Feedback for the prevention and rehabilitation of work-related musculoskeletal disorders: A systematic review
Abstract
BACKGROUND:
Work-related musculoskeletal disorders (WRMSDs) remain a challenge despite research aimed at improving their prevention and treatment. Extrinsic feedback has been suggested for the prevention and rehabilitation of WRMSDs to improve sensorimotor control, and ultimately to reduce pain and disability. However, there are few systematic reviews on the effectiveness of extrinsic feedback for WRMSDs.
OBJECTIVE:
To perform a systematic review investigating the effect of extrinsic feedback for the prevention and rehabilitation of WRMSDs.
METHODS:
Five databases (CINAHL, Embase, Ergonomics Abstract, PsycInfo, PubMed) were searched. Studies of various designs assessing the effects of extrinsic feedback during work tasks on three outcomes (function, symptoms, sensorimotor control) in the context of prevention and rehabilitation of WRMSDs were included.
RESULTS:
Forty-nine studies were included, for a total sample of 3387 participants (including 925 injured) who performed work-related tasks in the workplace (27 studies) or in controlled environments (22 studies). The use of extrinsic feedback was shown to be effective in controlled environments for short-term prevention of functional limitations and sensorimotor alterations (very limited to moderate evidence) and for improving, in injured participants, function, symptoms and sensorimotor control (moderate evidence). In the workplace, it was shown to be effective for short-term prevention of functional limitations (limited evidence). There was conflicting evidence regarding its effect for WRMSD rehabilitation in the workplace.
CONCLUSION:
Extrinsic feedback is an interesting complementary tool for the prevention and rehabilitation of WRMSDs in controlled environments. More evidence is needed regarding its effect for the prevention and rehabilitation of WRMSDs in the workplace.
1Introduction
Despite research aimed at improving their prevention and treatment, work-related musculoskeletal disorders (WRMSDs) continue to cause significant economic, social and individual burdens [1]. WRMSDs are challenging due to the multifactorial risk factors associated with their development and persistence (e.g., physical work demands, poor posture, altered sensorimotor control, biopsychosocial context) [2]. Previous systematic reviews on WRMSDs have mainly focused on specific treatments against pain [3, 4] and stiffness [5], neglecting other impairments that could explain their high prevalence and rate of chronicity, such as sensorimotor disturbance.
Sensorimotor disturbance is a discordance between motor output and peripherical feedback [6]. It may modulate pain sensation [6] and movement control [7, 8] due to changes in the central nervous system [9, 10]. Outcomes related to sensorimotor disturbance may be important to consider, as recent publications have promoted sensorimotor rehabilitation for musculoskeletal disorders [11, 12]. Therefore, awareness of sensorimotor disturbances could be key for the prevention and rehabilitation of WRMSDs. The use of extrinsic feedback has been proposed as a good option to decrease sensorimotor disturbance, and thus improve movement control [13, 14].
Feedback is described as sensory afferent information resulting from the movement of our body in relation to the environment [14]. Intrinsic (or internal, or proprioception) feedback refers to the brain’s awareness of somatic information occurring during movement, while extrinsic (or augmented) feedback is defined as external information provided by a third party regarding movement outcomes or how the movement is performed [15]. Using extrinsic feedback can enhance intrinsic feedback and help people learn and improve their motor skills [13]. Extrinsic feedback (referred to as “feedback” for the rest of this manuscript) can be produced from various sources: health professionals (e.g., therapists providing manual or auditory feedback), “simple” interface (e.g., mirror, videos) or sensors (e.g., surface electromyography [sEMG], inertial measurement units [IMUs]). Feedback can be delivered using different sensory modalities such as audio (e.g., voice or alarm), visual, or tactile modalities individually or in combination. They can also be set at various frequencies: continuous, intermittent or faded, during or after a task [16, 17]. In addition, feedback can be self-controlled or imposed by a health professional [17]. Finally, feedback provided to the individual can relate either to the movement outcome (e.g., accuracy, time) at the end of the task (knowledge of results[KR]) or to movement characteristics (e.g., joint angle, muscle activation) during the task (knowledge of performance [KP]) [13].
Three previous systematic reviews explored the effect of feedback for the prevention or rehabilitation of WRMSDs, but these reviews only targeted the upper extremities. Two systematic reviews [18, 19] found conflicting effects of feedback for prevention, while one systematic review found no effect for workplace rehabilitation [20]. The interpretation of these results is limited due a lack of studies (total of six included studies for the three systematic reviews), the various outcomes evaluated, and their focus on the upper extremity only. Evaluating the effectiveness of feedback in prevention and treatment of WRMSDs with common comparison variables (function, symptoms, sensorimotor control) could lead to more optimal use of feedback by rehabilitation professionals.
The main objective of this systematic review was to determine whether feedback is effective in the prevention and rehabilitation of WRMSDs by evaluating its effects on function (e.g., work ability, disabilities), symptoms (e.g., discomfort, pain) and sensorimotor control (e.g., posture, muscle activation) in individuals with and without WRMSDs.
2Methods
This systematic review was conducted in accordance with the Preferred Reporting Items for Systematic Review and Meta-analysis (PRISMA) [21] statement and registered on PROSPERO (CRD42020188863).
The search strategy was developed in collaboration with a university librarian. Five bibliographic databases (CINAHL, Embase, Ergonomics Abstract, PsycInfo, PubMed) were searched from inception to August 2022 (2022/08/02). Specific search strategies were developed in OVID (for PubMed, PsycInfo), Embase and EBSCO (for CINAHL, Ergonomics Abstracts) using Medical Subject Headings (MeSH) and free text. Three concepts were exploited for the database search: (1) Population: workers with or without WRMSDs or healthy people performing work-related tasks, AND (2) Intervention: prevention or rehabilitation, AND (3) Modality: feedback. The screening and management of duplicates were performed using Covidence [22], an online software for systematic reviews.
All titles and abstracts were screened independently by two authors using the following inclusion criteria: 1) study design: cross-sectional studies, prospective studies, quasi-randomized controlled studies, cross-over studies, randomized controlled trials; 2) population: individuals with or without WRMSDs; 3) setting: work tasks performed in a work environment or in a controlled environment (e.g., laboratory, clinics, home); 4) intervention: any prevention or rehabilitation intervention using feedback; 5) outcome measures: function, symptoms and sensorimotor control.
Two reviewers (AF and MH or CP or EC) independently screened the titles and abstracts of all retrieved articles, then screened full-text articles. In the case of disagreements, a consensus was reached by discussion, or a third reviewer (JSR) was consulted if needed. Study data were extracted by the first reviewer (AF) and audited for accuracy by a second reviewer (MH or CP). A data extraction form was created, and the data extracted included study design, intervention, characteristics of feedback studied, location of MSK injury (if any), clinical stage (prevention, acute, chronic), research setting, work activity level (sedentary, physical, mixed [23]), outcome measures, and results.
Two reviewers (AF and MH or CP) independently assessed the risk of bias and the quality of evidence using the Downs and Black checklist [24] and a consensus was reached for the final score. Any disagreements were resolved through discussion. The pre-consensus inter-rater agreement between reviewers for total scores was calculated using Gwet’s gamma coefficient [25] and the intraclass correlation coefficient (ICC). The Downs and Black checklist evaluates risk of bias using a 27-item scale in three domains: reporting, external validity, and internal validity. It has good inter-rater reliability (ICC 0.73 to 0.94) [26, 27]. The maximal score that corresponds with a minimal risk of bias is 28. Articles with scores >20 are considered strong, 14–20 moderate, 7–13 limited and <7 poor [28].
Two reviewers (AF and MH or CP) used the GRADE (Grading of Recommendations, Assessment, Development and Evaluations) qualitative approach [29] to determine the overall quality of evidence (Table 3) [30, 31]. Four domains were used to characterize the evidence of each outcome: 1) number of studies/participants (imprecision); 2) methodological quality (risk of bias); 3) methodological and outcome similarities (indirectness); and 4) direction of results (inconsistency) [32]. Quality of evidence is rated using a five-level classification system. Strong evidence was characterized by multiple high-quality studies with consistent results; moderate evidence was characterized by multiple studies including at least one high quality study or multiple moderate or low-quality studies presenting consistent results; limited evidence was characterized by multiple moderate quality or low-quality studies with inconsistent results, or one high quality study; very limited evidence was characterized by only one low or moderate quality study; conflicting evidence was characterized by multiple studies providing inconsistent results, regardless of the methodological quality.
Narrative reporting was used to synthesize results (see Table 1). Table 2 presents description of the various scales used as outcome measures in the included studies.
Table 1
Synthesis of the articles included
Author, year | Study design (group number) | Downs and Black grids | Population (n, occupation, gender, mean age). | Body zone aimed. Injury stage. | Intervention: place, task, frequency, intervention / control groups, duration. | Frequency, type, source, delivery (knowledge of results [KR] or performance [KP]) of feedback | Measuring period: during, just after (T0), at short, middle, or long-term. | Outcome measures | Reported effects |
PREVENTION | |||||||||
Agruss et al., 2004 | Cross-sectional laboratory study (3 groups) | 14 | n = 28, college students, 64% women, 20 to 33 years. | Low back. | In laboratory, lifting plastic crate with additional weight, until 3400 N of lumbar compression. 1 control and 2 intervention groups (1/week, 2 week). | 1 group: faded verbal feedback on lumbar acceleration index based on 3D motion capture (KP). 1 goup: faded audio feedback based on errector spinae sEMG (KP). | Short (day 7) | Lumbar compression (acceleration index) with 3D motion capture analysis and errector spinae sEMG. | All groups improved: 183 N for the control group, 380 N for the verbal feedback group, and 261 N for the sEMG feedback group. The verbal acceleration group reduced the peak lumbosacral forces more than the control group (post-hoc test, p < 0.01). The sEMG feedback group did not differ notably from the control group (p > 0,2). |
Alavosius et al., 1986 | Prospective cohort study | 10 | n = 6, physical providers, gender not specified, 20–43 years. | Low back. | In a residential school for the mentally retarded, two client transfer techniques. Feedback 1/week, after 5 week, feedback 1/2week until safe transfers. | Verbal and visual final faded feedback based on checklist (KR). | Short, middle and long (weeks 1-2, months 1-2-7) | Observational recording of percentage of safely performance of two transfer tasks: stand pivot or total lift. | Performance variable during baseline. Following initial feedback, the variability in technique persisted (total lift 38 % and stand pivot 33% unsafely). With additional feedback, safety further improved (total lift 17%, stand pivot 5% unsafely). |
Alavosius et al., 1990 | Prospective cohort study | 15 | n = 4, physical providers, 100% women, 28–38 years. | Low back. | In a medical service unit of residential facility for mentally retarded, transfer technics, positioning and feeding. 5 months of weekly visit, after 2 months biweekly and monthly visit, until participant completed 90% of the criteria correctly. | Three schedules of feedback: continuous (many times, each day) or intermittent verbal and visual (written) (1/week, 3 consecutive performance) feedback (KR) or no feedback. | Short, middle (weeks 1-2-3, months 3-4) | Observational recording of percentage of safely performance of three health car routines (feeding, positioning and transferring). | Baseline: 63.5% ±11.5% of the tasks performed correctly. All responses treated with feedback improved (93.8% ±1.4%), although continuous feedback delivery changed much faster the percentage of safely performance than intermittent feedback. At Month 3-4, performance tended to be maintained at relatively high level (91.8% ±5.4%). |
Bazazan et al., 2019 | RCT (2 groups) | 19 | n = 188, computer user, 0% women, 33±5 years. | Shoulder, upper back, neck, low back. | In control room operator, seated posture monitoring. 1 control group, 12 weeks; 2/days, 30 min. | Intermittent audio (alarm) or tactile (vibration) feedback on incorrect shoulder and trunk position based on IMU (KP). | Long (months 6 and 12) | Rapid Upper Limb Assessment (RULA). Musculoskeltal Symptoms (Nordic Musculoskeletal Questionnaire). | After the intervention, RULA score for the case group was decreased (p < 0,01), and there were differences in RULA scores of the 2 groups (p < 0,05). The prevalence of the symptoms (p < 0,05) decreased after the intervention, with a difference between the 2 groups (p < 0,05). |
Blasch et al., 2013 | Pseudo randomised trial (4 groups) | 16 | n = 93, office workers, 60% women, 40±8,8 years. | Neck, shoulder, arms, hands, low back and leg. | Feedback in workplace and assessment in a test room. 1 waiting list (control group) and 3 groups with a choice of exercise: nordic walking (NW), or balance exercise on a wobble board (BAL), or feedback group (BFB), 8 weeks. | Visual and continuous feedback (based on skin conductance level and finger temperature) assited relaxation and stretching (KP). | T0 and middle (months 2 and 3) | Musculoskeletal complaints and pain (Freiburger Beschwerdenliste, FBL). Upper trapezius neuromuscular activity (sEMG). | Any interventions had an effect on sEMG-activity. BAL did not show any effects. NW was associated with an improvement (p < 0.05) of the frequency of neck, shoulder and arm pain, respectively. BFB, was associated with a decrease (p < 0.05) of the frequency of musculoskeletal tension and low back pain at T0. |
Boocock et al., 2019 | Cross-sectional laboratory study (2 groups) | 17 | n = 36, students, gender not specified, 25,7±4,6 years. | Low back. | In laboratory, lift a 13 kg box at 10 lifts per minute for up to 20 min. 1 control group. | Audio intermittent feedback on 80% threshold lumbar flexion based IMU fixed on lumbar spine (KP). | T0 | Movement and posture (IMU, 3D motion capture analysis and ground force reaction plate). | Feedback group adopted less peak lumbosacral flexion (p < 0,05) than the control group. This was accompanied by increased peak hip (p < 0,001) and knee (p < 0,008) joint angular velocities in the feedback group. Lower limb moments did not significantly differ between groups. |
Brandeburg et al., 2005 | Cross-sectional laboratory study (2 groups) | 11 | n = 16, students, 50% women, 19–53 years. | Neck. | In laboratory, screw driving task that stress on the cervicobrachial region, 2 days, 90 min each session. | Ergonomic information, continous visual (miror) feedback (KP) with or without intermittent verbal positive reinforcement (KR). | T0 | Discomfort survey (scale 0–10 for each body region). | The use of a mirror reduced the discomfort level (p < 0,05). No significant effect on the discomfort with the positive verbal reinforcement. |
Chan et al., 2021 | Cross-sectional laboratory study (2 groups) | 19 | n = 26, occupation not specified (any paradmedic training), 10 female, 23,2±2,95 years. | Low back. | In laboratory, 50 lifting tasks (box, medication bag, paramedic backboard) with instruction (visual and oral) before the tasks with or without feedback. | Intermittent tactile feedback and faded terminal verbal feedback based on 3D motion capture(spine flexion angle) (KP). | T0 and short (1week) | Sagittal spine motion (absolute/relative peak flexion, peak velocity). | In both groups, reductions on spine motion at T0 and short time, with a greater reduction with the augmented feedback in 5/12 conditions (p < 0,05). |
De Kraker et al., 2008 | RCT (2 groups) | 11 | n = 91, call center employees, 74% women, 38±9,8 years. | Neck and upper extremity. | In workplace, computer task with regular or feedback computer mouse, 2 weeks. | Intermittent tactile mouse feedback (when 10 sec without using to put the hand on the table) (KR). | T0 | Hovering behaviour (The Observer, V5, Noldus Information Technology, Wageningen, the Netherlands). Discomfort (Lokaal Ervaren Ongemak). | The discomfort did not show any significant differences between the groups. The rate of the occurrences “hand above the mouse” increased in the intervention group (p < 0,03) and did not change in the control group (p < 0,08). The rate of occurrences “hand next to the mouse” did not change in the intervention group (p < 0,2) or in the control group. |
Faucett et al., 2002 | RCT (3 groups) | 16 | n = 79, electronics manufacturer (sedentary tasks), 62% women, 39±9 years. | Upper extremity. | In workplace, two intervention groups: feedback and muscle learning therapy, or learning and cognitive behavioral techniques. 1 group control (work station ergnomics guideline). 6 weeks. | Visual continuous feedback based on bilateral upper trapezius and forearm/hand muscle sEMG (KP) associated with muscle learning therapy. | T0, short-middle and long term | Muscle Tension, Symptoms diaries (Visual Analog Scale and body chart) Occurrence of upper extremity injuries | In feedback group, reduced muscle tension in the trapezius areas at T0 and at 32 weeks, and partially for the forearm areas (p < 0,05). For the symptom, group differences (intervention versus control) only at Week 6 (p < 0,05). Any statistically difference in the occurrence of injuries in the 3 groups. |
Ferrone et al., 2021 | Prospective cohort study | 11 | n = 9 females, occupation not specified (from university campus), 26,0±11,2 years. | Low back. | In laboratory, three common nurse activities: patient transfer, boosting in bed, reaching tasks (each task3*3repation with 1 min brake). Four parts: familiarization, baseline, feedback, “retention". | Intermittent haptic feedback based on two IMUs at L4 and T5 spine levels (KP). | During and T0 | Posture (maximum peak of lateral bending, forward/backward or twisting angle based on IMUS) | During feedback session (vs baseline) improvement in average percentage of peak angle for forward/backward 48,3% ±52%, twisting angle 37,1% ±21,2% but not lateral bending 58,9% ±45,7%. During “retention” session: improvement in average percentage of peak angle for forward/backward 11,1% ±6,0%, twisting angle 17,0% ±9,8% but not lateral bending – 26,3% ±11,3% (p < 0,05). |
Gaffney et al., 2016 | Cross-sectional laboratory study (2 groups) | 12 | n = 20, computer users, 50% women, 36,5±13,9 years (men) and 30,4±9,9 years (women). | Neck and shoulder. | In laboratory, postural correction during typing, 15 min. | Continous verbal feeback (postural coaching)±continous visual feedback (activity ratio between sEMG trapezius activations) (KP). | During intervention | High density sEMG distribution on upper, middle and lower trapezius. | Trapezius muscle activity was located 12,74±3,73 mm more inferior, the scapula was 2,58±118 mm more adducted and 0.23±0.24 mm more depressed in comparison to verbal postural coaching alone (p < 0,05). |
Gerard et al., 2002 | Prospective cohort study (3 groups) | 14 | n = 12, typists, gender not specified, 35±9 years. | Upper extremity. | Typing, in laboratory. 1 control group. 2 days, 2 trials /days. | Auditory intermittent feedback based on peak sEMG activity (flexor digitorum superficialis, extensor digitorum communis) or peak typing force (KP). | T0, and short (weeks 1-2) | Subjective rating of discomfort (Visual Analog Scale). Typing force (keyboard force monitor), finger flexor/extensor force (% sEMG MVC), muscle fatigue (mean frequency). | Feedback with various source induced a 10–20% reduction in 90th percentile typing force, finger flexor EMG, and finger extensor EMG. Fatigue: no significant changes. After 1 week no differences in typing force or sEMG activity in 3 groups. Any significant differences in discomfort between the different test conditions (p < 0,05). |
Henning et al., 1996 | Cross-section laboratory study (2 groups) | 11 | n = 31, undergraduate typists, gender and age not specified. | Specific anatomical location not specified. | In laboratory, typists entered lines of randomized word and self-managed their rest breaks under standard (typing single lines of words) or cognitive conditions (reverse-typed one word in each data line tasks). | Visual and continuous single or double feedback: 1 group could only see the time bar display, 1 group see the time bar display and a rest breaks bar display (KR). | T0 | Musculoskeletal discomfort (5 body chart, 5-point scale) | No significant differences on musculoskeletal discomfort. During cognitive demanded condition, less back discomfort in the feedback (M = 1,64) than in the control (M = 2,43) condition (F(2,27) = 4.86, p < 0,05). |
Holtermann et al., 2008 | RCT (3 groups) | 14 | n = 164, computer user gender and age not specified. | Neck and Shoulder. | In workplace. 2 groups with feedback and 1 group control with computer mouse more than 50% of time. All groups received ergonomic information and adjustments. (5 sessions, 1 weekly, 20 min) | Two groups working with computer mouse more than 50% (BF50) and less than 25% (BF25) of their work time. Audiovisual continuous feedback based on dominant trapezius sEMG (KP). | T0 and short (week 1) | Bilateral sEMG activity (amplitude, frequency of gaps). | A decrease in the sEMG amplitude of both trapezius in the 3 groups at T0 (p < 0,05). Differences between the intervention and control group (p < 0,05) but not between the intervention groups (p < 0,58). Changes in frequency of gaps after the intervention significantly different between the interventions and control group only in the dominant trapezius muscle. |
Kamachi et al., 2021 | Cross-sectional Laboratory Study (2 groups) | 15 | n = 20, novice caregivers, 10 women, 18–40 years. | Low back. | Training in simulated home environment: educational video and care tasks with or without feedback (4 set, 1 h, 2 days). | Conitnous (day 1) and intermittent (day 2) auditory feedback based on IMUs at T10 and sacrum spinal levels (KP). | T0, short (two weeks, middle (two months) | Lumbar spine flexion. | The intervention group have a decreased end-range (80th and 95th percentile) spine flexion compared to controls after intervention, at short and middle term (p < 0,05). |
Kernozek et al., 2006 | Prospective cohort study (2 groups) | 15 | n = 22, warehouse employers, 0% women, age not specified. | Low back. | In warehouse, repetitive (6) material-handling jobs under two different conditions (high-to-low and low-to-high) with different cases (13 to 18Kg). 1 control group. (6 weeks) | Continuous auditory feedback (based on electromagnetic tracking system, and coaching) (15 min, weeks 2 and 4) (KP). | Short (2 weeks) | Side-bending, flexion and rotation moments (electromagnetic tracking system). | Significant group-time interactions for the side-bending moment (p < 0,05) and the flexion moment (p < 0.05), but not the rotation moment (p > 0,05). The flexion and side-bending moments decreased by 43% and 70%, respectively, in the experimental group, versus 1% and 10%, respectively in the control group. |
King et al., 2013 | RCT Pilot | 16 | n = 23, office workers in computer intensive environment, gender and age not specified. | Neck and upper extremity. | In the workplace, assess relative computer mouse use with or without feedback. (25 weeks) | Tactile intermittent feedback (vibrated mouse) if the mouse is handled more than 12 sec (KR). | During intervention and at T0 | Posture (relative mouse using). Pain and discomfort (daily symptom survey) | At T0 less use in the control group while the feedback group came back to baseline (p < 0,05). Significant less shoulder pain and discomfort in the intervention group compared to control at T0 (38,7% less, p < 0.05). Previous instances of shoulder pain during intervention and T0 explained only 46% of the variance in shoulder pain at T0. |
Lind et al., 2020 | Cross-sectional laboratory study | 15 | n = 16, population of university, 56% women, 25±8 years. | Neck and upper extremity. | In laboratory, 7 simulated sessions of 30 mail sorting tasks under two conditions (predetermined or self-controlled) with verbal ergonomics instruction with or without haptic feedback. | Tactile (vibration) intermittent or terminal feedback based on IMU fixed T1-T2 and shoulder (KP). | T0 (after each session) | Discomfort/pain (Borg CR 10, body map). Posture (3 IMUs on T1-T2 and shoulder) | The discomfort results (shoulder n = 2, elbow n = 1, upper/lower back n = 2) were not significant. Less time in upper arm elevation (30–40–60°) and percentile upper arm elevation angle with verbal instruction and feedback (50–90–95th) (p≤0,005). |
Levanon et al., 2013. | RCT (3 groups) | 20 | n = 66, office workers, 65% women, 37,33±9,35 years. | Upper extremity. Prevention, acute, chronic. | Intervention in workplace and assessment in laboratory, typing task (five times, about one minute each). Ergonomics intervention (posture adjustments, muscle activity training) with or without feedback, and a control group. (10 weeks) | Auditory intermittent feedback (ergonomics intervention), or continuous auditory feedback (based to wrist extensor and upper trapezius sEMG0, both to relax muscle activity (KP). | Short (2 weeks) | Rapid Upper Limb Assessment. Pain (Standard Nordic Questionnaire). Upper extremity kinematics (3D motion capture system) and sEMG. | Only the ergonomics group alone reduced the mean angular velocity compared to the control group (25,12, p = 0,003). No significant differences in the muscle activity index between the three groups. Lower post-intervention RULA in the intervention groups (p = 0,000). Standard Nordic Questionnaire scores after intervention: increase in the control group (not significantly) and decreased in the intervention groups (p = 0,001). |
Lorenz et al., 2002 | Prospective cohort study | 16 | n = 955, material handling workers, 5% women, 33±9,3 years. | Low back. | In workplace, lifting case (3 to 36 Kg), 5 training sessions with a coach (30 minutes each session, 0–1–3–6–9 months period). | Verbal by coach intermittent and final feedback based on a magnetic motion measurement system (KP). | T0 | Spinal compression at L5/S1 (peak forward bending, twisting, and side bending based on magnetic measurement system) | Mean reductions in forward bending ranged from 5 to 11%, twisting reductions ranged from 7 to 13%, and the range of side bending reduction was 10 to 22%. The largest absolute reductions or largest percentage-wise reductions occurred in same session for forward (session 4) and side bending (session 1) while it occurred in various session (1 or 4) for the twisting (p < 0,05). |
Oppici et al., 2021 | Cross-sectional laboratory pilot study | 15 | n = 20, students, 7 female, 30±6 years. | Low back. | In laboratory, repetitive lifting tasks (30, 10 lifts/min) with or without feedback, followed by retention repetitions after 5 min of rest. | Continuous tactile (Leukotape applied on extensor muscle from T12 to S1 spinal levels) or intermittent auditory feedback of sacro-lumbar peak flexion angle (KP). | During and at T0. | Sacro-lumbar peak flexion angle (3D motion capture system). | During acquisition, sacro-lumbar angle in control condition>auditory (p < 0,01)>tactile(p < 0,01). In learning condition, control >tactile (p < 0,01)>auditory (p = 0,048). |
Owlia et al., 2020 | Cross-sectional laboratory Study (2 groups) | 16 | n = 20, novice care givers, control group 60% women, 24,7±2,7 years, intervention group 40% women, 28,1±–6,4 years. | Low back. | In a home simulated environment, 11 simulated care activities like patient handling tasks. 1 control group, 1 training group with feedback and video training. (8 times, 2 days) | Continuous audio feedback with a threshold at a spine flexion angle >70° based on IMUs (KP). | T0 | Spine flexion (IMUs) | Training group with reductions in end-range lumbar spine flexion during the simulated patient handling tasks at T0 compared to their baseline trials (p = 0,002) while there was no change for the control group. |
Partido et al., 2019 | RCT (2 groups) | 15 | n = 30, dental educators, gender and mean age not specified. | Whole body. | In workplace, feedback and self-assessment to improve dental educators’ posture (3 weeks). | Final (each week) and visual feedback based on ergonomic self-assessment with or without visual and verbal final feedback by the principal investigator based on photography (KR). | T0 | Posture (photography and Modified Dental Operator Posture Assessment Instrument) | Significant differences in ergonomic scores between the self-assessment groups and self-assessment plus photography groups at week 4 (F(1) = 6,295, p < 0.01) but not at week 1 (F(1) = 0,012, p > 0,05). |
Pinto et al., 2018 | Cross-sectional laboratory study | 13 | n = 11, occupation not specified, 36% women, 23±11 years. | Low back. | In laboratory, a repeated lifting task (3Kg) under four conditions: baseline, tape, tape plus instruction, retention without tape and instruction. 5 min of rest between each condition. | Tactile continuous feedback based on Leukotape (applied to extensor muscle from T12 to S1 spinal levels) and then verbal intermittent feedback to pay attention to it (KP). | During intervention and short (5 min) | Movement (peak lumbar flexion angle, peak hip flexion angle, peak knee flexion angle based on electromagnetic sensors) | Positive effects on the three dependent variables (all p < 0,0001): peak lumbar spine flexion angle decreased, peak hip flexion angle increased, and peak knee flexion increased compared to the baseline. |
Ribeiro et al., 2020 | RCT (2 groups) | 21 | n = 130, health care workers (physical), 84,6% women; 45,3 years. | Low back. All clinical stage. | During working hours, feedback group or sham group to modify postural behavior. (4 weeks). | Intermittent auditory postural feedback based on IMU measuring lombopelvic forward bending (KP). | Short (1 week), middle (1–3 months) and long (6–12 months) | Postural behaviour (number of times with postural threshold exceeded). | There were no within-group changes on the number of times postural threshold exceeded at 1-week follow-up, and no differences between shame group and feedback group. At 12-month follow-up, no significant changes were observed for the both groups. |
Ribeiro et al., 2014 | RCT feasibility (3 groups) | 20 | n = 62, health care organisation (72% physical and 28% sedentary), 92% women, 49±12 years. | Low back. All clinical stage. | During daily work-related activities, feedback device (2 types) for modifying postural behavior. 1 group control. (6 weeks) | Intermittent audio feedback (1week on /1week off) or continuous audio feedback based on IMU, number of cumulative postural behavior (4 weeks, KR). | Short (1 week) | Postural pattern change (frequency, accelerometer). | No significant differences were found for postural pattern between baseline and the follow-up period for the control group and intermittent feedback group. The post-hoc test revealed no statistically significant differences between the groups. |
Roossien et al., 2017 | Prospective cohort study | 15 | n = 49, office workers, 58% women, 43±11 years. | Thoracic and lumbar and hip. | In workplace, measuring sitting behavior with a “smart” office chair (vibration). 12 weeks. | Intermittent tactile feedback based on pressure sensors fixed on the chair when prolonged period in unfavourable sitting posture (6 weeks, KP). | T0 | Posture (pressure sensors and activity tracker). Pain (Local Musculoskeltal Discomfort). | Between the study phases small changes in mean sitting duration, posture and discomfort. After turning off the feedback signal, a slight increase in sitting duration (10 min, p = 0,04), a slight decrease in optimally supported posture (2,8%, p < 0,01), and musculoskeletal discomfort (0,8, p < 0,01). |
Sharma et al., 2014 | RCT (2 groups) | 14 | n = 166, army recruits, gender and mean age not specified. | Lower extremity. | In laboratory and during usual military training regimen. One intervention group to increase neuro muscular control and flexibility against tibial stress syndrom. 1 control group. 26 weeks. | Faded final visual and verbal feedback based on plantar pressure system and delayed intermittent verbal feedback by physiotherapist during the exercises (3/week, KP). | T0 | Incidence of medial tibial stress syndrome. Foot balance and time to reach peak heel rotation (treadmill). | Moderate effect of the intervention on foot balance (–14 N*cm-2) and on time to reach peak heel rotation (2,8%). The feedback was associated with a reduced instantaneous relative risk of medial tibial stress syndrome (adjusted HR of 0,25, p = 0,05). The number needed to treat to observe one additional injury-free recruit in intervention versus control at 20 weeks was 14 (11 to 23) participants. |
Thanathornwong et al., 2015 | Cross-over study | 12 | n = 16, dental students, 50% women, 21–23 years. | Low back, neck and shoulder. | Dental student posture during scaling. Two randomised sequence interventions: feedback or control. Study duration not specified. | Final vibration feedback based on accelerometer on upper back (KP). | T0 | Tilt angle of back (accelerometer) and upper trapezius activity (sEMG). | Dental students with feedback had lower tilt angles (X and Y) at 10th, 50th, 90th percentile of back, and a muscular load different (p < 0,05) from those without feedback. A significant improvement of 3,62–8,47° for forward movements, and 6,12–8,88° for sideways movements. |
Thomas et al., 2015 | Prospective cohort study (2 groups) | 13 | n = 10, line workers, 100% women, 39,3 years. | Wrist. | Lightweight hardware assembly on a moving conveyor belt (sedentary task). 1 control group. 8 weeks. | Intermittent audio feedback based on flexor/extensor forearm sEMG (1 h/week, KP) | T0 | Subjective discomfort (body chart, 0–10 scale). Grip strength (dynamometer), nervous conductance velocity (electroneurometer). | No significant differences (in time and between groups) in strength (p > 0,01), motor nerve conduction (p > 0,5), and body comfort (p > 0,5). |
Vedsted et al., 2011 | Cross-sectional laboratory study | 10 | n = 11, computer worker, 100% women, 40,4±9,3 years. | Neck and upper extremity. | In laboratory, standardised computer work for 3 min in different conditions different working condition (17): time constrain/no constrain, feedback locations, type and modes. | Intermittent audio or visual feedback based on right trapezius or extensor digitorum communis sEMG or right trapezius mechachanomyography by Inertial Measurement Unit (KP). | During | sEMG activity (both trapezius and right wrist) | During feedback, only the extensor digitorum communis activity (p = 0,003) was reduced compared to the control, no significant effect if the size were different. During the time constraint condition, there was a significant reduction only in the extensor digitorum communis activity (p = 0,002). Upper trapezius activity measurement did not significantly changed in all conditions. |
Vignais et al., 2013 | Cross-sectional laboratory study (2 groups) | 13 | n = 12, 0% women, students, 22,5±2,5 years. | Neck and upper body. | In living lab, manual industrial manufacturing tasks: turning two hand levers by 90°, removing four fuses, unscrewing screws of a transducer and putting it down (4.4 lbs max and 4 min). 1 control group. | Intermittent audio (global scores) and visual feedback (local scores) based on RULA and 7 IMUs placed on upper extremity, sternum, and spine (KR). | T0 | RULA | Feedback group have a lower global RULA score (3,95±0,83) than the control group (4,35±0,54, p < 0,05). The right and the left RULA scores separately were higher for the control group, but not significantly different. Differences between groups for percentage of time spent in each RULA range 3–4 and 5–6 (p < 0,05). Subjects’ articulations and segments of the control group have significantly higher RULA local score (p < 0,05). |
Yoo et al., 2006 | Cross-sectional study | 17 | n = 20, visual display terminal worker, 0% women, 23,2±2,4 years | Head, shoulder, trunk. | During visual display terminal work, two randomised sequence interventions (15 min) with a proximity-sensing chair to improve spine posture. | Intermittent auditory feedback (posture alarm), based on proximity sensors on T10 and T12 (KP). | During intervention | Posture (3D motion capture analysis system) | The forward head (6,86°vs 10,72°), forward shoulder (8,38° vs 15,83°), and trunk flexion (9,46° vs 18,32°) were significantly lower when using the feedback than without (p < 0,05). |
REHABILITATION | |||||||||
Author, year | Study design (group number) | Downs and Black grids | Population (n, occupation, gender, mean age) | Anatomical location addressed Clinical stage (acute, chronic) | Intervention (place, task, frequency, intervention groups without feedback, control group) | Types of feedback in intervention group(s) | Measurement timeline: Baseline (B), immediately after intervention (T0), and after intervention (short, middle, long term) | Outcome measures (parameters/function/pain) | Reported effects |
Alghadir et al., 2021 | Cross-sectional study | 18 | n = 50, teachers,44 females, 36,48±2,41 years. | Neck. Stage not specified. | In controlled environment, deep cervical flexor muscle training in addition to conventional exercise (stretching and strengthening). Control group with conventional exercise only. (4 weeks). | Continuous, visual feedback of craniocervical flexion pressure based on a unit of pressure biofeedback (KP). | T0 | Pain (NPRS), cranio-vertebral angle (digital photograph technique). | For both intervention groups (feedback vs control), pain (–2,18±1,35 vs –2,11±1,25) and cranio-vertebral angle (3,1°±3,86° vs 1,70°±3,90°) means improved, but more in the group using feedback (p < 0,05). |
Dellve et al., 2011 | RCT (3 groups) | 17 | n = 61, service organization workers (mixed physically demanding jobs), 100% women, 35–60 years. | Neck. Chronic. | In home environment, feedback training or short intensive muscular strenght training (5–10 min 2/days, 6 days/week for 1 month). 1 control group. | Final verbal feedback by physiotherapist 1/week, based on upper trapezius sEMG recordings, minimum 8 h a week (KP). | T0, short (month 1) and middle (month 3) | Work ability. Numeric pain scale (Von Korff). | For both intervention groups, pain was lowered over time compared with the control group (p = 0,0481). Muscular strength training was associated with increased self-rated work ability and feedback with increased work ability (p < 0,05). |
Dos Santos et al., 2021 | Prospective cohort study | 17 | n = 183 (RCS group n = 117, ASI group n = 66), occupation not specified, 48 female (RCS group) and 37 female (ASI group), 41,1±12,2 years (RCS group) and 26,7±10,3 years (ASI group). | Shoulder: rotator cuff related pain syndrom [RCS] and anterior shoulder instability [ASI]. Acute to chronic. | In laboratory, scapular-focused exercise and feedback (4weeks). | Intermittent visual feedback based on sEMG amplitude 3SD above the baseline of lower trapezieus, serratus anterior, anterior deltoid (KP). | T0, short (4 weeks) and long term (2 years). | Glenohumeral range of motion (goniometer), flexor and abductor strength (isometric manual testing), neuromuscular activity and control (% of MVC, observation of scapular dyskinesis), function (SPADI, DASH), pain (NPRS). | After the intervention all outcomes improved compared to the baseline (p < 0,05). No differences between RCS-ASI groups at T0 and long-term for DASH 1st part and SPADI (p < 0,05) and NPRS. 15,8% of recurrence. |
Golebowicz et al., 2015 | Prospective cohort study | 13 | n = 12, computer operator, 50% women, 34,25±8,8 years | Upper extremity. Acute or chronic. | In workplace, feedback to relax to the sEMG amplitude and ergonomics intervention. (2-3 min min twice a week, 4 to 6 weeks). | Verbal and final (by telerehabilitation) feedback based on wrist, finger extensor, upper trapezius activity sEMG (KP). | Short (weeks 4–6) | Rapid Upper Limb Assessment; Symptoms (Standard Nordic Questionnaire) | There was a significant difference between pre (6,66/7) and post-intervention (4/7) Rapid Upper Limb Score (p = 0,003, Z = –296). A significant reduction in the number of upper extremity symptoms was reported after intervention (p = 0,003, Z = –3,00). |
Iqbal et al., 2021 | RCT (2 groups) | 18 | n = 65, teacher,50% women. experimental group: 36,33±6, control group 36,45±5,95 years. | Neck. Chronic. | In controlled environment, deep cervical flexor muscle training (5 days/week) in addition to conventional exercise (stretching and strengthening, 4 days/week). Control group with conventional exercise only. | Continuous, visual feedback of craniocervical flexion pressure based on a unit of pressure biofeedback (KP). | During and T0 | Disability (Neck Disability Index). Pain (Numeric Pain Rating Scale). Muscle endurance (pressure feedback). | After initiating the intervention, although there were improvements in both groups, there was improvement in muscle endurance (5,26 vs 2,83 mmHg), pain (2,00 vs 0,9 points), and disability (6,77 versus 2,78 points) in subjects who received additional training with pressure biofeedback (p < 0,05). |
Iqbal et al., 2013 | RCT (2 groups) | 17 | n = 30, secondary teacher school, 80% women, 25–45 years. | Neck. Chronic and acute. | In school medical room, deep cervical flexor muscle training in addition to conventional exercise (stretching and strengthening, 4 days/week). Control group with conventional exercises only. | Continuous, visual feedback of craniocervical flexion pressure based on a unit of pressure biofeedback (KP). | Short (2 weeks) | Function (Neck Disability Index). Pain (numeric pain rating scale) | The mean reduction in NDI scores in feedback group was 6,715±0,67 at p = 0,000, and the mean reduction in control group was 2,207±0,1059 at p = 0,000, with significant difference between the groups (p < 0,05). In the comparison of pain, the values showed improvements in both groups (p < 0,05). The mean reduction in NPRS scores in feedback group was 3,137±0,41 and in control group was 1,797±0,1514, with difference between the groups (p < 0,05). |
Kuo et al., 2019 | Cross-sectional laboratory study | 17 | n = 21, office workers, 62% women, 23,8±3,5 years. | Neck. Chronic. | In laboratory, computer typing tasks (copy and type an electronic book, 2*1hours) with and without feedback. | Tactile (vibration) intermittent feedback (based on IMU) from wrong posture position (KP). | T0 | Self-reported neck and shoulder pain (Numeric rating scale). Spinal posture (3D motion capture system), muscle activity (cervical erector spinae, upper trapezius, and thoracic erector spinae). | Neck flexion (33°, 95%), upper cervical (3,3°, 95%), and lower thoracic (1,6°, 95%) angle were smaller with biofeedback than without (p < 0,05). For the sEMG, participants under the feedback condition exhibited lower muscle activity of the right (24,9%, 95%) and left (24,6%, 95%) cervical erector spinae (p < 0,05). Neck pain increased from 1,5 to 3,1 at the end (p < 0,001), and shoulder pain scores increased from 1,8 to 3,7 at the end (p < 0,001). |
Levanon et al., 2013. | RCT (3 groups) | 20 | n = 66, office workers, 65% women, 37,33±9,35 years. | Upper extremity. Prevention, acute, chronic. | Intervention in workplace and assessment in laboratory, typing task (five times, one minute each). Ergonomics intervention (posture adjustments, muscle activity training) with or without additional feedback, and a control group. (10 weeks) | Auditory intermittent feedback (ergonomics intervention), or continuous auditory feedback to relax muscle (wrist extensor and upper trapezius sEMG, KP). | Short (2 weeks) | Rapid Upper Limb Assessment. Pain (Standard Nordic Questionnaire). Upper extremity kinematics (3D motion capture system) and sEMG. | Only the ergonomics without biofeedback group reduced their mean angular velocity compared to the control group (25,12, p = 0,003). There were no significant group differences in the muscle activity index between the three groups. The post-intervention RULA was lower in the intervention groups (p = 0,000). Differences in the Standard Nordic Questionnaire scores were found between the study groups after intervention: increase in the control group (but not significantly) and decreased in the intervention groups (p = 0,001). |
Ma et al., 2010 | RCT (4 groups) | 16 | n = 60, computer user students, 67% women, 33,3±9,7 years. | Neck or shoulder. Chronic. | Standardized exercise program group: (4/day, strengthening neck and shoulder muscles). Passive treatment group: interferential therapy and hot packs (2/week). Control group: education booklet on office ergonomic. 1 feedback group (6 weeks). | Intermittent auditory feedback (on the bilateray upper trapezius activity) 2hours / days, 2 day / week as a minimum while performing computer work (KP). | T0 and long (6 months only pain) | Function (Neck Disability Index). Pain (Visual Analog Scale). Upper trapezius activity (sEMG). | The 4 groups had significantly different results in terms of their preintervention and postintervention sEMG amplitudes at T0. Pain score and NDI scores of the intervention groups had decreased significantly, and significantly more than in the control group (p = 0,012); the biofeedback group were significantly lower than in the other 3 groups. After 6 months, pain in the biofeedback group was still significantly lower than in the other 3 groups; and there was also a significant difference between the active exercise group and the passive treatment and control groups; and no significant difference between passive treatment and control groups. |
Neblett et al., 2010 | Prospective cohort study (3 groups) | 23 | n = 170, occupation not specified, 39 % women, 42,8 years. | Low back. Chronic. | Restoration treatment program (2–5 days/ week, 160 to 240 hours) with or without feedback; or control group (healthy participants) to correct abnormal flexion-relaxation. | Continuous visual and/or auditory feedback (based on sEMG) to assist stretching (KP). | T0 | Flexion-relaxation (mean errector spinae sEMG, gross lumbar flexion, pelvic flexion, true lumbar flexion with inclinometer) | Pretreatment range of motion and maximum voluntary flexion sEMG measures were similar in both treatment groups, but were very different than the control group (p = 0,000). At post-treatment, the functional restoration only group remained different than the control group on maximum voluntary flexion sEMG (p < 0,000) and gross lumbar/ pelvic flexion (p < 0,05), but the feedback group was statistically equivalent to the control participants on all post-treatment measures, including the ability to show flexion-relaxation. |
Nezamuddin et al., 2013. | RCT (2 groups) | 22 | n = 50, visual display terminal workers, 56% women, 20–35 years. | Neck. Acute or chronic. | In medical room, deep cervical flexor muscle training in addition to conventional exercises (stretching and strengthening). 5 days/week, 6 weeks.. | Continuous, visual feedback of craniocervical flexion pressure based on a unit of pressure biofeedback (KP). | T0 | Pain (Visual Analog Scale). Muscle performance (mmHg). | Difference at the end of the 6th week (p < 0,010) between the two groups for cranio-cervical flexion. During the 6-week treatment period, the mean improvement of cranio cervical flexor training score in the experimental group and control group was found to be 3,36 and 1,84, respectively. Pain in the feedback group decrease compare to the conventional exercise group at T0 (p < 0,004). |
Park et al., 2018 | RCT (2 groups) | 16 | n = 31, sedentary (sitting and standing) workers, 85% women, 33,1±13,1 years. | Low back. Acute or chronic. | In workplace, training posture program with feedback versus pedometer in static workers. 3 weeks. | Visual final feedback (at the end of each day) based on pedometer, or intermittent tactile (vibration) feedback (when not good body posture), based on accelerometer (KP). | T0 | Level of physical Activity (pedometer). Pain (Cornell Musculoskeletal Discomfort Questionnaire) | There was no statistical difference in level of physical activity by analyzing average steps between the experimental and control groups. In the CMDQ only the LBP discomfort was statistically significant when comparing pretest and posttest in the CMDQ mean score in all subjects [F(1, 18) = 6,25, p = 0,02], while there was no significant evidence of reducing LBP experience and LBP interference. However, there are no significant differences between the groups for CMDQ score. |
Ribeiro et al., 2020 | RCT (2 groups) | 21 | n = 130, health care workers (physical), 84,6% women; 45,3 years. | Low back. All clinical stage. | During working hours, feedback group or sham group to modify postural behavior. (4 weeks). | Auditory intermittent feedback based on IMU measuring lombopelvic forward bending (KP). | Short (1 week), middle (1–3 months) and long (6–12 months) | Postural behaviour (number of times with postural threshold exceeded). | There were no within-group changes on the number of times postural threshold was exceeded at 1-week follow-up, and no differences between shame group and feedback group. At 12-month follow-up, no significant changes were observed for the both groups. |
Ribeiro et al., 2014 | RCT feasibility (3 groups) | 20 | n = 62, health care organisation (72% physical and 28% sedentary), 92% women, 49±12 years. | Low back. All clinical stage. | During daily work-related activities, feedback device for modifying postural behavior, 1 group control (6 weeks). | Intermittent audio feedback (1week/2) or continuous audio feedback based on IMU (number of cumulative postural outcomes, KR) (4 weeks). | Short (1 week) | Postural pattern change (frequency, accelerometer). | No significant differences were found for postural pattern between baseline and the follow-up period for the control group and intermittent feedback group. The post-hoc test revealed no statistically significant differences between the groups. |
Sandsjo et al., 2010 | RCT (2 groups) | 12 | n = 65, computer users, 100% women, 45±11 years. | Neck and shoulder. Chronic. | In workplace, feedback-based on teletreatment or a conventional care in a private clinic. 4 weeks. | Visual continuous feedback based on trapezius sEMG (min 8 h/week, 2d/weeks and during 2hours) and verbal final feedback (teleconsultation each week, KP). | T0 and short (3 months) | Function (Work Ability Index). Pain intensity (Visual Analog Scale), Pain-related (Pain disability Index) | Improvement in terms of work ability for both groups taken together, with no differences between them. Improvement in terms of pain for both groups taken together, with no differences between them. Non-parametric tests showed an intervention effect in pain related disability for both groups together and no differences between them when tested at baseline, T0 and T3. |
Spence et al., 1995 | RCT (4 groups) | 12 | n = 48, mixed occupation, 84% women, 42±7,86 years. | Upper extremity and neck. Chronic. | At home, relaxation training, sEMG biofeedback, combined approach (by a psychologist) or wait-list control. 2/week, 4 weeks. | Auditory intermittent feedback based on muscle tension levels with forearm flexor or trapezius sEMG under pain area (KP). | T0 and long (6 months without control group) | Pain (Pain Beliefs Questionnaire [PBQ], West Haven Yale Multidimensional Pain Inventory [WHYMPI], Self-Monitored Pain Index) | Patients in all 3 treatment conditions showed reductions in pain (PBQ and self-monitored pain index, p < 0,05) over time at T0. At 6-month follow-up a change over time was found for the 3 treatment groups combined (Self-Monitored Pain Index, p < 0,01). There was no difference between treatments over time. WHYMPDI did not show significant reduction at T0 and 6 months. |
Voerman et al., 2007a | RCT (2 groups) | 17 | n = 79, computer workers, 52,0±58, years. | Neck. Chronic | During occupational activities ergonomic counseling with feedback. Measurement in laboratory (typing task, mouse stress and precision tasks). 4 weeks. | Continuous tactile (vibration) and audio feedback based on upper trapezius to relax (KP). (2hours/day and 2days/week minimum) | T0, middle (3 months) and long (6 months) | Pain disability index. Neck, shoulder, upper back pain (Visual analog scale) | Disability levels changed over time (F = 17,68, p < 0,01), without additional effects. Pain intensity in the neck-shoulder region changed over time (F = 12,08, p≤01), without additional effects. Post Hoc comparisons revealed that the VAS score was reduced at T0, T3, and T6 (p < 0,01) compared to baseline but also the reduction between T0 and T3 was significant (t = 2,85, p = 0,01). |
Voerman et al., 2007b | 19 | Muscle activation (sEMG) | No clear pattern of change. Post Hoc analysis revealed that small increased RMS values were found during the precision task at the left side (F = 5,74, p = 0.01) at T0 compared to baseline. RMS values during the stress task were reduced at the right side at T3 compared to baseline (F = 4,05, p = 0,05) but no other main effects or interactions were observed (p > 0,08). |
Table 2
Descriptions of the various scales used as outcome measures in the included studies
Name of the scale or outcome | Description |
Daily Symptom Survey | Assess respondent’s level of pain or discomfort in nine body areas |
Disabilities of the Arm, Shoulder and Hand | Questionnaire with 30-item scale (0–100) that measure physical disability and symptom of upper extremity |
Flexion-relaxation phenomenon | In standing position, lumbar flexion is generally associated with erector spinae muscle relaxation in healthy controls, but not in individuals with chronic low back pain |
Functional restoration treatment program | Full-day multidisciplinary program lasting 3 to 6 weeks for chronic low-back pain |
Localized Musculoskeletal Discomfort | Assess discomfort caused by static posture in 12 body areas |
Modified-Dental Operator PostureAssessment Instrument | A self-assessment posture tool for the hip, trunk, neck and upper extremities, based on 12 criteria |
Muscle Learning Therapy | Operant conditioning procedures to decrease muscle tension using sEMG feedback |
Neck Disability Index | Assess how neck pain affected the ability to manage everyday life |
Numeric Pain Rating Scale | Capture patient’s level of pain (0–10) |
Pain Beliefs Questionnaire | Assess disability, expectation, self-efficacy, depression, and pain as a threat |
Pain Disability Index | Assess chronic pain interference in various life activity |
Rapid and Upper Limb Assessment | Assess upper limb posture and indicate the level of intervention required to reduce the risk of injury due to physical loading on the worker |
Self-Monitored Pain | Assess the number of hours of pain, the degree of interference in daily living and type and quantity of medication |
Shoulder Pain and Disability Index | Self-reported questionnaire 13-item scale (0–100) to measure pain and disability associated with shoulder pathology |
Standard Nordic Questionnaire | Assess symptoms in various daily and working life activities with multiple choice questions of various body areas |
Von Korff rating scale | Numeric pain scale for specific time frames |
Work Ability Index | Measure seven dimensions of work ability |
3Results
The database search yielded 4,931 articles after removing duplicates. After full text analysis, 49 studies were included (See Fig. 1 and Table 1). There were 14 cross-sectional laboratory studies (CSLS), 2 cross-sectional workplace studies, 11 prospective cohort studies (PCS), 1 crossover study, 2 pilot randomized controlled trials (RCT), 1 pseudo RCT and 18 RCT. The studies enrolled healthy participants who performed tasks in controlled environments (n = 16) or in the workplace (n = 15), and/or injured workers who performed tasks in the workplace (n = 10) and controlled environments (n = 5). Three studies included both healthy and injured participants in the workplace. Work activity levels were sedentary (e.g., static sitting or standing position, n = 31), physical (n = 11), mixed or nonspecific (n = 7) (See Table 1, Fig. 2). Feedback focused on the neck and upper extremities (n = 24), low back (n = 16), lower extremities (n = 1), and mixed or nonspecific locations (n = 8). The total number of participants was 3387: 2204 healthy, 925 injured, and 258 undefined participants. The main sources of feedback used included sEMG (n = 14), IMUs (n = 11), and cranio-cervical pressure (n = 4), while 5 studies mixed two sources of feedback. The feedback sensory modalities used were audio (n = 8), tactile (n = 10), visual (n = 9), mixed (n = 12), and two studies compared the effects of different sensory modalities [33, 34] (See Fig. 2). The frequency of the feedback was continuous (n = 14), intermittent (n = 21), final (n = 4), mixed (n = 10), and two studies compared the effects of different frequencies of feedback [34, 35] (See Fig. 2). The delivered feedback pertained to the knowledge of performance (n = 40) or results (n = 9), and one study compared the effects of different methods of delivery [33]. Studies explored the effects of the feedback intervention alone (n = 35) or in combination with other interventions (exercise n = 7, ergonomic adjustments n = 7). The effect of feedback was evaluated during (n = 8), just after the intervention (n = 34), in the short term (<2 months, n = 17), in the midterm (<6 months, n = 9), and in the long term (≥6 months, n = 9).
Fig. 1
Study flowchart.
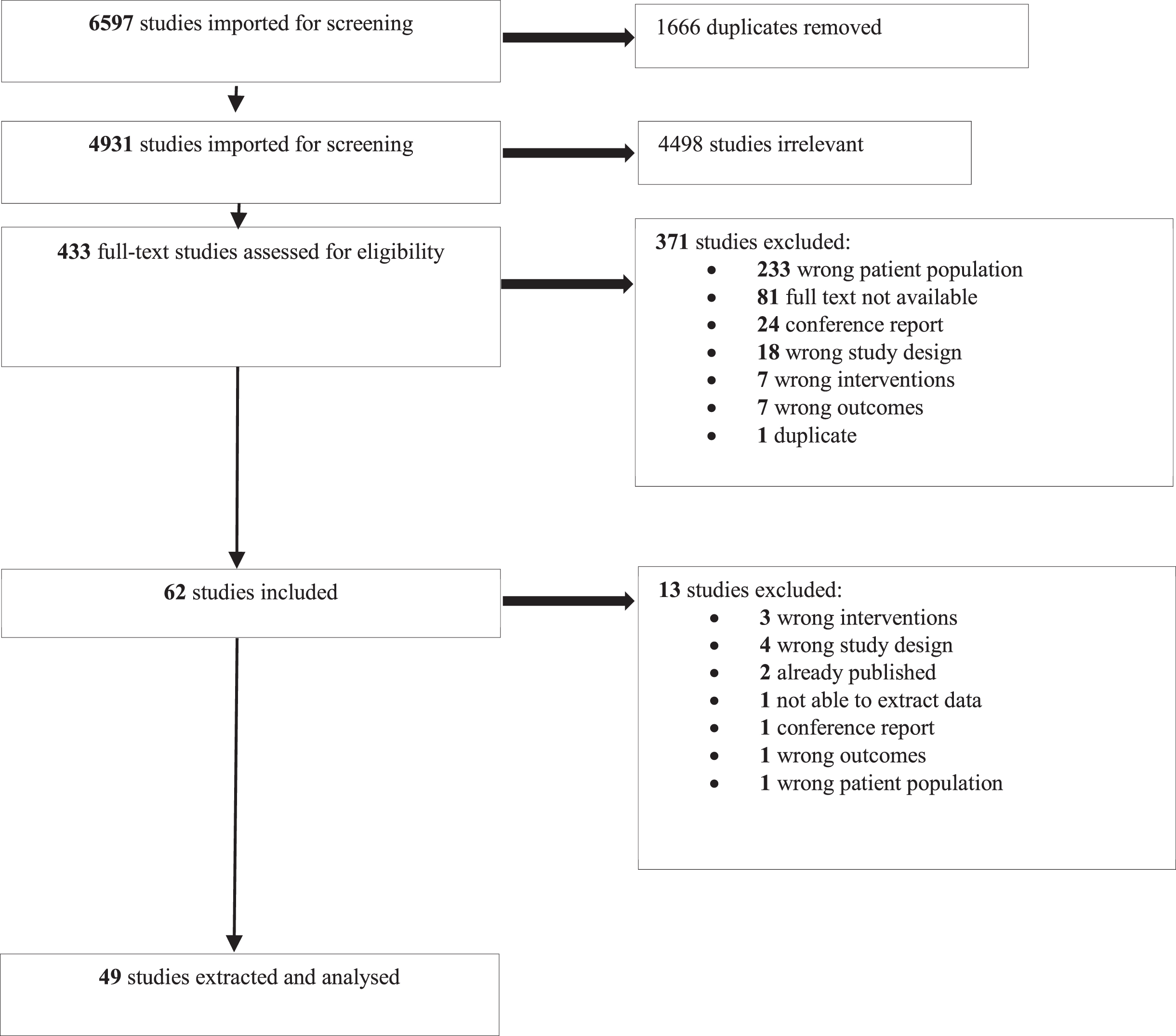
Fig. 2a
Occupation, modality and frequency of feedback and their effects in prevention. ++: effect superior compared to another intervention or to a control group. +: positive effect (not compared to another intervention). ±: partial positive effect. ∅: inferior or no additional positive effect compared to another intervention. -: negative effect.
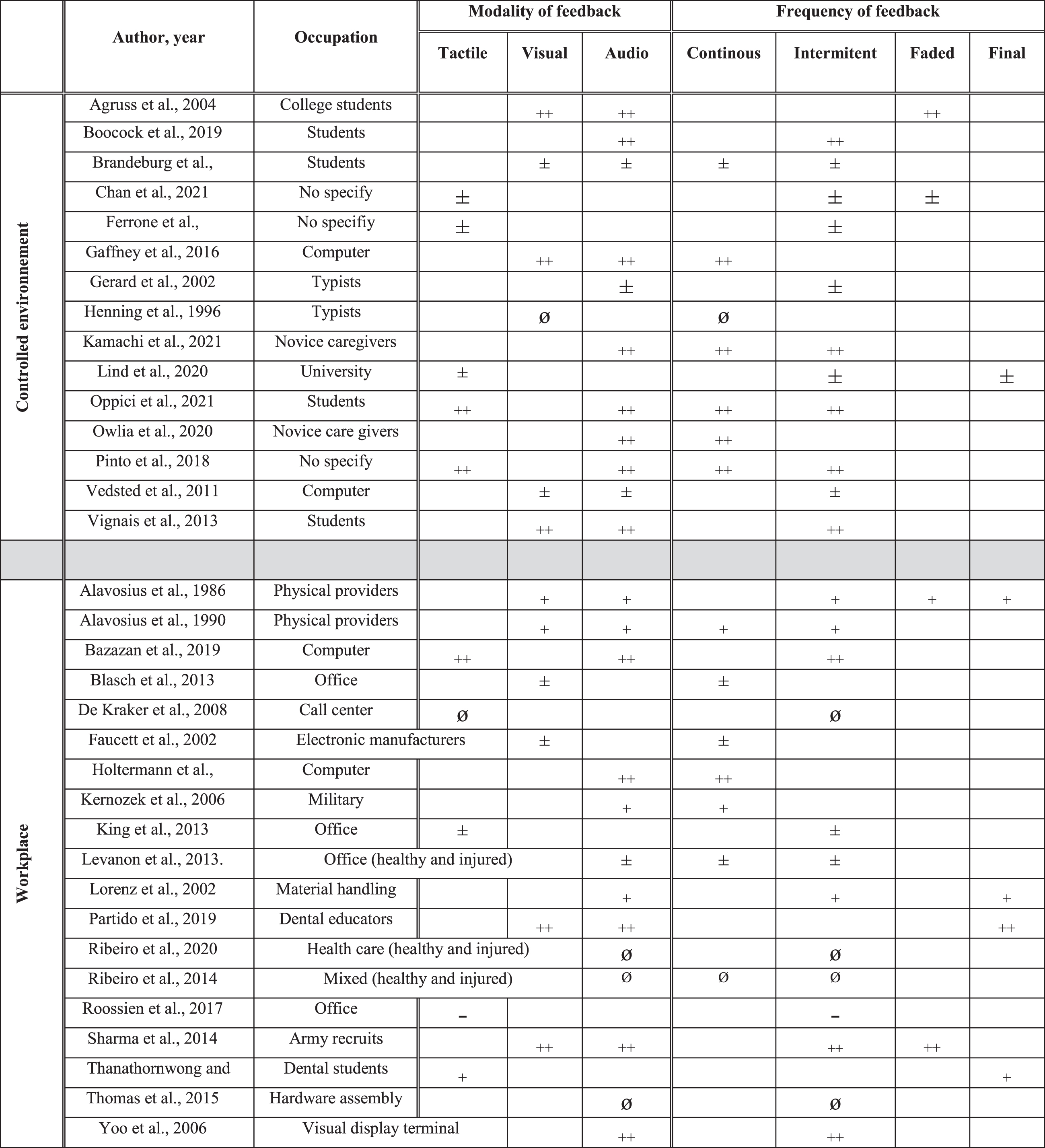
Fig. 2b
Occupation, modality and frequency of feedback and their effects in rehabilitation. ++: effect superior compared to another intervention or to a control group. +: positive effect (not compared to another intervention). ±: partial positive effect. ∅: inferior or no additional positive effect compared to another intervention.
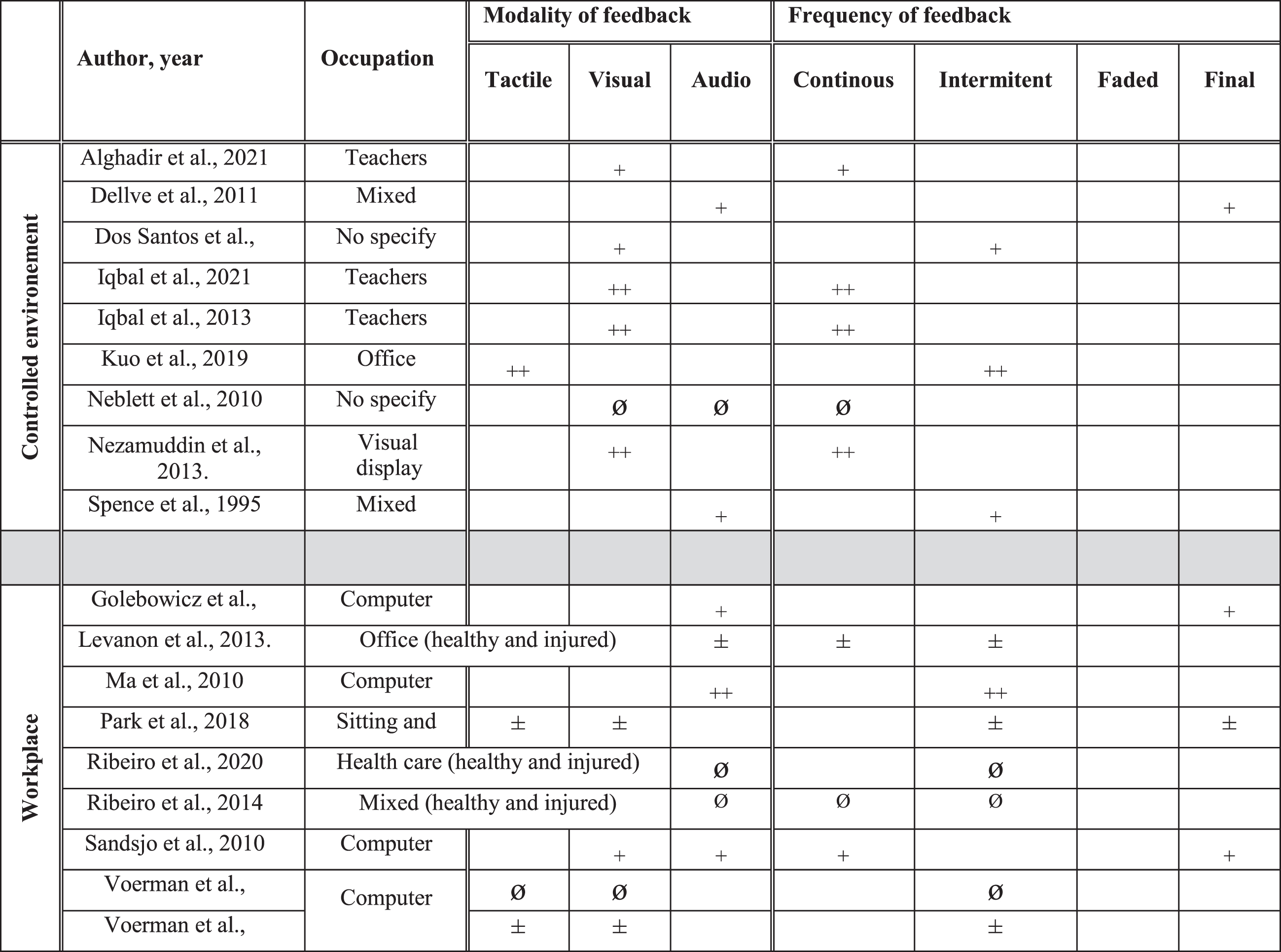
The pre-consensus inter-rater agreement was considered good between reviewers for each item (Gwet’s gamma coefficient = 0.84) and for total scores of the Downs and Black checklist (ICC = 0.86). Fourteen studies were of limited quality, 32 were of moderate quality, and 3 were of strongquality.
Results are organized by stage (prevention, rehabilitation), environment (controlled, workplace) and outcomes (function, symptoms, sensorimotor control). For each outcome, the studies are organized by work type (in terms of activity level) and type of effect (positive, null, negative). For each study, the p value, the study design and risk of bias are indicated. For details on the type of feedback used, see Table 1 and Fig. 2, for the synthesis of the body of evidence see Table 3.
Table 3a
Synthesis of body of evidence by outcome (function, symptoms and sensory-motor control) in prevention (CSS: cross-sectional study, LB: low back, LL: lower limb, LQ: low quality, M: mixed, MQ: moderate quality, NUL: neck and upper limb, NSP: not specified, NS: not significant, PCS: prospective cohort study, P: physical, PQ: poor quality, RCT: randomized controlled trial, S: significant, Se: sedentary, SQ: strong quality, T0: just after intervention)
Imprecision | Methodological quality | Indirectness | Inconsistency | Quality of evidence | ||||
n of study | n of subject | Type of study | Downs and Black checklist | Work types | MSK injury location | Statistical effect and time assessment | ||
Prevention in controlled environment | ||||||||
Fonctional level | 1 | 12 | CSS = 1 | LQ | P = 1 | NUL = 1 | S at T0 (n = 1) | Very limited evidence |
Symptoms | 4 | 75 | CSS = 2 | MQ = 1 | Se = 4 | NUL = 3 | S at T0 (n = 2) | Conflicting evidence |
PCS = 2 | LQ = 2 | NS = 1 | NS at T0 (n = 2) | |||||
Sensorimotor control | 12 | 269 | CSS = 10 | MQ = 8 | P = 9 | NUL = 4 | S at T0 (n = 11) and at short (n = 3) and middle term (n = 1) | Moderate evidence |
PCS = 2 | LQ = 4 | Se = 3 | LB = 8 | NS at short term (n = 1, only on sEMG fatigue, PCS, LQ, Gerard et al.) | ||||
Prevention in the workplace | ||||||||
Fonctional level | 6 | 473 | RCT = 4 | MQ = 5 | Se = 3 | NUL = 1LB = 2 | S at T0 (n = 2); at short (n = 3), at middle (n = 2); at long term (n = 2) | Limited evidence |
PCS = 2 | LQ = 1 | P = 3 | LL = 1ML = 2 | NS in short, middle, and long term (n = 1, RCT MQ, Faucett et al.) | ||||
Symptoms | 7 | 533 | RCT = 3 | MQ = 5 | Se = 7 | NUP = 4 | S at T0 (n = 2), at middle(n = 1), at long term (n = 2) | Conflicting evidence |
Pseudo RCT = 2 | ||||||||
PCS = 2 | LQ = 2 | ML = 3 | NS at T0 (n = 2), at short (n = 1), at middle (n = 1), at long term (n = 1) | |||||
Sensorimotor control | 12 | 1688 | RCT = 4 | MQ = 9 | Se = 9 | NUL = 5 | S at T0 (n = 7), at short (n = 1), at long term (n = 1) | Conflicting evidence |
LB = 2 | Negatively S at T0 (n = 2) | |||||||
Pseudo RCT = 2other = 6 | LQ = 3 | P = 3 | LL = 1ML = 4 | NS at T0 (n = 4), at short (n = 1), at middle (n = 1), at long term (n = 1) |
Table 3b
Synthesis of body of evidence by outcome in rehabilitation (CSS: cross-sectional study, LB: low back, LL: lower limb, LQ: low quality, M: mixed, MQ: moderate quality, NUL: neck and upper limb, NSP: not specified, NS: not significant, PCS: prospective cohort study, P: physical, PQ: poor quality, RCT: randomized controlled trial, S: significant, Se: sedentary, SQ: strong quality, T0: just after intervention)
Imprecision | Methodological quality | Indirectness | Inconsistency | Quality of evidence | ||||
n of study | n of subject | Type of study | Downs and Black | Work types | MSK injury location | Statistical effect and time assessment | ||
Rehabilitation in controlled environment | ||||||||
Fonctional level | 4 | 339 | RCT = 3 | MQ = 4 | Se = 2 | NUL = 4 | S at T0 (n = 4), short (n = 1) and long term (n = 1) | Moderate evidence |
M = 1 | ||||||||
PCS = 1 | NSP = 1 | |||||||
Symptoms | 8 | 508 | CSS = 1 | SQ = 1 | Se = 5 | NUL = 8 | S at T0 (n = 6), at short (n = 2), middle (n = 1) and long (n = 2) term | Moderate evidence |
PCS = 2 | MQ = 6 | M = 2 | ||||||
RCT = 5 | LQ = 1 | NSP = 1 | Negatively S at T0 (n = 1, CSS, MQ, Kuo et al) | |||||
Sensorimotor control | 6 | 539 | RCT = 2 | SQ = 2 | Se = 3 | NUL = 5 | S at T0 (n = 5), at short (n = 1) and long (n = 1) term | Moderate evidence |
CSS = 2 | MQ = 4 | M = 1 | LB = 1 | NS at T0 (n = 1, PCS, SQ, Neblett et al.) | ||||
PCS = 2 | NSP = 2 | |||||||
Rehabilitation in the workplace | ||||||||
Fonctional level | 5 | 282 | RCT = 4 | MQ = 3 | Se = 5 | NUL = 5 | S at T0 (n = 2), at short (n = 2) | Conflicting evidence |
PCS = 1 | LQ = 2 | NS at T0 (n = 1), short (n = 1), long (n = 1) | ||||||
Symptoms | 6 | 313 | RCT = 5 | MQ = 4 | Se = 6 | NUL = 5 | S at T0 (n = 2), at short (n = 2), at middle (n = 1), at long term (n = 1) | Conflicting evidence |
PCS = 1 | LQ = 2 | LB = 1 | NS at T0 (n = 2), at short (n = 1), at long term (n = 1) | |||||
Sensorimotor control | 5 | 397 | RCT = 5 | MQ = 5 | Se = 3 | NUL = 3 | S at T0 (n = 1, RCT with 4 groups, MQ, Ma et al.) | Limited evidence for no effect |
Pseudo RCT = 1 | M = 1 | LB = 2 | NS at T0 (n = 1), at short (n = 3), at middle term (n = 2), long (n = 1) |
a. Feedback effects for prevention in controlled environments
The body of evidence on the effect of feedback for prevention in controlled environments on function (one study, total of 12 participants) is considered very limited and suggests a positive effect. The single study on this topic found a positive effect: audio and visual feedback based on a computerized Rapid Upper Limb Assessment (RULA) [36] led to a decrease in injury risk during four simulated industrial manufacturing tasks (p < 0.05, CSLS, LQ)[37].
The body of evidence on the effect of feedback for prevention in controlled environments on symptoms (4 studies, total of 75 participants) is considered conflicting. Two studies found positive effects. During typing tasks, the addition of rest breaks to work time, using a visual display to manage the length of rest breaks, led to a decrease in low back discomfort during a cognitive task, but not during a standard condition (p < 0.05, CSLS, LQ) [38]. During a screwdriving task, the use of a mirror to improve neck posture reduced discomfort; the addition of a verbal reinforcement by a researcher caused no further reduction (p < 0.05, CSLS, LQ) [33]. In two other studies, the use of feedback had no effect on musculoskeletal symptoms. Auditory feedback based on finger sEMG or on keyboard force monitors did not influence pain during a typing task (p < 0.05, PCS, MQ) [39]. Instructions with or without vibration feedback to reduce adverse arm movements and posture during mail sorting tasks did not influence symptoms (CSLS, MQ) [40].
The body of evidence on the effect of feedback for prevention in controlled environments on sensorimotor control is considered moderate and suggests positive effects (twelve studies, total of 264 participants). The 12 studies found positive effects. During computer tasks, audio and visual feedback based on sEMG led to a decrease in muscle activity (p < 0.05; PCS, MD; CSLS, LQ) [39, 41]. The addition of continuous visual feedback (based on high density sEMG) to verbal feedback related to posture also led to a change in the spatial distribution of trapezius activity and in shoulder position (p < 0.05, CSLS, LQ) [42]. The addition of vibration feedback based on IMUs to verbal feedback also led to a decrease in arm elevation during mail sorting tasks (p < 0.005, CSLS, MQ) [40]. Various types of feedback led to improved lumbar posture during handling tasks: audio feedback based on lumbar IMUs to assess lumbar flexion (p < 0.05, CSLS, MQs) [43, 44], lumbar rigid strapping tape (p < 0.0001, CSLS, LQ) [45], final faded verbal feedback (based on 3D motion analysis), intermittent audio feedback based on sEMG to reduce lumbosacral compression (p < 0.01, CSLS, MQ) [46], and intermittent vibration feedback and terminal verbal feedback (based on 3D motion analysis, p < 0.005, CSS, MQ) [47]. During repetitive lifting tasks, auditory feedback (based on 3D motion analysis) led to decrease sacro-lumbar peak flexion more than continuous tactile feedback (based on tape applied, p < 0.05, CSS, MQ) [34]. In addition during care tasks, intermittent haptic or faded intermittent auditory feedback (based on IMUs) led to improve lumbar posture in forward/backward (p < 0.05, PCS, LQ) [48, 49].
b. Feedback effects for prevention in the workplace
The body of evidence on the effect of feedback for prevention in the workplace on function is considered limited and suggests a positive effect (six studies, 473 participants). The six included studies found positive effects. Audio or vibration feedback based on IMUs to control upper-back posture in control room operators led to a decrease in RULA scores in the long term (p < 0.05, RCT, MQ) [50]. In dental educators aiming to self-evaluate specific ergonomic criteria, the addition of feedback that was based on pictures of posture improved posture ergonomic score more than ergonomic self-assessment [51] alone (F = 6.295, p < 0.01, RCT, MQ) [52]. When verbal and visual feedback on transfer techniques (e.g., knee bending, maintaining a straight spine) was offered by therapists based on a checklist, the percentage of safely performed transfers in residential facilities was significantly increased in the short and long term (p unknown, 2 PCS, LQ [53] and MQ [54]). Pressure sensors (treadmill) feedback in combination with verbal feedback from therapists decreased the incidence of tibial stress syndrome in army recruits (p = 0.05, RCT, MQ) [55]. However, either visual feedback based on sEMG combined with Muscle Learning Therapy [56] or cognitive behavioral techniques were not more effective than application of ergonomics guidelines on the occurrence of upper extremity injuries in electronics manufacturers (p < 0.05, RCT, MQ) [57].
The body of evidence on the effect of feedback for prevention in the workplace on symptoms is considered conflicting (seven studies, 533 participants). Five studies found positive effects. Audio or vibration feedback based on IMUs to assess shoulder and trunk position (Nordic Musculoskeletal Questionnaire, p < 0.05, RCT, MQ) [50], and vibration feedback provided from chair pressure sensors to improve sitting behavior (Localized Musculoskeletal Discomfort [58], p < 0.01, PCS, MQ) [59] led to a decrease in the occurrence of musculoskeletal symptoms. Similarly, use of a vibrating computer mouse to modify hand posture (Daily Symptom Survey [60], p < 0.05, RCT pilot, MQ) [61], and visual feedback based on skin conductance level and finger temperature to assist relaxation and stretching (frequency of symptoms immediately after the intervention and in the mid-term, p < 0.05, pseudo RCT, MQ) [62] in sedentary seated workers led to a decrease in the occurrence of musculoskeletal symptoms. Visual feedback based on sEMG combined with Muscle Learning Therapy decreased upper extremity symptoms (Visual Analog Scale and body chart) in electronic manufacturers compared to cognitive behavioral techniques or ergonomics guidelines in the short term (p < 0.05, RCT, MQ) [57]. However, three studies did not observe such changes in symptoms for types of feedback such as: computer mouse feedback (Localized Musculoskeletal Discomfort, RCT, LQ) [63], feedback based on sEMG alone (audio, body chart, PCS, LQ) [64], or feedback based on sEMG with Muscle Learning Therapy (visual, VAS and body chart, immediately after intervention, in the mid- and long term, RCT, MQ) [57] to discourage awkward hand posture in line workers.
The body of evidence on the effect of feedback for prevention in the workplace on sensorimotor control is considered conflicting (12 studies, total of 1688 participants). Seven studies found positive effects. Vibration feedback from pressure sensors fixed on a chair led to modified sitting (p < 0.05, CSS, MQ) [65], while vibration feedback from one accelerometer on the upper back led to improved posture of dental educators and to a decrease in upper trapezius sEMG (p < 0.05, crossover study, LQ) [66]. Audiovisual feedback based on trapezius sEMG alone (p < 0.05, RCT, MQ) [67] or combined with Muscle Learning Therapy (p < 0.04, RCT, MQ) [57] in office workers led to a decrease in muscle activity. Auditory feedback based on magnetic motion measurement of lumbar moments combined with verbal therapists’ feedback decreased lumbar flexion and side bending torque during material handling (p < 0.05, PCS, MQ [68] and RCT, MQ [69]). Visual feedback based on pressure sensors (in a treadmill) in combination with a therapist’s verbal feedback to prevent tibial stress syndrome improved foot balance and time to reach peak heel rotation in army recruits (p = 0.05, RCT, MQ) [55]. However, five studies did not find any positive effect. Audio feedback based on forearm sEMG did not influence grip strength in electronics manufacturers (PCS, LQ) [64]. Visual feedback based on skin conductance level and finger temperature to assist relaxation and stretching did not influence computer users’ upper trapezius’ sEMG activity (pseudo RCT, MQ) [62]. Vibration feedback, provided by pressure sensors fixed on the operator’s chair, did not modify sitting behaviors (e.g., sitting duration, PCS, MQ) [59]. Computer mouse feedback (vibration), meant to decrease awkward computer mouse hand posture, increased awkwardness of computer mouse hand posture after feedback exposure (p < 0.03, RCT, LQ) [63], (p < 0.05, RCT pilot, MQ) [61].
c. Feedback effects for rehabilitation in controlled environments
The body of evidence on the effect of feedback for rehabilitation in controlled environments on function is considered moderate (four studies, 339 participants). All four studies found positive effects. Visual pressure feedback used to manage cranio-cervical flexion exercises in addition to conventional exercises led to greater improvement in teachers’ Neck Disability Index [70] scores after the intervention (p < 0.05, RCT, MQ) [71] and in the short term (p < 0.05, RCT, MQ) [72]. Verbal feedback by a therapist (based on sEMG) led to improved Work Ability Index [73] scores in the short and mid-term follow-ups in a chronic neck pain population (p < 0.05, RCT, MQ) [74]. Intermittent visual feedback (based on sEMG) associated with scapular focused exercises led to improve DASH (Disabilities of the Arm, Shoulder and Hand) [75] and SPADI (Shoulder Pain and Disability Index) [75] scores (p < 0.05, PCS, MQ) [76].
The body of evidence on the effect of feedback for rehabilitation in controlled environments on symptoms is considered moderate (eight studies, 508 participants) and suggests positive effects. Five studies found positive effects. Visual pressure feedback for cranio-cervical flexion exercises in addition to conventional exercises led to a greater decrease in pain (Numeric Pain Rating Scale [77]) than conventional exercises alone [78], in both teachers [71, 72] and visual display terminal workers [79] (p < 0.05, RCTs, MQ and SQ) immediately after the intervention [71, 79] and in the short term (p < 0.05, RCT, MQ) [72]. Feedback based on sEMG alone [74, 80] or in combination with relaxation training [80] in a population of physical and sedentary workers led to a decrease in neck pain (p = 0.05, RCT, MQ, Von Korff rating scale [81]) [74] and in upper extremity pain (p < 0.05, RCT, LQ, Pain Beliefs Questionnaire [82] and Self-Monitored Pain [80]) immediately after the intervention [74, 80] and in the mid-term [74]. Intermittent visual feedback (based on sEMG) associated with scapular focused exercises led to improve NPRS scores (p < 0.05, PCS, MQ) [76]. However, one study concluded that vibration feedback based on IMUs to monitor upper body posture led to an increase in neck and shoulder pain (Numeric Pain Rating Scale) in office workers just after the intervention (p < 0.001, CSLS, MQ) [83].
The body of evidence on the effect of feedback for rehabilitation in controlled environments on sensorimotor control is considered moderate (six studies, total of 539 participants) and suggests positive effects. Three studies found positive effects. Visual pressure feedback for cranio-cervical flexion exercises in addition to conventional exercises led to improved neck muscle endurance compared to conventional exercises alone (p < 0.05, RCT, MQ [71] and RCT, SQ [79]) or cranio vertebral angle (p < 0.05, CSS, MQ) [78]. Vibration feedback based on IMUs to monitor upper body posture led to a decrease in cervical erector spinae sEMG amplitude and to decreased neck and thoracic flexion measured in office workers after the intervention (p < 0.05, CSLS, MQ) [83]. Intermittent visual feedback (based on sEMG) associated with scapular focused exercises led to improve range of motion, strength and neuro muscular control (p < 0.05, PCS, MQ) [76]. However, the addition of visual and audio sEMG feedback to a functional restoration treatment program [84] for low back pain did not lead to greater improvement of muscle relaxation during standing flexion [85] (PCS, SQ) [86].
d. Feedback effects for rehabilitation in the workplace
The body of evidence on the effect of feedback for rehabilitation in the workplace on function is considered conflicting (six studies, 282 participants). Four studies found positive effects. Verbal or visual [87, 88] feedback through telerehabilitation based on upper extremity sEMG data alone [88], or with an ergonomic intervention [87] aiming to increase muscle relaxation, led to decreased physical loading (RULA, p = 0.003, PCS, LQ) [87] and to improved Work Ability Index and Pain Disability Index [89] scores, similarly to effects observed with conventional care, immediately after the intervention and in the short term (p < 0.05, RCT, LQ) [88] in computer operators with neck and shoulder pain. Audio feedback based on sEMG improved computer users’ Neck Disability Index scores more than shoulder muscle strengthening, passive treatment or educational ergonomics booklets (p = 0.012, RCT, MQ) [90]. On the other hand, two studies did not observe any positive effect in computer operators when tactile [91] and audio [69, 91] feedback based on sEMG were added to ergonomics counseling. Outcomes were Pain Disability Index scores immediately after the intervention and in the mid and long term (RCT, MQ) [91], and physical loading (RULA, RCT, SQ) [69].
The body of evidence on the effect of feedback for rehabilitation in the workplace on symptoms is considered conflicting (six studies, 313 participants). Four studies found positive effects. Verbal or visual [87, 88] feedback through telerehabilitation based on upper extremity sEMG data alone [88] or with an ergonomic intervention [87] led to a decrease in pain in the short term (Standard Nordic Questionnaire [92], p = 0.003, PCS, LQ) [87] or an effect similar to conventional care immediately after the intervention and in the short term (Visual Analog Scale, p < 0.05, RCT, LQ) [88]. Audio feedback based on sEMG led to a decrease in computer users’ pain (Visual Analog Scale), more effectively than shoulder muscle strengthening, passive treatment or ergonomics booklets, immediately after the intervention and in the long term (p < 0.05, RCT, MQ) [90]. Visual feedback based on pedometers and vibration based on lumbar posture (IMUs) led to reduced low back pain (Cornell Musculoskeletal Discomfort Questionnaire) after the intervention in standing and sitting workers (p = 0.02, RCT, MQ) [93]. Two studies did not observe such positive effects. In computer workers, the addition of tactile [91] and audio [69, 91] feedback based on sEMG to ergonomics counseling did not significantly influence symptoms immediately after the intervention (Visual Analog Scale, RCT, MQ), in the short term (Standard Nordic Questionnaire, RCT, SQ) [69] or in the long term (Visual Analog Scale, RCT, MQ) [91].
The body of evidence on the effect of feedback for rehabilitation in the workplace on sensorimotor control is considered limited and suggests no effect (five studies, total of 397 participants). One study found positive effects: audio feedback based on sEMG reduced muscle activity of office workers compared to strengthening exercises, passive treatment, or educational ergonomics booklets (p < 0.05, RCT, MQ) [90]. On the other hand, four studies did not observe any positive effect. Continuous or intermittent audio feedback based on IMUs to limit excess forward bending posture did not significantly improve lumbar posture compared to a control group in physically active [35, 94] and sedentary [35] health workers (RCTs, SQ) in the short [35, 94] and long term [94]. The addition of tactile [91] and audio [69, 91] feedback based on sEMG to ergonomics counseling in computer workers did not significantly modify sEMG activity [69, 91] and upper extremity kinematics immediately after the intervention, and in the short (RCT, SQ) [69] and long term (RCT, MQ) [91].
4Discussion
This systematic review of 49 studies offers a large overview of the effects of extrinsic feedback used during simulated or real working tasks in the prevention and rehabilitation of WRMSDs. For the prevention of WRMSDs, findings show that feedback leads to improved function in controlled environments (very limited evidence) and in the workplace (limited evidence), and to improved sensorimotor control in controlled environments (moderate evidence). Its effects on symptoms, however, are conflicting. For the rehabilitation of WRMSDs, feedback mostly had a positive effect on function, symptoms and sensorimotor control (moderate evidence) in controlled environments, while its effects in the workplace were conflicting for function and symptoms and null for sensorimotor control. The lack of studies comparing the different characteristics of feedback (e.g., sensory modalities, frequency) made it impossible to suggest specific feedback. These findings are more exhaustive than previous systematic reviews [18–20] which reported conflicting evidence based on a very small number of studies on the effect of different types of feedback for the upper extremity.
Our review is the first to attempt to address the effects of feedback in two different environments, namely controlled (clinics or research laboratories) and real-world environments (workplace). Specific working tasks (e.g., lifting a patient out of their bed) may not be similarly conducted and experienced by the therapist in controlled environments versus in the workplace. This is due to the possible unforeseen events present in the real world (e.g., medical bed blocked in a low position), work-related fatigue (e.g., therapist may have previously lifted multiple patients), and the biopsychosocial context of the workplace [95–97] (e.g., stress [98], organization [99]). These differences between environments could explain the discrepancy between results obtained in controlled and real environments.
The advantages of using feedback in controlled environments are isolation of the specific working task and, hopefully, improvement of movement quality. The positive outcomes conclusions reported in controlled environments show the clinical relevance of using feedback in clinics as their use in the included studies can be compared to a typical session in the clinic where clinicians use external feedback during movement or task simulations. Although multiple devices or sensors can be used to give feedback to patients, there are various non-technological types of feedback that can easily be used by clinicians. Examples include ergonomics counseling (verbal feedback) based on the therapist’s observations or based on a checklist, visual feedback based on cranio-cervical flexion pressure, and tactile feedback from rigid strapping tape to improve posture sensation. Therapists can therefore use feedback in their clinical practice to prevent or decrease pain and disability, and to optimize sensorimotor control during simulated work tasks before returning to work.
Feedback could be a useful tool in the prevention of WRMSDs, as certain studies in controlled environments have shown that it may reduce factors associated with the development of WRMSDs, e.g., decreased muscle activity during computer tasks or reduced lumbosacral compression during lifting tasks. These results are in line with findings concerning the use of feedback for the prevention of sport-related injuries. Examples include using feedback for gait retraining in runners [100] (leading to reduced ground reaction force variables) or for modifying jump-landing technique for the prevention of anterior cruciate ligament injury [101] (leading to reduced vertical ground reaction forces and knee abduction/flexion angle).
However, most WRMSDs develop gradually, over several months or years, due to repetitive movements, sustained posture or exposure to stress [2, 102]. The lack of high-quality, long-term studies evaluating the effect of feedback for the prevention of WRMSDs in the workplace prevents us from concluding on the potential of feedback to decrease the prevalence of WRMSDs. In addition, most included studies recruited young, healthy individuals without any symptoms, who had fewer risk factors for developing WRMSDs [103], and only a small margin for the outcomes progression. Further long-term longitudinal studies with workers should be conducted to determine whether feedback can be used for the prevention of WRMSDs.
The effect of feedback in the workplace was found to be null to very limited. These results are not surprising as several studies compared feedback to ergonomic adjustment and demonstrated the superiority of ergonomic adjustment in the workplace. By optimizing the working environment according to the physical characteristics of the worker, ergonomic adjustment is the most comprehensive, simple and effective intervention in the workplace to correct posture or movement [20, 104, 105]. Feedback, perceived as a more complex intervention by workers, should be used once work ergonomics have been optimized. Further studies could explore the value of adding feedback after ergonomic interventions.
The findings of this systematic review allow us to propose different clinical implications. Firstly, feedback can be used to preserve function in both controlled (very limited evidence) and work (moderate evidence) environments, and to improve/preserve sensorimotor control in controlled environments (moderate evidence). Secondly, clinicians can include interventions using feedback in controlled environments, as they have been shown to reduce symptoms and enhance function and sensorimotor control. Thirdly, feedback alone is not indicated for the rehabilitation of WRMSDs in the workplace as ergonomic adjustment should be performed first. More evidence is needed from long term studies before recommending the use of feedback for WRMSD prevention or in workplace rehabilitation as a complement to ergonomic interventions.
The main source of limitations in this systematic review is the heterogeneity of the included studies which prevented us from concluding on the specific effects of the different types of feedback (for instance, whether faded verbal feedback is more effective than continuous verbal feedback for pain prevention) and from performing meta-analyses. In addition, the lack of RCT and high-quality studies decreased the level of evidence and the possibility of producing clear recommendations. Still, the inclusion of cross-sectional and non-randomized studies allowed us to explore the effect of feedback on various body regions and environments. Moreover, 17 of the 43 included studies combined verbal feedback with another feedback modality, without assessing the effect of each type of feedback. Therefore, it is impossible to determine whether the use of a second type of feedback contributed to the observed outcomes. Finally, the paucity of studies with long-term assessments only allowed us to conclude on the immediate and short-term effects of feedback.
5Conclusion
Extrinsic feedback is a valuable complementary tool for the management of WRMSDs and future studies should further evaluate its clinical applications in prevention and workplace rehabilitation.
Ethical approval
Not applicable (systematic review).
Informed consent
Not applicable (systematic review).
Conflict of interest
The authors declare that they have no conflict of interest.
Acknowledgments
The authors would like to thank Marie-Marthe Gagnon, librarian, for her assistance in database searches, Isabelle Poitras for her advice and Émilie Champagne for her involvement.
Funding
AF is supported by a scholarship the Fonds de recherche du Québec-Santé. J-SR is supported by a career award from the Fonds de recherche du Québec-Santé.
References
[1] | McGee R , Beven S , Quadrello T . Fit For Work? Musculoskeletal Disorders and the Canadian Labour Market. The work fondation; (2009) . |
[2] | Punnett L , Wegman DH . Work-related musculoskeletal disorders: The epidemiologic evidence and the debate. J Electromyogr Kinesiol. (2004) ;14: (1):13–23. |
[3] | Geneen LJ , Moore RA , Clarke C , Martin D , Colvin LA , Smith BH . Physical activity and exercise for chronic pain in adults: An overview of Cochrane Reviews. Cochrane Pain, Palliative and Supportive Care Group, éditeur. Cochrane Database Syst Rev [Internet]. 24 avr 2017 [cité 29 janv 2021]; Disponible sur: http://doi.wiley.com/10.1002/14651858.CD011279.pub3 |
[4] | Meyer C , Denis CM , Berquin AD . Secondary prevention of chronic musculoskeletal pain: A systematic review of clinical trials. Ann Phys Rehabil Med. (2018) ;61: (5):323–38. |
[5] | Paige NM , Miake-Lye IM , Booth MS , Beroes JM , Mardian AS , Dougherty P , et al. Association of spinal manipulative therapy with clinical benefit and harm for acute low back pain: Systematic review and meta-analysis. JAMA. (2017) ;317: (14):1451. |
[6] | Don S , Voogt L , Meeus M , Kooning MD , Nijs J . Sensorimotor incongruence in people with musculoskeletal pain: A systematic review. Pain Pract. (2017) ;17: (1):115–28. |
[7] | Sterling M , Jull G , Vicenzino B , Kenardy J , Darnell R . Development of motor system dysfunction following whiplash injury. Pain. (2003) ;103: (1):65–73. |
[8] | Sung W , Abraham M , Plastaras C , Silfies SP . Trunk motor control deficits in acute and subacute low back pain are not associated with pain or fear of movement. Spine J Août. (2015) ;15: (8):1772–82. |
[9] | Nijs J , Meeus M , Van Oosterwijck J , Roussel N , De Kooning M , Ickmans K , et al. Treatment of central sensitization in patients with ‘unexplained’ chronic pain: What options do we have? Expert Opin Pharmacother. (2011) ;12: (7):1087–98. |
[10] | Roy JS , Bouyer LJ , Langevin P , Mercier C . Beyond the joint: The role of central nervous system reorganizations in chronic musculoskeletal disorders. (2017) ;5. |
[11] | Charlton JM , Eng JJ , Li LC , Hunt MA . Learning gait modifications for musculoskeletal rehabilitation: Applying motor learning principles to improve research and clinical implementation. Phys Ther. (2021) ;101: (2):pzaa207. |
[12] | Ribeiro DC , Sole G , Haxby Abbott J , Milosavljevic S . A rationale for the provision of extrinsic feedback towards management of low back pain. Man Ther. (2011) ;16: (3):301–5. |
[13] | Magill RA , Anderson D . Motor learning and control: Concepts and applications. Tenth edition. New York, NY: McGraw-Hill; (2014) . |
[14] | Schmidt RA , Lee TD , Winstein CJ , Wulf G , Zelaznik HN . Motor control and learning. A behavioral emphasis. Sixth.Human Kinetics Publishers; (2019) . (Human Kinetics Publishers.). |
[15] | Schmidt R , Wrisberg C . Motor learning and performance: A situation-based learning approach. (2008) . (Human Kinetics Publishers.). |
[16] | Marschall F , Bund A , Wiemeyer J . Does Frequent Augmented Feedback Really Degrade Laerning? A mete-Analysis. E-J Beweg Train. (2007) ;1: :75–86. |
[17] | Sigrist R , Rauter G , Riener R , Wolf P . Augmented visual, auditory, haptic, and multimodal feedback in motor learning: A review. Psychon Bull Rev. (2013) ;20: (1):21–53. |
[18] | Kennedy CA , Amick III BC , Dennerlein JT , Brewer S , Catli S , Williams R , et al. Systematic review of the role of occupational health and safety interventions in the prevention of upper extremity musculoskeletal symptoms, signs, disorders, injuries, claims and lost time. J Occup Rehabil. (2010) ;20: (2):127–62. |
[19] | Van Eerd D , Munhall C , Irvin E , Rempel D , Brewer S , van der Beek AJ , et al. Effectiveness of workplace interventions in the prevention of upper extremity musculoskeletal disorders and symptoms: An update of the evidence. Occup Environ Med. (2016) ;73: (1):62–70. |
[20] | Hoosain M , de Klerk S , Burger M . Workplace-based rehabilitation of upper limb conditions: A systematic review. J Occup Rehabil. (2019) ;29: (1):175–93. |
[21] | Liberati A , Altman DG , Tetzlaff J , Mulrow C , Gøtzsche PC , Ioannidis JPA , et al. The PRISMA statement for reporting systematic reviews and meta-analyses of studies that evaluate health care interventions: Explanation and elaboration. J Clin Epidemiol. (2009) ;62: (10):e1–34. |
[22] | Kellermeyer L , Harnke B , Knight S . Covidence and rayyan. J Med Libr Assoc [Internet]. 2018. [cité 10 sept 2020];106(4). Disponible sur. http://jmla.pitt.edu/ojs/jmla/article/view/513 |
[23] | Thivel D , Tremblay A , Genin PM , Panahi S , Rivière D , Duclos M . Physical activity, inactivity, and sedentary behaviors: Definitions and implications in occupational health. Front Public Health. (2018) ;6: :288. |
[24] | Downs SH , Black N . The feasibility of creating a checklist for the assessment of the methodological quality both of randomised and non-randomised studies of health care interventions. J Epidemiol Community Health. (1998) ;52: (6):377–84. |
[25] | Gwet KL . Computing inter-rater reliability and its variance in the presence of high agreement. Br J Math Stat Psychol. (2008) ;61: (1):29–48. |
[26] | Hootman JM , Driban JB , Sitler MR , Harris KP , Cattano NM . Reliability and validity of three quality rating instruments for systematic reviews of observational studies. Res Synth Methods. (2011) ;2: (2):110–8. |
[27] | O’Connor SR , Tully MA , Ryan B , Bradley JM , Baxter GD , McDonough SM . Failure of a numerical quality assessment scale to identify potential risk of bias in a systematic review: A comparison study. BMC Res Notes. (2015) ;8: (1):224. |
[28] | Patricio P , Roy JS , Rohel A , Gariépy C , Emond C , Hamel E , et al. The effect of noninvasive brain stimulationto reduce nonspecific low back pain: A systematic review and meta-analysis. Clin J Pain. (2021) ;37: (6):475. |
[29] | van Tulder M , Furlan A , Bombardier C , Bouter L . Updated method guidelines for systematic reviews in the cochrane collaboration back review group: Spine. (2003) ;28: (12):1290–9. |
[30] | Schünemann HJ , Higgins J , Vist G , Glasziou P , Skoetz N , Guyatt G . Cochrane Handbook for Systematic Reviews of Interventions version 6.1 (updated September 2020). Chapter 14: Completing ‘Summary of findings’ tables and grading the certainty of the evidence. [Internet]. The Cochrane Collaboration; 2020. Disponible sur: http://www.training.cochrane.org/handbook |
[31] | Guyatt GH , Oxman AD , Vist GE , Kunz R , Falck-Ytter Y , Alonso-Coello P , et al. GRADE: An emerging consensus on rating quality of evidence and strength of recommendations. BMJ. (2008) ;336(7650):924–6. |
[32] | Poitras I , Bielmann M , Campeau-Lecours A , Mercier C , Bouyer LJ , Roy JS . Validity of wearable sensors at theshoulder joint: Combining wireless electromyography sensors and inertial measurement units to perform physical workplace assessments. Sensors. (2019) ;19: (8):1885. |
[33] | Brandenburg DL , Mirka GA . Assessing the effects of positive feedback and reinforcement in the introduction phase of an ergonomic intervention. Hum Factors J Hum Factors Ergon Soc. (2005) ;47: (3):526–35. |
[34] | Oppici L , Grutters K , Garofolini A , Rosenkranz R , Narciss S . Deliberate practice and motor learning principles to underpin the design of training interventions for improving lifting movement in the occupational sector: A perspective and a pilot study on the role of augmented feedback. Front Sports Act Living. (2021) ;3(101765780):746142. |
[35] | Ribeiro DC , Sole G , Abbott JH , Milosavljevic S . The effectiveness of a lumbopelvic monitor and feedback device to change postural behavior: A feasibility randomized controlled trial. J Orthop Sports Phys Ther. (2014) ;44: (9):702–11. |
[36] | McAtamney L , Nigel Corlett E . RULA: A survey method for the investigation of work-related upper limb disorders. Appl Ergon. (1993) ;24: (2):91–9. |
[37] | Vignais N , Miezal M , Bleser G , Mura K , Gorecky D , Marin F . Innovative system for real-time ergonomic feedback in industrial manufacturing. Appl Ergon. (2013) ;44: (4):566–74. |
[38] | Henning RA , Callaghan EA , Ortega AM , Kissel GV , Guttman JI , Braun HA . Continuous feedback to promote self-management of rest breaks during computer use. Int J Ind Ergon. (1996) ;18: (1):71–82. |
[39] | Gerard MJ , Armstrong TJ , Rempel DA , Woolley C . Short term and long term effects of enhanced auditory feedback on typing force, EMG, and comfort while typing. Appl Ergon. (2002) ;33: (2):129–38. |
[40] | Lind CM , Diaz-Olivares JA , Lindecrantz K , Eklund J . A wearable sensor system for physical ergonomics interventions using haptic feedback. Sensors. (2020) ;20: (21):6010. |
[41] | Vedsted P , Søgaard K , Blangsted AK , Madeleine P , Sjøgaard G . Biofeedback effectiveness to reduce upper limb muscle activity during computer work is muscle specific and time pressure dependent. J Electromyogr Kinesiol. (2011) ;21: (1):49–58. |
[42] | Gaffney BM , Maluf KS , Davidson BS . Evaluation of novel EMG biofeedback for postural correction during computer use. Appl Psychophysiol Biofeedback. (2016) ;41: (2):181–9. |
[43] | Boocock M , Naudé Y , Taylor S , Kilby J , Mawston G . Influencing lumbar posture through real-time biofeedbackand its effects on the kinematics and kinetics of a repetitive lifting task. Gait Posture. (2019) ;73: :93–100. |
[44] | Owlia M , Kamachi M , Dutta T . Reducing lumbar spine flexion using real-time biofeedback during patient handling tasks. Work. (2020) ;66: (1):41–51. |
[45] | Pinto BL , Beaudette SM , Brown SHM . Tactile cues can change movement: An example using tape to redistribute flexion from the lumbar spine to the hips and knees during lifting. Hum Mov Sci. (2018) ;60: :32–9. |
[46] | Agruss C , Williams K , Fathallah F . The effect of feedback training on lumbosacral compression during simulated occupational lifting. Ergonomics. (2004) ;47: (10):1103–15. |
[47] | Chan VCH , Welsh TN , Tremblay L , Frost DM , Beach TAC . A comparison of augmented feedback and didactic training approaches to reduce spine motion during occupational lifting tasks. Appl Ergon [Internet]. 2022;99((Chan VCH, Welsh TN, Tremblay L, Frost DM) Faculty of Kinesiology and Physical Education, University of Toronto, Toronto, ON, Canada). Disponible sur: https://www.embase.com/search/results?subaction=viewrecord&id=L2015314784&from=export |
[48] | Ferrone A , Garcia Patino A , Menon C . Low back pain-behavior correction by providing haptic feedbacks: A preliminary investigation. Sensors. (2021) ;21(21). |
[49] | Kamachi M , Owlia M , Dutta T . Evaluating a wearable biofeedback device for reducing end-range sagittal lumbar spine flexion among home caregivers. Appl Ergon [Internet]. 2021;97((Kamachi M, Owlia M, Dutta T, tilak.dutta@uhn.ca) KITE Research Institute, Toronto Rehabilitation Institute, University Health Network, 550 University Ave., Toronto, ON, Canada). Disponible sur: https://www.embase.com/search/results?subaction=viewrecord&id=L2013915631&from=export |
[50] | Bazazan A , Dianat I , Feizollahi N , Mombeini Z , Shirazi AM , Castellucci HI . Effect of a posture correction–based intervention on musculoskeletal symptoms and fatigue among control room operators. Appl Ergon. (2019) ;76: :12–9. |
[51] | Partido BB , Wright BM . Self-assessment of ergonomics amongst dental students utilising photography: RCT. Eur J Dent Educ. (2018) ;22: (4):223–33. |
[52] | Partido BB , Henderson RP , Kennedy M . Improving the awareness of musculoskeletal disorder risks among dental educators. J Dent Educ. (2020) ;84: (1):5–12. |
[53] | Alavosius MP , Sulzer-Azaroff B . The effects of performance feedback on the safety of client lifting and transfer. J Appl Behav Anal. (1986) ;19: (3):261–7. |
[54] | Alavosius MP , Sulzer-Azaroff B . Acquisition and maintenance of health-care routine as a function of feedback density. J Appl Behav Anal. (1990) ;23: (2):151–62. |
[55] | Sharma J , Weston M , Batterham AM , Spears IR . Gait retraining and incidence of medial tibial stress syndrome in army recruits: Med sci sports exerc. (2014) ;46: (9):1684–92. |
[56] | Ettare D , Ettare R . Clinical EMG for Surface Recordings, II. Muscle learning therapy: A treatment protocol. Cram JR. Nevada City; 1990, pp. 197-234. |
[57] | Faucett J , Garry M , Nadler D , Ettare D . A test of two training interventions to prevent work-related musculoskeletal disorders of the upper extremity. Appl Ergon. (2002) ;33: (4):337–47. |
[58] | Mansfield N , Naddeo A , Frohriep S , Vink P . Integrating and applying models of comfort. Appl Ergon. (2020) ;82: :102917. |
[59] | Roossien CC , Stegenga J , Hodselmans AP , Spook SM , Koolhaas W , Brouwer S , et al. Can a smart chair improve the sitting behavior of office workers? Appl Ergon. (2017) ;65: :355–61. |
[60] | Amick BC , Robertson MM , DeRango K , Bazzani L , Moore A , Rooney T , et al. Effect of office ergonomics intervention on reducing. Spine. (2003) ;28: (24):2706–11. |
[61] | King TK , Severin CN , Van Eerd D , Ibrahim S , Cole D , Amick B , et al. A pilot randomised control trial of the effectiveness of a biofeedback mouse in reducing self-reported pain among office workers. Ergonomics. (2013) ;56: (1):59–68. |
[62] | Blasche G , Pfeffer M , Thaler H , Gollner E . Work-site health promotion of frequent computer users: Comparing selected interventions. Work. (2013) ;46: (3):233–41. |
[63] | De Kraker H , De Korte EM , Van Mil FLK , Rijs BP , Bongers PM . The effect of a feedback signal in a computer mouse on hovering behaviour, productivity, comfort and usability in a field study. Ergonomics. (2008) ;51: (2):140–55. |
[64] | Thomas RE , Vaidya SC , Herrick RT , Congleton JJ . The effects of biofeedback on carpal tunnel syndrome. Ergonomics. (1993) ;36: (4):353–61. |
[65] | Yoo WG , Yi CH , Kim MH . Effects of a proximity-sensing feedback chair on head, shoulder, and trunk postures when working at a visual display terminal. J Occup Rehabil. (2006) ;16: (4):631–7. |
[66] | Thanathornwong B , Suebnukarn S . The improvement of dental posture using personalized biofeedback. IOS Press. (2015) ;216: :756–60. |
[67] | Holtermann A , Søgaard K , Christensen H , Dahl B , Blangsted AK . The influence of biofeedback training on trapezius activity and rest during occupational computer work: A randomized controlled trial. Eur J Appl Physiol. (2008) ;104: (6):983–9. |
[68] | Lorenz EP , Lavender SA , Andersson GBJ . Determining what should be taught during lift-training instruction. Physiother Theory Pract. (2002) ;18: (4):175–91. |
[69] | Levanon Y , Gefen A , Lerman Y , Givon U , Ratzon NZ . Reducing musculoskeletal disorders among computer operators: Comparison between ergonomics interventions at the workplace. Ergonomics. (2012) ;55: (12):1571–85. |
[70] | Vernon H , Mior S . The Neck Disability Index: A study of reliability and validity. J Manipulative Physiol Ther. (1991) ;14: (7):409–15. |
[71] | Iqbal ZA , Alghadir AH , Anwer S . Efficacy of deep cervical flexor muscle training on neck pain, functionaldisability, and muscle endurance in school teachers: A clinical trial. Kaya D, éditeur. BioMed Res Int. (2021) ;2021:1–6. |
[72] | Iqbal ZA , Rajan R , Khan SA , Alghadir AH . Effect of deep cervical flexor muscles training using pressure biofeedback on pain and disability of school teachers with neck pain. J Phys Ther Sci. (2013) ;25: (6):657–61. |
[73] | Ilmarinen J . The work ability index (WAI). Occup Med. (2006) ;57: (2):160–160. |
[74] | Dellve L , Ahlstrom L , Jonsson A , Sandsjö L , Forsman M , Lindegård A , et al. Myofeedback training andintensive muscular strength training to decrease pain and improve work ability among female workers on long-termsick leave with neck pain: A randomized controlled trial. Int Arch Occup Environ Health. (2011) ;84: (3):335–46. |
[75] | Roy JS , MacDermid JC , Woodhouse LJ . Measuring shoulder function: A systematic review of four questionnaires. Arthritis Care Res. (2009) ;61: (5):623–32. |
[76] | Dos Santos C , Jones MA , Matias R . Short- and long-term effects of a scapular-focused exercise protocol for patients with shoulder dysfunctions-A prospective cohort. Sensors. (2021) ;21(8). |
[77] | Mintken PE , Cleland JA , Whitman JM , George SZ . Psychometric properties of the fear-avoidance beliefs questionnaire and tampa scale of kinesiophobia in patients with shoulder pain. Arch Phys Med Rehabil. (2010) ;91: (7):1128–36. |
[78] | Alghadir AH , Iqbal ZA . Effect of Deep Cervical Flexor Muscle Training Using Pressure Biofeedback on Pain and Forward Head Posture in School Teachers with Neck Pain: An Observational Study. BioMed Res Int [Internet]. 2021;2021((Alghadir A.H., aha@ksu.edu.sa; Iqbal Z.A., z_iqbal001@yahoo.com) Rehabilitation Research Chair, College of Applied Medical Sciences, King Saud University, Riyadh, Saudi Arabia). Disponible sur: https://www.embase.com/search/results?subaction=viewrecord&id=L2012833414&from=export |
[79] | Nezamuddin Md , Anwer S , Khan SA , Equebal A . Efficacy of pressure biofeedback guided deep cervical flexor training on neck pain and muscle performance in visual display terminal operators. J Musculoskelet Res. (2013) ;16: (03):1350011. |
[80] | Spence SH . Cognitive-behavior therapy in the management of chronic, occupational pain of the upper limbs. Behav Res Ther. (1989) ;27: (4):435–46. |
[81] | Von Korff M , Deyo RA , Cherkin D , Barlow W . Back pain in primary care. Outcomes at 1 Year. (1993) ;18: (7):855–62. |
[82] | Gottlieb BS . Development of the Pain Beliefs Questionnaire: A preliminary report. In: Annual Conference of the Association for the Advancement of Behaviour Therapy. Philadelphia, USA; (1984) . |
[83] | Kuo YL , Wang PS , Ko PY , Huang KY , Tsai YJ . Immediate effects of real-time postural biofeedback on spinal posture, muscle activity, and perceived pain severity in adults with neck pain. Gait Posture. (2019) ;67: :187–93. |
[84] | Poiraudeau S , Rannou F , Revel M . Functional restoration programs for low back pain: A systematic review. Ann Réadapt Médecine Phys. (2007) ;50: (6):425–9. |
[85] | Colloca CJ , Hinrichs RN . The biomechanical and clinical significance of the lumbar erector spinae flexion-relaxation phenomenon: A review of literature. J Manipulative Physiol Ther. (2005) ;28: (8):623–31. |
[86] | Neblett R , Mayer TG , Brede E , Gatchel RJ . Correcting abnormal flexion-relaxation in chronic lumbar pain: Responsiveness to a new biofeedback training protocol. Clin J Pain. (2010) ;26: (5):403–9. |
[87] | Golebowicz M , Levanon Y , Palti R , Ratzon NZ . Efficacy of a telerehabilitation intervention programme using biofeedback among computer operators. Ergonomics. (2015) ;58: (5):791–802. |
[88] | Sandsjö L , Larsman P , Huis in ’t Veld RM , Vollenbroek-Hutten MM . Clinical evaluation of a myofeedback-based teletreatment service applied in the workplace: A randomized controlled trial. J Telemed Telecare. (2010) ;16: (6):329–35. |
[89] | Pollard CA . Preliminary validity study of the pain disability index. Percept Mot Skills. (1984) ;59: (3):974–974. |
[90] | Ma C , Szeto GP , Yan T , Wu S , Lin C , Li L . Comparing biofeedback with active exercise and passive treatment for the management of work-related neck and shoulder pain: A randomized controlled trial. Arch Phys Med Rehabil. (2011) ;92: (6):849–58. |
[91] | Voerman GE , Sandsjö L , Vollenbroek-Hutten MMR , Larsman P , Kadefors R , Hermens HJ . Effects of ambulant myofeedback training and ergonomic counselling in female computer workers with work-related neck-shoulder complaints: A randomized controlled trial. J Occup Rehabil. (2007) ;17: (1):137–52. |
[92] | Kuorinka I , Andersson G . Standardised nordic questionnaires for the analysis. Appl Ergon. (1987) ;18.3: (18.3):233–7. |
[93] | Park SH . Tools for assessing fall risk in the elderly: A systematic review and meta-analysis. Aging Clin Exp Res. (2018) ;30: (1):1–16. |
[94] | Ribeiro DC , Milosavljevic S , Terry J , Abbott JH . Effectiveness of a lumbopelvic monitor and feedback device to change postural behaviour: The ELF cluster randomised controlled trial. Occup Environ Med. (2020) ;77: (7):462–9. |
[95] | Faucett J . Integrating ‘psychosocial’ factors into a theoretical model for work-related musculoskeletal disorders. Theor Issues Ergon Sci. (2005) ;6: (6):531–50. |
[96] | Kristensen TS . Intervention studies in occupational epidemiology. Occup Environ Med. (2005) ;62: (3):205–10. |
[97] | Noonan J , Wagner SL . A biopsychosocial perspective on the management of work-related musculoskeletal disorders. AAOHN J. (2010) ;58: (3):105–14. |
[98] | Vinstrup J , Sundstrup E , Andersen LL . Psychosocial stress and musculoskeletal pain among senior workers from nine occupational groups: Cross-sectional findings from the Senior Working Life study. BMJ Open. (2021) ;11: (3):e043520. |
[99] | Stock SR , Nicolakakis N , Vézina N , Vézina M , Gilbert L , Turcot A , et al. Are work organizationinterventions effective in preventing or reducing work-related musculoskeletal disorders? A systematic review ofthe literature. Scand J Work Environ Health [Internet]. 2017 [cité 3 juill 2020]; Disponible sur: http://www.sjweh.fi/show_abstract.php?abstract_id=3696 |
[100] | Agresta C , Brown A . Gait retraining for injured and healthy runners using augmented feedback: A systematic literature review. J Orthop Sports Phys Ther. (2015) ;45: (8):576–84. |
[101] | Armitano CN , Haegele JA , Russell DM . The use of augmented information for reducing anterior cruciate ligament injury risk during jump landings: A systematic review. J Athl Train. (2018) ;53: (9):844–59. |
[102] | Taibi Y , Metzler YA , Bellingrath S , Müller A . A systematic overview on the risk effects of psychosocial work characteristics on musculoskeletal disorders, absenteeism, and workplace accidents. Appl Ergon. (2021) ;95: :103434. |
[103] | Okunribido OO , Wynn T , Lewis D . Are older workers at greater risk of musculoskeletal disorders in the workplace than young workers? – A literature review. Occup Ergon. (2011) ;10: (1-2):53–68. |
[104] | Frutiger M , Res M , Borotkanics R . Systematic review and meta-analysis suggest strength training and workplace modifications may reduce neck pain in office workers. Pain Pract. (2020) ;21: (1):130–1. |
[105] | Sundstrup E . A systematic review of workplace interventions to rehabilitate musculoskeletal disorders among employees with physical demanding work. J Occup Rehabil. (2020) ;25. |