Quantitative body symmetry assessment during neurological examination
Abstract
BACKGROUND:
A lack of movement coordination characterized by the undershoot or overshoot of the intended location with the hand, arm, or leg is often found in individuals with multiple sclerosis (MS). Standardized as Finger-to-Nose (FNT) and The Heel-to-Shin (HST) tests are the most frequently used tests for qualitative examination of upper and lower body coordination. Inertial sensors facilitate in performing quantitative motion analysis and by estimating body symmetry more accurately assess coordination lesion and imbalance.
OBJECTIVES:
To assess the body symmetry of upper and lower limbs quantitatively, and to find the best body symmetry indices to discriminate MS from healthy individuals (CO).
METHODS:
28 MS patients and 23 CO participated in the study. Spatiotemporal parameters obtained from six Inertial Measurement Units (IMUs) were placed on the upper and lower extremities during FNT and HST tests. All data were analyzed using statistical methods in MATLAB.
RESULTS:
Asymmetry indices of temporal parameters showed a significant increase in upper body and lower body asymmetry of MS compared to CO. However, CO have a greater kinematic asymmetry compared to MS.
CONCLUSION:
Temporal parameters are the most sensitive to body asymmetry evaluation. However, range of motion is completely inappropriate if it is calculated for one movement cycle.
1.Introduction
Balance and coordination of movements depend on sensory and motor proper functioning. The brain receives and processes information about the body’s position in space, including visual, vestibular, proprioceptive, and tactile signals. These signals generate an appropriate motor response to maintain stability. Therefore, disruption of any of these factors can cause a person to lose balance and coordination of limbs in space [1, 2, 3, 4]. Dysmetria is the term that describes a lack of coordination of movement characterized by the undershoot or overshoot of the intended location with the hand, arm, leg, or eye. It is a type of ataxia. It can also include an inability to judge distance or scales. It is often found in individuals with multiple sclerosis (MS), amyotrophic lateral sclerosis, and persons who have suffered from tumors or strokes. The typical MS pattern is caused by demyelination of neural tissues. This leads to delay, and finally even to the interruption of the electrical signals transmitted by the nerves, which in turn causes more and more progressive symptoms and movement disturbances.
Typically, people with MS experience multiple lesions at different CNS locations that may affect the functional systems that are involved in walking and motor control [5, 6]. This can result in muscle weakness [7], fatigue [8, 9, 10, 11], reduced sensory sensitivity [12, 13], spasticity [14], and intentional tremor [15], which interfere with different motor skills. Impaired functions such as endurance, coordination, and balance have a negative impact on daily life activities [16, 17, 18]. Visual impairment is also quite common in people with MS, and the person’s response to environmental stimuli, control of body segments, and balance are also reduced. Depending on the brain injury, one side of the body is usually affected, resulting in an asymmetry of all the listed symptoms and locomotion between different parts of the body. The greater the asymmetry, the more it affects balance and coordination [19, 20]. Therefore, patients with MS often have a greater risk of falling [18, 21, 22], and use various support measures [23, 24]. Thus, coordination assessment is a key component of the neurological examination. A number of studies have been performed on balance and movement coordination of impaired locomotion involving qualitative and quantitative methods. Examining MS in particular, it is observed that the most common parameters are slowing down of movements, decreased repeatability of repetitive movements, trajectory accuracy when targeting, differences in joint amplitudes, increased variation and asymmetry between damaged and undamaged sides of the body [22, 25, 26, 27, 28]. Movement variability is considered as a clinical measure to assess the locomotor coordination in various motor dysfunctions. Coordination is evaluated by testing the patient’s ability to perform rapidly alternating and point-to-point movements correctly. Standardized functional tests such as Finger-to-Nose (FNT), 9-Hole Peg Test (9HPT), Box-and-Block test (BBT) are commonly used qualitatively to assess damaged upper limb coordination. The Heel-to-Shin test (HST) is used to examine lower limb coordination; the Timed 25-Foot Walk test (T25-FW), Tandem Walking is used to assess coordination during gait. It can be seen, that routine clinical evaluation of a patient involves a large number of tests that reveal the functional capabilities of different body segments. In general, whole-body coordination is best assessed by only two tests. HST with FNT are the most frequently used qualitative examination for upper and lower body coordination on a conventional basis. Thus, the sum of the two tests enables the observation of the whole picture of locomotor gross and fine functional abilities. These tests are analogous to each other and help to evaluate rapidly alternating performance as well as point-to-point movement. Moreover, they allow to evaluate the slowdown of movements, joint amplitudes, and accuracy [29, 30, 31]. Examination of all these parameters on different sides of the body allows to estimate the asymmetry. Simple and complex quantitative methods are used for more accurate coordination testing: starting from dynamometer and metronome to comprehensive motion capture and posturographic systems [32]. However, we did not find a single test, either qualitative or quantitative, for assessing MS whole-body (upper and lower) asymmetry.
The asymmetry between right and left lower limbs during walking, running, jumping and similar are often used to evaluate impaired coordination, balance, gait, etc. [33, 34, 35, 36]. Most of the scientists use different methodologies to evaluate the symmetry of the human body. Most often symmetry indices are calculated. They can be as follows: Ratio Index (RI), which uses the ratio of the values for the two limbs [37]; Symmetry Index (SI) calculated from an average of the absolute values for both limbs [38]; Symmetry Index (S.I.) calculated from an average of the absolute maximal and minimal values for both limbs [39]; Gait Asymmetry (GA) index is a logarithmic transform of the RI [40], Symmetry Angle (SA) is a factor calculated for the angle of the vector plotted from the right and left values of discrete gait parameters in relation to the OX axis [41]; or Normalized Symmetry Index (NSI), which can be used easily in any setting and can serve as a universal index for assessing symmetry in a clinical context [42].
The motivation for our study arose from the above-discussed needs for analyzing MS overall body coordination. Specifically, we hypothesized that MS patients would demonstrate impaired coordination of both upper and lower limbs across most of the dependent variables, and not only in the task performance time. In particular, this study was designed in line with four main aims: a) to analyze HST and FNT movements quantitatively; b) to find indicators to discriminate MS from healthy subjects; c) to evaluate the relationship between upper and lower limb asymmetry; and d) to define asymmetry of the body for MS and healthy subjects.
2.Methodology
2.1Participants
In total 28 MS patients (age range from 18 to 59 years, 38
Figure 1.
Study flowchart for subjects’ selection, assessment, and analysis.
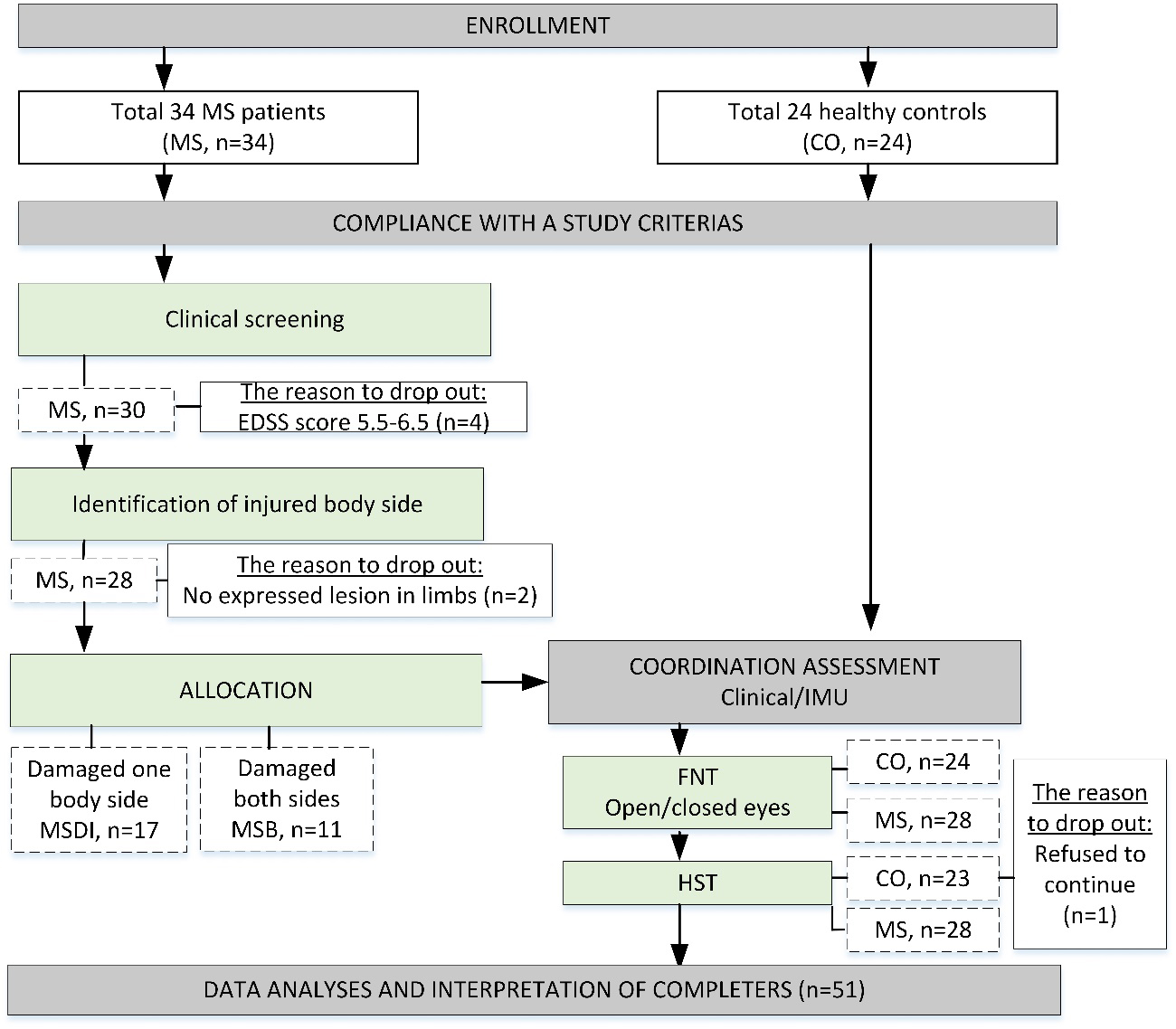
2.2Experimental procedure
Throughout the study, two coordination assessment tests were performed: FNT (open/closed eyes) and HST. Abnormalities were identified when the subjects were unable to target their finger to the nose or to keep their foot on the shin. The standardized procedure of FNT and HTS tests was explained and demonstrated by a neurologist for all participants (Fig. 2).
Quantitative analysis of movements performed processing the acquired kinematic data from six Inertial Measurement Units (IMUs; Shimmer Research, Dublin, Ireland), placed on the upper and lower extremities during FNT and HTS tasks. Linear acceleration, angular velocity, and magnetic heading in three dimensions were recorded. The initial position of movement was determined when the subject is sitting on the chair with fully extended arms (FNT) or in a supine position (HST) and range of motion (ROM) in joints were zero. While performing FNT, the patient is instructed to touch his or her own nose and return to the initial position (as indicated in Fig. 2a). This position was determined as the end of the movement. After the subject is instructed to place the heel of one foot onto the anterior part of the shin just below the knee joint of the opposite leg and then slide the heel down the shin towards the ankle (as indicated in Fig. 2b). The end of the movement was determined when the leg is returned to the initial position (this is considered as one full cycle). The movement was repeated three times for each arm (FNT) and leg (HST). The clinician scores were calculated according to the accuracy and smoothness and whether the subject was able to maintain heel contact with the shin.
Figure 2.
The standardized procedure of FNT (a) and HTS (b) tests.
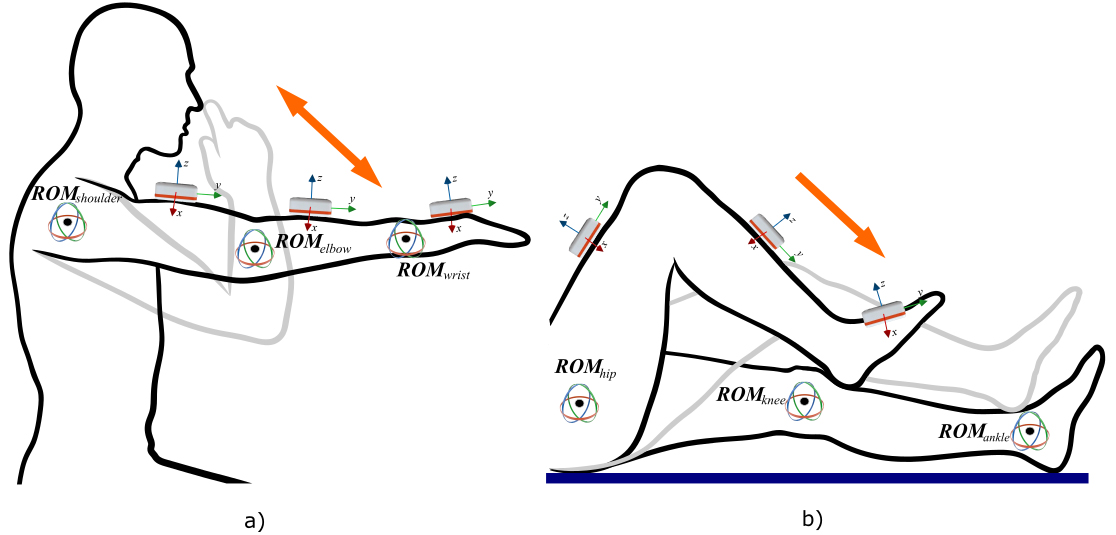
2.3Data processing and analysis
All collected data were analyzed using MATLAB software (Mathworks Inc., USA). The gyroscope, accelerometer, and magnetometer data were processed to determine spatiotemporal parameters. IMUs signals were sampled at 256 Hz and stored on the personal computer. The time interval between the movement start to end was defined as movement time (
Raw data from IMUs were pre-processed in three dimensions according to Madgwick’s Attitude and Heading Reference Systems (AHRS) algorithm [43]. Both sides of the body were evaluated and respectively Euler’s angles of the wrist, elbow, and shoulder joints (in FNT) or ankle, knee, and hip joints (in HST) were calculated. In FNT Euler’s
The range of motion (ROM) of joints was calculated in three dimensions:
(1)
Symmetry was determined based on four usually used symmetry indices. For MS
(2)
(3)
(4)
(5)
If the values of RI, SI, SA, and GA are equal to zero, it indicates full symmetry, while RI, SI, GA, and SA are
Figure 3.
Box plots of all symmetry indices (RI, SI, SA, GA) for knee flexion/extension ROMs
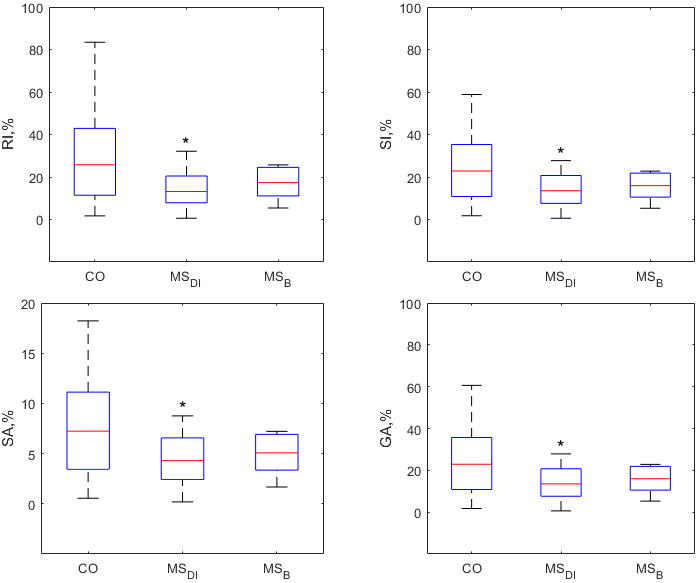
2.4Statistical analysis
The following tests were used to verify the existence of a possible relationship between the variables examined: Shapiro-Wilk normality test (
Table 1
Results of temporal variables,
Variables | Group | Index | HST | FNT eyes opened | FNT eyes closed | |||
---|---|---|---|---|---|---|---|---|
Median (MAD) | IQR | Median (MAD) | IQR | Median (MAD) | IQR | |||
CO | RI,% | 4.4 (7.6) | 2.2–15.9 | 6.1 (3.9) | 3.8–11.1 | 5.5 (3.7) | 2.3–9.6 | |
MS | 20.1 (15.3) | 9.2–37.9 | 14.3 (15.5) | 7.9–32.8 | 23.6 (26.3) | 12.6–59.9 | ||
MS | 15.2 (7.8) | 7.7–22.5 | 10.3 (3.4) | 6.7–12.1 | 5.8 (7.1) | 3.6–14.7 | ||
CO | SI,% | 4.3 (6.9) | 2.5–14.4 | 5.9 (4.3) | 3.7–11.8 | 5.7 (3.5) | 2.5–9.2 | |
MS | 20.9 (14.5) | 10.0–35.1 | 14.3 (13.9) | 8.0–29.9 | 26.8 (20.1) | 14.7–48.3 | ||
MS | 16.5 (7.1) | 8.4–20.9 | 10.2 (3.5) | 6.6–12.3 | 5.8 (6.6) | 3.6–13.9 | ||
CO | SA,% | 1.4 (2.2) | 0.8–4.6 | 1.9 (1.4) | 1.2–3.8 | 1.8 (1.1) | 0.8–2.9 | |
MS | 6.6 (4.5) | 3.2–10.9 | 4.6 (4.4) | 2.6–9.4 | 8.5 (6.2) | 4.7–14.9 | ||
MS | 5.2 (2.3) | 2.7–6.6 | 3.2 (1.1) | 2.1–3.9 | 1.8 (2.1) | 1.2–4.4 | ||
CO | GA,% | 4.3 (6.9) | 2.5–14.5 | 5.9 (4.4) | 3.7–11.9 | 5.7 (3.5) | 2.5–9.2 | |
MS | 20.9 (14.9) | 9.9–35.7 | 14.3 (14.1) | 8.0–30.4 | 26.9 (21.0) | 14.6–50.1 | ||
MS | 16.5 (7.2) | 8.4–21.0 | 10.2 (3.5) | 6.6–12.4 | 5.8 (6.7) | 3.6–14.0 |
The significant differences presented as follow: Bold – a significant difference of MS with CO; Bold italic – significant difference between MS
Figure 4.
Box plots of all symmetry indices (RI, SI, SA, GA) for elbow flexion/extension ROM
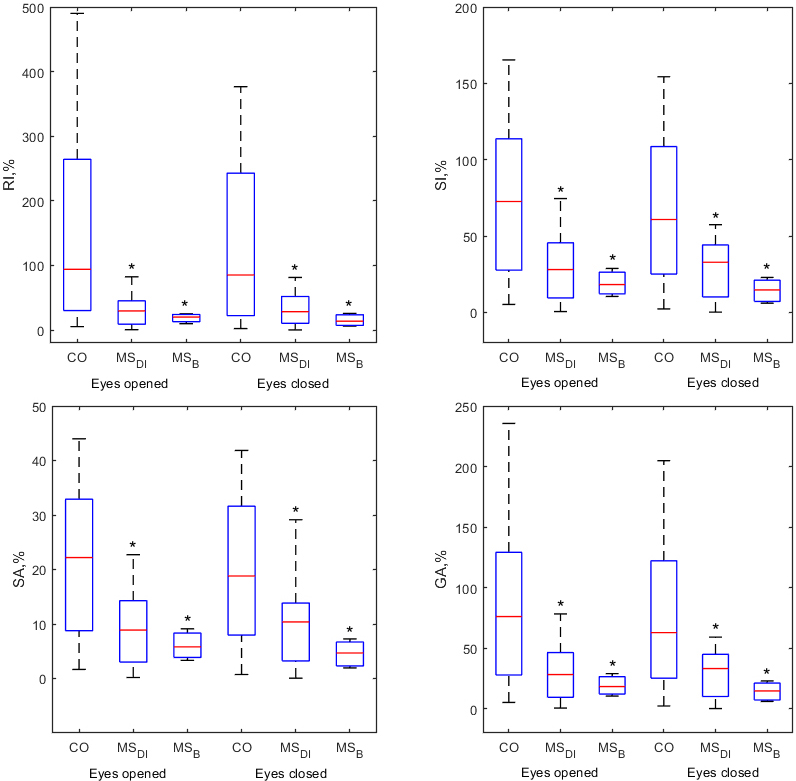
3.Results
Since no significant differences between variables in the groups were observed in the frontal and transverse planes, the results demonstrate only meaningful changes in the sagittal plane.
Asymmetry indices of temporal parameters showed statistically significant differences in both, upper body (FNT) and lower body (HST) asymmetry of MS compared to CO (Table 1). Moreover, all indices enclose meaningful shifts between upper body sides (FNT eyes closed). Higher symmetry indices were found in MS groups.
Figure 5.
Medians
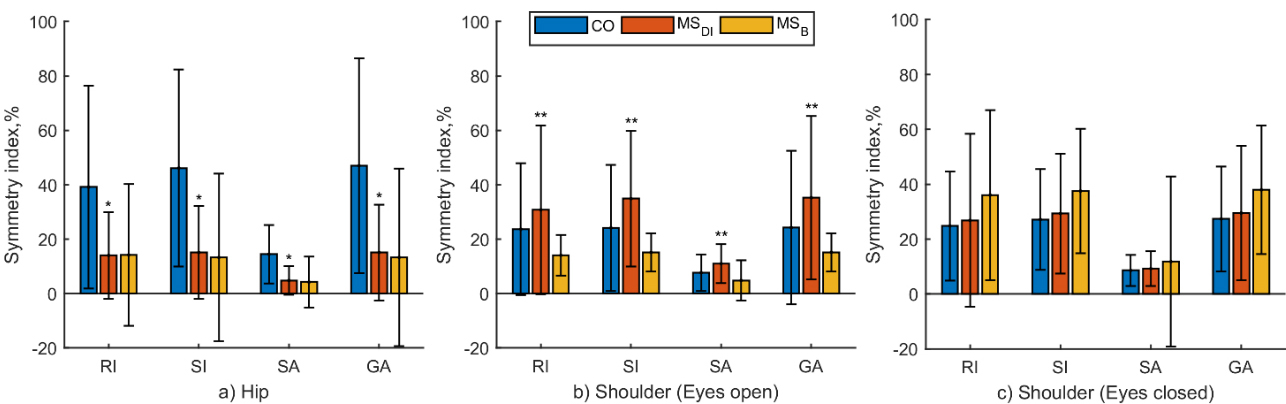
Figure 6.
Medians
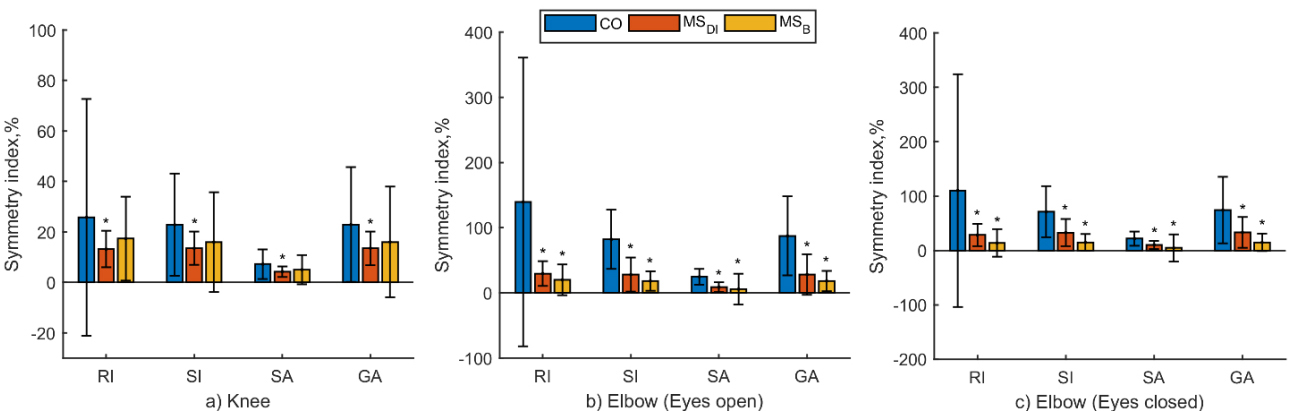
Comparison of symmetry indices calculated from kinematic parameters of a particular segment of the limb are presented in Figs 3–4. We did not find significant differences in symmetry indices obtained from ankle and wrist ROMs. Knee, hip, elbow, and shoulder demonstrated significant asymmetry of physical performance. All symmetry indices of the knee (Fig. 3) and elbow (Fig. 4) best discriminated MS
Symmetry index values by limb segments demonstrate upper and lower body asymmetry and are presented in Figs 5 and 6. The asymmetry of the hip joint is significantly greater in the CO group than in the MS
Comparing the symmetry of the knee and elbow joints, the same tendency was observed that the CO group performs movements with significantly less symmetry than the MS
Spearman correlation coefficients between the upper and lower limb temporal and kinematic symmetry indices showed that there is a weak relationship in the CO group (
4.Discussion
This study examined the associations between upper and lower body asymmetry and identified parameters that can differentiate between MS and CO. The results of this study demonstrated that asymmetries of the upper and lower body in MS patients have no relation. Symmetry indices [37, 38, 40, 41] for the upper and lower extremities were calculated from clinical trial data for the upper and lower limb. Obtained spatiotemporal parameters (ROMs and time of movement) demonstrated different results of an individual’s body symmetry.
Statistically significant differences of temporal symmetry indices of lower (HST) and upper (FNT) limbs were found among MS versus CO for upper and lower limbs. They were sensitive to differentiate MS from CO and even MS types (MS
Kinematic lower body asymmetry is usually evaluated from the gait parameters [19, 26, 33, 34, 35, 36, 39, 40]. The scientific literature reveals similar rates of asymmetry from gait parameters in MS patients compared to CO, although they were slightly higher among MS. Also, MS demonstrated higher variability [34, 36]. Previously reported comparison of damaged versus intact sides in MS showed that strength asymmetries may not play an important role in prolonged walking performance [35] or knee extensor power asymmetry was associated with fatigue and walk times [19]. Interestingly, mostly lower body symmetry is assessed among MS to result in MS levels [36] or predict the probability of falling [33]. We attempted to verify the fact of MS lower body asymmetry from HST kinematic data (ROMs of limb segments). However, our results showed an inverse distribution of symmetry among individuals, although MS differed significantly from the healthy group. Even though these findings lead to the rejection of our hypothesis, we believe that we obtained such results because the HST motion is relatively short and has only one cycle. In addition, MS patients performed the test at a much slower rate, adapting in an effort to complete it as accurately as possible. Also, MS patients were severe (up to EDSS 5.0). Healthy individuals have a considerably greater movement asymmetry compared to MS according to our obtained kinematic symmetry indices. It might be associated with a fact of higher joint variability in CO [42, 45], that has been found over a short period of movement.
Assessment of upper limb symmetry is not a common phenomenon in the literature. Only several papers present the results, in which the symmetry of the lower and upper limbs is compared. One of them found that the hand data are, in general, more asymmetric than lower limb data, even among healthy subjects, and confirmed that symmetry indices can distinguish individuals with Parkinson’s from healthy ones [46]. Another found that there were no differences in upper limb strength and lower limb flexibility between the dominant and non-dominant sides [47]. In our study symmetry indices of the upper body obtained from ROMs differentiate MS from CO. The shoulder ROMs revealed a statistically significant difference in asymmetry among MS. Moreover, the asymmetry was found considerably less in CO versus MS. Elbow movement evaluation demonstrated the same manner like lower limbs: higher asymmetry was found in CO, although significantly differentiated MS from CO. In addition, when assessing the symmetry of the upper extremities in the sagittal plane, the values of some symmetry indices, such as RI, SI, and GA, exceed the limits. Therefore, such results are impossible and cannot be interpreted accurately. This is also noted by other researchers [42].
To conclude, the less sensitive to movement parameters is SA symmetry index and it seems to be the most objective for body asymmetry evaluation from clinical trial data. Apparently healthy individuals demonstrated considerably higher lower and upper body kinematic asymmetry versus MS, with the exception of shoulder movements. Temporal upper and lower asymmetry significantly discriminated MS from CO. Statistically significantly greater asymmetry of both lower and upper limbs was indicated in MS. These findings are unique to this study because they have not previously been studied.
5.Conclusion
The results of our study indicated that time parameters are the most sensitive to body asymmetry. However, ROMs are completely inappropriate if they are calculated for one movement cycle. In clinical testing, it is sufficient to assess the time of movement, as it properly identifies the extent of asymmetry.
Acknowledgments
Special thanks to Gintaras Kaubrys, the head of the Santaros Neurology Center and his team Gyte Pakulaite-Kazliene, as well as to Donatas Luksys and Vigita Apanskiene for help in data collection.
Conflict of interest
None to report.
References
[1] | Horowitz G, Ungar OJ, Levit Y, et al. The impact of conductive hearing loss on balance. Clin Otolaryngol. (2020) ; 45: : 106-110. doi: 10.1111/coa.13473. |
[2] | Dierijck J, Kennefick M, Smirl J, et al. Attention is required to coordinate reaching and postural stability during upper limb movements generated while standing. J Mot Behav. (2020) ; 52: : 79-88. doi: 10.1080/00222895.2019.1587351. |
[3] | Hunter MC, Hoffman MA. Postural control: Visual and cognitive manipulations. Gait Posture. (2001) ; 13: : 41-48. doi: 10.1016/S0966-6362(00)00089-8. |
[4] | Agmon M, Lavie L, Doumas M. The association between hearing loss, postural control, and mobility in older adults: A systematic review. J Am Acad Audiol. (2017) ; 28: : 575-588. doi: 10.3766/jaaa.16044. |
[5] | Haines JD, Inglese M, Casaccia P. Axonal damage in multiple sclerosis. Mt Sinai J Med A J Transl Pers Med. (2011) ; 78: : 231-243. doi: 10.1002/msj.20246. |
[6] | Nantes JC, Zhong J, Holmes SA, et al. Cortical damage and disability in multiple sclerosis: Relation to intracortical inhibition and facilitation. Brain Stimul. (2016) ; 9: : 566-573. doi: 10.1016/j.brs.2016.01.003. |
[7] | Eken MM, Richards R, Beckerman H, et al. Quantifying muscle fatigue during walking in people with multiple sclerosis. Clin Biomech. (2020) ; 72: : 94-101. doi: 10.1016/j.clinbiomech.2019.11.020. |
[8] | Manjaly Z-M, Harrison NA, Critchley HD, et al. Pathophysiological and cognitive mechanisms of fatigue in multiple sclerosis. J Neurol Neurosurg Psychiatry. (2019) ; 90: : 642-651. doi: 10.1136/jnnp-2018-320050. |
[9] | Van Kessel K, Babbage DR, Reay N, et al. Mobile Technology use by people experiencing multiple sclerosis fatigue: Survey methodology. JMIR mHealth uHealth. (2017) ; 5: : e6. doi: 10.2196/mhealth.6192. |
[10] | McLoughlin JV, Barr CJ, Patritti B, et al. Fatigue induced changes to kinematic and kinetic gait parameters following six minutes of walking in people with multiple sclerosis. Disabil Rehabil. (2016) ; 38: : 535-543. doi: 10.3109/09638288.2015.1047969. |
[11] | Chalah MA, Riachi N, Ahdab R, et al. Fatigue in multiple sclerosis: Neural correlates and the role of non-invasive brain stimulation. Front Cell Neurosci. 9. Epub ahead of print 30 November 2015. doi: 10.3389/fncel.2015.00460. |
[12] | Soysal Tomruk M, Uz MZ, Kara B, et al. Effects of Pilates exercises on sensory interaction, postural control and fatigue in patients with multiple sclerosis. Mult Scler Relat Disord. (2016) ; 7: : 70-73. doi: 10.1016/j.msard.2016.03.008. |
[13] | Engel-Yeger B, DeLuca J, Hake P, et al. The role of sensory processing difficulties, cognitive impairment, and disease severity in predicting functional behavior among patients with multiple sclerosis. Disabil Rehabil. (2019) ; 1-8. doi: 10.1080/09638288.2019.1653998. |
[14] | Patejdl R, Zettl UK. Spasticity in multiple sclerosis: Contribution of inflammation, autoimmune mediated neuronal damage and therapeutic interventions. Autoimmun Rev. (2017) ; 16: : 925-936. doi: 10.1016/j.autrev.2017.07.004. |
[15] | Alusi SH. Evaluation of three different ways of assessing tremor in multiple sclerosis. J Neurol Neurosurg Psychiatry. (2000) ; 68: : 756-760. doi: 10.1136/jnnp.68.6.756. |
[16] | Cattaneo D, Rabuffetti M, Bovi G, et al. Assessment of postural stabilization in three task oriented movements in people with multiple sclerosis. Disabil Rehabil. (2014) ; 36: : 2237-2243. doi: 10.3109/09638288.2014.904933. |
[17] | Schmidt F, Zimmermann H, Mikolajczak J, et al. Severe structural and functional visual system damage leads to profound loss of vision-related quality of life in patients with neuromyelitis optica spectrum disorders. Mult Scler Relat Disord. (2017) ; 11: : 45-50. doi: 10.1016/j.msard.2016.11.008. |
[18] | Vister E, Tijsma ME, Hoang PD, et al. Fatigue, physical activity, quality of life, and fall risk in people with multiple sclerosis. Int J MS Care. (2017) ; 19: : 91-98. doi: 10.3109/09638288.2016.1148784. |
[19] | Chung LH, Remelius JG, Van Emmerik REA, et al. Leg power asymmetry and postural control in women with multiple sclerosis. Med Sci Sport Exerc. (2008) ; 40: : 1717-1724. doi: 10.3109/09638288.2016.1148784. |
[20] | Ryew C-C, Lee A-R, Hyun S-H. Effect of muscle mass asymmetric between upper and lower limbs on the postural stability and shock attenuation during landing. J Exerc Rehabil. (2019) ; 15: : 488-492. doi: 10.12965/jer.1938188.094. |
[21] | Tijsma M, Vister E, Hoang P, et al. A simple test of choice stepping reaction time for assessing fall risk in people with multiple sclerosis. Disabil Rehabil. (2017) ; 39: : 601-607. doi: 10.3109/09638288.2016.1148784. |
[22] | Salehi R, Mofateh R, Mehravar M, et al. Comparison of the lower limb inter-segmental coordination during walking between healthy controls and people with multiple sclerosis with and without fall history. Mult Scler Relat Disord. (2020) ; 41: : 102053. doi: 10.1016/j.msard.2020.102053. |
[23] | Afzal T, Kern M, Tseng S-C, et al. Cognitive demands during wearable exoskeleton assisted walking in persons with multiple sclerosis. In: 2017 International Symposium on Wearable Robotics and Rehabilitation (WeRob). IEEE, pp. 1-2. doi: 10.1109/WEROB.2017.8383853. |
[24] | Finlayson M, Guglielmello L, Liefer K. Describing and predicting the possession of assistive devices among persons with multiple sclerosis. Am J Occup Ther. (2001) ; 55: : 545-551. doi: 10.5014/ajot.55.5.545. |
[25] | Gibson-Horn C. Balance-based torso-weighting in a patient with ataxia and multiple sclerosis: A case report. J Neurol Phys Ther. (2008) ; 32: : 139-146. doi: 10.1097/NPT.0b013e318185558f. |
[26] | Gianfrancesco MA, Triche EW, Fawcett JA, et al. Speed- and cane-related alterations in gait parameters in individuals with multiple sclerosis. Gait Posture. (2011) ; 33: : 140-142. doi: 10.1016/j.gaitpost.2010.09.016. |
[27] | Sebastião E, Sandroff BM, Learmonth YC, et al. Validity of the timed up and go test as a measure of functional mobility in persons with multiple sclerosis archives of physical medicine and rehabilitation. Arch Phys Med Rehabil. Epub Ahead of Print. (2016) ; 97: (7): 1072-1077. doi: 10.1016/j.apmr.2015.12.031. |
[28] | Craig JJ, Bruetsch AP, Lynch SG, et al. Instrumented balance and walking assessments in persons with multiple sclerosis show strong test-retest reliability. J Neuroeng Rehabil. Epub Ahead of Print. (2017) ; 14: (1): 43. doi: 10.1186/s12984-017-0251-0. |
[29] | Johansson GM, Grip H, Levin MF, et al. The added value of kinematic evaluation of the timed finger-to-nose test in persons post-stroke. J Neuroeng Rehabil. (2017) ; 14: : 1-12. |
[30] | Gagnon C, Mathieu J, Desrosiers J. Standardized finger-nose test validity for coordination assessment in an ataxic disorder. Can J Neurol Sci/J Can des Sci Neurol. (2004) ; 31: : 484-489. |
[31] | Krishna R, Pathirana PN, Horne M, et al. Quantitative assessment of cerebella ataxia through automated upper limb functional tests. In: Mao K, Jiang X (eds) Tenth International Conference on Signal Processing Systems. SPIE, p. 25. doi: 10.1117/12.2520693. |
[32] | Banach M, Wasilewska A, Dlugosz R, Pauk J. Novel techniques for a wireless motion capture system for the monitoring and rehabilitation of disabled persons for application in smart buildings, Technology and Health Care. (2018) ; 26: (S2): S671-S677. |
[33] | Kalron A. Symmetry in vertical ground reaction force is not related to walking and balance difficulties in people with multiple sclerosis. Gait Posture. (2016) ; 47: : 48-50. doi: 10.1016/j.gaitpost.2016.04.010. |
[34] | Dujmovic I, Radovanovic S, Martinovic V, et al. Gait pattern in patients with different multiple sclerosis phenotypes. Mult Scler Relat Disord. (2017) ; 13: : 13-20. doi: 10.1016/j.msard.2017.01.012. |
[35] | Proessl F, Ketelhut NB, Rudroff T. No association of leg strength asymmetry with walking ability, fatigability, and fatigue in multiple sclerosis. Int J Rehabil Res. (2018) ; 41: : 267-269. doi: 10.1097/MRR.0000000000000278. |
[36] | Motta C, Palermo E, Studer V, et al. Disability and fatigue can be objectively measured in multiple sclerosis. PLoS One. (2016) ; 11: : e0148997. doi: 10.1371/journal.pone.0148997. |
[37] | Ganguli S, Mukherji P, Bose KS. Gait evaluation of unilateral below knee amputees fitted with patellar tendon bearing prostheses. J Indian Med Assoc. |
[38] | Robinson RO, Herzog W, Nigg BM. Use of force platform variables to quantify the effects of chiropractic manipulation on gait symmetry. J Manipulative Physiol Ther. |
[39] | Marinakis GNS. Interlimb symmetry of traumatic unilateral transtibial amputees wearing two different prosthetic feet in the early rehabilitation stage. J Rehabil Res Dev. (2004) ; 41: : 581. doi: 10.1682/JRRD.2003.04.0049. |
[40] | Plotnik M, Giladi N, Balash Y, et al. Is freezing of gait in Parkinson’s disease related to asymmetric motor function? Ann Neurol. (2005) ; 57: : 656-663. doi: 10.1002/ana.20452. |
[41] | Zifchock RA, Davis I, Higginson J, et al. The symmetry angle: A novel, robust method of quantifying asymmetry. Gait Posture. (2008) ; 27: : 622-627. doi: 10.1016/j.gaitpost.2007.08.006. |
[42] | Queen R, Dickerson L, Ranganathan S, et al. A novel method for measuring asymmetry in kinematic and kinetic variables: The normalized symmetry index. J Biomech. (2020) ; 99: : 109531. doi: 10.1016/j.jbiomech.2019.109531. |
[43] | Madgwick SOH. An efficient orientation filter for inertial and inertial/magnetic sensor arrays. Rep x-io Univ Bristol. (2010) ; 25: : 113-118. doi: 10.1109/ICORR.2011.5975346. |
[44] | Buckley C, Micó-Amigo ME, Dunne-Willows M, et al. Gait asymmetry post-stroke: Determining valid and reliable methods using a single accelerometer located on the trunk. Sensors. (2019) ; 20: : 37. doi: 10.3390/s20010037. |
[45] | Stalioraitis V, Robinson K, Hall T. Side-to-side range of movement variability in variants of the median and radial neurodynamic test sequences in asymptomatic people. Man Ther. (2014) ; 19: : 338-342. doi: 10.1016/j.math.2014.03.005. |
[46] | Sant’Anna A, Salarian A, Wickstrom N. A new measure of movement symmetry in early Parkinson’s disease patients using symbolic processing of inertial sensor data. IEEE Trans Biomed Eng. (2011) ; 58: : 2127-2135. doi: 10.1109/TBME.2011.2149521. |
[47] | Caraballo I, González-Montesinos JL, Alías A. Bilateral and Unilateral Asymmetries of Strength and Flexibility in Young Elite Sailors: Windsurfing, Optimist and Laser Classes. Symmetry (Basel). (2020) ; 12: : 184. doi: 10.3390/sym12010184. |