Hyperthermia Improves Solubility of Intravesical Chemotherapeutic Agents
Abstract
BACKGROUND:
Nearly 70% of all new cases of bladder cancer are non-muscle invasive disease, the treatment for which includes transurethral resection followed by intravesical therapy. Unfortunately, recurrence rates approach 50% in part due to poor intravesical drug delivery. Hyperthermia is frequently used as an adjunct to intravesical chemotherapy to improve drug delivery and response to treatment.
OBJECTIVE:
To assess the solubility profile of intravesical chemotherapies under varying conditions of pH and temperature.
METHODS:
Using microplate laser nephelometry we measured the solubility of three intravesical chemotherapy agents (mitomycin C, gemcitabine, and cisplatin) at varying physical conditions. Drugs were assessed at room temperature (23°C), body temperature (37°C), and 43°C, the temperature used for hyperthermic intravesical treatments. To account for variations in urine pH, solubility was also investigated at pH 4.00, 6.00, and 8.00.
RESULTS:
Heat incrementally increased the solubility of all three drugs studied. Conversely, pH largely did not impact solubility aside for gemcitabine which showed slightly reduced solubility at pH 8.00 versus 6.00 or 4.00. Mitomycin C at the commonly used 2.0 mg/mL was insoluble at room temperature, but soluble at both 37 and 43°C.
CONCLUSIONS:
Hyperthermia as an adjunct to intravesical treatment would improve drug solubility, and likely drug delivery as some current regimens are insoluble without heat. Improvements in solubility also allow for testing of alternative administration regimens to improve drug delivery or tolerability. Further studies are needed to confirm that improvements in solubility result in increased drug delivery.
INTRODUCTION
The physiologic purpose of the bladder epithelium (urothelium) is to provide a strong barrier against the absorption of physiologically undesirable molecules contained in the urine, a fact that has the unintended consequence of impairing intravesical drug delivery [1, 2]. Unfortunately, despite adjuvant and maintenance treatments, roughly half of non-muscle invasive bladder cancer (NMIBC) patients will experience tumor recurrences requiring intravesical treatments and/or subsequent procedures [3]. The application of heat to the bladder, a process known as hyperthermia, is an adjunct used to improve the efficacy of intravesical chemotherapy. Hyperthermia is thought to work by increasing drug delivery to the bladder, enhancing the anti-neoplastic effects of the therapeutic agents, and eliciting an anti-cancer immune response [4–10].
Intravesical drug bioavailability is dependent on solubility, permeability, metabolism, and efflux from urothelial, submucosal, and muscular layers [11]. Solubility is a modifiable contributor to bioavailability, and is affected by many factors including drug concentration, urine production rate, urine/drug pH, urine constituents, and temperature [11–13]. An optimized intravesical mitomycin C (MMC) dose of 2.0 mg/mL (40 mg/20 mL) has previously been proposed, but evidence suggests this concentration of MMC is insoluble without special preparation including sample heating prior to instillation [14, 15]. The precise effect of clinical bladder hyperthermia, where the entire bladder and its contents are heated to 43°C, on intravesical drug solubility has not been determined. Generally, heat improves drug solubility, and consequently some of the improved drug delivery that is shown with bladder hyperthermia might be due to improved drug solubility [16]. Optimized administration regimens of intravesical gemcitabine without hyperthermia exist, but there is a lack of data on the solubility of gemcitabine when heated [17]. Furthermore, individuals’ urinary pH varies significantly from 4.5–8.5 which may also impact intravesical drug solubility [18, 19].
Various methods of measuring kinetic drug solubility exist but nephelometry, the quantification of light scattering as it passes through a sample, is thought to be one of the most accurate and is commonly used during drug development for rapid, high-throughput solubility screens to identify viable drug candidates [12, 20]. As the number of insoluble particles increases, a higher fraction of the laser light passing through the solution is scattered, and detected by the nephelometer [20]. Nephelometry with serial drug dilutions is commonly used to determine a material’s kinetic solubility point at which further concentration increases result in a rapid drug precipitation and, consequently, increased laser light scatter in the nephelometer [12, 21, 22]. In this manuscript we study the impact of clinical hyperthermia at 43°C and pH on the solubility of three intravesical drugs commonly used to treat bladder cancer: MMC, gemcitabine, and cisplatin.
METHODS
Instruments, chemicals, materials
A NEPHELOstar Galaxy microplate reader (BMG LABTECH GmbH, Ortenberg, Germany) was used for rapid laser nephelometry testing. The machine passes a 635 nm laser through the sample and measures the fraction of scattered light due to insoluble particles, which is then quantified in Relative Nephelometry Units (RNUs). Outputs range from 0 to a maximum of 500,000 RNUs for insoluble samples. Calibration studies demonstrated a linear relationship (r2 = 0.999) between RNU and Nephelometric Turbidity Units (NTUs), another common measure of turbidity and therein solubility, such that 1 NTU is roughly equivalent to 5,000 RNUs [23]. The nephelometer was programmed such that each well was read for 1 second with a 0.5 second positioning delay, for a total plate reading time of 144 seconds. Laser intensity was set to 80% with beam focus of 1.8 mm per manufacturer recommendations given aliquot volume and well size. Given the device sensitivity, scratch resistant 96-well UV-transparent plates (Greiner Bio-One, Monroe NC. Item No. 5665-5801) were used.
Clinical grade mitomycin C (Accord Healthcare Inc., Durham NC, Lot PY03429), gemcitabine HCl (AAP Pharmaceuticals LLC, Paramus NJ, Lot 6018570), and cisplatin (WG Critical Care LLC, Schaumburg IL, Lot 9D05740) were obtained from the Duke University Hospital Cancer Center pharmacy (Durham, NC). Drugs were reconstituted and serially diluted with phosphate-buffered saline (PBS, pH 7.4, Mediatech Inc, Manassas VA, Lot 13518005), adjusted with HCl or NaHCO3 to achieve target pH. Measurements of pH were performed using a Fisherbrand Accumet micro-pH probe (Thermo Fisher Scientific, Waltham MA) and Mettler-Toledo 320 pH meter (Mettler-Toledo LLC, Columbus OH). Prior to use, the pH meter was calibrated using standard pH 4.01, 7.01, and 10.01 buffer solutions (Genesee Scientific, San Diego CA)
Experimental design
The initial concentration ranges studied for each drug were based on standard intravesical administration doses –mitomycin C 1.0–2.0 mg/mL, gemcitabine 20–40 mg/mL, and cisplatin 0.6–1.0 mg/mL [24–32]. For gemcitabine and cisplatin, when standard doses were shown to be easily soluble with hyperthermia, upper limits were increased to 80 and 3.5 mg/mL respectively to identify the heated kinetic solubility points.
Three temperatures were assessed: 23°C (room temperature, the standard temperature at which drug solutions are prepared), 37°C (standard body temperature, the highest temperature that a drug solution might get under normal physiologic circumstances), and 43°C (clinical hyperthermia). Initial exploratory studies were performed without pH adjustment, deemed standard pH preparations, with each drug serially diluted using un-adjusted PBS (pH 7.4). The resultant pH of these solutions stayed at 7.4 for both MMC and cisplatin, but gemcitabine decreased the pH to 3.5. To prepare pH adjusted samples, HCl or NaHCO3 was used to bring PBS to a pH of 4.00, 6.00, or 8.00 which was then used for the subsequent serial dilutions.
We attempted to prepare our samples in accordance with typical preparation in our institution’s pharmacy prior to clinical administration. Samples were prepared from powdered drug the same day as serial dilution, plating, heating, and nephelometric measurements to avoid long term storage of drug solutions.
As serial dilutions were performed at room temperature, there was visible drug precipitation in each of the 3 chemotherapies at their highest concentrations, which slowly dissolved with each serial dilution. Therefore, each solution was thoroughly vortexed prior to aliquoting to minimize concentration variation between aliquots. After each step in serial dilution for each concentration-pH permutation, drug solutions were aliquoted at 200μL volumes in quadruplicate and plated on 96-well microplates which were then sealed to minimize evaporative loss and condensation. Initial nephelometry measurements were obtained to represent room temperature readings, and plates were placed into water baths heated either to 37 or 43°C. Measurements were repeated at 60 and 120 min, standard dwell times for intravesical therapy. Of note, these measurements required removal of the plates from water baths for nephelometric measurement (144 seconds) after which they were returned to their respective water baths.
Data analysis
Raw data were exported from the MARS Data Analysis package (BMG Labtech, version 2.4.1) for analysis. To obtain a blank corrected value for each data point, the RNU reading from each well was corrected with an average of the four “blank” wells containing only solvent and no solute. Due to the sensitive nature of laser nephelometry, false positive readings are commonly seen from plate defects (scratches, fingerprints, inconsistencies in plastic) and competing particulates (bubbles, dust) and we took extensive measures to minimize these. While some authors have reported manually removing outlier data resulting from these issues after each nephelometer run [12], others have filtered out values deviating more than two standard deviations from the mean [21]. We defined outlier values as those deviating >2 standard deviations from the mean or >450,000 RNU for samples with a standard deviation >150,000 RNU. This appropriately identified the false positives as “device maximum” values that vastly increased the grouping’s standard deviation. Segmental linear regression was used to analyze nephelometric data, with the regression’s inflection or break point identifying the concentration at which there was an abrupt change in drug solubility (i.e. the sample’s kinetic solubility point under those physical conditions) [33–35]. Data were analyzed in R version 3.6.2 using RStudio 1.2.1 with the following packages installed: readxl, tidyverse, dplyr, tibble, janitor, ggplot2, segmented, gridExtra.
RESULTS
Overall, laser nephelometry was effective in quickly assessing the solubility of all three chemotherapies across a large range of physical conditions. Outlier detection rates were similar across groups –52 (5.6%) MMC datapoints, 41 (4.7%) gemcitabine datapoints, and 42 (4.5%) cisplatin. Samples at the highest concentration ranges were visibly turbid at room temperature but not after heating despite the nephelometer still detecting solute precipitation. For heated samples, we chose to compare 120-minute solubility data between drugs as, although similar in most cases, in a few select cases (gemcitabine 43°C pH 4.00 and 8.00) there was a continued improvement insolubility after 120 compared to 60 minutes (Appendix 1B).
Mitomycin C
As expected, the addition of heat incrementally increases the solubility of mitomycin C (Fig. 1A). A more substantial improvement in solubility is noted between 23°C and 37°C than between 37°C and 43°C. We did not demonstrate a consistent relationship between MMC solubility and solution pH, suggesting that MMC solubility is less affected by pH than previously thought (Fig. 2A). At 23°C the solubility point of MMC ranged from 0.8–0.9 mg/mL depending on solute pH (Table 1A). As shown in Fig. 3, the commonly used dose of 2.0 mg/mL is insoluble at 23°C, but its solubility is incrementally improved with temperature such that preparations at 37°C and 43°C become soluble. In contrast to MMC, commonly used dosages of gemcitabine and cisplatin did not appear to exhibit impaired solubility at room temperature (Fig. 3). A regression breakpoint was not identifiable for MMC samples heated to 37°C or 43°C as solutions even at the maximum of 2.0 mg/mL were soluble. Despite the lack of quantitative comparison, the regression slopes illustrate an appreciable improvement in raw RNUs with heat at concentrations above 1.0 mg/mL across all pH parameters (Figs. 1A, 2A). Comparison of solubility plots for heated samples showed similar results after 60 and 120 minutes of heating (Appendix 1A).
Fig. 1
Solubility plots and segmental regressions at room temperature (23°C, blue), body temperature (37°C, green) and hyperthermic temperature (43°C, red) for Mitomycin C (A), Gemcitabine (B), and Cisplatin (C). Each shows a scatter plot of nephelometric data (RNUs) versus concentration (mg/mL) and the subsequent segmental regression with inflection point corresponding to the preparation’s kinetic solubility point. Abbreviations: BP = Breakpoint, NBP = No breakpoint calculated (no clear inflection point of solubility plot).
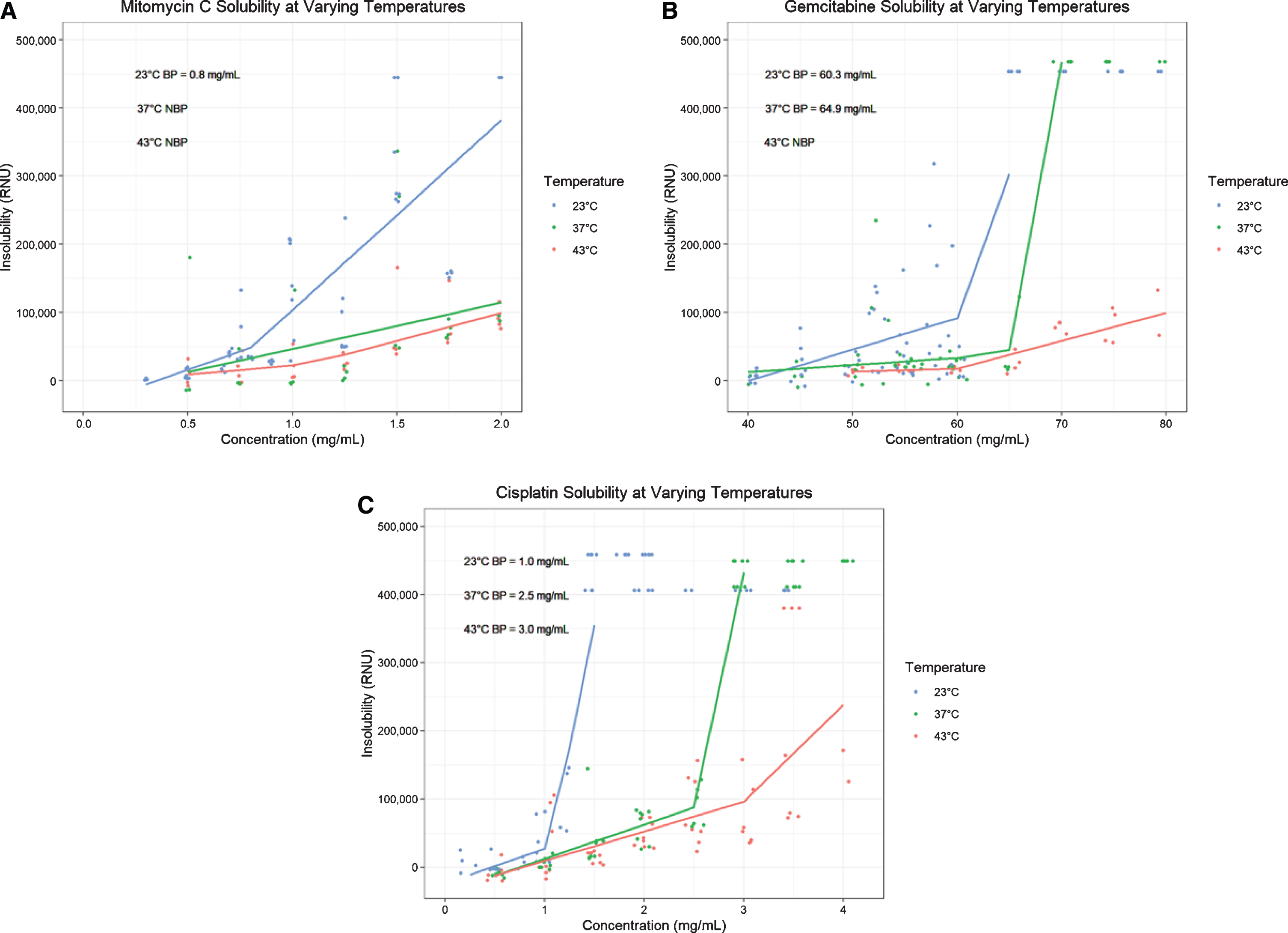
Fig. 2
Solubility plots and segmental regressions by temperature and pH for Mitomycin C (A), Gemcitabine (B), and Cisplatin (C). Each row of solubility plots representing a temperature (23°C, 37°C, 43°C), and each column a pH (4.00, 6.00, 8.00). The included breakpoints represent estimated kinetic solubility points derived from segmental linear regressions, all in mg/mL. Abbreviations: BP = Breakpoint, NBP = No breakpoint calculated (no clear inflection point of solubility plot).
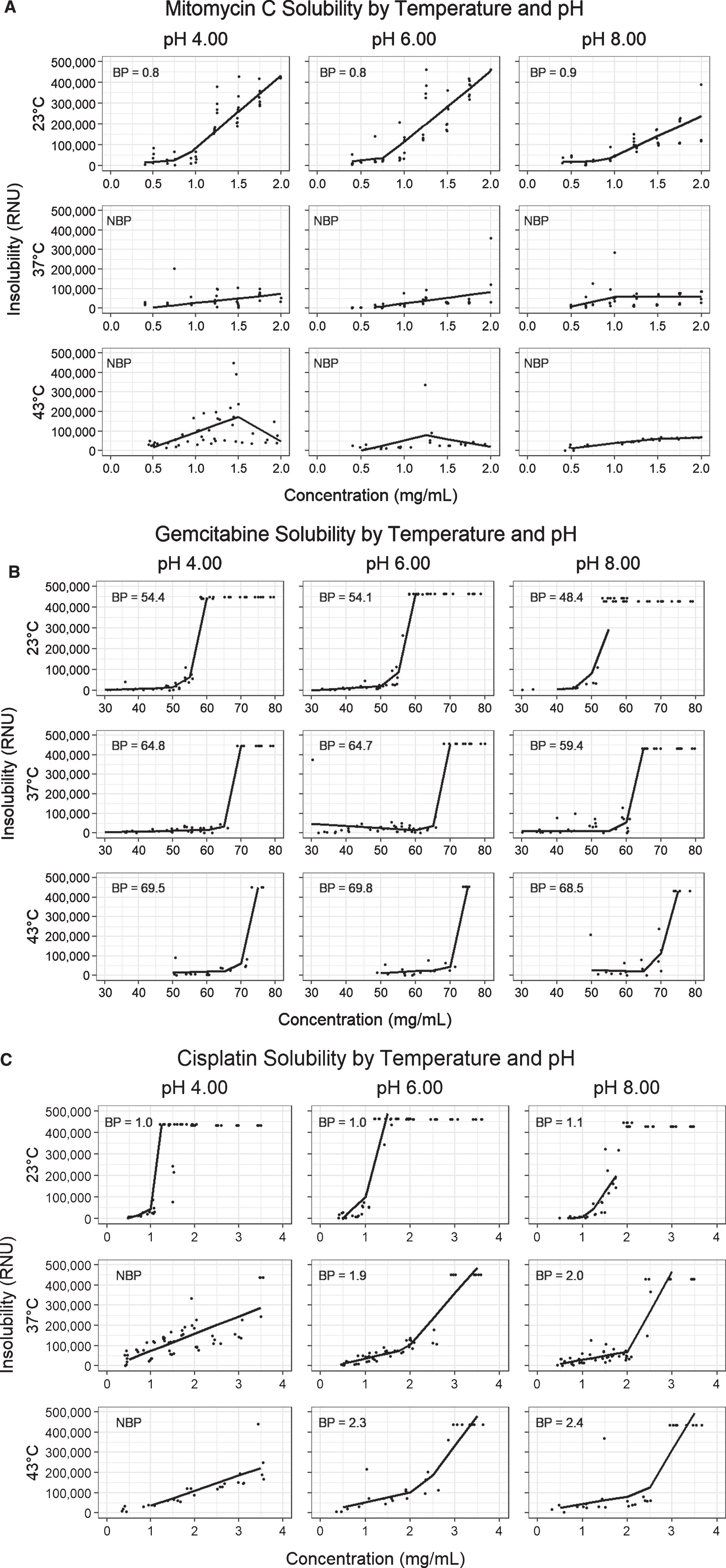
Table 1
Kinetic Solubility point estimates for Mitomycin C
A) Mitomycin C Kinetic Solubility Points (mg/mL) | ||||||
pH | ||||||
Standard | 4.00 | 6.00 | 8.00 | Mean | ||
23°C | 0.8 | 0.8 | 0.8 | 0.9 | 0.8 | |
37°C | 60 min | NBP | NBP | NBP | NBP | |
120 min | NBP | NBP | NBP | NBP | ||
43°C | 60 min | NBP | NBP | NBP | NBP | |
120 min | NBP | NBP | NBP | NBP |
Abbreviations: NBP = No breakpoint calculated.
Fig. 3
Solubility of common dosages of Mitomycin C (2.0 mg/mL), Gemcitabine (50 mg/mL), and Cisplatin (0.5 mg/mL) at 23°C, 37°C, and 43°C at unadjusted pH.
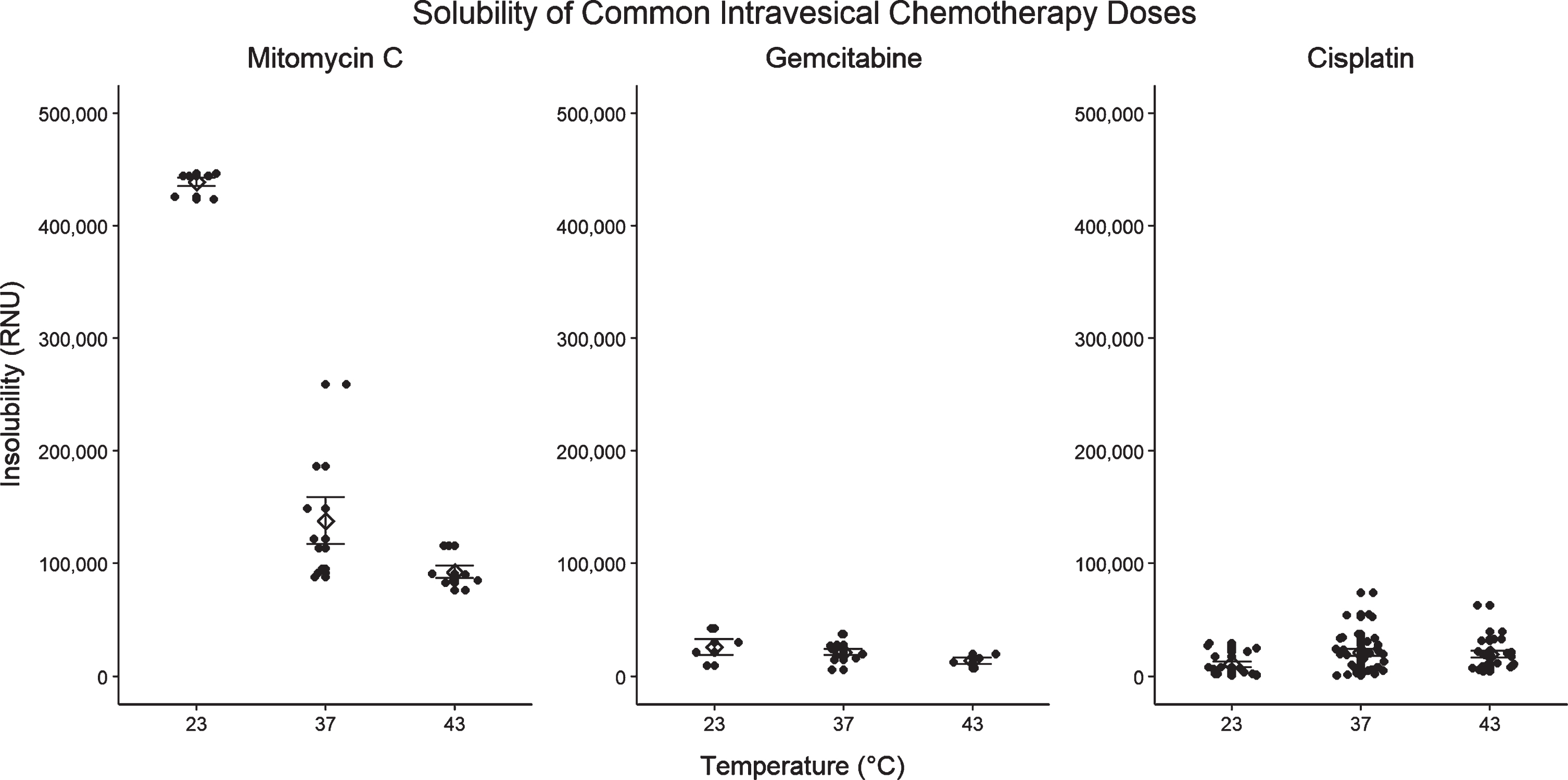
Gemcitabine
With increasing temperature, there is an incremental increase in the kinetic solubility point of gemcitabine, however observed solubility points are well above standard dosages even at room temperature (Figs. 1B, and 3) [30, 31]. At 23°C, gemcitabine precipitates at a mean of 54.3 mg/mL (range 48.4–60.3 depending on pH) compared to 63.5 mg/mL (range 59.4–64.9) and 69.3 mg/mL (range 68.5–69.8) after 120 minutes at 37°C and 43°C respectively (Table 1B). Our data show that dosages up to 60 mg/mL remain soluble even after 2 hours of hyperthermia at 43°C. For the most part, as with MMC, we did not observe a substantial difference in solubility between samples heated for 60 versus 120 minutes (Appendix 1B). Due to an experimental oversight, the solubility of gemcitabine at 43°C for 60-minutes was not assessed and is missing. At both 23°C and 37°C the solubility of pH 8.00 gemcitabine was slightly lower than that of the standard pH, pH 4.00, or pH 6.00 preparations (Fig. 2B).
Cisplatin
Of the three drugs studied, cisplatin demonstrated the strongest improvement in solubility with heat (Fig. 1C). This improvement was evident after 60 minutes of incubation, without substantial further improvement in solubility at 120 minutes (Appendix 1C). Average of the solubility points at 43°C was 2.6 mg/mL (range 2.3–3.0 depending on pH) compared to 2.1 mg/mL (range 1.9–2.5) at 37°C and 1.0 mg/mL (range 1.0–1.1) at 23°C (Table 1C). Like MMC, the drug’s solubility profile appears resistant to changes in pH (Fig. 2C).
DISCUSSION
Despite a randomized trial demonstrating improved intravesical MMC efficacy using concentrations of 2.0 mg/mL versus 1.0 mg/mL, this regimen is not universally used, in part due to concerns about the high concentration’s solubility when prepared under conventional methods [6, 14, 25, 36, 37]. Myers et al. report that standard preparations of 1.0 and 2.0 mg/mL are insoluble when prepared at room temperature. However, after heating at 50°C for 50 min, the drug dissolves and remains stable for 6 hours storage at 37°C [14]. Similarly, we demonstrated a solubility point of 0.8 mg/mL for room temperature MMC and show that even concentrations of 2.0 mg/mL become soluble when heated to 37°C or 43°C. Therefore, while a 2.0 mg/mL MMC solution is insoluble at preparation in the pharmacy, after it warms up in the human bladder it probably becomes soluble, but this takes time and reduces effective dwell time.
Initial preclinical investigations of intravesical gemcitabine in dogs showed that doses of 350 mg three times weekly (equivalent to a human dose of 1,000 mg/m2) were well tolerated without demonstrable side effects [38]. Phase 1 and 2 trials then used dosages of 500–2,000 mg in 100 mL normal saline, citing solubility as the reason higher concentrations were not included [26, 27]. Gemcitabine was detected in the serum of patients receiving 2,000 mg, however no dose limiting toxicities were observed [27]. Subsequent studies report doses up to 40 mg/mL are well tolerated despite systemic detection of low amounts of gemcitabine, <1μg/mL, and/or it’s inactive metabolite 2’,2’-difluorodeoxyuridine (dFdU) [28, 29, 37]. At 43°C, we show that gemcitabine exhibits a kinetic solubility point >60 mg/mL allowing for administration of much higher doses that may improve intravesical drug delivery, albeit likely with increased systemic absorption.
Enhanced solubility also allows for administration of higher concentrations that reduce a dose’s necessary volume which may improve tolerability and dwell time, especially in patients with small bladder capacities. Gontero et al. proposed an optimized intravesical gemcitabine regimen of 2,000 mg in 100 mL saline at pH 5.5 for 2 hours [17]. However, they notably had to exclude the 2,000 mg gemcitabine in 50 mL arms (concentration 40 mg/mL) due to insolubility. With hyperthermia, preparations of 40 and even 60 mg/mL are consistently soluble and may improve drug delivery over 100 mL preparations [25]. Gemcitabine has a pKa of 3.6, resulting in a solution pH of 2.7–3.2 when reconstituted which may cause chemical cystitis [26, 27]. As such, the pH of intravesical gemcitabine is often adjusted to 5.5–7 for intravesical use to improve tolerability [17, 26, 27]. At 23°C and 37°C, the solubility of gemcitabine at pH of 8.00 was slightly lower than at 4.00 or 6.00 (Fig. 2B), suggesting that over-correction of pH may impair solubility and therein drug delivery.
Table 2
Kinetic Solubility point estimates for Gemcitabine
B) Gemcitabine Kinetic Solubility Points (mg/mL) | |||||||
pH | |||||||
Standard | 4.00 | 6.00 | 8.00 | Mean | |||
23°C | 60.3 | 54.4 | 54.1 | 48.4 | 54.3 | ||
37°C | 60 min | 64.9 | 63.4 | 64.9 | 58.0 | 62.8 | |
120 min | 64.9 | 64.8 | 64.7 | 59.4 | 63.5 | ||
43°C | 60 min | NBP | 58.3 | 68.5 | 59.8 | 62.2 | |
120 min | NBP | 69.5 | 69.8 | 68.5 | 69.3 |
Abbreviations: NBP = No breakpoint calculated.
Table 3
Kinetic Solubility point estimates for Cisplatin
C) Cisplatin Kinetic Solubility Points (mg/mL) | ||||||
pH | ||||||
Standard | 4.00 | 6.00 | 8.00 | Mean | ||
23°C | 1.0 | 1.0 | 1.0 | 1.1 | 1.0 | |
37°C | 60 min | 2.3 | 3.0 | 2.3 | 2.0 | 2.4 |
120 min | 2.5 | NBP | 1.9 | 2.0 | 2.1 | |
43°C | 60 min | NBP | NBP | 2.6 | 2.3 | 2.5 |
120 min | 3.0 | NBP | 2.3 | 2.4 | 2.6 |
Abbreviations: NBP = No breakpoint calculated.
Intravesical cisplatin had been rarely studied after reports of a 14% anaphylaxis rate in a 1981 EORTC trial [39]. However, given its role as the mainstay of treatment for metastatic urothelial carcinoma, interest has recently rebounded in the form of pre-clinical and clinical trials [32, 40, 41]. Investigators are now using cisplatin as part of sequential multidrug intravesical regimens with MMC and doxorubicin or gemcitabine and cabazitaxel with promising initial results [32, 42–45]. As such, we report its solubility profiles under various physical states to aid in dose determination of future studies.
A major limitation of this work lies on the assumption that improvements in solubility with hyperthermia results in improved intravesical drug delivery and therein efficacy. However, while not yet studied in intravesical delivery, it is well understood in pharmacology that solubility is critical in oral drug bioavailability as gastrointestinal absorption is dependent on membrane permeability and concentration of drug in the aqueous form at the site of absorption [11, 46–48]. For this reason, solubility screens are performed early in the drug development process in order to identify viable candidates, as insoluble compounds are far less likely to achieve adequate bioavailability [11, 21, 49, 50]. While we believe the hypothesis that these improvements will translate into improved urothelial drug delivery, we plan to verify this with further studies in animal models.
A limitation of our experimental design is the requirement to remove microplates from their heated water baths for measurement. Due to these two minutes at room temperature, the actual solution temperatures were likely slightly lower than 37 and 43°C at the time of measurement, however we assume that all plates cooled a similar amount given consistent volumes.
As an in vitro analysis, this study is also limited in that it does not account for physiologic factors such as dilution and breakdown from urine constituents. The scope addresses physical stability and solubility but not the drugs chemical stability which may be impacted by extremes of heat and pH. Myers et al. noted that incubation at 50°C for 50 minutes leads to a 5–7% loss in MMC, so we anticipate >90% drug preservation after 2 hour at 43°C but this also warrants further investigation [14].
Overall, this study demonstrates and quantifies the improvements in solubility of three intravesical chemotherapies with adjunct intravesical hyperthermia. These results may be used to aid dosage determinations for future studies investigating whether the improvements in solubility translate to drug delivery.
ACKNOWLEDGMENTS
The authors have no acknowledgements.
FUNDING
DG is supported by the AUA Urology Care Foundation through a Resident Research Award (Award #629004). WT is supported by the Ruth L. Kirschstein NRSA Institutional Research Training Grant (T32 CA093245).
ETHICAL CONSIDERATIONS
As this is a test tube study not involving human subjects or tissues, informed consent and approval by an Institutional Review Board do not apply. Further, there were no cell lines used so there is no cell line identity to report.
CONFLICTS OF INTEREST
BI serves as a consultant/advisor for Ferring Pharmaceuticals, Combat Medical, and Taris Biomedical, and has participated in scientific studies/trials with FKD Therapies, Anchiano Therapeutics, Genentech Inc., Nucleix, Bristol-Meyers-Squibb, and Abbott Laboratories. The remaining authors have nothing to disclose.
AUTHOR CONTRIBUTIONS
Conception: DG, AS, WT, WE, IS, BI; Performance of work: DG, WT, WE; Interpretation or analysis of data: DG, AS, WT, WE, IS, BI; Writing the article: DG, AS, WT, WE, IS, BI.
SUPPLEMENTARY MATERIAL
[1] The appendix is available in the electronic version of this article: https://dx.doi.org/10.3233/BLC-200350.
REFERENCES
[1] | Douglass L , Schoenberg M . The Future of Intravesical Drug Delivery for Non-Muscle Invasive Bladder Cancer. Bladder Cancer 2: (3):285–92. |
[2] | Tan WS , Kelly JD . Intravesical device-assisted therapies for non-muscle-invasive bladder cancer. Nature Reviews Urology. (2018) ;15: (11):667. |
[3] | Cambier S , et al. EORTC Nomograms and Risk Groups for Predicting Recurrence, Progression, and Disease-specific and Overall Survival in Non–Muscle-invasive Stage Ta–T1 Urothelial Bladder Cancer Patients Treated with 1–3 Years of Maintenance Bacillus Calmette-Guérin. European Urology. (2016) ;69: (1):60–9. |
[4] | Lammers RJM , et al. The Role of a Combined Regimen With Intravesical Chemotherapy and Hyperthermia in the Management of Non-muscle-invasive Bladder Cancer: A Systematic Review. European Urology. (2011) ;60: (1):81–93. |
[5] | Sousa A , et al. A clinical trial of neoadjuvant hyperthermic intravesical chemotherapy (HIVEC) for treating intermediate and high-risk non-muscle invasive bladder cancer. International Journal of Hyperthermia.. (2014) ;30: (3):166–70. |
[6] | Arends TJH , et al. Results of a Randomised Controlled Trial Comparing Intravesical Chemohyperthermia with Mitomycin C Versus Bacillus Calmette-Guérin for Adjuvant Treatment of Patients with Intermediate- and High-risk Non–Muscle-invasive Bladder Cancer. European Urology. (2016) ;69: (6):1046–52. |
[7] | Hildebrandt B , et al. The cellular and molecular basis of hyperthermia. Critical Reviews in Oncology/Hematology. (2002) ;43: (1):33–56. |
[8] | Frey B , et al. Old and new facts about hyperthermia-induced modulations of the immune system. International Journal of Hyperthermia: The Official Journal of European Society for Hyperthermic Oncology. North American Hyperthermia Group. (2012) ;28: (6):528–42. |
[9] | Longo TA , et al. A systematic review of regional hyperthermia therapy in bladder cancer. International Journal of Hyperthermia. (2016) ;32: (4):381–9. |
[10] | van der Heijden AG , et al. Effect of hyperthermia on the cytotoxicity of 4 chemotherapeutic agents currently used for the treatment of transitional cell carcinoma of the bladder: an in vitro study. The Journal of Urology. (2005) ;173: (4):1375–80. |
[11] | Savjani KT , Gajjar AK , Savjani JK . Drug Solubility: Importance and Enhancement Techniques. ISRN Pharmaceutics. (2012) ;2012: . |
[12] | Dehring KA , et al. Automated robotic liquid handling/laser-based nephelometry system for high throughput measurement of kinetic aqueous solubility. Journal of Pharmaceutical and Biomedical Analysis.. (2004) ;36: (3):447–56. |
[13] | Martin AN , Sinko PJ , Singh Y . Martin’s physical pharmacy and pharmaceutical sciences: physical chemical and biopharmaceutical principles in the pharmaceutical sciences. 6th ed., 50th anniversary ed ed. 2011, Baltimore, MD: Lippincott Williams & Wilkins. 659. |
[14] | Myers AL , et al. Solubilization and Stability of Mitomycin C Solutions Prepared for Intravesical Administration. Drugs in R&D. (2017) ;17: (2):297–304. |
[15] | Beijnen JH , van Gijn R , Underberg WJ . Chemical stability of the antitumor drug mitomycin C in solutions for intravesical instillation. Journal of Parenteral Science and Technology: A Publication of the Parenteral Drug Association. (1990) ;44: (6):332–5. |
[16] | Owusu RA , Abern MR , Inman BA . Hyperthermia as Adjunct to Intravesical Chemotherapy for Bladder Cancer. BioMed Research International. (2013) ;2013: . |
[17] | Gontero P , et al. Pharmacokinetic study to optimize the intravesical administration of gemcitabine. BJU International. (2010) ;106: (11):1652–6. |
[18] | Bilobrov VM , Chugaj AV , Bessarabov VI . Urine pH variation dynamics in healthy individuals and stone formers. Urologia Internationalis. (1990) ;45: (6):326–31. |
[19] | Bergström CAS , Luthman K , Artursson P . Accuracy of calculated pH-dependent aqueous drug solubility. European Journal of Pharmaceutical Sciences.. (2004) ;22: (5):387–98. |
[20] | Green C , et al. AN 117- A fully automated kinetic solubility screen in 384-well plate format using nephelometry, in Application Notes. 2016, BMG Labtech: Ortenberg, Germany. pp. 271-2. 52 |
[21] | Bevan CD , Lloyd RS . A High-Throughput Screening Method for the Determination of Aqueous Drug Solubility Using Laser Nephelometry in Microtiter Plates. Analytical Chemistry. (2000) ;72: (8):1781–7. |
[22] | Fligge TA , Schuler A . Integration of a rapid automated solubility classification into early validation of hits obtained by high throughput screening. Journal of Pharmaceutical and Biomedical Analysis. (2006) ;42: (4):449–54. |
[23] | Peters C . AN 307 - Improving throughput for assessing nephelometric turbidity units (NTUs) using the NEPHELOstar® Plus, in Application Notes. 2017, BMG Labtech: Ortenberg, Germany. pp. 2. |
[24] | Sylvester RJ , Oosterlinck W , Witjes JA . The schedule and duration of intravesical chemotherapy in patients with non-muscle-invasive bladder cancer: a systematic review of the published results of randomized clinical trials. Eur Urol. (2008) ;53: (4):709–19. |
[25] | Au JL , et al. Methods to improve efficacy of intravesical mitomycin C: results of a randomized phase III trial. Journal of the National Cancer Institute. (2001) ;93: (8):597–604. |
[26] | Dalbagni G , et al. Phase I Trial of Intravesical Gemcitabine in Bacillus Calmette-Guérin–Refractory Transitional-Cell Carcinoma of the Bladder. Journal of Clinical Oncology. (2002) ;20: (15):3193–8. |
[27] | Dalbagni G , et al. Phase II Trial of Intravesical Gemcitabine in Bacille Calmette-Guérin–Refractory Transitional Cell Carcinoma of the Bladder. Journal of Clinical Oncology. (2006) ;24: (18):2729–34. |
[28] | De Berardinis E , et al. Intravesical administration of gemcitabine in superficial bladder cancer: a phase I study with pharmacodynamic evaluation. BJU international. (2004) ;93: (4):491–4. |
[29] | Laufer M , et al. Intravesical Gemcitabine Therapy for Superficial Transitional Cell Carcinoma of the Bladder: A Phase I and Pharmacokinetic Study. Journal of Clinical Oncology. (2003) ;21: (4):697–703. |
[30] | Messing EM , et al. Effect of Intravesical Instillation of Gemcitabine vs Saline Immediately Following Resection of Suspected Low-Grade Non–Muscle-Invasive Bladder Cancer on Tumor Recurrence: SWOG SRandomized Clinical Trial. JAMA. (2018) ;319: (18):1880–8. |
[31] | Shelley MD , et al. Intravesical gemcitabine therapy for non-muscle invasive bladder cancer (NMIBC): a systematic review. BJU international. (2012) ;109: (4):496–505. |
[32] | DeCastro GJ , et al. A phase I trial for the use of intravesical cabazitaxel, gemcitabine, and cisplatin (CGC) in the treatment of BCG-refractory nonmuscle invasive urothelial carcinoma of the bladder. Journal of Clinical Oncology. (2017) ;35: (6_suppl):313. |
[33] | Brand S , et al. Discovery and Optimization of 5-Amino-1,2,3-triazole-4-carboxamide Series against Trypanosoma cruzi. Journal of Medicinal Chemistry. (2017) ;60: (17):7284–99. |
[34] | Mus LM , et al. Vehicle development, pharmacokinetics and toxicity of the anti-invasive agent 4-fluoro-3’,4’,5’- trimethoxychalcone in rodents. PLoS ONE. (2018) ;13: (2). |
[35] | Hiltensperger G , et al. Quinolone Amides as Antitrypanosomal Lead Compounds with In Vivo Activity. Antimicrobial Agents and Chemotherapy. (2016) ;60: (8):4442–52. |
[36] | Geijsen ED , et al. Combining Mitomycin C and Regional 70 MHz Hyperthermia in Patients with Nonmuscle Invasive Bladder Cancer: A Pilot Study. The Journal of Urology. (2015) ;194: (5):1202–8. |
[37] | Witjes JA , Hendricksen K . Intravesical Pharmacotherapy for Non–Muscle-Invasive Bladder Cancer: A Critical Analysis of Currently Available Drugs, Treatment Schedules, and Long-Term Results. European Urology. (2008) ;53: (1):45–52. |
[38] | Cozzi PJ , et al. Toxicology and Pharmacokinetics of Intravesical Gemcitabine: A Preclinical Study in Dogs. pp. 10. |
[39] | Denis L . Anaphylactic reactions to repeated intravesical instillation with cisplatin. The Lancet. (1983) ;321: (8338):1378–9. |
[40] | Hadaschik BA , et al. Paclitaxel and cisplatin as intravesical agents against non-muscle-invasive bladder cancer. BJU International. (2008) ;101: (11):1347–55. |
[41] | Sasaki N , et al. Spatial and temporal profile of cisplatin delivery by ultrasound-assisted intravesical chemotherapy in a bladder cancer model. PLOS ONE. (2017) ;12: (11):e0188093. |
[42] | Kates M , et al. Preclinical Evaluation of Intravesical Cisplatin Nanoparticles for Non-Muscle-Invasive Bladder Cancer. Clinical Cancer Research: An Official Journal of the American Association for Cancer Research. (2017) ;23: (21):6592–601. |
[43] | Delto JC , et al. Preclinical analyses of intravesical chemotherapy for prevention of bladder cancer progression. Oncotarget. (2013) ;4: (2):269–76. |
[44] | Chen C-H , et al. A cocktail regimen of intravesical mitomycin-C, doxorubicin, and cisplatin (MDP) for non-muscle-invasive bladder cancer. Urologic Oncology: Seminars and Original Investigations. (2012) ;30: (4):421–7. |
[45] | DeCastro GJ , et al. A Phase 1 Trial of Intravesical Cabazitaxel, Gemcitabine, and Cisplatin for the Treatment of Non-muscle Invasive BCG Unresponsive or Recurrent/Relapsing Urothelial Carcinoma of the Bladder. J Urol. 2020:101097JU0000000000000919. |
[46] | Goodman LS , et al. Goodman & Gilman’s the Pharmacological Basis of Therapeutics. 11 ed. 2006: McGraw Hill. |
[47] | Vemula VR , Lagishetty V , Lingala S . International Journal of Pharmaceutical Sciences Review and Research Page 41 Available online at www.globalresearchonline.net. 2010. |
[48] | Bergström CAS . In silico Predictions of Drug Solubility and Permeability: Two Rate-limiting Barriers to Oral Drug Absorption. Basic & Clinical Pharmacology & Toxicology. (2005) ;96: (3):156–61. |
[49] | Lipinski CA , et al. Experimental and computational approaches to estimate solubility and permeability in drug discovery and development settings. Advanced Drug Delivery Reviews. (1997) ;23: (1):3–25. |
[50] | Bergström CAS , et al. Absorption Classification of Oral Drugs Based on Molecular Surface Properties. Journal of Medicinal Chemistry. (2003) ;46: (4):558–70. |