Characteristics of physiological data during asymmetric gait of healthy subjects using a two-belt treadmill
Abstract
BACKGROUND:
Mobile rehabilitation systems for patients with gait disorder are being developed. Safety functions to prevent patients from falling are considered during product development; however, few studies have been conducted on systems that have been prevalidated for healthy adults prior to application to patients.
OBJECTIVE:
This study analyzed the characteristics of lower extremity muscles and foot pressure in healthy adults during unbalanced walking with differences in the speed of left and right speed using a two-belt treadmill.
METHODS:
Twenty subjects performed gait motions with a difference in the weight support conditions (0% and 30%) and the left and right lower limb speeds (1–3 km/h). Each subject’s muscular activities and foot pressure signals were collected. The gait patterns of the faster side exhibit similar characteristics to the paralyzed leg, and the slower side is similar to the non-paralyzed leg.
RESULTS:
Weight-supporting healthy subjects showed asymmetric gait patterns, similar to hemiplegic patients, because of the difference in the speed of the left and right side.
CONCLUSIONS:
The quantitative results can be used to develop a training protocol for two-belt treadmills with differently controlled left and right speeds for gait rehabilitation in hemiplegic patients.
1.Introduction
Many people suffer from paralysis due to various reasons such as stroke or spinal cord injury. Patients with hemiplegia require many types of repetitive rehabilitation training to perform daily activities. These therapies can be physically demanding on the therapist. According to Bork et al. [1], physical therapists often have muscular skeletal diseases. Especially gait rehabilitation, which is nearly the last step of rehabilitation, requires considerable labor. To reduce the labors of the physical therapist, many cutting-edge technologies have attempted to use a gait rehabilitation training system. Many gait rehabilitation systems have been developed for paralysis patients using robots. These have numerous advantages such as cost effectiveness and motivating patients [2, 3, 4]. The robot gait rehabilitation systems must help support patients’ body weight to help with balance and prevent falls as well as strengthen muscles for walking [5]. Robot gait rehabilitation systems can be roughly divided into four sections for different symptoms: treadmill base, foot plate base, stationery robotic base, and mobile robotic base [6]. Among other applications, mobile robotic base gait rehabilitation systems are used for both the nearly last step of gait rehabilitation and for daily life assistance by inducing natural walking [7, 8, 9]. To function independently in everyday life, safety is the most important issue. For safety reasons, healthy people without gait disability are often used for evaluating systems before allowing patients to use them. However, these experiments are insufficient because there are many physical differences between healthy people and paralysis patients. It is important to reduce this testing gap for developing and growing systems.
Wentink et al. [10] attempted to induce hemiplegic gait in healthy people by restraint motion of joint angle. Choi and Park [11] used heavy clothes to imitate abnormal gait. There is a time difference between the paralyzed and non-paralyzed legs in the stance and swing phases of hemiplegic gait, which is rarely observed in healthy subjects. In addition, measurements of the EMG on skin like surface is difficult because the subjects wore some clothes for restraints. To develop a two-belt treadmill for gait rehabilitation protocol in hemiplegic patients, studies on the biomechanical analysis of asymmetrical gait in healthy adults are insufficient.
This study aimed to analyze the characteristics of lower extremity muscle and foot pressure in healthy adults for unbalanced walking with left and right speed difference using a two-belt treadmill. We assumed that an asymmetric gait can be imitated by a difference in the left and right side velocities of two-belt treadmill.
2.Experimental method
2.1Participants
Twenty healthy young male adults (25.35
2.2Procedures
A two-belt treadmill with different velocities for the right and left leg was used to induce abnormal gait. The experiments were divided into six cases, as summarized in Table 1. The body weight support rates were given at 0% and 30% of each subject’s weight. Each case was conducted in 3 min.
Table 1
Experimental cases of body weight support rate and left limb and right limb speed using two-belt treadmill
Case | Left velocity | Right velocity | Body weight support rate |
---|---|---|---|
1 | 2 km/h | 1 km/h | 0% |
2 | 3 km/h | 1 km/h | 0% |
3 | 3 km/h | 2 km/h | 0% |
4 | 2 km/h | 1 km/h | 30% |
5 | 3 km/h | 1 km/h | 30% |
6 | 3 km/h | 2 km/h | 30% |
2.3Two-belt type treadmill and harness
The GAT SYSTEM Pro treadmill (Cybermedia Inc., Korea) was used to control the velocity separately on the left and right side. The velocity can be adjusted in increments of 0.1 km/h through a display embedded in the treadmill. The range of the velocity was 0–8 km/h. The treadmill comprised a BLDC (Brushless direct current) motor (1.5 kW) and BLDC driver (750 W). The velocity feedback was used for precise control even at low velocities. The bodyweight support was a harness system (BIODEX, USA) that utilized a load cell to control bodyweight support rate by kg.
2.4Measurements
The foot pressure and surface EMG data were collected for evaluation during asymmetrical walking. We used the data after 1 min to reduce interference.
2.4.1Foot pressure
The foot pressure was measured with the Pedar-X system (Novel Gmbh, Germany). The 99 sensors embedded in the insole collected the foot pressure data, and Bluetooth communication was used. The raw data were divided according to nine sections of foot (Hallux, Toe, 1st Metatarsal, 2nd Metatarsal, 3rd Metatarsal, Medial Arch, Lateral Arch, Medial calcaneus, and Lateral calcaneus) and then roughly represented in two cases (1st: medal and lateral, 2nd: Anterior Posterior). Foot pressure data for the nine sections were analyzed by dividing the anterior, posterior, medial, and lateral side of sole.
2.4.2Surface EMG
The surface EMG was measured with a wireless type sensor, Noraxon Telemyo 2400T (NORAXON Inc., Scottsdale, AZ, USA). The bandpass filter (cut-off frequency: 10–250 Hz) was used for EMG signals, and the sampling rate was 1000 Hz. The EMG data were normalized with root mean square (RMS). The EMG data of each velocity with 30% bodyweight support were normalized based on 0% bodyweight support by each subject. The EMG data were measured in ten locations of the left and right lower limb muscles (Rectus femoris, RF; Biceps femoris, BF; Vastus Lateralis, VL; Tibialis anterior, TA; Lateral gastrocnemius, Lat.Gas).
2.5Statistical analysis
The statistical analysis of foot pressure and EMG data was performed with SPSS 18.0ver. All outcome variables were confirmed to be normally distributed by the Kolmogorov-Smirnov test. The paired t-test used for comparing foot pressure and EMG between 0% and 30% of bodyweight supports. To determine the correlation between each muscle and each section of foot pressure, Pearson’s correlational coefficient was used. Every significance level (
3.Results
3.1Foot pressure results
The total foot pressure (Table 2, Fig. 1) was higher on the faster side than on the slower side. When comparing pressure in one foot, the anterior was higher than posterior section on the faster side. In contrast, the posterior was higher than the anterior section on the slower side. Both sides of the posterior section were statistically significant (
Table 2
Foot pressure results of each case
LT medial | LT lateral | RT medial | RT lateral | LT anterior | LT posterior | RT anterior | RT posterior | |
2 km–1 km | ||||||||
30% | ||||||||
Average | 47.26 | 38.28 | 236.09 | 35.31 | 47.29 | 36.00 | 28.06 | 45.05 |
SD | 6.97 | 4.55 | 4.77 | 5.55 | 8.48 | 5.25 | 5.61 | 6.03 |
0% | ||||||||
Average | 48.34 | 46.26 | 41.74 | 41.73 | 50.04 | 43.61 | 35.12 | 50.01 |
SD | 5.07 | 7.18 | 6.18 | 6.28 | 9.64 | 4.54 | 8.57 | 6.23 |
3 km–2 km | ||||||||
30% | ||||||||
Average | 50.39 | 43.99 | 41.83 | 42.34 | 53.53 | 38.46 | 39.39 | 45.52 |
SD | 6.26 | 5.04 | 5.47 | 5.98 | 6.73 | 5.29 | 7.99 | 6.15 |
0% | ||||||||
Average | 52.96 | 51.92 | 46.66 | 46.68 | 57.48 | 46.00 | 43.79 | 50.28 |
SD | 6.79 | 7.40 | 7.83 | 7.25 | 8.31 | 4.27 | 11.04 | 6.90 |
3 km–1 km | ||||||||
30% | ||||||||
Average | 50.50 | 43.90 | 38.08 | 35.98 | 55.38 | 36.16 | 28.90 | 46.92 |
SD | 7.36 | 5.57 | 4.36 | 6.91 | 7.88 | 5.84 | 6.47 | 6.67 |
0% | ||||||||
Average | 54.46 | 51.42 | 42.47 | 41.47 | 59.89 | 43.87 | 33.93 | 51.91 |
SD | 6.20 | 7.59 | 6.04 | 6.76 | 8.88 | 5.52 | 9.11 | 6.49 |
1 km–2 km | ||||||||
30% | ||||||||
Average | 39.08 | 30.18 | 45.40 | 41.11 | 26.98 | 43.09 | 46.53 | 38.63 |
SD | 4.60 | 5.09 | 6.29 | 5.74 | 5.85 | 4.65 | 8.10 | 5.83 |
0% | ||||||||
Average | 42.82 | 40.12 | 47.67 | 45.82 | 35.12 | 49.07 | 50.20 | 42.19 |
SD | 4.67 | 7.76 | 6.17 | 6.82 | 7.57 | 5.58 | 8.62 | 6.61 |
1 km–3 km | ||||||||
30% | ||||||||
Average | 39.53 | 32.18 | 49.66 | 46.81 | 27.26 | 45.68 | 56.07 | 38.08 |
SD | 5.35 | 5.52 | 7.65 | 6.09 | 5.47 | 5.24 | 9.51 | 5.81 |
0% | ||||||||
Average | 43.13 | 40.15 | 53.48 | 50.01 | 33.81 | 51.06 | 59.15 | 42.06 |
SD | 4.78 | 7.73 | 7.39 | 7.16 | 6.65 | 5.27 | 9.34 | 5.61 |
2 km–3 km | ||||||||
30% | ||||||||
Average | 43.75 | 37.10 | 47.05 | 48.34 | 38.03 | 42.59 | 53.96 | 40.03 |
SD | 6.18 | 5.67 | 6.11 | 5.70 | 6.30 | 6.90 | 7.67 | 6.71 |
0% | ||||||||
Average | 47.56 | 45.07 | 52.12 | 51.39 | 42.39 | 50.91 | 57.12 | 44.95 |
SD | 5.99 | 8.15 | 7.16 | 6.94 | 8.06 | 5.16 | 10.06 | 5.72 |
Figure 1.
Foot pressure results.
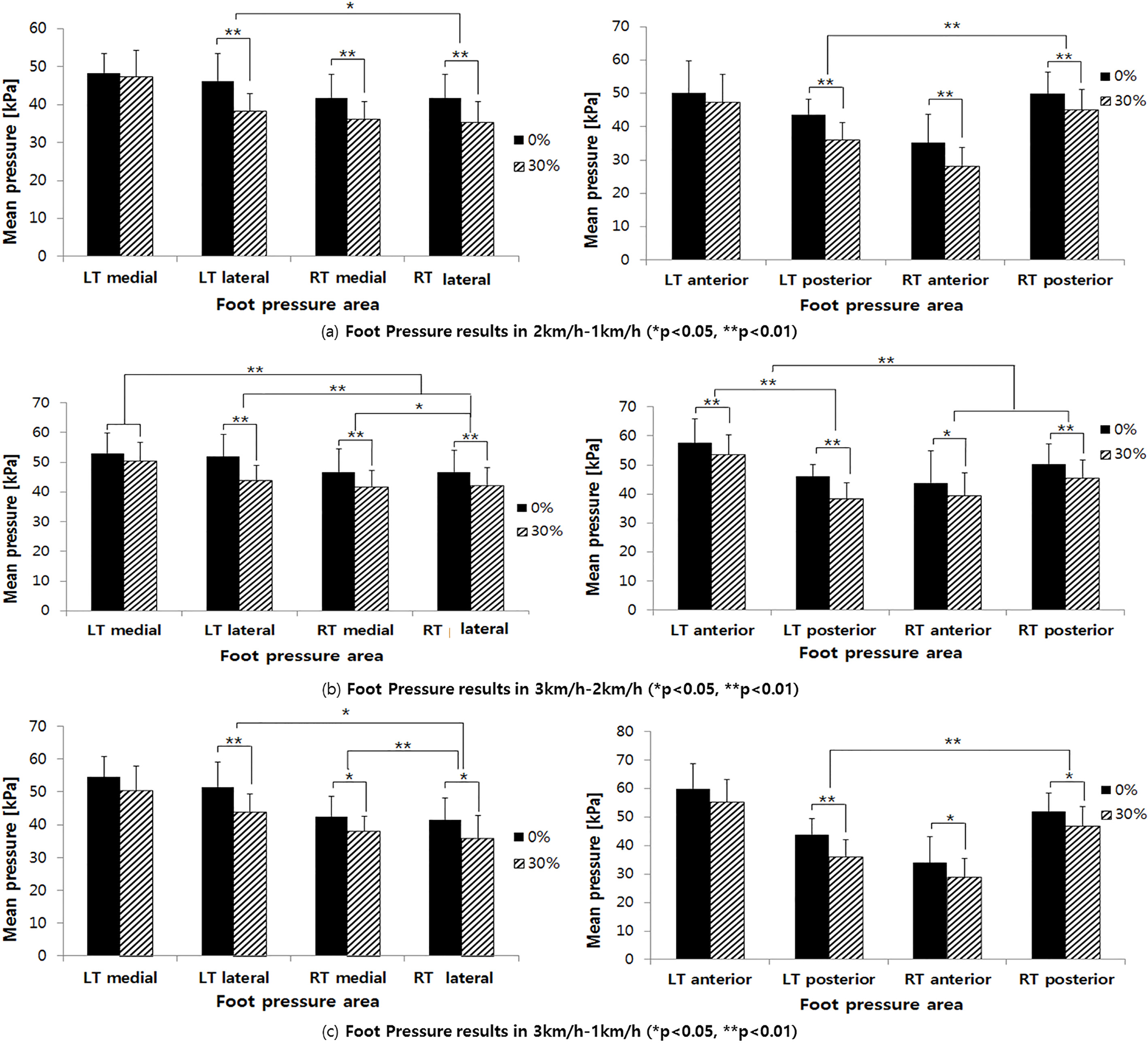
3.2 Surface EMG results
The EMG results (Table 3, Fig. 2) showed muscle activities of normalized EMG data of 30% body weight support in each muscle based on 0% body weight support. The RF and Lat.Gas muscles were more activated in the faster side than the slower side. In contrast, the BF muscle was more activated in the faster side. The TA muscle of the faster side and VL muscle of the slower side were correlated (
Table 3
EMG results of each case
LT RF | LT VLO | LT TA | LT BF | LT Lat. Gas | RT RF | RT VLO | RT TA | RT BF | RT Lat. Gas | |
---|---|---|---|---|---|---|---|---|---|---|
2 km–1 km | ||||||||||
30% | ||||||||||
Average | 1.65 | 0.75 | 0.39 | 0.43 | 1.28 | 1.26 | 0.69 | 0.39 | 0.73 | 1.12 |
SD | 0.94 | 0.33 | 0.08 | 0.27 | 0.94 | 0.93 | 0.33 | 0.09 | 0.51 | 0.99 |
3 km–2 km | ||||||||||
30% | ||||||||||
Average | 1.35 | 0.70 | 0.38 | 0.38 | 1.06 | 1.06 | 0.59 | 0.40 | 0.59 | 0.94 |
SD | 0.79 | 0.32 | 0.08 | 0.18 | 0.74 | 0.73 | 0.27 | 0.10 | 0.36 | 0.71 |
3 km–1 km | ||||||||||
30% | ||||||||||
Average | 1.45 | 0.72 | 0.33 | 0.32 | 1.08 | 1.06 | 0.61 | 0.37 | 0.69 | 0.97 |
SD | 0.86 | 0.35 | 0.07 | 0.15 | 0.75 | 0.74 | 0.28 | 0.10 | 0.52 | 0.88 |
1 km–2 km | ||||||||||
30% | ||||||||||
Average | 1.31 | 0.71 | 0.39 | 0.68 | 1.04 | 1.41 | 0.62 | 0.37 | 0.39 | 1.19 |
SD | 0.79 | 0.28 | 0.13 | 0.57 | 0.89 | 0.76 | 0.30 | 0.08 | 0.22 | 0.82 |
1 km–3 km | ||||||||||
30% | ||||||||||
Average | 1.25 | 0.71 | 0.40 | 0.75 | 1.00 | 1.40 | 0.70 | 0.35 | 0.36 | 1.20 |
SD | 0.81 | 0.26 | 0.13 | 0.44 | 0.84 | 0.81 | 0.38 | 0.11 | 0.16 | 0.87 |
2 km–3 km | ||||||||||
30% | ||||||||||
Average | 2.21 | 1.17 | 0.71 | 1.12 | 2.03 | 2.21 | 1.05 | 0.62 | 0.69 | 2.01 |
SD | 1.51 | 0.49 | 0.18 | 0.72 | 1.75 | 1.56 | 0.54 | 0.15 | 0.33 | 1.46 |
Figure 2.
EMG results when the left side is faster than the right side.
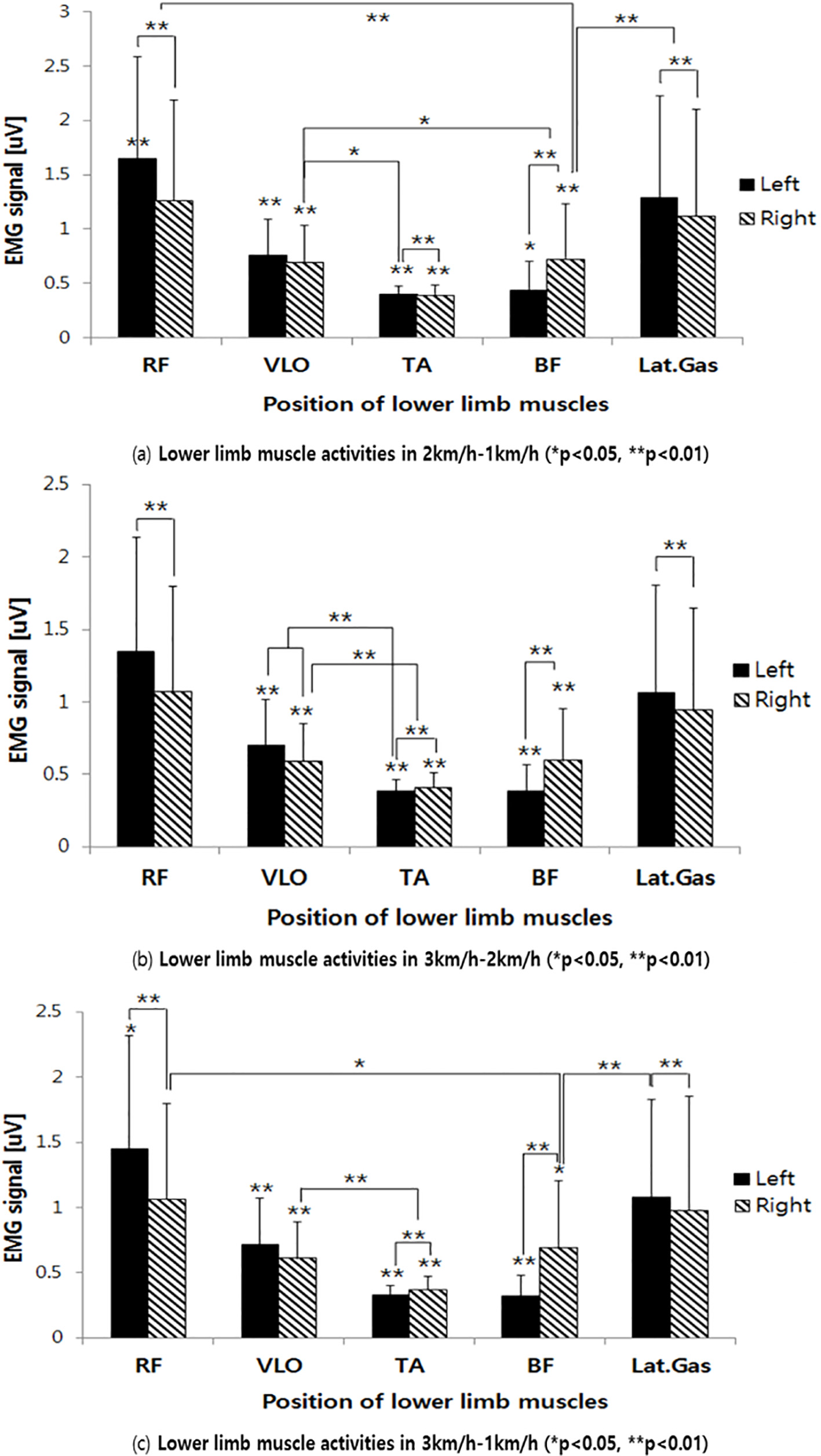
4.Discussion
Perry [12] and Hesse et al. [13] reported that hemiplegic patients have shorter stance phase and long swing phase on the sides of legs in paralysis. However, in non-paralysis, there are longer stance phase and short swing phase on the sides of legs to compensate the abnormal walking. Adjusting the velocity makes it possible to imitate the asymmetrical gait characteristics in a healthy subject. Because of the velocity, the faster side of the leg has a short stance phase and a long swing phase, which is similar to the gait characteristics in paralysis. However, there are longer stance phase and shorter swing phase in the slower side than the faster side. This is exact opposite to that observed in the gait of hemiplegic patients, who spend a longer time on the paralyzed leg [14, 15].
The foot pressure was concentrated more on the rearfoot than the forefoot in the slower side. This means that a pre-swing phase rarely occurs in the slower side of the leg. This is similar to the non-paralysis side of legs in hemiplegic patients [14, 15]. On the contrary, the faster side of foot pressure indicated exactly opposite results to the slower side. The concentration on the rearfoot on the faster side is caused by the toe-off phase; however, there is a difference in the hemiplegic patients’ gait patterns on the paralysis side [14, 15]. Because of the patients’ asymmetric patterns, the toe-off phase occurs. The asymmetry between the anterior and posterior foot pressures is large when the velocity gap is large. In other words, we expect that the degree of paralysis could be set by changing the velocity gap between two belts. According to the bodyweight support, the pressure on the lateral section of the foot statically decreased in both faster and slower sides. The decrease of pressure on the medial side of the foot pressure on slower side was also affected. The pressures on the anterior and posterior sides of the foot significantly decreased on both sides of the foot with bodyweight support, except on the anterior section of the faster side leg. The dissymmetry existed even with bodyweight support.
The EMG results show that the RF and Lat.Gas muscles were more activated on the faster side than the slower side. This is caused by the toe-off in pre-swing phase [15, 16, 17]. However, the TA and BF muscles were more activated on the slower side than the faster side because the slower side required a longer stance phase.
5.Conclusion
In this study we attempted to imitate asymmetrical gait patterns using two-belt type treadmill to aid in developing mobile a gait rehabilitation system with healthy subjects before using the system with patients.
We hypothesized that hemiplegic gait can be imitated using a speed difference between the left and right sides and verified the hypothesis through the experiments. We observed that the gait pattern of the slower side was similar to that of the non-paralyzed leg, and the gait pattern of the faster side to the paralyzed leg in hemiplegic patients. However, the gait pattern of the faster side indicated differences from the patients, because healthy people employ toe-off in pre-swing phase. In addition, we found that that the greater the speed difference, the greater the asymmetry in the foot and muscles action. Therefore, we can control the degree of paralysis by controlling this difference.
We also performed experiments with bodyweight support, which is a basic function of a gait rehabilitation system. Despite the bodyweight support, asymmetry was still present in both pressure concentration and muscles.
Despite its limitations, the proposed method improves the process of verifying novel gait rehabilitation systems before being used by hemiplegic patients. In the future, we will apply this method to a mobile robotic system, and we expect that we can set other asymmetrical gait patterns combined with velocity control and bodyweight support rate between the left and right sides. The quantitative results of this study can be used to develop a training protocol for two-belt treadmills with differently controlled speeds in the left and right sides for gait rehabilitation for hemiplegic patients.
Acknowledgments
This paper is based on work supported by the Basic Science Research Program through the National Foundation of Korea (NRF) funded by the Ministry of Education (no. NRF-2017R1A2B4011239).
Conflict of interest
None to report.
References
[1] | Bork ME, Cook TM, Rosecrance JC, Engelhardt KA, Thomason MEJ, Wauford IJ, et al. Work-related musculoskeletal disorders among physical therapists. Phys Ther. (1996) ; 76: (8): 827-835. |
[2] | Lum PS, Burgar CG, Shor PC, Majmundar M, Van der Loos M. Robot-assisted movement training compared with conventional therapy techniques for the rehabilitation of upper-limb motor function after stroke. Arch Phys Med Rehab. (2002) ; 83: (7): 952-959. |
[3] | Banala SK, Kim SH, Agrawal SK, Scholz JP. Robot assisted gait training with active leg exoskeleton (ALEX). IEEE Trans Neural Syst Rehabil Eng. (2009) ; 17: (1): 2-8. |
[4] | Patritti B, Romaguera F, Deming L, Mirelman A, Pelliccio M, Nimec D, et al. Enhancement and retention of locomotor function in children with cerebral palsy after robotic gait training. Gait Posture. (2009) ; 30: : S9-S10. |
[5] | Klarner T, Chan HK, Wakeling JM, Lam T. Patterns of muscle coordination vary with stride frequency during weight assisted treadmill walking. Gait Posture. (2010) ; 31: (3): 360-365. |
[6] | Schmidt H, Werner C, Bernhardt R, Hesse S, Krüger J. Gait rehabilitation machines based on programmable footplates. J Neuroeng Rehab. (2007) ; 4: (1): 1. |
[7] | Veneman JF, Kruidhof R, Hekman EE, Ekkelenkamp R, Van Asseldonk EH, Van Der Kooij H. Design and evaluation of the LOPES exoskeleton robot for interactive gait rehabilitation. IEEE Trans Neural Sys Rehab Eng. (2007) ; 15: (3): 379-386. |
[8] | Strickland E. Good-bye, wheelchair. IEEE Spectrum. (2012) ; 49: (1): 30-32. |
[9] | Chen B, Ma H, Qin LY, Gao F, Chan KM, Law SW, Qin L, Liao WH. Recent developments and challenges of lower extremity exoskeletons. Journal of Orthopaedic Traslation. (2016) ; 5: 26-37. |
[10] | Wentink EC, Beijen SI, Hermens HJ, Rietman JS, Veltink PH. Intention detection of gait initiation using EMG and kinematic data. Gait Posture. (2013) ; 37: : 223-228. |
[11] | Choi SW, Park MH. Effect of Senior Simulation on Geriatric Unit Nurses’ Attitude and Job Satisfaction toward the Elderly. Kor Gerontol Soc. (2009) ; 29: (2): 513-527. |
[12] | Perry J. Gait analysis: Normal and pathological function. SLACK Inc., (1992) . |
[13] | Hesse S, Bertelt C, Jahnke MT, Schaffrin A, Baake P, Malezic M, et al. Treadmill training with partial body weight support as compared to physiotherapy in non-ambulatory hemiparetic patients. J Stroke. (1995) ; 26: : 76-981. |
[14] | Mauritz KH. Gait training in hemiplegia. Eur J Neurol. (2002) ; 9: (S1): 23-29. |
[15] | Yoon HW, Lee SY, Lee HM. The comparison of plantar foot pressure in normal side of normal people, affected side and less affected side of hemiplegic patients during stance phase. J Kor Soc Phy Med. (2009) ; 4: (2): 87-96. |
[16] | Perry J, Giovan P, Harris LJ, Montgomery J, Azaria M. The determinants of muscle action in the nemiparetic lower extremity (and hteir effect on the examination procedure). Clin Orthop. (1978) ; 131: : 71-89. |
[17] | Winsters TF, Gage JR, Hicks R. Gait patterns in spastic hemiplegia in children and young adults. J Bone Joint Surg. (1987) ; 69: (A3): 437-441. |