Effects of a chair for ophthalmic microsurgery on pressure distribution and pelvic tilt in surgeons
Abstract
BACKGROUND:
Ophthalmic surgeons experience high levels of physical strain in the neck, lower back, and buttocks. While ergonomic interventions may help to solve these problems, only a few studies have reported chair designs for ophthalmic microsurgery.
OBJECTIVE:
To design a chair that reduces the physical strain on surgeons and examine its effectiveness in improving posture and reducing seat pressure.
METHODS:
A prototype chair with a three-dimensional seat surface and a sliding adjustment mechanism for the backrest was designed to fit the surgeon’s body. A conventional chair (A) and the prototype chair (B) were compared during microsurgeries performed by five surgeons. Seat pressure was measured using a pressure-sensing device, and the pelvic tilt angle was measured using a gyroscope sensor.
RESULTS:
A paired t-test indicated significant differences between the chairs: average seat pressure was 70.4±12.7 mmHg for A and 40.5±3.8 mmHg for B (p = 0.008); the maximum seat pressure was 242.2±19.7 mmHg for A and 170.5±38.5 mmHg for B (p = 0.024); contact area was 906.1±114.5 cm2 for A and 1,255.9±60.1 cm2 for B (p < 0.001); and relative value of the pelvic tilt angle was –13.7°±3.7° for A and –7.1°±4.9° for B (p = 0.032).
CONCLUSIONS:
The prototype chair was associated with lower seat pressure and maintenance of a more neutral posture than the conventional chair, indicating that it may help to reduce physical strain in ophthalmic surgeons.
1Introduction
Ophthalmic microsurgery using a microscope has allowed surgeons to treat micrometer-sized lesions, facilitating the management of various ocular diseases and recovery of visual function. Concurrent advancements in the performance of medical devices and improvements in surgical skill and clinical experience have further contributed to positive treatment outcomes [1]. However, ophthalmic surgeons are exposed to many physical demands while operating, which involves sitting on a chair while observing the surgical field under a microscope, performing repetitive movements of the hands and fingers, operating the foot switch, and occasionally moving the microscope vertically with the trunk and upper limbs (Fig. 1A). As a result, the surgeon’s body movement is restricted by the position of the microscope eyepiece, which is located at the site of surgery. The resultant excessive tension in the surgeon’s upper body may lead to physical strain, which may in turn affect performance and the quality of the procedure. Additionally, such strain may affect the longevity and quality of the surgeon’s career. Previous studies [1, 2] have highlighted several seat-related problems that may increase the risk of musculoskeletal disorders in surgeons, including sustained foot elevation; thighs/buttocks compression; lack of support for the forearm; excessive neck flexion; inability to recline; lack of support for the pelvis and back; prolonged rigid posture; and subjective fatigue of the neck, shoulders, and buttocks.
Fig. 1
(A) Image showing surgery using the conventional ophthalmic microscope. (B) Image showing surgery performed through a heads-up display system.
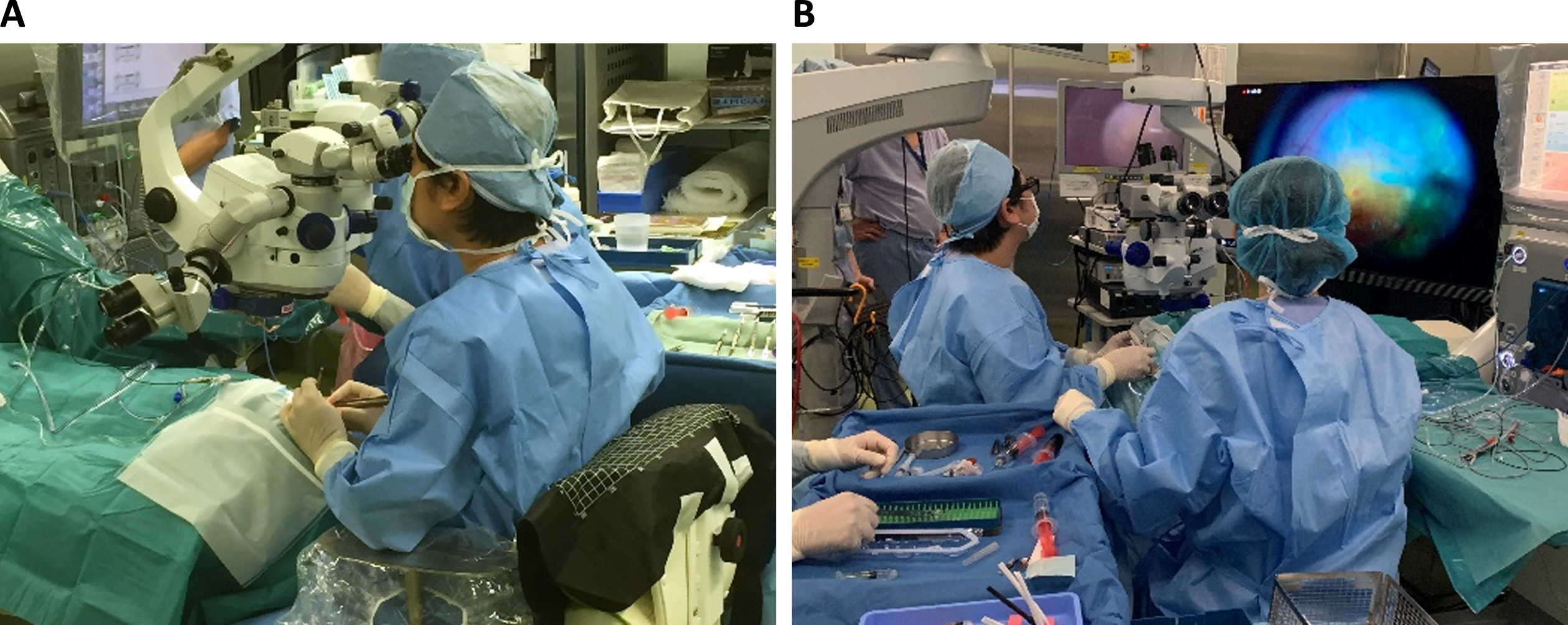
An analysis of data for 697 ophthalmologists indicated that the self-reported prevalence of neck, upper body, or lower back symptoms in the previous month was 51.8%, while the corresponding values for low back pain, upper-extremity symptoms, and neck pain were 39%, 32.9%, and 32.6%, respectively [3]. Approximately 15% of ophthalmologists experienced slight to moderate limitations in their work because of these symptoms [3]. Another survey of 518 ophthalmologists indicated that 57.1% used anti-inflammatory drugs, 40% required physiotherapy, and 5% underwent surgery for low back pain [4]. Furthermore, a study of 127 ophthalmologists indicated that surgeons with self-reported pain in the neck, upper/lower back, and upper extremity spent significantly longer time (hours/week) in surgery than those without pain (mean: 7.9 vs. 5.3 hours/week), suggesting an association between musculoskeletal pain and the time spent performing surgery [5].
Traditional ergonomic interventions, including instrument and device optimization and appropriate education, can limit the prevalence of musculoskeletal pain among eye care professionals [6]. The “Ergonomics Best Practice” course offered by the American Academy of Ophthalmology Ergonomics Task Force recommends stretches, daily posture improvement, and workplace modifications [7]. Several studies have demonstrated the effectiveness and benefits of such practices for surgeons in other disciplines. These ergonomic strategies include intraoperative microbreaks [8, 9], appropriate monitor positioning [10], custom-built ergonomic chairs for robotic-assisted laparoscopy [11], and a prototype open console for use during robotic surgery [12]. Recent innovations have enabled the development of heads-up digital visualization systems, which have been associated with ergonomic benefits such as improved neck, back, and visual comfort in ophthalmic surgeons [13–18]. Figure 1B presents an image of heads-up surgery1 in the operating room. The surgeon, who is wearing polarized glasses, performs the procedure while viewing the image displayed on a large screen using a three-dimensional camera. (Microscopic eyepieces are not used during surgery.) The heads-up digital visualization system allows freedom of neck and body movement, resulting in minimal physical strain. However, unless the current chair design is improved, the physical load on the surgeon’s buttocks, which reflects the pressure around the ischial tuberosity, will remain high even with the installation of a heads-up digital visualization system. Furthermore, since heads-up and conventional microscopic techniques are used in combination depending on the surgical procedure, the surgical chair must be able to accommodate both techniques [19–21].
Sitting comfort and discomfort are independent entities associated with different factors: Discomfort is related to pain/biomechanics and fatigue-related factors, while comfort is related to a sense of well-being and esthetics [22, 23]. Previous literature reviews have emphasized the lack of objective measures for evaluating sitting comfort [24]. A subsequent review on sitting comfort and discomfort and their relationships with objective measures—including posture analysis results, pressure measurements, and electromyography—reported that seat pressure distribution may be the objective measure exhibiting the clearest association with subjective ratings [25]. Another study indicated that peak pressure on the seat pan, pressure distribution on the backrest, and changes in the pattern of pressure appear to be reliable measures for quantifying comfort or discomfort [26]. The seat pressure on the buttocks generally ranges from 0 to 1.28 psi (unit conversion: 66.2 mmHg) [27], and values less than 50 mmHg are referred to as the “sitting comfort zone” [28]. A three-dimensional seat surface referred to as a “high-performance seat pan” has been proposed to increase the contact area and optimize seat pressure distribution [29]. The seat surface is divided into the lumbosacral region, buttocks, and thighs based on differences in composition and volume, and this concept has been applied to the design of the surface for surgical chairs [2]. Although the application differs, the seat position in a three-dimensional vehicle seat has also been reported to satisfy the ideal pressure distribution and thereby facilitate the reduction of lumbar disc pressure [30, 31].
The angle of the acetabulofemoral joint affects pelvic rotation and, consequently, lumbar curvature when seated [32, 33]. Traditional ergonomic chairs with a forward-tilted seat surface (tilting or kneeling chairs) aim to limit hip flexion and promote lumbar lordosis [33], including the Opsvik Balans chair [34], knee support [35], and the dynamic saddle chair known as “Back App” [36]. Tilting chairs may benefit surgeons, but an extremely forward-tilting seat may cause slippage of the buttocks, and knee support may interfere with the manipulation of the foot switch required for ophthalmic microsurgery. An alternative option is a wedge-shaped support placed at the rear end of the seat surface, called a “pelvic support,” which prevents the pelvis from rotating backward and stabilizes the pelvic area [37]. A convex support attached to the backrest commonly referred to as “lumbar support,” contributes to the maintenance of lumbar lordosis in the sitting position [38]. A reclining backrest with lumbar support improves intervertebral disc pressure and muscle activity in the lower back [39, 40] as well as subjective comfort [41]. While the backrest can reduce the strain on the lower back, a flat and hard design cannot fit the shape and natural movement of the human back. A more dynamic design is required for the chair backrest not only to support the human body but also to avoid interference with the natural movement of the back [42]. A dynamic backrest design, such as that found in ergonomic office chairs, aims to concurrently solve the conflicting issues of support and movement. Such backrests may prevent the surgeon from adopting a rigid posture during surgery and maximize the benefits of heads-up surgery in terms of the freedom of body movement. However, their structures and functions are complex, and the manufacturers of such chairs are limited.
Thus, in the present study, we aimed to design a chair that reduces physical strain in ophthalmic surgeons and examine its effectiveness in improving posture and reducing seat pressure. A chair specifically developed to fit the human body with a three-dimensional seat surface and adjustable functions would theoretically facilitate the reduction of seat pressure and achieve more neutral postures in ophthalmic surgeons [2]. Greater comfort would, in turn, allow surgeons to perform surgery more smoothly, thereby improving the quality of the procedure. Previous studies [20, 21] have reported the design and testing of a prototype chair backrest. In this paper, we report the design of a seat surface and an adjustment mechanism for the seat and backrest to reduce pressure on the thighs/buttocks and enhance the support of the sacral and pelvic regions. A clinical pilot test was also conducted to compare the prototype chair with a conventional chair during microsurgery in the operating room. We hypothesized that the prototype chair would reduce seat pressure and improve posture by preventing backward tilt of the pelvis when compared with the conventional chair.
2Methods
The study and surgical procedures were performed in the operating room at the University of Occupational and Environmental Health, Japan, University Hospital, after obtaining approval from the university’s ethics committee.
2.1Prototyping
An iterative approach was used for prototyping, which involved multiple rounds of design and testing to incorporate each ophthalmic surgeon’s feedback into the design specifications. Production of a prototype chair was conducted by a team that included ergonomists, ophthalmic surgeons, clinical laboratory technologists, designers, and manufacturers. Figure 2 shows a comparison of a conventional chair normally used in an operating room and the prototype chair. For the prototype chair, both the seat and the backrest were changed. The conventional chair had a flat seat surface and no lower support for the backrest (Fig. 2A), whereas the prototype chair featured a concave three-dimensional seat surface and a backrest with two roll supports (Fig. 2B).
Fig. 2
(A) Conventional chair used in the operating room. (B) Prototype chair.
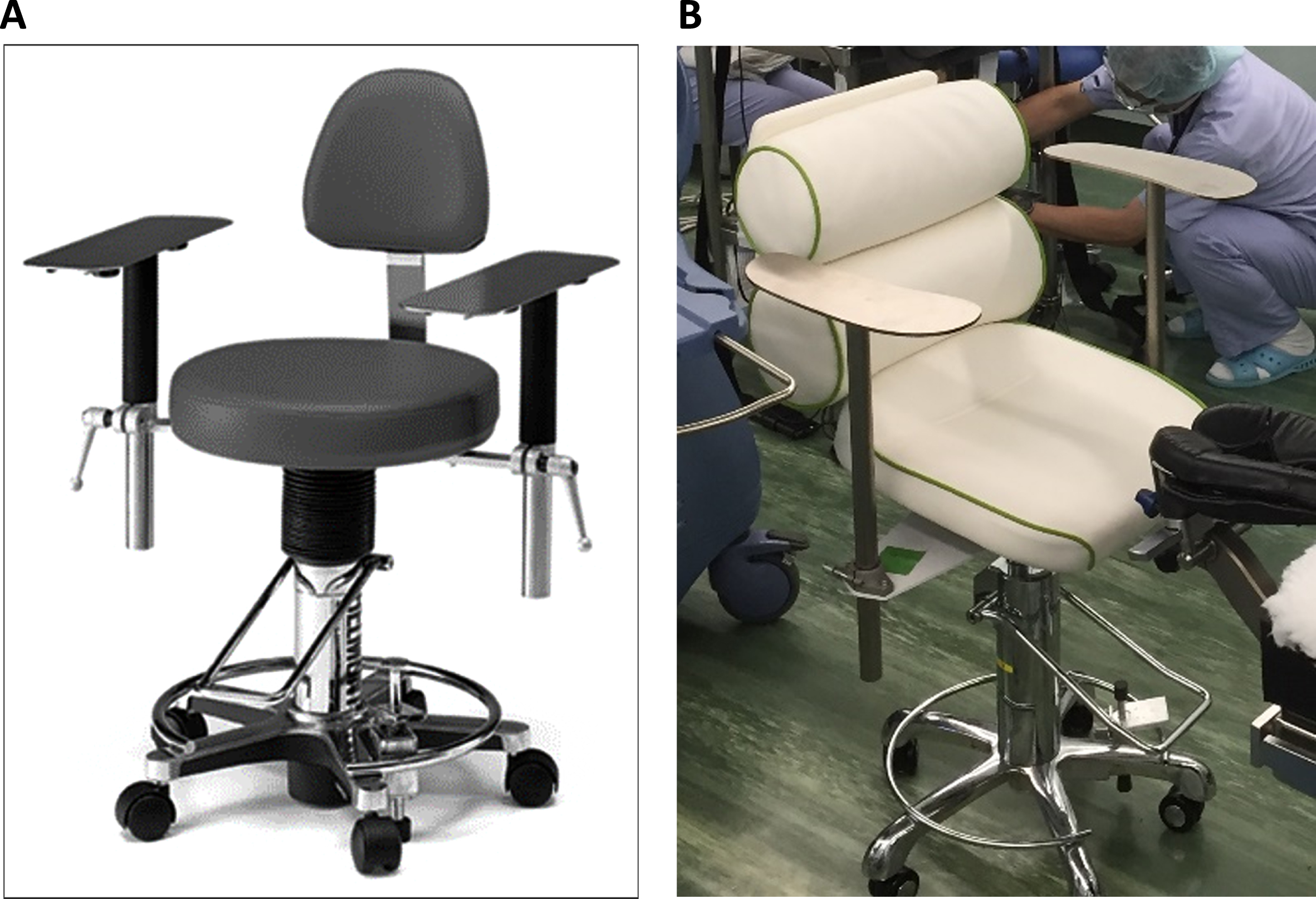
The seat surface of the prototype chair was based on the concept of a high-performance seat pan [2, 29]. The first study model reflects these functions: The wooden seat surface is divided into pelvic, ischial, and thigh zones [43]. The structure of the study model and a pre-scanned model of the adult human male buttocks (close to the 50th- percentile stature) (Fig. 3A) were emulated to create a three-dimensional model of the seat shell (Fig. 3B) using CAD software (Vectorworks, Vectorworks, Inc., Columbia, USA). The size of the seat shell was arranged to fit adult body sizes ranging from the 5th to 95th percentile based on data for the Japanese population [44]: The seat width was 400 mm to satisfy the hip breadth of the 95th-percentile adult male, while the seat depth was 395 mm to satisfy the buttock-popliteal length in the sitting position of the 5th-percentile adult female. The pelvic support, which has a wedged shape with an angle of approximately 20°, was placed at the seat’s rear end to prevent backward tilting of the pelvis, as reported by Wu et al. [37]. Side supports were installed to stabilize the surgeon’s body and distribute pressure. The front edge of the seat was also tilted downward to avoid pressure behind the thighs/knees. The seat surface was molded from fiber-reinforced plastic (Fig. 3C). Plastic entwined in a thread-like structure, which can be processed easily, was used as the main cushioning material instead of urethane (Fig. 3D).
Fig. 3
Prototyping of the seat surface. (A) Overlaid image of the first study model and a model of the buttocks shape. (B) 3D CAD seat shell. (C) Fiber-reinforced plastic seat shell. (D) Plastic coil cushion.
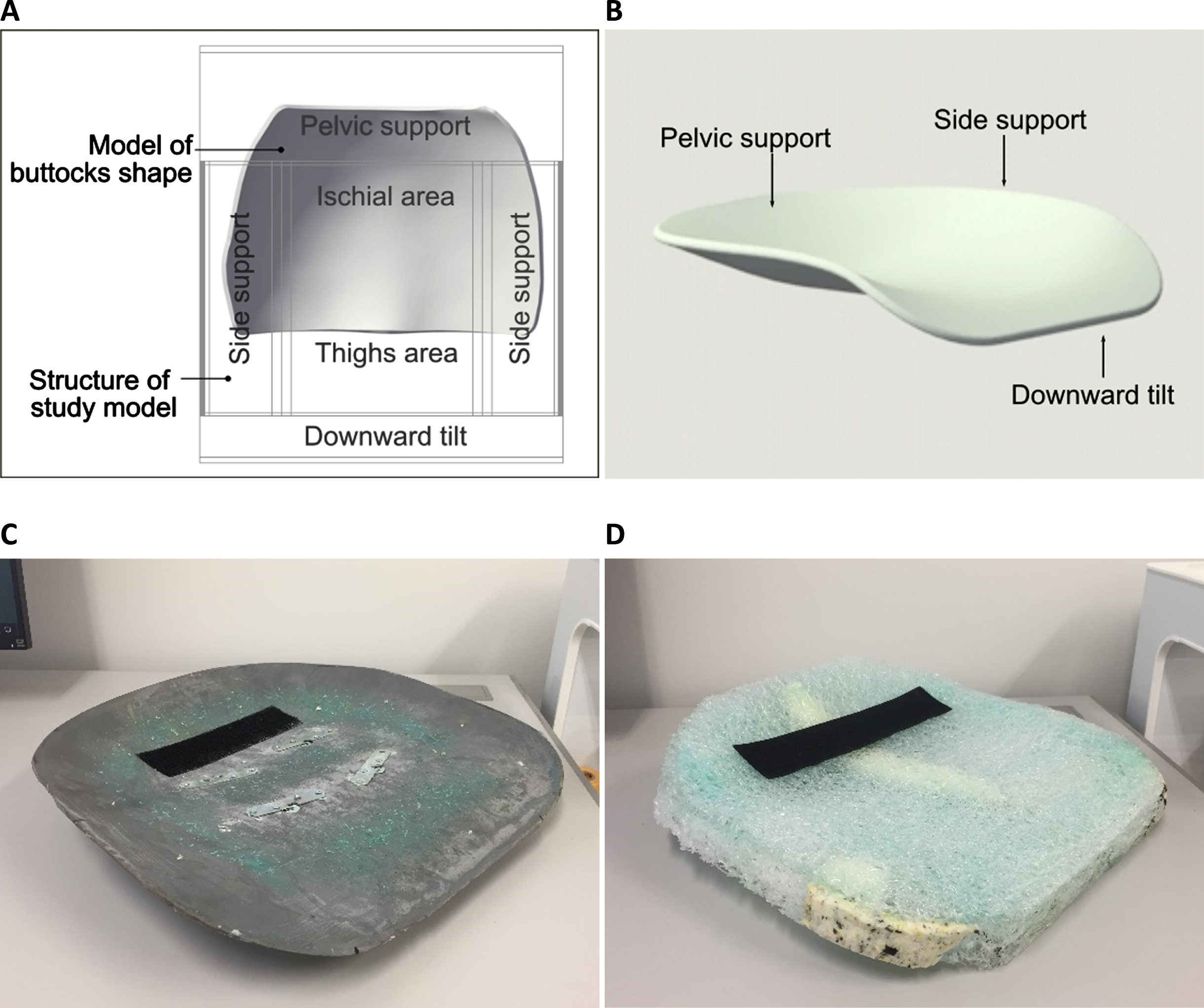
The backrest of the prototype chair consists of two roll supports with different diameters: The upper part supports the surgeon’s lumbar region, while the lower part supports the sacral and pelvic regions. The upper and lower rolls can also be inverted to adapt to both conventional microscopic and heads-up surgery. The functions of the backrest and the results of relevant tests have been reported in the literature [20, 21]. The novelty of the prototype chair is the incorporation of a sliding adjustment mechanism for the backrest (Fig. 4A), which enhances support in the sacral and pelvic regions. The sliding mechanism can fill the gap that tends to occur between the buttocks and the joint of the seat surface and backrest (Fig. 4B). When the backrest slides to fill the gap, the two types of stress shown in Fig. 4C are generated, allowing the surgeon to maintain a neutral posture. A vertical force (Fig. 4C-a) pushes the pelvis upward on the pelvic support of the seat surface, whereas a horizontal force (Fig. 4C-b) pushes the sacral region forward on the backrest, thereby enhancing the effect of the vertical force and preventing backward tilting of the pelvis in the sitting position. The slide mechanism also addresses the issue of “shallow sitting,” which occurs when surgeons almost never use the backrest since the seat depth can be adjusted according to individual differences in thigh length. An important aspect of this design is the ability to adjust the shaft of the backrest mounted on the rear side of the seat surface. Depth adjustment can be found in other chairs, but it is less common in the backrest.
Fig. 4
Sliding adjustment mechanism for the backrest. (A) View from below the seat surface. The backrest can be adjusted forward/backward and fixed with bolts below the seat surface. (B) Image showing a surgeon sitting in the prototype chair. (C) Image focusing on the area between the buttocks and the joint connecting the seat surface and backrest. The two types of stress generated by the slide mechanism for the backrest are shown. ‘a’ indicates the vertical force pushing the pelvis upward on the pelvic support in the seat surface, whereas ‘b’ indicates the horizontal force to pushing the sacrum region forward on the backrest, which enhances the effect of ‘a’ and thus prevents backward tilting of the pelvis.
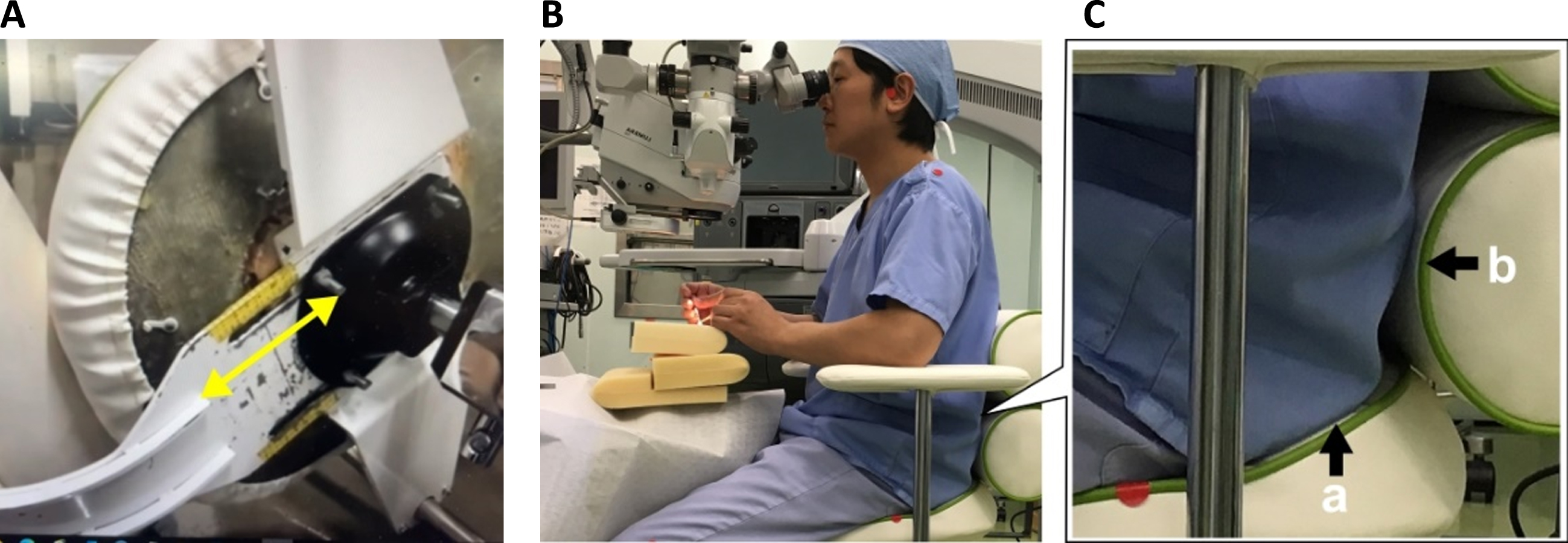
In contrast to office chairs with reclining and complex dynamic mechanisms (Fig. 5A), the prototype chair has a simple structure, adjustment function, and material combination (Fig. 5B). Although the prototype chair has a fixed backrest, the shape and displacement of the cushions allow the surgeon to lean backward or make slight movements. Other requirements for the surgeon’s tasks were also addressed using features such as armrests, foot pedal for the adjustment of seat height, and caster lock functions. The seat fabric was upholstered in wipe-cleanable, antibacterial, and alcohol-resistant vinyl leather. Disposable sterilized covers were attached to the armrests to prevent infection duringsurgery.
Fig. 5
Comparison of an ergonomic office chair and the prototype chair. (A) Dynamic backrest with a reclining mechanism found in ergonomic office chairs. (B) Fixed backrest found in the prototype chair. The adjustment mechanisms (vertical height, vertical inversion, and forward/backward) and two rolls aim to accommodate the requirements of static and dynamic seating.
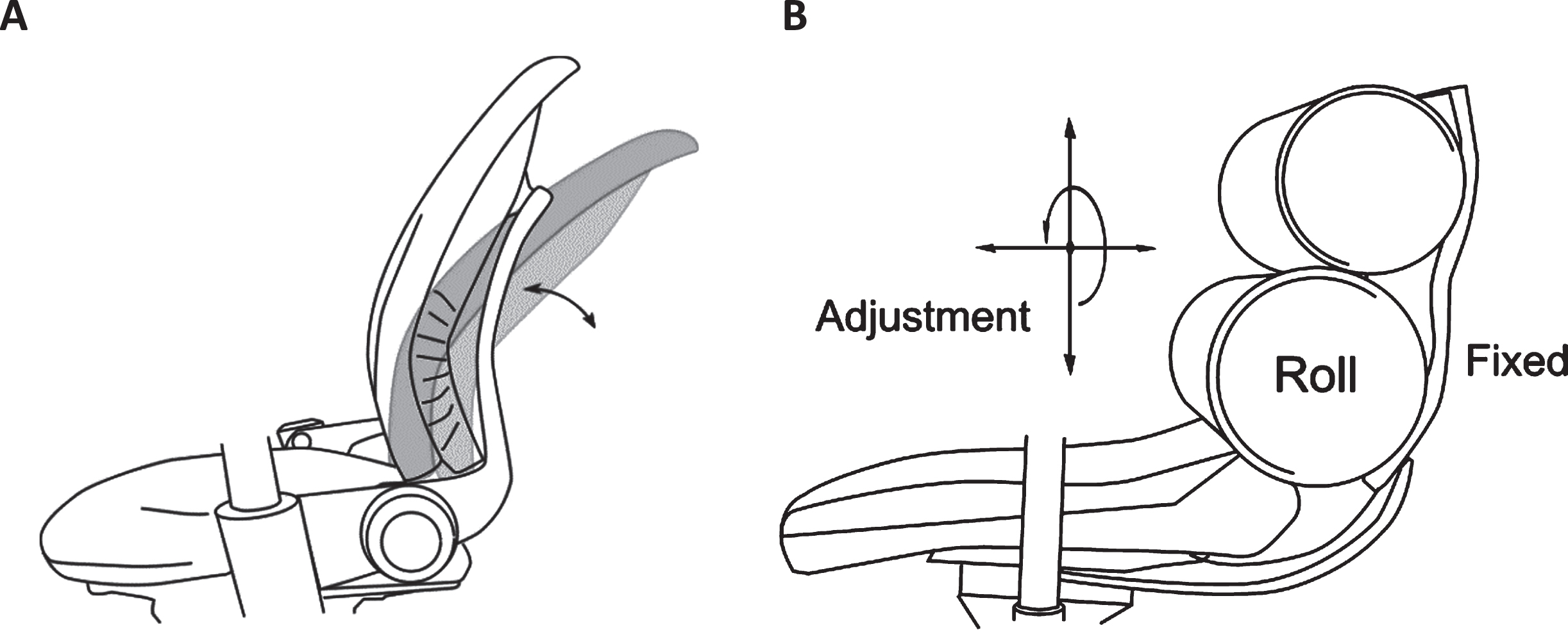
2.2Clinical pilot test
Five male ophthalmic surgeons participated in the study. The mean height and body mass index among the five surgeons were 169±3 cm and 23.1±2.6 kg/m2 (mean±standard deviation), respectively. The procedure involved vitreous surgery using a microscope, which is usually performed in the operating room (operation time: approximately 30–120 min). Two chairs were used during different surgeries: a conventional chair (A) and a prototype chair (B). The surgeons adjusted each part of the chair to fit the body before the operation. Each surgeon performed one surgery with each chair, and the order of exposure to each chair was randomized. Thus, ten observations were obtained.
The pressure distribution on the seat surface and pelvic tilt angle measurements, both of which have been used in previous studies [2, 19], were continuously recorded during surgery. The pressure distribution was measured using a pressure-sensing device (X-sensor X3, model PX100:36.36.02; XSENSOR Co., Calgary, Canada). The sensor sheet had a sensing area of 457×457 mm, a thickness of 0.8 mm, a sensor count of 36×36 (1,296), and a sensor pitch of 12.7 mm. The pressure ranged from 0 to 256 mmHg, and the measurement accuracy was approximately±10%.
Pelvic rotation is associated with lumbar alignment. Forward tilting of the pelvis causes lumbar lordosis, whereas backward tilting causes lumbar kyphosis. The pelvic tilt angle, which was used as an index to estimate lumbar alignment, was measured using a gyroscope sensor (VR-sensor, model GU-3013; Datatec Co., Tokyo, Japan) mounted on the iliac crest of the body surface, in accordance with Wu’s method [37]. The sensor system consisted of a gyro sensor and a control box containing an AD converter and a microcomputer. The sensor size was 35×35×29 mm, the sensor weight was 50 g, the detection angle (pitch angle) was±60°, and the measurement accuracy was±0.5°. The pelvic tilt angle was converted to a relative value based on the pre-measured value in the standing position (0°). Backward pelvic tilt was defined as negative. The sampling frequencies of the pressure and gyroscope sensors were both 1 Hz, and the start times were synchronized. The analyses were performed using the mean values of stable data collected over 30 s, starting from 30 min after the surgery began. This approach accounted for the differences in total operation time among surgeons.
Paired t-tests were used to analyze the following measurement items: average seat pressure, maximum seat pressure, contact area, and relative value of the pelvic tilt angle. Cohen’s d was used as a measure of the effect size. Cohen’s literature [45] describes as guide for use: small effect size is d = 0.2, medium effect size is d = 0.5, and large effect size is d = 0.8. If d is greater than 0.8, statistical power is generally considered sufficient. The correlation between the relative value of the pelvic tilt angle and each variable of the pressure distribution was examined, and Pearson’s correlation coefficient was calculated. The significance level was set at less than 5%. Statistical analyses were performed using IBM SPSS Statistics version 28 (IBM Corp., Armonk, N.Y., USA).
3Results
Figure 6 shows the results for the average seat pressure, maximum seat pressure, and contact area in the seat surface, as well as those for the pelvic tilt angle. The two chair types exhibited significant differences in all measurement items. The average seat pressure was 70.4±12.7 mmHg for A and 40.5±3.8 mmHg for B (p = 0.008, d = 2.159); maximum seat pressure was 242.2±19.7 mmHg for A and 170.5±38.5 mmHg for B (p = 0.024, d = 1.581); the contact area was 906.1±114.5 cm2 for A and 1,255.9±60.1 cm2 for B (p < 0.001, d = –4.272); and the relative values of the pelvic tilt angle were –13.7°±3.7° for A and –7.1°±4.9° for B (p = 0.032, d = –3.144). Furthermore, the relative value of the pelvic tilt angle was significantly negatively correlated with the average seat pressure (r = –0.892, p = 0.007) and maximum seat pressure (r = –0.914, p = 0.004).
Fig. 6
Comparison of (A) average pressure, (B) maximum pressure, (C) contact area in the seat surface, and (D) pelvic tilt angle in the conventional and prototype chairs. **p < 0.01, *p < 0.05.
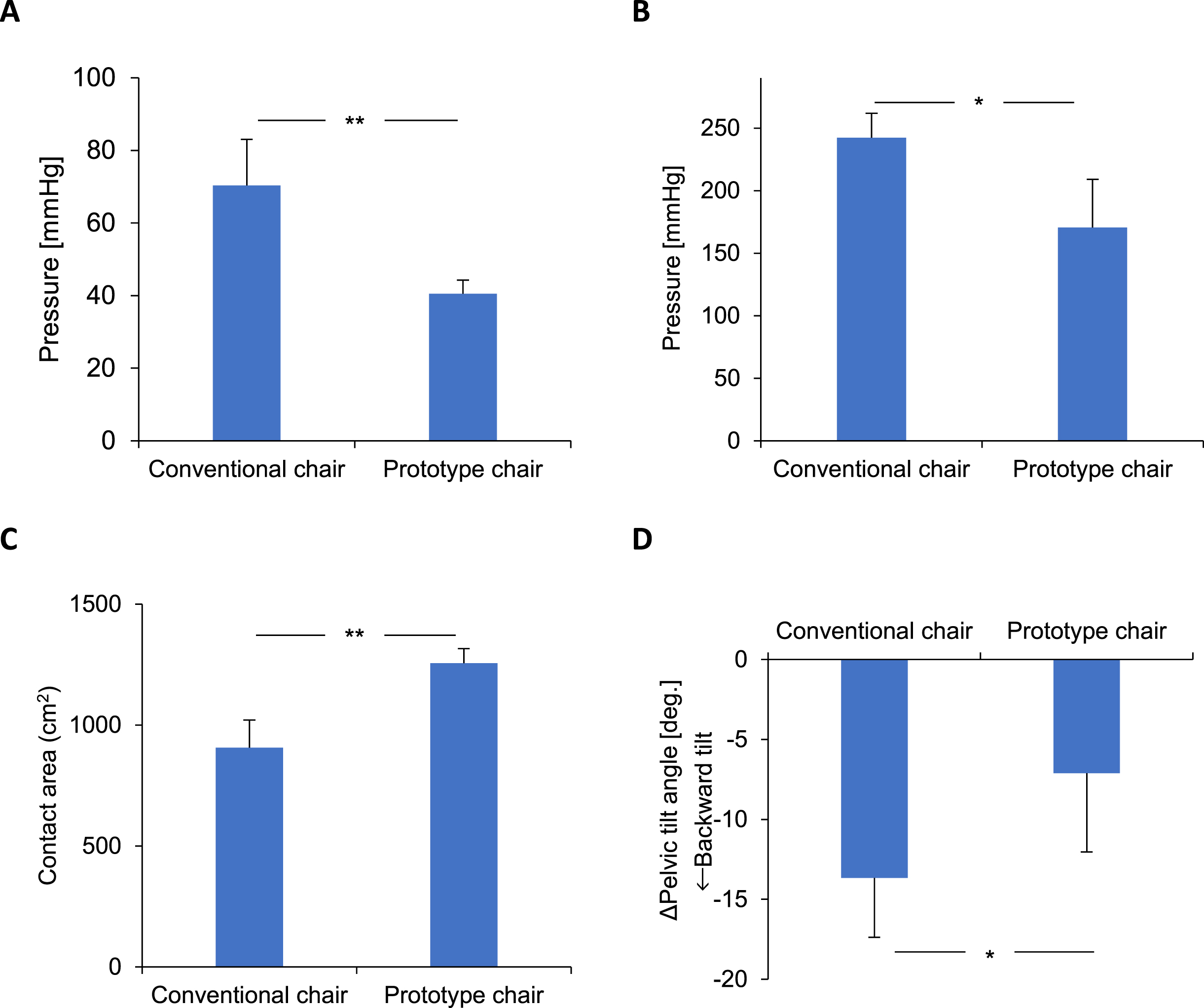
4Discussion
4.1Comparison of chairs
In this study, we compared a prototype chair designed to reduce physical strain with a conventional chair during ophthalmic surgery. Pressure values were lower, and the contact area was larger for the prototype chair than for the conventional chair, indicating that the prototype succeeded in reducing the pressure on the thighs/buttocks. The average seat pressure level with prototype chair in all participants was less than 50 mmHg, which is referred to as the “sitting comfort zone” [28]. The side supports of the seat surface may contribute to stabilizing the surgeon’s body and distributing the pressure, while the downward-tilted front edge of the seat surface may aid in reducing pressure behind the thighs/knees, which was notable in surgeon 5. Similarly, the cushioning material may contribute to reduction and dispersion of pressure, which was notable in surgeons 4 and 5. Images of each seat pressure map can be found in the literature [43].
When compared with the conventional chair, the prototype chair also prevented backward tilting of the pelvis, suggesting that the surgeon could maintain a more neutral seated posture that achieves pelvic and spinal alignment similar to that observed in the standing position. This positive effect may be due to the pelvic support on the seat surface and the function of the backrest. According to previous studies [20, 21], the backrest of the same prototype chair is in contact with the surgeon’s lower back at a low pressure (<20 mmHg, contact ratio of >80%). The synergistic effect of the seat surface and backrest allows for a stable work posture while minimizing the reaction force on the backrest and the forward displacement on the seat, even when the surgeon’s back touches the backrest.
The correlation analysis also indicated that the seat pressure increased with backward tilting of the pelvis. This is presumed to have occurred due to the change in the position of the ischial tuberosities caused by the rotation of the pelvis. Chair design approaches targeting the pelvic region may promote more neutral postures and reduce seat pressure.
4.2Study strength and limitations
The major strength of the present study was the use of a paired design to evaluate the effectiveness of a novel prototype chair for ophthalmic surgeons during actual microsurgery in the operating room. Although the chair was designed for surgical purposes, the findings may also be applicable to the development of general task chairs, such as office chairs. Based on these findings, we are also considering the development of a chair for daily use in an outpatient examination room.
A major limitation of this study was the small number of participating surgeons. A larger number of participants would have increased the validity of the findings; however, we were limited by the number of qualified microsurgeons who could participate at our institution. Another limitation was the relatively short duration of data collection/analysis to allow for detailed comparisons (30 s period after 30 min of surgery). Overall, the surgical procedure was performed with restrained body movements, thus even such a short duration analysis would be a sufficient substitute. However, a more detailed and longer experiment, including analysis, is required. Further studies are also required to elucidate the effects of long-term chair use on fatigue and performance in surgeons. Additionally, the measurement frequencies of both the pressure and gyroscope sensors were limited to 1 Hz because the microsurgery with the microscope was assumed to force the surgeon to a fixed posture. A higher sampling frequency would have been required for more detailed observation and analysis, but sitting is fairly static and therefore even at such a low sample rate there can still be confidence in the average angles measured (this would be similar to using radiographs to look at posture, i.e. just a single point in time measure, which still, however, gives an idea of the general posture). Lastly, our study may have been affected by intervention bias. The research group is the same group that designed the prototype chair. Although the multidisciplinary collaborative approach was useful for the development of the prototype chair, the potential for participant bias during evaluation cannot be excluded, even with objective measurements. Hence, blinded testing with more participants is therefore required.
Future work should include: a larger study with more participants, potentially a cluster randomized controlled trial with multiple sites if there are low numbers of surgeons at a single institution; longer data collection/analysis with higher sample rates; and potentially additional measures such as a low back angle (only one additional sensor at L1 that does not affect the surgery while minimizing interference with the chair backrest would be needed for this, e.g. a bodysuit type sensor).
5Conclusion
The results of this small study suggest that the prototype chair reduces seat pressure during surgery compared with the conventional chair while also maintaining a more neutral posture. These effects may translate to reduced physical strain in surgeons. Future studies should investigate this in greater detail using larger samples of surgeons and longer durations of exposure, ideally by adopting a randomized controlled design.
Disclaimer
This paper is complemented by the contents of the proceedings chapter, “Oyama et al. Fitting a chair to a surgeon’s body: Mechanism of a chair for ophthalmologic surgeon in an operating room” presented at the technical session “Healthcare Symposia - Ergonomics in Surgery” of the IEA Congress in 2021 (Vancouver, Canada).
Ethical approval
This study was approved by the Ethics Committee of the University of Occupational and Environmental Health, Japan (reference number: H28-219).
Informed consent
The participants provided written informed consent to participate in this study.
Conflict of interest
The authors have no potential conflicts of interest to report.
Acknowledgments
The authors would like to thank Prof. Dr. Hiroyuki Kondo at the University of Occupational and Environmental Health, Japan and all surgeons who participated in this study. They also would like to thank Handaya Co., Ltd. for their contribution to the manufacturing of the prototype chair.
Funding
This study was supported by the Dai-ichi Life Foundation.
References
[1] | Kondo H , Fujiki N , Mitsuya R , Oyama H , Togami H , Hachiya Y , et al. A study on working posture of microsurgeons. Research report. Tokyo: The Dai-ichi Life Foundation; 2016. (in Japanese). |
[2] | Noro K , Naruse T , Lueder R , Nao-I N , Kozawa M . Application of Zen sitting principles to microscopic surgery seating. Appl Ergon. (2012) ;43: (2):308–419. |
[3] | Dhimitri KC , McGwin GJr , McNeal SF , Lee P , Morse PA , Patterson M , et al. Symptoms of musculoskeletal disorders in ophthalmologists. Am J Ophthalmol. (2005) ;139: :179–81. |
[4] | Hyer JN , Lee RM , Chowdhury HR , Smith HB , Dhital A , Khandwala M . National survey of back & neck pain amongst consultant ophthalmologists in the United Kingdom. Int Ophthalmol. (2015) ;35: (6):769–75. |
[5] | Schechet SA , DeVience E , DeVience S , Shukla S , Kaleem M . Survey of musculoskeletal disorders among US ophthalmologists. Digit J Ophthalmol. (2020) ;26: (4):36–45. |
[6] | Marx JL . Ergonomics: Back to the future. Ophthalmology. (2012) ;119: :211–2. |
[7] | American Academy of Ophthalmology, Ergonomics Task Force. Ergonomics Best Practices [Internet]. San Francisco: American Academy of Ophthalmology; 2016 [cited 2021 Jul 30]. Available from: https://www.aao.org/course/ergonomics-best-practices-course |
[8] | Hallbeck MS , Lowndes BR , Bingener J , Abdelrahman AM , Yu D , Bartley A , et al. The impact of intraoperative microbreaks with exercises on surgeons: A multi-center cohort study. Appl Ergon. (2017) ;60: :334–41. |
[9] | Coleman Wood KA , Lowndes BR , Buus RJ , Hallbeck MS . Evidence-based intraoperative microbreak activities for reducing musculoskeletal injuries in the operating room. Work. (2018) ;60: (4):649–59. |
[10] | van Det MJ , Meijerink WJ , Hoff C , van Veelen MA , Pierie JP . Ergonomic assessment of neck posture in the minimally invasive surgery suite during laparoscopic cholecystectomy. Surg Endosc. (2008) ;22: (11):2421–7. |
[11] | Dalagera T , Jensen PT , Winthere TS , Savarimuthue TR , Markauskasc A , Mogensend O , et al. Surgeons’ muscle load during robotic-assisted laparoscopy performed with a regular office chair and the preferred of two ergonomic chairs: A pilot study. Appl Ergon. (2019) ;78: :286–92. |
[12] | Rhén IM , Fan X , Kjellman M , Forsman M . A possible revival of population-representing digital human manikins in static work situations - exemplified through an evaluation of a prototype console for robotic surgery. Work. (2021) ;70: (3):833–51. |
[13] | Zhang Z , Wang L , Wei Y , Fang D , Fan S , Zhang S . The preliminary experiences with three-dimensional heads-up display viewing system for vitreoretinal surgery under various status. Curr Eye Res. (2019) ;44: (1):102–9. |
[14] | Eckardt C , Paulo EB . Heads-up surgery for vitreoretinal procedures: An experimental and clinical study. Retina. (2016) ;36: (1):137–47. |
[15] | Mendez BM , Chiodo MV , Vandevender D , Patel PA . Heads-up 3D microscopy: An ergonomic and educational approach to microsurgery. Plast Reconstr Surg Glob Open. (2016) ;4: (5):1–4. |
[16] | Shibata T , Shiraki Y , Kamei M . Clinical utility of stereoscopic 3D displays in heads-up surgery. SID Symposium Digest of Technical Papers. (2018) ;49: (1):1327–30. |
[17] | Weinstock RJ , Ainslie-Garcia MH , Ferko NC , Qadeer RA , Morris LP , Cheng H , et al. Comparative assessment of ergonomic experience with heads-up display and conventional surgical microscope in the operating room. Clin Ophthalmol. (2021) ;15: :347–56. |
[18] | Bin Helayel H , Al-Mazidi S , AlAkeely A . Can the three-dimensional heads-up display improve ergonomics, surgical performance, and ophthalmology training compared to conventional microscopy? Clin Ophthalmol. (2021) ;15: :679–86. |
[19] | Watanabe A , Oyama H , Noro K , Togami H , Kondo H . Analysis and improvement of surgeons’ posture during ophthalmic microsurgery (part 1): Heads-up surgery with a 3D monitor. In: JägerM, editor. Proceedings of 64. Kongress der Gesellschaft für Arbeitswissenschaft (GfA). Dortmund: GfA Press; (2018) , pp. 1–6. |
[20] | Oyama H , Watanabe A , Togami H , Kondo H , Noro K . Analysis and improvement of surgeons’ posture during ophthalmic microsurgery (part 2): Development of a chair with backrest for forward-inclined posture. In: JägerM, editor. Proceedings of 64. Kongress der Gesellschaft für Arbeitswissenschaft (GfA). Dortmund: GfA Press; (2018) , pp. 1–6. |
[21] | Menozzi M , Neumayer N , Huang Y , Watanabe A , Oyama H , Fujimaki G , et al. Ergonomic aspects in the design of instrumentation for ophthalmic microsurgery. Z Arb Wiss. (2019) ;73: (1):23–34. |
[22] | Zhang L , Helander MG , Drury CG . Identifying factors of comfort and discomfort in sitting. Hum Factors. (1996) ;38: (3):377–89. |
[23] | Helander MG , Zhang L . Field studies of comfort and discomfort in sitting. Ergonomics. (1997) ;40: (9):895–915. |
[24] | Lueder RK . Seat comfort: A review of the construct in the office environment. Hum Factors. (1983) ;25: (6):701–11. |
[25] | de Looze MP , Kuijt-Evers LF , van Dieën J . Sitting comfort and discomfort and the relationships with objective measures. Ergonomics. (2003) ;46: (10):985–97. |
[26] | Zemp R , Taylor WR , Lorenzetti S . Are pressure measurements effective in the assessment of office chair comfort/discomfort? A review. Appl Ergon. (2015) ;48: :273–82. |
[27] | Congleton JJ , Ayoub MM , Smith JL . The design and evaluation of the neutral posture chair for surgeons. Hum Factors. (1985) ;27: (5):589–600. |
[28] | Noro K , Fujimaki G , Kishi S . A theory on pressure distribution and seat discomfort. In: VinkP, editor. Comfort and Design. Florida: CRC Press; (2005) , pp. 33–9. |
[29] | Noro K , Lueder R , Yamada S , Fujimaki G , Oyama H , Hashidate Y . Revisiting sitting cross-cultural aspects of seating. Proceedings of the Human Factors and Ergonomics Society 50th Annual Meeting. (2006) ;50: (7):814–9. |
[30] | Zenk R , Franz M , Bubb H , Vink P . Technical note: Spine loading in automotive seating. Appl Ergon. (2012) ;43: (2):290–5. |
[31] | Vink P , Franz M , Kamp I , Zenk R . Three experiments to support the design of lightweight comfortable vehicle seats. Work. (2012) ;41: :1466–70. |
[32] | Burandt U . Radiography of the position of the pelvis and vertebral column while seated on a forward tilted surface. Ergonomics. (1969) ;12: (2):356–64. |
[33] | Mandal AC . The seated man (Homo Sedens) the seated work position. Theory and practice. Appl Ergon. (1981) ;12: (1):19–26. |
[34] | Lueder R . Ergonomics review: Balans seating for VARIÉRUSA [Internet]. Texas: Humanics Ergonomics; 2010 [cited 2021 Aug 20]. Available from: https://www.humanics-es.com/BalansErgonomicsReview.pdf |
[35] | Vaucher M , Isner-Horobeti ME , Demattei C , Alonso S , Hérisson C , Kouyoumdjian P , et al. Effect of a kneeling chair on lumbar curvature in patients with low back pain and healthy controls: A pilot study. Ann Phys Rehabil Med. (2015) ;58: (3):151–6. |
[36] | O’Sullivan K , McCarthy R , White A , O’Sullivan L , Dankaerts W . Lumbar posture and trunk muscle activation during a typing task when sitting on a novel dynamic ergonomic chair. Ergonomics. (2012) ;55: (12):1586–95. |
[37] | Wu CS , Miyamoto H , Noro K . Research on pelvic angle variation when using a pelvic support. Ergonomics. (1998) ;41: (3):317–27. |
[38] | Williams MM , Hawley JA , McKenzie RA , van Wijmen PM . A comparison of the effect of two sitting posture on back and referred pain. Spine. (1991) ;16: (10):1185–91. |
[39] | Keegan JJ . The medical problem of lumbar spine flatting in automobile seats. SAE Technical Papers. (1964) ;838A 1–12. |
[40] | Andersson G , Ortengren R , Nachemson A , Elfström G . Lumbar disc pressure and myoelectric back muscle activity during sitting. IV. Studies on a car driver’s seat. Scand J Rehabil Med. (1974) ;6: (3):128–33. |
[41] | Grandjean E . Fitting the task to the man: A textbook of occupational ergonomics. 4th ed. Oxford: Taylor & Francis; (1988) , pp. 52–65. |
[42] | Allie P , Feiks F , Reinecke S . Supporting natural human motion while seated [Internet]. Michigan: Steelcase; 2002 [cited 2002 Apr 17]. At: https://www.steelcase.com/asia-ja/ (in Japanese translation). |
[43] | Oyama H , Watanabe A , Togami H , Kondo H , Noro K . Fitting a chair to a surgeon’s body: Mechanism of a chair for ophthalmologic surgeon in an operating room. In: BlackNL, NeumannWP, NoyI, editors. Proceedings of the 21st Congress of the International Ergonomics Association (IEA 2021). Cham: Springer; (2021) , pp. 410–6. |
[44] | Research Institute of Human Engineering for Quality Life. Japanese body size data book 2004–2006. Osaka: Research Institute of Human Engineering for Quality Life; 2011. |
[45] | Cohen J . Statistical power analysis for the behavioral sciences. 2nd ed. Hillsdale, NJ: Lawrence Earlbaum Associates, Publishers; (1988) . |
Notes
1 The term “heads-up surgery” is derived from the so-called “head-up display” that was first used in aircraft cockpits to allow pilots to raise their heads to view information without looking down at the instrument panel.