Stimulation Mapping of Myelinated Tracts in Awake Patients
Abstract
For a long time, although the functional anatomy of human cortex has extensively been studied, subcortical white matter tracts have received little consideration. Recent advances in tractography have opened the door to a non-invasive investigation of the subcortical fibers in vivo. However, this method cannot study directly the function of the bundles. Interestingly, for the first time in the history of cognitive neurosciences, direct axonal electrostimulation (DES) mapping of the neural pathways offers the unique opportunity to investigate the function of the connectomal anatomy. Indeed, this technique is able to perform real-time anatomo-functional correlations in awake patients who undergo brain surgery, especially at the level of the subcortical fibers. Here, the aim is to review original data issued from DES of myelinated tracts in adults, with regard to the functional connectivity mediating the sensorimotor, visuo-spatial, language, cognitive and emotional functions, as well as the interactions between these different sub-networks, leading ultimately to explore consciousness. Therefore, axonal stimulation is a valuable tool in the field of connectomics, that is, the map of neural connections, in order to switch from the traditional localizationist view of brain processing to a networking model in which cerebral functions are underpinned by the dynamic interactions of large-scale distributed and parallel sub-circuits. Such connectomal account should integrate the anatomic constraint represented by the subcortical fascicles. Indeed, post-lesional neuroplasticity is possible only on the condition that the white matter fibers are preserved, to allow communication and temporal synchronization among delocalized inter-connected networks.
INTRODUCTION
For more than one century, the neural foundations sustaining cognitive functions was conceived in a localizationist and rigid framework, in which the brain was thought to be organized in highly specialized critical zones (e.g., Broca’s and Wernicke’s areas [1, 2]) for which any injury induces severe and permanent functional deficit, and in “non-eloquent” regions – i.e. areas not generating persistent neurological worsening after injury. This principle of a fixed functional organization of the central nervous system led to dogmatically impose simplistic and static models of cognitive neurosciences. In this historical context, the subcortical connectivity has received little consideration, especially in humans. Indeed, in spite of regular reports on the structural anatomy of white matter tracts in monkeys, made possible owing to the use of anterograde and retrograde tracers [3], very few studies of anatomic dissection on cadavers have been performed in humans [4–6].
In the new era of connectomics (i.e. the map of neural connections), an alternative hodotopical account was proposed, in which brain functions are underpinned by the dynamic interactions of large-scale delocalized and parallel (while partially overlapped) sub-networks [7–9]. Recent advances diffusion tensor imaging (DTI), enabling a non-invasive tractography of white matter tracts in vivo, brought new insights into the subcortical pathways [10]. Nonetheless, DTI has many methodological limitations, should still benefit from a rigorous anatomical validation, and is not able to provide functional data: this is only an indirect reflect of the structural connectivity [11–14]. As a consequence, Klingler’s method has received renewed interest since DTI reconstruction can be correlated to actual anatomic evidence provided by such postmortem dissection of white matter bundles [15–17]. It is nonetheless worth noting that the traditional fiber dissection technique consists of extensive resection of surrounding cerebral tissue and hampers analysis of cortical terminations. Recently, an improvement of Klinger’s method was proposed, in which removal of cerebral tissue was kept to a minimum with the goal to preserve the cortex and relationships within the brain until the end of the dissection [17, 18]. Using this cortex-sparing fiber dissection, the trajectory and the orientation of white matter fibers, as well as their cortical terminations, can now be identified reliably and reproducibly, including in humans [16, 19].
In addition to structural considerations, the functional role of subcortical myelinated tracts can be investigated by means of intraoperative electrical mapping performed in awake patients undergoing surgery for brain tumor or epilepsy. Indeed, direct electrical stimulation (DES) of the brain offers a unique opportunity to study functional anatomy, in particular functional connectivity. In practice, it has become common clinical routine to awaken patients in order to assess the functional role of restricted cortical and subcortical regions, and to avoid neurological worsening. The surgeon can optimize the extent of resection, and thereby improve the oncological or epileptological outcomes, without causing permanent neurological deficits, owing to an individual mapping and preservation of critical structures. This means that the resection is achieved according to functional boundaries of each patient [20]. Patients perform several sensori-motor, visuospatial, language, cognitive or emotional tasks while the surgeon temporarily disrupts discrete cerebral structures using DES [21]. Thus, this biphasic electrical current (60 Hz, 1 msec, 1 to 4 mA) is mimicking a genuine “virtual transient lesion”, not only at the level of cortex, but also at the axonal level when the electrode is applied directly in contact with the white matter fascicles. If the patients stops moving, speaking or produces wrong response, the surgeon avoid removing the stimulated site [22].
The principle of DES is to interact locally with a small cortical or axonal site, but also non-locally, as the focal perturbation disrupts the whole (sub)network sustaining a given function. However, it has been shown that as long as the repetitive stimulation is <200 Hz, γ-aminobutyric acid-related inhibition prevents the stimulus from propagating beyond the first synapse [23]. Thus, conversely to functional neuroimaging, DES is able to identify the cortico-subcortical structures essential for brain functions by inhibiting a part of a sub-circuit during a few seconds – with the possibility to check whether the same functional disorders are reproduced when repeated stimulations are applied over the same area [24]. Interestingly, by gathering all cortical and axonal sites where the same type of errors were observed when stimulated, one can build up the sub-network of the disrupted sub-function. DES identifies with a great accuracy (about 5 mm) and reproducibility, in vivo in humans, the structures – not only cortex but also white matter tracts - that are crucial for cognitive functions [25]. Thus, this is currently the only way to investigate directly the functional role of the subcortical fascicles [26]. Indeed, combination of neurological disturbances elicited by intrasurgical DES with the anatomical data provided by post-operative MRI has enabled to perform reliable anatomo-functional correlations, at the level of both gray and white matters. Such correlations have allowed analysis of the anatomical location of the critical areas detected by DES, i.e. in essence, at the periphery of the cavity, where the resection was stopped according to functional limits – a methodology that has been extensively used and validated [27–32]. In other words, for the first time in the history of cognitive neurosciences, DES mapping of the neural pathways offers the unique opportunity to investigate the function of the connectomal anatomy, supporting a network organization of the brain, and resulting in the reappraisal of cognitive models[33].
In this article, the aim is to review original data issued from DES of myelinated tracts in adults, with regard to the functional connectivity mediating the sensorimotor, visuo-spatial, language, cognitive and emotional functions, as well as the interactions between these different sub-networks, leading ultimately to explore consciousness. Furthermore, the limiting role of axonal fibers in subcortical interindividual variability and neuroplasticity is discussed.
METHODOLOGY OF DIRECT BRAIN ELECTROSTIMULATION
Principles of stimulation
The membrane potential (MP) of the neuron at rest varies between –60 and –100 mV, because of an asymmetrical distribution of charges, which is maintained by active mechanisms and ion diffusion through lipid layers: negative charges predominate at the inner side of the membrane and positive charges at the outer side. The principle of electrical stimulations is to increase membrane excitability, via an initial phase of passive modification of the local MP in front of the cathode (the negative stimulating electrode), making the inner side of the membrane progressively less negative than the outer side, that is membrane depolarization. Conversely, the membrane becomes hyperpolarized in front of the anode. The intensity of this phenomenon depends both on stimulation parameters and membrane characteristics [34]. Indeed, membrane excitability if higher at the initial segment of the axon, and for larger and myelinated fibers [35]. As soon as the MP reaches the liminar depolarization threshold, a second phase starts with the opening of voltage-dependent ionic canals, which allow entry of Na+ ions and inverts the MP between +20 and +30 mV. A secondary output of K+ ions, associated with an inhibition of the flux of entering Na+ions, brings the MP back to its resting state. This corresponds to the “action potential”, which corresponds to an all-or-nothing phenomenon, that is, once generated, it has always the same characteristics, irrespective of the stimulation parameters.
Stimulation parameters: theoretical approach and the effect of myelination of fibers
An electrical stimulation is defined by its intensity (mA) and its impulse duration (ms). The product of both parameters gives the charge – represented in coulombs (C), or in C/phase in case of a biphasic current with alternating polarity. Charge density (or charge per unit of stimulated surface area) is expressed in C/cm2/phase. The product [intensity×resistance (or impedance, expressed in ohms)] gives the voltage (V). The product (charge×voltage) gives the energy, expressed in Joules (J)/phase. First and above all, these stimulations must be entirely safe for the cerebral parenchyma. Indeed, these stimulations could induce a lesion by accumulation of negative charge at the level of the cathode or through production of metal ions at the level of the anode [36]. This risk has been cancelled thanks to the use of biphasic impulses, since the second stimulus phase inverses the effects of the first. Tissue damage can also be caused, on the one hand, by excessive heat, which is produced specifically by hydrolysis that could cause vacuolization and chromatolysis; and on the other hand, by “leaking” of the intracellular current, which goes from the anode to the cathode through the cytoplasm, with a risk of lesion to the mitochondria and endoplasmic reticulum or even by alteration in homeostasis, when the neurons are activated in a manner that is too repetitive and synchronous [37]. These risks are directly linked to charge density, and studies based on animal experimentation allowed an upper safety limit to be set at 55 microcoulombs/cm2/phase [38]. Stimulations may also generate seizures. To prevent this complication, it is recommended to avoid the use of intensity above 4 mA, at least for myelinated fibers in adults [39]. However, the nonmyelination of fibers in young children may sometimes necessitate to increase the intensity of stimulation beyond this threshold, in order to induce functional response [40].
The second requirement for these stimulations is that they set off a reproducible response when they are applied to neural structures. The relationship between stimulation parameters and tissue response characteristics can be summed up by the intensity/duration curve, in which the current intensity is recorded as a function of impulse duration [40]. The rheobasis is thus defined as the minimal intensity that is required to generate an action potential for high impulse durations. The chronaxis is the impulse duration that is required to induce a response when the stimulus intensity is double the rheobasis. The chronaxis corresponds to the point on the intensity/duration curve where an action potential can be obtained with minimal energy: this is the optimal stimulation point (best benefit: risk ratio). The chronaxis depends on the characteristics of the tissue being stimulated,particularly its impedance. The infrequent studies that were made in this area [41] produced resistance values of 250 Ω for gray matter, 500 Ω for white matter and 65 Ω for cerebrospinal fluid. Moreover, chronaxis is significantly modified by the degree of cerebral maturation, notably by myelination: chronaxis is considerably longer (0.4–3.5 ms) for nonmyelined nerve fibers than myelined axons (0.05–0.4 ms) [40]. Fiber diameter seems also to be a factor, as axons of greater diameter are more easily excitable [35]. Finally, any pathological process, whether lesional (tumor) or nonlesional (epilepsy, postictal status), can interfere directly with tissue’s excitability [34]. Of note, based upon these electrophysiological properties, a safe and effective paradigm to functionally map the cortex using electrical stimulation has been specifically elaborated in childhood, because of nonmyelination of fibers [40].
As regards the frequency of electrical impulses, myelinated axons produce a single response for each stimulus delivered between 50 and 100 Hz [34]. Thus, in clinical practice, DES usually deliver a 60 Hz current to achieve both cortex and white matter tracts [42]. Actually, the absolute refractory period of the neuronal membrane covers a period of 0.6 to 2 ms, following the action potential. It is followed by a second phase of transient hyperexcitability, where the tissue can be stimulated by less intense currents than for the preceding stimulus, which implies a greater risk of seizure. Moreover, when neural structures are kept in a state of subthreshold depolarization, there is an increase of the MP threshold that must be reached in order to set off the impulse: this phenomenon is known as “accommodation”. Accommodation occurs only if the MP changes very progressively for example with sinusoïdal impulses. This is the reason why rectangular impulses are recommendedfor stimulation.
Although two electrodes are always required to produce a current, the stimulations are considered to be “monopolar” if only one of the electrodes (in general, the cathode) is “active”, that is, localized close to the target tissue, whereas the reference electrode (in general, the anode) is located at some distance. Even if the current density is distributed in a relatively uniform manner around the electrode, each tissue located in the current’s pathway can nevertheless be stimulated, most specifically if its depolarization threshold is lower than that of the target. In order to reduce this risk of a false positive, it is preferable to use a bipolar stimulation. In this case, both the cathode and the anode are “active”, that is, they are located close to the target tissue [43]. In this way, only these structures that are located between both electrodes are stimulated, with a lesser risk of current diffusion and therefore, a greater precision, as was demonstrated using the optical imaging method [44]. Therefore, when stimulating white matter tracts, bipolar probe is very accurate for detecting a specific sub-pathway involved in a given sub-function [26,45].
DES and cognitive neurosciences: an input gate into the eloquent neural networks
One of the major advantages of DES for brain mapping in adult patients is that they intrinsically do not cause any false negatives - if nevertheless the methodology is rigorously applied. Indeed, each eloquent structure, whatever its actual role in brain function, will be in essence electrically disturbed by DES, which necessarily will induce a functional consequence. This optimal sensitivity explains why DES is currently considered the gold standard in brain mapping. Indeed, this is the most important point for neurosurgeons, since intraoperative DES enables them to tailor the resection according to functional boundaries - thus minimizing the risk of permanent deficit [46] while optimizing the extent of resection. Moreover, this advantage also explains why DES is used to validate the non-invasive method of neurofunctional imaging (fMRI, transcranial magnetic stimulation and magnetoencephalography) [47–50] as well as DTI [51].
In addition, when stimulating the neuron (whatever the stimulated part), the action potential propragates by physiological conduction along the axon, and consequently, neurons at the end of the axons can be stimulated by these presynaptic currents. Similarly, when stimulating axonally, the action potential propagates both forward (in a physiological way) and backward (in a non-physiological way). So, in essence, DESs are highly non-local: they enter the whole network that sustains a function. The stimulated point (axonal or cortical) is only an input gate into this whole network. In other words, one should forget about localizationist models as well as a connectionist approach and shift toward a nonlocal theory when analyzing how DES works. It is worth noting that the perturbation induced by DES in a functional network is small enough that it propagates only in a “sub-circuit,” thus inhibiting solely a specific component of the tested function. This is why for cortical mapping of language function, depending on which “sub-circuit” is disturbed, one may observe phonological errors, speech apraxia, semantic paraphasias, anomia or syntactic mistakes. Of course, when increasing the stimulation intensity, one ultimately generates a speech arrest [24, 52]. Similarly, when stimulating axonally, one generates a dysfunction of a specific network. While in a connectionist point of view this dysfunction is mediated by a mere transient disconnection between two still effective areas (i.e., only the link is disturbed, corresponding to a connectionist point of view), the non-local theory assumes that one or both of the areas linked by the stimulated pathway will be disturbed. Anyway, the resulting effect mimics disconnection syndrome, as observed in lesions of white matter[26].
In summary, since DES allows the performance of on-line anatomofunctional correlations at both the cortical and axonal levels, and since each eloquent discrete site identified is only an input gate to a wider functional network, DES is consequently a perfect tool for the study of spatio-temporal brain connectivity, in vivo in humans.
Limitations of DES
Although DES opens up an array of opportunities, it also has some intrinsic limitations.
First, that the patients under investigation do not have an intact brain and, in the case of low-grade glioma, could exhibit reorganization of function before surgery. However, because subcortical plasticity is low [103], DES still has considerable value for investigating brain processing, especially in white matter tracts, even in patients with cerebral lesions. Indeed, if plasticity enabled compensation for all areas involved by the tumor, it would not be possible to identify crucial neural structures in a probabilistic map based on patients with glioma, as has been accomplished by Sarubbo et al. [105]. The ability to detect critical cortical as well as axonal structures with a high rate of probability by inducing a reproducible deficit during intraoperative DES - such as anarthria during stimulation of the SLF III [31], or semantic disturbances during stimulation of the IFOF [98] - despite cerebral reshaping, has considerable implications for understanding the normal functional anatomy of the brain. The connectivity of the brain is particularly amenable to this approach, owing to its low potential for reshaping [102].
Second, DES is only able to identify the cortico-subcortical structures essential for brain functions. Therefore, if DES does not provoke any transient deficit, this does not mean that the tested structure is not involved in the function, but this means that this structure can be compensated if removed. This has been supported by a recent prospective cohort with 374 patients who underwent awake surgery with resection of a brain tumor according to functional boundaries provided by intraoperative DES: three months after surgery, no patients had severe or disabling permanent worsening [39].
Finally, the limited time available during surgery does not permit the range of assessments and stimuli that are possible in standard neuropsychological evaluations. However, as mentioned above, the excellent functional outcomes following surgery plead in favor of a high reliability of the tests used intraoperatively, because they are adapted to the quality of life of the patient - depending not only on the location of the tumor, but also on the job and hobbies of the patient [88].
DES MAPPING OF THE PROJECTION FIBERS
Connectivity underlying sensorimotor function
Recent anatomo-functional DES studies have changed our view of movement, that is, motor execution and control, away from a hierarchical organization and toward parallel and interconnected circuits. First, DES have confirmed that the corticospinal tracts come from the primary motor cortex, run with a somatotopical organization within the corona radiata (with, from lateral to medial, the pyramidal tracts of the face, upper limb, and lower limb) and then within the posterior limb of the internal capsule (with, from anterior to posterior, the pyramidal tracts of the face, upper limb, and lower limb) before reaching the brainstem and the spinal cord [53, 54]. Furthermore, the existence of an additional “modulatory motor network” was recently evidenced, eliciting movement arrest or acceleration when stimulated in awake patients, with no loss of consciousness [30]. The subcortical stimulation sites were distributed veil-like, anterior to the primary motor fibers, suggesting descending pathways originating from premotor areas known for negative motor response characteristics, and running to the head of the caudate nucleus: it seems that this motor control circuit is mediated by the fronto-striatal tract [31]. Furthermore, these white matter bundles underpinning movement control were somatotopically distributed. Indeed, stimulation of the fibers from mesial to lateral directions and from posterior to anterior directions evoked arrest of movement of the lower limb (mesially and posteriorly), upper limb(s), and face/speech (laterally and anteriorly) [55]. Further stimulation sites in the anterior arm of the internal capsule indicated a large-scale motor control circuit [30]. More recently, the first evidence of bilateral negative motor responses elicited by unilateral subcortical DES has been reported. Such findings support the existence of a bilateral cortico-subcortical network connecting the premotor cortices, basal ganglia, and spinal cord, involved in the control of bimanual coordination [56].
Posterior thalamo-cortical somatosensory pathways and their somatotopy have also been investigated by DES, which generate dysesthesias or tingling in awake patients [53, 57]. Interestingly, axonal stimulation of the white matter under the retrocentral gyrus may also induce disturbances in movement control [58], possibly due to transient inhibition of U fibers within the rolandic region [59]. Moreover, in patients who experienced interference with movement during subcortical DES, fibers that induced inhibition or acceleration were located immediately posterior to thalamo-cortical somatosensory pathways. Therefore, a thalamo-parietal connection distinct from somatosensory pathways is likely [30, 58]. On the basis of these original DES findings, the existence of a wide fronto-thalamo-parietal sensory-motor network has been suggested [30, 58].
Visual tract
The optic radiations arise from the lateral geniculate body in three bundles. The anterior bundle curves anterolaterally above the temporal horn (Meyer’s loop) usually reaching beyond the anterior limit of the temporal horn and then loops backward along the inferolateral wall of the atrium. The middle bundle courses laterally around and turns posteriorly along the lateral wall of the atrium and the occipital horn. The posterior bundle courses directly backward, also along the lateral wall of the atrium and occipital horn. Recently, visual pathways have also been mapped in awake patients. A protocol was recently proposed in which two images, located in opposite quadrants on the same computer screen, are shown to the patient. It is thus possible to generate a transient visual field deficit subjectively described by the patient, which is confirmed objectively with this test (only one of the two objects can be seen and, therefore, described) during axonal DES of the optic radiations [60].
Interestingly, DES can generate either “negative effect” such as blurred vision or impression of shadow, or “positive effect” such as phosphenes in the contralateral visual field. In addition, complex responses such as visual hallucinations (e.g., zoopsia or distortion of pictures as metamorphopsia) have been described by patients during stimulation [61]. These findings might be explained by the fact that DES of bundles joining the calcarine fissure evokes visual suppression (homonymous hemianopia), whereas DES of fibres joining the association visual cortex for higher-order visual processing (outside the primary visual system) evokes visual illusion.
DES MAPPING OF THE ASSOCIATION FASCICLES
Tracts mediating language (Fig. 1A)
On the basis of DES findings, a dual model for visual language processing has recently been proposed, with a ventral stream involved in mapping visual information to meaning (the “what” pathway) and a dorsal stream dedicated to mapping visual information to articulation through visuo–phonological conversion [27]. These findings complete the seminal model by Hickok and Poeppel [62], that is, a pure cognitive model that did not take into account anatomical constraints, especially with regard to the white matter tracts.
Functional anatomy of the dorsal Superior longitudinal Fascicle (SLF)/Arcuate Fascicle (AF) complex
Fiber dissection in cadavers and DTI tractography studies in humans have investigated the structural anatomy of the SLF/AF complex [10, 19]. The different components of the perisylvian SLF were isolated and the tracts were followed until their cortical terminations. Three segments of the perisylvian SLF were detected: (i) anterior segment of the lateral SLF, that connects the supramarginal gyrus and superior temporal gyrus (in the region just posterior to the Heschl’s gyrus) with the ventral portion of the precentral gyrus (ventral premotor cortex), (ii) posterior segment of the lateral SLF, that connects the posterior portion of the middle temporal gyrus with the angular gyrus, and (iii) long segment of the AF, deeply located, stemming from the caudal part of the temporal lobe, mainly the inferior and middle temporal gyri, that arches around the insula and advances forward to end within the frontal lobe, essentially within the precentral gyrus and posterior portion of the inferior and middle frontal gyri. Based on these original data challenging the traditional view, it was suggested that the fibers from the posterior part of the superior temporal gyrus are part of the anterior portion of the perisylvian SLF and not of the AF[19].
In awake patients performing a picture-naming task, cortically, phonemic paraphasia have been generated by DES of the inferior parietal lobule and inferior frontal gyrus in the dominant hemisphere [63]. Axonally speaking, these phonemic paraphasias were evoked when stimulating the AF [19, 64], possibly associated with repetition disorders [65]. This is in agreement with the theory by Geschwind [66], who postulated that damages of this bundle would produce conduction aphasia, including phonemic paraphasia and repetition disturbances, and this supports the role of the sub-part of the dorsal stream mediated by the AF in phonological processing. Interestingly, the posterior cortical origin of the AF within the posterior part of the inferior temporal gyrus corresponds to the visual object form area [19]. Indeed, this region represents a functional hub, involved both in semantic and phonological processing dedicated to visual material [67, 68]. Therefore, phonological processing sustained by the AF is performed in parallel to the semantic processes implemented by the ventral route (see below). In addition to this direct dorsal route, the indirect dorsal stream composed of the lateral SLF is involved in articulation and phonological working memory, as demonstrated by DES. Cortical areas eliciting articulatory disorders are located in the ventral premotor cortex, supramarginal gyrus and posterior part of the superior temporal gyrus [69]. Axonally, stimulation of the white matter under the fronto-parietal operculum and supramarginal gyrus, laterally and ventrally to the AF, elicited anarthria as well [31]. This bundle corresponds to the part III of the SLF according to Makris et al. [70]. Indeed, this lateral operculo-opercular component of the SLF constitutes the articulatory loop, by connecting the supramarginal gyrus/posterior portion of the superior temporal gyrus (which receives feedback information from somatosensory and auditory areas) with the frontal operculum (which receives afferences bringing the phonological/phonetic information to be translated into articulatory motor programs and efferences toward the primary motor area)[31, 33].
Using the same paradigm, DES also supported that syntactic processing was mediated by delocalized cortical regions (left inferior frontal gyrus and posterior middle temporal gyrus) connected by a sub-part of the left SLF. Interestingly, this sub-circuit is interacting but independent of the sub-network involved in naming, as demonstrated by a double dissociation between syntactic (especially grammatical gender) and naming processing during DES. These findings support a parallel rather than serial theory, calling into question the principle of “lemma” [71].
Functional anatomy of the ventral route
The ventral stream connects the occipital and posterior temporal areas with the frontal lobe. This ventral route is referred by some authors as “extreme capsule fiber system” with reference to connectivity studies in the primate [72, 73]. It seems nonetheless more adapted to speak about fascicles rather than “extreme capsule”, because the latter only considers a discrete anatomical structure while the former considers actual neural pathways with their cortical termination, in a connectomal view of brain processing. Indeed, if one takes account of the sole subcortical region without any considerations regarding the cortical epicenters connected by these white matter fibers, this does not allow the understanding of the whole eloquent network.
Regarding structural anatomy, the ventral route is composed of direct and indirect pathways. The direct pathway is represented by the inferior fronto-occipital fascicle (IFOF), that has never been described in animals, explaining the controversy about its role [74]. In humans, the IFOF is a ventral long-distance association bundle that connects the occipital lobe, parietal lobe and the postero-temporal cortex with the frontal lobe. Recent anatomic dissections [18] combined with DTI have investigated the main course of the IFOF [16]. From the posterior cortex, it runs within the sagittal stratum in the superior and lateral part of the atrium; it reaches the roof of the sphenoidal horn in the temporal lobe; it joins the ventral part of the external/extreme capsule and it runs under the insula at the posterior two-thirds of the temporal stem; then it joins the frontal lobe [18]. Two layers of the IFOF have been described [16]. The superficial and dorsal layer connects the posterior portion of the superior and middle occipital gyri, the superior parietal lobule and the posterior part of the superior temporal gyrus to the inferior frontal gyrus (pars triangularis and opercularis). The deep and ventral subcomponent connects the posterior portion of the inferior occipital gyrus, the posterior temporal-basal area including the Fusa (fusiform area at the occipito-temporal junction) and the posterior part of the middle temporal gyrus to the frontal lobe - orbito-frontal cortex, middle frontal gyrus and dorsolateral prefrontal cortex.
In parallel, the ventral stream is underpinned by an indirect pathway, constituted by the anterior part of the inferior longitudinal fascicle (ILF) (running below the IFOF), that links the posterior occipitotemporal region (Fusa) and the temporal pole (TP), then relayed by the uncinate fasciculus (UF), that connects the TP to the basifrontal areas by running within the anterior third of the temporal stem (in front of the IFOF) [75]. Of note, the posterior part of the ILF links the occipital lobe to the posterior occipitotemporal junction (visual object form area) [76]. This means that this indirect route connects the occipital/Fusa to the orbito-frontal cortex - thus partially overlapped with the IFOF. Finally, while previously described in monkey, another pathway has recently been observed in humans: the middle longitudinal fascicle (MdLF) [77]. It connects the angular gyrus with the superior temporal gyrus up to the TP and courses under the superior temporal sulcus, lateral and superior to the IFOF [78, 79].
In the awake patient, during picture naming, DES of the IFOF, at least in the dominant hemisphere, elicited semantic paraphasias either associative (e.g. /key/ for /padlock/) or coordinate (e.g. /tiger/ for /lion/) in more than 85% of cases. It did not matter what portion of the IFOF was stimulated (parieto-occipital junction, temporal, subinsular or frontal part) [27]. These language disorders were mainly induced by stimulating the superficial layer of the IFOF. Interestingly, semantic paraphasias were never observed during stimulation of the dorsal route (SLF) [63]. In addition, IFOF stimulation may also generate verbal perseveration [80].
The functional role of the indirect ventral pathway is still debated. On one hand, this indirect route connects areas involved in verbal semantic processing such as Fusa and lateral frontal cortex [67]. Moreover, the major cortical relay between the ILF and UF is the TP, which is a “hub”, i.e. a functional epicenter allowing a plurimodal integration of the multiple data coming from the unimodal systems (subserved by ILF, UF and MdLF) – explaining its role in semantics and its implication in semantic dementia when bilaterally damaged [81]. On the other hand, except for the posterior part of the ILF for which injury generates alexia [68, 76, 82], the indirect pathway can be functionally compensated when unilaterally damaged [75, 83]. This was also confirmed by language recovery following anterior temporal lobectomy in tumor and in epilepsy surgery [84, 85]. Even if very mild and selective deficit may persist, as concerning proper name retrieval after resection of the UF, this is a good illustration of the concept of “subcortical plasticity”, in which a sub-network (IFOF, direct pathway) is able to bypass another sub-network (indirect pathway) and to functionally compensate it [83, 86]. Similarly, DES of MdLF and resection of its anterior part failed to induce any functional disorders [87], demonstrating that this fascicle converging to the TP can also be compensated.
In summary, DES has enabled a re–examination of the classical Broca–Wernicke localizationist model of language. The new findings from axonal stimulation provide a distributed framework for future studies of language networks and for management of patients with aphasia. This new model is based on multiple direct and indirect corticosubcortical interacting subnetworks involved in syntactic, semantic, phonological and articulatory processes. It offers several advantages in comparison with previous models, especially by explaining double dissociations during damage of the ventral versus dorsal stream (semantic and phonemic disorders, respectively). Also, it takes into account the cortical and subcortical anatomical constraints.
Fibers sustaining visuospatial and vestibular processing
In awake patients, axonal DES of the ILF has generated contralateral visual hemiagnosia, supporting the existence of an occipito–temporal pathway connecting occipital visual input to higher–level processing in temporal lobe structures, in particular the fusiform gyrus [88]. These stimulation findings support a crucial role for the ILF in visual recognition, with specialization of this bundle for visuospatial processing in the right hemisphere and language processing in the left hemisphere.
Stimulation of a specific part of the SLF (called the SLF II) in the right hemisphere can produce spatial cognition problems. Indeed, DES of this structure elicited rightward deviation in a line bisection test [28]. These data suggest that parietal–frontal communication is necessary for symmetrical processing of the visual scene. In other words, spatial awareness depends not only on the cortical areas of the temporal–parietal junction, but also on a larger parietal–frontal network communicating via the right SLF.
Finally, stimulation of another subcircuit in the right SLF can cause a central vestibular syndrome with vertigo, by disrupting the vestibular inputs assembled in the temporoparietal areas and the prefrontal cortex [89]. This finding demonstrates the role of the SLF in the network coordinating body posture and spatially oriented
Fibers subserving mentalizing
Regarding the network sustaining mentalizing, namely, the theory of mind, crucial for emotion and social cognition, intraoperative DES combined with pre- and post-operative behavioral examinations showed that this function is made possible by parallel functioning of two subsystems. The first, low-level, of mentalizing accuracy of identification (mirror system, i.e. the ability to appreciate other people’s emotions) is subserved by the AF/SLF complex; the second, high-level of inferential mentalizing, corresponding to the attribution of mental states is mediated by the cingulum [90–92].
These findings, which constitute the first experimental data on the structural connectivity of the mentalizing network, suggest the existence of a dual-stream connectomal model, and could lead to a better understanding of disorders that affect social cognition, as autism [92].
Fibers underlying cognitive functions
DES also evidenced the existence of an executive system (including prefrontal cortex, anterior cingulate and caudate nucleus) involved in the cognitive control of more dedicated subcircuit, as for example the subnetwork involved in language switching - itself constituted by a wide cortico-subcortical network comprising postero-temporal areas, supramarginal and angular gyri, inferior frontal gyrus and a sub-part of the SLF [93]. Moreover, the frontal aslant tract, that connects the pre-supplementary motor area and anterior cingulate with the inferior frontal gyrus [59, 94, 95] might play a role in language control, especially with regard to planning of speech articulation [32]. Therefore, DES of this tract may evoke stuttering [95]. In the same vein, a cortico-subcortical loop involving the deep grey nuclei, especially the caudate nucleus was also demonstrated as participating in the control of language (selection/inhibition), since DES of the head of the caudate nucleus in the left hemisphere generated perseverations with a high level of reliability [97]. Anatomically, this cortico-striatal loop seem to be supported by the fronto-striatal tract [32].
DES of the IFOF also induced non-verbal comprehension disturbances in more than 90% of cases during non-verbal semantic association test - e.g. Pyramid and Palm Trees Test. The patients were not able anymore to make a semantic choice during DES, with some of them still able to join a short verbal description of their feelings, like “I don’t know at all”, “what do I have to do?”, “I don’t understand anything” [98]. These comprehension disorders were mainly generated by stimulating the deep layer of the IFOF, and elicited a double dissociation: semantic paraphasia with normal non-verbal semantic choice during DES of superficial IFOF and vice versa during DES of deep IFOF. Thus, it was suggested that the existence of a superficial component involved in verbal semantics (see above) and a deep component involved in amodal semantic processing.
These data are in agreement with the cortical terminations of the IFOF (prefrontal, temporal-basal and parietal areas), that correspond with the cortical network involved in semantic control [99]. Consequently, from the new insights gained from axonal DES, an original anatomo-functional model of semantic processing has recently been proposed, in which the crucial pathway is represented by the IFOF. In this model, visual information is processed at the level of the occipital and temporal-basal associative cortices, and auditory information is processed at the level of the temporal and parietal associative cortices. They are transmitted directly on an amodal shape to the prefrontal areas, which exert a top down control over this amodal information in order to achieve a successful semantic processing in a given context. DES of this fascicle generates a disruption of these rapid direct connections. The transient semantic disorganization observed when stimulating the IFOF would therefore be caused by a dis-synchronization within this large-scale network, interrupting simultaneously the bottom up transmission and the top down control mechanisms [98]. Thus, IFOF might play a crucial role not only in verbal and non-verbal semantic processing, but also in the awareness of amodal semantic knowledge, namely noetic consciousness. From a phylogenic perspective, because recent studies in the primate failed to identify this tract, one could suggest that the IFOF is the proper human fascicle. This multi-function fascicle allows human to produce and understand language, to manipulate concepts, to apprehend and understand the world (i.e. metalinguistics, conceptualization and awareness of knowledge) and it contributes to make the human what he is, with his infinite wealth of mind.
In the same spirit, axonal DES has shown that disruption of the subcortical connectivity of the left posterior cingulate cortex reliably induced a breakdown in conscious experience [100, 101]. This acute phenomenon was mainly characterized by a transient behavioral unresponsiveness with loss of external connectedness (the patient described himself as in a dream, outside the operating room). This finding suggests that functional integrity of posterior cingulate connectivity is necessary to maintain consciousness of the external environment. In other words, axonal DES can open the door to the investigation of the connectomal anatomy underlying distinct levels of awareness, including the pathways involved in monitoring of the human level of consciousness related to semantic memory (noetic consciousness), as well as the pathways that sustain consciousness of the external world. Axonal DES can also be used to explore the dynamic interactions between these subcircuits, and possible functional regulation of the intrinsic activity of these subcircuits by other subnetworks, such as the cingulothalamic system [101].
To sum up, these original insights provided by DES strongly support the fact that cerebral functions are underpinned by extensive circuits comprising both the cortical epicenters (topos, i.e. sites) and connections between these “hubs”, created by associating bundles of white matter (hodos, i.e. pathways) [8]. In this hodopical model challenging the traditional localizationist view, neurological function comes from the synchronization between different epicenters, working in phase during a given task, and explaining why the same hub may take part in several functions depending on the other cortical areas with which it is temporarily connected at any one time. One step forward, it is crucial to consider that complex brain processes are possible only because of dynamic interactions exist between these parallel delocalized sub-networks, with different levels of sub-circuits recruitment according to the task required. Therefore, brain processing should not be conceived as the sum of several subfunctions. Rather, cerebral function results from the integration and potentiation of parallel (while partially overlapped) subnetworks.
The limiting role of MYELINATED TRACTS in NEUROplasticity
Although a huge plastic potential has been demonstrated in humans at the cortical level, subcortical plasticity is low, implying that axonal connectivity should be preserved to allow post-lesional compensation [9]. Indeed, lessons from stoke studies have taught us that a damage of the white matter pathways generates a more severe neurological outcome than lesions of the cortex. Recently, we proposed the elaboration of a probabilistic postsurgical residue atlas computed on a series of patients who underwent incomplete resection for a glioma on the basis of intraoperative DES [102, 103]. We gathered 231 postoperative MRI of patients operated on for a WHO grade II glioma under direct electrical cortico-subcortical stimulation, that is, with a tumor resection performed according to functional boundaries. Postoperative images were registered on the MNI template to construct an atlas of functional resectability for which each voxel represents the probability to observe residual nonresectable glioma, that is, non-compensable area [102, 103]. The anatomo-functional correlations obtained by combining the intrasurgical functional data with postoperative anatomical MRI findings provided new insights into the potentials and limitations of cerebral plasticity. This probabilistic atlas of functional plastic potential highlighted the crucial role of the axonal pathways in the reorganization of the brain after a lesion. It provided a general framework to establish anatomo-functional correlations by computing for each brain voxel its probability to be left – due to its functional role – on the postoperative MRI. Its overlap with the cortical MNI template and a DTI atlas offered a unique tool to analyze the potentialities and the limitations of inter-individual variability and plasticity, both for cortical areas and axonal pathways. We observed low probability of residual tumors on the cortical surface, whereas most of the regions with high probability of residual tumor were located in the deep white matter tracts. Thus, projection and association axonal pathways seem to play a critical role in the proper functioning of the brain. In other words, the functions sub-served by long-range axonal pathways seem to be less subject to inter-individual variability and reorganization than cortical sites [83, 104]. The reproducibility of these results may lead to suggest the existence of a “minimal common brain”, necessary for the basic cognitive functions - even if likely not sufficient for more complex functions such as multi-processing.
Of note, from a clinical point of view, in addition to surgical planning, this atlas may be a unique tool for predicting the likelihood of functional recovery in various neurological diseases. Moreover, this atlas may be critical for identifying patients who require functional rehabilitation following a cerebral damage. In other words, objective neuropsychological assessments after brain damage can be helpful for the elaboration of individualized programs of neurological, cognitive and behavioral rehabilitation, adapted to an improved knowledge of the connectome.
CONCLUSION
For the first time in the history of cognitive neurosciences, DES mapping of the white matter tracts offers a unique opportunity to investigate the function of the connectomal anatomy in humans. This original methodology, in which real-time anatomo-functional correlations are performed in awake patients, has provided new insights into the functional connectivity underlying the sensorimotor, visuospatial language and sociocognitive systems. On the basis of these unique results in the literature, the first functional atlas of human white matter has recently been built [105]. In addition, interactions between these neural networks and multimodal systems, such as working memory, attention, executive functions and even consciousness, can be investigated by axonal stimulation. In this networking model, cerebral function results from the integration and potentiation of parallel, but partially overlapping, subnetworks. In addition, this hodotopical account, supported by axonal DES, offers the possibility to better understand the mechanisms and limits of neuroplasticity within and between delocalized and dynamic large-scale subcircuits, both during development and in adults. Overall, these findings allow a reappraisal of the classic neuropsychological models of conation and cognition, which should integrate the anatomic constraint represented by the subcortical pathways. Therefore, it is time for neuroscientists to improve their knowledge of white matter circuitry underpinning the human brain connectome.
CONFLICT OF INTEREST
The author declare no conflict of interest.
REFERENCES
[1] | Broca P . Nouvelle observation d’aphémie produite par une lésion de la moitié postérieure des deuxième et troisième circonvolutions frontale. Bull Soc Anat. (1861) ;6: :337–393. |
[2] | Wernicke C . Der aphasische symptomen complex. Breslau: Cohn et Weigert, (1874) . |
[3] | Schmahmann JD , Pandya DN . Anatomical investigation of projections from thalamus to posterior parietal cortex in the rhesus monkey: A WGA-HRP and fluorescent tracer study. J Comp Neurol. (1990) ;295: :299–326. |
[4] | Déjerine J . Anatomie des Centres Nerveux. Tome 1. Paris: Rueff et Cie, (1895) . |
[5] | Klingler J . Erleichterung der makroskopischen Praeparation des Gehirns durch den Gefrierprozess. Schweiz Arch Neurol Psychiatr. (1935) ;36: :247–256. |
[6] | Ludwig E , Klinger J . Atlas cerebri humani. Boston, Toronto: Little, Brown, (1956) . |
[7] | Catani M . From hodology to function. Brain. (2007) ;130: :602–605. |
[8] | de Benedictis A , Duffau H . Brain hodotopy: From esoteric concept to practical surgical applications. Neurosurgery. (2011) ;68: :1709–1723. |
[9] | Duffau H . The huge plastic potential of adult brain and the role of connectomics: New insights provided by serial mappings in glioma surgery. Cortex. (2014) ;58: :325–337. |
[10] | Catani M , Jones DK , ffytche DH . Perisylvian language networks of the human brain. Ann Neurol. (2005) ;57: :8–16. |
[11] | Le Bihan D , Poupon C , Amadon A , Lethimonnier F . Artifacts and pitfalls in diffusion MRI. J Magn Reson Imaging. (2006) ;24: :478–488. |
[12] | Hubbard PL , Parker GJM . Validation of tractography. In: Diffusion MRI ( Johansen-Berg H , Behrens T , eds), pp. 353–376. Burlington: Academic Press, (2009) . |
[13] | Jbabdi S , Johansen-Berg H . Tractography: Where do we go from here? Brain Connect. (2011) ;1: :169–183. |
[14] | Duffau H . Diffusion Tensor Imaging is a research and educational tool, but not yet a clinical tool. World Neurosurg.e. (2014) ;82: :43–45. |
[15] | Fernandez-Miranda JC , Pathak S , Engh J , Jarbo K , Verstynen T , Yeh FC , et al., High-definition fiber tractography of the human brain: Neuroanatomical validation and neurosurgical applications. Neurosurgery. (2012) ;71: :430–453. |
[16] | Sarubbo S , De Benedictis A , Maldonado IL , Basso G , Duffau H . Frontal terminations for the inferior fronto-occipital fascicle: Anatomical dissection, DTI study and functional considerations on a multi-component bundle. Brain Struct Funct. (2013) ;218: :21–37. |
[17] | Martino J , De Witt Hamer PC , Vergani F , Brogna C , de Lucas EM , Vázquez-Barquero A , et al., Cortex-sparing fiber dissection: An improved method for the study of white matter anatomy in the human brain. J Anat. (2011) ;219: :531–541. |
[18] | Martino J , Brogna C , Gil Robles S , Vergani F , Duffau H . Anatomic dissection of the inferior fronto-occipital fasciculus revisited in the lights of brain stimulation data. Cortex. (2010) ;46: :691–699. |
[19] | Martino J , De Witt Hamer PC , Berger MS , Lawton MT , Arnold CM , de Lucas EM , et al., Analysis of the subcomponents and cortical terminations of the perisylvian superior longitudinal fasciculus: A fiber dissection and DTI tractography study. Brain Struct Funct. (2013) ;218: :105–121. |
[20] | Duffau H . Resecting diffuse low-grade gliomas to the boundaries of brain functions: A new concept in surgical neuro-oncology. J Neurosurg Sci. (2015) ;59: :361–371. |
[21] | Fernández Coello A , Moritz-Gasser S , Martino J , Matsuda A , Duffau H . Selection of intraoperative tasks for awake mapping based on relationships between tumor location and functional networks. J Neurosurg. (2013) ;119: :1380–1394. |
[22] | Duffau H . The challenge to remove diffuse low-grade gliomas while preserving brain functions. Acta Neurochir (Wien). (2012) ;154: :569–574. |
[23] | Logothetis NK , Augath M , Murayama Y , Rauch A , Sultan F , Goense J , et al., The effects of electrical microstimulation on cortical signal propagation. Nat Neurosci. (2010) ;13: :1283–1291. |
[24] | Mandonnet E , Winkler P , Duffau H . Direct electrical stimulation as an input gate into brain functional networks: Principles, adavantages and limitations. Acta Neurochir. (2010) ;152: :185–193. |
[25] | Duffau H . Brain Mapping: From Neural Basis of Cognition to Surgical Applications. Duffau H , (Ed.). Springer Wien, New York, (2011) . |
[26] | Duffau H . Stimulation mapping of white matter tracts to study brain functional connectivity. Nat Rev Neurol. (2015) ;11: :255–265. |
[27] | Duffau H , Gatignol P , Mandonnet E , Peruzzi P , Tzourio-Mazoyer N , Capelle L . New insights into the anatomo-functional connectivity of the semantic system: A study using cortico-subcortical electrostimulations. Brain. (2005) ;128: :797–810. |
[28] | Thiebaut de Schotten M , Urbanski M , Duffau H , Volle E , Levy R , Dubois B , et al., Direct evidence for a parietal-frontal pathway subserving spatial awareness in humans. Science. (2005) ;309: :2226–2228. |
[29] | Mandonnet E , Nouet A , Gatignol P , Capelle L , Duffau H . Does the left inferior longitudinal fasciculus play a role in language? A brain stimulation study. Brain. (2007) ;130: :623–629. |
[30] | Schucht P , Moritz-Gasser S , Herbet G , Raabe A , Duffau H . Subcortical electrostimulation to identify network subserving motor control. Hum Brain Ma. (2013) ;34: :3023–3030. |
[31] | van Geemen K , Herbet G , Moritz-Gasser S , Duffau H . Limited plastic potential of the left ventral premotor cortex in speech articulation: Evidence From intraoperative awake mapping in glioma patients. Hum Brain Ma. (2014) ;35: :1587–1596. |
[32] | Kinoshita M , Menjot de Champfleur N , Deverdun J , Moritz-Gasser S , Herbet G , Duffau H . Role of fronto-striatal tract and frontal aslant tract in movement and speech: An axonal mapping study. Brain Struct Funct. (2015) ;220: :3399–3412. |
[33] | Duffau H , Moritz-Gasser S , Mandonnet E . Are-examination of neural basis of language processing: Proposal of a dynamic hodotopical model from data provided by brain stimulation mapping during picture naming. Brain Lang. (2014) ;131: :1–10. |
[34] | Jayakar P . Physiological principles of electrical stimulation. In: Devinsky O , Beric A , Dogali M , editors. Electrical and magnetic stimulation of the brain and spinal cord. New York, Raven Press Ltd, (1993) . |
[35] | Ranck JB . Extracellular stimulation. In: Patterson MM , Kesner RP , editors. Electrical stimulation research methods. New York: Academic Press, (1981) :2–36. |
[36] | Agnew WF , McCreery DB . Considerations for safety in the use of extracranial stimulation for motor evoked potentials. Neurosurgery. (1987) ;20: :143–147. |
[37] | Yeomans JS . Principles of brain stimulation, New York: Oxford University Press, (1990) . |
[38] | Gordon B , Lesser RP , Rance NE , Hart J Jr , Webber R , Uematsu S , et al., Parameters for direct cortical electrical stimulation in the human: Histopathologic confirmation. Electroencephalogr Clin Neurophysiol. (1990) ;75: :371–377. |
[39] | Boetto J , Bertram L , Moulinié G , Herbet G , Moritz-Gasser S , Duffau H . Low rate of intraoperative seizures during awake craniotomy in a prospective cohort with 374 supratentorial brain lesions: Electrocorticography is not mandatory. World Neurosurg. (2015) ;84: :1838–1844. |
[40] | Jayakar P , Alvarez LA , Duchowny MS , Resnick TJ . A safe and effective paradigm to functionally map the cortex in childhood. J Clin Neurophysiol. (1992) ;9: :288–293. |
[41] | Geddes LA , Baker LE . The specific resistance of biological material - a compendium of data for the biomedical engineer and physiologist. Med Biol Eng Comput. (1967) ;5: :271–293. |
[42] | Duffau H . Contribution of cortical and subcortical electrostimulation in brain glioma surgery: Methodological and functional considerations. Neurophysiol Clin. (2007) ;37: :373–382. |
[43] | Nathan SS , Lesser RP , Gordon B , Thakor NV . Electrical stimulation of the human cerebral cortex. Theoretical approach. In: Devinsky O , Beric A , Dogali M , editors. Electrical and magnetic stimulation of the brain and spinal Cord, New York: Raven Press, Ltd; (1993) . |
[44] | Haglund MM , Ojemann GA , Bleasdel GG . Optical imaging of bipolar cortical stimulation. J Neurosurg. (1993) ;78: :785–793. |
[45] | Vincent M , Rossel O , Hayashibe M , Herbet G , Duffau H , Guiraud D , Bonnetblanc F . The difference between electrical microstimulation and direct electrical stimulation - towards new opportunities for innovative functional brain mapping? Rev Neurosci, (2015) Dec 8. pii: /j/revneuro.ahead-of-print/revneuro–2015–0029/revneuro–2015–0029.xml. doi: 10.1515/revneuro-2015-0029 . [Epub ahead of print]. |
[46] | Duffau H , Lopes M , Arthuis F , Bitar A , Sichez JP , Van Effenterre R , et al., Contribution of intraoperative electrical stimulations in surgery of low grade gliomas: A comparative study between two series without (1985-96) and with (1996-2003)functional mapping in the same institution. J Neurol Neurosurg Psychiatry. (2005) ;76: :845–851. |
[47] | Lehericy S , Duffau H , Cornu P , Capelle L , Pidoux B , Carpentier A , et al., Correspondence between functional magnetic resonance imaging somatotopy and individual brain anatomy of the central region: Comparison with intraoperative stimulation in patients with brain tumors. J Neurosurg. (2000) ;92: :589–598. |
[48] | Tarapore PE , Tate MC , Findlay AM , Honma SM , Mizuiri D , Berger MS , et al., Preoperative multimodal motor mapping: A comparison of magnetoencephalography imaging, navigated transcranial magnetic stimulation, and direct cortical stimulation. J Neurosurg. (2012) ;117: :354–362. |
[49] | Kuchcinski G , Mellerio C , Pallud J , Dezamis E , Turc G , Rigaux-Viodé O , et al., Three-tesla functional MR language mapping: Comparison with direct cortical stimulation in gliomas. Neurology. (2015) ;84: :560–568. |
[50] | Ille S , Sollmann N , Hauck T , Maurer S , Tanigawa N , Obermueller T , et al., Combined noninvasive language mapping by navigated transcranial magnetic stimulation and functional MRI and its comparison with direct cortical stimulation. J Neurosurg. (2015) ;123: :212–225. |
[51] | Leclercq D , Duffau H , Delmaire C , Capelle L , Gatignol P , Ducros M , et al., Comparison of diffusion tensor imaging tractography of language tracts and intraoperative subcortical stimulations. J Neurosurg. (2010) ;112: :503–511. |
[52] | Luders H , Lesser RP , Hahn J , Dinner DS , Morris HH , Wyllie E , et al., Basal temporal language area. Brain. (1991) ;114: :743–754. |
[53] | Duffau H , Capelle L , Denvil D , Sichez N , Gatignol P , Taillandier L , et al., Usefulness of intraoperative electrical subcortical mapping during surgery for low-grade gliomas located within eloquent brain regions: Functional results in a consecutive series of 103 patients. J. Neurosurg. (2003) ;98: :764–778. |
[54] | Maldonado IL , Mandonnet E , Duffau H . Dorsal fronto-parietal connections of the human brain: A fiber dissection study of their composition and anatomical relationships. Anat Rec. (Hoboken). (2012) ;295: :187–195. |
[55] | Rech F , Herbet G , Moritz-Gasser S , Duffau H . Somatotopic organization of the white matter tracts underpinning motor control in humans: An electrical stimulation study. Brain Struct Funct. (2015) Oct 12. [Epub ahead of print]. |
[56] | Rech F , Herbet G , Moritz-Gasser S , Duffau H . Disruption of bimanual movement by unilateral subcortical stimulation. Hum Brain Ma. (2014) ;35: :3439–3445. |
[57] | Maldonado IL , Moritz-Gasser S , de Champfleur NM , Bertram L , Moulinié G , Duffau H . Surgery for gliomas involving the left inferior parietal lobule: New insights into the functional anatomy provided by stimulation mapping in awake patients. J Neurosurg. (2011) ;115: :770–779. |
[58] | Almairac F , Herbet G , Moritz-Gasser S , Duffau H . Parietal network underlying movement control: Disturbances during subcortical electrostimulation. Neurosurg Rev. (2014) ;37: :513–516. |
[59] | Catani M , Dell’acqua F , Vergani F , Malik F , Hodge H , Roy P , et al., Short frontal lobe connections of the human brain. Cortex. (2012) ;48: :273–291. |
[60] | Gras-Combes G , Moritz-Gasser S , Herbet G , Duffau H . Intraoperative subcortical electrical mapping of optic radiations in awake surgery for glioma involving visual pathways. J Neurosurg. (2012) ;117: :466–473. |
[61] | Duffau H , Velut S , Mitchell MC , Gatignol P , Capelle L . Intra-operative mapping of the subcortical visual pathways using direct electrical stimulations. Acta Neurochir (Wien). (2004) ;146: :265–269. |
[62] | Hickok G , Poeppel D . The cortical organization of speech processing. Nat Rev Neurosci. (2007) ;8: :393–402. |
[63] | Maldonado IL , Moritz-Gasser S , Duffau H . Does the left superior longitudinal fascicle subserve language semantics? A brain electrostimulation study. Brain Struct Funct. (2011) ;216: :263–264. |
[64] | Duffau H , Capelle L , Sichez N , Denvil D , Bitar A , Sichez JP , et al., Intraoperative mapping of the subcortical language pathways using direct stimulations. An anatomo-functional study. Brain. (2002) ;125: :199–214. |
[65] | Moritz-Gasser S , Duffau H . The anatomo-functional connectivity of word repetition: Insights provided by awake brain tumor surgery. Front Hum Neurosci. (2013) ;7: :405. |
[66] | Geschwind N . The organization of language and the brain. Science. (1970) ;170: :940–944. |
[67] | Vigneau M , Beaucousin V , Herve PY , Duffau H , Crivello F , Houdé O , et al., Meta-analyzing left hemisphere language areas: Phonology, semantics, and sentence processing. Neuroimage. (2006) ;30: :1414–1432. |
[68] | Zemmoura I , Herbet G , Moritz-Gasser S , Duffau H . New insights into the neural network mediating reading processes provided by cortico-subcortical electrical mapping. Hum Brain Ma. (2015) ;36: :2215–2230. |
[69] | Duffau H , Capelle L , Denvil D , Gatignol P , Sichez N , Lopes M , et al., The role of dominant premotor cortex in language: A study using intraoperative functional mapping in awake patients. Neuroimage. (2003) ;20: :1903–1914. |
[70] | Makris N , Kennedy DN , McInerney S , Sorensen AG , Wang R , Caviness VS Jr , et al., Segmentation of subcomponents within the superior longitudinal fascicle in humans: A quantitative, in vivo, DT-MRI study . Cereb Cortex. (2005) ;15: :854–869. |
[71] | Vidorreta JG , Garcia R , Moritz-Gasser S , Duffau H . Double dissociation between syntactic gender and picture naming processing: A brain stimulation mapping study. Hum Brain Ma. (2011) ;32: :331–340. |
[72] | Saur D , Kreher BW , Schnell S , Kümmerer D , Kellmeyer P , Vry MS , et al., Ventral and dorsal pathways for language. Proc Natl Acad Sci U S A. (2008) ;105: :18035–18040. |
[73] | Makris N , Pandya DN . The extreme capsule in humans and rethinking of the language circuitry. Brain Struct Funct. (2009) ;213: :343–358. |
[74] | Schmahmann JD , Pandya DN . Fiber Pathways of the Brain. Oxford University Press, New York, (2006) . |
[75] | Mandonnet E , Nouet A , Gatignol P , Capelle L , Duffau H . Does the left inferior longitudinal fasciculus play a role in language? A brain stimulation study. Brain. (2007) ;130: :623–629. |
[76] | Mandonnet E , Gatignol P , Duffau H . Evidence for an occipito-temporal tract underlying visual recognition in picture naming. Clin Neurol Neurosurg. (2009) ;111: :601–605. |
[77] | Makris N , Papadimitriou GM , Kaiser JR , Sorg S , Kennedy DN , Pandya DN . Delineation of the middle longitudinal fascicle in humans: A quantitative, in vivo, DT-MRI study . Cereb Cortex. (2009) ;19: :777–785. |
[78] | Maldonado IL , de Champfleur NM , Velut S , Destrieux C , Zemmoura I , Duffau H . Evidence of a middle longitudinal fasciculus in the human brain from in vitro dissection . J Anat. (2013) ;223: :38–45. |
[79] | Menjot de Champfleur N , Maldonado IL , Moritz-Gasser S , Machi P , Le Bars E , Bonafé A , et al., Middle longitudinal fasciculus delineation within language pathways: A diffusion tensor imaging study in human. Eur J Radiol. (2013) ;82: :151–157. |
[80] | Khan OH , Herbet G , Moritz-Gasser S , Duffau H . The role of left inferior fronto-occipital fascicle in verbal perseveration: A brain electrostimulation mapping study. Brain Topogr. (2014) ;27: :403–411. |
[81] | Holland R , Lambon-Ralph MA . The anterior temporal lobe semantic hub is a part of the language neural network: Selective disruption of irregular past tense verb by rTMS. Cereb Cortex. (2010) ;20: :2771–2775. |
[82] | Chan-Seng E , Moritz-Gasser S , Duffau H . Awake mapping for low-grade gliomas involving the left sagittal stratum: Anatomofunctional and surgical considerations. J Neurosurg. (2014) ;120: :1069–1077. |
[83] | Duffau H . Does post-lesional subcortical plasticity exist in the human brain? Neurosci Res. (2009) ;65: :131–135. |
[84] | Duffau H , Gatignol P , Moritz-Gasser S , Mandonnet E . Is the left uncinate fasciculus essential for language? A cerebral stimulation study. J Neurol. (2009) ;256: :382–389. |
[85] | Duffau H , Thiebaut de Schotten M , Mandonnet E . White matter functional connectivity as an additional landmark for dominant temporal lobectomy. J Neurol Neurosurg Psychiatry. (2008) ;79: :492–495. |
[86] | Duffau H , Herbet G , Moritz-Gasser S . Toward a pluri-component, multimodal, and dynamic organization of the ventral semantic stream in humans: Lessons from stimulation mapping in awake patients. Front Syst Neurosci. (2013) ;7: :44. |
[87] | De Witt Hamer P , Moritz-Gasser S , Gatignol P , Duffau H . Is the human left middle longitudinal fascicle essential for language? A brain electrostimulation study. Hum Brain Ma. (2011) ;32: :962–973. |
[88] | Fernández Coello A , Duvaux S , De Benedictis A , Matsuda R , Duffau H . Involvement of the right inferior longitudinal fascicle in visual hemiagnosia: A brain stimulation mapping study. J Neurosurg. (2013) ;118: :202–205. |
[89] | Spena G , Gatignol P , Capelle L , Duffau H . Superior longitudinal fasciculus subserves vestibular network in humans. Neuroreport. (2006) ;17: :1403–1406. |
[90] | Herbet G , Lafargue G , Bonnetblanc F , Moritz-Gasser S , Duffau H . Is the right frontal cortex really crucial in the mentalizing network? A longitudinal study in patients with a slow-growing lesion. Cortex. (2013) ;49: :2711–2727. |
[91] | Herbet G , Lafargue G , Moritz-Gasser S , Bonnetblanc F , Duffau H . Interfering with the neural activity of mirror-related frontal areas impairs mentalistic inferences. Brain Struct Funct. (2015) ;220: :2159–2169. |
[92] | Herbet G , Lafargue G , Bonnetblanc F , Moritz-Gasser S , Menjot de Champfleur N , Duffau H . Inferring a dual-stream model of mentalizing from associative white matter fibres disconnection. Brain. (2014) ;137: :944–959. |
[93] | Moritz-Gasser S , Duffau H . Cognitive processes and neural basis of language switching: Proposal of a new model. Neuroreport. (2009) ;20: :1577–1580. |
[94] | Thiebaut de Schotten M , Dell’Acqua F , Valabregue R , Catani M . Monkey to human comparative anatomy of the frontal lobe association tracts. Cortex. (2012) ;48: :82–96. |
[95] | Vergani F , Lacerda L , Martino J , Attems J , Morris C , Mitchell P , et al., White matter connections of the supplementary motor area in humans. J Neurol Neurosurg Psychiatry. (2014) ;85: :1377–1385. |
[96] | Kemerdere R , de Champfleur NM , Deverdun J , Cochereau J , Moritz-Gasser S , Herbet G , et al., Role of the left frontal aslant tract in stuttering: A brain stimulation and tractographic study. J Neurol. (2016) ;263: :157–167. |
[97] | Gil Robles S , Gatignol P , Capelle L , Mitchell MC , Duffau H . The role of dominant striatum in language: A study using intraoperative electrical stimulations. J Neurol Neurosurg Psychiatry. (2005) ;76: :940–946. |
[98] | Moritz-Gasser S , Herbet G , Duffau H . Mapping the connectivity underlying multimodal (verbal and non-verbal) semantic processing: A brain electrostimulation study. Neuropsychologia. (2013) ;51: :1814–1822. |
[99] | Whitney C , Kirk M , O’Sullivan J , Lambon Ralph MA , Jefferies E . The neural organization of semantic control: TMS evidence for a distributed network in left inferior frontal and posterior middle temporal gyrus. Cereb Cortex. (2011) ;21: :1066–1075. |
[100] | Herbet G , Lafargue G , de Champfleur NM , Moritz-Gasser S , le Bars E , Bonnetblanc F , et al., Disrupting posterior cingulate connectivity disconnects consciousness from the external environment. Neuropsychologia. (2014) ;56: :239–244. |
[101] | Herbet G , Lafargue G , Duffau H . The dorsal cingulate cortex as a critical gateway in the network supporting conscious awareness. Brain. (2015) Dec 30. pii: awv381. [Epub ahead of print]. |
[102] | Ius T , Angelini E , de Schotten MT , Mandonnet E , Duffau H . Evidence for potentials and limitations of brain plasticity using an atlas of functional respectability of WHO grade II gliomas: Towards a “minimal common brain”. Neuroimage. (2011) ;56: :992–1000. |
[103] | Herbet G , Maheu M , Costi E , Lafargue G , Duffau H . Mapping neuroplastic potential in brain-damaged patients. Brain. (2016) ;139: :829–844. |
[104] | Duffau H . A two-level model of interindividual anatomo-functional variability of the brain and its implications for neurosurgery. Cortex, (2016) Jan 29. pii: S0010-9452(16)00011-3. doi: 10.1016/j.cortex.2015.12.009 . [Epub ahead of print]. |
[105] | Sarubbo S , de Benedictis A , Merler S , Mandonnet E , Balbi S , Granieri E , et al., Towards a functional atlas of human white matter. Hum Brain Ma. (2015) ;36: :3117–3136. |
Figures and Tables
Fig.1
A. Preoperative axial FLAIR-weighted MRI, coronal T2-weighted MRI and sagittal enhanced T1-weighted MRI, in a 42 year-old right-handed male who presented with partial seizures and language disorders on presurgical cognitive examination, showing a voluminous glioma involving the left temporal lobe. B: Intraoperative view before resection. The anterior part of the left hemisphere is on the right and its posterior part is on the left. Letter tags correspond to the projection on the cortical surface of the tumor limits identified using ultrasonography. Number tags show zones of positive DES mapping as follows, 1, 2 and 3: ventral premotor cortex (generating anarthria during DES); 4 (mid-part of the superior temporal gyrus): areas involved in lexical access (eliciting anomia during DES); 5 (posterior part of the superior temporal gyrus): areas involved in semantic processing (inducing semantic paraphasia during DES). C: Intraoperative view after resection, achieved up to eloquent structures, both at cortical and subcortical levels. Indeed, DES of white matter tracts allowed the detection of the ventral stream underpinned by the IFOF (eliciting semantic paraphasias when stimulated, tag 46); the detection of the inferior longitudinal fascicle (anterior part of the posterior segment, generating reading disturbances during DES, tag 47); the optic radiations more deeply located (inducing transient visual disturbances when stimulated, tag 50); as well as the detection of the temporal part of the SLF subserving the dorsal stream (eliciting phonological disorders when stimulated, tag 49). D: Immediate axial FLAIR-weighted MRI, coronal T2-weighted MRI and sagittal enhanced T1-weighted MRI, showing a complete tumor removal. The patient benefited from a cognitive rehabilitation within the weeks following resection, and improved in comparison with the presurgical status, on the postsurgical language assessment achieved 3 months later. The diagnosis of low-grade glioma was confirmed, and no adjuvant oncological treatment was administrated. The patient resumed a normal familial, social and professional life within 3 months following surgery, with no functional deficits (no neurological and no cognitive disorders, no seizures) thanks to the preservation of the subcortical connectivity - despite an extensive resection of the temporal lobe in the left hemisphere.
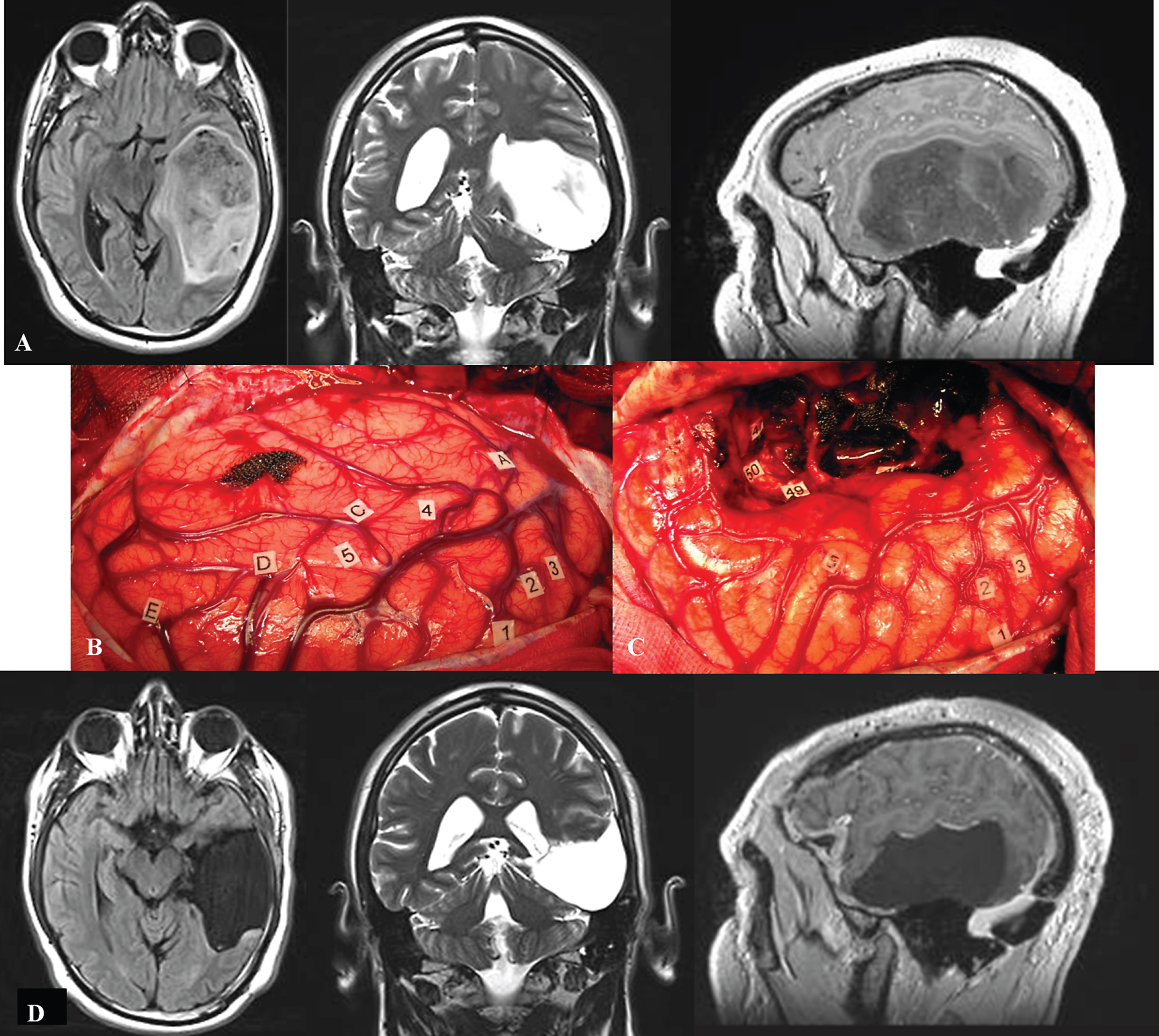