Vitamin B12 enhances the antitumor activity of 1,25-dihydroxyvitamin D3 via activation of caspases and targeting actin cytoskeleton
Abstract
BACKGROUND:
1,25-dihydroxyvitamin D3 (1,25(OH)2D3) is an effective anticancer agent, and when combined with other agents it shows superior activities. Vitamin B12 has been shown to contribute to increasing the effectiveness of anticancer drugs when used in combination. Thus, the current study aimed at investigating the anticancer potential of the combination of 1,25(OH)2D3 and vitamin B12.
METHODS:
MTT assay was used to determine the cytotoxic activity of combining 1,25(OH)2D3 and vitamin B12 against six different cancer cell lines and one normal cell line. The surviving fraction after clonogenic assay was measured, and the effects of 1,25(OH)2D3/B12 combination on the activity of different caspases, cell adhesion, actin cytoskeleton, cell morphology, and percentage of polarized cells were evaluated.
RESULTS:
Vitamin B12 did not cause cytotoxicity, however, it enhanced the cytotoxicity of 1,25(OH)2D3 against cancer cells. The cytotoxic effects of 1,25(OH)2D3 and its combination with vitamin B12 was not evident in the normal mammary MCF10A cell line indicating cancer cell-specificity. The cytotoxic effects of 1,25(OH)2D3/B12 combination occurred in a dose-dependent manner and was attributed to apoptosis induction which was mediated by caspase 4 and 8. Moreover, 1,25(OH)2D3/B12-treated cells showed enhanced inhibition of clonogenic tumor growth, reduced cell adhesion, reduced cell area, reduced percentage of cell polarization, and disorganized actin cytoskeleton resulting in reduced migratory phenotype when compared to cells treated with 1,25(OH)2D3 alone.
CONCLUSION:
1,25(OH)2D3 and vitamin B12 exhibited synergistic anticancer effects against different cancer cell lines. The combination therapy of 1,25(OH)2D3 and vitamin B12 may provide a potential adjunctive treatment option for some cancer types.
1Introduction
Worldwide, cancer is the second leading reason of death next to cardiovascular diseases. The three most common cancers are breast, lung, and colon cancers with lung cancer being the primary cause of cancer-related deaths [1, 2]. Common cancer treatments include surgery, chemotherapy, and radiation. For certain cancer types and cancer patients, the best approach is combining more than one treatment option or combining more than one therapeutic drug. Combined drug therapy enhances treatment effectiveness either in a synergistic or in an additive fashion [3].
Vitamin D is a set of fat-soluble secosteroids that regulate calcium-phosphate metabolism and homeostasis. The two forms of vitamin D are cholecalciferol (vitamin D3) and ergocalciferol (vitamin D2). Vitamin D3 is biologically inactive requiring a complex series of enzymatic reactions, either through the classical pathway in which CYP2R1 is the most important enzyme, [4–8] or the non-classical pathway mediated by CYP11A1, [9–12] to be converted to its active form 1α,25-dihydroxyvitamin D3 (1,25(OH)2D3). The activity of 1,25(OH)2D3 depends on its interaction with vitamin D receptor (VDR), inducing gene expression involved in cell proliferation and differentiation [13, 14]. 1,25(OH)2D3 and VDR have important roles in apoptosis, angiogenesis, and immune-modulatory properties by genomic and non-genomic pathways. Interestingly, vitamin D function and metabolism are dysregulated in many cancer types, reversing vitamin D antitumorigenic effect or causing resistance to it. Thus, understanding vitamin D dysregulation in cancer may be critical for development of new strategies intended for successful vitamin D-based cancer therapy [15].
Vitamin D concentrations in blood and tissues are highly regulated, and vitamin D deficiency has major consequences as it contributes to increased risk of diseases such as obesity, diabetes, cardiovascular disease, inflammatory diseases, cancers, and some neurological diseases. The link between vitamin D and cancer prevention through its anti-proliferative and pro-differentiation effects were reported [15]. The anti-cancer activities of vitamin D are mediated through apoptosis induction, differentiation stimulation, angiogenesis inhibition, and metastasis inhibition [15–17].
When U937 leukemia cells were treated with 1,25(OH)2D3, a rapid rise in cell proliferation was observed followed by growth inhibition and differentiation and induction of apoptosis [18]. In contrast, 1,25(OH)2D3-treated MCF-7 breast cancer cells directly entered into cell cycle arrest in G1 [19]. Thereby, cell death-enhancing effect of 1,25(OH)2D3 varies with cell type. 1,25(OH)2D3 induced apoptosis by the activation of cell cycle genes (p21, p27, and RB) and pro-apoptotic genes (BAK and BAX) and the inhibition of cyclin-dependent kinases (G1-cyclin/CDK complex) causing the G1/S transition blockage [20].
1,25(OH)2D3 has been reported to be a promising chemotherapeutic agent against breast cancer. Treating estrogen receptor (ER) positive breast cancer cells with 1,25(OH)2D3 resulted in reducing mRNA expression of ER, downregulating the pro-survival BCL2 protein, and upregulating cell cycle regulation genes and apoptotic genes [21, 22]. 1,25(OH)2D3-treated MCF-7 breast cancer cells (ER+) were stuck at the G0-G1 transition phase, which lead to mitochondrial stress, release of cytochrome c and accumulation of pro-apoptotic proteins [22]. On the other hand, Ozkaya and colleagues, 2017, found that 1,25(OH)2D3 exerted its cellular actions on treated MCF-7 breast cancer cell line via induction of the endoplasmic reticulum (ER)-stress-like response; [23] however, ER stress by itself was found to be insufficient to cause 1,25(OH)2D3-mediated cytotoxicity [24]. Remarkably, it was reported that the anti-tumor effects of 1,25(OH)2D3 are enhanced significantly when combined with other treatments [25–27].
Vitamin B12 (cobalamin) is an essential cofactor in different methylation processes such as DNA synthesis and mitochondrial metabolism which are crucial in maintaining neuronal health and hematopoiesis. When vitamin B12 enters the cell, it is transformed into two active coenzymes, methylcobalamin which maintains the homocysteine homeostasis, and adenosylcobalamin which maintains the methylmalonic acid homeostasis [28]. Vitamin B12 reduces superoxide levels in the cytosol and mitochondria and stimulates reactive oxygen species (ROS) scavenging by glutathione preservation, [29] its deficiency mediates ROS accumulation [30]. There is an association between antioxidant vitamins (such as vitamin B12) and prevention of some types of cancer such as pancreatic cancer [31]. Methylated B vitamins such as vitamin B12 are required for DNA stability, integrity and synthesis; [32] consequently, any defect will affect DNA stability and cell metabolism negatively. Vitamin B12 deficiency and elevated plasma homocysteine are positively correlated with increased micronucleus formation and carcinogenesis.
Studies have reported that deficiency of B vitamins, including B12, induces genetic modification by adding uracil base instead of thymine resulting in chromosomal breaks, accordingly, high intake of vitamin B12 reduces the risk of TT-genotype colon cancer mutation [33]. Most studies conducted using vitamin B12 have proven that when it is used in combined therapy it contributes to increasing the effectiveness of anticancer drugs. As a new promising therapeutic application, vitamin B12-conjugates such as vitamin B12-labeled poly(D,L-lactide-co-glycolide) and polyethylene glycol nanoparticles (PLGA-PEG-VB12 NPs), have been used with microRNA system in the form of miR-532-3p-loaded PLGA-PEG nanoparticles as an anti-proliferative agent for gastric cancer via activating the ARC/Bax/mitochondria-mediated apoptosis signaling pathway [34]. Additionally, it was shown that vitamin B12 contributes to a distinctive mechanism of cell death known as paraptosis-like cell death. The combination of vitamin B12 with diethyldithiocarbamate stimulated disulfiram oxiderivatives formation resulting in severe ER stress and extensive ER vacuolization leading to paraptosis-like cell death [35]. Moreover, a study done on HL-60 human lymphocytic leukemia cell line showed that the use of vitamin B12 in combination with thiol antioxidants such as dithiothreitol (DTT), glutathione, and N-acetylcysteine resulted in lysosome dysfunction followed by apoptosis [36].
Since the therapeutic role of vitamin B12 in cancer cells is to increase the effectiveness of other antitumor agents, the current study aimed at investigating the anticancer potential of the combination of 1,25(OH)2D3 and B12 on different cancer cell lines.
2Materials and methods
2.1Cell culture
Human BT549, MDA-MB-453, and SUM159PT triple negative breast cancer (TNBC) cells were cultured in high glucose Dulbecco’s Modified Eagle’s Medium (HGDMEM; Gibco, USA) supplemented with 10% fetal bovine serum (FBS; VWR, USA), human HeLa cervical cancer cells were cultured in LGDMEM (Gibco, USA) supplemented with 10% FBS, human HL-60 promyelocytic leukemia cells were cultivated in in RPMI 1640 medium (Gibco, USA) supplemented with 20% FBS, human MCF-7 breast cancer cells were cultured in RPMI-1640 medium supplemented with 10% FBS, and the mammary non-tumorigenic MCF10A cells were grown in DMEM/F12 (Gibco, USA) supplemented with 5% horse serum (Invitrogen, USA), EGF (20 ng/mL, Sigma), hydrocortisone (0.5 mg/mL, Sigma), cholera toxin (100 ng/mL, Sigma), and insulin (10 mg/mL, Sigma). For subculture, trypsin-EDTA (HyClone, USA) was used. Cell growth was carried out in a 5% carbon dioxide and 95% air atmosphere at 37 C°. HeLa, HL-60, MCF-7, and MCF10A cell lines were purchased from ATCC. MDA-MB-453, BT549 and SUM159PT cell lines were purchased from University of Colorado Cancer Center (UCCC) Tissue Culture Core.
2.2In vitro cytotoxicity (3-(4,5-dimethylthiazol-2-yl)–2,5-diphenyltetrazolium bromide) MTT test
Cytotoxicity of 1α,25-dihydroxyvitamin D3 (1,25(OH)2D3; Sigma-Aldrich, USA), vitamin B12 (Sigma-Aldrich, USA) and the combination (1,25(OH)2D3/B12) on the different cancer cell lines was evaluated by means of MTT test [37, 38]. Vitamin stock solutions were prepared in 10% DMSO with final DMSO concentration not exceeding 0.1%. For each compound, six concentrations (0.01, 0.1, 1, 10, 100, and 1000 nM) were prepared in growth media. The absorbance of each well at 570 nm was measured and then used to determine cell growth inhibition induced by the vitamins (Biotek Synergy HT Multi-Mode Microplate Reader, VT, USA). Growth percent was calculated according to: Growth (%) = OD treated/OD vehicle-treated control×100%. The concentration percent growth curve was used to calculate 50% growth inhibition (IC50) by linear interpolation from a semi-log plot of a dose response curve. Each experiment was repeated in triplicates.
2.3Detection of apoptosis
Apoptosis induction was detected with DAPI using a Nikon Eclipse Ti-E microscope visualizing nuclei after staining and confirmed by phosphatidylserine translocation to the cell surface using annexin V-FITC apoptosis detection kit (Invitrogen, USA).
2.4Clonogenic assay
Cells were subcultured and 2×105 were seeded and incubated in a humidified 5% CO2 incubator at 37°C for 24 h. Afterwards, cells were incubated for 3 h in the presence of increasing concentrations (0.01, 0.1, 1, 10, 100, and 1000 nM) of 1,25(OH)2D3 or B12 or the combination. One thousand HeLa, MCF-7, and SUM159PT cells were seeded on soft agar, while HL-60 cells were seeded on methylcellulose, [39, 40] then incubated for 12 days. Colonies were then stained with crystal violet (0.01%) and counted [40]. Surviving fraction (SF) was calculated as plating efficiency (PE) of treated sample/ PE of vehicle-treated control×100, where PE is the number of colonies counted/ number of cells seeded×100 [39]. The experiment was repeated in duplicates three times.
2.5Cytochrome c release apoptosis assay
Control and IC50-treated cells (5×107) were centrifuged at 2,900 RPM for 5 min and the cell pellet was washed with ice cold PBS and re-centrifuged at 2,900 RPM for 5 min, then re-suspended with cytosolic extraction buffer containing protease inhibitors and dithiothreitol (DTT). Cells were then incubated for 10 min on ice, homogenized and re-centrifuged for 10 min at 3,100 RPM. The cytosolic fraction (CF) was collected from the supernatant by centrifugation at 12,000 RMP for 30 min, while the mitochondrial fraction (MF) was collected from the pellet by suspending it in mitochondrial extraction buffer (Cytochrome c Release Apoptosis Assay Kit, Abcam, USA).
2.6Measurement of caspase activity
The activity of the different caspases (3/7, 4, 8 and 9) was measured using the Caspase-Glo assay according to the manufacturer instructions (Promega, USA).
2.7Western blot analysis
Proteins were resolved by electrophoresis from both control and IC50-treated cells (10% –12.5%) SDS-polyacrylamide gels. Proteins were transferred onto nitrocellulose membranes, then blocked with 2% (w/v) bovine serum albumin (BSA) in tris-buffered saline (TBS) at 4°C. The nitrocellulose membranes were exposed to the primary antibodies: mouse monoclonal anti-caspase 3 (1:500, Invitrogen, USA), rabbit polyclonal anti-caspase 4 (1:1000; Invitrogen, USA), mouse monoclonal anti-caspase 8 (1:1000; Invitrogen, USA), mouse monoclonal anti-caspase 9 (1:1000; Invitrogen, USA), rabbit polyclonal anti-smac/DIABLO (1:1000; R&D Systems, Germany), mouse monoclonal anti-GAPDH (1:6000; CHEMICON, USA), mouse monoclonal anti-tubulin (1:500, Sigma, USA) diluted in 1% BSA in a solution of TBS and 0.05% Tween 20. This was followed by incubation with secondary antibodies conjugated to 680 nm or 800 nm fluorescent dyes (IRDye 680 or IRDye800). Membranes were then washed and visualized on FluorChem R system (Oxford, UK). Quantification of the signals were carried out by AlphaView software (ProteinSimple, USA).
2.8Adhesion assay
Control and IC50-treated cells were suspended in serum-free media with 0.35% BSA and replated (5×104 cells/well) onto collagen I (10μg/ml)-precoated 96-well tissue culture dishes. After incubation at 37°C for 1 h, cells were washed with PBS two times, and adherent cells were fixed for 30 min by paraformaldehyde and stained with 1% methylene blue and 1% borax. Absorbance (at 630 nm) was measured after solubilization with 1% SDS [41].
2.9Cell staining and microscopy
On sterile glass cover slips, cells were plated for 24 h. Later, cells were treated with 1,25(OH)2D3, vitamin B12, and the combination of 1,25(OH)2D3/vitamin B12 at the IC50 concentration. Cells were fixed with 4% paraformaldehyde (Sigma-Aldrich, USA) in PBS for 45 min. Cells were washed with 0.1% Triton X-100 in PBS for three times (three min each). Fluorescent- conjugated phalloidin (Invitrogen) was used to stain F-actin for 1 h. Prolong Gold Antifade containing DAPI (Invitrogen) was used to mount the cells. Images were captured with a CCD camera and 60×NA 0.85 objective on Nikon Eclipse Ti-E microscope run by NIS Elements software.
2.10Statistical analysis
Results are expressed as mean±SD. GraphPad Prism version 7.0 (GraphPad Software, San Diego, CA, USA) was used to carry out statistical analysis. One-way ANOVA with Fisher’s LSD was used to determine the differences between 3 or more means, with p value < 0.05 as a significant value.
3Results
3.1Vitamin B12 enhances the cytotoxicity of 1,25(OH)2D3 against different types of cancer cells
The cytotoxic effects of 1,25(OH)2D3, vitamin B12 and the combination of 1,25(OH)2D3 and vitamin B12 (1,25(OH)2D3/B12) were evaluated against 6 different human cancer cell lines (BT549, HeLa, HL-60, MCF-7, MDA-MB-453, and SUM159PT) and one normal cell line (MCF10A) by means of MTT assay. The IC50 values are shown in Table 1. The results reveal that 1,25(OH)2D3 caused cytotoxicity in 4 out of the 6 cancer cell lines tested (Table 1), while vitamin B12 did not cause cytotoxicity in cancer cells even at the 1000 nM concentration after 72 h of treatment (Table 1). However, vitamin B12 enhanced the cytotoxic effects of 1,25(OH)2D3 significantly in HeLa, HL-60 and MCF-7 cancer cells but not in SUM159PT cells (Table 1). On the other hand, none of the vitamins used (1,25(OH)2D3 and B12) or their combination caused cytotoxicity in MCF10A normal cells even after 72 h of treatment with the highest concentration used (1000 nM) indicating cancer-cell specificity (Table 1), The amounts of 1,25(OH)2D3 and 1,25(OH)2D3/B12 combination used in subsequent experiments were the IC50 values from this table, unless stated then. In addition, and as shown in Fig. 1A-D, the cytotoxic effects of 1,25(OH)2D3 and 1,25(OH)2D3/B12 combination (but not vitamin B12) occurred in a dose-dependent mode, i.e., increasing the concentration of 1,25(OH)2D3 as well as 1,25(OH)2D3/B12 combination caused an increase in the percentage of non-viable cells.
Table 1
IC50 values of 1,25(OH)2D3,vitamin B12 and the combination(1,25(OH)2D3/B12) in different cell lines asdetermined bythe MTT assay
Cell line | 1,25(OH)2D3 | Vitamin B12 | 1,25(OH)2D3/B12 |
BT549 | No effectc | No effectc | No effectc |
HeLa | 653.6 nMa | No effectc | 473.7 nMaφφ |
HL-60 | 444.0 nMc | No effectc | 367.4 nMcφ |
MCF-7 | 453.5 nMa | No effectb | 301.4 nMaφφ |
MDA-MB-453 | No effectc | No effectc | No effectc |
SUM159PT | 1006.8 nMb | No effectb | 972.0 nMb |
MCF10A | No effectc | No effectc | No effectc |
a: after 24 h; b: after 48 h; c: after 72 h of vitamin(s) addition. φp < 0.05, φφp < 0.01 versus 1,25(OH)2D3 alone.
Fig. 1
Vitamin B12 enhances the dose-dependent cytotoxicity of 1,25(OH)2D3. Cells were treated with different concentrations (0.01, 0.1, 1, 10, 100, 1000 nM) of 1,25(OH)2D3, vitamin B12 and combination of both (1,25(OH)2D3/B12) for different time periods as shown in Table 1. The percentage of non-viable cells relative to control cells was measured by MTT assay for A) HeLa cells; B) HL-60 cells; C) MCF-7 cells; D) SUM159PT cells. Bars = mean±SD. Experiments was repeated in triplicates. *P < 0.05; **P < 0.01; ***P < 0.001 compared to DMSO-treated control cells, φP < 0.05; φφ P < 0.01 compared to 1,25(OH)2D3-treated cells.
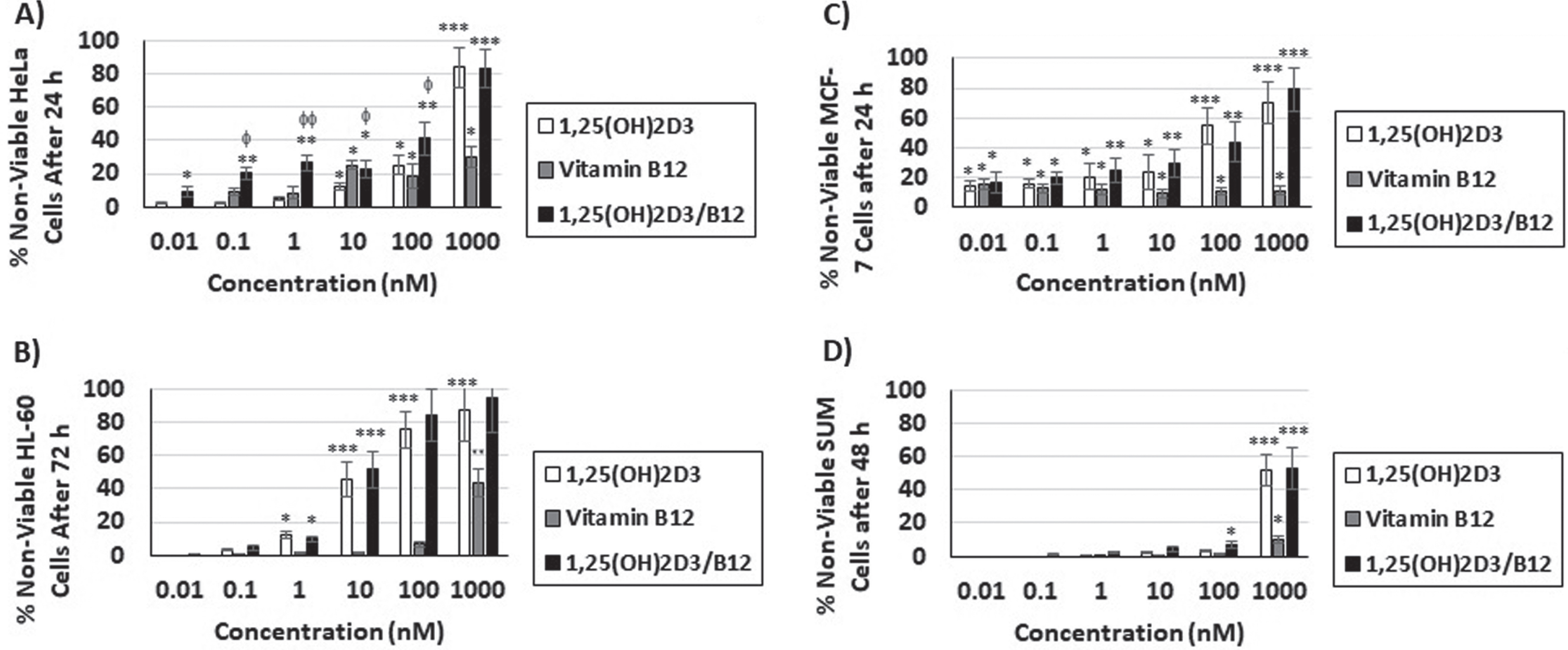
3.2Cytotoxicity of 1,25(OH)2D3 and the combination of 1,25(OH)2D3 and B12 is attributed to induction of apoptosis
To investigate the type of cell death (apoptosis versus necrosis) caused by 1,25(OH)2D3 and the combination (1,25(OH)2D3/B12), cells were treated at the IC50 concentration from Table 1 and the cells were stained with DAPI or subjected to flow cytometry. The results presented in Fig. 2 show that 1,25(OH)2D3 and the combination caused cytotoxicity in cancer cells by apoptosis induction that was detected by visualizing fragmented nuclei in a fluorescence microscope (Fig. 2A) and analyzed by annexin V-FITC and PI flow cytometry analysis (Fig. 2B).
Fig. 2
1,25(OH)2D3 and the combination of 1,25(OH)2D3 and B12 cause cytotoxicity by induction of apoptosis. Cells were treated at the IC50 concentration for 1,25(OH)2D3 and 1,25(OH)2D3/B12 from Table 1, and at 1000 nM for vitamin B12. A) Fluorescence images of treated HeLa, HL-60, MCF-7, and SUM159PT cancer cells showing apoptotic nuclei (fragmented nuclei) after DAPI staining. The image of 1,25(OH)2D3/B12-treated HL-60 nuclei is a composite image since very few cells remained attached to the cove slip. Scale bar: 10μm; B) Flow cytometry analysis for both control and treated HL-60 stained for annexin V-FITC and PI. Live cells (PI negative, Annexin V negative), apoptotic cells (PI negative, Annexin V positive) and necrotic cells (PI positive, Annexin V positive).
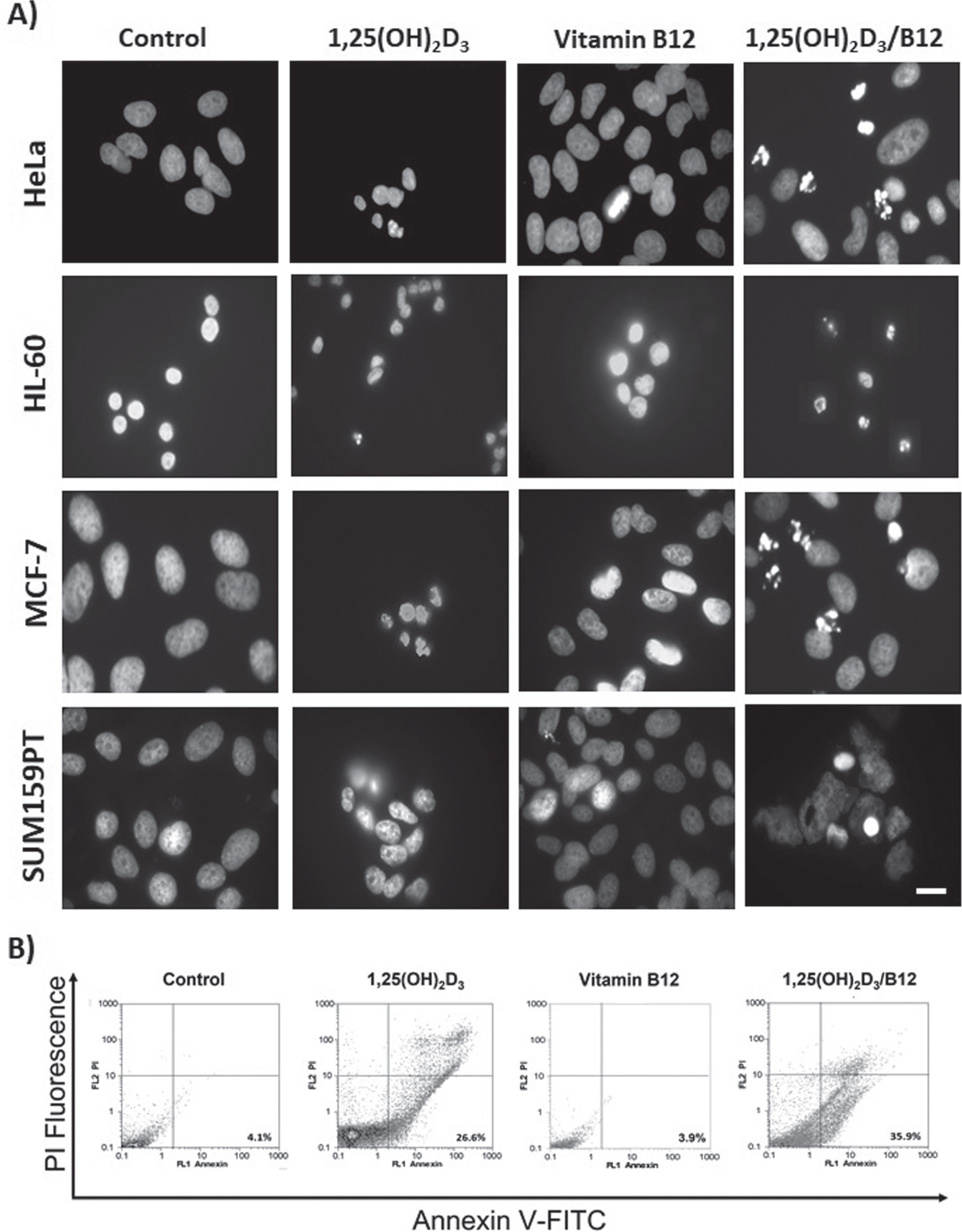
3.3Vitamin B12 augments 1,25(OH)2D3 inhibition of clonal tumor cell growth
The antiproliferative activity of 1,25(OH)2D3, vitamin B12 and the combination was also evaluated by studying cells clonal growth capacity (Fig. 3). Figure 3 showed that 3 h treatment of cancer cells with 1,25(OH)2D3 or the combination of 1,25(OH)2D3 and B12 caused a dose-dependent inhibition of clonogenic tumor growth in all cell lines tested, and that the combination (1,25(OH)2D3/B12) resulted in a better inhibition in HeLa cells (Fig. 3A), HL-60 cells (Fig. 3B), and MCF-7 cells (Fig. 3C) than when treating these cells with 1,25(OH)2D3 alone.
Fig. 3
The combination of vitamin B12 with 1,25(OH)2D3 enhances the inhibition of clonogenic tumor growth caused by 1,25(OH)2D3 alone. Cells were treated for 3 h with increasing concentrations of 1,25(OH)2D3, vitamin B12 and the combination of both and the number of colonies was quantified after 12 days. Bars = mean±SD of three different experiments performed in duplicates. *P < 0.05; **P < 0.01; ***P < 0.001 compared to DMSO-treated control cells, φP < 0.05; φφP < 0.01 compared to 1,25(OH)2D3-treated cells.
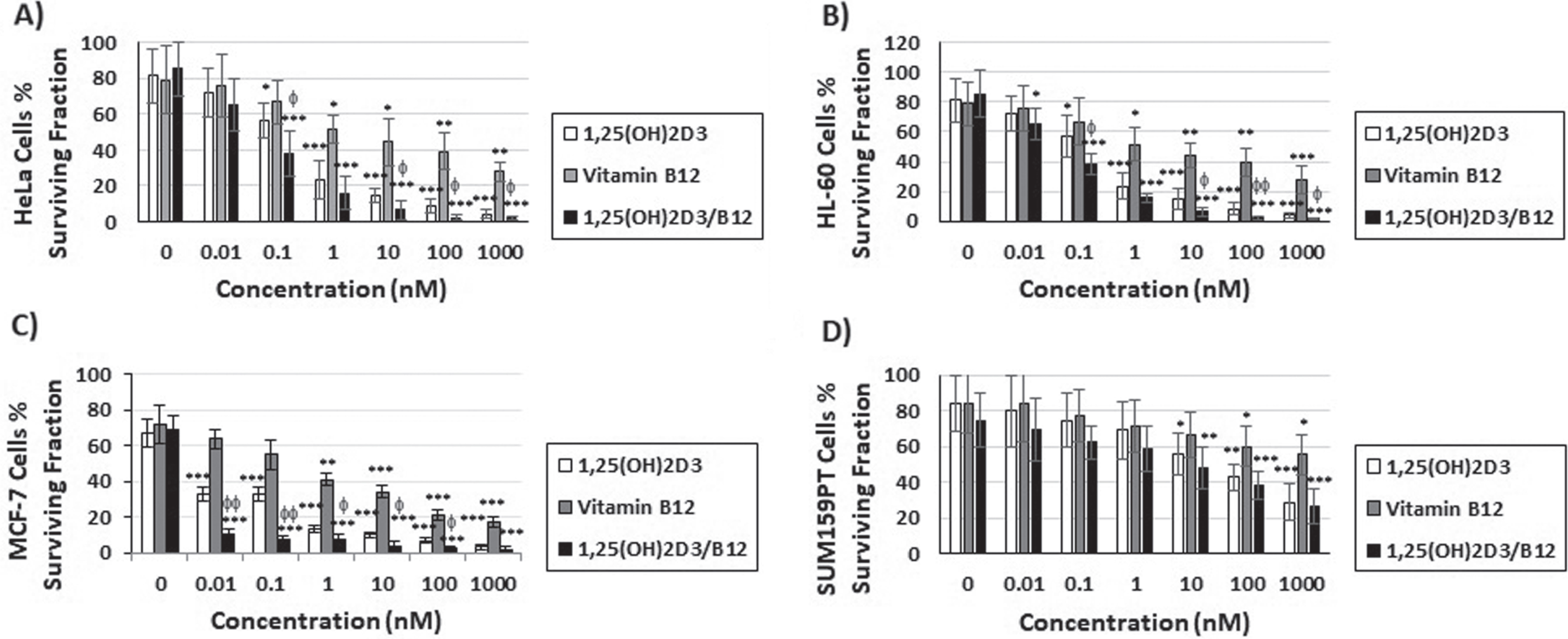
3.4Caspase 4 and 8 mediate 1,25(OH)2D3/B12-induced apoptosis
Since 1,25(OH)2D3 and the combination (1,25(OH)2D3/B12) induced apoptosis in cancer cells (Fig. 2), we aimed to investigate the role of different caspases in this process. The activity of the caspases 3/7, 4, 8 and 9 were measured using the Caspase-Glo assay after treatment with vitamin 1,25(OH)2D3, vitamin B12, and the combination of 1,25(OH)2D3 and vitamin B12 (Fig. 4A). Active caspase 3/7 and caspase 9 levels were lower in treated HL-60 cells when compared to control cells. On the other hand, the levels of active caspase 4 and caspase 8 were significantly increased in 1,25(OH)2D3-treated cells compared to control levels (Fig. 4A). In addition, combining vitamin B12 with 1,25(OH)2D3 increased the levels of active caspase 4 and caspase 8 in treated cells (Fig. 4A) more than when these cells were treated with 1,25(OH)2D3 by itself although insignificantly except for the IC50 treatment.
Fig. 4
1,25(OH)2D3 and 1,25(OH)2D3/B12 combination-induced apoptosis is dependent on caspase 4 and caspase 8 activation. A) Analysis of caspase 3/7, 4, 8 and 9 activation in treated cells by the Caspase-Glo assay compared to HL-60 control cells [fixed to 1.0 arbitrary unit]. The experiment was repeated in triplicate; B) Western blots presenting cleavage “activation’ of procaspase 4 to p20 active form and procaspase 8 to p42/44 active forms in treated HL-60 leukemia cells (IC50 for 1,25(OH)2D3 and 1,25(OH)2D3/B12, and 1000 nM for vitamin B12). The experiment was repeated three times and the corresponding quantification is shown in (C); C) Quantification of procaspase 3, 4, 8, and 9 and their cleaved forms in 1,25(OH)2D3-, vitamin B12-, and 1,25(OH)2D3/B12-treated HL-60 cells relative to the control values set as 1.0 obtained from pixel density of Western blots; D) Representative Western blot showing that 1,25(OH)2D3 or its combination with vitamin B12 caused the release of Smac/DIABLO (Smac) but not cytochrome c from the mitochondria (MF, mitochondrial fraction) to the cytosol (CF, cytosolic fraction) of treated cells (IC50) as compared to vehicle-treated cells. Equal protein loading was controlled by staining membranes with Ponceau S (an illustrative section of the stained membrane is shown). Similar results were achieved in the other cancer cell lines. The experiment was repeated three times and the corresponding quantification is shown in (E); E) Quantification of cytochrome c and Smac/DIABLO in control and IC50-treated HL-60 cells. *P < 0.05; **P < 0.01; ***P < 0.001 compared to DMSO-treated control cells, φP < 0.05 compared to 1,25(OH)2D3-treated cells.
![1,25(OH)2D3 and 1,25(OH)2D3/B12 combination-induced apoptosis is dependent on caspase 4 and caspase 8 activation. A) Analysis of caspase 3/7, 4, 8 and 9 activation in treated cells by the Caspase-Glo assay compared to HL-60 control cells [fixed to 1.0 arbitrary unit]. The experiment was repeated in triplicate; B) Western blots presenting cleavage “activation’ of procaspase 4 to p20 active form and procaspase 8 to p42/44 active forms in treated HL-60 leukemia cells (IC50 for 1,25(OH)2D3 and 1,25(OH)2D3/B12, and 1000 nM for vitamin B12). The experiment was repeated three times and the corresponding quantification is shown in (C); C) Quantification of procaspase 3, 4, 8, and 9 and their cleaved forms in 1,25(OH)2D3-, vitamin B12-, and 1,25(OH)2D3/B12-treated HL-60 cells relative to the control values set as 1.0 obtained from pixel density of Western blots; D) Representative Western blot showing that 1,25(OH)2D3 or its combination with vitamin B12 caused the release of Smac/DIABLO (Smac) but not cytochrome c from the mitochondria (MF, mitochondrial fraction) to the cytosol (CF, cytosolic fraction) of treated cells (IC50) as compared to vehicle-treated cells. Equal protein loading was controlled by staining membranes with Ponceau S (an illustrative section of the stained membrane is shown). Similar results were achieved in the other cancer cell lines. The experiment was repeated three times and the corresponding quantification is shown in (E); E) Quantification of cytochrome c and Smac/DIABLO in control and IC50-treated HL-60 cells. *P < 0.05; **P < 0.01; ***P < 0.001 compared to DMSO-treated control cells, φP < 0.05 compared to 1,25(OH)2D3-treated cells.](https://ip.ios.semcs.net:443/media/tub/2022/44-1/tub-44-1-tub211536/tub-44-tub211536-g004.jpg)
Moreover, the activation of the different caspases was assayed by Western blotting (Fig. 4B). Treating cells with 1,25(OH)2D3 or the combination resulted in the activation of caspase 4 (procaspase cleavage to p20 active form) and caspase 8 (procaspase cleavage to p42 and p44 active forms) but not caspase 3 or 9 (without cleavage of the procaspase to p17 active form, or p35 and p37 active forms, respectively) (Fig. 4B), interestingly, treating cells with 1,25(OH)2D3 showed enhanced caspase 8 cleavage compared to the combination of 1,25(OH)2D3 /B12, in contrast to caspase 4 cleavage (Fig. 4C). In addition, when cytochrome c release assay was performed, the results showed that treatment of HL-60 cancer cells with 1,25(OH)2D3 or the combination of 1,25(OH)2D3 and vitamin B12 showed no release of cytochrome c from mitochondria (mitochondrial fraction; MF) to the cytosol (cytosolic fraction, CF) (Fig. 4D). In contrast, the release of the protein Smac/DIABLO (Smac) was enhanced in response to this treatment (Fig. 4D). HeLa, MCF-7, and SUM159PT cells showed similar results (except for caspase 3 in MCF-7 cells) [42] (data not shown).
3.5Vitamin 1,25(OH)2D3/B12-treated cells are less adhesive than 1,25(OH)2D3-treated cells
Cell adhesion is an essential step during cell migration, [41] thus we analyzed the effects of 1,25(OH)2D3 and its combination with vitamin B12 on cell adhesion by subjecting control and treated cells to the adhesion assay (Fig. 5). The results indicate that vitamin B12 treatment reduced cell adhesion when compared to control cells, however insignificantly (P > 0.05). On the other hand, 1,25(OH)2D3-treated cells showed decreased adhesion compared to control cells, and HeLa cells as well as MCF-7 cells treated with both 1,25(OH)2D3 and vitamin B12 were even less adhesive than vitamin 1,25(OH)2D3-treated cells (Fig. 5).
Fig. 5
1,25(OH)2D3/B12-treated cells are less adhesive than 1,25(OH)2D3-treated cells. Control and treated cells (IC50 for 1,25(OH)2D3 and 1,25(OH)2D3/B12, and 1000 nM for vitamin B12) were subjected to the adhesion assay. Bars = mean±SD of three independent experiments. *P < 0.05; **P < 0.01; ***P < 0.001 compared to DMSO-treated control cells, φP < 0.05 compared to 1,25(OH)2D3-treated cells.
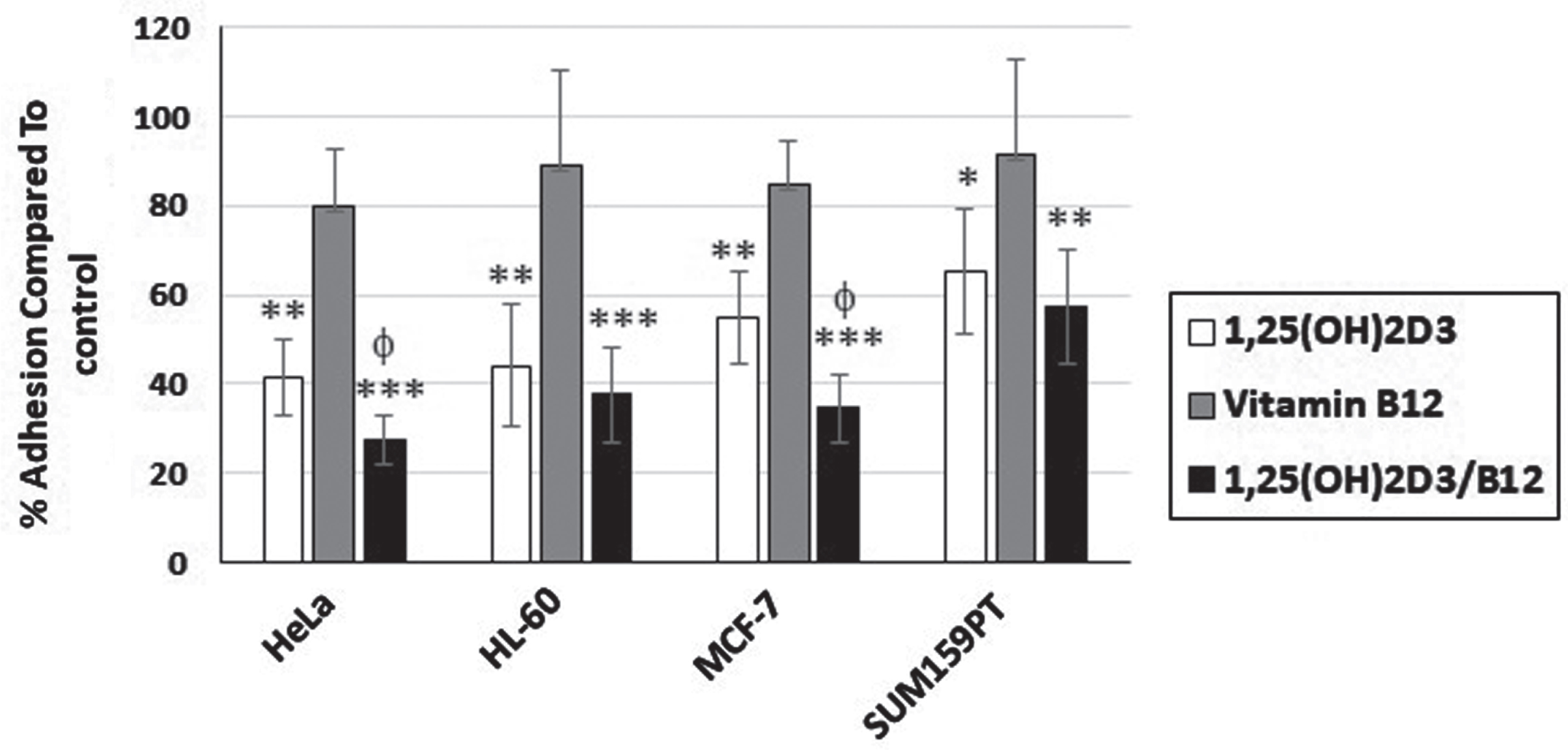
3.6Vitamin B12 intensifies the effects of 1,25(OH)2D3 on cell morphology and polarization
To study the effects of 1,25(OH)2D3 and the combination of 1,25(OH)2D3 and vitamin B12 on the morphology and polarization of cells, control and treated cells were stained with fluorescently labeled phalloidin. 1,25(OH)2D3-treated HeLa cells showed reduced length, width, and cell area (Table 2), disorganized and reduced actin filaments, as well as more cell protrusions and reduced cell migratory morphology as compared to control cells (Fig. 6A), similarly, 1,25(OH)2D3-treated MCF-7 cells were smaller in size (Table 2), showed actin aggregates and short actin filaments and most cells had thin lamellipodia (Fig. 6A). Combining vitamin B12 with 1,25(OH)2D3 caused cell rounding in both cell types, a common feature of apoptotic cells (Table 2, Fig. 6A). Control HL-60 cells have a round shape and cortical actin filaments distribution, 1,25(OH)2D3-treated cells lost that typical cell morphology and became smaller (Table 2), actin filaments got shorter and less cortical, and cells showed protrusion, the same was more evident in 1,25(OH)2D3/B12-treated cells (Fig. 6A). The effect of 1,25(OH)2D3 on SUM159PT cell morphology and actin cytoskeleton was not as pronounced, the most obvious effect was thinning of actin filaments (Fig. 6A), the same was observed in 1,25(OH)2D3/B12-treated cells in addition to the formation of thin protrusion. Cell area, height, and width of 1,25(OH)2D3/B12-treated SUM159PT cells were significantly smaller than control cells but not than 1,25(OH)2D3-treated cells (Table 2). In all cell lines, vitamin B12 did not cause a significant change to cell morphology, actin cytoskeleton or cell area (Fig. 6 and Table 2)
Table 2
Vitamin B12 enhances the effects of 1,25(OH)2D3on cell shape parameters.
Area (μm2) | Height (μm) | Width (μm) | ||||||||||
HeLa | HL-60 | MCF-7 | SUM | HeLa | HL-60 | MCF-7 | SUM | HeLa | HL-60 | MCF-7 | SUM | |
Control | 1569.4 ±593.3 | 654.9±148.1 | 2387.1 ±1674.8 | 2589.6±1389.4 | 48.7 ±20.7 | 27.5±15.3 | 67.2 ±30.8 | 71.4±31.6 | 45.7 ±28.4 | 24.4 ±10.0 | 62.2±27.0 | 65.4±18.4 |
1,25(OH)2D3 | 1209.2 ±359.9* | 406.3±114.1* | 1692.4 ±818.0** | 1989.2±712.9 | 40.5±18.5 | 17.7±21.4* | 59.1 ±29.6 | 63.2±22.3 | 35.0±21.3* | 14.0±9.5* | 49.3 ±26.9* | 53.4±19.4 |
Vitamin B12 | 1429.7±429.6 | 623.7±127.5 | 2019.7±989.4 | 2444.2±1002.1 | 42.3±16.5 | 24.1±12.8 | 60.0±26.6 | 73.2±28.8 | 41.3±29.6 | 23.3±9.3 | 62.4±25.4 | 61.8±22.4 |
1,25(OH)2D3/B12 | 799.3 ±205.9**φφ | 371.8±152.0* | 1087.8 ±905.4***φ | 1883.2±992.1* | 29.4±12.8**φ | 14.5±8.7* | 40.9±21.6**φ | 59.1±24.6* | 22.6±24.3***φφ | 9.5±7.5**φ | 39.0 ± 19.6** | 49.5±16.7** |
Data are expressed as mean±SD, n≥100 cells in each experiment, three independent experiments. *p < 0.05, **p < 0.01, ***p < 0.001 versus control, φP < 0.05; φφP < 0.01 compared to 1,25(OH)2D3-treated control cells
Fig. 6
Vitamin B12 intensifies the effects of 1,25(OH)2D3 on cell polarization. A) Control and treated cells (IC50 for 1,25(OH)2D3 and 1,25(OH)2D3/B12, and 1000 nM for vitamin B12) were stained with fluorescently-labeled phalloidin. The image of 1,25(OH)2D3/B12-treated HL-60 cells is a composite image since very few cells remained attached to the cove slip. Scale bar: 10μm; B) Control and treated HeLa, MCF-7, and SUM159PT cells were categorized as apolar, bipolar or polar (arrows show the leading edge and direction of movement) and the percentage of polarized cells was scored in (C); C) Control and vitamin-treated cells were scored for percentage of polarized cells. Bars = mean±SD of three independent experiments. *P < 0.05; **P < 0.01 compared to DMSO-treated control cells, φP < 0.05 compared to 1,25(OH)2D3-treated cells.
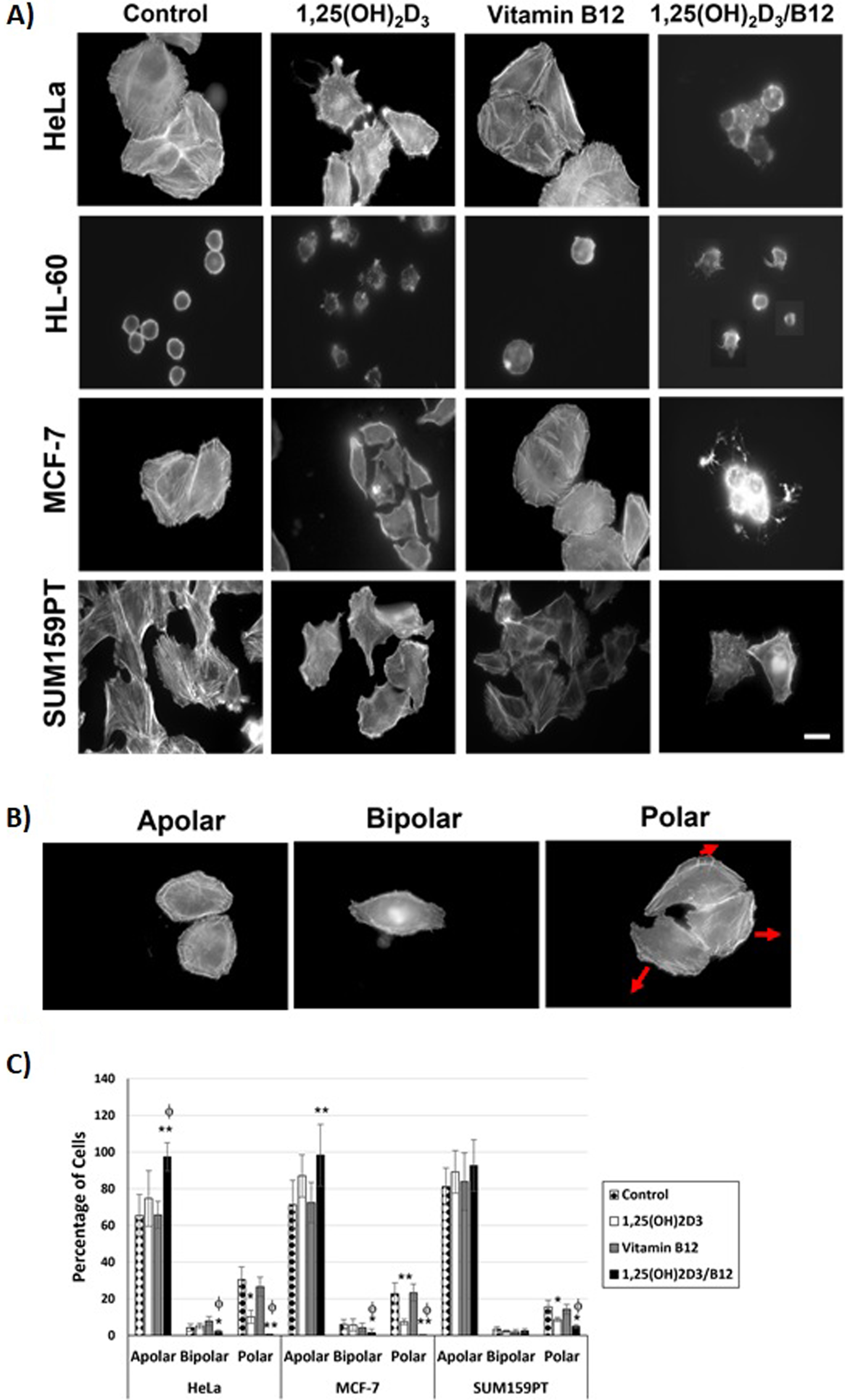
HeLa, MCF-7, and SUM159PT cells were classified as apolar (no or more than 2 lamellipodia), bipolar (2 lamellipodia) or polar (one leading edge/lamellipodium) (Fig. 6B). Figure 6C showed that the percentage of polar cells in 1,25(OH)2D3-treated cells was reduced compared to control, and that combining vitamin B12 with 1,25(OH)2D3 decreases the percentage of polar cells as compared to 1,25(OH)2D3 treated cells.
4Discussion
Most cancers are associated with activation of several oncogenic signal transduction pathways. Therefore, recent studies have focused on the development of combination therapies using multiple anticancer agents to efficiently inhibit compound oncogenic pathways. A previous clinical study reported the anticancer effects of vitamin D3; [43] additionally, several in vivo and in vitro studies showed that vitamin D3 induced cell growth inhibition [44, 45]. However, and up to our knowledge, no previous study investigated the synergistic antitumor effects of combined vitamin D3 or its active form (1,25(OH)2D3) and B12 on different cancer cell lines.
The biological activity of 1,25(OH)2D3 depends on its association with one of the binding sites of vitamin D receptor (VDR), either a genomic pocket (VDR-GP) or an alternative pocket (VDR-AP). In the genomic pathway, the 1,25(OH)2D3/VDR complex acts as a transcription factor that produces a heterodimeric complex with retinoid X receptor (RXR). This complex in turn binds to vitamin D responsive elements stimulating transcriptional co-activators or co-repressors that cause positive or negative downstream regulations [6]. On the other hand, 1,25(OH)2D3 via the non-genomic pathways, exerts short-term effects and acts as a protective agent against skin cancer and against sunlight-induced DNA damage [16]. It is worth mentioning that in addition to the VDR canonical pathway, some active forms of vitamin D such as 20(OH)D3 and 20,23(OH)2D3 bind other nuclear receptors such RORα and RORγ, [46] AhR, [47, 48], and liver X receptors (LXRs) [49].
The role of vitamin D3 in preventing cellular oncogenic transformation is mediated through several modulations in various stages of oncogenesis ranging from tumor initiation to progression, and cell-microenvironment interactions [10]. These modulations induce cell cycle arrest, differentiation, and apoptosis.
Vitamin B12 is a water-soluble vitamin essential to cell production, DNA synthesis and neurologic function. Moreover, vitamin B12 acts as a co-enzyme in the metabolism of different amino acids such as methionine, threonine, and valine [50]. Additionally, vitamin B12 was reported to have potential antioxidant properties by scavenging reactive oxygen species [51]. So far, there is no proof that dietary vitamin B12 alone decreases the risk of cancer. However, vitamin B12 may lessen the risk of breast cancer when taken with folate, vitamin B6, and methionine [52].
The results of this study demonstrated significant concentration-dependent cytotoxic effects of 1,25(OH)2D3 but not B12 on four out of the six cancer cell lines tested (HeLa, HL-60, MCF-7, and SUM159PT; Fig. 1). Moreover, a synergistic cytotoxic effect for combining 1,25(OH)2D3 and B12 together on HeLa, HL-60, and MCF-7 cancer cells was also shown (Fig. 1). However, this synergetic effect was not constant, some concentrations of the combination did not show a higher cytotoxic when compared to 1,25(OH)2D3 alone such as the 1000 nM in HeLa cells and 100 nM in MCF-7 cells. It was reported previously that some drug combinations achieve their optimum effects when the concentration of the combination is lower than the concentration of the best individual drug, sometimes this is referred to as antagonism or hyperantagonism, where the pair has a lower effect than either or one of the two single drugs.
Our results indicated that 1,25(OH)2D3 and B12 synergism inhibited the growth of these cancer cells, primarily due to apoptosis (Fig. 2). These results are in accordance with previous studies that showed that vitamin D3 treatment reduced the number of cancer stem cells in MCF-7 cell line, [53] comparable results were also reported [54].
The results presented in Table 1 indicate that 1,25(OH)2D3 treatment (and the combination) exhibited cytotoxic effects in only one of the TNBC cell lines tested (SUM159PT) but not in BT549 or MDA-MB-453 TNBC cell lines (Table 1). This was in contradiction to previous studies reviewed by Blasiak et al. (2020) [55]. Low vitamin D levels are noticed in TNBC patients, suggesting that vitamin D can be used as a protective agent. Vitamin D3 can decrease the metastatic potential of TNBC cells by down-regulating invasion and metastasis genes. Additionally, vitamin D3 can act as anti-proliferative agent by inducing growth arrest and DNA damage-inducible 45 alpha (GADD45) proteins as well as stimulating the synthesis of TNF-α in TNBC cells to prevent their propagation [55].
Fig. 7
Proposed scheme of various apoptotic pathways involved in 1,25(OH)2D3/B12-induced apoptosis. The combination of 1,25(OH)2D3 and vitamin B12 stimulates the death receptor and activates caspase 8, then the combination is internalized where it induces the ER stress pathway and then caspase 4. Caspase 4 and 8 then activate an executioner caspase such as caspase 6 which leads to activation of the apoptotic process such as nuclear fragmentation, dysregulation of actin cytoskeleton and reduced cell migration.
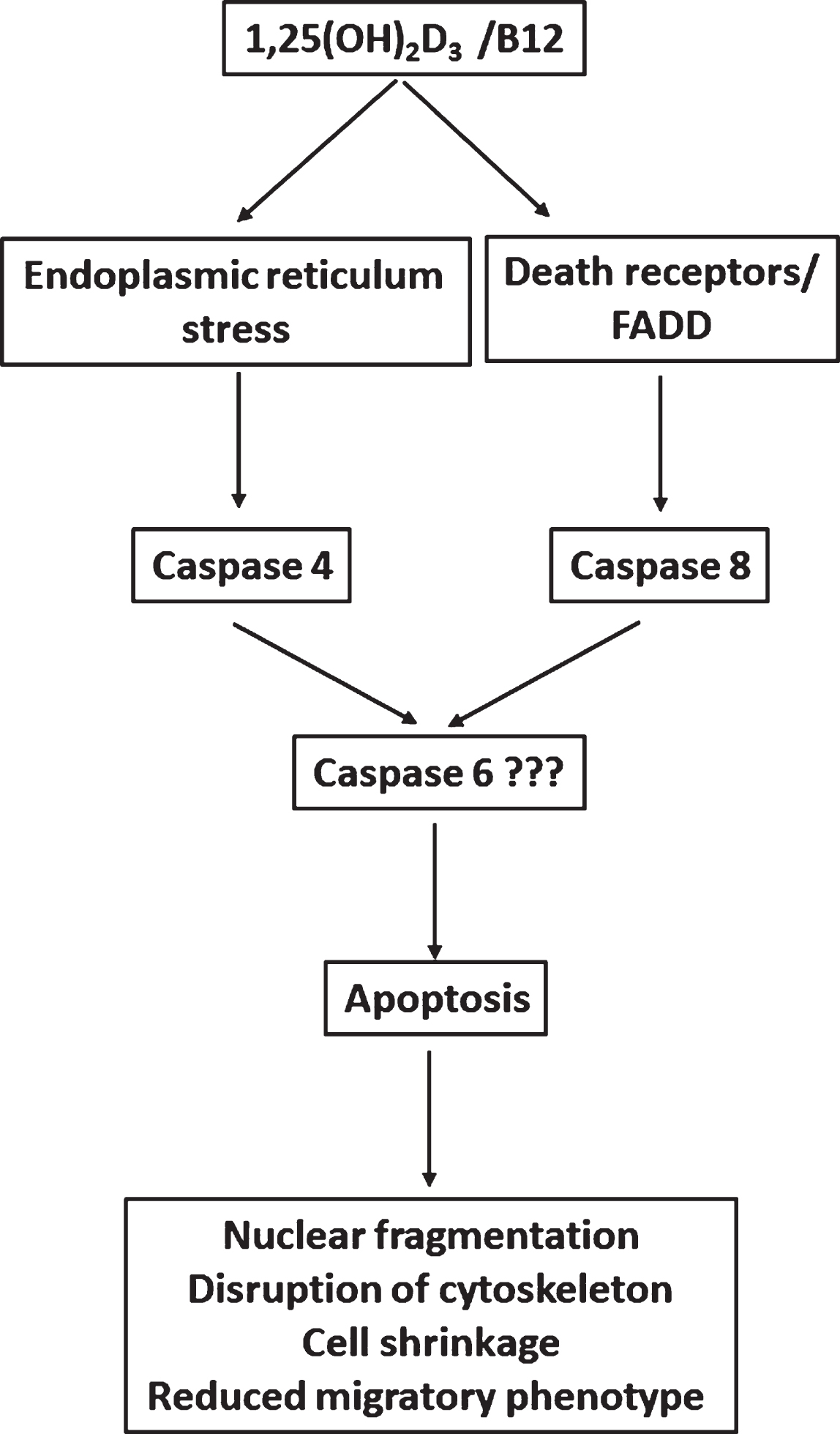
Apoptosis is an active cell suicide process that maintains homeostasis in cells. This process is classified as either caspase (cysteine protease)-dependent or caspase-independent. A caspase-dependent action is mediated via the mitochondrial-dependent intrinsic pathway characterized by cytochrome c release from the mitochondria and caspase 9 activation, and the death receptor-mediated extrinsic pathway characterized by caspase 8 activation [56]. The present study showed that caspase 8 was activated in treated cells as shown by the caspase activity assay and Western blot analysis (Fig. 4). Moreover, the result showed that neither 1,25(OH)2D3, vitamin B12 nor their combination stimulated cytochrome c release from the mitochondria (Fig. 4), and they did not stimulate caspase 3/7 or caspase 9 activation (Fig. 4). These results indicate that 1,25(OH)2D3 induced apoptosis in the tested cancer cells through the extrinsic apoptosis pathway.
Additionally, the results presented in Fig. 4 show that 1,25(OH)2D3 treatment (and the combination of vitamin B12 and 1,25(OH)2D3) worked through activating the ER stress pathway. ER stress is caused by dysregulation of protein folding, and if this is prolonged or if the repair system fails, then ER-mediated apoptosis signaling is activated. The hallmarks of this atypical apoptosis pathway are activation of caspase 4, enhanced phosphorylation of the eukaryotic translation initiation factor 2 (eIF2) and release of Smac/DIABLO into the cytosol [23, 57]. Previous work has reported that 1,25(OH)2D3 induced apoptosis in MCF-7 breast cancer cells by induction of the endoplasmic reticulum (ER)-stress-like response [23]. The collaborative activation of both caspases (4 and 8) in other processes such as in response to infection has been reported previously [58, 59].
Acquiring a polarized morphology is essential for cell migration and cancer metastasis. The dynamic reorganization of actin cytoskeleton regulates almost all steps of cell migration including formation pf protrusions, cell adhesion, cell body contractility and formation of invadopodia [60, 61]. The results presented in Fig. 6A show that treated cells exhibited disorganized actin cytoskeleton and consequently they were less adhesive (Fig. 5) and less polarized (Fig. 6C). Additionally, it was shown that cells treated with the combination of vitamin B12 with 1,25(OH)2D3 were less adhesive (Fig. 5) and showed reduced percentage of cell polarization (Fig. 6C) when compared to 1,25(OH)2D3-treated cells.
5Conclusions
In conclusion, the present study revealed the synergistic anticancer effects of 1,25(OH)2D3 and B12 against different cancer cell lines. 1,25(OH)2D3 induced cytotoxicity in HeLa, HL-60, MCF-7 and SUM159PT cancer cells by induction of apoptosis as evident by fragmented nuclei after DAPI staining and flow cytometry analysis. The combination of vitamin B12 with 1,25(OH)2D3 enhanced the cytotoxic effects of 1,25(OH)2D3 as well as its inhibition of clonal tumor cell growth. The induction of apoptosis by 1,25(OH)2D3/B12 was achieved through activation of both caspase 4 and 8. 1,25(OH)2D3/B12-treated cells were less adhesive, showed reduced cell area, disorganized actin cytoskeleton and reduced percentage of polarized cells and thus had reduced migratory phenotype (Fig. 7) compared to cells treated with 1,25(OH)2D3 alone. The combination therapy of 1,25(OH)2D3 and vitamin B12 may provide a potential adjunctive treatment option for some cancer types.
Acknowledgments
The authors are grateful to the Deanship of Scientific Research, The Hashemite University for supporting the current work (grant No. 11/2016). We thank Omar Hanbali for helpful comments and advice in the manuscript preparation.
Authors contributions
CONCEPTION: MA, LT and FA
DATA CURATION: LT, ZA and MR
ANALYSIS OF DATA: LT, FA, ZA and MR
PREPARATION OF THE MANUSCRIPT: MA, LT and FA
REVISION FOR IMPORTANT INTELLECTUAL CONTENT: All authors
Conflict of interest
The authors have no conflict of interest to declare.
Ethical considerations
This research was approved by the Hashemite University IRB committee (IRB/11/2016). The work was carried out from 2018 until 2020.
References
[1] | Atoum M , Alzoughool F . Vitamin D and Breast Cancer: Latest Evidence and Future Steps. Breast Cancer (Auckl). (2017) ;11: :1178223417749816. doi: 10.1177/1178223417749816. |
[2] | Cancer. World Health Organization (WHO); 2021. |
[3] | Mokhtari R , Homayouni T , Baluch N , Morgatskaya E , Kumar S , Das B , et al. Combination therapy in combating cancer. Oncotarget. (2017) ;8: (23):38022–43. doi: 10.18632/oncotarget.16723. |
[4] | Dusso A , Brown A , Slatopolsky E . Vitamin D. Am J Physiol Renal Physiol. (2005) ;289: (1):F8–28. doi: 10.1152/ajprenal.00336.2004. |
[5] | Bikle D . Vitamin D metabolism, mechanism of action, and clinical applications. Chem Biol. (2014) ;21: (3):319–29. doi: 10.1016/j.chembiol.2013.12.016. |
[6] | Jeon S , Shin E . Exploring vitamin D metabolism and function in cancer. Exp Mol Med. (2018) ;50: (4):1–14. doi: 10.1038/s12276-018-0038-9. |
[7] | Chang S , Lee H . Vitamin D and health - The missing vitamin in humans. Pediatr Neonatol. (2019) ;60: (3):237–44. doi: 10.1016/j.pedneo.2019.04.007. |
[8] | El-Sharkawy A , Malki A . Vitamin D signaling in inflammation and cancer: molecular mechanisms and therapeutic implications. Molecules. (2020) ;25: (14):3219. doi: 10.3390/molecules25143219. |
[9] | Slominski A , Kim T , Shehabi H , Semak I , Tang E , Nguyen M , Benson H , Korik E , Janjetovic Z , Chen J , Yates C , Postlethwaite A , Li W , Tuckey R . In vivo evidence for a novel pathway of vitamin D3 metabolism initiated by P450scc and modified by CYP27B1. FASEB J. (2012) ;26: (9):3901–15. doi: 10.1096/fj.12-208975. |
[10] | Slominski A , Kim T , Shehabi H , Tang E , Benson H , Semak I , Lin Z , Yates C , Wang J , Li W , Tuckey R . In vivo production of novel vitamin D2 hydroxy-derivatives by human placentas, epidermal keratinocytes, Caco-2 colon cells and the adrenal gland. Mol. Cell. Endocrinol. (2014) ;383: (1–2):181–92. doi: 10.1016/j.mce.2013.12.012. |
[11] | Slominski A , Li W , Kim T , Semak I , Wang J , Zjawiony J , Tuckey R . Novel activities of CYP11A1 and their potential physiological significance. J Steroid Biochem Mol Biol. (2015) ;151: :25–37. doi: 10.1016/j.jsbmb.2014.11.010. |
[12] | Slominski A , Kim T , Li W , Postlethwaite A , Tieu E , Tang E , Tuckey R . Detection of novel CYP11A1-derived secosteroids in the human epidermis and serum and pig adrenal gland. Sci Rep. (2015) ;5: :14875. doi: 10.1038/srep14875. |
[13] | Saracligil B , Ozturk B , Unlu A , Abusoglu S , Tekin G . The effect of vitamin D on MCF-7 breast cancer cell metabolism. Bratisl Lek Listy. (2017) ;118: (2):101–6. doi: 10.4149/BLL_2017_021. |
[14] | Abu el Maaty M , Dabiri Y , Almouhanna F , Blagojevic B , Theobald J , Büttner M , et al. Activation of pro-survival metabolic networks by 1,25(OH)2D3 does not hamper the sensitivity of breast cancer cells to chemotherapeutics. Cancer Metab. (2018) ;6: :11. doi: 10.1186/s40170-018-0183-6. |
[15] | Sang-Min Jeon , Eun-Ae Shin . Exploring vitamin D metabolism and function in cancer. Exp Mol Med. (2018) ;50: (4):20. doi: 10.1038/s12276-018-0038-9. |
[16] | Feldman D , Krishnan AV , Swami S , Giovannucci E , Feldman BJ . The role of vitamin D in reducing cancer risk and progression. Nat Rev Cancer. (2014) ;14: :342–57. doi: 10.1038/nrc3691. |
[17] | Pandolfi F , Franza L , Mandolini C , Conti P . Immune modulation by vitamin D: special emphasis on its role in prevention and treatment of cancer. Clin Ther. (2017) ;39: (5):884–93. doi: 10.1016/j.clinthera.2017.03.012. |
[18] | Rots NY , Iavarone A , Bromleigh V , Freedman LP . Induced differentiation of U937 cells by 1,25-dihydroxyvitamin D3 involves cell cycle arrest in G1 that is preceded by a transient proliferative burst and an increase in cyclin expression. Blood. (1999) ;93: (8), 2721–9. PMID: 10194452. |
[19] | Wu G , Fan R , Li W , Ko T , Brattain M . Modulation of cell cycle control by vitamin D3 and its analogue, EB1089, in human breast cancer cells. Oncogene. (1997) ;15: :1555–63. doi: 10.1038/sj.onc.1201329. |
[20] | Kawa S , Nikaido T , Aoki Y , Zhai Y , Kumagai T , Furihata K , et al. Vitamin D analogues upregulate p21 and p27 during growth inhibition of pancreatic cancer cell lines. Br J Cancer. (1997) ;76: (7):884–9. doi: 10.1038/bjc.1997.479. |
[21] | Deeb K , Trump D , Johnson C . Vitamin D signalling pathways in cancer: potential for anticancer therapeutics. Nat Rev Cancer. (2007) ;7: (9):684–700. doi: 10.1038/nrc2196. |
[22] | Moukayed M , Grant W . The roles of UVB and vitamin D in reducing risk of cancer incidence and mortality: A review of the epidemiology, clinical trials, and mechanisms. Rev Endocr Metab Disord. (2017) ;18: (2):167–82. doi: 10.1007/s11154-017-9415-2. |
[23] | Ozkaya AB , Ak H , Aydin HH . High concentration calcitriol induces endoplasmic reticulum stress related gene profile in breast cancer cells. Biochem Cell Biol. (2017) ;95: (2):289–94. doi: 10.1139/bcb-2016-0037. |
[24] | Haddur E , Ozkaya AB , Ak H , Aydin HH . The effect of calcitriol on endoplasmic reticulum stress response. Biochem Cell Biol. (2015) ;93: (3):268–71. doi: 10.1139/bcb-2014-0155. |
[25] | Bernardi RJ , Trump DL , Yu WD , McGuire TF , Hershberger PA , Johnson CS . Combination of 1alpha,25-dihydroxyvitamin D(3) with dexamethasone enhances cell cycle arrest and apoptosis: role of nuclear receptor cross-talk and Erk/Akt signaling. Clin Cancer Res. (2001) ;7: (12), 4164–73. PMID: 11751517. |
[26] | Sharabani H , Izumchenko E , Wang Q , Kreinin R , Steiner M , Barvish Z , et al. Cooperative antitumor effects of vitamin D3 derivatives and rosemary preparations in a mouse model of myeloid leukemia. Int J Cancer. (2006) ;118: (12):3012–21. doi: 10.1002/ijc.21736. |
[27] | Mily A , Rekha RS , Kamal SMM , Arifuzzaman ASM , Rahim Z , Khan L , et al. Significant effects of oral phenylbutyrate and vitamin D3 adjunctive therapy in pulmonary tuberculosis: a randomized controlled trial. PLoS ONE. (2015) ;10: (9):e0138340. doi: 10.1371/journal.pone.0138340. |
[28] | Hunt A , Harrington D , Robinson S . Vitamin B12 deficiency. BMJ. (2014) ;349: :g5226. doi: 10.1136/bmj.g5226. |
[29] | Karamshetty V , Acharya JD , Ghaskadbi S , Goel P . Mathematical modeling of glutathione status in type 2 diabetics with vitamin B12 deficiency. Front Cell Dev Biol. (2016) ;4: :16. doi: 10.3389/fcell.2016.00016. |
[30] | Tyagi N . Mechanisms of homocysteine-induced oxidative stress. AJP Heart Circ Physiol. (2005) ;289: :H2649–H2656. doi: 10.1152/ajpheart.00548.2005. |
[31] | Liu Y , Wang X , Sun X , Lu S , Liu S . Vitamin intake and pancreatic cancer risk reduction: A meta-analysis of observational studies. Medicine (Baltimore). (2018) ;97: (13):e0114. doi: 10.1097/MD.0000000000010114. |
[32] | Fenech M . The role of folic acid and Vitamin B12 in genomic stability of human cells. Mutat Res. (2001) ;475: (1-2):57–67. doi: 10.1016/s0027-5107(01)00079-3. |
[33] | Ryan-Harshman M , Aldoori W . Vitamin B12 and health. Can Fam Physician. (2008) ;54: (4):536–41.PMID: 18411381; PMCID: PMC2294088. |
[34] | Chen Z , Liang Y , Feng X , Liang Y , Shen G , Huang H , et al. Vitamin-B12-conjugated PLGA-PEG nanoparticles incorporating miR-532-3p induce mitochondrial damage by targeting apoptosis repressor with caspase recruitment domain (ARC) on CD320-overexpressed gastric cancer. Mater Sci Eng C Mater Biol Appl. (2021) ;120: :111722. doi: 10.1016/j.msec.2020.111722. |
[35] | Solovieva M , Shatalin Y , Fadeev R , Krestinina O , Baburina Y , Kruglov A , et al. Vitamin B 12b Enhances the Cytotoxicity of Diethyldithiocarbamate in a Synergistic Manner, Inducing the Paraptosis-Like Death of Human Larynx Carcinoma Cells. Biomolecules. (2020) ;10: (1):69. doi: 10.3390/biom10010069. |
[36] | Solovyeva ME , Faskhutdinova AA , Solovyev VV , Akatov VS . Thiol antioxidants in combination with vitamin B12 induce apoptotic death of human lymphocytic leukemia cells by destabilization of lysosomes with the involvement of iron ions. Bull Exp Biol Med. (2013) ;154: (4):449–52. doi: 10.1007/s10517-013-1974-y. |
[37] | Mosmann T . Rapid colorimetric assay for cellular growth and survival: application to proliferation and cytotoxicity assays. J Immunol Methods. (1983) ;65: :55–63. doi: 10.1016/0022-1759(83)90303-4. |
[38] | Fotakis G , Timbrell JA . In vitro cytotoxicity assays: comparison of LDH, neutral red, MTT and protein assay in hepatoma cell lines following exposure to cadmium chloride. Toxicol Lett. (2006) ;160: :171–7. doi: 10.1016/j.toxlet.2005.07.001. |
[39] | Franken NA , Rodermond HM , Stap J , Haveman J , van Bree C . Clonogenic assay of cells in vitro. Nat Protoc. (2006) ;1: :2315–9. doi: 10.1038/nprot.2006.339. |
[40] | Rafehi H , Orlowski C , Georgiadis GT , Ververis K , El-Osta A , Karagiannis TC . Clonogenic assay: adherent cells. J Vis Ex. (2011) ;(49):2573. doi: 10.3791/2573. |
[41] | Tahtamouni LH , Shaw AE , Hasan M ; Yasin SR ; Bamburg JR . Non-overlapping activities of ADF and cofilin-1 during the migration of metastatic breast tumor cells. BMC Cell Biol. (2013) ;14: :45(2013). doi: 10.1186/1471-2121-14-45. |
[42] | Semenov D , Aronov P , Kuligina E , Potapenko M , Richter V . Oligonucleosomal DNA fragmentation in MCF-7 cells undergoing palmitate-induced apoptosis. Biochemistry. (2003) ;68: :1335–41. doi: 10.1023/B:BIRY.0000011655.58235.44. |
[43] | Kumar SBV , Singh S , Verma R . Anticancer potential of dietary vitamin D and ascorbic acid: A review. Crit Rev Food Sci Nutr. (2017) ;57: (12):2623–35. doi: 10.1080/10408398.2015.1064086. |
[44] | Swami S , Krishnan AV , Wang JY , Jensen K , Peng L , Albertelli MA , et al. Inhibitory effects of calcitriol on the growth of MCF-7 breast cancer xenografts in nude mice: selective modulation of aromatase expression in vivo . Horm Cancer. (2011) ;2: (3):190–202. doi: 10.1007/s12672-011-0073-7. |
[45] | Nicolas S , Bolzinger MA , Jordheim LP , Chevalier Y , Fessi H , Almouazen E . Polymeric nanocapsules as drug carriers for sustained anticancer activity of calcitriol in breast cancer cells. Int J Pharm. (2018) ;550: (1-2):170–9. doi: 10.1016/j.ijpharm.2018.08.022. Epub 2018 Aug. |
[46] | Slominski A , Kim T , Takeda Y , Janjetovic Z , Brożyna A , Skobowiat C , Wang J , Postlethwaite A , Li W , Tuckey , Jetten A . RORα and ROR γ are expressed in human skin and serve as receptors for endogenously produced noncalcemic 20-hydroxy- and 20,23-dihydroxyvitamin D. FASEB J. (2014) ;28: :2775–89. doi: 10.1096/fj.13-242040. |
[47] | Slominski A , Kim T , Janjetovic Z , Brożyna A , Żmijewski M , Xu H , Sutter T , Tuckey R , Jetten A , Crossman D. . Differential and Overlapping Effects of 20,23(OH)2D3 and 1,25(OH)2D3 on Gene Expression in Human Epidermal Keratinocytes: Identification of AhR as an Alternative Receptor for 20,23(OH)2D3. Int J Mol Sci. (2018) ;19: (10):3072. https://doi.org/10.3390/ijms19103072. |
[48] | Slominski A , Chaiprasongsuk A , Janjetovic Z , Kim T , Stefan J , Slominski R , Hanumanthu V , Raman C , Qayyum S , Song Y , Song Y , Panich U , Crossman D , Athar M , Holick M , Jetten A , Zmijewski M , Zmijewski J , Tuckey R . Photoprotective Properties of Vitamin D and Lumisterol Hydroxyderivatives. Cell Biochem Biophys. (2020) ;78: (2):165–80. doi: 10.1007/s12013-020-00913-6. |
[49] | Slominski A , Kim T , Qayyum S , Song Y , Janjetovic Z , Oak A , Slominski R , Raman C , Stefan J , Mier-Aguilar C , Atigadda V , Crossman D , Golub A , Bilokin Y , Tang E , Chen J , Tuckey R , Jetten A , Song Y . Vitamin D and lumisterol derivatives can act on liver X receptors (LXRs). Sci Rep. (2021) ;11: :8002. doi: 10.1038/s41598-021-87061-w. |
[50] | Kapadia CR . Vitamin B12 in health and disease: part I–inherited disorders of function, absorption, and transport. Gastroenterologist. (1995) ;3: (4), 329–44. PMID: 8775094. |
[51] | van de Lagemaat E , de Groot L , van den Heuvel E . Vitamin B12 in relation to oxidative stress: A systematic review. Nutrients. (2019) ;11: (2):482. doi: 10.3390/nu11020482. |
[52] | Lajous M , Lazcano-Ponce E , Hernandez-Avila M , Willett W , Romieu I . Folate, vitamin B(6), and vitamin B(12) intake and the risk of breast cancer among Mexican women. Cancer Epidemiol Biomarkers Prev. (2006) ;15: (3):443–8. doi: 10.1158/1055-9965.EPI-05-0532. |
[53] | Koçak N , Nergiz S , Yildirim IH , Duran Y . Vitamin D can be used as a supplement against cancer stem cells. Cell Mol Biol (Noisy-le-grand). (2018) ;64: (12), 47–51. PMID: 30301502. |
[54] | Thyer L , Ward E , Smith R , Fiore MG , Magherini S , Branca JJV , et al. A novel role for a major component of the vitamin D axis: Vitamin D binding protein-derived macrophage activating factor induces human breast cancer cell apoptosis through stimulation of macrophages. Nutrients. (2013) ;5: (7):2577–89. doi: 10.3390/nu5072577. |
[55] | Blasiak J , Pawlowska E , Chojnacki J , Szczepanska J , Fila M , Chojnacki C . Vitamin D in HeLa-negative and BRCA1-deficient breast cancer-implications for pathogenesis and therapy. Int J Mol Sci. (2020) ;21: (10):3670. doi: 10.3390/ijms21103670. |
[56] | Elmore S . Apoptosis: a review of programmed cell death. Toxicol Pathol. (2007) ;35: :495–516. doi: 10.1080/01926230701320337. |
[57] | d’Azzo A , Tessitore A , Sano R . Gangliosides as apoptotic signals in ER stress response. Cell Death Differ. (2006) ;13: (3):404–14. doi: 10.1038/sj.cdd.4401834. |
[58] | Mandal P , Feng Y , Lyons J , Berger S , Otani S , DeLaney A , et al. Caspase-8 collaborates with caspase-11 to drive tissue damage and execution of endotoxic shock. Immunity. (2018) ;49: (1):42–55.e6. doi: 10.1016/j.immuni.2018.06.011. |
[59] | Pereira L , Assis P , de Araújo N , Durso D , Junqueira C , Ataíde M , et al. Caspase-8 mediates inflammation and disease in rodent malaria. Nat Commun. (2020) ;11: :4596. doi: 10.1038/s41467-020-18295-x. |
[60] | Lindberg U , Karlsson R , Lassing I , Schutt C , Höglund A . The microfilament system and malignancy. Semin Cancer Biol. (2008) ;18: (1):2–11. doi: 10.1016/j.semcancer.2007.10.002. |
[61] | Tahtamouni LH , Bamburg JR . Redundant and non-redundant functions of actin depolymerizing factor (ADF) and cofilin in metastasis- review. JJBS. (2011) ;4: (1):1–12. doi: 10.1016/j.cub.2017.01.018 |