Designing and manufacturing custom-made 3D printed assistive devices: A comparison of three workflows
Abstract
BACKGROUND:
Do-it-yourself technologies such as 3D printing offer interesting opportunities for creating custom-made assistive devices for people with disabilities. Although these opportunities are increasingly acknowledged, it remains unclear how 3D printing technology should be implemented in assistive devices delivery processes.
OBJECTIVE:
In three separate action research projects carried out in Belgium, Italy and The Netherlands, workflows were designed and evaluated that incorporate 3D printing as a standard option for providing clients with suitable assistive devices.
METHODS:
In this paper we describe and compare the three workflows that were conceived simultaneously, yet independently from each other.
RESULTS:
Based on the evaluations of these workflows, and the experiences of the researchers who developed the workflows, we provide recommendations for implementing 3D printing as a common approach in assistive device delivery processes in practice. Most importantly, designing and manufacturing should be done by means of a client-centered co-creation process by interdisciplinary teams of clinicians, clients, and 3D printing experts. We provide several recommendations for facilitating and supporting collaborations within such teams.
CONCLUSIONS:
The three workflows presented in this paper are strikingly similar and therefore provide a convincing starting point for interdisciplinary design teams who wish to embark on 3D printing custom-made assistive devices.
1.Introduction
People with disabilities often use assistive devices (ADs) to perform activities of daily living and to participate in society [1]. In the past, occupational therapists (OTs) were taught to create ADs for their clients by means of crafting techniques, such as knitting and woodworking [2]. Nowadays, however, most ADs that OTs provide to their clients are off-the-shelf, commercially available devices [3]. Unfortunately, since such off-the-shelf devices are not custom-made, it is not always possible to ensure an optimal fit, which can lead to abandonment of ADs [4]. Research shows that in Western countries almost a third of all ADs are abandoned after one year [3, 4, 5].
Although making ADs for individual clients has become less common in occupational therapy, the rise of so-called do-it-yourself (DiY) technologies, such as 3D printing, introduces interesting opportunities. Such technologies allow for the creation of custom-made solutions, which could reduce the risk of ill-fitting ADs. In addition, it allows for involving clients and their informal caregivers in the design process of ADs [6], which has been shown to improve acceptance and to reduce the risk of abandonment [7]. As such, modern DiY technologies might generate a revival of the traditional maker approach towards creating ADs in occupational therapy, and could result in custom-made solutions that meet clients’ needs better than off-the-shelf devices.
Several benefits of 3D printing for manufacturing medical devices such as ADs have been described in literature. First, 3D printing allows for the production of unique parts of ADs without the high costs associated with conventional production techniques [8]. Second, technological developments in commercially available 3D printing techniques and 3D printing materials make it possible to use 3D printing for an increasingly broad range of applications in the health care sector. For instance, 3D printing has been used in recent years to create personalized implants and hearing aids [9], orthoses and prostheses [10, 11], dental implants [12], etc. Third, digital design files for ADs can be shared, which creates opportunities for collaboration on improved designs as well as for reusing designs across end-users [13]. It is becoming increasingly common for AD makers to share their designs via online repositories such as Thingiverse.com [14], especially for simple and relatively small ADs such as custom-made cutlery, grips and holders for everyday objects, wheelchair mounts, prosthetic limbs, splints, tactile graphics, etc. Finally, the production of 3D printed ADs can be done locally, which has economical and sustainability advantages [15].
Despite the many benefits, 3D printing as a manufacturing technique for ADs has not been generally adopted by OTs yet. One of the hurdles for widespread implementation may lay in the fact that the process of designing and manufacturing custom-made 3D printed ADs is not straightforward [16]. It requires skills that are not part of the typical skillset of OTs, nor of occupational therapy teaching programmes. Designing 3D printable objects involves the drawing of digital 3D models with computer-aided design (CAD) software, which requires very different design skills than the crafting techniques that OTs used to be trained with. As a result, clinicians such as OTs are currently not typically involved in the digital design of custom-made ADs [13, 14]. In addition, the ever-growing range of available printing techniques and materials makes it very challenging for people with limited 3D printing experience to choose the best options for their designs. A final, yet important barrier, is the fact that designing and manufacturing custom-made 3D printed ADs has not yet been incorporated in existing assistive technology service delivery systems. This implicates, for instance, that the costs for creating this new class of ADs might not be reimbursed by default.
In many countries, AD delivery processes have been in place for years as part of their national or regional healthcare or welfare systems [5, 17]. These processes vary broadly within and across countries, in relation to national and regional disability policies, socio- economic context, and history [5, 17]. Such delivery processes are comprised of different steps through which clients are provided with ADs that meet their needs [18]. Despite the differences in national processes, common steps can be recognized. In 1994, the HEART study analyzed AD provision and service delivery systems in 16 European countries. According to a position paper on behalf of the Association for the Advancement of Assistive Technology in Europe (AAATE) and the European Assistive Technology Information Network (EASTIN) [19], the seven common steps resulting from this analysis are still relevant and adequate. These seven steps, which have been described by several authors (e.g. [1, 3, 17, 18, 19]) are: (1) initiative – first contact with the delivery system; (2) assessment – evaluation of needs; (3) selection of the assistive solution – defining the individual AD programme; (4) selection of the equipment – choosing the specific equipment within the AD programme; (5) authorisation – obtaining funding; (6) implementation – delivering the equipment to the user, fitting and training; (7) management and follow up – maintenance and periodic verification.
Figure 1.
Examples of 3D printed ADs that were created in the three projects. From left to right: Joystick in the Flemish project; Cup-holder in the Dutch project; Can-opener in the Italian project.
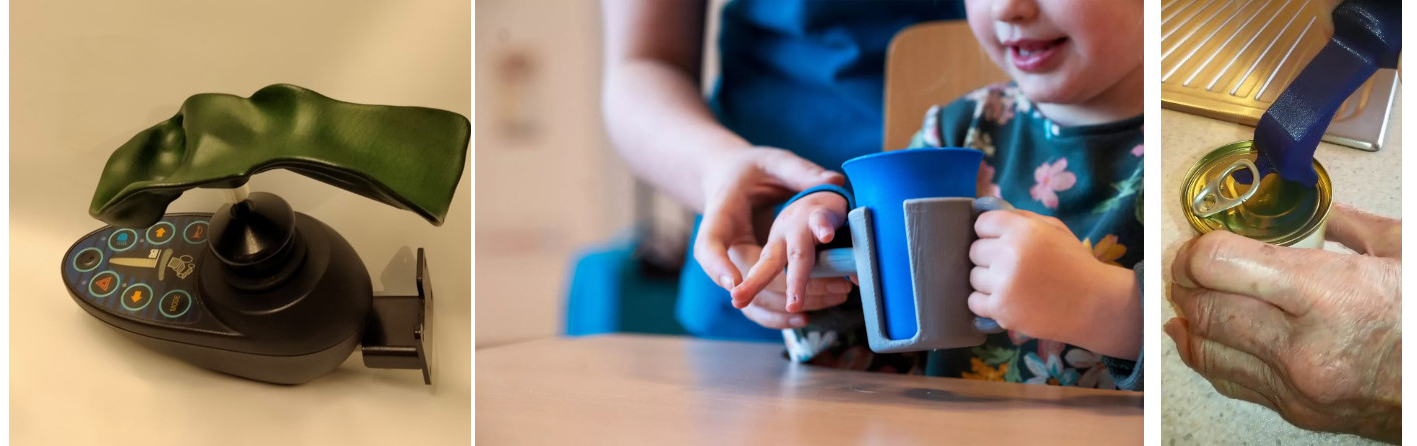
Figure 2.
The workflow developed in the Flemish project ‘Assist3D: 3D printing of bespoke aids’.
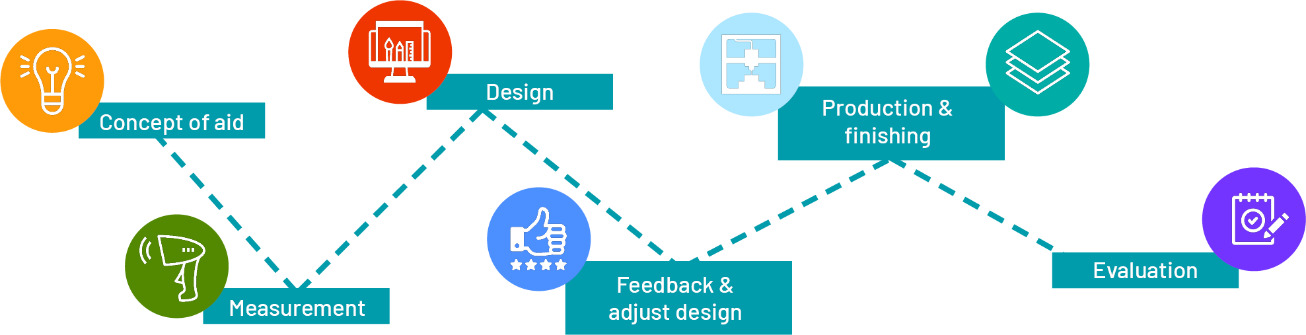
To understand how 3D printing would fit into existing AD delivery processes, the authors of this paper were involved in research projects that were independently but simultaneously carried out in three European countries (Belgium, Italy and The Netherlands). In each of these projects, new AD delivery workflows were designed that incorporate 3D printing as a standard option for providing clients with a suitable AD. In this paper, we – the researchers who were involved in the three projects – present the workflows that resulted from these projects. Based on a comparison of these workflows, and on reflections on our experiences with these workflows, we formulate recommendations for facilitating the adoption of 3D printing as a common approach for creating custom-made ADs.
2.Three AD delivery workflows including 3D printing
In each of the three research projects mentioned above, a workflow was designed to demonstrate how 3D printing could be incorporated in AD delivery processes. In this section, we provide background information about each project, and we describe the workflows that were developed. We briefly explain how the workflows were designed and evaluated. In the next section we compare the three workflows in detail.
2.1The Flemish project (Belgium)
‘Assist3D: 3D printing of bespoke aids’ was a research project carried out between 2018 and 2021 in the region of Flanders in Belgium. The project was funded by a regional funding programme for applied research to stimulate innovation. It was carried out by Thomas More University of Applied Sciences in collaboration with nine different healthcare and patient organizations for persons with motor, intellectual, visual and/or hearing disabilities, the Flemish Agency for People with Disabilities, six AD providers, and five organisations with expertise in 3D printing.
The Flemish project aimed to research how to lower the threshold for healthcare organisations and OTs to apply 3D printing techniques in order to improve the quality of life and participation of persons with disabilities in need for custom-made ADs. To meet this goal, an action research approach was adopted in which 3D printed ADs were designed and manufactured by an interdisciplinary team for 35 real client cases (see an example in Fig. 1).
2.1.1The Flemish workflow
The Flemish workflow was developed in co-creation with OTs and 3D printing experts. The starting point was a workflow that is typically used for the design of custom-made 3D printed orthopaedic devices [10], which was modified for designing and manufacturing ADs. The modified workflow (see Fig. 2) consists of six steps.
In the first step, called Concept of Aid, the care needs of the client are identified and assessed to determine if a 3D printed AD would be a suitable solution. For those care needs for which a 3D printed AD is a viable option, the concept and requirements are determined in a client-centered co-design session, involving the client, the OT, and a 3D printing expert. During this session, several design concepts are explored. Inspiration for these concepts is taken from clients’ and OTs’ positive and negative experiences with off-the-shelf ADs, as well as from existing digital 3D models that can be found online (e.g. via Thingiverse.com, an online sharing platform for digital design files).
In step 2, Measurement, the client, OT and 3D printing expert identify which measurements should be taken in order to realize the concept that was selected in the first step. They determine if the AD needs to fit other objects (e.g. a mobility aid) and whether it should be tailored to the client’s anatomy. They also select appropriate measurement techniques (e.g. tape measurements or 3D scanning) to take the actual measurements.
In the next step, Design, the measurements or scans are used to design a digital 3D model of the AD. Based on the complexity of the concept, appropriate CAD software is selected. In the research project, digital models of low complexity were designed by an OT, using low complexity software. Models for more complex ADs were made by an engineer using more complex software. The project also explored ways to facilitate OTs with little to no CAD experience to interact and adapt digital models of complex ADs.
Once a first digital model is ready, it is presented to the client (and the OT, if the model is designed by a 3D expert) so that they can provide feedback (step 4: Feedback and Adjust Design). For this presentation, which can be done face-to-face or online, a 3D web viewer can be used, which allows for the presentation of the 3D model and for adding annotations (e.g. remarks or questions) to certain elements of the model.
In the next step, Production and Finishing, the digital 3D model is printed. To choose the most suitable printing materials and techniques, several criteria are considered. For instance, some ADs need to be able to withstand large mechanical forces and are best printed with industrial grade printing techniques that are also used for orthopaedic devices (such as Laser Sintering or Multi Jet Fusion [10, 20]). Simpler ADs, such as pen grips, on the other hand, can be produced with consumer grade printers. Another example regards ADs that come into contact with food, for which post processing with food-safe coatings is recommended, or ADs that are worn on the skin for prolonged periods of time, for which chemical smoothing techniques can be applied to avoid skin irritation.
The sixth and final step, Evaluation, involves assessing the client’s satisfaction with the 3D printed AD by means of the D-QUEST questionnaire [21]. This is the Dutch version of the QUEST [22], a commonly used questionnaire to evaluate users’ satisfaction with assistive technology. The evaluation is done at least three weeks after delivery of the AD, together with the client (or, if necessary, with a family member or guardian), and under the supervision of the OT.
The concrete application of the Flemish workflow is described by Saey et al. [20] for two cases, a bespoke joystick handle for an electric wheelchair and a plate-raiser.
2.1.2Lessons learned regarding the Flemish workflow
During the research project, the Flemish workflow was used to print ADs for a total of 35 client cases. The evaluations with the D-QUEST showed that the clients were generally satisfied with their custom-made 3D printed AD. In discussions with the stakeholders involved in the research project, OTs generally expressed positive attitudes towards the potential added value of 3D printing technology for the manufacturing of custom-made ADs. They indicated that the biggest challenges for them were related to the design of digital 3D models with CAD software, as well as to the selection of suitable 3D printing techniques and materials, especially given the fact that these techniques are still evolving rapidly. As such, OTs considered it helpful to include basic courses on CAD design and 3D printing in occupational study programmes. Overall, the OTs involved in the project considered 3D printing as an interesting new tool in the OT’s toolbox, which allows them to help clients that cannot be helped with off-the-shelf ADs.
2.2The Italian project
‘3DPrint2Enable’ was an Italian project initiated and coordinated by the University of Modena and Reggio Emilia in 2017. It was co-financed with a private and independent non-profit bank foundation. The project involved a collaboration between the engineering department and the occupational therapy degree course of the university and the rheumatology unit of Modena University Hospital.
Figure 3.
The workflow developed in the Italian project ‘3DPrint2Enable’ (OT
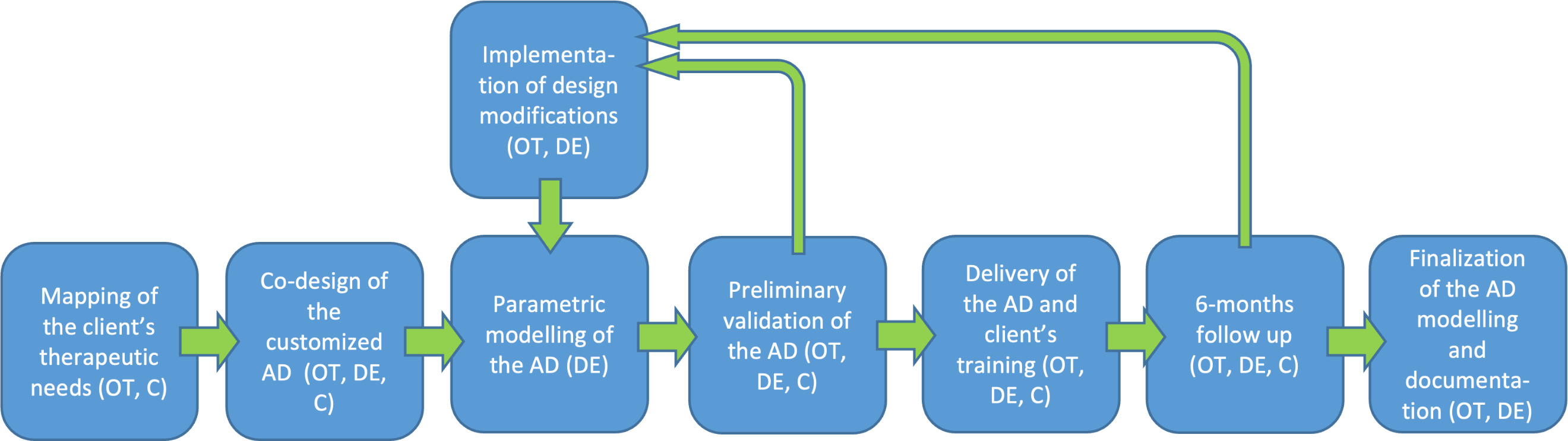
The main objective of the project was to develop custom-made 3D printed ADs for everyday activities by means of an interdisciplinary co-design approach. An action research approach was adopted which involved installation of 3D printers at the rheumatology unit. Based on care needs of real clients, interdisciplinary teams consisting of the client, their OT and an engineer collaborated in the design and manufacturing of custom-made 3D printed ADs.
2.2.1The Italian workflow
The Italian workflow to provide custom-made 3D printed ADs was developed in collaboration with OTs and engineers who combined principles from co-design with principles from occupational therapy. The workflow was applied in the development of several ADs, eight of which were described by [23].
The workflow (Fig. 3) starts when a client is admitted to the occupational therapy service. In the first step, the OT maps the client’s therapeutic needs. If a custom-made AD is deemed suitable for these needs, the second step is that the client, the OT, and an engineer form a co-design team to design and realize a custom-made AD. Co-designing this AD is done in three sub-phases: 1) identification of the key features of the AD; 2) prioritization and translation of the client’s needs into technical requirements and product specifications; and 3) creation of a preliminary 3D model for the AD, using either physical modelling material (e.g. clay) or 3D modelling software. Specific tools such as USERfit [24] and QFD [25] are used as a guide for identifying the key features of the AD and for prioritizing the needs.
In the third step of the workflow, the engineer creates a parametric digital 3D model, which allows to reproduce and adapt the AD if needed (e.g. for another client, or to improve the fit of the AD for the client). This parametric model is printed and presented to the client and the OT for validation (step five) from both a functional and aesthetic point-of-view. In case changes are required, these are immediately made in the parametric model (step six). This process is repeated until the client and OT are satisfied with the design. Once the design is deemed satisfactory, the AD is delivered to the client and, if necessary, the OT provides training and instructions (step seven). In this last phase, the OT uses the PIADS tool [26] to identify the client’s expectations about how the AD will influence their daily life.
Finally, the workflow has two follow-up steps. Three weeks after the 3D printed AD was delivered, the OT checks if the AD is still in use, if there were any problems during its usage, and again uses the PIADS tool to assess how the AD has influenced the client’s life. In addition, the client’s satisfaction with the AD and the supply service are measured with QUEST tool [22]. After six months, the OT once more verifies if the AD is still in use, whether problems have occurred, and whether the client is still satisfied (step eight).
2.2.2Lessons learned regarding the Italian workflow
Figure 4.
The workflow developed in the Dutch research project ‘Occupational Therapy and Do-it-Yourself Technology’.
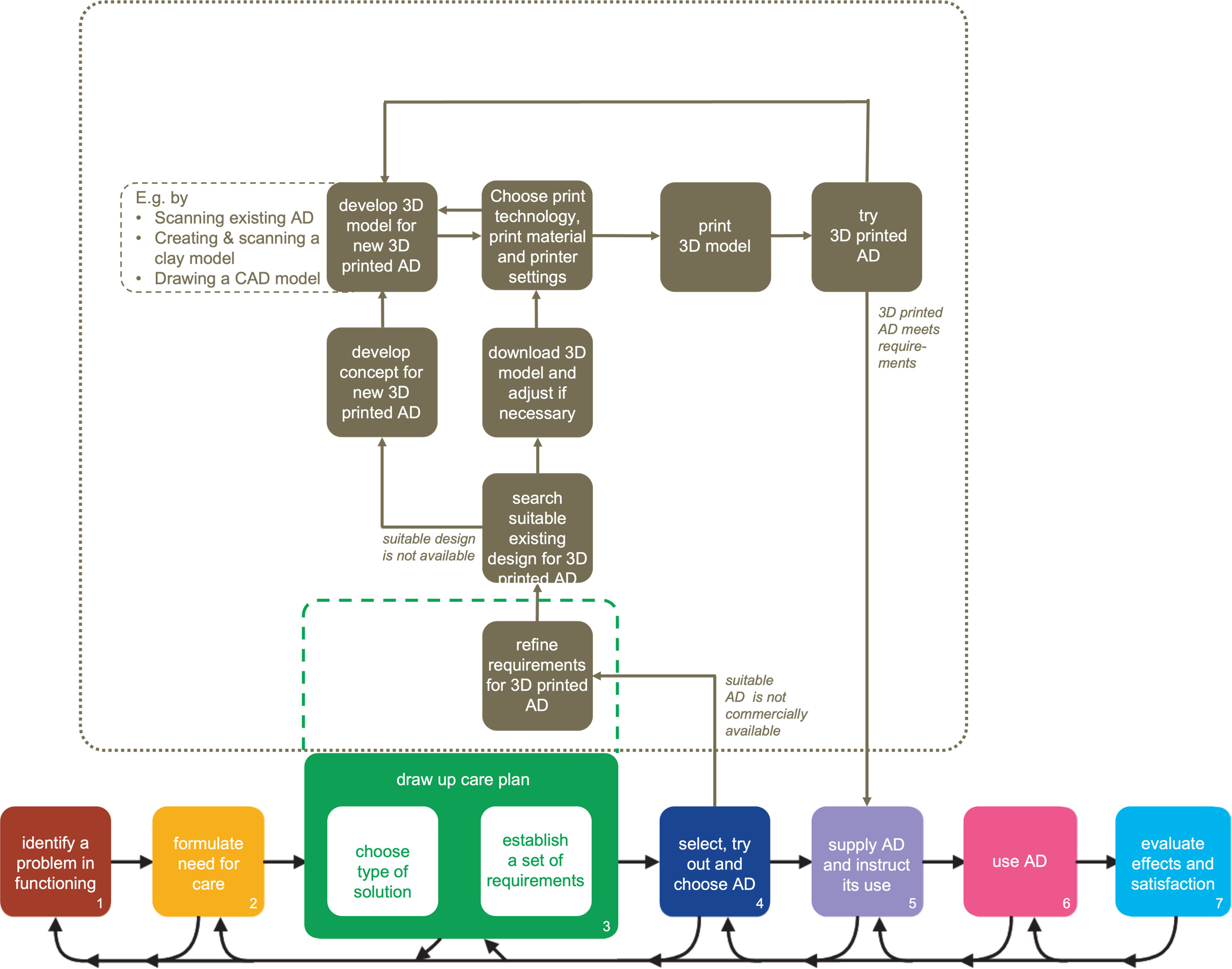
The workflow was used for designing 21 different ADs (seen an example in Fig. 1). Based on these cases, the workflow was iteratively adapted to allow for more interaction with clients during the design and manufacturing process. The research findings [23, 27] regarding these cases are encouraging. After six months, all ADs that had been created were still in use, and clients’ satisfaction generally remained unchanged. In a few cases, client’s satisfaction decreased, which was mostly related to damage to, or premature wear of the AD. Clients’ satisfaction with both the device and the delivery service was high, as were clients’ psychosocial impact scores (i.e. feelings of competence, adaptability and self-esteem due to using the AD). The 12-month follow-up evaluation showed that the psychosocial impact scores had decreased for some clients, which may be explained by the fact that using the AD had become a habit.
2.3The Dutch project
‘Occupational Therapy and Do-it-Yourself Technology’ was a two-year project (2020–2022) in The Netherlands funded by a national funding agency for applied research. The project was coordinated by Zuyd University of Applied Sciences, and involved three rehabilitation centers specialized in child, adult, and elderly rehabilitation, and a company specialized in 3D printing.
The main aim of the project was to research how OTs could integrate DiY technologies, such as 3D printing, into their daily practices of prescribing and delivering ADs. As in the other two projects, an action research approach was adopted for which 3D printers were installed at the three rehabilitation centers and learning communities were established at each center to realize custom-made 3D printed AD (see an example in Fig. 1). In these learning communities, OTs and their clients collaborated with (medical) engineers, adaptation technicians, lecturers and/or students of the engineering department of the university and engineers of the 3D printing company.
2.3.1The Dutch workflow
In the development of the Dutch workflow (see Fig. 4), the starting point was the basic guideline for the provision of assistive products that is used by OTs in the Netherlands [28, 29]. The numbered boxes in the bottom of Fig. 4 represent the seven steps of this guideline.
European regulations prescribe OTs that they can only supply custom-made ADs in case no suitable commercially available solutions are available [30]. Such a lack of suitable commercially available ADs would typically be discovered during the 4th step of the Dutch guideline. Therefore, new steps for creating custom-made 3D printed ADs were added to the workflow after this step (the boxes in the upper part of Fig. 4). These new steps were roughly based on the Additive Manufacturing Process [31]. This process describes product development involving additive manufacturing machines (such as a 3D printer) in eight steps: 1) conceptualization and creation of a digital 3D model; 2) conversion of the 3D model to a standard file type for 3D printing; 3) adjusting the printer settings; 4) sending the file to the 3D printer and refining it if necessary; 5) printing the product; and 6–8) removing the product from the printer and manually finishing it.
The steps in the Dutch workflow that describe how custom-made 3D printed ADs are designed and manufactured start with the decision whether 3D printing would be a suitable technique for creating a custom-made AD for a particular client. For this purpose, the set of requirements that is established in accordance with the existing Dutch guideline is further refined by establishing technical quality requirements to determine whether 3D printing is a viable option for creating the AD.
Once the technical requirements are clear, and 3D printing a custom-made AD is deemed feasible, the next step is to search whether digital designs for similar or suitable ADs exist already, that could serve as a starting point (e.g. models that have been shared by others via online repositories like Thingiverse.com, under licenses that allow for using and editing). In case suitable designs are found, these are downloaded and adjusted according to the set of requirements. If no suitable models exist, a concept for an entirely new 3D printed AD is co-designed by the client, the OT and a 3D printing expert. Once a concept for a new AD is generated, it is subsequently translated to a digital 3D model.
This creation of a digital 3D model for an entirely new 3D printed AD can be done in several ways. In the research project, participants experimented with 3D scanning existing AD, creating and 3D scanning clay models, and drawing digital 3D models from scratch with CAD software. Once the digital 3D model of the new (or adjusted) AD is finished, suitable printing technologies, printing materials and printer settings are selected, after which the digital file of the 3D model is sent to the printer. After printing, the client and OT test the AD, and further adjustments are made to the 3D model if required. Once the 3D printed AD meets all requirements, and both the client and the OT are satisfied, the traditional Dutch guideline for providing ADs is picked up again at the fifth step, in which the OT hands over the device to the client with instructions for the intended use.
2.3.2Lessons learned regarding the Dutch workflow
During the Dutch project, semi-structured interviews were conducted with the participants of all learning communities (OTs and engineers) who were asked to reflect on their experiences with the workflow. At that time, about 20 ADs were printed, including several hand grips for cutlery, an adapted game controller, writing aids, a pincer to open small butter cups, and a cup holder (see Fig. 1). Although their experiences with designing, printing and post-processing were still quite limited, participants indicated that they found the workflow to be clear and complete. Furthermore, participants considered interdisciplinary collaboration between stakeholders with healthcare backgrounds and stakeholders with engineering backgrounds to be essential for designing and manufacturing custom-made 3D printed ADs. They all enjoyed the ongoing collaborations and indicated that they did not deem it feasible for OTs and clients to complete the entire design and manufacturing process on their own. They especially felt that collaboration with engineers is important when designing digital 3D models and when technical issues arise.
We found that collaboration is important in all steps of the workflow. In steps 1 and 2 of the original guideline, OTs are usually in the lead. Once technical requirements of the AD are established, the need for cooperation with engineers emerges, as both clinical and technical expertise is required. The nature and intensity of the collaborations between OTs and engineers seemed to depend on several factors of the case at hand. In some cases, collaboration continued through the remainder of the 3D printing steps, while in other cases, OTs performed the step of testing the 3D printed ADs or prototype themselves and gave feedback on the effect of the solution and satisfaction of the client to the engineer. This differed between the learning communities and depended on the competencies and preferences of the participants involved. OTs and clients in general were satisfied with the 3D printed ADs, as they looked nice and professional.
Involvement of clients varied between the learning communities. In one learning community, clients themselves came up with a request for an AD. These clients were able to communicate and talk with the OT and engineer about their wishes and ideas. In the other communities, most clients were not involved due to cognitive impairments (e.g. of nursing home residents) or the age of the participants (e.g. very young children of 0–4 years old).
Since May 2020, the European Medical Devices Regulation (MDR) [30] applies for all organizations who manufacture ADs for their clients. Several tools (guideline and templates) were developed in the Dutch research project that facilitate OTs in manufacturing custom-made ADs while complying to this MDR, which we describe in [32]. During the development of these support tools, OTs expressed serious concerns about the feasibility of complying with the MDR in practice, as this involves detailed documentation and is very time consuming.
3.Lessons learned from the three workflows
In this section we compare the three workflows that resulted from the Flemish, Italian and Dutch research projects. Our main aim with this comparison was to identify similarities and differences, in order to formulate general recommendations for implementing 3D printing as a common approach in AD delivery processes.
3.1The three workflows compared
The comparison of the three workflows mostly shows striking similarities, especially given the fact that the workflows were developed independently from each other in different research projects, and in different countries and contexts. The steps for designing and manufacturing custom-made 3D printed ADs that were added to existing AD delivery guidelines in the Dutch and Italian workflows are highly similar to the steps in the Flemish workflow. Most importantly, all workflows include separate steps for co-designing a digital 3D model, steps for iteratively refining the model based on feedback by clients and OTs, and steps for producing the final 3D printed AD.
We also noticed differences between the workflows. Some of these are related to the way each workflow breaks up the main steps mentioned above into smaller steps. The Flemish and Dutch workflows, for instance, include a separate step for conceptual design, where the Italian workflow combines conceptual design with the design of a digital 3D model for the AD in one co-design step.
Other differences seem to stem from differences in the nature of the research projects. The Flemish workflow focuses slightly more on the technical details compared to the Italian and Dutch workflows, specifically on printing materials and techniques. This may be explained by the fact that the Flemish research team consisted of several engineers and one OT, while the main researchers in the Dutch research team, for instance, were OTs. Also related to the background or the researchers involved, some of the technical elements that are explicitly identified in the Italian and Dutch workflows were not included in the Flemish workflow, as these were considered to be extremely common from an engineer’s point-of-view (e.g. the option of using existing digital 3D models as a starting point).
The comparison of the workflows, the evaluations carried out during the research projects, and joint reflections on our experiences during the research projects, led us to formulate several recommendations. Below, we discuss these recommendations for designing and manufacturing custom-made 3D printed ADs in practice, and for supporting the collaboration between OTs, clients, and designers and/or engineers.
3.2Recommendations for 3D printing custom-made ADs
First and foremost, we believe that designing and manufacturing custom-made 3D printed ADs is a process that is best done using a user-centered co-design approach. More specifically, design-for-one approaches [33, 34] may provide valuable inspiration for methods and approaches in this respect, as the starting point for designing a custom-made 3D printed AD is usually a specific need of one individual client, rather than a general need of a group of potential clients.
Second, based on the workflows we have presented in this paper, designing and manufacturing custom-made 3D printed ADs requires close collaboration of an interdisciplinary design team. As knowledge from a clinical perspective, a user experience perspective, and a technical design perspective should be combined in one optimized design, such a team should consist of at least a clinician (e.g. OT, rehabilitation therapist, …), a client (and possibly informal caregivers), and a designer or engineer specialized in 3D printing. Consequently, workflows should allow each of these stakeholders to contribute from their own field of expertise in the different steps of the process.
As such an interdisciplinary team is likely to include novice users of 3D printing technology, the workflow that the team follows should explicitly cater for different levels of skills and knowledge and different individual learning goals regarding 3D printing. As such, workflows should be flexible in terms of the roles that different team members play in each step. For instance, when an OT is involved in designing and manufacturing a custom-made 3D printed AD for the first time, they (and their client) are likely to know and understand very little of the technical aspects of designing and 3D printing objects. In such a case, the designer or engineer in the team will have a leading role in many steps of the process. However, OTs who have designed and manufactured several custom-made 3D printed ADs might have acquired some design and manufacturing skills, and may be able to perform parts of the process themselves, as was found in the Flemish and the Dutch projects. Therefore, we recommend that workflows allow each stakeholder to contribute and collaborate in a flexible way, facilitating the alternation of the leading role.
As collaboration can be quite complex, we feel that it is important to provide interdisciplinary design teams with sufficient support tools. The workflows presented in this paper were all developed in combination with several tools and support material, including – but not limited to – instruction materials for novice 3D printer users, tools facilitating collaborations between clinicians, clients, and engineers/designers, connections with databases offering existing 3D models for ADs that can be used as a starting point, templates and guidelines to support compliance with medical regulations, etc. [20, 23, 32, 35].
On a more technical level, workflows for designing and manufacturing custom-made 3D printed ADs should include steps to translate clients’ needs into functional and technical requirements. This is a step that requires close collaboration between clinicians and designers and/or engineers, as clinical requirements need to be translated into technical design requirements. This step will also often involve tailoring the design of an AD to the client’s physical morphology as well as to their personal preferences (which may address a range of product qualities, including aesthetics). As such, the client needs to be involved closely in this step.
Building on the previous recommendation, workflows should include guidance to make sure that considerations regarding the choice of print technologies, print materials, printer settings and finishings meet both clinical requirements (including medical regulations) and user requirements. Regarding the clinical requirements, it is important to consider whether custom-made 3D printed ADs will get into contact with (e.g.) skin, or with food, as this implies certain hygiene requirements for both material and design [30]. Furthermore, one should carefully consider for which activities and purposes a custom-made AD will be used to determine which forces will be exercised upon the AD. In this respect, the European Medical Devices Regulation requires detailed documentation of both the design and manufacturing process, and of the evaluations that have been carried out to ensure product safety. We recommend – as mentioned above – to offer support tools that guide the design team in making sure that the AD they are designing and manufacturing is indeed safe for use, that sufficient tests and evaluations are carried out, and that the design and manufacturing process is documented according to the legal requirements. The toolbox accompanying the Dutch workflow contains dedicated templates for this purpose [32]. In addition, it is important that workflows include systematic testing to assess whether 3D printed ADs meet user requirements (e.g. criteria regarding aesthetics, comfort, usability, etc.).
Finally, we offer two more general suggestions. The first addresses the extent to which a custom-made 3D printed AD is novel. In countries in the European Union, the MDR prevents clinicians to create custom-made ADs for their clients whenever suitable products are commercially available [30]. This implies that a workflow for designing and manufacturing custom-made 3D printed ADs aimed at health care professionals by definition involves the design of novel ADs, or the adaptation of existing (3D printed) ADs, and excludes simply printing identical copies of off-the-shelf ADs. However, using existing digital 3D models or 3D scans of prefabricated ADs proved to be a good starting point for designing and manufacturing custom-made 3D printed ADs. Also, clients are not subject to the MDR if they decide to print an AD without intervention of a clinician. Therefore, we recommend that workflows include steps that allow for reuse and adaptation of existing digital 3D models that can be imported from dedicated databases offering AD models (Thingiverse.com offers such models, for instance).
Finally, in all three research projects leading to the workflows discussed in this paper, researchers encountered client cases for which 3D printing was found not to be a suitable manufacturing technique. Therefore, we suggest that workflows for designing and manufacturing custom-made 3D printed ADs include a clear go/no go decision as to whether 3D printing is the most suitable manufacturing technique for creating a solution for a specific functional problem. Such a go/no go decision may be based on criteria such as complexity of the problem, quality aspects (hygiene, safety), expected time investment, costs, etc. Assessing these issues specifically and early in the design process may help to prevent spending resources on a design process that will later turn out to be wasted.
4.Discussion and conclusion
In this paper we presented and compared three workflows for designing and manufacturing custom-made 3D printed ADs by OTs. Based on this comparison and on our experiences, we have formulated general recommendations directed to interdisciplinary teams of clients (and informal caregivers), their OTs, and designers/engineers specialized in 3D printing. In each of the three projects discussed in this paper, researchers concluded that implementing 3D printing in existing AD delivery systems provides a clear added value for custom-made AD. The clear similarities that were found between the workflows, as well as the positive experiences that OTs reported, suggest that the proposed workflows offer a usable approach for designing and manufacturing custom-made 3D printed ADs.
The differences between the workflows show that they offer flexibility to adapt the design and manufacturing process to national differences, or even to individual differences based on the preferences of the stakeholders involved. In addition, these differences provide starting points for further development and improvement of the workflows, as well as for the actual implementation of such workflows in practice. Most importantly, in this respect, we recommend future research to focus on the roles of all stakeholders in collaborative design and manufacturing processes, and on ways to further facilitate such collaboration. Another important research topic involves the question of how designing and manufacturing custom-made 3D printed ADs can be implemented in practice while ensuring economic viability as well as durable collaborations that can adapt to ongoing technical developments and changes in healthcare systems.
What is important to keep in mind, is the fact that all three workflows were developed in European countries, and as such are tailored to highly similar legal frameworks, most importantly the Medical Devices Regulation issued by the European Parliament [30]. This regulation dictates that custom-made 3D printed ADs are legally seen as medical devices and need to comply with specified safety and documentation requirements. It remains unclear how the process outlined in the workflows translates to non-European countries, which may have different regulations. In this respect, it would be especially interesting to further understand how the workflows generalize to emerging economies, for which it has been argued before [34] that the added value of 3D printing for providing ADs is particularly promising. Advantages such as the ability to create one’s own AD locally, and using (or recycling) available materials, may allow for an entirely new and more accessible category of ADs in this context. We expect that the workflows that we discussed in this paper will still be usable – at least partly – as designing and manufacturing custom-made 3D printed ADs will always involve collaboration between different stakeholders, as well as the basic steps of designing or adapting a 3D model, printing and finishing the AD, etc.
Besides researching whether the workflows allow for implementation in other national, cultural, or economic contexts, the workflows presented need further evaluation themselves. The same holds for the long-term use of, and satisfaction with, 3D printed AD, which was only evaluated to some extent in the Italian project. Such evaluations need to be performed systematically on several levels, including the quality of the manufactured ADs, clients’ and OTs’ satisfaction with such ADs, and the user experiences of all stakeholders involved in the design and 3D printing process. In addition, the workflows might need to be extended in order to further support interdisciplinary design teams to make suitable material choices and to test the safety of their designs (e.g. in terms of forces, impact of temperature, moist, sunlight, etc.), which is a prerequisite of the previously mentioned Medical Devices Regulation.
Another question for future research that we consider to be important is the sharing of ideas and designs for custom-made 3D printable ADs. The current workflows now refer to existing databases such as Thingiverse.com, but these databases have not specifically been set up for 3D printed ADs. It remains unclear how ideas and designs can be shared according to open design principles, while simultaneously complying with national and international legal frameworks, while allowing designers to protect their intellectual property.
Summarizing, the three workflows presented in this paper provide a convincing starting point for interdisciplinary design teams who wish to embark on 3D printing custom-made ADs. In addition, the development and comparison of these workflows raises many questions that require further research. We hope that this paper helps interdisciplinary teams of clinicians, clients, and 3D printing experts around the world to improve their workflows and collaborations, and as such to create better custom-made 3D printed ADs. Furthermore, we hope to have inspired researchers in the fields of 3D printing and assistive technology to further explore the opportunities for designing and manufacturing custom-made 3D printed ADs.
Author contributions
CONCEPTION: Karin Slegers, Tessa Delien, Valentina Bettelli, Monique Lexis, Tom Saey and Ramon Daniëls.
PERFORMANCE OF WORK: Karin Slegers, Tessa Delien, Valentina Bettelli, Monique Lexis and Tom Saey.
INTERPRETATION OR ANALYSIS OF DATA: Karin Slegers, Tessa Delien, Valentina Bettelli, Monique Lexis and Tom Saey.
PREPARATION OF THE MANUSCRIPT: Karin Slegers, Tessa Delien, Valentina Bettelli, Monique Lexis, Tom Saey, David Banes and Ramon Daniëls.
REVISION FOR IMPORTANT INTELLECTUAL CONTENT: Karin Slegers, Tessa Delien, Valentina Bettelli, Monique Lexis, Tom Saey, David Banes and Ramon Daniëls.
SUPERVISION: Ramon Daniëls.
Ethical considerations
The research performed in the Flemish project was approved by the Ethical Review Committee of Jessa Hospital (19.57/reva19.02). Participants or their legal guardians gave informed consent to participate in the study before taking part.
The research performed in the Italian project was approved by the Modena and Reggio Emilia Ethical Committee. Participants or their legal guardians gave informed consent to participate in the study before taking part.
The research performed in the Dutch project was approved by the joint Medical Research Ethics Committee of Zuyderland Hospital and Zuyd University of Applied Sciences (METCZ20200197). Participants or their legal guardians gave informed consent to participate in the study before taking part.
Acknowledgments
The Flemish research project Assist3D: 3D Printing of Bespoke Aids (HBC.2018.0039) was funded by Flanders Innovation & Entrepreneurship (VLAIO).
The Italian research project 3DPrint2Enable (SIME 2017.0345) was funded by Fondazione Cassa di Risparmio di Modena.
The Dutch research project Occupational Therapy and Do-it-Yourself Technology (RAAKPub06.013) was funded by the Taskforce for Applied Research SIA.
Conflict of interest
The authors have no conflicts of interest to report.
References
[1] | Brandt Å, Hansen EM, Christensen JR. The effects of assistive technology service delivery processes and factors associated with positive outcomes – a systematic review. Disabil Rehabil Assist Technol. (2020) ; 15: : 590-603. doi: 10.1080/17483107.2019.1682067. |
[2] | Radomski M, Trombly Latham CA. Occupational Therapy for Physical Dysfunction. 7th ed. Baltimore: Lippincott Williams & Wilkins; (2014) . |
[3] | Larsson Ranada Å, Lidström H. Satisfaction with assistive technology device in relation to the service delivery process – A systematic review. Assistive Technology. (2019) ; 31: : 82-97. doi: 10.1080/10400435.2017.1367737. |
[4] | Tedesco Triccas L, McLening B, Hendrie W, Peryer G. Is there a standard procedure for assessing and providing assistive devices for people with neuro-disabling conditions in United Kingdom? A nation-wide survey. Disabil Health J. (2019) ; 12: : 93-7. doi: 10.1016/j.dhjo.2018.08.003. |
[5] | Federici S, Borsci S. Providing assistive technology in Italy: the perceived delivery process quality as affecting abandonment. Disability and Rehabilitation: Assistive Technology. (2016) ; 11: : 22-31. doi: 10.3109/17483107.2014.930191. |
[6] | Desmond D, Layton N, Bentley J, Boot FH, Borg J, Dhungana BM, et al. Assistive technology and people: a position paper from the first global research, innovation and education on assistive technology (GREAT) summit. Disabil Rehabil Assist Technol. (2018) ; 13: : 437-44. doi: 10.1080/17483107.2018.1471169. |
[7] | Pousada García T, Garabal-Barbeira J, Porto Trillo P, Vilar Figueira O, Novo Díaz C, Pereira Loureiro J. A Framework for a New Approach to Empower Users Through Low-Cost and Do-It-Yourself Assistive Technology. Int J Environ Res Public Health. (2021) ; 18: : 3039. doi: 10.3390/ijerph18063039. |
[8] | Berman B. 3-D printing: The new industrial revolution. Business Horizons. (2012) ; 55: : 155-62. doi: 10.1016/j.bushor.2011.11.003. |
[9] | Sandström CG. The non-disruptive emergence of an ecosystem for 3D Printing – Insights from the hearing aid industry’s transition 1989–2008. Technological Forecasting and Social Change. (2016) ; 102: : 160-8. |
[10] | Creylman V, Muraru L, Pallari J, Vertommen H, Peeraer L. Gait assessment during the initial fitting of customized selective laser sintering ankle foot orthoses in subjects with drop foot. Prosthet Orthot Int. (2013) ; 37: : 132-8. doi: 10.1177/0309364612451269. |
[11] | Michalski MH, Ross JS. The shape of things to come: 3D printing in medicine. JAMA. (2014) ; 312: : 2213-4. doi: 10.1001/jama.2014.9542. |
[12] | Dawood A, Marti Marti B, Sauret-Jackson V, Darwood A. 3D printing in dentistry. Br Dent J. (2015) ; 219: : 521-9. doi: 10.1038/sj.bdj.2015.914. |
[13] | Hofmann M, Williams K, Kaplan T, Valencia S, Hann G, Hudson SE, et al. “Occupational therapy is making”: Clinical rapid prototyping and digital fabrication. New York, New York, USA: ACM Press; (2019) . pp. 1-13. doi: 10.1145/3290605.3300544. |
[14] | Buehler E, Branham S, Ali A, Chang JJ, Hofmann MK, Hurst A, et al. Sharing is Caring: Assistive Technology Designs on Thingiverse. In: Proceedings of the 33rd Annual ACM Conference on Human Factors in Computing Systems. New York, NY, USA: ACM; (2015) . pp. 525-34. doi: 10.1145/2702123.2702525. |
[15] | McDonald S, Comrie N, Buehler E, Carter N, Dubin B, Gordes K, et al. Uncovering Challenges and Opportunities for 3D Printing Assistive Technology with Physical Therapists. New York, New York, USA: ACM Press; (2016) . pp. 131-9. doi: 10.1145/2982142.2982162. |
[16] | Slegers K, Kouwenberg K, Loučova T, Daniels R. Makers in Healthcare: The Role of Occupational Therapists in the Design of DIY Assistive Technology. In: Proceedings of the 2020 CHI Conference on Human Factors in Computing Systems. New York, NY, USA: Association for Computing Machinery; (2020) . pp. 1-11. doi: 10.1145/3313831.3376685. |
[17] | Desideri L, Stefanelli B, Bitelli C, Roentgen U, Gelderblom G-J, de Witte L. Satisfaction of users with assistive technology service delivery: An exploratory analysis of experiences of parents of children with physical and multiple disabilities. Developmental Neurorehabilitation. (2016) ; 19: : 255-66. doi: 10.3109/17518423.2014.988303. |
[18] | de Witte L, Steel E, Gupta S, Ramos VD, Roentgen U. Assistive technology provision: towards an international framework for assuring availability and accessibility of affordable high-quality assistive technology. Disabil Rehabil Assist Technol. (2018) ; 13: : 467-72. doi: 10.1080/17483107.2018.1470264. |
[19] | Andrich R, Mathiassen NE, Hoogerwerf EJ, Gelderblom GJ. Service delivery systems for assistive technology in Europe: An AAATE/EASTIN position paper. Technology and Disability. (2013) ; 25: : 127-46. doi: 10.3233/TAD-130381. |
[20] | Saey T, Creylman V, Sevit R, Raeve ED, Arenas DM, Muraru L. A digital workflow for personalized design of the interface parts integrated in a powered ankle foot orthosis (PAFO). In: 2021 43rd Annual International Conference of the IEEE Engineering in Medicine Biology Society (EMBC). (2021) . pp. 4840-3. doi: 10.1109/EMBC46164.2021.9631049. |
[21] | Wessels RD, de Witte LP, Weiss-Lambrou R, Demers L, Wijlhuizen G. A Dutch version of QUEST (D-QUEST) applied as a routine follow-up within the service delivery process. Helsinki: (1998) . |
[22] | Demers L, Weiss-Lambrou R, Ska B. Development of the Quebec User Evaluation of Satisfaction with assistive Technology (QUEST). Assist Technol. (1996) ; 8: : 3-13. doi: 10.1080/10400435.1996.10132268. |
[23] | Gherardini F, Mascia MT, Bettelli V, Leali F. A Co-Design Method for the Additive Manufacturing of Customised Assistive Devices for Hand Pathologies. J Integr Des Process Sci. (2018) ; 22: : 21-37. doi: 10.3233/jid-2018-0002. |
[24] | Poulson D, Richardson S. USERfit – a framework for user centred design in assistive technology. Technology and Disability. (1998) ; 9: : 163-71. doi: 10.3233/TAD-1998-9307. |
[25] | Gherardini F, Renzi C, Leali F. A systematic user-centred framework for engineering product design in small- and medium-sized enterprises (SMEs). Int J Adv Manuf Technol. (2017) ; 91: : 1723-46. doi: 10.1007/s00170-016-9857-9. |
[26] | Demers L, Monette M, Descent M, Jutai J, Wolfson C. The Psychosocial Impact of Assistive Devices Scale (PIADS): translation and preliminary psychometric evaluation of a Canadian-French version. Qual Life Res. (2002) ; 11: : 583-92. doi: 10.1023/a:1016397412708. |
[27] | Gherardini F, Petruccioli A, Dalpadulo E, Bettelli V, Mascia M, Leali F. A Methodological Approach for the Design of Inclusive Assistive Devices by Integrating Co-design and Additive Manufacturing Technologies. (2020) . Available from: https://www.semanticscholar.org/paper/A-Methodological-Approach-for-the-Design-of-Devices-Gherardini-Petruccioli/5fe3cd1df381f83e370f9d7155e6217aec4930e5. |
[28] | Heerkens YF, Bougie T, Jonker HK. A basic guideline for the provision of assistive products in the Netherlands. Everyday Technology for Independence and Care. (2011) ; 1149-54. doi: 10.3233/978-1-60750-814-4-1149. |
[29] | Nictiz. Procesbeschrijving Hulpmiddelenzorg [Description of the process of providing care related to assistive products]. (2009) . |
[30] | European Parliament. MDR 2017/745. (2017) . |
[31] | Gibson I, Rosen D, Stucker B. Generalized Additive Manufacturing Process Chain. Additive Manufacturing Technologies: 3D Printing, Rapid Prototyping, and Direct Digital Manufacturing. Springer New York; (2015) . pp. 43-61. doi: 10.1007/978-1-4939-2113-3_3. |
[32] | De Jone I, Lexis M, Slegers K, Tuijthof G. Medical Device Regulation: Requirements for Occupational Therapists in The Netherlands who Prescribe and Manufacture Custom-made Devices. under review. |
[33] | Den Haan MC, Brankaert R, Lu Y. Design for One: Personalisation and Experiences of Design Researchers and Participants. In: Proceedings of the 6th International Conference on Design Creativity, ICDC 2020. (2020) . pp. 279-86. doi: 10.35199/ICDC.2020.35. |
[34] | Wilkinson A. Designing for one: how designing for one enriches the student design process. PhD thesis. University of Leeds; (2020) . |
[35] | Case Study Overview of the ASSIST3D Project. ASSIST3D n.d. Available from: https://www.assist3d.be/cases.html (accessed December 15, 2022). |
[36] | Zuniga J, Katsavelis D, Peck J, Stollberg J, Petrykowski M, Carson A, et al. Cyborg beast: a low-cost 3d-printed prosthetic hand for children with upper-limb differences. BMC Research Notes. (2015) ; 8: : 10. doi: 10.1186/s13104-015-0971-9. |
[37] | ten Kate J, Smit G, Breedveld P. 3D-printed upper limb prostheses: a review. Disability and Rehabilitation: Assistive Technology. (2017) ; 12: : 300-14. doi: 10.1080/17483107.2016.1253117. |