botond G , Molnar T , Perczel A , Hudecz F , Mezo G , Erdei A , Tirian L , Lehotzky A , Gelpi E , Budka H , Ovadi J ((2004) ) Natively unfolded tubulin polymerization promoting protein TPPP/p25 is a common marker of alpha-synucleinopathies. Neurobiol Dis 17: , 155–162. |
[507] | Lehotzky A , Lau P , Tokesi N , Muja N , Hudson LD , Ovadi J ((2010) ) Tubulin polymerization-promoting protein (TPPP/p25) is critical for oligodendrocyte differentiation. Glia 58: , 157–168. |
[508] | Hlavanda E , Kovacs J , Olah J , Orosz F , Medzihradszky KF , Ovadi J ((2002) ) Brain-specific p25 protein binds to tubulin and microtubules and induces aberrant microtubule assemblies at substoichiometric concentrations. Biochemistry 41: , 8657–8664. |
[509] | Lindersson E , Lundvig D , Petersen C , Madsen P , Nyengaard JR , Hojrup P , Moos T , Otzen D , Gai WP , Blumbergs PC , Jensen PH ((2005) ) p25alpha stimulates alpha-synuclein aggregation and is co-localized with aggregated alpha-synuclein in alpha-synucleinopathies. J Biol Chem 280: , 5703–5715. |
[510] | Ota K , Obayashi M , Ozaki K , Ichinose S , Kakita A , Tada M , Takahashi H , Ando N , Eishi Y , Mizusawa H , Ishikawa K ((2014) ) Relocation of p25 inverted question mark/tubulin polymerization promoting protein from the nucleus to the perinuclear cytoplasm in the oligodendroglia of sporadic and COQ2 mutant multiple system atrophy. Acta Neuropathol Commun 2: , 136. |
[511] | Kovacs GG , Breydo L , Green R , Kis V , Puska G , Lorincz P , Perju-Dumbrava L , Giera R , Pirker W , Lutz M , Lachmann I , Budka H , Uversky VN , Molnar K , Laszlo L ((2014) ) Intracellular processing of disease-associated alpha-synuclein in the human brain suggests prion-like cell-to-cell spread. Neurobiol Dis 69: , 76–92. |
[512] | Ettle B , Reiprich S , Deusser J , Schlachetzki JC , Xiang W , Prots I , Masliah E , Winner B , Wegner M , Winkler J ((2014) ) Intracellular alpha-synuclein affects early maturation of primary oligodendrocyte progenitor cells. Mol Cell Neurosci 62: , 68–78. |
[513] | May VE , Ettle B , Poehler AM , Nuber S , Ubhi K , Rockenstein E , Winner B , Wegner M , Masliah E , Winkler J ((2014) ) alpha-Synuclein impairs oligodendrocyte progenitor maturation in multiple system atrophy. Neurobiol Aging 35: , 2357–2368. |
[514] | Yazawa I , Giasson BI , Sasaki R , Zhang B , Joyce S , Uryu K , Trojanowski JQ , Lee VM ((2005) ) Mouse model of multiple system atrophy alpha-synuclein expression in oligodendrocytes causes glial and neuronal degeneration. Neuron 45: , 847–859. |
[515] | Kragh CL , Fillon G , Gysbers A , Hansen HD , Neumann M , Richter-Landsberg C , Haass C , Zalc B , Lubetzki C , Gai WP , Halliday GM , Kahle PJ , Jensen PH ((2013) ) FAS-dependent cell death in alpha-synuclein transgenic oligodendrocyte models of multiple system atrophy. PLoS One 8: , e55243. |
[516] | Urbizu A , Canet-Pons J , Munoz-Marmol AM , Aldecoa I , Lopez MT , Compta Y , Alvarez R , Ispierto L , Tolosa E , Ariza A , Beyer K ((2015) ) Cystatin C is differentially involved in multiple system atrophy phenotypes. Neuropathol Appl Neurobiol 41: , 507–519. |
[517] | Fellner L , Jellinger KA , Wenning GK , Stefanova N ((2011) ) Glial dysfunction in the pathogenesis of alpha-synucleinopathies: Emerging concepts. Acta Neuropathol 121: , 675–693. |
[518] | Weiss G , Goodnough LT ((2005) ) Anemia of chronic disease. N Engl J Med 352: , 1011–1023. |
[519] | Csencsits-Smith K , Suescun J , Li K , Luo S , Bick DL , Schiess M ((2017) ) Serum lymphocyte-associated cytokine concentrations change more rapidly over time in multiple system atrophy compared to Parkinson disease. Neuroimmunomodulation 23: , 301–308. |
[520] | Rydbirk R , Elfving B , Andersen MD , Langbol MA , Folke J , Winge K , Pakkenberg B , Brudek T , Aznar S ((2017) ) Cytokine profiling in the prefrontal cortex of Parkinson’s disease and multiple system atrophy patients. Neurobiol Dis 106: , 269–278. |
[521] | Wullner U , Weller M , Kornhuber J , Bornemann A , Schulz JB , Riederer P , Klockgether T ((2000) ) Altered expression of calcium- and apoptosis-regulating proteins in multiple system atrophy Purkinje cells. Mov Disord 15: , 269–275. |
[522] | Tanji K , Odagiri S , Maruyama A , Mori F , Kakita A , Takahashi H , Wakabayashi K ((2013) ) Alteration of autophagosomal proteins in the brain of multiple system atrophy. Neurobiol Dis 49: , 190–198. |
[523] | Schwarz L , Goldbaum O , Bergmann M , Probst-Cousin S , Richter-Landsberg C ((2012) ) Involvement of macroautophagy in multiple system atrophy and protein aggregate formation in oligodendrocytes. J Mol Neurosci 47: , 256–266. |
[524] | Nakamura S , Kawamoto Y , Kitajima K , Honjo Y , Matsuo A , Nakano S , Akiguchi I ((2001) ) Immunohistochemical localization of phosphoinositide 3-kinase in brains with multiple system atrophy. Clin Neuropathol 20: , 243–247. |
[525] | Furukawa Y , Vigouroux S , Wong H , Guttman M , Rajput AH , Ang L , Briand M , Kish SJ , Briand Y ((2002) ) Brain proteasomal function in sporadic Parkinson’s disease and related disorders. Ann Neurol 51: , 779–782. |
[526] | Xilouri M , Brekk OR , Stefanis L ((2016) ) Autophagy and alpha-synuclein: Relevance toarkinson’s disease and related synucleopathies. Mov Disord 31: , 178–192. |
[527] | Ubhi K , Low P , Masliah E ((2011) ) Multiple system atrophy: A clinical and neuropathological perspective. Trends Neurosci 34: , 581–590. |
[528] | Beraud D , Hathaway HA , Trecki J , Chasovskikh S , Johnson DA , Johnson JA , Federoff HJ , Shimoji M , Mhyre TR , Maguire-Zeiss KA ((2013) ) Microglial activation and antioxidant responses induced by the Parkinson’s disease protein alpha-synuclein. J Neuroimmune Pharmacol 8: , 94–117. |
[529] | Kuzdas-Wood D , Fellner L , Premstaller M , Borm C , Bloem B , Kirik D , Wenning GK , Stefanova N ((2015) ) Overexpression of alpha-synuclein in oligodendrocytes does not increase susceptibility to focal striatal excitotoxicity. BMC Neurosci 16: , 86. |
[530] | Castro Caldas A , Levin J , Djaldetti R , Rascol O , Wenning G , Ferreira JJ ((2017) ) Critical appraisal of clinical trials in multiple system atrophy: Toward better quality. Mov Disord 32: , 1356–1364. |