Methyl-Beta-Cyclodextrin Restores KIR Channel Function in Brain Endothelium of Female Alzheimer’s Disease Mice
Abstract
Background:
As the sixth-leading cause of death in the United States, Alzheimer’s disease (AD) entails deteriorating endothelial control of blood flow throughout the brain. In particular, reduced inward-rectifying K+ (KIR) channel function in animal models of aging and AD compromises endothelial function and optimal perfusion of brain parenchyma. Deficient endothelial KIR channels may result from aberrant interaction with plasma membrane cholesterol as a primary regulator of membrane fluidity and ion channels.
Objective:
We tested the hypothesis that mild methyl-β-cyclodextrin (MβCD) treatment to reduce membrane cholesterol may restore endothelial KIR channel function in brain endothelium of old AD mice.
Methods:
Membrane potential was continuously measured in isolated endothelial tubes from posterior cerebral arteries of young (1 to 3 months) and old (16 to 19 months) female 3xTg-AD mice before and after mild treatment with the cholesterol-removing agent MβCD (1 mmol/L). Elevated extracellular potassium ([K+]E; 15 mmol/L) and NS309 (1μmol/L) activated KIR and Ca2+-activated K+ (SKCa/IKCa) channels respectively before and after MβCD treatment.
Results:
SKCa/IKCa channel function for producing hyperpolarization remained stable regardless of age group and MβCD treatment (ΔVm: ∼–33 mV). However, as deficient during AD, KIR channel function was restored (ΔVm: –9±1 mV) versus pre-MβCD conditions (–5±1 mV); a progressive effect that reached –14±1 mV hyperpolarization at 60 min following MβCD washout.
Conclusion:
In female animals, MβCD treatment of brain endothelium selectively restores KIR versus SKCa/IKCa channel function during AD. Thus, the endothelial cholesterol-KIR channel interface is a novel target for ameliorating perfusion of the AD brain.
INTRODUCTION
Alzheimer’s disease (AD) is the leading type of dementia (∼6.2 million people in the United States alone) and continues to rise in parallel with an increasingly aging demographic of individuals of age 65 years and older [1]. Moreover, females in the general population indicate a higher prevalence, severity, and rate of AD pathogenesis even after correcting for age [2, 3]. Thus, especially in the context of biological sex, the etiology of AD remains obscure with no effective treatment strategies in place accordingly. Emerging evidence suggests maintenance or restoration of cerebrovascular health for optimal brain perfusion as a solution. In particular, how endothelial function may be harnessed to prevent dementia per conditions of aging [4, 5] and development of AD pathology [6–8].
In addition to pre-capillary small- and intermediate-Ca2+-activated K+ (SKCa/IKCa) channels, endothelial inward-rectifying K+ (KIR) channels are integral to cerebral blood flow regulation [9, 10]. Our group has demonstrated that the hyperpolarizing function of endothelial KIR channels of posterior cerebral arteries (corresponding to vasodilation and maintenance of cerebral blood flow) is reduced during conditions of aging [5] and AD [7]. Further, another recent study observed lower KIR2 channel current density in brain capillary endothelium in 5xFAD mice (age, 12 to 13 months; severe AD pathology as neuronal loss [11]), with overall consequences of diminished functional hyperemia in response to both whisker stimulation and elevated K+ [8]. In turn, this study identified a potential therapeutic role for membrane lipid regulation via enhanced phosphatidylinositol 4,5-bisphosphate (PIP2) supply to restore deficient cerebrovascular KIR2 channels [8].
As an additional key component of lipid regulation while integral to development of AD pathology [12–14], membrane cholesterol may also inactivate vascular KIR2 channels during conditions of health [15] and hypercholesterolemia [16, 17], with the latter most pronounced for diminished endothelial KIR2 channel function in particular. This regulatory role for cholesterol has not been tested using direct electrophysiological measurements of freshly isolated and intact endothelium in a study model of AD. Thus, we have tested the hypothesis that mild methyl- β-cyclodextrin (M βCD) treatment to reduce membrane cholesterol may restore KIR channel function in brain endothelium of old AD mice. With a central emphasis on females, membrane potential (Vm) was measured in posterior cerebral endothelial tubes of young (1 to 3 months) and old (16 to 19 months) 3xTg-AD mice. The function of SKCa/IKCa and KIR2 channels underlying endothelium-derived hyperpolarization (EDH) [18, 19] were examined before and after mild treatment with the cholesterol-removing agent methyl-β-cyclodextrin (MβCD; 1 mmol/L). SKCa/IKCa channel function was stable throughout conditions regardless of MβCD treatment. Remarkably, reduced KIR2 channel function during advanced AD was restored to pre-AD conditions following presence of MβCD. This selective restoration of KIR2 channel function was likely due to mild membrane cholesterol removal and/or disruption in cerebrovascular endothelium during AD pathology. Altogether, cholesterol-KIR channel interaction plays a key role in cerebrovascular endothelial KIR2 channel downregulation during AD and direct modification of membrane cholesterol may ameliorate associated deficits in cerebral perfusion and dementia.
METHODS
Animal care and use
All animal care use and experimental protocols for this study were approved by the Institutional Animal Care and Use Committee of Loma Linda University and performed in accord with the National Research Council’s “Guide for the Care and Use of Laboratory Animals” (8th Edition, 2011). Experiments were performed using young Pre-AD (1–3 months; females, 31 animals) and old AD (16–19 months; females, 39 animals) 3xTg-AD mice [20] generated from homozygous breeding pairs obtained from the Jackson Laboratories [Wilmington, MA, USA; (B6;129-Tg (APPSwe,tauP301L) 1Lfa Psen1tm1Mpm/Mmjax); Mutant Mouse Resource and Research Center (MMRRC) stock #034830]. All animals were housed on a 12:12 h light-dark cycle at 22–24°C with fresh water and food available ad libitum. For individual experiments, animals were selected randomly with respect to group and biological sex.
Solutions and reagents
Preparation and composition of all solutions have been previously described for measurements of Vm in intact endothelium freshly isolated from mouse posterior cerebral arteries [5, 7, 21, 22]. Briefly, physiological salt solution (PSS; pH 7.4) was prepared for continuous superfusion of cerebral endothelial tubes [(in mmol/L): 140 NaCl, 5 KCl, 2 CaCl2, 1 MgCl2, 10 HEPES, 10 Glucose]. For dissection of brain and arteries, PSS lacking CaCl2 contained 0.1%bovine serum albumin. For isolation of intact endothelium, an enzyme cocktail [0.31 mg/mL papain (P4762, Sigma), 0.5 mg/mL dithioerythritol (D8255, Sigma), 0.75 mg/mL collagenase (C8051, Sigma), and 0.13 mg/mL elastase (E7885, Sigma)] was prepared in dissociation PSS containing reduced Ca2+ (0.1 mmol/L CaCl2) and 0.1%bovine serum albumin. The 15 mmol/L KCl solution was prepared with an equimolar decrease of NaCl accordingly in order to maintain osmolarity. All reagents were purchased from Sigma-Aldrich (St. Louis, MO, USA) or ThermoFisher Scientific (Pittsburgh, PA, USA) unless otherwise indicated.
Dissection of cerebral arteries and isolation of endothelial tubes
Sequential dissection of mouse brain for preparation of posterior cerebral arteries and isolation of arterial endothelium have been previously described and illustrated in our previous studies [5, 7, 21, 22]. Briefly, following isoflurane inhalation for anesthesia and decapitation, the brain was acutely removed and placed in chilled (4°C) dissection PSS. Posterior cerebral arteries were carefully dissected from the brain and cut into segments. Arterial segments were partially digested in dissociation PSS containing the enzyme cocktail at 34°C for 10 to 12 min. Following digestion, arterial segments were triturated using borosilicate glass capillary tubes [1B100-4, World Precision Instruments (WPI), Sarasota, FL, USA] with an internal tip diameter of 80–120μm to remove adventitia, smooth muscle cells, and internal elastic lamina to isolate intact endothelial tubes. Endothelial tubes were continuously superfused with PSS at 37°C.
Recording of intracellular Vm
Recording of Vm in mouse cerebral endothelium has been previously described and illustrated [5, 7, 21, 22]. Briefly, Vm of isolated endothelial tubes was recorded with Axoclamp electrometers (2B and/or 900A; Molecular Devices, Sunnyvale, CA) using microelectrodes pulled (P-97, Sutter Instruments) from glass capillary tubes (GC100F-10, Warner Instruments, Holliston, MA, USA). Under continuous superfusion with PSS, an endothelial cell was penetrated with a microelectrode backfilled with 2 mol/L KCl (tip resistance: ∼150 MΩ) while viewing the endothelial tube at 400×magnification. Amplifier outputs were connected to a data acquisition system (Digidata 1550A; Molecular Devices), whereby all data were acquired at a 10 Hz frequency on a Hewlett-Packard personal computer using Axoscope software (Molecular Devices). Each experiment included a continuous intracellular Vm recording for ∼2 h.
Cholesterol assay of cerebral blood vessels
Cholesterol content was determined in total blood vessel content in the brain using Cholesterol Quantification Assay Kit (Sigma) in accord with manufacturer’s instructions. Brain microvessel fragments were isolated from the mouse brains using a previously illustrated protocol [23]. Cholesterol content (μmol/L) was normalized to total phospholipid (μmol/L) determined from the same blood vessel isolates using Phospholipid Assay Kit (Sigma) according to manufacturer’s instructions.
Cholesterol imaging of endothelial cell tubes
Endothelial cell tubes were secured on a glass coverslip with glass micropipettes using two micromanipulators and superfused with PSS while gradually raising the bath temperature to 37°C. Endothelial cell membrane cholesterol was detected by incubating cells with Filipin-III (50μg/ml, Sigma) at 37°C for ∼1.5 h under light-protected conditions. The cells were then washed with fresh PSS and images were acquired using a fluorescent microscope (Eclipse Ti-S; Nikon), a 60X objective [Plan Apo λ (numerical aperture: 0.95); Nikon)], a solid-state light source containing a violet channel (MIRA Light Engine; Lumencor, Inc., Beaverton, OR, USA), a 16-megapixel monochrome camera (DS-Qi2, Nikon), and Nikon imaging software (NIS Elements-F 4.60.00). For unpaired image acquisitions prior to staining with Filipin, endothelial cell tubes were treated with methyl-β-cyclodextrin (MβCD) for 20 min followed by 1 h washout period at 37°C. For ideal visibility, all images illustrating Filipin staining were pseudo-colored green.
Pharmacology
An effective half-maximal concentration of NS309 (EC50, ∼1μmol/L; 3895, Tocris, Bristol, England) was used to directly evaluate function of SKCa/IKCa channels to produce hyperpolarization [5, 22]. As consistent with our previous studies [22], DMSO solvent (≤0.1%) for working stocks of NS309 was found to have no effect on endothelial Vm on its own for≤5 min applications used for respective drug treatments in cerebrovascular endothelial tubes. Elevated level of extracellular KCl ([K+]o: 15 mmol/L) was used to stimulate endothelial KIR2 channels for hyperpolarization [22, 24]. MβCD (1μmol/L; 332615, Sigma) was prepared in PSS to reduce cholesterol in the endothelial cell membrane [25, 26]. Respective K+ channel activating agents were applied before and after washout of a 20 min application of MβCD treatment. Resting Vm was typically allowed≥2 min to stabilize before application of a pharmacological agent, whereby each application was allowed sufficient time (≥3 min) to record peak Vm responses. In between individual applications, the endothelial tube was washed with PSS to baseline conditions.
Data and statistical analysis
For quantification of cholesterol levels normalized to total phospholipid content, n = 1 entails one experiment using a total blood vessel sample pooled from three mouse brains of each group (pre-AD versus AD). For Vm recordings, data analyses included resting Vm (mV) and change in Vm (ΔVm) = peak response Vm –preceding baseline Vm. Summary data represent values averaged over 10 s during stable recordings. Filipin-III image acquisitions and Vm recordings represent the number (n) of independent experiments, each representing one endothelial tube from one mouse.
For simultaneous comparisons among both age groups±MβCD treatment, statistical analysis included a two-way Analysis of Variance (ANOVA) with Tukey’s post hoc correction for multiple comparisons. One-way ANOVA with Tukey’s post hoc examined statistical significance for respective AD groups for pre-MβCD conditions versus post-MβCD conditions at both 30 min and 60 min time points. The two-tailed unpaired t-test was used for select comparisons between two groups as pre-AD versus AD data sets (e.g., cholesterol measurements, pre- or post-MβCD treatment). Two-tailed paired student’s t-tests were used to analyze select comparisons for pre-MβCD versus post-MβCD within the Pre-AD or AD group. All graph preparation and statistical analyses were performed using GraphPad Prism (La Jolla, CA, USA). Differences between groups were accepted as statistically significant with p < 0.05. All summary data are presented as the mean±SEM. Note that the absence of statistically significant differences does not necessarily rule out physiological or pathological importance of respective study group comparisons that indicate p > 0.05.
RESULTS
The goal of this study was to ascertain the mechanism of how cerebrovascular endothelial KIR2 channel function is reduced during AD pathology as observed in our recent study [7]. In accord with our current hypothesis, we examined the potential impact of membrane cholesterol on endothelial K+ channel function for hyperpolarization of Vm as components of EDH during development of AD pathology. As the most impacted biological sex in the clinical AD population, we chose to center the current studies on use of females [2, 3]. It should be noted that female 3xTg-AD mice also live consistently to≥16 months in age [27, 28]. Relatively high concentrations of MβCD (5 mmol/L, 60 min) enhance endothelial KIR2 channel currents by > 3-fold during conditions of hypercholesterolemia [16]. Thus, we applied a relatively mild MβCD treatment (1 mmol/L, 20 min; [25]) in an attempt to restore deficient KIR2 channel-dependent hyperpolarization in cerebral endothelium during AD to pre-AD conditions if possible. Continuous Vm recordings were applied in endothelial tubes (width: ∼90μm, length:≥300μm) freshly isolated from 3xTg-AD mouse posterior cerebral arteries for ∼2 hr [7]. Changes in Vm were recorded in response to NS309 (SKCa/IKCa channel opener) and 15 mmol/L KCl (KIR2 channel activator) before and after treatment of endothelial cells with MβCD followed by washout for up to 60 min. With application of this procedure, a significant shift in Vm was not evident during the presence and washout of MβCD alone (ΔVm: –1±1 mV). Here, we demonstrate selective restoration of KIR2 channel function in freshly isolated and intact cerebrovascular endothelium of old female AD animals via targeting membrane cholesterol.
Total cholesterol content in cerebral blood vessels during conditions of pre-AD and AD
Cholesterol dyshomeostasis is known to underlie conditions of atherosclerosis per aging [29] while the presence of the apolipoprotein E (APOE) ɛ4 allele in particular is a major risk factor for development of AD [14, 30] and associated cerebrovascular pathology [31]. Thus, we first sought to determine cholesterol levels in isolated cerebral vessels of 3xTg-AD animals during pre-AD and AD conditions. Although the mean value for the total cholesterol:phospholipid ratio is ∼40%higher for AD (0.345±0.120) versus Pre-AD (0.242±0.068), this difference is not statistically significant (p = 0.48; unpaired t-test) (Fig. 1; n = 5 independent experiments for each group, 3 animals pooled per experiment; 15 animals total per group) and should be interpreted with caution. Thus, these data suggest that cerebral blood vessels may or may not be enriched with excessive levels of cholesterol among individual animals during conditions of AD.
Fig. 1
Cerebrovascular cholesterol content among pre-AD and AD groups. Data represent molar ratio of cholesterol content per total phospholipid content for respective groups. n = number of experiment for each group (Pre-AD: 5 & Old AD: 5), whereby each group consisted of pooled purified cerebral blood vessels isolated from three female mice.
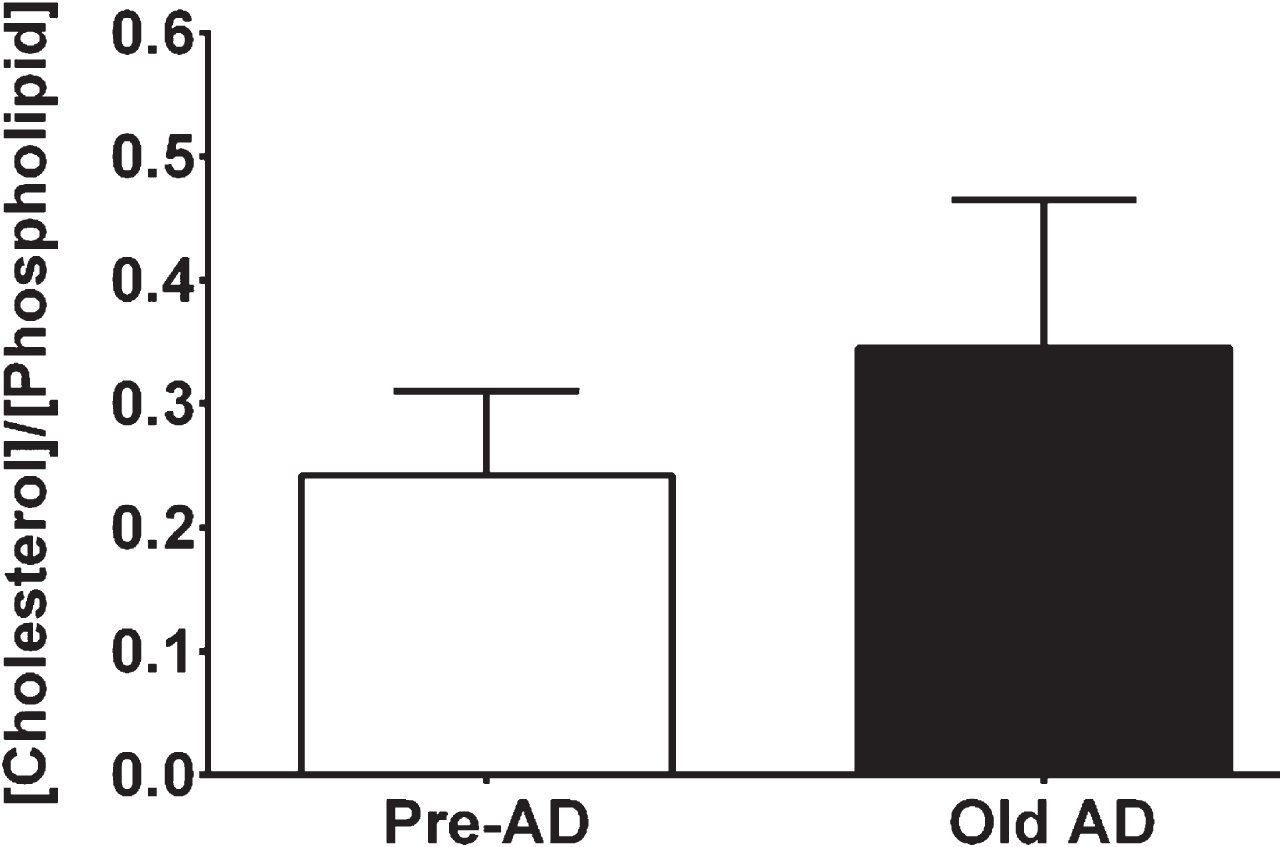
Membrane cholesterol in endothelial cell tubes during conditions of pre-AD and AD
Presence of cholesterol inclusion bodies or “crystals” appear in endothelial cells during conditions of disease such as atherogenesis [26] and can be removed using MβCD [32]. With use of Filipin to stain membrane cholesterol [26, 32], we observed fluorescent crystals in endothelial tubes during AD pathology (Fig. 2F; 15.3±0.7 crystals/mm2) that were significantly reduced in number (Fig. 2H; 3.6±0.9 crystals/mm2) following mild MβCD treatment (1μmol/L; 20 min incubation, 1 h washout) or in young, pre-AD animals regardless of MβCD treatment (Fig. 2B, D; 0.2±0.1 crystals/mm2). These data demonstrate that membrane cholesterol crystals are a feature of cerebrovascular endothelium during AD pathology which may impact function of K+ channels crucial to endothelial function underlying perfusion of the brain.
Fig. 2
Presence of cholesterol crystals in AD animals and reduction following MβCD treatment. Representative (A, C, E, G) brightfield and (B, D, F, H) Filipin-III images (green pseudo-color) of cerebrovascular endothelial cell tubes isolated from female animals. A, B) Pre-AD images without MβCD treatment. C, D) As shown in (A) & (B) respectively after MβCD treatment (1 mmol/L, 20 min incubation, & 1 h wash). E-H) As shown in (A), (B), (C), and (D) respectively for AD animals. White arrows indicate cholesterol “crystals” in endothelial tubes during AD conditions in the absence of MβCD treatment. The scale bar throughout panels represents 20μm. I) Summary data for number of crystals per unit area (mm2). n = number of animals and respective cerebral endothelial tubes (Pre-AD & Old AD: 4; Pre-AD & Old AD after MβCD: 3). *p < 0.05 (two-way ANOVA & paired t-test), Pre-AD or Old AD female Post-MβCD versus respective Pre-MβCD group; #p < 0.05 (two-way ANOVA & paired t-test), Old AD female Pre-MβCD versus Old AD female Post-MβCD.
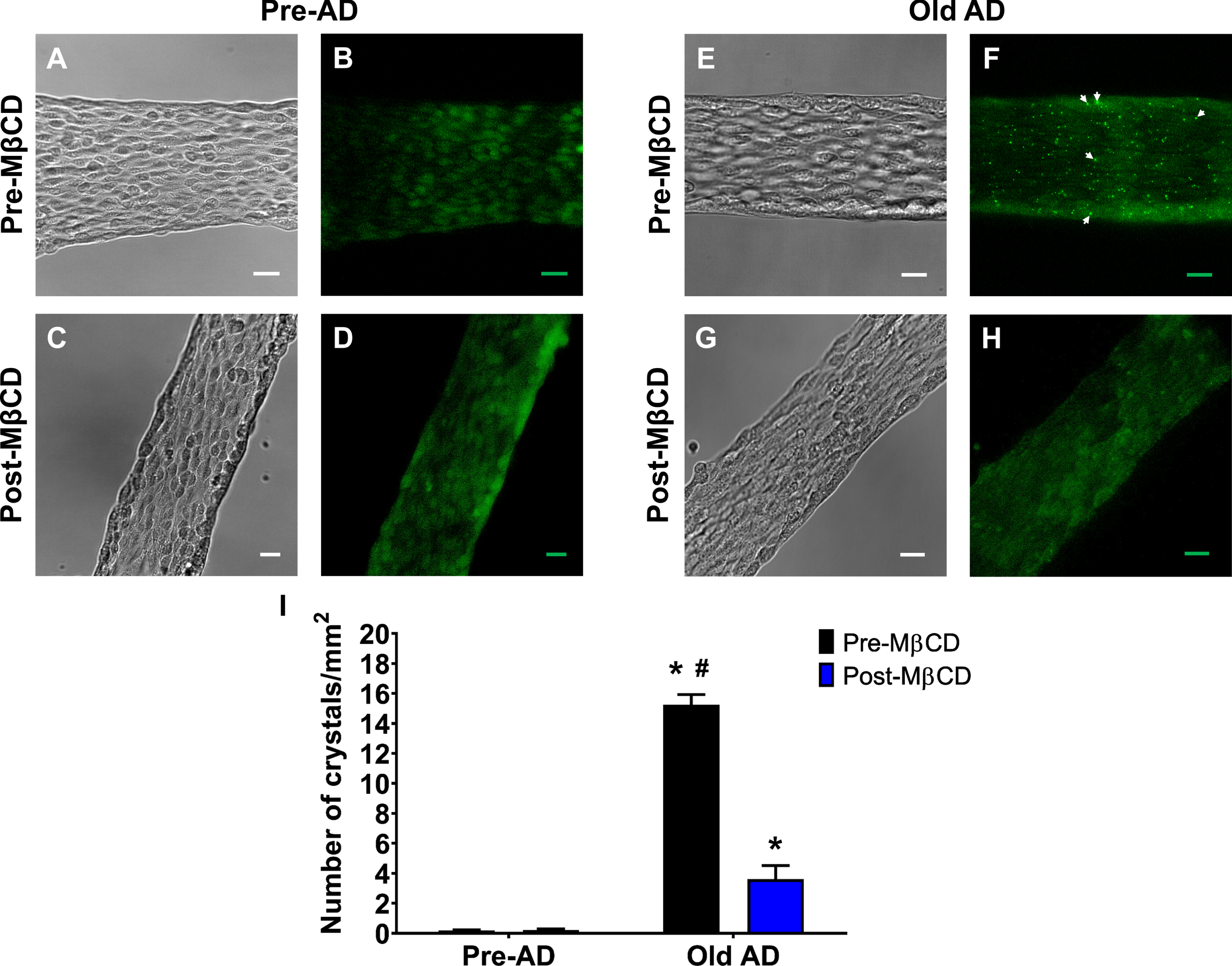
Effect of MβCD on cerebrovascular endothelial SKCa/IKCa channel function
Endothelial SKCa/IKCa channels are integral to a major blood flow regulation pathway as EDH in cerebral arteries [22, 33] and arterioles [34]. We have previously found that SKCa/IKCa channel function is preserved in females while modestly enhanced (∼20%) in males during AD pathology [7]. Accordingly, we found that peak hyperpolarization to NS309 (1μmol/L) as SKCa/IKCa channel activation was not altered by advanced AD pathology or MβCD treatment (1μmol/L) (Fig. 3; p > 0.05; ANOVA or t-tests). SKCa/IKCa channel-mediated hyperpolarization was as follows across female groups: Pre-AD (Pre-MβCD, 34.2±1.2 mV & Post-MβCD, 33.0±0.7 mV; n = 11) and Old AD (Pre-MβCD, 33.1±1.6 mV & Post-MβCD, 31.1±1.0 mV; n = 12) (Fig. 3B). Altogether, these data demonstrate that cerebrovascular endothelial SKCa/IKCa channel stability during AD pathology is not altered by MβCD treatment.
Fig. 3
SKCa/IKCa channel function is not impacted by conditions of AD or membrane cholesterol regulation. A) Representative recordings of SKCa/IKCa channel hyperpolarization in Old AD females in response to NS309 (1μmol/L) before and after 30 min washout of MβCD (1 mmol/L, 20 min). B) Summary data for peak ΔVm in response to SKCa/IKCa activation in Pre-AD versus Old AD females before and after MβCD treatment (30 min washout). n = number of animals and respective cerebral endothelial tubes (Pre-AD: 11; Old AD: 12).
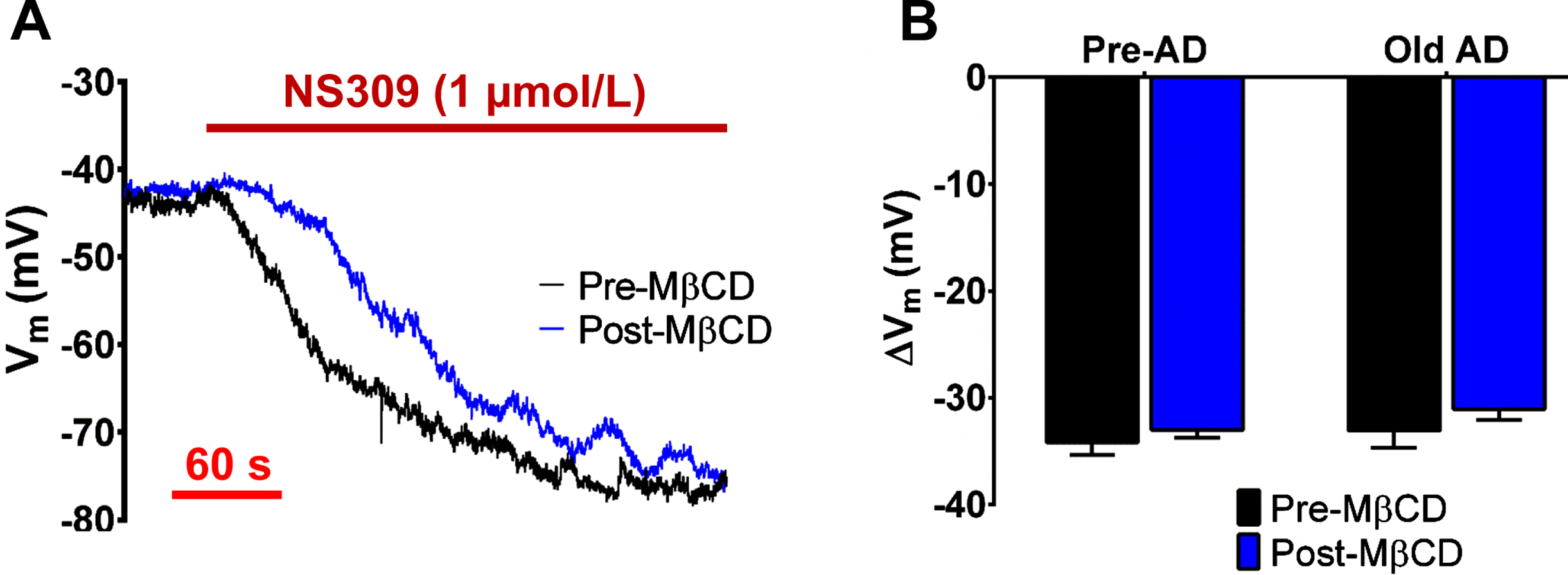
Impact of MβCD treatment on cerebrovascular endothelial KIR2 channel function
KIR2 channel function is crucial for cerebral vasodilation and blood flow [10, 15] and is impacted by conditions of hypercholesterolemia [16, 17]. Also, we have recently found reduced KIR2 channel function with advancing age [5] while exacerbated by conditions of AD [7] in both biological sexes. Thus, we tested whether treating with a relatively low dose of MβCD, an agent that lowers membrane cholesterol [25, 26], could restore KIR2 channel function in endothelial tubes from AD mice. Relative to SKCa/IKCa channel activation, there is a modest Vm hyperpolarization response to activation of cerebrovascular endothelial KIR2 channels with 15 mmol/L KCl (ΔVm ∼–5 to –10 mV; [5, 22]) (Fig. 4A). In female animals, we have found that hyperpolarization to 15 mmol/L KCl decreased by ∼40%(p < 0.05, unpaired t-test) from young pre-AD (7.3±0.6 mV) to old AD (4.5±0.8 mV) (Fig. 4B). As an approximate wild-type reference to the AD animals, age-matched (16–19 months) C57BL/6 females respond to 15 mmol/KCl with 7.0±1.1 mV of hyperpolarization (n = 5) [5]; similar to the young Pre-AD group. Following MβCD treatment (30 min washout), both pre-AD and AD hyperpolarization responses were similar as ∼9±1 mV (pre-AD, 9.3±1.2 mV & AD, 8.5±0.8 mV). In such manner, a statistically significant increase by ∼2-fold (p < 0.05; two-way ANOVA & paired t-test) was only observed in AD animals versus paired pre-MβCD measurements (Fig. 4A, B). Furthermore, we found that hyperpolarization responses were additionally enhanced following a 60-min washout time period after MβCD treatment, whereby hyperpolarization responses for both pre-AD (13.3±1.9 mV) and AD (13.6±1.2 mV) females were statistically significant versus respective pre-MβCD conditions (p < 0.05; One-way ANOVA). As a reference, an approximate time period of incubation with PSS (washout solution and vehicle for MβCD) did not significantly alter hyperpolarization to 15 mM KCl (n = 4 AD animals; 0 h, 4.3±0.6 mV & 1.5 h, 5.3±0.9 mV). Altogether, these data suggest that a relatively low concentration of MβCD, an agent known to reduce membrane cholesterol, can restore (with potential to further enhance) cerebrovascular endothelial KIR2 channel function during conditions of advanced AD in females.
Fig. 4
Reduction of membrane cholesterol restores endothelial KIR2 channel function during AD pathology. A) Representative recordings of KIR2 channel-mediated hyperpolarization in Old AD females in response to 15 mM KCl before and after 30 min washout of MβCD (1 mmol/L, 20 min). B) Summary data for peak ΔVm in response to KIR2 channel activation in Pre-AD versus Old AD females before and after MβCD treatment (30 min washout). Note that both Pre-AD and Old AD females show similar hyperpolarization to 15 mM KCl as ∼9 mV following MβCD treatment. n = number of animals and respective cerebral endothelial tubes (Pre-AD: 9; Old AD: 12). *p < 0.05 (unpaired t-test) for Old AD Female Pre-MβCD versus Pre-AD Female Pre-MβCD; #p < 0.05 (two-way ANOVA & paired t-test), Old AD female Post-MβCD versus Old AD female Pre-MβCD.
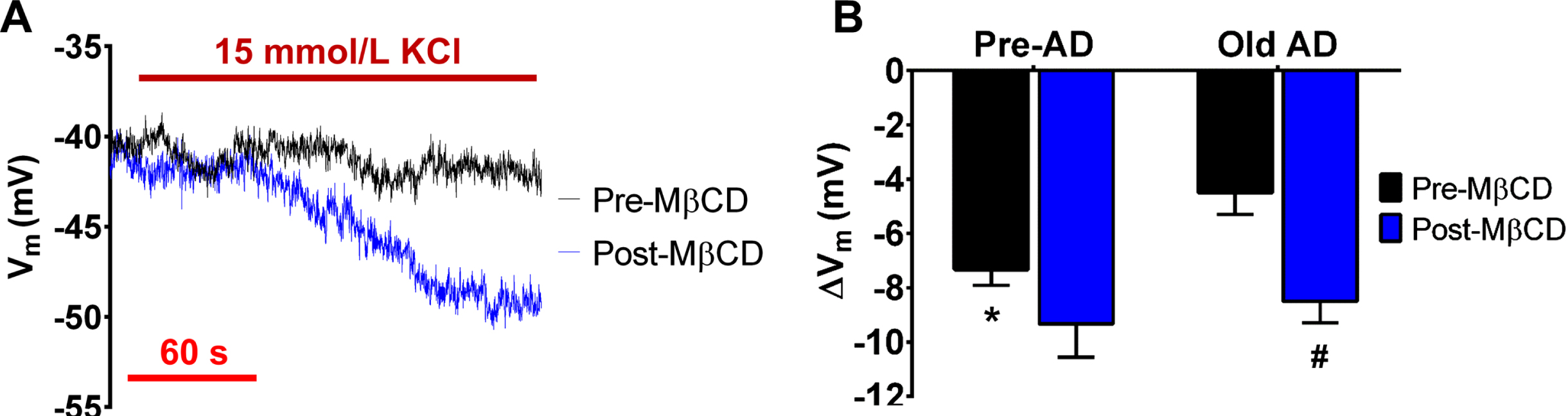
DISCUSSION
Understanding blood flow regulation throughout the cerebrovascular network is crucial because chronic, inadequate delivery of oxygen and nutrients throughout the brain contributes to neurodegenerative pathology such as AD [6, 35]. Further, with recognition of the endothelium as an organ that “feeds” all other organs throughout the body [18], naturally, endothelial function has become pivotal for fundamental studies of brain health [9, 36, 37], the aging brain [4, 5, 38], and select conditions of AD [7, 8]. In particular for AD, there are remaining knowledge gaps for potentially altered functions of cerebrovascular endothelial ion channels per blood flow regulation, primarily as the transient receptor potential [39–41] and K+ channels (e.g., KCa and KIR subtypes; [7, 8, 39, 42]). In a recent study [7], we have demonstrated that endothelial KIR channel function of posterior cerebral arteries is reduced during AD by ∼50%, whereas SKCa/IKCa channels are relatively stable. This general finding has also been reinforced for KIR channel function in capillary endothelium during AD [8], altogether establishing a premise for mechanistic investigation and corresponding therapeutic strategies involving cerebrovascular K+ channels. With a basis of KIR channels modulated by membrane cholesterol [17, 43] and cholesterol dysregulation playing a key role in AD etiology [14, 31], the goal of this study was to investigate whether mild MβCD treatment to reduce membrane cholesterol would significantly improve cerebrovascular KIR channel function in old 3xTg-AD animals. Whereas SKCa/IKCa channel function was not altered as otherwise stable from pre-AD to AD, treatment with MβCD effectively restored KIR channel function to the level of pre-AD, supporting cholesterol-KIR channel interaction as a key therapeutic target during neurodegenerative disease.
Endothelial membrane cholesterol and use of cyclodextrin for treatment of AD
Cholesterol is essentially present in the plasma membrane of all mammalian cells as one of the major lipid components for cellular growth and function, whereby its excess beyond physiological levels is generally associated with the development of disease including AD [14, 44–46]. Although not statistically significant, we have found that the mean for cerebrovascular cholesterol (normalized to total phospholipid content) was ∼40%higher in AD versus pre-AD animals (Fig. 1). Thus, our data for cellular cholesterol content suggest that cerebral blood vessels may or may not be enriched with excessive levels of cholesterol among individual animals during conditions of AD. However, with use of fluorescent Filipin staining, we observed apparent cholesterol crystals in endothelial membranes of AD animals that were substantially reduced in number by ∼75%following MβCD treatment (Fig. 2). In females, elevated cholesterol levels in human frontal cortex [47] and rodent endothelial plasma membranes [48] have been shown during conditions of aging as a key risk factor for cognitive decline towards AD [1].
Cyclodextrins such as MβCD are cyclic oligosaccharide compounds and have been widely used to bind to cholesterol in a concentration-, time-, temperature-, and cell type-dependent manner [25]. With a reference point of 5 mmol/L MβCD (30 to 60 min incubation) for patch-clamp measurements of rodent vascular cells (brain [15], mesentery [16]), we determined that 1 mmol/L MβCD (20 min) was sufficient for restoration of reduced KIR channel-dependent hyperpolarization for AD animals following 30 min of MβCD washout. In addition to the precision in time for various drug-response measurements, it should be noted that a major strength of our electrophysiological abilities encompasses a scale of hours (versus seconds or minutes) [21] and thus, we encompassed paired examinations of Pre-MβCD and Post-MβCD conditions throughout individual endothelium preparations.
SKCa/IKCa channel function is unaffected, whereas KIR channel function is restored by mild MβCD treatment of cerebrovascular endothelial membrane cholesterol during AD
Endothelial SKCa/IKCa channels are vital for cerebral blood flow regulation [33, 34], whereby their function is not impaired by conditions of AD alone [7]. Accordingly, the current study demonstrates that MβCD has no significant impact on SKCa/IKCa channel activity to produce membrane hyperpolarization (Fig. 3), suggesting that membrane cholesterol does not play a major role for regulation of cerebrovascular SKCa/IKCa during health and disease. Overall, this lack of effect of MβCD treatment on SKCa/IKCa channel function is consistent with previous studies (reviewed in [49]). As highly expressed in smooth muscle cells [50], large conductance KCa (BKCa) channels are suppressed [51] or activated in response to membrane-enriched cholesterol depending on expression of KCNMB1 and trafficking of BKCa β1 subunits [52].
KIR channels are integral to neurovascular coupling and the overall coordination of cerebral pre-capillary vasodilation and blood flow [10, 53] with a unique capacity for also boosting SKCa/IKCa channel hyperpolarization [54, 55]. Elevated membrane cholesterol suppresses endothelial KIR2 channel currents [16, 17, 45] by interacting with a CD loop region of the C-terminus as a cytosolic component of the channel [56]. Remarkably, we found that KIR2 channel-dependent hyperpolarization increased progressively with time of washout following MβCD treatment to ∼9 mV and ∼14 mV for both female Pre-AD and AD animals at 30 min and 60 min respectively (Fig. 4 and Results text). The enhancement of hyperpolarization responses in pre-AD animals was unexpected but previous evidence suggests that, even in healthy humans, normal cholesterol levels can suppress endothelial function [57]. Also, we should note that mechanisms of local control of KIR channels can occur via glycocalyx regulation as well [43]. Relative contributions of membrane cholesterol-dependent versus -independent modulation of endothelial ion channels remain to be resolved for conditions of AD.
Experimental considerations and potential treatment strategies
Although KIR2 channels are also present on cerebrovascular smooth muscle cells [10, 15], we studied the key component of coordinated electrical signaling of cerebral blood flow control as the endothelium [9, 22]. In addition to retention of in vivo properties as Vm regulation and intercellular electrical coupling [22], the mechanical stability of endothelial cells (versus smooth cells) for continuous, long-term electrophysiological recordings is an experimental advantage as well. It should be noted that another recent study found that cholesterol primarily regulates cerebral arterial smooth muscle versus endothelial KIR2 channels in young (10 to 12 weeks) female rats [15]. Thus, with knowing that cholesterol can also impact smooth muscle KIR2 channels in intact cerebral arteries [15], we believe that the current study demonstrates the general concept that mild treatment with MβCD can alleviate suppressed KIR2 channel function during conditions of AD [7, 8]. Therapy via PIP2 restoration may also restore capillary endothelial KIR2 channel function for improved neurovascular coupling and cerebral blood flow during AD [8]. However, PIP2 is also a key dynamic component of constitutive, moment-to-moment physiology (e.g., intracellular Ca2+ signaling), whereby its hydrolysis following activation of Gq-protein coupled receptors [58], can notably facilitate TRPV4 activation while suppressing KIR2 channels [59]. Although such investigations of PIP2 are mechanistically informative, we suggest that a mild reduction of membrane cholesterol may serve as a more effective strategy for improving endothelial KIR2 channel function with significantly less impact on constitutive events governing basal cerebral blood flow.
SUMMARY AND CONCLUSIONS
Impairment of cerebral blood flow during AD is due, in large part, to endothelial dysfunction in the brain. Therefore, it is crucial to locate mechanisms and therapies that center on improvement of cerebral perfusion. With following up from our recent studies demonstrating a functional decrease of endothelial KIR2 channels, the current study was strategically undertaken to restore KIR2 channel-dependent hyperpolarization in response to elevated extracellular K+. We found that mild application of a cholesterol removal agent as MβCD progressively restored and even enhanced KIR2 channel-dependent hyperpolarization while SKCa/IKCa channels were unaffected. Further, this finding was observed in females as the representative biological sex of the clinical AD population [2, 3]. Altogether, these findings suggest that the endothelial cholesterol-KIR channel interface may be a novel therapeutic target for ameliorating cerebrovascular function to optimize perfusion of the AD brain.
ACKNOWLEDGMENTS
This research has been supported by National Institutes of Health grant R56AG062169 (to E.J.B.). The content of this original article is solely the responsibility of the authors and does not necessarily represent the official views of the National Institutes of Health.
CONFLICT OF INTEREST
The authors have no conflict of interest to report.
REFERENCES
[1] | Alzheimer’s Association ((2021) ) 2021 Alzheimer’s disease facts and figures. Alzheimers Dement 17: , 327–406. |
[2] | Pike CJ ((2017) ) Sex and the development of Alzheimer’s disease. J Neurosci Res 95: , 671–680. |
[3] | Fisher DW , Bennett DA , Dong H ((2018) ) Sexual dimorphism in predisposition to Alzheimer’s disease. Neurobiol Aging 70: , 308–324. |
[4] | Toth P , Tarantini S , Csiszar A , Ungvari Z ((2017) ) Functional vascular contributions to cognitive impairment and dementia: Mechanisms and consequences of cerebral autoregulatory dysfunction, endothelial impairment, and neurovascular uncoupling in aging.H1-H. Am J Physiol Heart Circ Physiol 312: , 20. |
[5] | Hakim MA , Chum PP , Buchholz JN , Behringer EJ ((2020) ) Aging alters cerebrovascular endothelial GPCR and K+ channel function: Divergent role of biological sex. J Gerontol A Biol Sci Med Sci 75: , 2064–2073. |
[6] | Di Marco LY , Venneri A , Farkas E , Evans PC , Marzo A , Frangi AF ((2015) ) Vascular dysfunction in the pathogenesis of Alzheimer’s disease–A review of endothelium-mediated mechanisms and ensuing vicious circles. Neurobiol Dis 82: , 593–606. |
[7] | Hakim MA , Behringer EJ ((2020) ) Development of Alzheimer’s disease progressively alters sex-dependent KCa and sex-independent KIR channel function in cerebrovascular endothelium. J Alzheimers Dis 76: , 1423–1442. |
[8] | Mughal A , Harraz OF , Gonzales AL , Hill-Eubanks D , Nelson MT ((2021) ) PIP2 improves cerebral blood flow in a mouse model of Alzheimer’s disease. Function 2: , zqab010. |
[9] | Longden TA , Dabertrand F , Koide M , Gonzales AL , Tykocki NR , Brayden JE , Hill-Eubanks D , Nelson MT ((2017) ) Capillary K+-sensing initiates retrograde hyperpolarization to increase local cerebral blood flow. Nat Neurosci 20: , 717–726. |
[10] | Longden TA , Nelson MT ((2015) ) Vascular inward rectifier K+ channels as external K+ sensors in the control of cerebral blood flow. Microcirculation 22: , 183–196. |
[11] | Oakley H , Cole SL , Logan S , Maus E , Shao P , Craft J , Guillozet-Bongaarts A , Ohno M , Disterhoft J , Van Eldik L , Berry R , Vassar R ((2006) ) Intraneuronal beta-amyloid aggregates, neurodegeneration, and neuron loss in transgenic mice with five familial Alzheimer’s disease mutations: Potential factors in amyloid plaque formation. J Neurosci 26: , 10129–10140. |
[12] | Bos I , Vos SJB , Schindler SE , Hassenstab J , Xiong C , Grant E , Verhey F , Morris JC , Visser PJ , Fagan AM ((2019) ) Vascular risk factors are associated with longitudinal changes in cerebrospinal fluid tau markers and cognition in preclinical Alzheimer’s disease. Alzheimers Dement 15: , 1149–1159. |
[13] | Day GS , Cruchaga C , Wingo T , Schindler SE , Coble D , Morris JC ((2019) ) Association of acquired and heritable factors with intergenerational differences in age at symptomatic onset of Alzheimer disease between offspring and parents with dementia. JAMA Netw Open 2: , e1913491. |
[14] | Refolo LM , Malester B , LaFrancois J , Bryant-Thomas T , Wang R , Tint GS , Sambamurti K , Duff K , Pappolla MA ((2000) ) Hypercholesterolemia accelerates the Alzheimer’s amyloid pathology in a transgenic mouse model. Neurobiol Dis 7: , 321–331. |
[15] | Sancho M , Fabris S , Hald BO , Brett SE , Sandow SL , Poepping TL , Welsh DG ((2019) ) Membrane lipid-KIR2.x channel interactions enable hemodynamic sensing in cerebral arteries. Arterioscler Thromb Vasc Biol 39: , 1072–1087. |
[16] | Fancher IS , Ahn SJ , Adamos C , Osborn C , Oh MJ , Fang Y , Reardon CA , Getz GS , Phillips SA , Levitan I ((2018) ) Hypercholesterolemia-induced loss of flow-induced vasodilation and lesion formation in apolipoprotein E-deficient mice critically depend on inwardly rectifying K+ channels. J Am Heart Assoc 7: , e007430. |
[17] | Fang Y , Mohler ER , 3rd, Hsieh E , Osman H , Hashemi SM , Davies PF , Rothblat GH , Wilensky RL , Levitan I ((2006) ) Hypercholesterolemia suppresses inwardly rectifying K+ channels in aortic endothelium in vitro and in vivo. Circ Res 98: , 1064–1071. |
[18] | Behringer EJ ((2017) ) Calcium and electrical signaling in arterial endothelial tubes: New insights into cellular physiology and cardiovascular function. Microcirculation 24: , 10.1111/micc.12328. |
[19] | Behringer EJ , Hakim MA ((2019) ) Functional interaction among KCa and TRP channels for cardiovascular physiology: Modern perspectives on aging and chronic disease. Int J Mol Sci 20: , E1380. |
[20] | Oddo S , Caccamo A , Shepherd JD , Murphy MP , Golde TE , Kayed R , Metherate R , Mattson MP , Akbari Y , LaFerla FM ((2003) ) Triple-transgenic model of Alzheimer’s disease with plaques and tangles: Intracellular Abeta and synaptic dysfunction. Neuron 39: , 409–421. |
[21] | Hakim MA , Behringer EJ ((2019) ) Simultaneous measurements of intracellular calcium and membrane potential in freshly isolated and intact mouse cerebral endothelium. J Vis Exp 143: , 10.3791/58832. |
[22] | Hakim MA , Buchholz JN , Behringer EJ ((2018) ) Electrical dynamics of isolated cerebral and skeletal muscle endothelial tubes: Differential roles of G-protein-coupled receptors and K+ channels. Pharmacol Res Perspect 6: , e00391. |
[23] | Boulay AC , Saubamea B , Decleves X , Cohen-Salmon M (2015) Purification of mouse brain vessels. J Vis Exp, e53208. |
[24] | Jackson WF ((2005) ) Potassium channels in the peripheral microcirculation. Microcirculation 12: , 113–127. |
[25] | Zidovetzki R , Levitan I ((2007) ) Use of cyclodextrins to manipulate plasma membrane cholesterol content: Evidence, misconceptions and control strategies. Biochim Biophys Acta 1768: , 1311–1324. |
[26] | Baumer Y , McCurdy S , Weatherby TM , Mehta NN , Halbherr S , Halbherr P , Yamazaki N , Boisvert WA ((2017) ) Hyperlipidemia-induced cholesterol crystal production by endothelial cells promotes atherogenesis. Nat Commun 8: , 1129. |
[27] | Rae EA , Brown RE ((2015) ) The problem of genotype and sex differences in life expectancy in transgenic AD mice. Neurosci Biobehav Rev 57: , 238–251. |
[28] | Kane AE , Shin S , Wong AA , Fertan E , Faustova NS , Howlett SE , Brown RE ((2018) ) Sex differences in healthspan predict lifespan in the 3xTg-AD mouse model of Alzheimer’s disease. Front Aging Neurosci 10: , 172. |
[29] | Felix-Redondo FJ , Grau M , Fernandez-Berges D ((2013) ) Cholesterol and cardiovascular disease in the elderly. Facts and gaps. Aging Dis 4: , 154–169. |
[30] | Pappolla MA , Bryant-Thomas TK , Herbert D , Pacheco J , Fabra Garcia M , Manjon M , Girones X , Henry TL , Matsubara E , Zambon D , Wolozin B , Sano M , Cruz-Sanchez FF , Thal LJ , Petanceska SS , Refolo LM ((2003) ) Mild hypercholesterolemia is an early risk factor for the development of Alzheimer amyloid pathology. Neurology 61: , 199–205. |
[31] | Suri S , Mackay CE , Kelly ME , Germuska M , Tunbridge EM , Frisoni GB , Matthews PM , Ebmeier KP , Bulte DP , Filippini N ((2015) ) Reduced cerebrovascular reactivity in young adults carrying the APOE epsilon4 allele. Alzheimers Dement 11: , 648–657 e641. |
[32] | Reversi A , Rimoldi V , Brambillasca S , Chini B ((2006) ) Effects of cholesterol manipulation on the signaling of the human oxytocin receptor. Am J Physiol Regul Integr Comp Physiol 291: , R861–869. |
[33] | Marrelli SP , Eckmann MS , Hunte MS ((2003) ) Role of endothelial intermediate conductance KCa channels in cerebral EDHF-mediated dilations. Am J Physiol Heart Circ Physiol 285: , H1590–1599. |
[34] | Hannah RM , Dunn KM , Bonev AD , Nelson MT ((2011) ) Endothelial SKCa and IKCa channels regulate brain parenchymal arteriolar diameter and cortical cerebral blood flow. J Cereb Blood Flow Metab 31: , 1175–1186. |
[35] | Kisler K , Nelson AR , Montagne A , Zlokovic BV ((2017) ) Cerebral blood flow regulation and neurovascular dysfunction in Alzheimer disease. Nat Rev Neurosci 18: , 419–434. |
[36] | Chen BR , Kozberg MG , Bouchard MB , Shaik MA , Hillman EM ((2014) ) A critical role for the vascular endothelium in functional neurovascular coupling in the brain. J Am Heart Assoc 3: , e000787. |
[37] | Chow BW , Nunez V , Kaplan L , Granger AJ , Bistrong K , Zucker HL , Kumar P , Sabatini BL , Gu C ((2020) ) Caveolae in CNS arterioles mediate neurovascular coupling. Nature 579: , 106–110. |
[38] | De Silva TM , Modrick ML , Dabertrand F , Faraci FM ((2018) ) Changes in cerebral arteries and parenchymal arterioles with aging: Role of Rho kinase 2 and impact of genetic background. Hypertension 71: , 921–927. |
[39] | Zhang L , Papadopoulos P , Hamel E ((2013) ) Endothelial TRPV4 channels mediate dilation of cerebral arteries: Impairment and recovery in cerebrovascular pathologies related to Alzheimer’s disease.. Br J Pharmacol 170: , 661–670. |
[40] | Park L , Wang G , Moore J , Girouard H , Zhou P , Anrather J , Iadecola C ((2014) ) The key role of transient receptor potential melastatin-2 channels in amyloid-beta-induced neurovascular dysfunction. Nat Commun 5: , 5318. |
[41] | Thakore P , Alvarado MG , Ali S , Mughal A , Pires PW , Yamasaki E , Pritchard HA , Isakson BE , Tran CHT , Earley S ((2021) ) Brain endothelial cell TRPA1 channels initiate neurovascular coupling. Elife 10: , e63040. |
[42] | Chi X , Sutton ET , Thomas T , Price JM ((1999) ) The protective effect of K+ channel openers on beta-amyloid induced cerebrovascular endothelial dysfunction. Neurol Res 21: , 345–351. |
[43] | Fancher IS , Le Master E , Ahn SJ , Adamos C , Lee JC , Berdyshev E , Dull RO , Phillips SA , Levitan I ((2020) ) Impairment of flow-sensitive inwardly rectifying K+ channels via disruption of glycocalyx mediates obesity-induced endothelial dysfunction. Arterioscler Thromb Vasc Biol 40: , e240–e255. |
[44] | Kruth HS ((2001) ) Lipoprotein cholesterol and atherosclerosis. Curr Mol Med 1: , 633–653. |
[45] | Levitan I ((2009) ) Cholesterol and Kir channels. IUBMB Life 61: , 781–790. |
[46] | Steinberg D ((2002) ) Atherogenesis in perspective: Hypercholesterolemia and inflammation as partners in crime. Nat Med 8: , 1211–1217. |
[47] | Diaz M , Fabelo N , Ferrer I , Marin R ((2018) ) “Lipid raft aging” in the human frontal cortex during nonpathological aging: Gender influences and potential implications in Alzheimer’s disease. Neurobiol Aging 67: , 42–52. |
[48] | Hashimoto M , Hossain S , Masumura S ((1999) ) Effect of aging on plasma membrane fluidity of rat aortic endothelial cells. Exp Gerontol 34: , 687–698. |
[49] | Levitan I , Fang Y , Rosenhouse-Dantsker A , Romanenko V ((2010) ) Cholesterol and ion channels. Subcell Biochem 51: , 509–549. |
[50] | Ledoux J , Werner ME , Brayden JE , Nelson MT ((2006) ) Calcium-activated potassium channels and the regulation of vascular tone. Physiology (Bethesda) 21: , 69–78. |
[51] | Romanenko VG , Roser KS , Melvin JE , Begenisich T ((2009) ) The role of cell cholesterol and the cytoskeleton in the interaction between IK1 and maxi-K channels. Am J Physiol Cell Physiol 296: , C878–888. |
[52] | Bukiya AN , Leo MD , Jaggar JH , Dopico AM ((2021) ) Cholesterol activates BK channels by increasing KCNMB1 protein levels in the plasmalemma. J Biol Chem 296: , 100381. |
[53] | Hearon CM Jr. , Richards JC , Racine ML , Luckasen GJ , Larson DG , Dinenno FA ((2019) ) Amplification of endothelium-dependent vasodilatation in contracting human skeletal muscle: Role of KIR channels. J Physiol 597: , 1321–1335. |
[54] | Jackson WF ((2017) ) Boosting the signal: Endothelial inward rectifier K+ channels. Microcirculation 24: , 10.1111/micc.12319. |
[55] | Sonkusare SK , Dalsgaard T , Bonev AD , Nelson MT ((2016) ) Inward rectifier potassium (Kir2.1) channels as end-stage boosters of endothelium-dependent vasodilators. J Physiol 594: , 3271–3285. |
[56] | Epshtein Y , Chopra AP , Rosenhouse-Dantsker A , Kowalsky GB , Logothetis DE , Levitan I ((2009) ) Identification of a C-terminus domain critical for the sensitivity of Kir2.1 to cholesterol. Proc Natl Acad Sci U S A 106: , 8055–8060. |
[57] | Steinberg HO , Bayazeed B , Hook G , Johnson A , Cronin J , Baron AD ((1997) ) Endothelial dysfunction is associated with cholesterol levels in the high normal range in humans. Circulation 96: , 3287–3293. |
[58] | Harraz OF , Longden TA , Dabertrand F , Hill-Eubanks D , Nelson MT ((2018) ) Endothelial GqPCR activity controls capillary electrical signaling and brain blood flow through PIP2 depletion. Proc Natl Acad Sci U S A 115: , E3569–E3577. |
[59] | Harraz OF , Longden TA , Hill-Eubanks D , Nelson MT ((2018) ) PIP2 depletion promotes TRPV4 channel activity in mouse brain capillary endothelial cells. Elife 7: , e38689. |