Energy absorption at lower limb joints in different foot contact strategies while descending stairs
Abstract
BACKGROUND:
Joint loads in different walking strategies during stair descent have been investigated in terms of the joint moment in association with the risk of osteoarthritis. However, the absorption mechanisms of the potential energy loss are not known.
OBJECTIVE:
This study aims to compare the mechanical energy absorptions in lower limb joints in different initial foot contact strategies.
METHODS:
Nineteen young subjects walked down on instrumented stairs with two different strategies, i.e., forefoot and rearfoot strike. Power and energy at lower limb joints during stance phase were compared between strategies.
RESULTS:
Lower limb joints absorbed 73
CONCLUSION:
The results suggest that a leg absorbs most of the potential energy while descending stairs irrespective of the walking strategies and that any reduction of energy absorption at one joint is compensated by other joints. Greater energy absorption at the knee joint compared to the other joints suggests high burden of knee joint muscles and connective tissues during stair-descent, which is even more significant for the rearfoot strike strategy.
1.Introduction
Walking is one of the essential activities of human living, and it requires complex coordination of multiple muscles, tendons, and ligaments [1, 2]. Mechanical energy flows manipulated by the coordination of multiple joints may provide a profound understanding of the walking mechanisms [3]. For example, the impact of the leading leg on the ground dissipates (absorbs) mechanical energy and the trailing leg generates mechanical power during double stance phase of level walking [4, 5]. In this process, the total joint moment may cause energy to be generated, absorbed, and transferred between body segments [6, 7, 8, 9]. One of the major techniques for the investigation of the mechanical energy flows include the joint power analysis [8].
In the literature, many studies reported the mechanical energy flows during walking on level surface [3, 10] and inclined ramp [11]. Also reported was the reduction in energy cost by using spring-like ankle foot orthoses [12]. However, there has been no report on the mechanical energy flows while descending stairs, in the best of our knowledge. Stair descent is a common but one of the most hazardous activities of daily life [13] which is characterized by the absorption of the potential energy caused by the lowering of center of mass [14]. Moreover, stair descent was known to induce significantly greater knee joint moment (associated with knee joint diseases) compared to level walking [14] and also to stair ascent [15]. In this respect, attempts were made to find a better foot contact strategy to reduce joint loads during stair descent at the ankle [15] and the knee [16]. However, the coordination of multiple joints was not investigated so that the energy absorption mechanisms in different foot contact strategies are unknown. The results that forefoot strike (FFS) resulted in less knee joint moments [16] but greater ankle joint moments and forces [17] compared to those of rearfoot strike (RFS) support the possibility of multiple joints’ coordination in energy absorption.
Therefore, this study aims to investigate the energy absorption mechanisms in different foot contact strategies while descending stairs. We hypothesized that 1) the total amount of absorbed energy in lower limb joints would be similar in both strategies and 2) any reduction/increase in energy absorption at one joint would be compensated by the other joints.
2.Materials and methods
2.1Experiment
Nineteen young men (age: 23.4
All subjects provided informed consents and the experimental procedures were in accordance with the guidelines and regulations of the Institutional Bioethics Committee (HR-174) of Konkuk University Korea.
2.2Data analysis and statistics
Three dimensional joint power normalized by subject’s body mass were calculated at the ankle, knee and hip joints by using NEXUS2.0 (Vicon, UK) Net joint mechanical energy was calculated by the time integration of the joint mechanical power.
Figure 1.
Potential energy loss during one step descent and single leg energy absorption during stance.
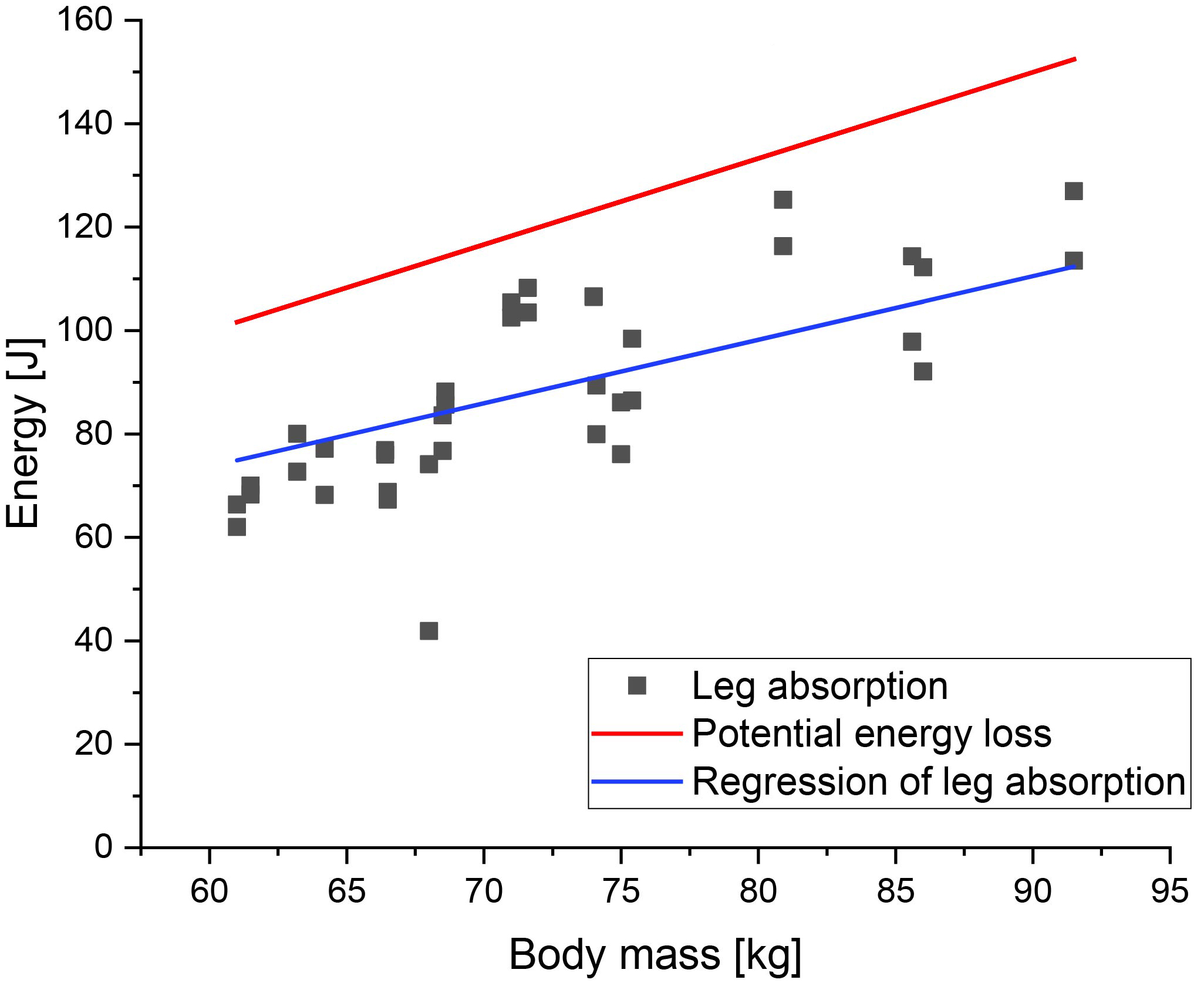
First, we investigated how much of the potential energy loss is absorbed by the lower limb joints. That is, the net energy absorption of single leg was compared with the potential energy which was lost in the process of descending one step. The potential energy loss of the body while descending one step (Eq. (1)) was calculated by the multiplication of the body weight and the rise height of one step (0.17 m). Assuming that two legs absorb equal amount of energy during one stride (two steps) of stair descent, one leg should absorb the potential energy loss of one step descent. Single leg in the stance period works as the leading leg in the earlier phase and also trailing leg in the later phase. Total energy absorption of single leg joints (Eq. (2.2)) was calculated by the summation of net energy in ankle, knee, and hip joints during stance period and its linear regression was derived with respect to the body mass. Any difference in mechanical energy absorption in single leg during stance period was investigated between foot contact strategies.
The overall distribution of leg joint energy to three joints was compared between strategies by using the ratio (Eq. (3)) of each joint’s net energy with respect to the total absorbed energy of the single leg joints. To understand the energy absorption in different phases of stair descent, joint energy in each of three phases of stair descent, i.e., 1
(1)
(2)
(3)
All comparisons of variables (single leg energy, joint absorption ratio, phase absorption) between strategies were performed by Wilcoxon signed-rank test, because some of the variables did not pass the normality test. All statistical analyses were performed by using SPSS ver. 24 for Windows (IBM Corp., Armonk, NY, USA).
3.Results
3.1Potential energy loss and single leg energy absorption
Figure 1 shows the potential energy loss and the energy absorption in single leg joints with respect to the body mass. Regression of single leg joint energy with respect to the body mass was significant, which explained 60% of the variability (R-square) in single leg joint energy. The single leg joints absorbed 73
Table 1
Comparison of joint absorption ratio [%] between strategies
Joint | Foot contact strategy | Difference | |||
---|---|---|---|---|---|
RFS | FFS | ||||
Ankle | 10.8 |
| 31.2 | *** | |
Knee | 90.9 |
| 74.9 | *** | 15.4 |
Hip |
| *** | 5.51 |
Positive value represents absorbed energy and vice versa. ***
Figure 2.
Ratio of each joint energy to the total leg joint energy.
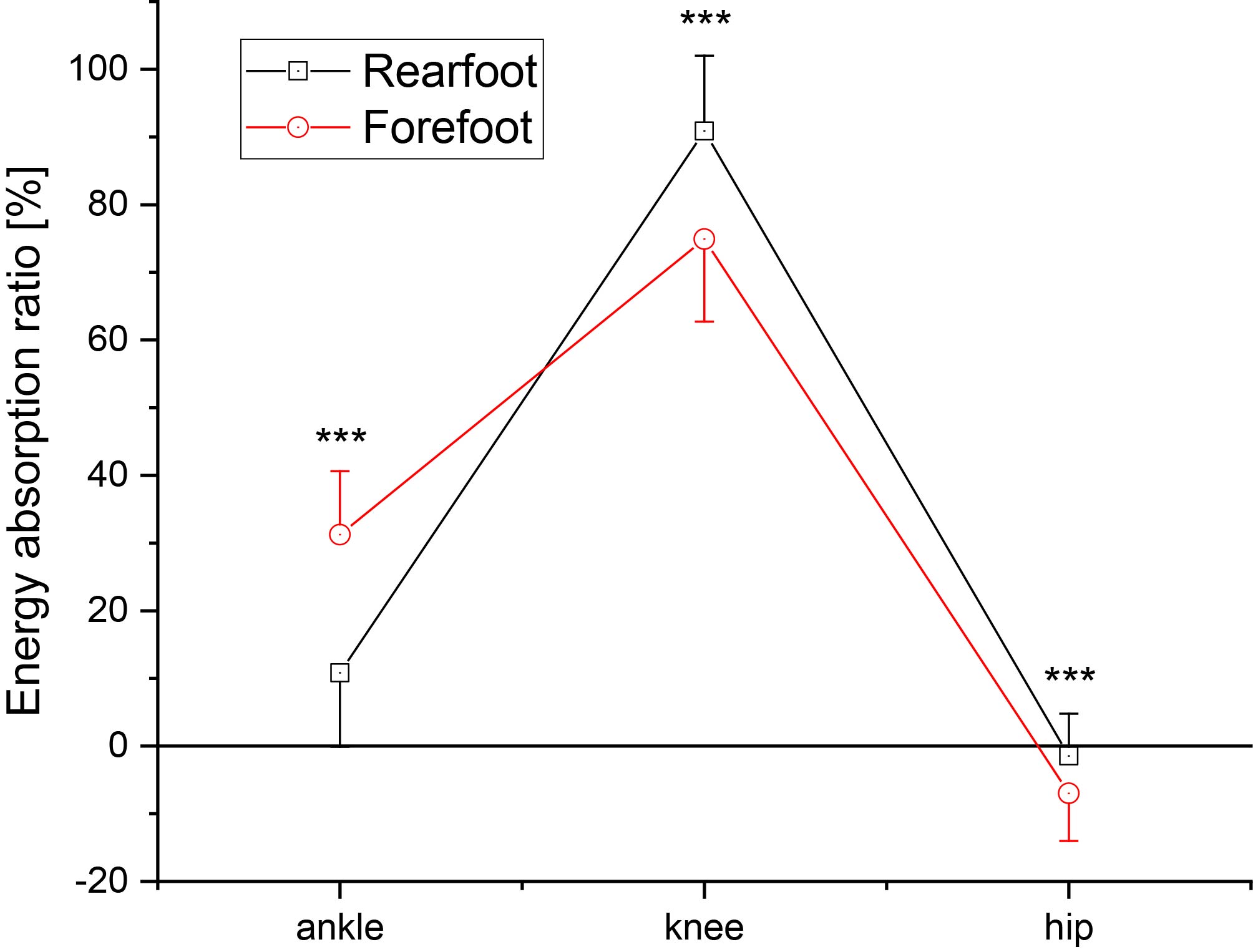
3.2The ratio of each joint energy to single leg total joint energy
Figure 2 and Table 1 compare the percentage of each joint energy to the total leg joint energy between foot contact strategies. At the ankle joint, energy absorption in FFS was 3 times greater than RFS (
3.3Joint absorption energy
Figure 3 shows the joint power trajectories. Table 2 shows absorbed energy and its strategy difference in three phases of stance. Figure 4 shows the detailed phase classification during stance including both ipsilateral and contralateral legs. The ipsilateral leg in the stance period works as the leading leg in the earlier double stance phase (0%–25.6%) and also trailing leg in the later double stance phase (77%–100%)
The only case that absorbed energy was greater in FFS than RFS was at the ankle joint in the 1
Table 2
Phase-specific energy absorption in each joint
Joint | Phase | Energy absorption in single joint [J] | ||||
RFS | FFS | Difference | ||||
Ankle | 1st | 1.57 |
| 22.4 | *** | |
2nd | 10.1 |
| 7.23 | ** | 2.9 | |
3rd | 0.573 | |||||
Knee | 1st | 3.22 |
| 0.51 | * | 2.7 |
2nd | 15.1 |
| 2.67 | *** | 12.4 | |
3rd | 61.2 | 63.4 | 0.687 | |||
Hip | 1st | 1.16 | 1.62 | 0.355 | ||
2nd | 1.34 |
| 0.36 | * | 1.0 | |
3rd |
| *** | 4.6 |
Positive value represents absorbed energy and vice versa. *
Figure 3.
Joint power during stance period of the stair decent averaged for all subjects (a. ankle, b. knee, c. hip). R and F indicate a significantly greater energy absorption for the rearfoot- and forefoot strike, respectively. Numbers in parentheses indicates the amount of difference in absorbed energy [J]
![Joint power during stance period of the stair decent averaged for all subjects (a. ankle, b. knee, c. hip). R and F indicate a significantly greater energy absorption for the rearfoot- and forefoot strike, respectively. Numbers in parentheses indicates the amount of difference in absorbed energy [J] *p< 0.05, p**< 0.01, p***< 0.001.](https://ip.ios.semcs.net:443/media/thc/2021/29-S1/thc-29-S1-thc218041/thc-29-thc218041-g003.jpg)
Figure 4.
Stance phase in stair descent.
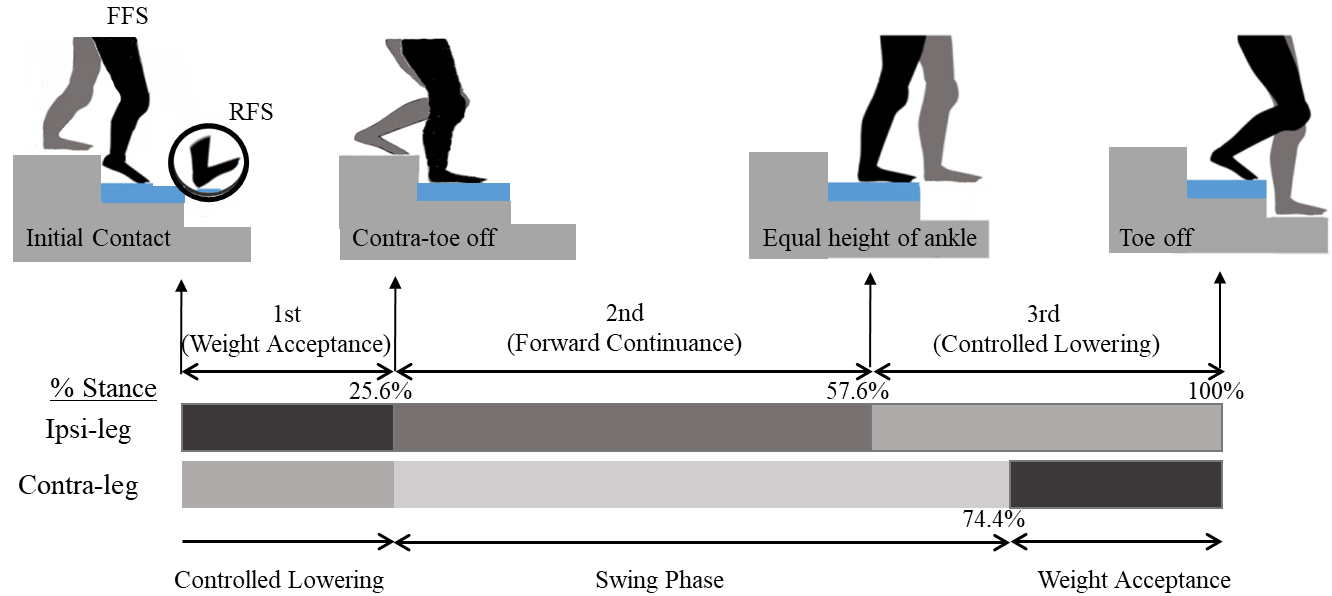
The major compensations were performed by the knee joint in the 2nd phase (12.4J) and by the hip joint in the 3rd phase (4.6J). Minor compensations were performed by the ankle joint itself in the 2nd phase (2.9J), by the knee joint in the 1st phase (2.7J), and by the hip joint in the 2nd phase (1.0J).
4.Discussion and conclusion
4.1Leg energy absorption of potential energy loss
Because the human body mass goes down the riseheight of stairs per one step of descending, the potential energy loss of human body should be proportional to the body mass and need to be absorbed by some body parts irrespective of walking strategies. Figure 1 showed that the major site of absorption is lower limb joints during stance phase (73% of the potential energy) and that the absorbed energy is proportional to body mass. Moreover, the total amount of absorbed energy was not different between strategies, which is in agreement to our hypothesis no. 1. The results provide a rationale for the comparison of energy absorption between strategies.
4.2Joint energy absorption in different foot contact strategies
Figure 2 and Table 1 showed that the energy absorption is greater in the order of knee, ankle, and hip joints irrespective of the walking strategies, which suggests high burden of knee joint and its surrounding muscles and connective tissues during stair descent. In contrast, the generation of energy was significant during controlled lowering (3rd) phase in ankle and hip joint (Fig. 3), which would be associated with the ankle plantarflexion and hip flexion to push-off the ground and lift the ipsilateral leg for the preparation of subsequent swing. It is noted that net energy at the hip joint was generation instead of absorption (Table 1), suggesting that the major role of hip joint during stance phase is concentric hip flexion to prepare for swing.
The second hypothesis was that a reduction/increase in absorbed energy at any joint would be compensated by the other joints, which is proved by Fig. 2 and Table 1. That is, smaller absorption at the ankle joint (in case of RFS) was compensated by greater absorption at the knee joint and less generation at the hip joint. Similarly, greater absorption at the ankle joint (in case of FFS) was compensated by the smaller absorption at the knee joint and greater generation at the hip joint.
Figure 3 and Table 2 showed the detailed absorption profile in different phase of descent. Absorption in RFS was 21J smaller than that of FFS only at the ankle joint in the 1st (WA) phase. The major compensation of this shortage was done by the knee in the 2nd (FC) phase and by the hip joint in the 3rd (CL) phase, which indicates that the early difference at the ankle joint was nullified by delayed compensation in the knee and hip joints. The compensation may be associated with earlier onset of the energy absorption at the knee and later onset of hip concentric flexion (generation) in RFS, resulting in the elongated supporting of body weight on the ipsilateral leg.
4.3Further studies
The energy absorbed by lower limb joints during stair descent was 73% of the potential energy loss. The rest 27% of the potential energy may be handled by linear mechanical work in lower limb segments [3]. Therefore, for the better understanding of the energy absorption mechanism in different walking strategies, it is desirable to consider flow of linear mechanical energy as well, which requires further investigations.
We considered the energy absorption of single leg during stance period where the leg works as both the leading and trailing leg. Further investigations with two force plates for the measurement of ground reaction forces of both legs would provide better understanding of the energy absorption mechanism in the leading vs. trailing legs.
Acknowledgments
This paper was written as part of the Konkuk University’s research support program for its faculty on sabbatical leave in 2020. This research was supported by the Basic Science Research Program through the National Research Foundation of Korea (NRF) funded by the Ministry of Education (2020R1A6A3A01099516).
Conflict of interest
None to report.
References
[1] | Ferris DP, Louie M, Farley CT. Running in the real world: adjusting leg stiffness for different surfaces. Proceedings of the Royal Society of London.Series B: Biological Sciences. (1998) ; 265: (1400): 989-994. |
[2] | Kuo AD. The six determinants of gait and the inverted pendulum analogy: A dynamic walking perspective. Human movement science. (2007) ; 26: (4): 617-656. |
[3] | Umberger BR, Augsburger S, Resig J, Oeffinger D, Shapiro R, Tylkowski C. Generation, absorption, and transfer of mechanical energy during walking in children. Med Eng Phys. (2013) ; 35: (5): 644-651. |
[4] | Donelan JM, Kram R, Kuo AD. Simultaneous positive and negative external mechanical work in human walking. J Biomech. (2002) ; 35: (1): 117-124. |
[5] | Adamczyk PG, Kuo AD. Redirection of center-of-mass velocity during the step-to-step transition of human walking. J Exp Biol. (2009) ; 212: (16): 2668-2678. |
[6] | Caldwell GE, Forrester LW. Estimates of mechanical work and energy transfers: demonstration of a rigid body power model of the recovery leg in gait. Med Sci Sports Exerc. (1992) ; 24: (12): 1396-1412. |
[7] | Fregly BJ, Zajac FE. A state-space analysis of mechanical energy generation, absorption, and transfer during pedaling. J Biomech. (1996) ; 29: (1): 81-90. |
[8] | Gordon D, Robertson E, Winter DA. Mechanical energy generation, absorption and transfer amongst segments during walking. J Biomech. (1980) ; 13: (10): 845-854. |
[9] | Siegel KL, Kepple TM, Stanhope SJ. Joint moment control of mechanical energy flow during normal gait. Gait Posture. (2004) ; 19: (1): 69-75. |
[10] | Jin L, Hahn ME. Modulation of lower extremity joint stiffness, work and power at different walking and running speeds. Human Movement Science. (2018) ; 58: : 1-9. |
[11] | Franz JR, Lyddon NE, Kram R. Mechanical work performed by the individual legs during uphill and downhill walking. J Biomech. (2012) ; 45: (2): 257-262. |
[12] | Bregman D, Harlaar J, Meskers C, De Groot V. Spring-like Ankle Foot Orthoses reduce the energy cost of walking by taking over ankle work. Gait Posture. (2012) ; 35: (1): 148-153. |
[13] | Larsen AH, Puggaard L, Hämäläinen U, Aagaard P. Comparison of ground reaction forces and antagonist muscle coactivation during stair walking with ageing. Journal of Electromyography and Kinesiology. (2008) ; 18: (4): 568-580. |
[14] | Riener R, Rabuffetti M, Frigo C. Stair ascent and descent at different inclinations. Gait Posture. (2002) Feb; 15: (1): 32-44. |
[15] | Silverman AK, Neptune RR, Sinitski EH, Wilken JM. Whole-body angular momentum during stair ascent and descent. Gait Posture. (2014) Apr; 39: (4): 1109-1114. |
[16] | Jeon H, Choi E, Heo J, Eom G. Influence of the initial foot contact strategy on knee joint moments during stair and ramp descent. Scientific Reports. (2020) ; 10: (1): 1-8. |
[17] | Jeon HM, Choi EB, Heo JH, Eom GM. Ankle joint moments in different foot strike methods during stair descent. Journal of Mechanics in Medicine and Biology. (2019) ; 19: (7): 1940031. |
[18] | Song YS, Ha S, Hsu H, Ting LH, Liu CK. Stair negotiation made easier using novel interactive energy-recycling assistive stairs. PLoS One. (2017) Jul 12; 12: (7): e0179637. |