Serum tumor markers for response prediction and monitoring of advanced lung cancer: A review focusing on immunotherapy and targeted therapies
Abstract
BACKGROUND:
The value of serum tumor markers (STMs) in the current therapeutic landscape of lung cancer is unclear.
OBJECTIVE:
This scoping review gathered evidence of the predictive, prognostic, and monitoring value of STMs for patients with advanced lung cancer receiving immunotherapy (IT) or targeted therapy (TT).
METHODS:
Literature searches were conducted (cut-off: May 2022) using PubMed and Cochrane CENTRAL databases. Medical professionals advised on the search strategies.
RESULTS:
Study heterogeneity limited the evidence and inferences from the 36 publications reviewed. While increased baseline levels of serum cytokeratin 19 fragment antigen (CYFRA21-1) and carcinoembryonic antigen (CEA) may predict IT response, results for TT were less clear. For monitoring IT-treated patients, STM panels (including CYFRA21-1, CEA, and neuron-specific enolase) may surpass the power of single analyses to predict non-response. CYFRA21-1 measurement could aid in monitoring TT-treated patients, but the value of CEA in this context requires further investigation. Overall, baseline and dynamic changes in individual or combined STM levels have potential utility to predict treatment outcome and for monitoring of patients with advanced lung cancer.
CONCLUSIONS:
In advanced lung cancer, STMs provide additional relevant clinical information by predicting treatment outcome, but further standardization and validation is warranted.
1Introduction
The past two decades have witnessed outstanding advances in thoracic oncology. The nature of these advances is three-fold: first, a deeper understanding of the molecular determinants of cancer initiation and progression; second, advances in the development and clinical use of novel anticancer therapies, such as targeted treatments and immunotherapies; and third, the application of increasingly sophisticated technologies and diagnostic tools, such as next-generation sequencing, tissue-based scores, artificial intelligence, and radiomics in lung cancer medicine [1–5].
Immunotherapy and targeted therapy are treatment options for patients with lung cancer, alongside surgery, radiotherapy, and chemotherapy. Immunotherapy uses monoclonal antibodies to interact with cytotoxic T cells or ligands on tumor cells to induce tumor cell apoptosis [3]. Immune checkpoint inhibitors (ICIs) with mechanisms of action against programed death receptor-1 (PD-1; e.g., nivolumab, pembrolizumab), programed death-ligand 1 (PD-L1; e.g., atezolizumab), and cytotoxic T-lymphocyte-associated protein 4 (CTLA-4; e.g., ipilimumab) are available options [3, 4]. Treatment with ICIs alone, or in combination with chemotherapy, is recommended in the first-line setting for patients with advanced non-small cell lung cancer (NSCLC) whose tumors do not have actionable driver mutations, such as alterations in the epidermal growth factor receptor (EGFR) or anaplastic lymphoma kinase (ALK) genes [4]. Patients whose tumors do harbor actionable mutations may benefit from targeted therapy with tyrosine kinase inhibitors (TKIs), multi-kinase inhibitors, serine/threonine kinase inhibitors, or other small molecule inhibitors; many such agents are approved for the treatment of NSCLC [6].
Precision medicine and personalized medicine are rightly advocated as the most promising path forward in oncology, although their clinical application remains challenging due to a lack of infrastructure, in-depth fundamental knowledge, and validated data from clinical trials. There has been a focus on molecular markers to stratify patients for targeted or immune treatments and international guidelines recommend that all patients with advanced NSCLC undergo testing for mutations in EGFR, BRAF, Kirsten rat sarcoma virus (KRAS), ALK rearrangements, and PD-L1 expression [7]. However, in this quest for precision and personalization, more dated predictive circulating protein biomarkers have been somewhat neglected, as they have been shown to provide inadequate or insufficient information. Several issues are apparent in the existing literature: small, heterogeneous patient populations that may have concomitant diseases capable of influencing marker levels; use of different methods to assess serum tumor marker (STM) values; and lack of comparison with other existing predictive markers [8, 9].
The rapid evolution of therapeutic options brings the need to revisit and critically assess all tools at our disposal to support and guide clinical decision making. Recent studies suggest that STMs hold therapeutic promise, though published literature around this topic is scarce [10–13]. The European Society for Medical Oncology (ESMO) 2017 and 2019 Clinical Practice Guidelines (European and Pan-Asian adapted) do not recommend routine measurement of STMs for staging and risk assessment in patients with early or advanced NSCLC, and the ESMO 2021 guidelines state that there are currently no validated STMs with predictive value for small cell lung cancer (SCLC) [14–16]. These recommendations, however, are based on historical data, including evidence from a decade old review focusing on measurement of carcinoembryonic antigen (CEA) in patients following surgery, chemotherapy, or radiotherapy only [17]. The 2022 National Comprehensive Cancer Network and American Society of Clinical Oncology guidelines contain no information about STMs [7, 18–20]. Nonetheless, STMs have all the attributes to be considered “companion diagnostics”: STMs reflect tumor size or biochemical activity [21]; they can be assessed quantitatively, quickly, robustly, and with high levels of quality control on automated instruments; assessments are cost-efficient; and testing can be performed in a serial manner before, during, and after therapy to monitor local and systemic tumor control. However, to aid clinical decision making and to interpret individual STM levels and kinetics, several values and measures need to be developed, including defined criteria for appropriate time points, and relevant thresholds or individual value changes over time.
Predictive markers are defined as those that are measured at baseline and are indicative of therapeutic efficacy; they can discriminate between patients who will or will not respond to a specific therapy [22, 23]. Prognostic markers indicate the likelihood of disease recurrence, progression-free survival (PFS), or overall survival (OS), and are independent of therapy received as they reflect innate tumor behavior [23]. In this review, “prognosis” is considered after the initiation of immunotherapy or targeted treatment. In addition, some biomarkers have monitoring value, in that they can be measured serially to evaluate disease status or inform on the effect of a medical or biologic agent over time [23]. Importantly, some biomarkers are both predictive and prognostic, whilst also providing monitoring value (Fig. 1) [22, 23].
Fig. 1
Summary of predictive, prognostic, and monitoring STMs for lung cancer. Biomarkers may have multiple clinical applications during the course of the disease, depending on the timing of the test and the exact measurements and comparisons that are to be made. The arrow represents the time from initial histologic diagnosis and staging in arbitrary units. CT: computed tomography, NSCLC: non-small cell lung cancer, SCLC: small cell lung cancer, STMs: serum tumor markers.
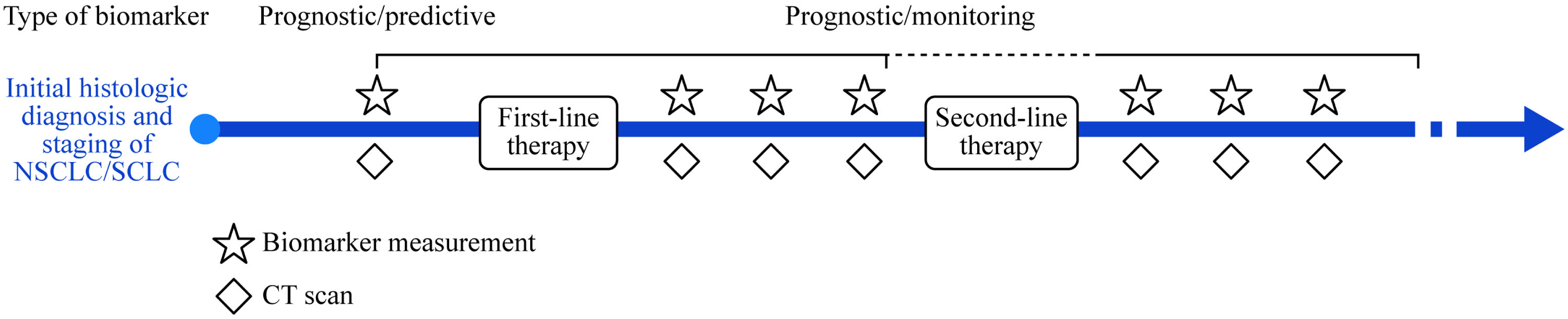
We conducted a literature search to gather current evidence around the predictive, prognostic, and monitoring value of circulating STMs, including: CEA, cytokeratin 19 fragment antigen (CYFRA21-1), carbohydrate antigen 19-9 (CA19-9), cancer antigen 125 (CA125), cancer antigen 15-3 (CA15-3), squamous cell carcinoma antigen (SCCA), neuron-specific enolase (NSE), progastrin-releasing peptide (ProGRP), human epididymis protein 4 (HE4), and antibodies against tumor protein 53 (as described in Table 1), for patients with advanced stage lung cancer, including NSCLC and SCLC, receiving immunotherapy or targeted therapy.
Table 1
STMs included in the search strategy
STM | Biologic function | Application in diagnostics |
CEA | CEA is a glycoprotein produced during embryonic development that is reported to be involved in cell adhesion, immunity, and apoptosis [24, 25] | CEA is overexpressed in many malignant tumors, including NSCLC, and is readily detectable in serum [26] |
CYFRA21-1 | CYFRA21-1 is a fragment of cytokeratin 19, a structural protein in the cytoskeleton of epithelial cells, that is soluble in serum [27, 28] | Degradation of cytokeratin 19 occurs at an accelerated rate in epithelial neoplasms, and elevated circulating CYFRA21-1 occurs in NSCLC, particularly squamous cell carcinoma [28] |
CA19-9 | CA19-9 is a tumor-associated antigen that binds to the Lewis (a) antigen on a mucin [29, 30] | Elevated levels of CA19-9 have been observed in cases of gastric, lung, colon, and pancreatic cancer [31] |
CA125 | CA125 is a glycoprotein encoded by MUC16 that is expressed on the surface of epithelial cells [32] | CA125 is involved in cancer cell proliferation and expressed in various gynecologic and non-gynecologic cancers, including lung cancer [32, 33] |
CA15-3 | CA15-3 is a mucin encoded by MUC1; under normal conditions, mucins play a protective role on the surface of epithelial cells [34] | Elevated levels of circulating CA15-3 have been identified in colorectal, lung, ovarian, breast, and pancreatic cancers, using the monoclonal antibodies DF3 and 115D8 [34] |
SCCA | SCCA is a subfraction of the glycoprotein TA-4 that acts as an enzyme inhibitor [35, 36] | Alterations in the expression of SCCA have been identified in squamous cell carcinomas, including NSCLC [37] |
NSE | NSE is a cell-specific isoenzyme encoded by ENO2; under normal pathophysiologic conditions, NSE is found in the cytoplasm of neurons and neuroendocrine cells, and is involved in glycolytic energy metabolism [38] | Elevated levels of circulating NSE have been reported during malignant proliferation, and NSE has been used as a marker for SCLC and NSCLC [38, 39] |
ProGRP | ProGRP is a precursor for GRP, a regulatory neuropeptide implicated in an array of physiologic processes [40] | ProGRP may function as an autocrine growth factor in various types of cancer, including SCLC [40, 41] |
HE4 | HE4 is a protein encoded by WFDC2 that inhibits trypsin degradation and belongs to the WFDC protein family [42, 43] | HE4 has been suggested to be a biomarker for various cancers including ovarian, endometrial, and lung [42–44] |
Anti-p53 | p53 is a tumor suppressor involved in the regulation of cell growth, DNA repair, and apoptosis [45] | Mutations in TP53 can result in overexpression of p53 and thereby induce circulating p53 antibodies (anti-p53) in various types of cancer, including NSCLC [45, 46] |
Anti-p53: antibodies against tumor protein p53, CA125: cancer antigen 125, CA15-3: cancer antigen 15-3, CA19-9: carbohydrate antigen 19-9, CEA: carcinoembryonic antigen, CYFRA21-1: cytokeratin 19 fragment antigen, DNA: deoxyribonucleic acid, ENO2: enolase 2, GRP: gastrin-releasing peptide, HE4: human epididymal protein 4, MUC1: mucin 1, MUC16: mucin 16, NSCLC: non-small cell lung cancer, NSE: neuron-specific enolase, ProGRP: progastrin-releasing peptide, SCCA: squamous cell carcinoma antigen, SCLC: small cell lung cancer, STMs: serum tumor markers, TP53: tumor protein p53, WFDC: whey acidic four-disulfide core, WFDC2: whey acidic four-disulfide core domain 2.
2Literature search methodology
Using PubMed and Cochrane CENTRAL databases, we conducted literature searches with a cut-off date of May 9, 2022. A team of medical professionals advised on the design of the search strategies, and these were adapted for each database. The Boolean operators “AND", “OR", and “NOT” were used to combine keywords related to the STMs of interest (Table 1), NSCLC, or SCLC, and a set of “Core terms” that defined the outcomes of interest and treatment, including immunotherapy and targeted therapy specific for NSCLC and SCLC. Searches were restricted to records of papers published after 2011 and non-human studies were excluded. Full search strategies for both PubMed and Cochrane CENTRAL are provided in Appendices 1–4.
Search results were imported into a shared spreadsheet and duplicate records removed. Two team members screened each abstract for eligibility. For records marked for potential inclusion, full texts were evaluated by the wider review team. Of 362 publications identified, 229 titles and abstracts were screened and 72 full texts were of potential interest and assessed for eligibility. In total, 36 publications were included in the review, of which only one concerned SCLC. Overall, 326 publications were excluded due to being duplicates (n = 132) or not fulfilling the inclusion criteria as follows: irrelevant study design (n = 134), case reports (n = 36), wrong patient population/setting (n = 15), not English language (n = 7), irrelevant outcome(s) (n = 2) (Fig. 2).
Fig. 2
Flow diagram of search results. NSCLC: non-small cell lung cancer, SCLC: small cell lung cancer, STMs: serum tumor markers.
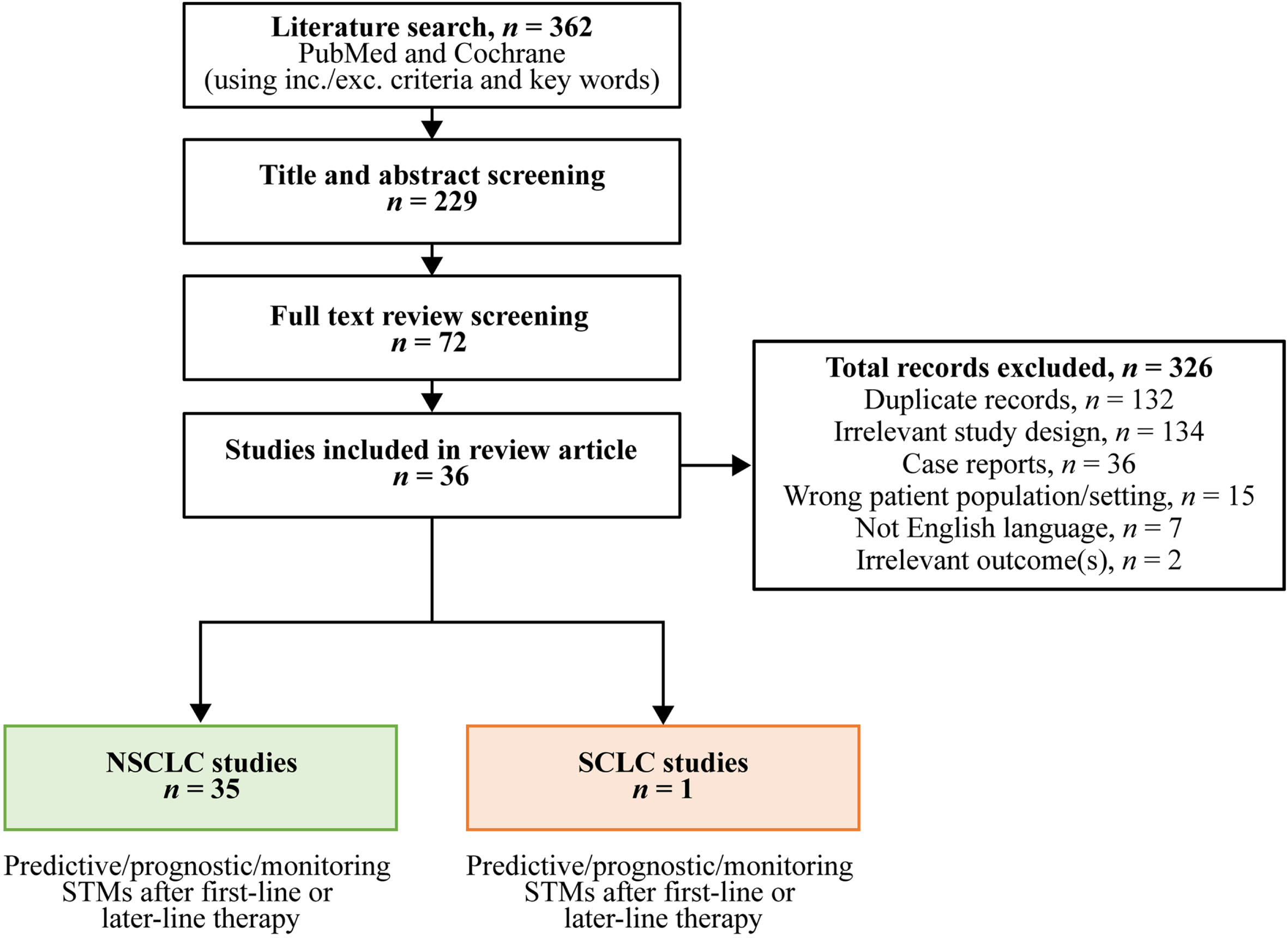
3Predictive STMs for treatment response (measured at baseline)
A key objective of identifying predictive STMs in patients with lung cancer is to enable appropriate use of immunotherapy or targeted therapies and to personalize treatment by predicting response prior to treatment initiation, using baseline samples. Several STMs have demonstrated predictive value for treatment response in patients with advanced NSCLC receiving immunotherapy or targeted therapy (Table 2 and Fig. 3A and B).
Table 2
Summary of outcomes for predictive STMs in
Reference | Study | Disease | STM | Assay | PFS | OS | RR | Other | |||
(year) | type | (stage), histology, n | (cut off) | method (kit/ instrument) | Median PFS (95% CI), P-value | HR (95% CI), P-value | Median OS (95% CI), P-value | HR (95% CI), P-value | % Responders, P-value | HR (95% CI), P-value | parameters |
Predictive STMs in patients treated with immunotherapy | |||||||||||
Shirasu (2018) | Retrospective | Advanced NSCLC (stage IV), adenocarcinoma, n = 50 | CEA (<5.0 vs. ≥ 5.0 ng/mL) | CLIA (Architect kit; Abbott) | – | Univariate analysis: 1.05 (0.54–2.03), 0.888 | – | – | – | – | – |
CYFRA21-1 (≥2.2 vs. <2.2 ng/mL) | CLEIA (Lumipulse Presto kit; Fujirebio) | 155 (65–275) vs. 51.5 (36–70) days – | Multivariate analysis: 0.44 (0.23–0.85), 0.015 | – | – | – | – | – | |||
Dall’Olio (2020) | Retrospective | Advanced NSCLC (stage IIIB/IV), squamous, non-squamous, test set, n = 133; validation set, n = 74; chemotherapy control set, n = 89 | CEA (>8.0 ng/mL) | CLIA (Access CEA kit/DXI instrument; Beckman Coulter) | – | – | – | Pooled multivariate analysis: 1.58 (1.06–2.33), 0.024 | – | – | – |
CYFRA21-1 (>8.0 vs. ≤8.0 ng/mL) | TRACE (Kryptor compact plus; Thermo Fisher Scientific) | – | – | Pooled analysis: 3.0 (1.9–4.1) vs. 17.7 (11.4–24.0) months, – | Pooled multivariate analysis: 1.90 (1.24–2.93), 0.003 | – | – | – | |||
Kataoka (2018) | Retrospective | Advanced NSCLC (–), squamous, non-squamous, n = 189 | CEA (≥13.8 vs. <13.8 ng/mL) | CLEIA (ND) | – | Adjusted HR: 1.72 (1.17–2.53), 0.005 | – | – | – | – | – |
CYFRA21-1 (≥5.05 vs. <5.05 ng/mL) | CLEIA (ND) | – | Adjusted HR: 1.19 (0.82–1.73), 0.36 | – | – | – | – | – | |||
Huang (2020) | Retrospective | Advanced NSCLC (stage IIIB/IV), squamous cell carcinoma, adenocarcinoma, n = 61 | CEA (≤5.0 vs. >5.0 ng/mL) | ND | – | 0.437 (0.225–0.846), 0.014 | – | 0.513 (0.240–1.099), 0.086 | – | – | – |
Lang (2019) | Retrospective | Advanced NSCLC (stage III/IV/CM), adenocarcinoma, squamous cell carcinoma, n = 84 | CYFRA21-1 (baseline levels) | ECLIA (CYFRA21-1 kit/cobas® e 801 instrument; Roche Diagnostics) | – | – | – | – | – | – | AUC (progression/death): 71.8% AUC (death): 68.9% |
CA19-9 (baseline levels) | ECLIA (CA19-9 kit/cobas e 801 instrument; Roche Diagnostics) | – | – | – | – | – | – | AUC (progression/death): 67.6% AUC (death): 64.7% | |||
CEA (baseline levels) | ECLIA (CEA kit/cobas e 801 instrument; Roche Diagnostics) | – | – | – | – | – | – | AUC (progression/death): 51.1% AUC (death): 54.6% | |||
NSE (baseline levels) | ECLIA (NSE kit/cobas e 801 instrument; Roche Diagnostics) | – | – | – | – | – | – | AUC (progression/death): 44.1% AUC (death): 53.2% | |||
Chai (2020) | Retrospective | Recurrent/advanced NSCLC (stage IIIB/IV), adenocarcinoma, squamous cell carcinoma, large cell carcinoma, mixed adenosquamous, n = 110 | CEA | ND | – | – | – | Univariate analysis: 1.00 (1.00–1.00), 0.005 | – | – | – |
CYFRA21-1 | IRMA (ND) | – | – | – | Univariate analysis: 1.01 (1.00–1.02), 0.021 Multivariate analysis: 1.04 (1.01–1.06), 0.002 | – | – | – | |||
SCLC | |||||||||||
Li (2021) | Retrospective | Advanced SCLC (stage IIIB/IV), –, n = 102 | NSE (≥24.0 vs. <24.0 ng/mL at baseline) | ECLIA (Access NSE kit/cobas e 601 instrument; Roche Diagnostics) | 4.7 (–) vs. 8.7 (–) months, 0.006 | Multivariate analysis: 1.93 (1.18–3.17), 0.009 | 15.2 (–) vs. 23.8 (–) months, 0.014 | Multivariate analysis: 2.41 (1.14–5.10), 0.021 | – | – | – |
NSE (≥24.0 vs. <24.0 ng/mL at 3 weeks) | ECLIA (Access NSE kit/cobas e 601 instrument; Roche Diagnostics) | 4.5 (–) vs. 8.4 (–) months, 0.0002 | – | 7.4 (–) vs. 23.3 (–) months, <0.0001 | – | – | – | – | |||
Predictive STMs in patients treated with targeted therapy | |||||||||||
Tanaka (2013) | Retrospective | Advanced NSCLC (stage IIIB/IV, post-surgical relapse), adenocarcinoma, squamous, large cell, with EGFR mutation, n = 160 | CEA (>5.0 vs. ≤5.0 ng/mL) | ECLIA (Architect i2000SR system; Abbott) | 8.6 (7.6–11.9) vs. 11.2 (7.1–16.6) months, 0.242 | 0.99 (0.76–1.17), 0.918 | 26.0 (20.3–36.5) vs. 39.0 (26.5–61.3) months, 0.163 | 1.13 (0.88–1.46), 0.352 | – | – | – |
CYFRA21-1 (>2.0 vs. ≤2.0 ng/mL) | ECLIA (Elecsys® 2010 system; Roche Diagnostics) | 7.5 (6.2–9.1) vs. 13.3 (10.6–18.2) months, <0.001 | 1.27 (1.11–1.40), 0.002 | 24.8 (20.3–36.5) vs. 37.8 (26.4–52.7) months, 0.104 | 1.10 (0.85–1.41), 0.484 | 48.1 vs. 42.2, 0.818 | – | – | |||
Fiala (2014a) | Retrospective | Advanced NSCLC (stage IIIB/IV), adenocarcinoma, squamous cell carcinoma, other, n = 144 | CEA (≥3.0 vs. <3.0μg/L) | CLIA (DXI 800i instrument; Beckman) | 1.9 (–) vs. 2.9 (–) months, 0.046 | Univariate analysis: 1.44 (1.00–2.08), 0.049Multivariate analysis: 1.72 (1.16–2.56), 0.007 | 8.6 (–) vs. 16.1 (–) months, 0.116 | Univariate analysis: 1.46 (0.91–2.33), 0.119Multivariate analysis: 1.38 (0.85–2.24), 0.200 | – | – | – |
CYFRA21-1 (≥2.5 vs. <2.5μg/L) | IRMA (titration; Beckman-Immunotech) | 1.9 vs. 3.4 months, <0.001 | Univariate analysis: 2.06 (1.45–2.95), <0.001Multivariate analysis: 2.17 (1.48–3.19), <0.001 | 6.1 (–) vs. 23.8 (–) months, <0.001 | Univariate analysis: 3.73 (2.30–6.07), <0.001 Multivariate analysis: 2.74 (1.63–4.61), <0.001 | – | – | – | |||
Takeuchi (2017) | Retrospective | Advanced NSCLC (stage IIIB/IV), squamous cell carcinoma, non-squamous cell carcinoma, adenocarcinoma, large cell carcinoma, non-small cell carcinoma, n = 95 | CEA (>5 vs. ≤5 ng/mL) | ECLIA (HISCL-5000 system; Sysmex) | 124 (–) vs. 97 (–) days, 0.757 | – | 542 (–) vs. 357 (–) days, 0.059 | – | – | – | – |
CYFRA21-1 (>3.5 vs. ≤3.5 ng/mL) | ECLIA (Lumipulse Presto II system; Fujirebio) | 99 (–) vs. 123.5 (–) days, 0.011 | Multivariate analysis: 2.17 (1.38–3.40), <0.001 | 385 (–) vs. 607 (–) days, 0.001 | Multivariate analysis: 1.07 (0.57–1.99), 0.838 | – | – | – | |||
Zhao (2017) | Retrospective | Advanced NSCLC (stage IIIB/IV), adenocarcinoma, with EGFR mutation, n = 177 | CEA (>10.0 vs. ≤10.0 ng/mL) | CLIA (ND) | 5.3 (3.6–7.0) vs. 7.8 (7.0–8.6) months, 0.029 | 1.450 (1.047–2.008), 0.025 | 11.8 (8.5–15.1) vs. 18.8 (13.4–24.2) months, <0.0001 | 2.133 (1.444–3.151), <0.0001 | 43.4 vs. 69.2, 0.001 | 0.322 (0.166–0.625), 0.001 | – |
CYFRA21-1 (>3.3 vs. ≤3.3 ng/mL) | CLIA (ND) | 5.9 (4.5–7.3) vs. 7.8 (6.6–9.0) months, 0.230 | 1.217 (0.871–1.702), 0.250 | 16.5 (12.0–21.0) vs. 14.5 (10.6–18.4) months, 0.677 | 0.864 (0.583–1.282), 0.468 | 50.0 vs. 61.3, 0.134 | 0.595 (0.294–1.201), 0.147 | – | |||
NSE (>13.7 vs. ≤13.7 ng/mL) | CLIA (ND) | 6.9 (4.7–9.1) vs. 6.6 (4.6–8.6) months, 0.995 | 0.838 (0.598–1.173), 0.302 | 14.9 (11.3–18.5) vs. 14.8 (10.6–19.0) months, 0.909 | 0.896 (0.610–1.316), 0.576 | 56.2 vs. 53.1, 0.674 | 1.724 (0.861–3.452), 0.124 | – | |||
CA19-9 (>35.0 vs. ≤35.0 U/mL) | CLIA (ND) | 5.6 (4.2–7.1) vs. 7.7 (6.1–9.3) months, 0.472 | 1.108 (0.807–1.521), 0.527 | 14.4 (9.5–19.3) vs. 14.9 (11.0–18.8) months, 0.306 | 1.277 (0.898–1.816), 0.174 | 50.6 vs. 58.2, 0.317 | 0.788 (0.416–1.492), 0.464 | – | |||
Yanwei (2016) | Retrospective | Advanced NSCLC (stage IIIA/IIIB/IV), adenocarcinoma, non-adenocarcinoma, with EGFR mutation, n = 200 | CEA (≥5.0 vs. <5.0 ng/mL) | ECLIA (Architect i2000SR system; Abbott) | 12.0 vs. 8.3 months, 0.055 | – | – | – | – | – | – |
CEA (≥20.0 vs. <20.0 ng/mL) | ECLIA (Architect i2000SR system; Abbott) | 12.8 vs. 8.7 months, 0.016 | Multivariate analysis: 1.412 (1.042–1.913), 0.026 | – | – | – | – | – | |||
CYFRA21-1 (≥3.3 vs. <3.3 ng/mL) | ECLIA (Elecsys 200 system; Roche Diagnostics) | 9.2 vs. 12.5 months, 0.086 | – | – | – | – | – | – | |||
CA125 (≥35.0 vs. <35.0 U/mL) | ELISA (3rd generation kit; Can Ag) | 10.0 vs. 12.0 months, 0.154 | – | – | – | – | – | – | |||
Chen (2015) | Retrospective | Advanced NSCLC (–), EGFR mutation, n = 241 | CEA (>32.0 vs. 5.0–32.0 vs. <5.0 ng/mL) | ND | 8.8 vs. 11.3 vs. 14.4 months, <0.001 | >32.0 vs. <5.0 ng/mL: 1.715 (1.178–2.495), 0.005 5.0–32.0 vs. <5.0 ng/mL: 1.181 (0.804–1.734), 0.40 | 17.8 (–) vs. 22.0 (–) vs. 27.9 (–) months, 0.01 | >32.0 vs. <5.0 ng/mL: 1.718 (1.060–2.782), 0.03 5.0–32.0 vs. <5.0 ng/mL: 1.526 (0.927–2.512), 0.10 | – | – | – |
CEA (trend and normalization of CEA response “yes and <5.0” vs. “yes but >5.0” vs. “no response”) | ND | 14.3 (–) vs. 10.6 (–) vs. 7.1 (–) months, <0.001 | – | 29.7 (–) vs. 20.0 (–) vs. 16.2 (–) months, <0.001 | – | – | – | – | |||
Wu (2019b) | Retrospective | Advanced NSCLC (stage IIIB/IV), adenocarcinoma, squamous cell carcinoma, other, with or without EGFR mutation, n = 301 | CEA (≥5.0 vs. <5.0 ng/mL) | ND | 9.6 (–) vs. 12.0 (–) months, 0.013 | 1.594 (–), – | – | – | – | – | – |
Wei (2016) | Retrospective | NSCLC with CM (stage I–IV), adenocarcinoma, squamous cell carcinoma, with EGFR mutation, n = 66 | CEA (>10.0 vs. ≤10.0μg/mL) | ND | 4.1 (–) vs. 9.3 (–) months, 0.035 | – | 8.7 (–) vs. 16.0 (–) months, 0.031 | – | – | – | – |
Romero-Ventosa (2015) | Cohort | NSCLC (stage I–IV), adenocarcinoma, squamous cell carcinoma, unknown, n = 58 | CEA (≥5.0 vs. <5.0 ng/mL) | ECLIA (CEA kit/Elecsys 2010 instrument; Roche Diagnostics) | 2.8 (1.2–4.3) vs. 2.8 (1.9–3.7) months, 0.155 | 0.58 (0.3–1.2), 0.161 | 10.2 (5.9–14.5) vs. 4.4 (2.7–6.1) months, <0.001 | 0.23 (–), 0.001 | – | – | – |
CYFRA21-1 (≥3.3 vs. <3.3 ng/mL) | ECLIA (CYFRA21-1 kit/Elecsys 2010 instrument; Roche Diagnostics) | 2.8 (2.5–3.0) vs. 3.2 (0.0–7.8) months, 0.317 | 1.51 (–), 0.321 | 6.5 (4.3–8.6) vs. 15.0 (0.0–31.9) months, 0.056 | 2.34 (0.95–6.0), 0.064 | – | – | – | |||
SCCA (≥1.5 vs. <1.5 ng/mL) | TRACE (Kryptor Brahms-Atom instrument; Thermo Fisher Scientific) | 2.7 (2.0–3.3) vs. 2.8 (2.0–3.7) months, 0.500 | 1.36 (0.55–3.36), 0.503 | 6.5 (4.7–8.3) vs. 7.7 (3.7–11.7) months, 0.184 | 1.87 (0.73–4.76), 0.192 | – | – | – | |||
Facchinetti (2015) | Retrospective | Advanced NSCLC (stage IIIB/IV), adenocarcinoma, other, with or without EGFR mutation, n = 79 | CEA (≥5.0 vs. <5.0 ng/mL) | CLIA (Access CEA kit/UniCel DXI 800 instrument; Beckman Coulter) | 5.4 (1.8–9.0) vs. 1.9 (0.4–3.5) months, 0.087 | – | 10.3 (8.4–12.1) vs. 5.1 (0.0–11.0) months, 0.09 | – | – | – | – |
Ding (2019) | Prospective | Advanced NSCLC (–), with EGFR mutation, n = 28 (CEA levels available for n = 22) | CEA (≥3.0 vs. <3.0μg/L) | ECLIA (cobas e 602 instrument; Roche Diagnostics) | – | – | – | 1.65 (–), 0.4 | – | – | – |
CEA (fall at 4 weeks) | ECLIA (cobas e 602 instrument; Roche Diagnostics) | – | – | – | 1.8 (–), 0.3 | – | – | – | |||
Han (2017) | Prospective | Advanced NSCLC (stage IIIB/IV), adenocarcinoma, squamous cell carcinoma, adenosquamous carcinoma, with EGFR mutation, n = 100 | CEA (>10 [high] vs. 5–10 [low] vs. <5.0 ng/mL [normal]) | Sequential CLIA (Immulite 2000 system; Siemens Healthineers) | 6.4 (–) vs. 4.5 (–) vs. 3.0 (–) months, <0.0001 | 1.25 (1.09–1.39), – | 11.9 (–) vs. 9.4 (–) vs. 7.8 (–) months, <0.0001 | – | 65.3 vs. 38.0 vs. 33.3% high vs. low: 0.035 high vs. normal: 0.006 | – | – |
Pan (2014) | Retrospective | Advanced NSCLC, (–), adenocarcinoma, n = 48 | CA19-9 (≥35.0 vs. <35.0 U/mL) | CLEIA (system not identified; Shu Kang Biotechnology) | – | – | – | – | – | – | ORR: 0.032 (0.001–0.763), 0.033 |
CEA (≥5.0 vs. <5.0 ng/mL) | CLEIA (system not identified; Shu Kang Biotechnology) | – | – | – | – | – | – | ORR: 0.077 (0.009–0.623), 0.016 | |||
Ramalingam (2015) | Randomized controlled trial | Advanced NSCLC (stage IIIB/IV), adenocarcinoma, large cell, other, n = 138 | STM panel comprising: CEA (>3.0 ng/mL) and CYFRA21-1 (<7.0 ng/mL) | ELISA (Architect system; Abbott) | linifanib 7.5 mg: 10.2 (3.9–NR) linifanib 12.5 mg: 8.3 (4.8–NR) months | linifanib 7.5 mg: 0.49 (–), 0.049 linifanib 12.5 mg: 0.38 (–), 0.029 | linifanib 7.5 mg: 12.5 (6.2–NR) linifanib 12.5 mg: 17.4 (12.9–NR) months | linifanib 7.5 mg: 1.02 (–), 0.758 linifanib 12.5 mg: 0.54 (–), 0.137 | – | – | – |
Fiala (2014b) | Non-randomized experimental | Advanced NSCLC (stage IIIB/IV), adenocarcinoma, squamous cell carcinoma, other, n = 163 | NSE (≥12.5 vs. <12.5μg/L) | IRMA (titration; Beckman-Immunotech) | 1.1 (0.8–1.3) vs. 2.6 (1.8–3.4) months, 0.002 | 2.36 (1.34–4.17), 0.003 | 3.7 (3.2–4.2) vs. 11.6 (7.4–15.9) months, 0.003 | 1.90 (0.95–3.80), 0.071 | – | – | – |
Suh (2016) | Retrospective | Advanced NSCLC (stage IIIB/IV), adenocarcinoma, other, with EGFR mutation, n = 151 | NSE (≥16.3 vs. <16.3 ng/mL) | ECLIA (cobas e 170 instrument; Roche Diagnostics) | 10.5 (8.8–12.3) vs. 15.4 (10.2–20.5) months, 0.034 | 1.656 (1.083–2.534), 0.020 | 17.0 (11.8–25.9) vs. 29.1 (21.8–36.4) months, <0.001 | 2.671 (1.612–4.427), <0.001 | – | – | – |
Inomata (2015) | Retrospective | Advanced NSCLC (stage IIIB/IV), adenocarcinoma, other, with EGFR mutation, n = 41 | Serum ProGRP (≥30.0 vs. <30.0 pg/mL) | CLEIA (ND) | No difference | – | No difference | – | – | – | – |
Plasma NSE (≥13.0 vs. <13.0 ng/mL) | RIA before December 2013 and ECLIA from December 2013 (ND) | P = 0.013 | 4.69 (1.27–19.12), 0.021 | P = 0.014 | 10.31 (2.26–59.2), 0.0024 | – | – | – |
AUC: area under curve, CA125: cancer antigen 125, CA19-9: carbohydrate antigen 19-9, CEA: carcinoembryonic antigen, CI: confidence interval, CLEIA: chemiluminescent enzyme immunoassay, CLIA: chemiluminescent immunoassay, CM: cerebral metastases, CYFRA21-1: cytokeratin 19 fragment antigen, ECLIA: electrochemiluminescence immunoassay, EGFR: epidermal growth factor receptor, ELISA: enzyme-linked immunosorbent assay, HR: hazard ratio, IRMA: immunoradiometric assay, ND: not disclosed, NSCLC: non-small cell lung cancer, NR: not reached, NSE: neuron-specific enolase, ORR: objective response rate, OS: overall survival, PD-1: programmed death receptor-1, PD-L1: programmed death-ligand 1, PFS: progression-free survival, ProGRP: progastrin-releasing peptide, RIA: radioimmunoassay, RR: response rate, SCCA: squamous cell carcinoma antigen, SCLC: small cell lung cancer, STMs: serum tumor markers, TKI: tyrosine kinase inhibitor, TRACE: time-resolved amplified cryptate emission.
Fig. 3
Summary of STM results across reviewed literature by A) predictive value of STMs in patients treated with immunotherapy; B) predictive value of STMs in patients treated with targeted therapy; C) prognostic/monitoring value of STMs in patients treated with immunotherapy; D) prognostic/monitoring value of STMs in patients treated with targeted therapy. a: Patients in the test set were treated with second-line nivolumab or atezolizumab; patients in the validation set were treated with first-line pembrolizumab. b: Correlation is based on a positive predictive value of non-response rather than on outcome. An elevation of CYFRA21-1, CEA, or NSE (≥50% compared to baseline) gave a positive predictive value of 90.3% (95% CI: 40.7–59.3) of non-response. c: Treatment modalities included: targeted treatment, immunotherapy, chemo-immunotherapy, chemotherapy, chemo-radiotherapy, radiotherapy, surgery + adjuvant chemotherapy. CA125: cancer antigen 125, CA15-3: cancer antigen 15-3, CA19-9: carbohydrate antigen 19-9, CEA: carcinoembryonic antigen, CYFRA21-1: cytokeratin 19 fragment antigen, EGFR-TKI: epidermal growth factor receptor-tyrosine kinase inhibitor, HE4: human epididymal protein 4, IT: immunotherapy, NSE: neuron-specific enolase, PD-L1: programmed death-ligand 1, ProGRP: progastrin-releasing peptide, SCCA: squamous cell carcinoma antigen, STMs: serum tumor markers, TT: targeted therapy.
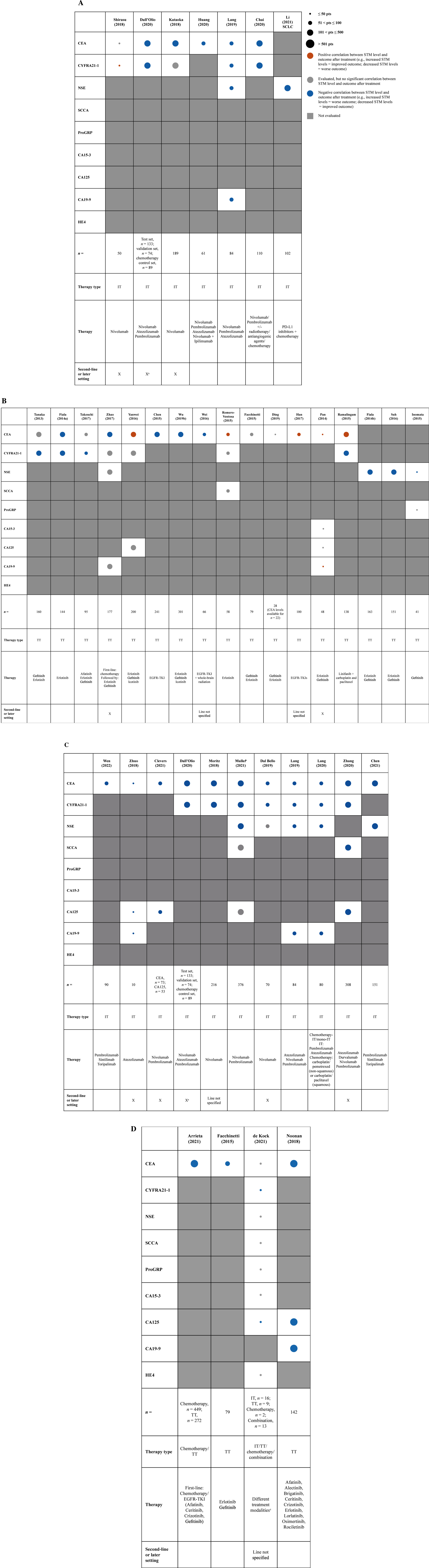
3.1Predictive STMs in patients treated with immunotherapy
Increased baseline levels of serum CYFRA21-1 and CEA may predict immunotherapy treatment response in patients with advanced NSCLC or SCLC. Shirasu et al. [47] described pre-treatment CYFRA21-1 levels ≥2.2 ng/mL as an independent predictor of prolonged PFS in patients with advanced NSCLC (n = 50) receiving second- or later-line nivolumab (median PFS 155 vs. 51.5 days for CYFRA21-1 <2.2 ng/mL) [47]. In contrast, Dall’Olio et al. [48] reported that patients with stage IIIB/IV NSCLC who received anti PD-1/PD-L1 treatment and had baseline CYFRA21-1 levels >8 ng/mL had shorter OS than patients with baseline CYFRA21-1 levels ≤8 ng/mL, whilst baseline CEA levels >8 ng/mL were not correlated with OS [48].
In a retrospective study, Kataoka et al. [49] found that high CEA expression (≥13.8 ng/mL) was independently associated with poorer PFS in patients who received nivolumab as a second- or later-line treatment for advanced NSCLC (n = 189) [49]. Similarly, in patients treated with anti-PD-1/anti-PD-L1/anti-CTLA-4 therapies (n = 61), Huang et al. [50] reported that elevated pre-treatment CEA levels ≥5 ng/mL were associated with significantly shorter PFS [50]. Thus, measurement of pre-treatment serum CYFRA21-1 or CEA levels may aid prediction of response to immunotherapy; however, the appropriate cut-off must be examined further.
Lang et al. [51] examined the predictive performance of a panel of STMs, including CEA, CA19-9, CYFRA21-1, and NSE in patients with advanced NSCLC treated with ICI monotherapy (n = 84) [51]. Using receiver operating characteristic curve analyses, baseline levels of CYFRA21-1 were found to have the best predictive power for disease progression or death, followed by CA19-9, CEA, and NSE [51]. Chai et al. [52] developed an OS probability nomogram to evaluate individual risk for patients prior to PD-1 inhibitor treatment (n = 110). The study reported that several of the parameters examined, including baseline CEA and CYFRA21-1 levels, were positively correlated with the individual’s risk score, such that higher baseline levels were associated with a higher risk score and hence a shortened OS [52]; however, this study has several limitations. Given the small sample size and, most strikingly, the absence of a comparison against data from patients receiving standard or no therapy, the predictive role of the analyzed biomarkers emerging from this study can only be speculated.
In patients with advanced SCLC receiving first-line PD-1/PD-L1 inhibitors plus chemotherapy (n = 102), Li et al. [53] noted that increased serum NSE levels at baseline and at 3 weeks post-treatment were correlated with poorer clinical outcomes [53]. Further investigation into the value of baseline serum NSE levels for predicting therapy response in a larger population of patients with advanced SCLC appears warranted.
3.2Predictive STMs in patients treated with targeted therapy
Several studies have reported an association between high pre-treatment CYFRA21-1 levels and shorter PFS in patients with advanced NSCLC treated with TKI therapy. Tanaka et al. [54] found that high CYFRA21-1 levels (>2 ng/mL) were associated with significantly shorter PFS in patients with EGFR-mutated NSCLC (n = 160) receiving EGFR-TKIs [54]. Fiala et al. [55] also reported shorter PFS in patients with advanced NSCLC treated with erlotinib (n = 144) who had high vs. low pre-treatment CYFRA21-1 levels (≥2.5 vs. <2.5μg/L) [55]. Takeuchi et al. [11] noted that patients with advanced NSCLC treated with EGFR-TKIs (n = 95) and presenting elevated baseline serum CYFRA21-1 levels had shorter PFS than those with normal baseline serum CYFRA21-1, regardless of EGFR status [11]. Conversely, Zhao et al. [8] and Yanwei et al. [9] found no significant association between PFS and baseline serum CYFRA21-1 levels in patients with advanced NSCLC treated with EGFR-TKIs (n = 177 and n = 200, respectively) [8, 9]. Yanwei et al. [9] also reported no significant difference in PFS between patients with normal and high serum CA125 levels [9]. Discrepancies between the results of these two studies and most other studies regarding CYFRA21-1 may be due to differences in ethnicity of the patient population, as both studies were conducted in China, or might indicate that the cut-off value chosen (3.3 ng/mL) was not sufficiently discriminatory.
High pre-treatment levels of CEA have also been found to be an independent predictor of outcomes in patients with advanced NSCLC treated with EGFR-TKIs, although the results are again conflicting, possibly due to differences in trial design, patient characteristics, or the differing cut-off thresholds used. Fiala et al. [55] observed significantly shorter PFS in patients with a high vs. low pre-treatment CEA level (≥3 vs. <3μg/L; n = 144) [55]. Zhao et al. [8] reported that baseline CEA expression ≤10 ng/mL predicted favorable outcomes in patients with advanced NSCLC and EGFR mutations (n = 177) [8]. Chen et al. [56] investigated the predictive value of CEA in patients with advanced EGFR-positive NSCLC treated with first-line EGFR-TKIs (n = 241) and found that patients with elevated baseline CEA levels had reduced PFS [56]. Wu et al. [57] also reported a significant association between higher pre-treatment CEA levels (≥5μg/mL) and shorter PFS in patients with EGFR mutations treated with first-line EGFR-TKI therapy [57]. In a subset of patients with EGFR-positive NSCLC with brain metastases (n = 66) who had undergone whole-brain radiation therapy prior to EGFR-TKI treatment, Wei et al. [58] noted that pre-treatment CEA levels ≤10μg/mL were associated with significantly longer PFS and OS compared with CEA >10μg/mL [58]. Conversely, Romero-Ventosa et al. [59] reported that patients (n = 58) with CEA levels ≥5 ng/mL prior to treatment with the EGFR-TKI erlotinib had significantly longer OS compared with patients who had lower CEA levels, but changes in PFS were not significant [59]. The authors concluded that pre-treatment CEA levels may provide similar predictive information to EGFR mutation status. The study also reported no significant correlation between pre-treatment CYFRA 21-1 or SCCA levels and PFS or OS.
Facchinetti et al. [60] examined the correlation between survival and baseline CEA levels (≥5 vs. <5 ng/mL) in patients with EGFR mutations or wild-type/unknown EGFR status and found no significant relationship between OS and CEA levels in the total patient population (n = 79) [60]. Similarly, Yanwei et al. [9] found that in patients with advanced EGFR-mutated NSCLC who received EGFR-TKI treatment (n = 200), patients with higher baseline CEA levels (≥20 ng/mL) had significantly prolonged PFS as compared to those with lower CEA levels [9]; however, PFS did not differ significantly between the patients when using a cut-off of 5 ng/mL. This may be due to an inappropriate threshold being used. Ding et al. [61] reported that for patients with advanced EGFR-positive NSCLC (n = 22) treated with gefitinib or erlotinib, high baseline CEA levels (>3μg/L) did not predict higher metastatic disease burden or reduced survival times [61]. In addition, no correlation between an early decrease (within 4 weeks of beginning of treatment) in CEA levels and treatment response or survival was observed. Han et al. [62] examined whether CEA levels could predict acquired EGFR-TKI resistance in patients enrolled for palliative care with EGFR-TKI therapy (n = 100). Whilst the study found that pre-treatment CEA levels did not predict acquired EGFR-TKI resistance, patients with high pre-treatment CEA levels (>10 ng/mL) benefited more from treatment in terms of PFS and OS than patients with low (5–10 ng/mL) or normal (<5 ng/mL) CEA levels [62].
In a retrospective cohort study, Pan et al. [63] analyzed the correlation between pre-treatment STM levels and response to EGFR-TKIs in patients with stage IIIB/IV lung adenocarcinoma (n = 48). Patients with elevated pre-treatment levels of serum CEA (≥5 ng/mL) and CA19-9 (≥35 U/mL) had a greater disease control rate (DCR; defined as a partial or complete response or stable disease ≥6 weeks) and prolonged survival time, but pre-treatment serum CA15-3 and CA125 levels were not related to outcomes [63]. Furthermore, in a randomized controlled trial, Ramalingam et al. [64] evaluated a panel comprising CEA and CYFRA21-1 for its predictive response to treatment with linifanib (a multi-targeted receptor TKI), in combination with carboplatin and paclitaxel as first-line therapy for patients with advanced, non-squamous NSCLC (n = 138) [64]. A high CEA (>3 ng/mL) and low CYFRA21-1 (<7 ng/mL) signature at baseline was associated with significantly improved PFS in both treatment arms (linifanib 7.5 and 12.5 mg) [64]. Conversely, Takeuchi et al. [11] reported no association between elevated pre-treatment CEA levels (>5 ng/mL) and either PFS or OS in patients with advanced NSCLC who were treated with first- and second-generation TKIs (n = 95) [11].
An association has also been reported between baseline plasma NSE levels and response to treatment in patients receiving targeted therapy. Fiala et al. [65] reported significantly shorter PFS in patients with high (≥12.5μg/L) vs. low (<12.5μg/L) pre-treatment NSE levels receiving EGFR-TKIs (erlotinib or gefitinib; n = 163) [65]. Suh et al. [66] also found that increased baseline NSE levels were associated with significantly shorter PFS following treatment with erlotinib or gefitinib (n = 151) [66]. However, Zhao et al. [8] found no significant difference in PFS between patients with low (≤13.7 ng/mL) or elevated (>13.7 ng/mL) serum NSE levels (n = 177) [8]. The predictive value of CA19-9 was also evaluated in this study (cut-off: 35 U/mL), but no significant difference in PFS was observed between low and high expression of this marker [8]. In gefitinib-treated patients with EGFR-mutated NSCLC and available baseline data (n = 22), Inomata et al. [67] found that higher pre-treatment NSE levels were significantly associated with shorter PFS and OS; however, there was no association between pre-treatment serum ProGRP level (n = 31) and PFS or OS in this setting [67].
4Monitoring STMs (measured serially)
4.1Monitoring STMs in patients treated with immunotherapy
Several studies have investigated the value of dynamic changes in STMs for monitoring immunotherapy treatment response, to allow for cost-effective and timely changes in treatment, if required (Table 3; Fig. 3C).
Table 3
Summary of outcomes for monitoring STMs in
Reference | Study | Disease | STM | Assay | PFS | OS | RR | Other | |||
(year) | type | (stage), histology, n | (cut off) | method (kit/instrument) | Median PFS (95% CI), P-value | HR (95% CI), P-value | Median OS (95% CI), P-value | HR (95% CI), P-value | % Responders, P-value | HR (95% CI), P-value | parameters |
Monitoring STMs in patients treated with immunotherapy | |||||||||||
Wen (2022) | Retrospective | Advanced NSCLC (stage IIIB/IV), adenocarcinoma, squamous, n = 90 | CEA (increase or decrease, 6 weeks post treatment) | ND | – | Multivariate analysis: 1.81 (1.091–3.003), 0.022 | – | – | – | – | – |
Zhuo (2018) | Observational | Advanced NSCLC (stage IIIB/IV), adenocarcinoma, squamous, n = 10 | CEA, CA125, or CA19-9 (at least two >50% increase over baseline) | Microparticle CLIA (Architect i2000SR system; Abbott) | – | – | – | – | – | – | – |
Clevers (2021) | Retrospective, observational | Advanced NSCLC (stage IIIB/IV), adenocarcinoma, squamous, other, n = 136a | CEA (increase or decrease vs. baseline) | ECLIA (cobas 3000 analyzer series; Roche Diagnostics) | – | – | – | – | – | – | AUC for disease progression (95% CI): 0.6487 (0.525–0.771) |
CA125 (increase or decrease vs. baseline) | ECLIA (cobas 3000 analyzer series; Roche Diagnostics) | – | – | – | – | – | – | AUC for disease progression (95% CI): 0.5871 (0.424–0.751) | |||
Dall’Olio (2020) | Retrospective | Advanced NSCLC (stage IIIB/IV), squamous, non-squamous, test set, n = 133; validation set, n = 74; chemotherapy control set, n = 89 | CEA (≥20% decrease vs. no decrease) | CLIA (Access CEA kit/DXI instrument; Beckman Coulter) | – | – | NR (NR–NR) vs. 4.0 (2.1–5.9) months, – | 0.12 (0.04–0.33), <0.001 | – | – | DCR (95% CI), P-value: 12.28 (2.57–58.59), 0.002 |
CYFRA21-1 (≥20% decrease vs. no decrease) | TRACE (Kryptor compact plus; Thermo Fisher Scientific) | – | – | NR (NR–NR) vs. 4.0 (2.0–5.0) months, – | 0.19 (0.07–0.55), 0.002 | – | – | DCR (95% CI), P-value: 7.50 (1.73–33.03), 0.008 | |||
Moritz (2018) | Diagnostic test accuracy | Metastatic NSCLC (–), –, n = 216 | CEA, CYFRA21-1 | ECLIA (cobas 6000 analyzer series; Roche Diagnostics) | – | – | – | – | Increases of >50% in CYFRA21-1 levels were associated almost exclusively with non-response; CEA increases were associated with an increased percentage of non-responding patients | – | – |
Muller (2021) | Prospective, observational | NSCLC (–), adenocarcinoma, squamous cell, other, n = 376 | CEA (6μg/L) 6 weeks after treatment initiation | ECLIA (cobas 6000 analyzer series; Roche Diagnostics) | – | – | – | – | – | – | Specificity (95% CI): 98.3% (90.9–100) |
CYFRA21-1 (4μg/L) 6 weeks after treatment initiation | ECLIA (cobas 6000 analyzer series; Roche Diagnostics) | – | – | – | – | – | – | Specificity (95% CI): 91.8% (81.9–97.3) | |||
NSE (20μg/L) 6 weeks after treatment initiation | ECLIA (cobas 6000 analyzer series; Roche Diagnostics) | – | – | – | – | – | – | Specificity (95% CI): 96.5% (87.9–99.6) | |||
SCCA (3.5μg/L) 6 weeks after treatment initiation | TRACE (Kryptor system; Thermo Fisher Scientific) | – | – | – | – | – | – | Specificity (95% CI):96.5% (88.1–99.6) | |||
CA125 (65 U/mL) 6 weeks after treatment initiation | ECLIA (cobas 6000 analyzer series; Roche Diagnostics) | – | – | – | – | – | – | Specificity (95% CI):86.0% (74.2–93.7) | |||
Dal Bello (2019) | Non-randomized experimental | Advanced NSCLC (stage IIIB/IV), adenocarcinoma, squamous cell, other, n = 70 | CEA (≥20 vs. <20% reduction from baseline) | Microparticle CLIA (Architect system; Abbott) | 7.1 (–) vs. 1.9 (–) months, 0.028 | – | 15.0 (12.7–17.3) vs. 9.9 (8.5–11.3) monthsb, 0.026 | – | 43.5 vs. 56.5, 0.021 | – | – |
CYFRA21-1 (≥20 vs. <20% reduction from baseline) | IRMA (Cytokeratin 19 Fragment Kit; Beckman Coulter) | 7.9 (–) vs. 1.9 (–) months, <0.001 | 0.35 (0.20–0.60), <0.001 | 14.6 (12.4–16.8) vs. 10.0 (8.4–11.6) monthsb, 0.019 | 0.55 (0.28–1.07), 0.079 | 62.5 vs. 37.5, <0.001 | – | – | |||
NSE (≥20 vs. <20% reduction from baseline) | IRMA (NSE Kit; Beckman Coulter) | 4.7 (–) vs. 1.9 (–) months, 0.300 | – | 12.4 (9.8–15.0) vs. 11.6 (9.9–13.4) monthsb, 0.950 | – | 47.6 vs. 52.4, 0.21 | – | – | |||
Lang (2019) | Retrospective | Advanced NSCLC (stage IV; stage III if otherwise untreatable/CM), adenocarcinoma, squamous cell carcinoma, n = 84 | STM panel comprising: CEA, CA19-9, CYFRA21-1, NSE (STM panel decrease vs. increase <two-fold vs. increase ≥two-fold from baseline) | ECLIA (individual kits/cobas e 801 instrument; Roche Diagnostics) | 11 (7–19) vs. 6 (3–8) vs. 2 (1–2) months, <0.001 | Multivariate analysis: Increase <two-fold: 1.826 (–), 0.076Increase ≥two-fold: 9.075 (–), <0.001 | NR (NR) vs. 14 (12–26) vs. 4 (3–7) months, <0.001 | Multivariate analysis: Increase <two-fold: 1.576 (–), 0.305Increase ≥two-fold: 21.123 (–), <0.001 | – | – | – |
Lang (2020) | Retrospective | Advanced NSCLC (stage III/IV), adenocarcinoma, squamous cell carcinoma, n = 80 | CEA, CYFRA21-1, CA19-9, and NSE (increase from baseline to re-staging) | ECLIA (individual kits/cobas e 801 instrument; Roche Diagnostics) | 3.5 (2–6) months, <0.001 | – | NR (7–NR) months, 0.055 | – | – | – | – |
CEA, CYFRA21-1, CA19-9, and NSE (decrease from baseline to re-staging) | ECLIA (individual kits/cobas e 801 instrument; Roche Diagnostics) | 16 (7–NR) months, <0.001 | – | NR (NR–NR) months, 0.055 | – | – | – | – | |||
CEA, CYFRA21-1, CA19-9, and NSE (increase from baseline to re-staging) | ECLIA (individual kits/cobas e 801 instrument; Roche Diagnostics) | 5 (3–6) months, 0.042 | – | NR (10–NR) months, 0.363 | – | – | – | – | |||
CEA, CYFRA21-1, CA19-9, and NSE (decrease from baseline to re-staging) | ECLIA (individual kits/cobas e 801 instrument; Roche Diagnostics) | 9 (5–12) months, 0.042 | – | 15 (10–NR) months, 0.363 | – | – | – | – | |||
Zhang (2020) | Observational | Advanced NSCLC (stage IIIB/IV), adenocarcinoma, squamous cell, other, n = 308 | CEA, CA125, CYFRA21-1, SCCA (improvement in ≥2 vs. <2 out of 4 STMs) | ECLIA (CEA, CA125, and CYFRA21-1 kits; Roche Diagnostics) and microparticle CLIA (SCCA; Architect SCC kit; Abbott); instruments ND | 12.5 (–) vs. 5.4 (–) months, <0.001 | Original: 0.45 (0.34–0.59) –Weighted: 0.48 (0.36–0.62), <0.001 | 25.6 (–) vs. 11.7 (–) months, <0.001 | Original: 0.45 (0.33–0.62) –Weighted: 0.45 (0.32–0.61), <0.001 | – | – | Original ORR (95% CI), P-value: 0.35 (0.25–0.45) vs. 0.08 (0.04–0.12), <0.001Weighted ORR (95% CI), P-value: 0.36 (0.25–0.45) vs. 0.07 (0.04–0.12), <0.001 |
Chen (2021) | Retrospective | Advanced or relapsed NSCLC (stage IIIB/IV), adenocarcinoma, squamous, non-squamous, other, n = 151 | CEA (decrease vs. increase at 6 weeks post-treatment) | ND | 10.5 (8.6–12.4) vs. 6.6 (5.4–7.8) months, <0.001 | 0.477 (0.320–0.710),<0.0001 | 16.5 (15.6–17.4) vs. 13.5 (12.6–14.4) months, 0.001 | 0.543 (0.339–0.871), 0.011 | – | – | – |
CEA (decrease vs. increase at 12 weeks post-treatment) | ND | 11.2 (10.2–12.2) vs. 6.0 (4.6–7.4) months, <0.001 | 0.406 (0.270–0.609),<0.0001 | 16.2 (15.1–17.3) vs. 13.8 (12.5–15.1) months, 0.065 | 0.620 (0.390–0.986), 0.043 | ORR: 2.469 (1.134–5.375), 0.023 | – | – | |||
NSE (decrease vs. increase at 6 weeks post-treatment) | ND | – | – | 15.8 (14.6–17.0) vs. 13.6 (12.7–14.5) months, 0.006 | 0.619 (0.386–0.994), 0.047 | – | – | – | |||
NSE (decrease vs. increase at 12 weeks post-treatment) | ND | – | – | 15.3 (13.5–17.1) vs. 15.3 (12.9–17.7) months, 0.020 | 0.578 (0.353–0.947), 0.029 | – | – | – | |||
Monitoring STMs in patients treated with targeted therapy | |||||||||||
Arrieta (2021) | Retrospective | Advanced NSCLC (stage III/IV), adenocarcinoma, other, n = 748 | CEA (10% decrease from baseline absent vs. 10% decrease from baseline present) | Sequential CLIA (Immulite 2000 system; Siemens Healthineers) | 7.7 (4.6–16.7) vs. 5.9 (2.9–9.2) months, – | Adjusted: 0.71 (0.574–0.885), 0.002 | – | 0.75 (0.613–0.919), 0.006 | – | – | – |
CEA (20% decrease from baseline absent vs. 20% decrease from baseline present) | Sequential CLIA (Immulite 2000 system; Siemens Healthineers) | 11.9 (5.1–23.6) vs. 7.3 (2.9–11.9) months, – | Adjusted: 0.67 (0.503–0.887), 0.005 | – | 0.77 (0.585–1.011) – | – | – | – | |||
Facchinetti (2015) | Retrospective | Advanced NSCLC (stage IIIB/IV), adenocarcinoma, other, with or without EGFR mutation, n = 79 | CEA (≥20% reduction vs. <20% reduction) | CLIA (Access CEA kit/UniCel DXI 800 instrument; Beckman Coulter) | 8.0 (–) vs. 2.1 (–) months, 0.002 | Multivariate analysis: 0.4 (0.2–0.7), 0.003 | 15.5 (–) vs. 7.7 (–) months, 0.0019 | Multivariate analysis: 0.8 (0.4–1.4), 0.380 | 87 vs. 13, <0.001 | – | – |
de Kock (2021) | Non-randomized experimental | NSCLC, n = 39; SCLC, n = 1 (stage IB–IVB), adenocarcinoma, squamous cell, not otherwise specified, unknown, n = 40 | CA125 | ECLIA (Elecsys CA125 II kit; Roche Diagnostics) | – | – | – | – | – | – | Median percentage change (Q1, Q3): PR: –63 (–83, –30)PD: 90 (54, 102)PR vs. PD: P = 0.042 |
CYFRA21-1 | ECLIA (Elecsys CYFRA21-1 kit; Roche Diagnostics) | – | – | – | – | – | – | Median percentage change (Q1, Q3):PR: –67 (–76, –59) PD: –6 (–10, 19)PR vs. PD: P = 0.020 | |||
CEA | ECLIA (Elecsys CEA kit; Roche Diagnostics) | – | – | – | – | – | – | NS | |||
CA15-3 | ECLIA (Elecsys CA15-3 II kit; Roche Diagnostics) | – | – | – | – | – | – | NS | |||
HE4 | ECLIA (Elecsys HE4 kit; Roche Diagnostics) | – | – | – | – | – | – | NS | |||
NSE | ECLIA (Elecsys NSE kit; Roche Diagnostics) | – | – | – | – | – | – | NS | |||
ProGRP | ECLIA (Elecsys proGRP kit; Roche Diagnostics) | – | – | – | – | – | – | NS | |||
SCCA | ECLIA (Elecsys SCCA kit; Roche Diagnostics) | – | – | – | – | – | – | NS | |||
Noonan (2018) | Retrospective | Advanced NSCLC (stage IV), adenocarcinoma with EGFR or KRAS mutation or ALK or ROS1 rearrangement, n = 142 | CEA, CA125, CA27-29, CA19-9 | ND | – | – | – | – | – | – | Of the patients with systemic disease progression, 53% with elevated CEA at baseline also had elevated tumor markers at progression. Of the patients with CNS only progression, 22% with elevated tumor markers at baseline had an increase (≥10% from nadir) at CNS progression |
a: n = 136 patients recruited in total, n = 73 patients with CEA levels measured, and n = 53 patients with CA125 levels measured. b: Mean OS is presented rather than median OS as median OS was not reached. ALK: anaplastic lymphoma kinase, AUC: area under curve, CA125: cancer antigen 125, CA15-3: cancer antigen 15-3, CA19-9: carbohydrate antigen 19-9, CA27-29: cancer antigen 27–29, CEA: carcinoembryonic antigen, CI: confidence interval, CLIA: chemiluminescent immunoassay, CM: cerebral metastases, CNS: central nervous system, CYFRA21-1: cytokeratin 19 fragment antigen, DCR: disease control rate, ECLIA: electrochemiluminescence immunoassay, EGFR: epidermal growth factor receptor, HE4: human epididymal protein 4, HR: hazard ratio, IRMA: immunoradiometric assay, KRAS: Kirsten rat sarcoma virus; ND: not disclosed, NR: not reached, NS: not significant, NSCLC: non-small cell lung cancer, NSE: neuron-specific enolase, ORR: objective response rate, OS: overall survival, PD: progressive disease, PFS: progression-free survival, PR: partial response, ProGRP: progastrin-releasing peptide, Q1: first quartile, Q3: third quartile, ROS1: proto-oncogene 1, receptor tyrosine kinase, RR: response rate, SCCA: squamous cell carcinoma antigen, SCLC: small cell lung cancer, STMs: serum tumor markers, TRACE: time-resolved amplified cryptate emission.
Wen et al. [68] reported that patients (n = 90) with decreased CEA levels 6 weeks after anti-PD-1-based immunotherapy relative to baseline had significantly improved disease remission rates and significantly prolonged PFS [68]. In a small observational study (n = 10), Zhuo et al. [69] found that an increase in CEA, CA125, or CA19-9 of >50% from baseline was associated with disease progression after treatment with atezolizumab, although inferences from this study must be made cautiously due to the very small number of patients enrolled [69]. Clevers et al. [70] reported that CEA or CA125 levels elevated from baseline could be predictive of tumor progression in patients receiving PD-L1 inhibitors, with positive predictive values of 80% (n = 73) and 75.9% (n = 53), respectively [70]. Dall’Olio et al. [48] reported that a 20% decrease from baseline in CEA or CYFRA21-1 levels after the third anti-PD-1/PD-L1 treatment cycle was associated with a significant increase in DCR and OS [48].
To support the predictive validation and clinical interpretation of longitudinal STMs, Moritz et al. [71] developed a biomarker response characteristic plot and demonstrated an association between CEA and CYFRA21-1 levels and clinical outcome (“non-response”, defined as progressive disease based on radiologic observations after 6 months of nivolumab treatment) in a cohort of patients with metastatic NSCLC (n = 216) [71]. Based on these plots, CEA and CYFRA21-1 tests were designed for the detection of treatment failure, with a specificity of 96% and sensitivity of 34% and 35% respectively.
The value of serially assessing a panel of STMs has also been examined. Muller et al. [72] showed that “non-response” (defined as disease control for <6 months, determined by radiologic assessment) could be demonstrated using a panel of STMs comprising CEA, NSE, SCCA, CYFRA21-1, and CA125 in patients with NSCLC treated with nivolumab or pembrolizumab (n = 376) [72]. By comparing baseline STM values with follow-up values measured 6 weeks after initiation of immunotherapy, specificities of 86% for CA125, 92% for CYFRA21-1, and >96% for CEA, NSE, and SCCA were calculated in the validation cohort. Moreover, combined measurement of CYFRA21-1, CEA, and NSE could predict non-response in 32.2% of patients with a specificity of 95.2% [72]. The authors concluded that these serum marker tests can accurately detect a lack of response to treatment and may have value in supporting a decision to discontinue treatment.
Serial measurement of a panel of STMs could also have value in monitoring for response instead of non-response to immunotherapy; however, while this may instill confidence relating to continuation of treatment, it will likely have less impact on clinical care than monitoring for non-response. In a small cohort of patients with advanced NSCLC (n = 70), Dal Bello et al. [12] reported that a decrease in CEA of ≥20% below the baseline measurement after four cycles of nivolumab was significantly associated with favorable DCR, PFS, and OS [12]. In addition, a decrease in CYFRA21-1 of ≥20% below baseline was significantly predictive of DCR and PFS [12]. The authors concluded that a reduction in NSE was not significant for monitoring the efficacy of nivolumab; however, it must be noted that only 20% of patients had NSE pre-treatment levels above the upper limit of normal (ULN), compared with 57% who had pre-treatment CEA levels and 64% with pre-treatment CYFRA21-1 levels above the ULN, thereby restricting the inferences that can be drawn from these data [12]. In a retrospective cohort study that evaluated the monitoring and prognostic value of serum CEA, CA19-9, CYFRA21-1, and NSE levels in patients treated with ICIs (n = 84), Lang et al. [51] reported that a model based on a leading STM from a panel of several markers surpassed the power of single analyses for STM dynamics [51]. Specifically, a decrease in the leading STM change at the first restaging was predictive of prolonged PFS and OS [51]. In a subsequent report in patients with advanced NSCLC receiving chemo-immunotherapy (n = 80; first-line or later), Lang et al. [10] found that a decrease in the leading serum marker level at first restaging under chemotherapy-ICI combination therapy was associated with significantly longer PFS [10]. Decreased STM levels were also associated with significantly longer PFS in patients receiving subsequent mono-ICI maintenance therapy, demonstrating that change in leading STM levels can provide additional value to radiologic monitoring. Conversely, Zhang et al. [13] found that a ≥20% decrease in levels of fewer than two of the four STMs measured (CEA, CA125, CYFRA21-1, and SCCA) after 6 weeks of treatment was associated with a significantly lower objective response rate, and shorter median PFS and OS in a cohort of Chinese patients with advanced NSCLC (n = 308) [13]. However, baseline elevations of the STMs varied considerably (55%, 52%, 60%, and 30% of patients, respectively, had levels of CEA, CA125, CYFRA21-1, and SCCA above the ULN), potentially confounding the data. Chen et al. [73] reported that, in patients receiving PD-1 inhibitor-based combination therapy (n = 151) with a median follow-up duration of 20.4 months, decreased post-treatment levels of CEA were independent predictors of treatment response (6 weeks post-treatment, odds ratio [OR]: 4.209; 12 weeks post-treatment, OR: 7.267) [73]. Taken together, the findings from these studies suggest that a panel of STMs including CYFRA21-1, CEA, NSE, CA125, CA19-9, and SCCA could aid treatment monitoring in patients with advanced lung cancer receiving immunotherapy.
4.2Monitoring STMs in patients treated with targeted therapy
The monitoring value of STMs has also been investigated in patients with advanced NSCLC receiving targeted therapies (Table 3; Fig. 3D). Arrieta et al. [74] reported that prolonged PFS and OS correlated with a decrease in CEA levels from baseline throughout first-line chemotherapy or TKI therapy (n = 721) [74]. Facchinetti et al. [60] reported that, in patients with tumors harboring EGFR mutations or with wild-type/unknown EGFR status, those showing a ≥20% reduction compared with baseline CEA levels 4 weeks post TKI-treatment had significantly prolonged OS and PFS compared with patients who had a smaller reduction in CEA [60]. The effect on OS was maintained in a subset of patients with wild-type/unknown EGFR status but not in patients with EGFR mutation-positive tumors. These findings suggest that serum CEA measurement could provide a non-invasive method for monitoring in advanced NSCLC, but further investigation and validation, particularly surrounding the relationship with EGFR mutation status, will be necessary to confirm this.
de Kock et al. [75] evaluated the value of CA125, CEA, CA15-3, CYFRA21-1, HE4, NSE, ProGRP, and SCCA in patients with NSCLC (n = 39) and SCLC (n = 1) who received immunotherapy (40%), targeted therapy (23%), chemotherapy (5%), or a combination of these [75]. The STMs with a baseline concentration exceeding specified cut-off values were monitored. Of these, the median percent changes in CA125 and CYFRA21-1 levels were significantly lower (CA125: n = 20, P = 0.042; CYFRA21-1: n = 25, P = 0.020) in patients with partial response compared with levels in patients who had progressive disease at the first computed tomography scan. No significant change was observed for the other STMs evaluated. Thus, measurement of CYFRA21-1 and possibly CA125 levels could aid monitoring therapy response and early detection of disease progression in patients with lung cancer.
Noonan et al. 2018 measured CEA, CA125, CA19-9, and cancer antigen 27–29 (CA27-29) levels during TKI treatment in patients whose tumors harbored EGFR (n = 50) or KRAS (n = 28) mutations, or ALK (n = 60) or ROS1 (n = 4) rearrangements, and reported that a biomarker increase of ≥10% from nadir (the lowest level reached after starting treatment before disease progression) was observed in 53% of patients with systemic progression and 22% of patients with central nervous system-only progression [76]. CEA was elevated in 82% of patients diagnosed with NSCLC at stage IV but was only elevated in 50–52% of patients diagnosed at earlier stages, highlighting the stage dependency of CEA.
5Discussion
This scoping review gathered current evidence around the predictive and monitoring value of existing circulating STMs for patients with advanced NSCLC or SCLC receiving immunotherapy or targeted therapy. The search retrieved 362 publications, of which 36 relevant articles were included in this review. Of the 36 included, 29 described retrospective studies, two were observational studies, three were non-randomized experimental studies, one was a randomized controlled study, and one was a diagnostic test accuracy study. Only one SCLC study was identified by the search and included in this review (Li et al. [53]), highlighting a clear knowledge gap for this indication. The majority of studies assessed individual biomarkers, and the STMs most often examined were CEA, CYFRA21-1, and NSE. Measuring a panel of STMs also appears to be a promising approach, based on both the published articles found in our literature search and data presented at recent international congresses [77–81]. Overall, we observed that i) STMs may add value for predicting treatment response in patients with advanced lung cancer receiving immunotherapy (Fig. 3A), and ii) STMs may be useful as a monitoring tool for patients with advanced lung cancer receiving either targeted therapy or immunotherapy (Fig. 3C and D). The predictive value of STMs for patients treated with targeted therapy (Fig. 3B) is less clear, with several studies reporting conflicting results, possibly due to differences in the timing of baseline measurements, patient ethnicity, and the STM level cut-off thresholds used [8, 9, 11]. Another explanation might be that for targeted treatments, the mechanism of treatment resistance and the onset thereof has more of an influence on PFS and OS than STM levels prior to treatment.
It is important to note that many of the studies evaluated did not discriminate between predictive STMs (those measured at baseline and being indicative of therapeutic effect) and prognostic STMs (those indicative of patient outcome, independent of therapeutic agent received). Instead, these studies only showed a relationship between baseline STM measurements and patient outcome. Moreover, separating prognostic significance and the prediction of treatment effects remains difficult, particularly in studies lacking a control arm, or when there is no direct mechanistic connection between a given STM and a therapeutic agent. In light of this, we must consider whether high STM levels at baseline could be indicative of a more advanced or aggressive disease at treatment initiation (compared with lower STM levels), rather than specifically being predictive of an unfavorable response to treatment.
Whilst the predictive benefit of STMs is unclear, particularly for patients receiving targeted treatments, one could compare them to other indicators of therapeutic response such as PD-L1 staining [82], tumor mutational burden [83], and EGFR-mutation status [84]. These indicators are not overly precise or specific, nor do they have widely accepted cut-off thresholds, yet they are still useful for guiding therapeutic decision making. Provided STMs give clear predictive information on the effectiveness or non-effectiveness of immunotherapies and targeted therapies for a proportion of patients (e.g., for 30% of patients with 95% specificity), they could have real clinical value, particularly as more treatment options become available and predictive STMs become better characterized and validated.
When serial STM measurements were used to monitor response to treatment for both immunotherapy and targeted therapy, all studies evaluated presented a negative correlation between STM concentration change and clinical outcome (Fig. 3C and D), meaning that increases in STM levels after the start of treatment were associated with worse clinical outcomes and vice versa. Since all studies showed this relationship, this demonstrates the potential utility of STMs as a tool to monitor targeted treatment and immunotherapy in patients with advanced lung cancer. As cancer treatment becomes ever more personalized, based on the precise genetic alterations detected in each tumor, continued research into the most appropriate STM for use during therapeutic monitoring will also be necessary. Increasing coordination between drug and diagnostics development arms is likely to be paramount in the future, to develop both a mutational test and a specific monitoring assay to correlate with each precisely targeted treatment agent.
Based on this review, there is currently no consensus on i) the optimal STM sampling time points for baseline and serial measurements, ii) the cut-off thresholds and change in STMs that can be used to predict or monitor response/non-response, iii) the relevant clinical events to predict or monitor therapeutic efficacy, and iv) the required sensitivity, specificity, or positive/negative predictive values to enable clinical application. An additional relevant consideration concerns the unmet clinical need and for what clinical purpose the STM can be of most value. For monitoring, this could be accurate detection of response or non-response to treatment to support either continuation or discontinuation of treatment. For instance, monitoring STMs may provide clinical utility in patients treated with immunotherapy where radiologic follow-up may be difficult to interpret due to phenomena like pseudo-progression or the absence of target lesions.
Measurement of STMs has several advantages, including low costs, general availability, low risk to the patient, and short turn-around times. STM monitoring may qualify as informative companion diagnostics during treatment and over the course of the disease. A major challenge and complication, however, is that STM tests are not well harmonized, with various methodologies and systems used across the studies evaluated herein, and it is important to note that results obtained for one measurement system might differ from another [85]. For the time being, therapeutic decisions should not and cannot be based on STM measurements alone but must always be considered in the context of other factors, including a patient’s tolerance to treatment and imaging results. Stepwise diagnostic pathways, incorporating STMs with other clinical and radiologic measures should be the focus of future development, with the ultimate aim of i) improving patient guidance; ii) limiting the harm caused by treatment side effects; and iii) reducing economic burden of lung cancer treatment.
Our review emphasizes that evidence for the clinical application of STMs is not sufficient for high-grade clinical guideline recommendations and further research is needed; however, given the current therapeutic landscape, using STMs for monitoring purposes seems most promising, with the majority of evidence concerning CEA and CYFRA21-1. There is a clinical need for better monitoring of response to immunotherapies on a biochemical level. STMs that correlate with tumor mass or tumor cell activity may be accurate and cost-efficient tools for at least a portion of patients and therefore could be of added value, particularly when combined with other molecular or radiologic exams. Inclusion of such STMs, particularly in prospective immunotherapy studies, is therefore recommended. In clinical practice, there is currently little need for predictive STMs as treatment options for advanced lung cancer are limited and therapeutic decisions are based on histopathologic and molecular tumor characteristics, radiologic staging, and patient performance status. Given their inherent limitations, STMs alone are unlikely to change current predictive practices; however, there may be a place for them in combination with other biomarkers in future predictive models.
In conclusion, we found that baseline and dynamic changes in STM levels may be of added value in the context of prediction of treatment outcome and monitoring of patients with advanced lung cancer during and after treatment with targeted or immunotherapies. However, further research into the utility of STMs in routine clinical care of patients with advanced lung cancer is warranted.
Acknowledgments
The authors would like to thank Birgit Wehnl (Roche Diagnostics GmbH), Daniela Bruni, and Anna-Giorgia Hjelmer (Roche Diagnostics International Ltd) for their contributions to the literature search, conception/design, and critical review of the manuscript. Third-party medical writing assistance, under the direction of the authors, was provided by Chloe Fletcher, MSc and Anna King, PhD, of Ashfield MedComms, an Inizio company, and was funded by Roche Diagnostics International Ltd (Rotkreuz, Switzerland). COBAS and ELECSYS are trademarks of Roche. All other product names and trademarks are the property of their respective owners.
Author contributions
CONCEPTION: All authors
DATA CURATION: DL
ANALYSIS OF DATA: All authors
PREPARATION OF THE MANUSCRIPT: All authors
REVISION FOR IMPORTANT INTELLECTUAL CONTENT: All authors
Conflict of interest
Michel van den Heuvel has received research funding or honoraria from AbbVie, AstraZeneca, Bristol Myers Squibb, Eli Lilly, Janssen Pharmaceuticals, Merck & Co., Inc., Merck Sharp & Dohme, Novartis, PamGene, Pfizer, Roche, and Stichting Treatmeds. He is a guest editor in the special issue “Lung Cancer in Tumor Markers” but had no participation in the peer review process of this paper.
Stefan Holdenrieder has received research funding or honoraria from Bristol Myers Squibb, Merck KGaA, Roche Diagnostics, and Volition SPRL. He is a board member of Tumor Biology but had no participation in the peer review process of this paper.
Milou Schuurbiers and Inga Trulson have no conflicts of interest.
Daniel Cigoianu is an employee of and holds bonds in Roche Diagnostics International Ltd.
Huub van Rossum is the owner and director of Huvaros B.V. and holds stock in SelfSafeSure Blood Collections B.V. He is a board member of Tumor Biology but had no participation in the peer review process of this paper.
David Lang has received speaker’s honoraria and served as an advisor to Boehringer Ingelheim, Bristol Myers Squibb, Eli Lilly, Merck Sharp & Dohme, and Roche, and has received travel/accommodation funding from Boehringer Ingelheim and Roche.
Supplementary material
[1] The supplementary material is available in the electronic version of this article: https://dx.doi.org/10.3233/TUB-220039.
References
[1] | Tunali I , Gillies RJ , Schabath MB . Application of radiomics and artificial intelligence for lung cancer precision medicine. Cold Spring Harb Perspect Med. (2021) ;11: (8):a039537. doi: 10.1101/cshperspect.a039537 |
[2] | Remon J , Lacroix L , Jovelet C , Caramella C , Howarth K , Plagnol V , et al. Real-world utility of an amplicon-based next-generation sequencing liquid biopsy for broad molecular profiling in patients with advanced non–small-cell lung cancer. JCO Precis Oncol. 2019: (3):1–14. doi: 10.1200/po.18.00211 |
[3] | Nasser NJ , Gorenberg M , Agbarya A . First line immunotherapy for non-small cell lung cancer. Pharmaceuticals (Basel). (2020) ;13: (11):373. doi: 10.3390/ph13110373 |
[4] | Shields MD , Marin-Acevedo JA , Pellini B . Immunotherapy for advanced non–small cell lung cancer: A decade of progress. Am Soc Clin Oncol Educ Book. (2021) ;41: :e105–27. doi: 10.1200/edbk_321483 |
[5] | Tan AC , Tan DSW . Targeted therapies for lung cancer patients with oncogenic driver molecular alterations. J Clin Oncol. (2022) ;40: (6):611–25. doi: 10.1200/jco.21.01626 |
[6] | Konig D , Savic S , Rothschild SI Targeted therapy in advanced and metastatic non-small cell lung cancer. An update on treatment of the most important actionable oncogenic driver alterations. Cancers (Basel). (2021) ;13: (4):804. doi: 10.3390/cancers13040804 |
[7] | Ettinger DS , Wood DE , Aisner DL , Akerley W , Bauman JR , Bharat A , et al. Non–small cell lung cancer, version 3.2022, NCCN clinical practice guidelines in oncology. J Natl Compr Canc Netw. (2022) ;20: (5):497–530. doi: 10.6004/jnccn.2022.0025 |
[8] | Zhao XM , Zhao J , Xing KL , Sun S , Luo ZG , Wang HJ , et al. Prognostic and predictive value of serum carcinoembryonic antigen levels in advanced non-small cell lung cancer patients with epidermal growth factor receptor sensitive mutations and receiving tyrosine kinase inhibitors. Oncotarget. (2017) ;8: (41):70865–73. doi: 10.18632/oncotarget.20145 |
[9] | Yanwei Z , Bo J , Yuqing L , Rong L , Xueyan Z , Song H , et al. Serum carcinoembryonic antigen levels predicts the efficacy of EGFR-TKI in non-small cell lung cancer harboring EGFR mutations. J Cancer Res Ther. (2016) ;12: (1):254–8. doi: 10.4103/0973-1482.153666 |
[10] | Lang D , Haslinger W , Akbari K , Scala M , Hergan B , Asel C ,et al. Serum tumor marker dynamics as predictive biomarkers in NSCLC chemo-immunotherapy and mono-immunotherapy maintenance: A registry-based descriptive study. Lung Cancer (Auckl). (2020) ;11: :113–21. doi: 10.2147/lctt.S286228 |
[11] | Takeuchi A , Oguri T , Sone K , Ito K , Kitamura Y , Inoue Y , et al. Predictive and prognostic value of CYFRA 21-1 for advanced non-small cell lung cancer treated with EGFR-TKIs. Anticancer Res. (2017) ;37: (10):5771–6. doi: 10.21873/anticanres.12018 |
[12] | Dal Bello MG , Filiberti RA , Alama A , Orengo AM , Mussap M , Coco S ,et al.The role of CEA, CYFRA21-1 and NSE in monitoring tumor response to nivolumab in advanced non-small cell lung cancer (NSCLC) patients. J Transl Med. (2019) ;17: (1):74. doi: 10.1186/s12967-019-1828-0 |
[13] | Zhang Z , Yuan F , Chen R , Li Y , Ma J , Yan X , et al. Dynamics of serum tumor markers can serve as a prognostic biomarker for Chinese advanced non-small cell lung cancer patients treated with immune checkpoint inhibitors. Front Immunol. (2020) ;11: :1173. doi: 10.3389/fimmu.2020.01173 |
[14] | Postmus PE , Kerr KM , Oudkerk M , Senan S , Waller DA , Vansteenkiste J , et al. Early and locally advanced non-small-cell lung cancer (NSCLC): ESMO clinical practice guidelines for diagnosis, treatment and follow-up. Ann Oncol. (2017) ;28: (Suppl. 4):iv1–21. doi: 10.1093/annonc/mdx222 |
[15] | Dingemans AC , Früh M , Ardizzoni A , Besse B , Faivre-Finn C , Hendriks LE , et al. Small-cell lung cancer: ESMO clinical practice guidelines for diagnosis, treatment and follow-up. Ann Oncol. (2021) ;32: (7):839–53. doi: 10.1016/j.annonc.2021.03.207 |
[16] | Wu YL , Planchard D , Lu S , Sun H , Yamamoto N , Kim DW , et al. Pan-Asian adapted clinical practice guidelines for the management of patients with metastatic non-small-cell lung cancer: A CSCO-ESMO initiative endorsed by JSMO, KSMO, MOS, SSO and TOS. Ann Oncol. (2019) a; 30: (2):171–210. doi: 10.1093/annonc/mdy554 |
[17] | Grunnet M , Sorensen JB . Carcinoembryonic antigen (CEA) as tumor marker in lung cancer. Lung Cancer. (2012) ;76: (2):138–43. doi: 10.1016/j.lungcan.2011.11.012 |
[18] | Daly ME , Singh N , Ismaila N , Antonoff MB , Arenberg DA , Bradley J , et al. Management of stage III non–small-cell lung cancer: ASCO guideline. J Clin Oncol. (2022) ;40: (12):1356–84. doi: 10.1200/jco.21.02528 |
[19] | Ganti AKP , Loo BW , Bassetti M , Blakely C , Chiang A , D’Amico TA , et al. Small cell lung cancer, version 2.2022,NCCN clinical practice guidelines in oncology. J Natl Compr Canc Netw. (2021) ;19: (12):1441–64. doi: 10.6004/jnccn.2021.0058 |
[20] | Singh N , Temin S , Baker S Jr, Blanchard E , Brahmer JR , Celano P , et al. Therapy for stage IV non–small-cell lung cancer with driver alterations: ASCO living guideline. J Clin Oncol. (2022) ;40: (28):3310–22. doi: 10.1200/jco.22.00824 |
[21] | Molina R , Holdenrieder S , Auge JM , Schalhorn A , Hatz R , Stieber P . Diagnostic relevance of circulating biomarkers in patients with lung cancer. Cancer Biomark. (2010) ;6: (3–4):163–78. doi: 10.3233/cbm-2009-0127 |
[22] | FDA-NIH Biomarker Working Group BEST (Biomarkers, endpoints, and other tools) resource. Silver Spring, MD & Bethesda, MD, USA. Food and Drug Administration & National Institutes of Health. (2016) Available from: https://www.ncbi.nlm.nih.gov/books/NBK326791/. |
[23] | Califf RM . Biomarker definitions and their applications. Exp Biol Med (Maywood). (2018) ;243: (3):213–21. doi: 10.1177/1535370217750088 |
[24] | Hammarström S . The carcinoembryonic antigen (CEA) family: Structures, suggested functions and expression in normal and malignant tissues. Semin Cancer Biol. (1999) ;9: (2):67–81. doi: 10.1006/scbi.1998.0119 |
[25] | Screaton RA , Penn LZ , Stanners CP . Carcinoembryonic antigen, a human tumor marker, cooperates with Myc and Bcl-2 in cellular transformation. J Cell Biol. (1997) ;137: (4):939–52. doi: 10.1083/jcb.137.4.939 |
[26] | Arrieta O , Villarreal-Garza C , Martínez-Barrera L , Morales M , Dorantes-Gallareta Y , Peña-Curiel O , et al. Usefulness of serum carcinoembryonic antigen (CEA) in evaluating response to chemotherapy in patients with advanced non small-cell lung cancer: A prospective cohort study. BMC Cancer. (2013) ;13: 254. doi: 10.1186/1471-2407-13-254 |
[27] | Moll R , Franke WW , Schiller DL , Geiger B , Krepler R . The catalog of human cytokeratins: Patterns of expression in normal epithelia, tumors and cultured cells. Cell. (1982) ;31: (1):11–24. doi: 10.1016/0092-8674(82)90400-7 |
[28] | Ono A , Takahashi T , Mori K , Akamatsu H , Shukuya T , Taira T , et al. Prognostic impact of serum CYFRA 21–1 in patients with advanced lung adenocarcinoma: A retrospective study. BMC Cancer. (2013) ;13: (1):354. doi: 10.1186/1471-2407-13-354 |
[29] | Koprowski H , Steplewski Z , Mitchell K , Herlyn M , Herlyn D , Fuhrer P . Colorectal carcinoma antigens detected by hybridoma antibodies. Somatic Cell Genet. (1979) ;5: (6):957–71. doi: 10.1007/bf01542654 |
[30] | Magnani JL , Steplewski Z , Koprowski H , Ginsburg V . Identification of the gastrointestinal and pancreatic cancer-associated antigen detected by monoclonal antibody 19-9 in the sera of patients as a mucin. Cancer Res. (1983) ;43: (11):5489–92. |
[31] | Lamerz R . Role of tumour markers, cytogenetics. Ann Oncol. (1999) ;10: (Suppl. 4):145–9. |
[32] | Bast RC Jr, Feeney M , Lazarus H , Nadler LM , Colvin RB , Knapp RC . Reactivity of a monoclonal antibody with human ovarian carcinoma. J Clin Invest. (1981) ;68: (5):1331–7. doi: 10.1172/jci110380 |
[33] | Bast RC , Xu FJ , Yu YH , Barnhill S , Zhang Z , Mills GB . CA The past and the future. Int J Biologic Markers. (1998) ;13: (4):179–87. doi: 10.1177/172460089801300402 |
[34] | Duffy MJ . CA 15-3 and related mucins as circulating markers in breast cancer. Ann Clin Biochem. (1999) ;36: (5):579–86. doi: 10.1177/000456329903600503 |
[35] | Kato H , Torigoe T . Radioimmunoassay for tumor antigen of human cervical squamous cell carcinoma. Cancer. (1977) ;40: (4):1621–8. doi: 10.1002/1097-0142(197710)40:4<1621::aid-cncr2820400435>3.0.co;2-i |
[36] | Schick C , Kamachi Y , Bartuski AJ , Cataltepe S , Schechter NM , Pemberton PA ,et al. Squamous cell carcinoma antigen 2 is a novel serpin that inhibits the chymotrypsin-like proteinases cathepsin G and mast cell chymase. J Biol Chem. (1997) ;272: (3):1849–55. doi: 10.1074/jbc.272.3.1849 |
[37] | Zhu H . Squamous cell carcinoma antigen: Clinical application and research status. Diagnostics (Basel). (2022) ;12: (5):1065. doi: 10.3390/diagnostics12051065 |
[38] | Isgrò MA , Bottoni P , Scatena R . Neuron-specific enolase as a biomarker: Biochemical and clinical aspects. Adv Exp Med Biol. (2015) ;867: :125–43. doi: 10.1007/978-94-017-7215-0_9 |
[39] | Lu L , Zha Z , Zhang P , Wang P , Liu X , Fang X , et al. Neuron-specific enolase promotes stem cell-like characteristics of small-cell lung cancer by downregulating NBL1 and activating the BMP2/Smad/ID1 pathway. Oncogenesis. (2022) ;11: (1):21. doi: 10.1038/s41389-022-00396-5 |
[40] | Uchida K , Kojima A , Morokawa N , Tanabe O , Anzai C , Kawakami M , et al Expression of progastrin-releasing peptide and gastrin-releasing peptide receptor mRNA transcripts in tumor cells of patients with small cell lung cancer. J Cancer Res Clin Oncol. (2002) ;128: (12):633–40. doi: 10.1007/s00432-002-0392-8 |
[41] | Miyake Y , Kodama T , Yamaguchi K . Pro-gastrin-releasing peptide(31-98) is a specific tumor marker in patients with small cell lung carcinoma. Cancer Res. (1994) ;54: (8):2136–40. |
[42] | Hellström I , Raycraft J , Hayden-Ledbetter M , Ledbetter JA , Schummer M , McIntosh M , et al. The HE4 (WFDC2) protein is a biomarker for ovarian carcinoma. Cancer Res. (2003) ;63: (13):3695–700. |
[43] | Kim KK , Turner R , Khazan N , Kodza A , Jones A , Singh RK , et al. Role of trypsin and protease-activated receptor-2 in ovarian cancer. PLoS One. (2020) ;15: (5):e0232253. doi: 10.1371/journal.pone.0232253 |
[44] | Sun ML , Yang ZY , Wu QJ , Li YZ , Li XY , Liu FH , et al. The role of human epididymis protein 4 in the diagnosis and prognosis of diseases: An umbrella review of systematic reviews and meta-analyses of observational studies. Front Med (Lausanne). (2022) ;9: :842002. doi: 10.3389/fmed.2022.842002 |
[45] | Mattioni M , Soddu S , Prodosmo A , Visca P , Conti S , Alessandrini G , et al. Prognostic role of serum p53 antibodies in lung cancer. BMC Cancer. (2015) ;15: :148. doi: 10.1186/s12885-015-1174-4 |
[46] | Laudanski J , Burzykowski T , Niklinska W , Chyczewski L , Furman M , Niklinski J . Prognostic value of serum p53 antibodies in patients with resected non-small cell lung cancer. Lung Cancer. (1998) ;22: (3):191–200. doi: 10.1016/S0169-5002(98)00088-9 |
[47] | Shirasu H , Ono A , Omae K , Nakashima K , Omori S , Wakuda K , et al. CYFRA 21-1 predicts the efficacy of nivolumab in patients with advanced lung adenocarcinoma. Tumour Biol. (2018) ;40: (2):1010428318760420. doi: 10.1177/1010428318760420 |
[48] | Dall’Olio FG , Abbati F , Facchinetti F , Massucci M , Melotti B , Squadrilli A , et al. CEA and CYFRA 21-1 as prognostic biomarker and as a tool for treatment monitoring in advanced NSCLC treated with immune checkpoint inhibitors. Ther Adv Med Oncol. (2020) ;12: :1758835920952994. doi: 10.1177/1758835920952994 |
[49] | Kataoka Y , Hirano K , Narabayashi T , Hara S , Fujimoto D , Tanaka T ,et al. Carcinoembryonic antigen as a predictive biomarker of response to nivolumab in non–small cell lung cancer. Anticancer Res. (2018) ;38: (1):559–63. doi: 10.21873/anticanres.12259 |
[50] | Huang L , Li L , Zhou Y , Yang Z , Wang M , Gao Y , et al. Clinical characteristics correlate with outcomes of immunotherapy in advanced non-small cell lung cancer. J Cancer. (2020) ;11: (24):7137–45. doi: 10.7150/jca.49213 |
[51] | Lang D , Horner A , Brehm E , Akbari K , Hergan B , Langer K ,et al. Early serum tumor marker dynamics predict progression-free and overall survival in single PD-1/PD-L1 inhibitor treated advanced NSCLC - a retrospective cohort study. Lung Cancer. (2019) ;134: 59–65. doi: 10.1016/j.lungcan.2019.05.033 |
[52] | Chai R , Fan Y , Zhao J , He F , Li J , Han Y . Prognostic nomogram on clinicopathologic features and serum indicators for advanced non-small cell lung cancer patients treated with anti-PD-1 inhibitors. Ann Transl Med. (2020) ;8: (17):1078. doi: 10.21037/atm-20-4297 |
[53] | Li L , Zhang Z , Hu Y . Neuron - specific enolase predicts the prognosis in advanced small cell lung cancer patients treated with first-line PD-1/PD-L1 inhibitors. Medicine (Baltimore). (2021) ;100: (36):e27029. doi: 10.1097/md.0000000000027029 |
[54] | Tanaka K , Hata A , Kaji R , Fujita S , Otoshi T , Fujimoto D , et al. Cytokeratin 19 fragment predicts the efficacy of epidermal growth factor receptor-tyrosine kinase inhibitor in non-small-cell lung cancer harboring EGFR mutation. J Thorac Oncol. (2013) ;8: (7):892–8. doi: 10.1097/JTO.0b013e31828c3929 |
[55] | Fiala O , Pesek M , Finek J , Benesova L , Minarik M , Bortlicek Z , et al. Predictive role of CEA and CYFRA 21-1 in patients with advanced-stage NSCLC treated with erlotinib. Anticancer Res. (2014) a;34: (6):3205–10. |
[56] | Chen Y-M , Lai C-H , Chang H-C , Chao T-Y , Tseng C-C , Fang W-F , et al. Baseline, trend, and normalization of carcinoembryonic antigen as prognostic factors in epidermal growth factor receptor-mutant nonsmall cell lung cancer patients treated with first-line epidermal growthfactor receptor tyrosine kinase inhibitors. Medicine. (2015) ;94: (50):e2239. doi: 10.1097/MD.0000000000002239 |
[57] | Wu Z , Dai Y , Chen LA . The prediction of epidermal growth factor receptor mutation and prognosis of EGFR tyrosine kinase inhibitor by serum ferritin in advanced NSCLC. Cancer Manag Res. (2019) b;11: :8835–43. doi: 10.2147/cmar.S216037 |
[58] | Wei H , Su M , Lin R , Li H , Zou C . Prognostic factors analysis in EGFR mutation-positive non-small cell lung cancer with brain metastases treated with whole brain-radiotherapy and EGFR-tyrosine kinase inhibitors. Oncol Lett. (2016) ;11: (3):2249–54. doi: 10.3892/ol.2016.4163 |
[59] | Romero-Ventosa EY , Blanco-Prieto S , González-Piñeiro AL , Rodríguez-Berrocal FJ , Piñeiro-Corrales G , Páez de la Cadena M . Pretreatment levels of the serum biomarkers CEA, CYFRA21–1, SCC and the soluble EGFR and its ligands EGF,TGF-alpha, HB-EGF in the prediction of outcome in erlotinib treatednon-small-cell lung cancer patients. SpringerPlus. (2015) ;4: :171. doi: 10.1186/s40064-015-0891-0 |
[60] | Facchinetti F , Aldigeri R , Aloe R , Bortesi B , Ardizzoni A , Tiseo M . CEA serum level as early predictive marker of outcome during EGFR-TKI therapy in advanced NSCLC patients. Tumour Biol. (2015) ;36: (8):5943–51. doi: 10.1007/s13277-015-3269-6 |
[61] | Ding PN , Becker TM , Bray VJ , Chua W , Ma YF , Lynch D , et al. The predictive and prognostic significance of liquid biopsy in advanced epidermal growth factor receptor-mutated non-small cell lung cancer: A prospective study. Lung Cancer. (2019) ;134: :187–93. doi: 10.1016/j.lungcan.2019.06.021 |
[62] | Han J , Li Y , Cao S , Dong Q , Zhao G , Zhang X , et al. The level of serum carcinoembryonic antigen is a surrogate marker for the efficacy of EGFR-TKIs but is not an indication of acquired resistance to EGFR-TKIs in NSCLC patients with EGFR mutationsm. Biomed Rep. (2017) ;7: (1):61–6. doi: 10.3892/br.2017.914 |
[63] | Pan JB , Hou YH , Zhang GJ . Correlation between efficacy of the EGFR tyrosine kinase inhibitor and serum tumor markers in lung adenocarcinoma patients. Clin Lab. (2014) ;60: (9):1439–47. doi: 10.7754/clin.lab.2013.131002 |
[64] | Ramalingam SS , Shtivelband M , Soo RA , Barrios CH , Makhson A , Segalla JG , et al. Randomized phase II study of carboplatin and paclitaxel with either linifanib or placebo for advanced nonsquamous non-small-cell lung cancer. J Clin Oncol. (2015) ;33: (5):433–41. doi: 10.1200/jco.2014.55.7173 |
[65] | Fiala O , Pesek M , Finek J , Benesova L , Minarik M , Bortlicek Z , et al. The role of neuron-specific enolase (NSE) and thymidine kinase (TK) levels in prediction of efficacy of EGFR-TKIs in patients with advanced-stage NSCLC [corrected]. Anticancer Res. (2014) b;34: (9):5193–8. |
[66] | Suh KJ , Keam B , Kim M , Park YS , Kim TM , Jeon YK , et al. Serum neuron-specific enolase levels predict the efficacy of first-line epidermal growth factor receptor (EGFR) tyrosine kinase inhibitors in patients with non-small cell lung cancer harboring EGFR mutations. Clin Lung Cancer. (2016) ;17: (4):245–52e1. doi: 10.1016/j.cllc.2015.11.012 |
[67] | Inomata M , Hayashi R , Yamamoto A , Tokui K , Taka C , Okazawa S , et al. Plasma neuron-specific enolase level as a prognostic marker in patients with non-small cell lung cancer receiving gefitinib. Mol Clin Oncol. (2015) ;3: (4):802–6. doi: 10.3892/mco.2015.568 |
[68] | Wen S , Du X , Chen Y , Xia J , Wang R , Zhu M , et al. Association between changes in thioredoxin reductase and other peripheral blood biomarkers with response to PD-1 inhibitor-based combination immunotherapy in non-small cell lung cancer: A retrospective study. Transl Lung Cancer Res. (2022) ;11: (5):757–75. doi: 10.21037/tlcr-22-300 |
[69] | Zhuo M , Chen H , Zhang T , Yang X , Zhong J , Wang Y , et al. The potential predictive value of circulating immune cell ratio and tumor marker in atezolizumab treated advanced non-small cell lung cancer patients. Cancer Biomark. (2018) ;22: (3):467–76. doi: 10.3233/cbm-171089 |
[70] | Clevers MR , Kastelijn EA , Peters BJM , Kelder H , Schramel FMNH . Evaluation of serum biomarker CEA and Ca-125 as immunotherapy response predictors in metastatic non-small cell lung cancer. Anticancer Res. (2021) ;41: (2):869–76. doi: 10.21873/anticanres.14839 |
[71] | Moritz R , Muller M , Korse CM , van den Broek D , Baas P , van den Noort V , et al. Diagnostic validation and interpretation of longitudinal circulating biomarkers using a biomarker response characteristic plot. Clin Chim Acta. (2018) ;487: :6–14. doi: 10.1016/j.cca.2018.09.015 |
[72] | Muller M , Hoogendoorn R , Moritz RJG , van der Noort V , Lanfermeijer M , Korse CM , et al. Validation of a clinical blood-based decision aid to guide immunotherapy treatment in patients with non-small cell lung cancer. Tumour Biol. (2021) ;43: (1):115–27. doi: 10.3233/tub-211504 |
[73] | Chen Y , Wen S , Xia J , Du X , Wu Y , Pan B ,et al. Association of dynamic changes in peripheral blood indexes with response to PD-1 inhibitor-based combination therapy and survival among patients with advanced non-small cell lung cancer. Front Immunol. (2021) ;12: :672271. doi: 10.3389/fimmu.2021.672271 |
[74] | Arrieta O , Varela-Santoyo E , Cardona AF , Sánchez-Reyes R , Lara-Mejía L , Bassarmal SS ,et al. Association ofcarcinoembryonic antigen reduction with progression-free and overallsurvival improvement in advanced non-small-cell lung cancer. ClinLung Cancer. (2021) ;22: (6):510–22. doi: 10.1016/j.cllc.2021.03.014 |
[75] | de Kock R , van den Borne B , Youssef–El Soud M , Belderbos H , Stege G , de Saegher M , et al. Circulating biomarkers for monitoring therapy response and detection of disease progression in lung cancer patients. Cancer Treat Res Commun. (2021) ;28: :100410. doi: 10.1016/j.ctarc.2021.100410 |
[76] | Noonan SA , Patil T , Gao D , King GG , Thibault JR , Lu X , et al. Baseline and on-treatment characteristics of serum tumor markers in stage IV oncogene-addicted adenocarcinoma of the lung. J Thorac Oncol. (2018) ;13: (1):134–8. doi: 10.1016/j.jtho.2017.08.005 |
[77] | Mang A , Zou W , Rolny V , Reck M , Cigoianu D , Schulze K , et al. P22.02 combined use of CYFRA 21-1, CA125 and CRP predicts survival of metastatic NSCLC patients with stable disease.. J Thorac Oncol. (2021) ;16: (10 Suppl.):S1024–5. doi: 10.1016/j.jtho.2021.08.356 |
[78] | van Delft F , Schuurbiers M , Muller M , Burgers S , van Rossum H , Ijzerman M , et al. 194P Modelling strategies to combine multiple serum tumor biomarkers for early prediction of immunotherapy non-response in non-small cell lung cancer. Ann Oncol. (2022) ;33: (Suppl. 2):S119. doi: 10.1016/j.heliyon.2022.e10932 |
[79] | Wang J , Tang Y , Sun Y , Yin B , Guan Y , Feng D . A novel combination score based on early serum tumor markers and neutrophil-to-lymphocyte ratio dynamics predicts response to PD-1/PD-L1 inhibitors in advanced non-small cell lung cancer. J Clin Oncol. (2021) ;39: : (15 Suppl):e21095. |
[80] | Schuurbiers M , van Delft F , Koffijberg H , Burgers S , Ijzerman M , Van Rossum H , et al. P Early prediction of non-response to immunotherapy by a combined serum tumor marker model in non-small cell lung cancer patients. Ann Oncol. (2022) ;33: :S1036. doi: 10.1016/j.annonc.2022.07.1179 |
[81] | Muley T , Schneider M , Meister M , Thomas M , Heussel C , Kriegsmann M , et al. P23.04 Serum biomarkers enhance prognostic value of computed tomography (CT) in patients with non-small cell lung cancer (NSCLC). J Thorac Oncol. (2021) ;16: (10 Suppl.):S1028–9. doi: 10.1016/j.jtho.2021.08.364 |
[82] | Brody R , Zhang Y , Ballas M , Siddiqui MK , Gupta P , Barker C , et al. PD-L1 expression in advanced NSCLC: Insights into risk stratification and treatment selection from a systematic literature review. Lung Cancer. (2017) ;112: :200–15. doi: 10.1016/j.lungcan.2017.08.005 |
[83] | Reck M , Schenker M , Lee KH , Provencio M , Nishio M , Lesniewski-Kmak K , et al. Nivolumab plus ipilimumab versus chemotherapy as first-line treatment in advanced non-small-cell lung cancer with high tumour mutational burden: Patient-reported outcomes results from the randomised, open-label, phase III CheckMate 227 trial. Eur J Cancer. (2019) ;116: :137–47. doi: 10.1016/j.ejca.2019.05.008 |
[84] | Jiang W , Cai G , Hu PC , Wang Y . Personalized medicine in non-small cell lung cancer: A review from a pharmacogenomics perspective. Acta Pharm Sin B. (2018) ;8: (4):530–8. doi: 10.1016/j.apsb.2018.04.005 |
[85] | Sturgeon C . Standardization of tumor markers - priorities identified through external quality assessment. Scand J Clin Lab Invest Suppl. (2016) ;245: :S94–910.1080/00365513.2016.1210334 |