Targeted neurorehabilitation strategies in post-stroke aphasia
Abstract
Background:
Aphasia is a debilitating language impairment, affecting millions of people worldwide. About 40% of stroke survivors develop chronic aphasia, resulting in life-long disability.
Objective:
This review examines extrinsic and intrinsic neuromodulation techniques, aimed at enhancing the effects of speech and language therapies in stroke survivors with aphasia.
Methods:
We discuss the available evidence supporting the use of transcranial direct current stimulation (tDCS), repetitive transcranial magnetic stimulation, and functional MRI (fMRI) real-time neurofeedback in aphasia rehabilitation.
Results:
This review systematically evaluates studies focusing on efficacy and implementation of specialized methods for post-treatment outcome optimization and transfer to functional skills. It considers stimulation target determination and various targeting approaches. The translation of neuromodulation interventions to clinical practice is explored, emphasizing generalization and functional communication. The review also covers real-time fMRI neurofeedback, discussing current evidence for efficacy and essential implementation parameters. Finally, we address future directions for neuromodulation research in aphasia.
Conclusions:
This comprehensive review aims to serve as a resource for a broad audience of researchers and clinicians interested in incorporating neuromodulation for advancing aphasia care.
1Targeted neurorehabilitation strategies in post-stroke aphasia
Conservative estimates indicate that 2,500,000 people in the US are currently living with an acquired communication disorder, called aphasia (Simmons-Mackie & Cherney, 2018). Aphasia is a debilitating disorder that can disrupt multiple aspects of language, such as speaking, understanding, writing or reading (Brookshire, Wilson, et al., 2014). The number of people affected by aphasia is likely to grow, because an estimated 250,000 (32%) of new stroke survivors in the US each year are diagnosed with this acquired language impairment, majority of whom demonstrate persisting deficits at least 1 year later (Flowers et al., 2016; Pedersen et al., 2004).
Clinically available speech and language therapies (SLT) are the current standard of care but provide modest benefits at best. The outpatient therapy services available to aphasia survivors last up to 5-6 months after stroke, or up to insurance coverage limits (Babbitt et al., 2015). After this time, aphasia survivors are often left to live with residual language impairments, without continuous access to aphasia therapy (Abrams, 2017; Babbitt et al., 2015; L. Johnson et al., 2019). There is a growing gap between the need for language rehabilitation in a large population living with aphasia and the current availability of highly effective and accessible treatments. In the current review, we focus on neuromodulation techniques that aim to enhance the effects of SLT in people with post-stroke aphasia (PWA). Our primary goal is to foster in-depth understanding of neuromodulation techniques and procedures relevant to PWA, and to discuss methodologies to help increase the effectiveness of these techniques. This review was inspired by our (PSB, OB, AK) symposium presentation at the 2022 annual meeting of the American Society for Neurorehabilitation and the subsequent questions from the audience.
The term neuromodulation in our review refers to the process of modifying or regulating brain activity using extrinsic or intrinsic methodologies paired with behavioral strategies. In the context of this review, extrinsic methods of neuromodulation alter excitability of neuronal networks by delivering an external electrical or magnetic pulse generated by a noninvasive brain stimulation (NIBS) device. The magnitude of extrinsic neuromodulation depends on the strengths of the applied pulse. In contrast, intrinsic methodologies, such as neurofeedback, promote network activity that is generated and controlled by neurons within that neural network or within other connected brain areas. Thus, the magnitude of intrinsic neuromodulation depends on present and past network activity which determines its current state. The methodologies discussed are restricted to NIBS (electrical, electromagnetic) and neurofeedback approaches that are both safe and result in short and long-term alterations in brain activity and behavior (M.D. Johnson et al., 2013; Krames et al., 2009).
In this review, we will focus on procedural aspects of two neuromodulation techniques, namely transcranial direct current stimulation (tDCS) and functional MRI (fMRI) related real-time neurofeedback (fMRI NFB). In the interest of space, while we will discuss repetitive transcranial magnetic stimulation (rTMS), the rTMS sections will be relatively concise and focused. We will also evaluate specialized methods that are implemented using these techniques to optimize therapeutic benefits in PWA.
Briefly, tDCS and rTMS are extrinsic neuromodulation techniques because they use an external device to generate the neuromodulation effects. TDCS uses subthreshold, electrical stimulation to alter the excitability of the stimulated neuronal populations (Nitsche & Paulus, 2001). RTMS uses time-varying magnetic fields to induce an electrical current in the cortical neurons and generate action potentials (Hallett, 2007). Whereas real-time fMRI NFB is an intrinsic neuromodulation technique because it uses self-generated regulation strategies in combination with feedback about concurrent brain activity. This is an innovative approach allowing patients to regulate their own brain activity while being guided by feedback which aims to produce a particular pattern of brain activation.
These methods have all been applied in research settings as potential treatments for PWA. The majority of original tDCS studies and meta-analyses provide compelling evidence in favor of tDCS (Elsner et al., 2019, 2020; Shah-Basak et al., 2016), reporting significant improvements in language functions, including picture naming accuracy and latency (Flöel et al., 2011; Fridriksson, Rorden, et al., 2018; Kang et al., 2011), auditory verbal comprehension (You et al., 2011), spontaneous speech production (Marangolo et al., 2013; Norise et al., 2017) and overall reductions in aphasia severity (Shah-Basak et al., 2015) in PWA. The positive effects of rTMS on language recovery are reported both when administered alone and with SLT (Gholami et al., 2022; Kielar et al., 2022). More recently, learning-induced changes in cognitive and motor function have been demonstrated with repeated efforts to self-regulate brain activity. Early proof-of-concept studies indicate that real-time fMRI NFB may be beneficial for the treatment of post-stroke impairments, including aphasia (Sreedharan et al., 2019).
We provide a scoping overview of the current neuromodulation research with emphasis on individualized approaches and potential for generalization. In the following sections, we begin with in-depth discussions of NIBS parameters that strongly influence the ensuing neuromodulatory effects. Special consideration is given to approaches that localize anatomical targets for the administration of tDCS/rTMS coupled with language therapy to maximize benefits. This includes topics on targeting precise anatomical areas for stimulation and topics on targeting brain areas with residual language function vs. brain areas that exhibit suboptimal post-stroke function, indicated for example by reduced regional cerebral blood flow (CBF) or abnormal electrophysiological signal. We also discuss future directions and recommendations to promote translation of research-based neuromodulation interventions to clinical practice.
In the latter sections, we cover the relatively scarce literature on fMRI NFB in aphasia rehabilitation. While a large number of fMRI NFB publications in healthy adults and in psychiatric populations (e.g., Major Depressive Disorder) (Young et al., 2017) demonstrates efficacy of this neuromodulation technique, only a handful of studies have been published using it for stroke rehabilitation. We discuss these studies, as well as fMRI NFB procedures, important parameters, and promising directions for future research in aphasia.
2Noninvasive brain stimulation (NIBS)
2.1Transcranial magnetic stimulation (TMS)
RTMS uses magnetic fields to noninvasively stimulate neurons in the brain with no serious side effects when applied following published safety parameters. TMS employs the principle of electromagnetic induction. A brief and time-varying magnetic field is delivered via a TMS coil placed on the scalp. This magnetic field penetrates through the skull and an electrical current is induced in the cortical neurons near the coil. The magnitude, direction and precise location of the electrical current is determined by a complex interaction among stimulation intensity, coil shape and coil orientation. This current is sufficient to depolarize affected neuronal membranes and generate action potentials (Hallett, 2007). The intensity, frequency, train duration, and intertrain interval of TMS pulses are important parameters that determine the direction of its effect on cortical excitability. Typically, low-frequency rTMS (< 5 Hertz [Hz]) is characterized by decreased cortical excitability (Chen et al., 1997), whereas high-frequency rTMS (≥5 Hz) is characterized by enhanced excitability (Berardelli et al., 1998). Recently, a new rTMS protocol— theta burst stimulation (TBS)— was introduced which can produce longer lasting and more stable changes in cortical excitability compared to standard rTMS protocols. TBS consists of delivering multiple pulses very rapidly (at 50 Hz or bursts), which can either be interrupted every few seconds (intermittent TBS [iTBS]) or can be uninterrupted (continuous TBS [cTBS]). ITBS typically increases cortical excitability, while cTBS decreases excitability (Huang et al., 2005, 2011). Depending on rTMS parametric settings, the effects after a single session outlast the period of stimulation for a few seconds to up to a few hours. Multiple sessions are needed to affect long-term plasticity (Klomjai et al., 2015).
2.2Transcranial direct current stimulation (tDCS)
TDCS— a subthreshold, electrical stimulation technique— affords safe and tolerable stimulation that is easy to apply, portable and cost-effective. One advantage of tDCS is that it can be easily combined with behavioral interventions. TDCS involves low-intensity electric currents that are delivered for 20–30 minutes via two or more scalp electrodes. Short-term tDCS (20–30 minutes during a single session) induces incremental shifts in resting neuronal membrane potentials and alters neuronal firing rates (Nitsche & Paulus, 2001). Depending on electrode polarity (anode or cathode) and current strength (1 to 2 milliamperes [mA]), tDCS can transiently facilitate or inhibit the excitability of the affected neuronal populations. Similar to rTMS, multiple sessions (5 to 15 sessions) of tDCS typically over 1–3 weeks are needed to promote long-term neural plasticity (Stagg et al., 2018). The changes in resting membrane potentials after a single session of stimulation are short-lasting and are distinct from the lasting neuroplasticity changes associated with protracted treatment protocols. Long-term neural plasticity after multiple sessions of stimulation involves changes in long-term potentiation or depression (LTP/LTD) by strengthening or weakening of activity-dependent synaptic connections through N-methyl-D-aspartate receptor activity (Agboada et al., 2020). In addition, there is emerging evidence indicating that tDCS can modulate neuroplasticity by interacting with brain derived neurotrophic factor (BNDF) gene that facilitates synapse formation (Fridriksson, Elm, et al., 2018; Gersner et al., 2011).
Some PWA respond to tDCS more favorably than others even when parameters such as electrode locations, duration and intensity are kept constant (Chew et al., 2015; Shah-Basak et al., 2015). We have hypothesized that a one-size-fits-all approach to treatment of aphasia, which includes the type of language therapy and the parameter selection for tDCS, is suboptimal and may significantly contribute to the observed interindividual variability (Shah-Basak et al., 2015, 2020, 2021). To maximize treatment effectiveness with tDCS, standardized methods are needed for methodical selection of tDCS parameters (Fig. 1) in individual patients. In this review, we focus on two such parameters of tDCS.
Fig. 1
Parameter and design characteristics involving tDCS treatment studies.
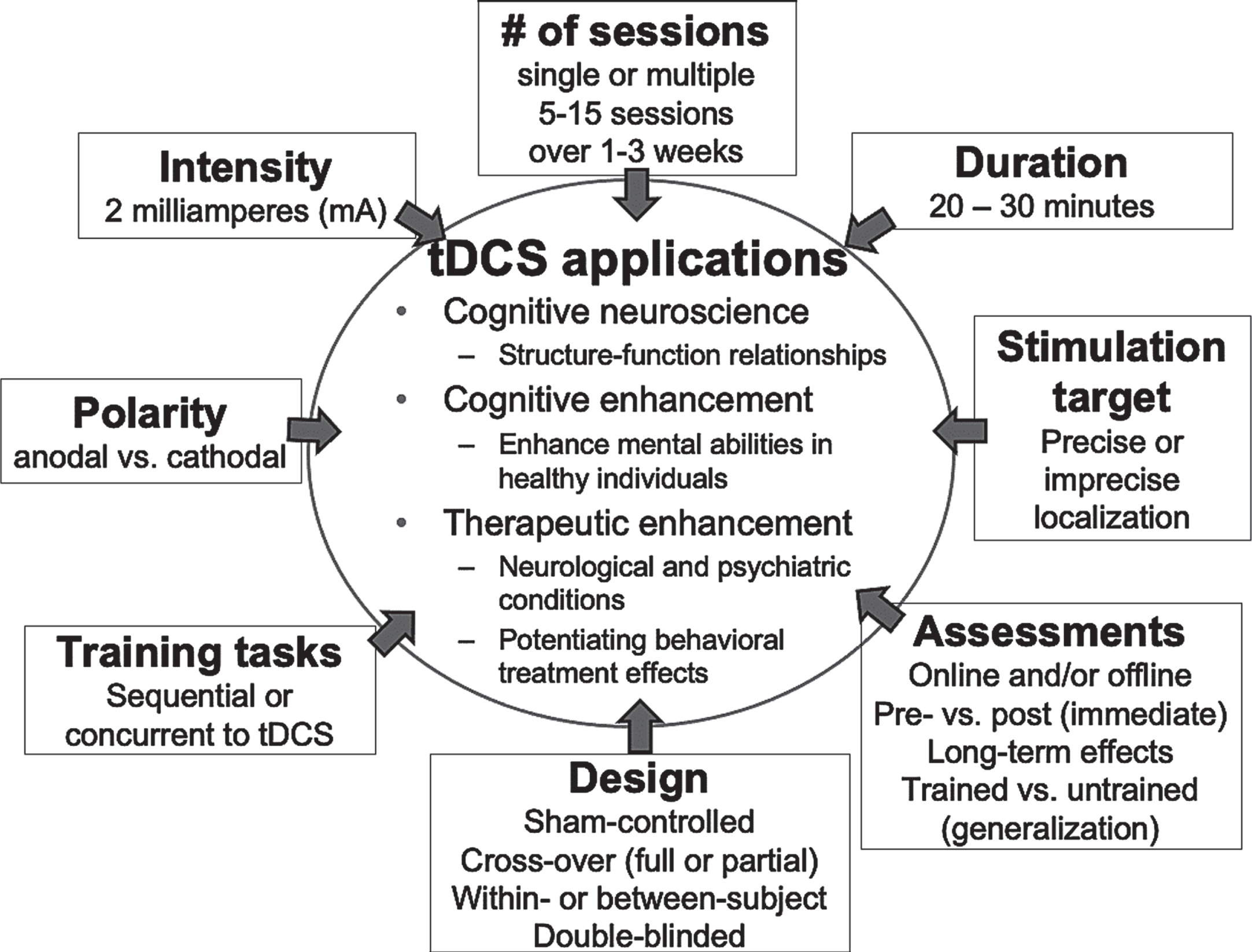
Foremost, the placement of tDCS electrodes is crucial to determining tDCS effects (Shah-Basak et al., 2020). The main issue in most prior studies is the use of approaches that do not respect the boundaries of structural damage or potential differences in language network reorganization across PWA (Kang et al., 2011; Marangolo et al., 2013; Monti et al., 2008; You et al., 2011). This question of individualized targeting becomes more pertinent as tDCS technology moves toward relatively more focal current delivery with high-definition or HD-tDCS. HD-tDCS replaces large rubber electrodes that cover large sections of the brain with multiple smaller electrodes that are placed strategically on the scalp for relatively focal stimulation of brain areas directly underneath the electrodes (Datta et al., 2009; Villamar et al., 2013). Individualized targeting approaches that take structural and functional characteristics into account can help increase the consistency of tDCS treatment response across patients and thus strengthen its efficacy (Shah-Basak et al., 2020). Currently there is no consensus on best practices that can be used for individualized targeting to maximize aphasia recovery using tDCS.
Secondly, our prior work and that of others suggests that cognitive demands of the task during tDCS (or training tasks) can profoundly influence the effects of tDCS (Gill et al., 2015). Evidence indicates that outcomes of tDCS depend on “the state of neuronal activation at the time of stimulation” (Nozari et al., 2014). That is, the neural plasticity resulting from tDCS reflects a dynamic interaction between tDCS and the activity of neural tissue undergoing stimulation (Hsu et al., 2016; Nozari et al., 2014). Two main “active ingredients” that likely determine these effects include the location of tDCS electrodes (stimulation targets) and the cognitive demand of tasks performed during tDCS. This evidence has direct relevance to maximizing tDCS efficacy in PWA. It underscores the need to pair language therapies with targeted tDCS to optimize overall neuromodulatory effects. Relatedly, recent evidence from motor studies indicates that the timing of tDCS in relation to the training tasks (i.e before, during or after task completion) can influence the effects of tDCS (Liao et al., 2020). Thus, pairing of therapies need not be concurrent (tDCS during training) to tDCS but can be sequential (tDCS before training, or tDCS after training).
We discuss different approaches that have been implemented for determining stimulation targets in PWA and behavioral strategies (or SLT) to increase transfer of behavioral treatment effects to clinically meaningful gains (e.g., functional communication) and the current evidence on pairing these strategies with NIBS to boost therapeutic benefits.
3Stimulation target determination
3.1Targeting right or left hemispheres – traditional models of recovery
The review of the emerging literature suggests nuanced roles of the residual left and right hemisphere areas over the course of aphasia recovery. Longitudinal fMRI studies of language in aphasia suggest that stroke may temporarily reduce brain activity and functional connectivity in the affected left hemisphere. For example, Saur et al. (2006) investigated auditory sentence comprehension in 14 PWA using fMRI over 3 sessions (1.8 days post-stroke (dps), 12.1 dps, and 321 dps) (Saur et al., 2006) and found early depression in left brain activity, followed by a subacute increase bilaterally, particularly in the right frontal activity. In the chronic stage more successful language recovery was associated with decreased right hemisphere activation and return to left-lateralized brain activity. Siegel et al. (2016) found that language deficits were associated with decreased left-hemisphere and homotopic interhemispheric functional connectivity assessed 2 weeks post-stroke (Siegel et al., 2016) (see also Sandberg and colleagues (2017) for similar results). Nair et al. (2015) found decreased functional connectivity of 23 bilateral language nodes in acute stroke patients (5 dps), which improved at 4.5 months post-stroke (Nair et al., 2015). Thus, the initial decreases in left hemisphere activation and bilateral connectivity are followed by subsequent increases that accompany recovery.
Evidence on the recruitment of contralesional (right hemisphere) areas during recovery is conflicting, with findings indicating both adaptive and maladaptive roles. Across different neuroimaging studies and task paradigms, activation in right regions in PWA is shown to be functionally homologous to left areas that normally subserve language in healthy individuals. Activation of right-sided regions is shown to support language recovery in some studies (Heath et al., 2012; Mohr et al., 2014). Whereas increasing involvement (or disinhibition) of contralesional, right hemisphere regions in other studies is shown to disrupt the balance in interhemispheric inhibition, suggesting a maladaptive pattern of brain activity. Consistent with this disinhibition account, activation of pars triangularis (PTr, BA45) of the right IFG is shown in some cases to interfere with recovery.
Brain activity following stroke and aphasia is shown to rely on the extent and location of the lesion. As mentioned previously, during the early stages of language recovery, changes in brain activity represent a transient reliance on right-brain homologues (Stockert et al., 2016; Thompson & den Ouden, 2008). But the exact pattern of right hemisphere recruitment may depend on the location of lesions in the left hemisphere (Hartwigsen & Saur, 2019). For example, in the Saur et al. study patients with damage to the left IFG more reliably activated the right homologous area, than those without left frontal damage (Turkeltaub et al., 2011).
Re-organization of the language system following stroke can also involve the spared areas in the left hemisphere that are distant from the lesion. For example, a meta-analysis of neuroimaging studies (Turkeltaub et al., 2011) found that PWA consistently recruit spared areas of the left language network and new left hemisphere areas (see also Hartwigsen and Saur (2019)). The size and location of lesions determines the exact pattern of the involvement of these areas. Adaptive role of perilesional areas (structurally intact areas surrounding the lesion) in reorganized language networks is also reported (Geranmayeh et al., 2016; Saur et al., 2006), such that increased recruitment of the perilesional areas is shown to result in better language outcomes (Fridriksson, 2010; Fridriksson et al., 2010, 2012; Heath et al., 2012). It is thought that these areas may subserve or are able to take on a similar function to the lesioned tissue because of their proximity and therefore, suitability for supporting recovery.
Emerging literature suggests that when enough of the left hemisphere is spared, restoration of left-dominant brain activity patterns tends to be associated with greater language gains (Crosson et al., 2007; Gainotti, 1993; Price & Crinion, 2005; Rubi-Fessen et al., 2015). But limited ‘structural reserve’ (or damage to key left language areas) and factors such as premorbid right hemisphere language dominance may determine how right regions are recruited to support or inhibit language recovery.
Which of these patterns becomes more dominant may also be determined by specific linguistic skills that are impaired or retained (Perani et al., 2003). For example, using magnetoencephalography (MEG), we have shown that recruitment of right homologous temporoparietal areas was associated with better semantic performance, whereas more accurate processing of syntactic information was related to bilateral superior temporoparietal and right frontal activity after stroke (Kielar et al., 2015).
Finally, some studies report the involvement of domain-general functional networks. For example, Dreyer et al. (2021) found that gains on a clinical aphasia assessment following constraint induced language therapy were linearly associated with activity in the default mode network.
Prior NIBS protocols take one or more of these models of recovery into consideration for the selection of stimulation targets, stimulation frequency (rTMS) or polarity (tDCS). Improvements have been reported in response to both facilitation (Flöel et al., 2011) and inhibition of right homologue regions (Kang et al., 2011; Lee et al., 2013; You et al., 2011) and of the left hemisphere regions (Shah-Basak et al., 2015). The most commonly applied protocols using rTMS are inhibitory (low frequency or theta burst) rTMS to the intact right regions with several studies targeting the right PTr (IFG) (Barwood et al., 2013; Georgiou & Kambanaros, 2022; Heikkinen et al., 2019; Heiss et al., 2013; Kindler et al., 2012; Medina et al., 2012; Naeser et al., 2005; Rubi-Fessen et al., 2015; Seniów et al., 2009; Thiel et al., 2013; Tsai et al., 2014; Wang et al., 2014; Winhuisen et al., 2005). These protocols operate under the interhemispheric inhibition hypothesis that suppression of abnormally activated right regions can release spared, perilesional areas for recruitment and thus induce recovery. In a recent meta-analysis of rTMS studies, Kielar et al. (2022) reported that inhibitory rTMS applied to right PTr administered daily over 2-3 weeks resulted in a significant improvement of language skills that persisted for up to 12 months post-intervention in PWA (Kielar et al., 2022). The effects of rTMS were modulated by stroke chronicity and language task. In subacute PWA, the greatest gains were observed for naming, writing, repetition, and speech production. In chronic patients, most reliable improvement was found for naming, and gains on repetition and speech production were less consistent. Excitatory stimulation using high frequency rTMS or theta burst to the right IFG and directly to the left perilesional areas has also facilitated language abilities in PWA (Chieffo et al., 2014; Griffis et al., 2016; Hu et al., 2018; Szaflarski et al., 2011; Turkeltaub, 2015).
A majority of tDCS studies used anodal tDCS to stimulate the left hemisphere regions (Norise & Hamilton, 2016; Shah et al., 2013). Relatively fewer studies have targeted the right regions with anodal or cathodal tDCS. With naming accuracy of nouns as an outcome measure, two recent meta-analyses indicated superior effects of active tDCS compared to sham tDCS (Elsner et al., 2019, 2020; Shah-Basak et al., 2016), whereas one other meta-analysis that only included studies implementing cathodal tDCS (3 studies with a total of 32 participants) found non-significant effects (Otal et al., 2015). A recent pilot trial that compared anodal, cathodal and sham tDCS at 1 mA intensity applied to the left perilesional areas during SLT found greater gains in language skills with both active forms of tDCS compared to sham tDCS (Cherney et al., 2021). This study could not conclusively confirm superiority of one polarity (anodal or cathodal) over the other but noted that cathodal tDCS resulted in increased bilateral brain activity.
The stimulation intensity for tDCS ranged from 1-2 mA in most prior aphasia treatment studies, and a linear relationship was assumed with polarity: anodal-tDCS facilitates and cathodal-tDCS inhibits neuronal excitability. However, recent evidence refutes such straightforward relationships, in particular in response to cathodal tDCS (Batsikadze et al., 2013). The supposition that cathodal-tDCS is inhibitory has been questioned in several studies since the early 2010 s. Batsikadze and colleagues (2013) found unequivocal evidence of reversal in cortical excitability with increasing intensity of cathodal tDCS. They replicated previous findings that 1 mA cathodal tDCS was inhibitory, whereas they found that 2 mA cathodal tDCS was excitatory. Additionally, a meta-analysis of tDCS studies conducted to modulate cognitive processes (as opposed to motor) found that increasing intensity of cathodal tDCS affects cortical excitability in unpredictable ways and that relationship may be nonlinear (Jacobson et al., 2012). In our own work in PWA, we have found improvements after 2 mA cathodal tDCS over left perilesional areas, arguing against the inhibitory effects of cathodal tDCS (Shah-Basak et al., 2015). Consistent with this, the pilot trial that we previously described comparing anodal, cathodal and sham tDCS applied to left areas at 1 mA found that cathodal tDCS resulted in increased brain activity (Cherney et al., 2021). This new understanding of the relationship between intensity and polarity brings under scrutiny the findings from prior studies that applied cathodal tDCS to right homolog areas to reduce their activation (and release the left areas for reintegration during functional reorganization, see (Norise & Hamilton, 2016) for a review), in particular if tDCS was applied at 2 mA intensity.
Another critical point of consideration is that we still do not fully understand the different patterns of neural activity changes in aphasia recovery and their determinants. The findings from a recent review by Wilson and Schneck (2021) attests to this limitation. Wilson and Schneck critically appraised the claims of language reorganization and patterns of brain activity changes in PWA across 86 functional neuroimaging studies. They found that PWA compared to healthy controls show decreased activation in the left language network, including IFG pars opercularis and triangularis and posterior superior temporal gyrus (STG), and increased activation in the right IFG pars opercularis. Increased activation of the left IFG PTr was associated with improved language function. They failed to support the hypothesis that domain-general network activation is associated with aphasia recovery and provided only modest evidence for recruitment of the right homotopic areas during recovery. Overall, the authors noted that their conclusions are constrained by the fact that the majority of currently published studies have important limitations, including confounds related to task performance, the validity of task comparisons and statistical correction for multiple comparisons. With an incomplete understanding of neural reorganization patterns in aphasia, the selection of specific NIBS parameters to induce permanent neural changes underlying recovery remains a challenge. The selection of NIBS parameters is further complicated by the observed variability in lesion, aphasia severity and/or language impairment characteristics. Thus, as we have previously argued, adopting a single stimulation protocol across PWA based on hypothetical models of recovery may be an ineffective strategy.
3.2Individualized targeting
Traditional reorganization models guided earlier, proof-of-concept studies by defining a priori stimulation targets that were the same across all PWA, irrespective of structural and functional integrity of those brain regions in individual PWA. Although this early work did not individualize target localization, the results did help establish feasibility, safety profile and as noted earlier, preliminary efficacy of NIBS in improving linguistic outcomes after stroke. But a large degree of individual variability was reported across these studies in response to NIBS treatments, particularly tDCS, bringing the overall treatment effect sizes down (Li et al., 2015). In the recent decade, the field is undergoing a major paradigm shift with a focus on individual PWA and developing strategies to maximize NIBS’ therapeutic potential with individual patients in mind.
One strategy that we advocate for increasing NIBS efficacy is localization of stimulation targets in individual PWA (or individualized targeting) to reduce heterogeneity in treatment response. Accounting for factors such as lesion location and extent, language impairment profiles, brain activation profiles and planned SLTs, researchers have already implemented several different individualized targeting approaches (Table 1) that we discuss in detail below and have broadly categorized as:
(1) Behavioral targeting;
(2) Targeting for strengthening or enhancing brain function; and
(3) Targeting for normalizing or restoring function (or dysfunction) within the language network.
Table 1
Summary of rTMS and tDCS studies that implemented individualized targeting approaches in post-stroke aphasia
Source | Aphasia characteristics | Stimulation details | Localizer task/ modality | Pre-post Tx imaging modality/task | Assessment time points | Treatment method | Sham | Outcome/activation change post-Tx | Study design |
Behavioral Targeting | |||||||||
rTMS Studies | |||||||||
Hamilton et al., 2010 | n = 1, Age: 61 yrs old, male Chronic: TPO 7 yrs, nonfluent | Protocol: 1 Hz rTMS (1200 pulses at 90% rMT), Target: optimal target in the posterior gyral portion of right BA45 Duration: 10 daily 20 min sessions over 12 days | Behavioral localizer picture naming task: 6 sessions, 1 Hz rTMS (600 pulses at 90% rMT) administered at 6 sites in the right frontal lobe, Target: most responsive during picture naming (motor cortex, BA44, BA45, BA44/45, BA47) | Structural MRI only | Pre-rTMS, 2 mo, 6 mo, 10 mo follow up | rTMS only | not performed | -1 Hz rTMS to the right anterior BA 44/45: ventral posterior BA45: decrease in naming accuracy -LFS to right BA45: improved object naming and action naming -Improved picture description at 2, 6, and 10 mo after rTMS -Improvement on the WAB: spontaneous speech. | Single case |
Harvey et al. (2017) | n = 9 Age: 61±8 yrs, Males: 7 Females: 2 Chronic; TPO 55±33 mo, nonfluent; mild-moderate ischemic stroke | Protocol: 1 Hz rTMS, 90% rMT Target: right BA 45 (n = 8) BA47 (n = 1) Duration: 10 sessions over 2 weeks, 20 min/session administered to the optimal target | Behavioral localizer picture naming task; alternating 10 picture-naming blocks 2 runs, (3 trials per block) and 10 pattern-viewing blocks (2 trials per block) 1 Hz rTMS applied to 6 different sites within the right frontal lobe. -target: most responsive site | fMRI- pre-post rTMS: picture-naming and pattern-viewing alternating blocks | Baseline pre rTMS, 2 mo, 6 mo | rTMS only | not performed | -LFS to right hemisphere: improved naming related to recruitment of posterior right BA 6, 44, and 46 (post rTMS). -At 6 mo post-rTMS vs. baseline: greater activation for picture naming vs. pattern viewing were left lateralized, except right POp and right STG. | single group |
Harvey et al., 2019 | n = 11 Mean age: 55.5 yrs; Range: 29–79 yrs Chronic: Mean TPO 64.6 mo; Range: 6–173 mo 4 Broca’s, 6 anomic, 1 conduction | Protocol: cTBS, 50 Hz triplets of TMS pulses at 5 Hz for a total of 600 pulses (40 s, 80% of AMT). Target: right pars triangularis BA45 administered to the optimal target Duration: a single session of cTBS or sham delivered on different days in randomized order | Task related localizer naming task and structural MRI Items used for naming during stimulation were named incorrectly in one or both baseline sessions (i.e., inconsistent vs. wrong items) | none | Pre-post | cTBS only | rTMS coil to the vertex | -cTBS to the right BA45: improved naming following cTBS for low performers -improved naming of inconsistent, but not wrong items -baseline phonological but not semantic naming impairment severity correlated with improved accuracy and decreased phonological errors | Single session, cTBS or shamWithin group |
Martin et al., 2004 | n = 4 Age: 52–58 yrs Chronic: TPO 5–11 yrs 5 nonfluent 1 anomic | Protocol: 1 Hz rTMS, 90% rMT Target: right BA45, BA44 Duration:10 daily 20 min sessions (2 weeks), administered to the optimal target with best naming response | Behavioral localizer picture-naming task: 1 Hz rTMS for 10 min (90% of RMT) to 4 right perisylvian language homologue ROI, 4 rTMS sessions right BA 45, right BA 44, right BA 22, right motor cortex (M1) mouth area | none | Pre rTMS, 2 weeks post, 2 mo post | rTMS only | not performed | -LF rTMS to right BA45: improved picture naming -LF rTMS to right BA44: decreased naming accuracy and slower reaction times | Single group, case series |
Medina et al. (2012) | n = 10, n = 5 rTMS, n = 5 Sham Males: 4 Females: 6 Age: M = 61.60±8.32 yrs Chronic, TPO: M = 49.8±29.6 mo Nonfluent, mild-moderate, ischemic | Protocol: 1 Hz rTMS, 90% rMT Target: right BA45, BA47 rTMS to the optimal target Duration: 10 sessions over 2 weeks, duration 20 min | Behavioral localizer naming task, 1 Hz rTMS delivered to targets in the right IFG Optimal site: greatest improvement on the picture naming task | not performed | 2 mo | rTMS only | rTMS coil perpendicular to the head | -rTMS>Sham, improved discourse production | Within group, randomized, sham-controlled, single blind, cross-over. |
Turkeltaubet al. (2012) | n = 1 Age = 72 yrs old, Female Global aphasia/nonfluent Chronic: TPO 4 yrs | Protocol: 1 Hz rTMS 90% rMT Target: inferior posterior right BA45 Duration: 10 daily sessions, 20 min, over 2 weeks; treatment target: site with the largest % increase in naming accuracy | Behavioral localizer picture naming task, 1 Hz rTMS at 90% rMT for 10 min to 6 RH targets in separate sessions. (motor cortex, BA44, BA45, BA44/45, BA47) -sites selected based on gyral anatomy, not fMRI activity | fMRI pre-post: overt picture naming vs. pattern viewing (3 runs) | Multiple baseline, 2 mo 6 mo after rTMS fMRI acquisition: first day of treatment, prior to rTMS, &immediately after. | rTMS only | not performed | -1 Hz rTMS: reduction of activity in RBA45; inhibition of RBA45: improved naming - decreased activity in the medial prefrontal cortex, right caudate, right posterior insula, and the left STG -increased activity in the bilateral superior parietal cortices, left SMG and left pTemp | Single case (part of a larger trial) |
Behavioral Targeting | |||||||||
tDCS studies | |||||||||
Shah-Basak et al., (2015) | n = 12, Age: M = 63.6±8.6 yrs, Range: 53–78 yrs 10 males, 2 females Chronic: TPO M = 31.0±29.7 mo | Protocol: tDCS, anodal, cathodal, or sham Target: F4 or F3 sites Reference: contralateral mastoid Duration: 2 mA, 20 min (current density:0.80μA/mm2), sponge 5 cm2 electrodes, 30-s ramp-up/ ramp-down | Optimal montage finding naming task with tDCS: stimulated with 4 active montages (left/right, anode or cathode) and one sham montage optimal montage: best accuracy after stimulation | not performed | Multiple baseline, 2 weeks, 2 mo | tDCS + concurrent naming task | Sham montage, (randomized to receive F3-anode and cathode on right mastoid or F4-anode and cathode on left mastoid), Sham ramped up to 2.0 mA, then down to 0 mA in the first 60 s | -7/12 subjects showed transient improvement in picture-naming. -Aphasia severity improved at 2-weeks and 2-mo following real-tDCS but not sham. -Naming improvement after left-frontal cathodal-stimulation vs. sham. | Randomized cross-over, sham controlled, single-blind |
Targeting Function | |||||||||
rTMS studies with fMRI-localizer | |||||||||
Abo et al. (2012) | n = 24 rTMS Age: M = 55.9±8.8 yrs Males: 22 Females: 2 Chronic: TPO M = 34.7±20.5 mo, nonfluent, fluent, mild-moderate, stroke: ischemic, hemorrhagic | Protocol: 1 Hz rTMS, 90% rMT, Target: right IFG for nonfluent, Left STG for fluent aphasia Duration: 10 sessions: 40-min/session (11 days) | fMRI localizer: word repetition task | fMRI localizer pre-TMS only | immediate, 4 weeks | rTMS+SLT (expressive production, 10 sessions: 60 min/ session) | not performed | -LF-rTMS to the right IFG for nonfluent aphasia and to the left STG for fluent aphasia. post_rTMS > baseline improvement: nonfluent -aphasia: auditory and reading comprehension, repetition. Fluent aphasia: spontaneous speech. | single group |
Griffis et al., 2016 | n = 8 Age: M = 54.4±12.7 yrs Chronic: M = 5.25±3.62 yrs 4 anomic, 1 conduction, 4 nonfluent/ Broca’s | Protocol: iTBS 50 Hz every 200 millis in 2-s trains at 10-s intervals over a 200-s period, 600 pulses, 80% AMT Target: perilesional left IFG, Optimal target location Duration: 10 days over 2 weeks | fMRI localizer semantic decision/tone decision: iTBS applied to residual language-responsive cortex in or near the left IFG identified using an fMRI localizer task | fMRI data pre-post: alternating block-design covert verb generation task -silent verb generation alternating 30 s blocks in response to binaurally presented nouns -control condition: bilateral sequential finger tapping | fMRI before and after iTBS | iTBS only | not performed | -Post iTBS: IFG responses during covert verb generation were more strongly left-lateralized. -Post-iTBS higher levels of BOLD activity in left IFG and right IFG during covert verb generation compared to finger tapping. -Shift from right to left-lateralized activation | single group, Single session |
Hara et al. (2015) | n = 50 n = 29 right LF-rTMS n = 21 left-LF-rTMS Age: right-LF-rTMS: M = 59.9 yrs, left-LF-rTMS: M = 60.9 yrs Males: 40 Females: 10 Chronic: TPO right-LF-rTMS: M = 56.2±33, left-LF-rTMS group: M = 55.6±43.2 28 nonfluent, 23 fluent mild to severe ischemic, hemorrhagic, subarachnoid | Protocol: 1 Hz rTMS, 90% rMT Target: right or left: STG or IFG Location based on pretreatment activation: LF-rTMS to the right IFG or LF-rTMS to the left IFG Duration: 10 sessions: 40 min/session (2 weeks) | fMRI localizer word repetition task, 1-week pre-rTMS 4 runs with each run consisting of 12 cycles of 60-s rest and overt word repetition periods | fMRI: target localization, SPECT: pre-post TMS evaluation | SPECT at admission &3 mo post-TMS | rTMS+SLT (expressive production, 10 sessions: 60 min/session) | not performed | -Overall aphasia severity decreased, improved speaking, reading, writing and listening -Right hemisphere-LF rTMS: improvement in the total SLTA score, SLTA speaking and writing correlated with recruitment of perilesional left hemisphere regions. -Left hemisphere-LF-rTMS group: association between SLTA total score and change in laterality were not observed. -Speaking subscale scores correlated with transition of laterality index from left to right hemisphere | single group |
Hara et al. (2017) | n = 8 n = 4 LF-rTMS, n = 4 HF-rTMS Age: LF-rTMS: M = 63 yrs HF rTMS: M = 68.25 yrs Chronic: TPO 1 Hz = 20.79 mo 10 Hz = 55.15 mo Males: 6 Females: 2 nonfluent, fluent mild to severe, ischemic, hemorrhagic | Protocol: 1 or 10 Hz, 90% rMT Target: right IFG - left hemisphere activation received 1-Hz rTMS to the right inferior frontal gyrus (RIFG), – right hemisphere activation received 10-Hz rTMS to the RIFG Duration: 10 sessions: 40 min/session (2 weeks) | fNIRS- rTMS target: 3 cycles of 15-s rest &30-s overt word-repetition periods. | fNIRS-pre vs. post: word repetition task | 1-week pre-rTMS, 3 mo post-rTMS | rTMS+SLT, (expressive production, 10 sessions: 60 min/session) | not performed | -Post_rTMS > baseline, overall aphasia severity, listening, speaking, reading, and writing -LFS to right BA45: post-rTMS reduced bilateral activation and shift to the left hemisphere activity. -fNIRS showed resolution of interhemispheric inhibition -LFS to right BA45: left hemisphere > right hemisphere -HFS right BA45: post rTMS increase in the right hemisphere activity, also increased activation in the left hemisphere -HFS group: regions contralateral to the lesion > perilesional left hemisphere | single group |
Szaflarski et al. (2011) | n = 8 Age: M = 54.±12.7 yrs Chronic: TPO M = 5.3±3.6 yrs Males: 4, Females: 4 Nonfluent-fluent, Broca’s, Wernicke’s, global, moderate-severe, ischemic | Protocol: iTBS, 50 Hz 600 pulses, 80% AMT Target: left BA 45 Duration: 10 sessions: 3–10 min/session (2 weeks) | fMRI–localizer, semantic decision vs. tone decision task | fMRI pre vs. post activation: semantic decision vs. tone decision task | immediate pre-post rTMS | iTBS only, none | none | -Post iTBS > baseline, improved semantic fluency, self-reported improvement in communication abilities. -iTBS to the LH: increased activation in left fronto-temporo-parietal language networks, shift to the left frontal, and left temporo-parietal regions. -Improvement in language performance were correlated with increased lateralization to the left hemisphere. | single group single blind |
Szaflarski et al. (2018) | n = 12 Age: M = 49±12.63 yrs, Range 26–66 yrs Males: 9 Females: 3 Chronic: TPO M = 3.42±2.87 yrs nonfluent, (fluent), anomic, Broca’s, global, mild-moderate, type of stroke not stated | Protocol: iTBS, 50 Hz 600 pulses, 80% AMT Target: left BA 45 Duration: 10 sessions: 3–10 min/session (2 weeks) | fMRI localizer: semantic decision vs. tone decision task | fMRI pre vs. post activation: semantic decision vs. tone decision task | T1: baseline, T2: right after iTBS (2 weeks), T3:3 mo after iTBS | rTMS+SLT, (mCIAT, 10 sessions: 45 min/session) | none | -Post iTBS > baseline, improved aphasia severity (WAB-AQ) and object naming (BNT) -iTBS to the LH: improvements in WAB-AQ from T2 to T3 and decreased activation in left inferior parietal lobe, improvements on BNT from T1 to T3 with decreased activation in the right inferior frontal gyrus. -Shift of activation to the perilesional LH regions | single group single blind |
Targeting Function | |||||||||
tDCS studies with fMRI-localizer | |||||||||
Baker et al. (2010) | n = 10 Age: M = 65.±11.44 yrs, Range 45–81 yrs Chronic: TPO M = 64.6±68.4 mo, Range 10 to 242 mo 6 fluent, 4 nonfluent | Protocol: Anodal tDCS and sham: 1 mA with 5 cm2 sponge electrodes Target: left frontal cortex optimal site reference: right shoulder Duration: 5 days of anodal tDCS (1 mA, 20 min day) and 5 days of sham tDCS | Optimal site finding. T1-MRI and fMRI activation associated with an overt picture naming task was used to determine placement of the anode on a patient by-patient basis. Optimal target: area of the left frontal cortex with the greatest activation during correct naming | not performed | Baseline, 1 week | Anodal tDCS + self-administered computerized anomia treatment (1 week each sham or active) | Sham montage, sham turned off following 30 s of stimulation | -tDCS over the left frontal cortex improved naming accuracy -Those with the most improvements had perilesional targets closest to the stimulation site | cross-over, randomized, sham controlled, single-blind |
Cherney et al. (2021) | n = 12 Age: M = 56.7±7.14 yrs Chronic: TPO M = 34.8±42 mo 8 males 4 females non-fluent aphasia unilateral left hemisphere stroke | Protocol: anodal-tDCS, cathodal-tDCS, or sham tDCS 8 cm2 oblong (2×4) saline-soaked sponge electrode Target: perilesional left hemisphere reference anode (48 cm2) on the forehead, directly above the right orbit. Duration: 5 days a week for 6-weeks tDCS: 1 mA, first 13 min of the 90-minute speech–language treatment session 5 days a week | fMRI localizer task: guided identification of tDCS sites. Tasks: 1. semantic categorization, 2. oral reading of a word within a sentence, 3. imitation of consonant-vowel syllables fMRI-navigated electrode placement Individualized stimulation site based on overlapping fMRI activation in the left hemisphere across three speech–language task | fMRI task pre vs. post: Tasks: semantic categorization oral reading of a word within a sentence, imitation of consonant-vowel syllables | Baseline, post Tx, follow-up | tDCS+STL (90 min computerized SLT with virtual therapist) 2 sequential 15-min intervals of Oral Reading for Language in Aphasia (ORLA®). The first 15 min of ORLA® was paired with the tDCS 30-min interval of Aphasia Scripts incorporates reading aloud of sentences, embedded in scripted conversation The last 30-min of treatment was continued reading aloud practice via ORLA®. | Sham: a ramp-like fashion for 30 s, and then shut off | -WAB-IQ and oral reading improved for anodal and cathodal vs. sham -Active tDCS: greater gains on standardized probes, and caregiver-reported measures of functional language-Cathodal stimulation to the lesioned left hemisphere: increased areas of cortical activation across both hemispheres, and in perilesional regions. -Effects interacted with severity, less severe aphasia exhibited greater activation of the left hemisphere post treatment, than more severe aphasia | between group, randomized single-blind placebo-controlled clinical pilot study |
Fridriksson et al. (2011) | n = 8 Age: M = 68.1±10.4 yrs Range 53–79 yrs Chronic: TPO, M = 58.4±44.6 mo, Range 10–150 mo, fluent aphasia | anodal-tDCS (A-tDCS) sham tDCS (S-tDCS) (1 mA) Target: left posterior cortex reference cathode on the right forehead. Duration: 10 sessions of computerized anomia treatment; 5 (1 week) sessions, 20-minute anodal tDCS and 5 (1 week) included Sham tDCS | fMRI localizer overt picture naming task: Anode placed over perilesional brain regions that showed the greatest activation on a pretreatment fMRI task | no pre vs. post fMRI reported | Baseline, after Tx, 3 weeks | tDCS+10 sessions of computerized anomia treatment; began 5 min before the start of tDCS | Sham stimulator was turned off after 45 s | -Reduction in RT during naming of trained items after A-tDCS compared with S-tDCS immediately after treatment and at 3-week follow-up testing. -Greater response generalization after A-tDCS - A-tDCS in the absence of behavioral training did not improve task performance | randomized, double-blind, sham-controlled crossover trial |
Fridriksson et al., 2018 | n = 74 Age: M = 60±10 yrs 52 Males 22 Females Chronic: TPO M = 44±40 mo Mixed aphasia types Single ischemic stroke | A-tDCS, sham tDCS (S-tDCS), 1 mA, 20-minute Target: left temporal regions, Duration: 3 weeks (15 sessions) | fMRI localizer overt picture naming task | No pre vs. post fMRI reported | Baseline, 1-week postTx, 6 mo follow- up | Anodal tDCS + SLT (3 weeks, 15 sessions, 45 min each computerized anomia treatment) | sham tDCS | -Naming accuracy improved after anodal tDCS compared to sham | randomized, double-blind, sham-controlled between-group |
Ulm et al., 2015 | n = 1, Age = 51 yrs old, female Chronic: TPO 4.7 yrs Mild anomic aphasia | Protocol: anodal tDCS+fMRI (simultaneous) 1 mA, 5×7 cm3 sponges Target: Anode = F3 (left IFG) corresponded to ∼75% upwards from F7 to F3 cathode (10 cm×10 cm) over right supraorbital region Duration: Single session anodal ramped-up 10 s prior to the start of the picture naming, stimulated for 20 min | fMRI localizer overt picture naming task: Peak activity located in the spared left inferior frontal gyrus and this area was stimulated during a subsequent cross-over phase. | fMRI concurrent with tDCS: picture naming task, pictures named correctly during baseline | Baseline, post tDCS | Anodal or sham + picture naming task in fMRI | Sham turned off after 30 s | Anodal vs. sham resulted in selectively increased activity at the stimulation site | Single case, sham-tDCS controlled, Single session |
Richardson et a., 2015 | n = 8 Age: M = 60.±8.88 yrs, Range 51–74 yrs Chronic: TPO, M = 100.2±92, Range 9–312 mo 4 females 4 males 5 Broca’s 3 anomic | Protocol: compared conventional sponge-based (CS) tDCS to HD-tDCS, CS-tDCS: 1 mA, 20 min, 5×5 cm2, HD-tDCS: 8-channel, 9th reference, 1 mA, per electrode (2 anodes, 2 cathodes), Target: optimal fMRI location, Duration: 20 min, 30 s ramp-up and down. | High-resolution T1- and T2- MRI scans, fMRI language task used to determine electrode placement on a patient-by-patient basis. Optimal target: highest fMRI activation in the perilesional cortex on correctly named trials Individualized modeling of targeting | not performed | assessed at 12 different time points: twice at Phase 1 baseline (P1-B), twice immediately post final treatment session (P1-IP), twice at one-week follow-up (P1-FU), twice at P2-B, twice at P2-IP, and twice at P2-follow up. | tDCS + Computerized anomia treatment, 5 days during each treatment arm. | not performed | -CS-tDCS and HD-tDCS comparable outcomes -Naming accuracy and response time improved for both stimulation conditions. | randomized, cross-over |
Targeting pathology or dysfunction | |||||||||
Shah-Basak et al., 2020 | n = 14 Age: M = 58.8±12.8 yrs, 7 males 7 females Chronic: TPO: M = 8.0±5.2 yrs Range 4–21 yrs nonfluent, conduction, Anomic, Fluent | Protocol: HD-tDCS vs. sham, 2 mA, using a 3×1 center-surround configuration, Targets: anodal-tDCS: LH perilesional, right cathodal-tDCS: RH homolog or sham-tDCS, Duration: Single 20-min session, separated by 48h. | rsMEG localizer: Location of the center electrode determined individually based on rsMEG abnormalities | MEG: delayed word reading task | Baseline, pre vs. post | HD-tDCS + cued and uncued repetition task, easy and hard task adjusted to severity level | Sham vs. post-tDCS repetition performance | -Anodal-tDCS: improved repetition accuracy -Improvements depended on task difficulty level. -Anodal-tDCS to perilesional areas increased low gamma and signal complexity in the higher frequencies and reduced contralateral theta and signal complexity -Changes to theta power in contralateral areas after perilesional anodal-tDCS associated with improved repetition accuracy -Homolog cathodal-tDCS was only weakly effective -Cathodal-tDCS: oscillatory changes not correlated with repetition accuracy | randomized, double-blind, within-subjects |
Targeting ancillary systems | |||||||||
Motor cortex | |||||||||
Datta et al., 2011 | n = 1 Age: 60-year-old male, Chronic: TPO 64 mo Broca’s aphasia | Protocol: anodal tDCS (A-tDCS 1 mA, 20 min), Target: left perilesional frontal cortex Duration: 5 days of A-tDCS and 5 days of sham tDCS (S-tDCS, 20 min) | computerized model of current flow | – | tDCS or sham + computerized anomia treatment | reference cathode electrode placed on right shoulder | Single-subject | ||
Darkow et al., 2017 | n = 16 Age: M = 56.8±10.1 yrs, chronic: TPO M = 54.36±45.3 mo 6 females, 10 males mild aphasia | Protocol: Concurrent tDCS+fMRI, MR compatible Anodal vs sham tDCS (1 mA, 20 min), 5×7 cm2 Target: Left primary motor cortex (M1) Duration: Single session of active or sham to M1. | C3 based on the 10–20 EEG system, | fMRI: object naming task the naming task during tDCS-fMRI: pictures that could be named correctly during repeated behavioral baseline assessment | Pre vs. post tDCS Activation change pre vs. post | tDCS only | Sham, turned off after 30 s | - greater activity during anodal- compared with sham-tDCS in the language regions, but not in the visual or motor regions -Anodal-tDCS: “normalization” of task-related activity and network characteristics. -Stimulation effects task dependent, M1-tDCS did not affect the motor network. not determined by the stimulation site. | randomized, cross-over |
Meizner et al., 2016 | n = 26 Age: M = 60±11.8 yrs, TPO: M = 42±22.3 yrs, 18 males 8 females Chronic aphasia, Broca’s, Wernicke’s, global | Protocol: Anodal tDCS vs sham Target: anode to left primary motor cortex (M1) (over C3) twice daily, 5×7 cm2 cathode (10×10 cm2) over the right supraorbital region Duration: 2 weeks 8 days, twice daily for 1.5h/day, ramped up and down for 10 s | C3 based on 10–20 EEG system) | Baseline, Post Tx, 6 mo follow-up | Pre and post tDCS after 6 mo | Anodal tDCS + SLT (naming therapy, 2 weeks (8 days, 2×1.5 h/day) | Sham: ramped up and remained at 1 mA for 30 s before ramping down | -Naming ability for trained items improved after the intervention in both the anodal and sham-tDCS -Better maintenance in the anodal-tDCS group 6 mo later -Transfer to untrained items larger in the anodal-tDCS group after training and during the 6-month follow-up-Improved functional communication in the anodal-tDCS vs. sham | parallel group, randomized, sham-controlled, double-blind |
Xu et al., 2021 | n = 16 Age: M = 55.6±11.8 yrs, 12 males 4 females TPO: M = 5.1±2.3 mo, Range 2–9 mo, ischemic or hemorrhagic stroke | Protocol: iTBS 800 pulses for 300 s, three pulses at 50 Hz in each burst at 5 Hz, and 2 s, 8 s intervals, 80% rMT Target: left primary motor cortex (M1) Duration: Single session | single-pulse rTMS over the primary motor area (M1) where the largest MEP was evoked | Resting state fMRI before, immediately after the iTBS | Baseline, Pre vs. post, MRI Pre and immediately after iTBS session | iTBS only, No SLT | not performed | Changes in functional connectivity after iTBS | Single group |
Cerebellum | |||||||||
Demarco et al., 2021 | n = 24 Active: n = 10 Sham: n = 14 Age: M = 60.2±10.5 yrs, Range 42–81 yrs 16 males 8 females, chronicity = TPO: M = 43.4±39.9 mo Range 6.8–177.6 mo | Protocol: Anodal tDCS, 5×5-cm2, 2 mA Target: right posterolateral cerebellum cathode placed on the upper right bicep. Duration: applied during the first 20 min of speech therapy | No localizer task Cerebellum: 1 cm down and 4 cm to the right of the inion | No imaging | 2×before treatment, once immediately post treatment, and at the 3-month follow-up | Anodal tDCS + multimodal speech therapy targeting anomia | Sham results used from a different study, Two sham: anodes placed at left IFG (F7 and F5), and two cathodes placed at the right-IFG (F6 and F8). The current was ramped up to 1.5 mA over 30 s, followed immediately by a 30-s ramp-down. | -Improvement on number of utterances produced -Active = sham no significant post treatment behavioral gains -No significant gains on measures that engage the cerebellum | between group, sham controlled |
Marangolo et al., 2018 | n = 12 6 males females | Protocol: cathodal tDCS, 2 mA, 5×7 cm2, sham Target: right cerebellum Duration: 20 min, 5 daily sessions/1 week | Location:1 cm down and 4 cm to the right of the inion | No imaging | Baseline, Post Tx, 1-week follow-up | tDCS + verb generation task, tDCS + verb naming task in different conditions 5 sessions, over a week, 6 days break | Sham turned off after 30 s, -reference: right shoulder over deltoid muscle. | -Cathodal tDCS improved verb generation but not verb naming. | randomized, double-blind, within group |
Sebastian et al., 2017 | n = 1 age = 57 yrs old male TPO = 5 yrs ischemic stroke | Protocol: Anodal tDCS and sham, 25 cm2 saline soaked sponge electrodes Sham first, tDCS second Target: right cerebellum Duration: 20 min/session, 3–5 per week | Right cerebellum 1 cm under, and 4 cm lateral to the inion -Modeled current flow in the right cerebellum | MRI image, resting fMRI | before, immediately after, and 2 mo post-treatment for each condition | cerebellar tDCS+ spelling therapy (15 sessions) 15 training sessions 3–5 per week, separated by 2 mo tDCS administered for the first 20 min of the 45-min spelling treatment | Sham ramped down to zero after 30s, cathode was placed on the right deltoid muscle | -Greater improvement with tDCS than with sham for trained and untrained words -Immediately after and 2 mo post-treatment, improved spelling-to-dictation for trained and untrained words-Generalization to written picture naming in tDCS condition -Immediately after and 2 mo post-treatment, connectivity increased between the right cerebellum and the LH and RH ROIs -Improved spelling accompanied by an increase in cerebro-cerebellar connectivity. | double-blind, within-subject crossover trial design, with random order of treatments |
rTMS: repetitive transcranial magnetic stimulation, tDCS: transcranial direct current stimulation; HD-tDCS: High definition transcranial direct current stimulation; CS-tDCS HF: Conventional sponge tDCS; high frequency, LF: low frequency; cTBS: continuous theta burst stimulation; iTBS: Intermittent theta burst stimulation; rMT: resting motor threshold; AMT: active motor threshold; MEP: motor evoked potential; SLT: speech language therapy; rTMS+SLT: repetitive transcranial magnetic stimulation combined with speech language therapy. TPO: time post onset; SLT: speech-language therapy, LMCA: left middle cerebral artery; LH: left hemisphere; RH: right hemisphere, ROIs: regions of interest. *values calculated based on the information provided in the text. ΔLI: change in laterality index; LFS: low frequency stimulation; HFS: high frequency stimulation; fNIRS: functional near infrared spectroscopy; SPECT: single-photon emission computed tomography; fMRI: functional magnetic resonance imaging; PET: positron emission tomography; PET AVI: positron emission tomography activation volume; MEG: Magnetoencephalography; rsMEG: resting-state MEG; CBF: cerebral blood flow. WABAQ: Western Aphasia Battery Aphasia Quotient; CCAT: Chinese Concise Aphasia Test; BDAE: Boston Diagnostic Aphasia Examination; AAT: Aachen Aphasia Test; ASRS: Aphasia Severity Rating Scale. BA: Broadman’s Area; STG: superior temporal gyrus; IFG: inferior frontal gyrus; FT: frontal-temporal lobe; pIFG: posterior inferior frontal gyrus; pSTG: posterior superior temporal gyrus; pT: posterior temporal; SMG supramarginal gyrus; min: minutes; hr: hour; ILAT: intensive language action therapy, mCIAT: modified constraint-induced aphasia therapy; BDAE: Boston Diagnostic Aphasia Examination.
3.2.1Behavioral targeting
Behavioral targeting approaches are those where transient effects of rTMS or tDCS are evaluated first at several targets (and polarity) to identify the target that results in more accurate language performance in an individual (Hamilton et al., 2011; Medina et al., 2012; Shah-Basak et al., 2015). In several studies, transient change in naming ability in response to single sessions of 1 Hz rTMS applied to six regions within the right IFG (including PTr, POp, pars orbitalis, and mouth area of the motor cortex) was used as a metric for identifying optimal targets to inhibit in individuals with nonfluent aphasia (Hamilton et al., 2010; Harvey et al., 2017; Medina et al., 2012; Naeser et al., 2005; Turkeltaub et al., 2012). The target finding sessions were separated by 5 days and patients performed a naming task before and immediately after rTMS. The site with the largest increase in naming accuracy from pre to post rTMS was selected as a treatment target. During a subsequent longer treatment trial, 10 daily 20-minute treatment sessions of 1 Hz TMS were administered to this optimal target over two weeks followed by a naming therapy. Across several studies, significant improvement in naming accuracy and on measures of discourse production was reported immediately and up to 3-months after stimulation. The long-term benefits were associated with a shift of activity from right PTr before the intervention, to more posterior regions of the right IFG (BA 44/6/46). Improved naming performance after treatment was associated with increased activity in the right posterior STG and left perilesional regions (Harvey et al., 2017). Right PTr was the optimal site for most patients, with a few exceptions where right pars orbitalis was the optimal site (P. Martin et al., 2004; Medina et al., 2012). Gains in naming ability are also reported after inhibitory stimulation with cTBS over the right BA45, especially in those with more severe baseline naming deficit (Harvey et al., 2019).
A similar approach was used for a treatment study involving tDCS. Naming accuracy changes in response to 4 active tDCS electrode montages (left hemisphere anodal or cathodal; right hemisphere anodal or cathodal) and 1 sham montage were examined for each PWA prior to protracted treatment trial. The montage with largest change was selected as a therapeutic target. Large variability was reported in 12 patients, and the greatest improvement was reported for cathodal montage over the left frontal regions. Optimal montage finding procedure was followed by a sham-controlled cross-over trial in which tDCS was administered concurrently with constrained induced language therapy (CILT), over 10-days using the optimal montage configuration. Reduction in overall aphasia severity was found after two weeks, which was maintained at the 2-month follow-up (Shah-Basak et al., 2015).
3.2.2Targeting function
In other studies, functional neuroimaging with language tasks closely related to planned language therapy was performed prior to the NIBS trial to select the target based on individual’s lesion as well as functional activation patterns (Cherney et al., 2021; Fridriksson, Rorden, et al., 2018; Griffis et al., 2016; Richardson et al., 2015; Ulm et al., 2015). The rationale was to maximize the effects of therapy by strengthening activity using NIBS in the most therapy-responsive region.
FMRI-guided target-finding approach has been previously implemented for several rTMS studies. For example, stimulation targets in individual patients were selected based on the type of aphasia and fMRI activation patterns during a repetition task in a study by Abo et al. (2012). Targets for patients with nonfluent aphasia were within the right IFG and for those with fluent aphasia within the left STG. Each patient subsequently received 10 treatment sessions consisting of 40-minute 1-Hz rTMS to the brain area contralateral to the region identified during fMRI, and 60 minutes of intensive SLT. Patients with nonfluent aphasia improved on auditory comprehension, reading comprehension and repetition, whereas those with fluent aphasia showed improvement in spontaneous speech. These effects persisted 4 weeks after the intervention (Abo et al., 2012). In a subsequent study, Hara et al. (2015) adopted a similar fMRI guided approach but targeted both left and right hemisphere targets. After the treatment, overall aphasia severity decreased. Improved performance was observed on subtests of speaking, reading, writing and listening at 3 months after the intervention (Hara et al., 2015).
More recently, Hara et al. (2017) modified their stimulation protocol and localized stimulation targets using functional near infrared spectroscopy (fNIRS) during a repetition task. Patients exhibiting left hemisphere activation on the fNIRS localizer task received 1-Hz stimulation to the right IFG, and those with right hemisphere activation received 10-Hz rTMS directly to the right IFG. Significant improvement was found for both groups on the overall aphasia severity, measured with subtests for listening, speaking, reading and writing. Reduced bilateral activation and a shift to left hemisphere activation, relative to pre-intervention, was reported in patients who received inhibitory rTMS to the right IFG. Patients who received 10-Hz to the right IFG exhibited increased activation in the stimulated right hemisphere relative to pre-intervention time point (Hara et al., 2017).
In a study by Szaflarski et al. (2011), patients performed a semantic fMRI task to localize optimal targets within the left hemisphere perilesional areas. For 7 out of 8 patients, the left IFG was the most activated region. Over 10 daily sessions, patients received excitatory stimulation using iTBS. Compared to baseline, significant improvements on semantic fluency and a trend toward better self-reported communication abilities were found. Additionally, changes after 10 sessions of iTBS treatment were measured using fMRI with a semantic vs. tone decision task. Observed improvements were significantly correlated with a shift to the left hemisphere after left IFG stimulation (Szaflarski et al., 2011). In particular, increased activation in the left fronto-temporo-parietal language networks was reported. In a later study by Szaflarski et al. (2018), stimulation targets were determined individually using a fMRI task and were localized to left frontal regions in most patients. Treatment trial involved iTBS to these targets, accompanied by modified constraint-induced aphasia therapy. Significant reductions in overall aphasia severity (WAB-AQ) and increases in naming accuracy (on the BNT) were reported immediately after the treatment, which lasted 3 months after the intervention was discontinued (Szaflarski et al., 2018).
FMRI-guided targeting has also been implemented to guide electrode positioning during tDCS to ensure that stimulation is applied over responsive brain regions (Richardson et al., 2015; Ulm et al., 2015). Baker et al. (2010) administered anodal tDCS (1 mA, 20-min) and sham tDCS to individually determined left perilesional locations in 10 patients with chronic aphasia over two separate weeks combined with a computerized anomia treatment. The electrode positioning was guided by fMRI activation patterns acquired during an overt naming task for each individual on correct trials. This procedure ensured that the active electrode was placed over structurally preserved cortex engaged during naming, directly relevant to the anomia treatment provided during tDCS. Naming accuracy improved after active tDCS compared to sham (Baker et al., 2010). Similar fMRI protocol for tDCS target localization has been used in other studies (Fridriksson et al., 2011; Fridriksson, Rorden, et al., 2018). This includes a recent clinical trial that found that individually targeted anodal tDCS to left temporal regions, guided by fMRI naming task, was feasible and non-futile, warranting further study for the treatment of aphasia.
A more recent study that investigated the effects of extended six-week application of anodal, cathodal and sham tDCS (13 min, 1 mA daily) on language skills and brain activation adopted a targeting approach guided by 3 fMRI tasks: semantic decision, word reading and consonant-vowel imitation. It was expected that these fMRI tasks would recruit regions needed for therapies planned during tDCS, namely Oral Reading for Language in Aphasia or ORLA and reading scripts out loud. Compared to sham both anodal and cathodal tDCS groups showed reduction in aphasia severity (WAB-AQ) and improvement on WAB language quotient. In addition, active tDCS resulted in improved reading comprehension and increased functional communication scores. FMRI results indicated that cathodal tDCS to the left perilesional regions resulted in increased activation in both hemispheres and increased activation in the perilesional regions. These effects interacted with severity, such that participants with less severe aphasia tended to exhibit greater activation of the left hemisphere after the treatment, than participants with more severe aphasia (Cherney et al., 2021).
3.2.3Targeting pathology or dysfunction
Another targeting approach implements neuroimaging to identify regions that are structurally intact but exhibit physiologically abnormal activity. These aberrant physiological signals are thought to reflect dysfunction, in comparison to healthy regions within the same individual or compared to matched healthy controls (Chu et al., 2015; Kielar et al., 2016; Shah-Basak et al., 2020). The rationale is to normalize or restore activity in functionally compromised regions using NIBS, and potentially maximize recruitment of these regions during planned therapy.
One study by Shah-Basak et al. (2020) used resting-state MEG (rsMEG) to localize region(s) exhibiting perilesional oscillatory dysfunction in individual PWA during chronic stages of recovery. These regions were then targeted using HD-tDCS to correct or normalize the abnormalities. This study examined transient changes after a single 20-minute session of anodal, cathodal or sham-tDCS. Specifically, perilesional area(s) that exhibited peak pathological oscillatory slowing was selected as a stimulation target in individual patients. These areas were targeted with anodal HD-tDCS directly, or cathodal HD-tDCS was applied to the homolog right hemisphere regions. During stimulation, patients performed a graded sentence repetition task. Anodal HD-tDCS significantly improved repetition accuracy, whereas cathodal HD-tDCS showed weaker effects, compared to sham tDCS. Effects of anodal tDCS interacted with the difficulty of the task conducted during stimulation such that the improvement was greater in patients with less severe aphasia who could undergo the more difficult version of the task. Anodal HD-tDCS applied over the perilesional targets resulted in a reduction of oscillatory abnormalities in the left hemisphere and in the contralateral right hemisphere areas. The reduction in theta power (4–7 Hz) in the right hemisphere areas contralateral to the left hemisphere stimulation sites was associated with greater improvement in repetition accuracy. For cathodal stimulation, changes in oscillatory activity did not significantly correlate with repetition accuracy (Shah-Basak et al., 2020).
While the mechanisms of oscillatory slowing in perilesional areas are unclear, prior work by Kielar et al. (2016) has found significant associations between reduced cerebral blood flow (CBF) in PWA and increases in slow (pathological) oscillatory activity in these regions, as measured with rsMEG. In comparison to age and education matched controls, PWA exhibit decreased CBF in perilesional regions (Kielar et al., 2016).
Taking this evidence together, we hypothesize that partial restoration of perfusion with NIBS in the structurally intact areas of the affected hemisphere may be an integral part of post-stroke aphasia recovery. But changes in perilesional perfusion can unfold differently throughout recovery (with rapid changes early after stroke that level out chronically) and these changes may be differentially important at different stages of stroke recovery. These characteristics would have to be accounted for in the planning of NIBS targeting protocols.
In general, we know that stroke can induce deficits in temporal dynamics of CBF (Roc et al., 2006; Treger et al., 2007). Diminished blood circulation in a particular part of the brain results in decreased delivery of oxygen and nutrients. In brain areas with extremely limited circulation (or hypoperfusion), apoptosis is very likely (Baron & Marchal, 1999). In other areas, where hypoperfusion is sub-baseline, neurons can remain alive. However, such sub-baseline hypoperfusion can last for weeks after stroke (Brumm et al., 2010; Hillis, 2007) and can impact language function (Cloutman et al., 2011). For example, following a left-hemisphere stroke, chronic hypoperfusion within 0–6 mm band in the perilesional area was associated with worse language impairment (Thompson et al., 2017).
Studies in the hyperacute stroke period (< 2 days post-stroke) have supported a relationship between cerebral perfusion and language ability. For example, hypoperfusion of Wernicke’s area and BA 37 seen on perfusion MRI was associated with poor naming and word comprehension, whereas subsequent reperfusion coincided with an improvement of those abilities (Hillis et al., 2005; Hillis & Heidler, 2002). Furthermore, Hillis (2007) showed that promoting reperfusion via a pharmacologically-induced increase in blood pressure in a patient with hypoperfused left perisylvian language cortex improved naming and comprehension. As suggested by this and other studies, cerebral circulation following stroke may undergo changes across different stages of recovery. A study by Boukrina and colleagues (Boukrina et al., 2019), which examined post-stroke recovery of reading, showed that subacute perfusion in the intact parts of the left reading (ipsilesional) network was positively associated with chronic accuracy on a pseudoword rhyming task. Consistent with prior findings, Walenski and colleagues (2022) showed that cerebral perfusion in perilesional areas was abnormal in both hemispheres of chronic PWA. However, they did not observe a change in perfusion over time or any changes associated with recovery resulting from a language treatment. The authors suggested that functional re-organization in their cohort may have accounted for improvements observed after language treatments, with changes occurring beyond the perilesional areas. Abbott et al. (2021) used individualized hypoperfusion cutoffs and found associations between hypoperfusion in left hemisphere language areas outside lesion boundaries and standardized scores on the WAB (Kertesz, 2007) and the BDAE (Goodglass et al., 2000). This indicates a long-term decrease in cerebral perfusion after stroke and that increased reperfusion in the affected left hemisphere is positively associated with post-stroke language outcome.
To our knowledge, no treatment studies have used individualized protocols to identify hypoperfused areas in post-stroke aphasia during chronic phases for NIBS applications. CBF measures are typically used to examine post-stimulation effects but they are rarely used to localize stimulation targets. Several studies in healthy volunteers demonstrate a correlation between the effects of tDCS and regional changes in CBF, but the exact nature of changes varies with the stimulation target and polarity (anodal vs. cathodal). For example, Zheng et al. (2011) used an alternating on-off sampling to detect changes in cerebral perfusion as a result of anodal or cathodal tDCS of varying strengths (0.8–2 mA) in 14 healthy adult participants. The sponge electrodes were placed above the right motor region, with reference at the contralateral supraorbital region. Perfusion changes were found within the spherical region in the motor cortex below the electrodes during and after the stimulation. Specifically, anodal tDCS caused a ∼17% increase in CBF, which persisted in the post-stimulation period. The increase in CBF correlated with current strength. Cathodal tDCS caused a ∼5% increase in CBF, and a subsequent immediate decrease, which continued during post-stimulation period. Anodal tDCS also resulted in CBF increases in the ipsilateral motor and premotor areas, and to a lesser degree in contralateral motor and premotor regions. Stagg et al. (2013) also reported changes in CBF post-tDCS but their results are somewhat at odds with those of Zheng et al. (2011). Like Zheng et al., they found that CBF increased during sham-controlled anodal and decreased during cathodal tDCS of the left dorsolateral prefrontal cortex (DLPFC). Specifically, anodal tDCS resulted in increases in CBF in left primary sensory cortex, midcingulate, and paracingulate cortex relative to baseline. Cathodal tDCS decreased perfusion in bilateral thalamus and right temporal cortex relative to baseline. However, after the stimulation ended, CBF was decreased for both stimulation polarities. Compared to CBF during anodal tDCS, post-stimulation CBF decreased in bilateral frontal lobe, cerebellum, and the precuneus. Frontal and precuneus CBF decreases were also evident after the stimulation when comparing to baseline CBF. After cathodal tDCS, CBF decreased in the cerebellum and bilateral occipital cortex. Compared to baseline, after cathodal tDCS CBF was decreased in bilateral frontal and temporal lobes. CBF changes have also been reported post-rTMS. For example, Moisa et al. (2010) showed intensity dependent increases in relative CBF following rTMS to the motor, premotor, and supplementary motor areas. It is currently unknown what mediates or determines the relationship between CBF and tDCS (and other NIBS methods). Several potential mechanisms have been proposed. First, perfusion changes may coincide with tDCS effects through neurovascular coupling (Figeys et al., 2021). For example, electrical stimulation of cerebellar parallel fibers in anesthetized rats increased dilation of local arterioles and larger arterioles upstream of stimulation. This vasodilation correlated with increased regional CBF (Iadecola et al., 1997). Additionally, tDCS may have an indirect effect on CBF through the function of excitatory and inhibitory neurotransmitters. An MR spectroscopy study showed that anodal tDCS reduced local concentrations of GABA (gamma Aminobutyric acid) while cathodal tDCS resulted in both reduced glutamate and GABA among 11 healthy volunteers (Stagg et al., 2009).
While evidence of positive effects of tDCS on CBF during chronic stages of stroke recovery is minimal, recently the TESSERACT trial assessed the feasibility, tolerability, and safety of HD cathodal tDCS delivered to the ischemic regions within minutes after stroke, or acute ischemic stroke, to salvage tissue undergoing stroke-related damage (Bahr-Hosseini et al., 2023). Based on preclinical evidence of vasodilation and neuroprotective abilities of cathodal tDCS, the trial assessed for the first time in humans the preliminary efficacy of tDCS to inhibit “peri-infarct excitotoxic effects and enhance collateral perfusion” during acute stages of ischemic stroke. Ten patients (7 active and 3 sham) were randomized to receive either active HD cathodal tDCS or sham tDCS at doses of 1 mA or 2 mA within 24 hours from stroke onset. Individualized HD montages based on computational modeling, predefined to cover vascular territories undergoing ischemia, were used. The HD cathodal tDCS was well tolerated (quantified by the rate of patients completing the stimulation 10 out of 10) and feasible (time from randomization to tDCS initiation was on average 12.5 minutes (9–15 minutes). Penumbral tissue appeared to be salvaged at higher rates and hypoperfusion in ischemic regions was reduced in patients receiving active compared to sham tDCS. Increased regional cerebral blood volume and higher rate of early recanalization were other findings after active stimulation. While safety could not be fully addressed due to the small sample sizes, the results are quite promising and suggest that targeted HD cathodal tDCS can be an efficient strategy to reduce stroke-related damage during the hyperacute stages. The results further reinforce the relationship between NIBS and CBF and warrant clinical trials focusing on safety and efficacy of this approach in larger samples.
It is possible that the effects of tDCS on CBF vary across age and disease states (Figeys et al., 2021). The greatest modulation of CBF by tDCS seems to occur in healthy younger adults and decreases with age and disease, as does the regional CBF. With the caveat that CBF may not be as malleable in older age, targeting tDCS to restore perfusion in intact areas of the affected hemisphere may prove to be an effective treatment strategy during chronic stages of post-stroke aphasia recovery, and should be further explored in future studies. Hyperacutely, the use of cathodal tDCS can help salvage affected tissue and could thus have long-lasting impact on motor and cognitive outcomes after stroke. The full potential of this treatment remains to be explored in future studies.
3.2.4Optimal montage configurations
With tDCS, once individual stimulation targets are localized, another important consideration is determining optimal montage configurations. The positioning of active and reference electrodes is critical to achieving maximal current flow at the intended target location. The intracranial current distribution and peak electric field magnitude are affected by the brain tissue geometry and electrical properties (conductivity) of the brain layers and tissue between the scalp electrodes and cortical brain targets (Datta et al., 2009; Dmochowski et al., 2013; Neuling et al., 2012; Saturnino et al., 2015). Recent advances in high-resolution MRI-based finite element (FE) modeling have made it possible to estimate tDCS induced electric fields with both conventional and HD configurations. Modeling demonstrates that focality and specificity of tDCS can be modified by changing the electrode size, placement and number of active or reference electrodes (Alam et al., 2016). A common HD-tDCS configuration is 4×1 or ring configuration, shown to result in increased focality with the peak induced electric field directly under the center electrode. The conventional tDCS with large rectangular sponge electrodes results in diffused electric field distribution and the peak field magnitude is found between the active and reference electrodes (Datta et al., 2009).
Modeled current flow is also shown to predict behavioral response to tDCS (Mendonca et al., 2011). For example, a study testing different (conventional) tDCS montages in patients with fibromyalgia found increased analgesic effects with supraorbital-extracephalic (active-reference) montage but no effects with M1-extracephalic montage. The clinical effects were consistent with modeling results. Head modeling indicated that the supraorbital montage produced current flow across anterior prefrontal structures, associated with the pain matrix, whereas the M1 montage produced current flow in unrelated temporoparietal regions. Thus, montage configuration, as guided by current modeling, can directly impact the efficacy of tDCS (van der Cruijsen et al., 2021).
Recently, FE models have been developed to guide electrode positioning by reviewing “hotspots” of induced electric fields. Most prior studies developed single FE models from a healthy adult brain template to estimate cortical current distribution as a function of different montage configurations. But differences in brain anatomy and head geometry can produce differential current distribution across individuals in targets of interest. Ideally, individualized FE models using participant’s own head MRI are needed to optimize montages for maximal focality or intensity at the target. Several free and open-source software packages are now available such as simNIBS (Saturnino et al., 2015) that enable processing of electric field directions and magnitude for a given montage. This software can also automatically determine optimal montages using electrode placements from an EEG cap. In this case, the electric field induced by each individual electrode is calculated and is used to estimate the field generated by different combinations of electrodes. The FE modeling presents some challenges with stroke lesions or skull defects that dramatically change the current flow, and thus they must be accounted for in the processing steps. Nonetheless, these models, especially individualized models, are a great tool with a potential to substantially increase tDCS efficacy.
3.3Implementation of targeting approaches
The targeting approaches that we have discussed have a component of “precision” using behavioral exploration of optimal montages, pre-treatment functional mapping of spared language areas, or individualized current modeling. We acknowledge that from a practical and clinical standpoint, determining precise stimulation targets and montages is time consuming, expensive and can require specialized software and expertise (e.g., fMRI, FE modeling) that may not be readily available in a clinical setting. This creates a significant barrier for implementation of focal stimulation (rTMS, HD-tDCS) approaches in clinical practice. Several clinically inclined groups have recognized this barrier and note that these approaches may not be feasible outside specialized research labs (Meinzer et al., 2016).
For TMS/rTMS applications, neuronavigational systems that incorporate structural MRI data are often used to enable spatial precision. The same systems can also be used for tDCS applications. However, historically, localization of targets for tDCS has been imprecise compared to TMS. For conventional tDCS, the use of 10–20 EEG International coordinate system is common to roughly estimate electrode placement.
One may argue that precise targeting is excessive for conventional tDCS that affects large sections of the brain and may not sufficiently increase efficacy to justify complicating procedures, say with pre-treatment fMRI mapping. The same argument can also apply to HD-tDCS, which is more focal than conventional tDCS but not as focal as TMS and may not require precision targeting. To address these questions, more empirical research is needed to quantify and compare the treatment effect sizes with and without targeting across neurological cohorts and applications of tDCS, including in PWA. We hypothesize that precise targeting with conventional or HD-tDCS would improve tDCS efficacy, particularly in patients with brain damage, by reducing individual variability, but this remains to be tested in future studies.
3.4Implementation of HD-tDCS
To our knowledge, only 2 published studies have implemented HD-tDCS with linguistic therapies for the treatment of aphasia after stroke. Richardson et al. compared the efficacy of sponge-based vs. HD-tDCS (5 sessions per arm, 1 mA) during a computerized anomia treatment in 8 PWA. Individualized targeting procedures were implemented to determine stimulation targets. While numerically higher naming accuracy was found after HD-tDCS in most patients, the difference was not significant compared to sponge-based tDCS (Richardson et al., 2015). The other study in PWA is from our group and evaluated transient changes after a single 20-minute session of HD-tDCS on sentence repetition accuracy. As described earlier, rsMEG analysis was used to localize region(s) exhibiting perilesional oscillatory dysfunction in individual PWA, which were then targeted using HD-tDCS to correct or normalize the abnormalities. Anodal HD-tDCS significantly improved repetition accuracy, and cathodal HD-tDCS showed weaker effects, both compared to sham tDCS (Shah-Basak et al., 2020).
While evidence for the implementation of HD-tDCS in PWA is lacking, several studies have implemented HD-tDCS in normal healthy volunteers to modulate language processes (Table 2), involving vocal motor control, rapid talker adaptation, action sentence processing, noun and verb retrieval, lexical-semantic integration, assembled phonology and creativity in verb generation. We summarize these studies below.
Table 2
Summary of HD-tDCS studies in healthy controls
Source | Sample size | Stimulation protocol | Target and Montage | Sham Condition | Language process assessed | Outcomes | Study design |
Behroozmand et al. (2020) | Experiment 1: N = 30. Age: Mean = 21, Range = 18–25 Experiment 2: N = 26. Age: Mean = 22, Range = 18–28 | Protocol: Experiment 1: Participants were pseudorandomly assigned to one of three groups (N = 10) matched for age and gender, to receive anodal, cathodal or sham stimulation. Each subject had two experimental sessions with minimum of 4 days apart, for an average of 11.6 days (SD 7.6; range 4–32). Intensity: 2 milliamps (mA) Experiment 2: Participants were pseudorandomly assigned to one of two groups (N = 13) matched for age and gender, to receive either 1 mA or 2 mA cathodal stimulation. | Target: Left ventral portion of the pre-central gyrus (MNI: x = –57, y = 3, z = 14) Montage: Electrodes were positioned at locations FC5, F7, C1, TP7, and AF7 using standard 10–20 EEG cap | Current was applied at 1 mA with two electrodes positioned over the inferior occipital cortex. | Vocal pitch motor control | In experiment 1, both anodal and cathodal HD-tDCS enhanced voice motor control. The pitch frequency of the vocalized vowel sounds was more stable and less susceptible to externally altered auditory feedback under both anodal and cathodal tDCS conditions. In experiment 2, cathodal tDCS conditions with 1 mA vs. 2 mA effects were comparable. | Blinded, between-subject design |
Choi and Perrachione (2019) | N = 60 Age: Mean = 20.4, Range = 18–31 | Protocol: Participants were assigned to groups receiving either sham (N = 20), anodal (N = 20), or cathodal (N = 20) while performing a word identification task. Intensity: 2 mA | Target: Left superior temporal cortex Montage: Electrode placement used the standard 10-10 EEG system were at T7 and TP7 and return electrodes at C3, CP3, PO7 and F7. | Current was ramped up to 2 mA over 30 seconds then ramped back down over the next 30 seconds. | Rapid talker adaptation in speech processing | Compared to sham, both anodal and cathodal stimulation significantly disrupted rapid talker adaptation in connected speech on a timescale as short as within a sentence, but not its ability to adapt over longer timescales. No differences in processing isolated words were found across stimulation conditions. | Mixed between and within-subjects design |
Perceval et al. (2017) | N = 50 Age: Mean = 23.16, SD = 3.79 | Protocol: Participants were stratified by baseline learning ability and pairwise randomized to active anodal (20 mins; N = 25) or sham (40 seconds; N = 25). Current was ramped up before the first training block (word-picture learning) over 10 seconds to 1 mA, remained constant for 20 mins, then ramped down over 10 seconds. Intensity: 1 mA | Target: Left temporo-parietal cortex Montage: Electrodes placement used a 10–20 EEG system with center electrode at CP5 with surrounding return electrodes. | The current was ramped up and down in the same way as anodal but remained constant for 40 seconds. | Novel word learning | Active HD-tDCS resulted in faster retrieval of correct word-picture pairs, but accuracy was unaffected | Double-blind, between-subjects design |
Price et al. (2016) | N = 18 Age: Mean = 25.3, Range = 20–39 | Protocol: Each participant completed three separate brain-stimulation sessions (anodal left angular gyrus (AG), anodal right AG, and sham), for a total of 54 sessions. The sessions were scheduled at the same time of day for each participant and were a minimum of 48 h apart. HD-tDCS is administered while participants perform a two-word comprehension task. For the active anodal brain-stimulation sessions, a constant current of 2 mA was delivered for a period of 20 min, preceded and followed by linear ramp-up and ramp-down periods of 30s. Intensity: 2 mA | Target: Left AG (MNI: –52, –56, 22). Right AG (MNI: 52, –56, 22) Montage: Electrodes placement used a 10–20 EEG system in a 4×1 montage. Left AG central electrode was placed at CP5, surrounded by return electrodes at C3, T7, P7, and P3. Right AG used a mirror coordinate montage. | A constant current of 2 mA for a period of 30 s preceded and followed by the same ramp-up and ramp-down periods of 30 s, and then followed by 19.5 min of no stimulation. | Lexical-semantic integration | Compared to sham and right AG, anodal stimulation to the left AG resulted in faster comprehension of semantically meaningful combinations. The size of the effect from brain stimulation correlated with the degree of semantic coherence between word pairs, demonstrating left AG integrates lexical-semantic information and HD-tDCS modulate integrative processes in semantic memory. | Within-subjects design, blinded design |
Malyutina and Ouden (2015) | N = 27 Age: Mean = 22.1, SD 3.2, Range 18–31 | Protocol: Participants received stimulation either of Broca’s area (N = 14) or of the left AG (N = 13), each received 20 min of anodal, cathodal, and sham stimulation on separate days. Stimulation sessions separated by a minimum of 24 hr (Mean 2.8 days, SD 3.0, range 1–10), followed by naming and lexical decision tasks with single-word verb and noun stimuli. Intensity: 2 mA | Target: Left Broca’s (MNI: –49, 16, 24). Left AG (MNI: –27, –57, 51) Montage: Electrodes placement used a 10-10 EEG cap. Frontal target anodal and cathodal electrodes were placed at FC5 and AFz. AG electrodes were at CP5 and POz. Frontal target density was 0.61 V/m, AG was 0.16 V/m. | Corresponding frontal sham electrodes were placed at AFz and FPz or FC5 and FC3 with density estimated to be < = 0.11 V/m at both locations. For AG stimulation, sham is placed at CP5 and TP7. | Noun and Verb Retrieval in Naming and Lexical Decision | Cathodal stimulation over both areas increased naming speed for verbs and nouns, but no effects were found for the lexical decision task. | Randomized, blinded design |
Johari et al. (2021) | N = 21 Age: Mean = 24.2, SD 3, Range 18–29 | Protocol: Half of the subjects received cathodal stimulation in the first session following with sham stimulation in the second session. The other half was reversed. Stimulation sessions were separated by a minimum of 24 hr (range: 1–24 days). Half of the sentences from each condition were used during stimulation of the targeted area, while the other half was used during sham. Stimulation lasted 20 min. Intensity: 1.85 mA | Target: Left-hemisphere hand motor cortex (MNI: –57, 3, 14) Montage: Electrode placement used a 10–20 EEG cap. In the cathodal group, electrodes were positioned at CP3, Cz, C1, FC3, and FC1. | Electrodes were positioned at FC3, FC1, CP1, and CP3. | Figurative and literal action sentence processing | Cathodal HD-tDCS to the motor cortex significantly facilitated performance on literal, idiomatic, and metaphoric action sentences relative to the visual sentences control condition.The effect of this modulation differs depending on the action-related content of the sentences. | Randomized, blinded design |
Green et al. (2017) | N = 23 Age: Mean = 21.69, SD = 3.09 | Protocol: Half of the participants received anodal tDCS and the other half received sham for the first 20 min of the testing session. Stimulation was delivered for the first 20 minutes with a 30 seconds ramp up and down of an analogy finding task and slice creativity verb generation task. Intensity: 2 mA | Target: Left frontopolar cortex Montage: Electrode placement used a 10-10 EEG cap in a 4×1 montage. Anodal electrode at AF3, return electrodes at FPz, Fz, F7, and FC3. | Sham was applied with a 30 seconds ramp up, then 15 seconds later ramped down over 30 seconds. | Creative analogical reasoning and creativity in verb generation | HD-tDCS was effective in eliciting more creative analogical reasoning without decreasing the meaningfulness of analogies. Participants interacted more with creativity cueing to generate creative verbs. | Randomized, blinded design |
Xue et al. (2017) | N = 46 Age: Mean = 19.67, SD = 1.81, Range = 18 to 25 | Protocol: Two matched groups of participants received anodal stimulation either on left temporoparietal cortex (LTPC) or on the control site at visual cortex (VC) prior to training at Session 1 (day 1) and Session2 (day 3). Stimulation was applied for 20 min, with a 30 seconds ramp up and down. Intensity: 1.5 mA | Target: LTPC or VC Montage: Electrode placement used a 10–20 EEG cap in a 4×1 montage. LTPC electrodes were halfway between T3 and P3, with four return electrodes at P5, CP1, Pz, and PO7. | Control site was at the VC, with a montage electrodes placement at Oz, with return electrodes at PO3, O1, PO4, and O2. | Assembled phonology, learning to read an alphabetic language (map graphemes to phonemes) | LPTC HD-tDCS enhanced learning of assembled phonology and its effect lasted for four days post-stimulation. | Randomized, blinded design |
Behroozmand et al. (2020) used HD-tDCS targeted to the left ventral motor cortex to modulate vocal pitch motor control in response to pitch-shift stimuli in 30 healthy participants. Participants were pseudorandomized to receive anodal-, cathodal- or sham-tDCS. In the anodal- and cathodal-tDCS groups, intensity was set to 2 mA and electrodes were positioned at locations FC5, F7, C1, TP7, and AF7 using standard 10–20 EEG cap. In the sham group, HD-tDCS was applied at 1 mA with two electrodes positioned over the inferior occipital cortex. Both anodal and cathodal HD-tDCS enhanced voice motor control. The pitch frequency of the vocalized vowel sounds was more stable and less susceptible to externally altered auditory feedback under both anodal and cathodal tDCS conditions (Behroozmand et al., 2020). In a sub-experiment with a different cohort of 26 participants, cathodal tDCS conditions with 1 mA vs. 2 mA were compared. The effects of 1 mA and 2 mA were comparable.
Choi and Perrachione (2019) used HD-tDCS to modulate rapid talker adaptation and speech processing in 60 healthy participants, randomly assigned to receive anodal-, cathodal-, or sham-tDCS. In the anodal and cathodal groups, HD-tDCS was applied at 2 mA targeted to the left superior temporal cortex. Electrode placement was the same across participants using the standard a 10-10 EEG system at T7 and TP7 and return electrodes at C3, CP3, PO7 and F7. In sham, current was ramped up to 2 mA over 30 seconds then ramped back down over the next 30 seconds. Participants completed a word identification task during stimulation. Compared to sham, both anodal and cathodal stimulation significantly disrupted rapid talker adaptation in connected speech. No differences in processing isolated words were found across stimulation conditions. The authors concluded that stimulation of the left superior temporal lobe impaired the ability to rapidly adapt to a talker on a timescale as short as within a sentence, but not its ability to adapt over longer timescales (Choi & Perrachione, 2019).
Perceval et al. (2017) used HD-tDCS to study access to newly learned words in 50 healthy participants, randomized to active anodal or sham groups. HD-tDCS was applied at 1 mA intensity targeted over the left temporo-pareital cortex for 20 minutes per session. Electrodes were positioned using a 10–20 EEG system with center electrode at CP5 with a surrounding return electrodes. Participants learned associations between line drawings of Finnish farming equipment and a non-word “name”. Active HD-tDCS resulted in faster retrieval of correct word-picture pairs, but accuracy was unaffected (Perceval et al., 2017).
Price et al. (2016) examined lexical-semantic integration using HD-tDCS in 18 healthy participants. HD-tDCS was applied at 2 mA for 20 minutes over the left angular gyrus (AG), right AG or sham in a within-subject design. Electrodes were positioned using the 10–20 EEG system in a 4×1 montage. For left AG, central electrode was placed at CP5, surrounded by return electrodes at C3, T7, P7, and P3. For right AG, a mirror coordinate montage was used. During stimulation, participants completed a two-word comprehension task. Left AG anodal stimulation modulated semantic integration with faster comprehension of semantically meaningful combinations compared to sham-tDCS (A.R. Price et al., 2016).
Malyutina and Ouden (2015) examined noun and verb retrieval in naming and lexical decision with HD-tDCS in 27 healthy participants, randomly assigned to receive left inferior frontal (N = 14) or left AG stimulation (N = 13). HD-tDCS was applied at 2 mA using a 10-10 EEG cap. Electrode montages were chosen based on FE modeling using a template adult brain and HD-TargetTM and HD-ExploreTM software packages. Electrode placements optimized current intensity at the target location, while minimizing the intensity at the non-target. For frontal stimulation, anodal and cathodal active electrodes were placed at FC5 and AFz, which resulted in 0.61 V/m density in frontal target compared to 0.16 V/m in AG. Corresponding sham, electrodes were placed at AFz and FPz or FC5 and FC3 with density estimated to be < = 0.11 V/m at both locations. For AG stimulation, anodal and cathodal electrodes were placed at CP5 and POz, and sham placed at CP5 and TP7. The sham montages were chosen such that the current would bypass the cortex. During stimulation, participants completed a jigsaw puzzle. After stimulation, participants completed overt naming and lexical (word or non-word) decision tasks. Cathodal stimulation over both areas increased naming speed for verbs and nouns, but no effects were found for the lexical decision task (Malyutina & Ouden, 2015).
Johari et al. (2021) used HD-tDCS to modulate figurative and literal action sentence processing in 21 healthy participants, randomly assigned and blinded to receive either cathodal or sham stimulations first. HD-tDCS was applied at 1.85 mA for 20 minutes targeting the left-hand motor cortex using a standard 10–20 EEG cap. In the cathodal group, electrodes were positioned at CP3, Cz, C1, FC3, and FC1. In the sham group, electrodes were positioned at FC3, FC1, CP1, and CP3 so current can bypass the cortex. Cathodal HD-tDCS to the motor cortex significantly facilitated comprehension of literal, idiomatic, and metaphoric action sentences relative to the visual sentences control condition (Johari et al., 2021).
Green et al. (2017) examined creativity in verb generation using HD-tDCS in 23 healthy participants, randomly assigned to receive anodal- or sham- tDCS. HD-tDCS was applied at 2 mA over the left frontopolar cortex using a 10-10 EEG cap. A 4×1 montage was used with anodal electrode at AF3, and return electrodes at FPz, Fz, F7, and FC3. Stimulation was delivered for the first 20 minutes of an analogy finding task and slice creativity verb generation task. HD-tDCS was effective in eliciting more creative analogical reasoning without decreasing the meaningfulness of analogies. Participants interacted more with creativity cueing to generate creative verbs (Green et al., 2017).
Xue et al. (2017) used HD-tDCS to modulate assembled phonology in 26 healthy native Chinese speakers, who were randomly assigned and blinded to receive anodal stimulation on left temporoparietal cortex (LTPC) or the control site on the visual cortex. HD-tDCS was applied at 1.5 mA in a 4×1 ring montage using a 10–20 EEG cap. For anodal LTPC stimulation, electrodes were located halfway between T3 and P3, with four return electrodes at P5, CP1, Pz, and PO7. For the control visual cortex stimulation, electrodes were located at Oz, with return electrodes at PO3, O1, PO4, and O2. Stimulation was delivered prior to two training sessions of an artificial language based on Korean Hangul characters. LPTC HD-tDCS enhanced learning of assembled phonology and its effect lasted for four days post-stimulation (Xue et al., 2017).
While different electrode configurations were used across these studies, the 4×1 ring configuration appears to be the most popular montage for language studies. Template modeling of current flow was implemented in one study to guide placement of electrodes to maximize intensity at the target location (Malyutina & Ouden, 2015). None of the studies adopted an individualized approach to targeting. The coarse location of stimulation targets was informed by prior functional imaging studies, linking brain regions to the language function of interest. HD-tDCS was found to be safe and tolerable in studies that did report these data. Across these studies, mild tingling, mild pain, and mild burning sensations are typically reported with HD-tDCS. But overall pain, discomfort or unpleasantness are rated low (Behroozmand et al., 2020; Choi & Perrachione, 2019; Malyutina & Ouden, 2015; Perceval et al., 2017; A.R. Price et al., 2016). The sensations are reported to gradually reduce over the duration of a session (Behroozmand et al., 2020). Two studies that reported blinding outcomes indicated that participants could not distinguish scalp sensations between sham and active stimulation (Behroozmand et al., 2020; Choi & Perrachione, 2019). Overall, it appears that the use of HD-tDCS would be acceptable in PWA with minimal side effects.
Future studies should further evaluate the relative effect sizes of conventional vs. HD-tDCS to determine whether focal stimulation provides any benefit over diffused stimulation modality, particularly in patients with brain damage. It is also unclear from the current literature whether individualized targeting with HD-tDCS or conventional tDCS would be superior to no targeting (as in using 10/20 EEG system) in patients and should be systematically studied in future applications of tDCS.
3.5Targeting ancillary systems
Several other investigators have also explored the effectiveness of stimulation targets that are located outside of the canonical language network but are structurally and functionally connected to it. The rationale for this approach is to modulate excitability in preserved systems supporting language. Previous models that we discussed involved targeting seemingly spared perilesional regions. But these regions may be affected by encephalomalacia, and surrounding lesions filled with cerebrospinal fluid may distort or shunt current flow in unpredictable ways, weakening the effect of stimulation (van der Cruijsen et al., 2021). The problem with targeting right hemisphere is variability in recruitment of these areas and only a partial understanding of its role during recovery (Heath et al., 2012; Mohr et al., 2014; Turkeltaub, 2015). There is a risk that inhibiting these regions would interfere with recovery or impair language skills normally associated with these regions (e.g., semantics, pragmatic skills, prosody) (de Aguiar, Paolazzi, et al., 2015).
Two ancillary systems that may indirectly facilitate processing of the residual language regions are primary motor cortex (Darkow et al., 2017; Meinzer et al., 2016; Xu et al., 2021) and cerebellum (Sebastian et al., 2017, 2020; Turkeltaub et al., 2016). Both primary motor cortex and cerebellum are usually preserved in individuals with perisylvian lesions and thus may provide targets that can be easily administered and feasible in clinical environments (Grimaldi et al., 2016; P.A. Pope & Miall, 2012; Pulvermüller, 2005; Vukovic et al., 2021). Previous studies indicate a tight link between motor and language systems in healthy controls and PWA (Darkow et al., 2017; Meinzer et al., 2016). In healthy controls, stimulation of the motor cortex with anodal tDCS is shown to facilitate language function, whereas cathodal stimulation is shown to impair language processing (Pulvermüller, 2005; Vukovic et al., 2021).
In one PWA, Datta et al. (2011) reported improved naming after administration of anodal tDCS to the left primary motor cortex (M1) (Datta et al., 2011). In a larger randomized, double-blind and sham controlled study, Meinzer et al. (2016) investigated effectiveness of 2 weeks of anodal tDCS administered to left M1 in combination with intensive naming therapy in 26 PWA. Significant improvements on naming accuracy of trained items and generalization to untrained items were reported in active tDCS compared to the sham group, and the effects were maintained at 6 months follow up. They also reported significant gains in functional communication after anodal tDCS assessed immediately after the intervention and 6-months later (Meinzer et al., 2016). In a different study, Xu et al. (2011) used iTBS to stimulate left M1 hand area in 16 PWA and measured its immediate effects on brain connectivity using resting-state fMRI. The immediate functional effects of the iTBS intervention were assessed using regional, seed-based connectivity and graph-based measures. Induced functional connectivity changes were reported in the motor and language networks (Xu et al., 2021). These results suggest that stimulation of preserved primary motor cortex may induce extensive neuroplastic changes including in the language network.
Recently several studies have targeted the right cerebellum using tDCS. Although traditionally cerebellum has been associated with motor and articulatory functions, more recent evidence points to its involvement in multiple cognitive and language processes (Mariën et al., 2014). Right cerebellum often coactivates with left hemisphere regions during a variety of language tasks, including verbal fluency, verb generation, and semantic association tasks (De Smet et al., 2013; Mangano et al., 2015; Schmahmann, 2019; Senaha et al., 2005). Damage to the cerebellum is associated with deficits in phonemic and semantic fluency, syntactic processing, naming and grammar (De Smet et al., 2013; Mariën et al., 2014). The right posterolateral cerebellum has anatomical and functional connections with the left temporal, parietal and inferior frontal language regions. Given right cerebellum’s role in learning and language, and connections with the left language cortical areas, it has been proposed that cerebellar neuromodulation can facilitate excitability of left hemisphere language regions and in turn aphasia recovery (D’Mello et al., 2017; Pope & Miall, 2012; Sebastian et al., 2017; Turkeltaub et al., 2016). As the mechanism of action, it has been suggested that cerebellar tDCS modulates activity in the Purkinje cells and this affects level of activity in the deep cerebellar output nuclei, which exerts facilitatory or inhibitory input on the distant cortical regions (Marangolo et al., 2018). For example, anodal tDCS to the cerebellum through its excitatory effect on Purkinje cells has been shown to increase the inhibitory input from the cerebellar nuclei to the frontal cortex, whereas cathodal stimulation reduces Purkinje cells inhibition and increases activity in the left cerebral language cortices (Pope & Miall, 2014).
Consistent with this idea, Turkeltaub et al. (2016) applied anodal, cathodal or sham tDCS to the right posterolateral cerebellum in healthy participants during articulation and verbal fluency tasks. Both anodal and cathodal cerebellar tDCS were effective in improving verbal fluency (Turkeltaub et al., 2016). Anodal tDCS increased functional connectivity between the cerebellum and cortical areas involved in the motor control of speech and enhanced the correlations between language and speech-motor regions in the left hemisphere. Following on the results of that study, DeMarco et al. (2021) applied anodal tDCS during SLT to the right posterolateral cerebellum in 24 PWA. The only reliable effect of active vs. sham stimulation was found for the mean utterance length on the picture description task (DeMarco et al., 2021). In a different study, Sebastian et al. (2017) applied 15 sessions of anodal tDCS to the right cerebellum combined with spelling treatment in one PWA. They documented greater improvement on spelling to dictation with anodal tDCS compared to sham for both trained and untrained words (Sebastian et al., 2017). Generalization to written picture naming was observed with tDCS but not sham condition. Resting state functional connectivity analysis revealed increased connectivity between right cerebellum and perilesional language regions 2 months after the intervention and it was related to improvement in spelling. In another study, Marangolo et al. (2018) applied cathodal and sham tDCS to the right cerebellum in 12 PWA during a verb generation task and a verb naming task, over 5 days. Significant improvements were found after cathodal tDCS on the verb generation task. There were no differences between tDCS and sham conditions for the naming task (Marangolo et al., 2018).
These preliminary results suggest that neuromodulation of a distal site that is functionally and/or anatomically connected to the left hemisphere language regions can be an effective approach for language neurorehabilitation in chronic post-stroke aphasia.
4Translation to clinical practice
Another important consideration is the functional efficacy of NIBS treatments. A recent meta-analysis on aphasia studies using tDCS showed no evidence of treatment transfer from specific linguistic outcome to functional communication (i.e., translation of specific improvements in naming a picture of an object to day-to-day communication) (Elsner et al., 2019, 2020).
The ultimate goal of language rehabilitation with NIBS is to achieve clinically meaningful gains with lasting impact on daily communication abilities. Improving functional communication is vital to translating the use of NIBS to routine clinical practice. An essential intermediate step(s) to achieve this goal is to examine factors that are important for generalization or transfer of effects from therapies paired with NIBS that target specific linguistic skills to broader gains in functional communication and real-world social interactions. We rationalize that for NIBS to be accepted widely as part of routine clinical practice, it must promote generalization with much larger effect sizes than aphasia therapy alone and make a significant impact on daily communicative needs. In the sections below, we define the term generalization in the context of aphasia therapies and functional communication, summarize behavioral strategies that are implemented to strengthen language generalization, and the small evidence base on generalization of aphasia therapies paired with NIBS with a focus on tDCS studies.
4.1Generalization
Broadly, generalization is described as “effects that extend beyond the immediate focus of treatment” to untreated behaviors, observed in different contexts or domains of communication (Stokes & Baer, 1977; Webster et al., 2015a). Based on psycholinguistic and neurobiological models of language processing, several strategies have been adopted for developing aphasia therapies that facilitate generalization, which we will summarize in the section below.
Generalization can be assessed across at least 3 dimensions, including distance, context, and modality/level. Given a language therapy, comparing performance on treated vs. untreated items is more proximal (near) to the therapy than assessment of performance changes on everyday communication abilities (far), if not directly targeted during therapy. Generalization can be elicited in different environments or contexts, say if treated items after therapy are used with people and/or in everyday scenario outside of routine clinical setting. Generalization can occur cross-modally, (e.g., therapies targeting production benefit comprehension abilities), or across-linguistic levels, (e.g., therapy focused on production of single words benefits production of sentences). Relatedly, generalization is often described as either response generalization or “emergence of untrained language response”, or stimulus generalization or “transfer of trained behavior in a different context” (Webster et al., 2015a). Improvement on treated and untreated words after a word retrieval therapy is an example of response generalization, whereas retrieval of treated words (restricted to specific words that are treated during therapy) evoked under different conditions (e.g., in a narrative context) is an example of stimulus generalization. Cross-linguistic generalization requires change in stimulus or condition that evokes the response and thus falls under stimulus generalization. Changes within a linguistic level fall under response generalization, as the type of stimulus that evokes the response is the same (Webster et al., 2015a).
4.2Aphasia treatment strategies to strengthen generalization
Numerous linguistically motived strategies have been adopted to strengthen generalization of aphasia therapies, published as single case studies, case series and small group studies. While comprehensive discussion of all these strategies is beyond the scope of this review (excellent reviews are already published (e.g., Webster et al., 2015b)), we summarize a few strategies with promising findings from case-series, group-level studies, and meta-analyses (Table 3).
Table 3
Aphasia treatment strategies to strengthen generalization
Treatment | Broad impairment targets | Specific language process targets | Training | Studies | Generalization findings |
Semantic feature analysis (SFA) | Word retrieval difficulties | Lexical-semantics | Patient names a picture of an object based on a description of its semantic features (e.g., its superordinate group, physical properties, usage, etc.). The training includes lists of words representing several object categories, with the premise that training of overlapping feature sets will strengthen the semantic system and augment generalization to untrained words. | Meta-analysis by Quiques, Y, et al. (2019); Gilmore, N, et al. (2020). | SFA promotes generalization for naming untreated or untrained stimuli, with a larger generalization for related items. |
Phonomotor treatment (PMT) | Word retrieval difficulties | Phonological To rebuild and strength phonological representations with a variety of tasks. | Patient engages phonology initially through recognizing, producing, and manipulating phonemes in isolation, then progressing to longer phoneme sequences in words and non-words. Tasks involve a variety of representations that include visual, acoustic, orthographic, motor and tactile (e.g., visual feedback, auditory perceptual discrimination tasks, etc.). | Kendall et al. (2015); Silkes et al. (2019); Madden et al. (2021); Brookshire et al. (2014). | PMT promotes generalization for naming trained nouns, and untrained nouns with sustained effects; improvements in discourse production reading are also reported. |
Treatment Sequence for Phonological Alexia/Agraphia | Reading, spelling, and written narratives | Sublexical phonological skills To train the interactive use of sublexical and lexical information. | In a two-stage treatment procedure, patient is first retrained on the grapheme to -phoneme correspondence and then engages in phonological manipulation tasks (e.g. blending of phonemes into words and non-words or deleting/replacing phonemes in words and non-words) | Beeson et al. (2010, 2018) DeMarco et al. (2010) | Significant generalization to reading and spelling of words and non-words as well as written narratives (grammatical construction, functors, and morphological markers). |
Complexity Account of Treatment Efficacy (CATE) | Word retrieval difficulties | Semantic, phonological or syntactic domain | For e.g., CATE in the syntactic domain: Patients are trained to comprehend and produce atypical or complex sentence structures with wh-movement (e.g., from least to most complex: who-questions, object clefts, object-relative constructions). Patients are tested with untrained sentences and narrative language samples. | Thompson and Shapiro (2007); Swiderski et al (2021). | Significant generalization to simpler sentence structures from complex, treated sentences, limited to the same structural family. |
Verb network Strengthening Treatment (VNeST) | Word retrieval difficulties | Lexical-semantics. Retrieval of verbs and their thematic roles to facilitate access to content words | Patient generates an agent (doer of action or thematic roles e.g., carpenter, chef) and patient (receiver of action e.g., lumber sugar) pairs to a target transitive verb (e.g., measure). Patient generates transitive verbs (e.g., gives or plays), reads the generated sentences, expanding on them using wh-questions to activate their related contexts, makes semantic judgments about similar generated sentences, and produces the target verb independently. | Edmonds et al. (2009); Edmonds et al. (2015); Edmonds (2016). | VNeST promotes generalization for naming single noun and verbs and retrieval in sentences (containing trained and untrained items), including cross-linguistic generalization to discourse production or connected speech and to untrained sentence and discourse contexts. |
ACTION | Word retrieval difficulties | Lexical verb retrieval by addressing grammatical properties of verbs | Training at lexical level during action naming, training at syntactic and morphosyntactic levels during sentence completion tasks with infinitive and finite verbs, and the final step requiring sentence construction | de Aguiar et al. (2015) | ACTION promotes improvement on untrained infinitives and finite verbs; generalization to verbal communication also reported. |
Integrated discourse treatment for aphasia (IDTA) | Discourse difficulties | Multilevel cross-linguistic. Problem-based learning | Patients are trained to solve real-world problems (e.g., conversing on a topic), incorporate personal relevance (e.g., using names of family members), and training of specific linguistic components (word retrieval based on semantic, phonological and orthographic cueing hierarchy, and sentence production) as well as their usage in connected speech (discourse training with response elaboration treatment). | Milman (2016) | IDTA allows generalization to related linguistic structures, standardized tests of language and cognition, and discourse production. *The evidence is considered preliminary due to small sample sizes. |
Novel Approach to Real-life communication: Narrative Intervention in Aphasia (NARNIA) | Discourse difficulties | Multilevel cross-linguistic. Metalinguistic premise | Patients are trained with picture sequences, including identifying events in pictures, accessing verbs and relevant nouns, and creating complete argument structures within sentences. The patients are then trained on structural elements of everyday discourse genres (e.g., story-telling or narrative, explaining a procedure or procedural, event recounting, and providing opinions or exposition), including sentences to set the scene (the beginning), events taking place (the middle) and conclusion of the story (the end). Other genres are introduced using a similar framework. A mind-mapping format with visual prompts is used to help and is gradually decreased as therapy progresses. Once coherence aspects are learned, cohesion is targeted through the use of connectives. | Whitworth et al. (2015) | NARNIA promotes generalization for verb and argument structures production, untreated nouns and verbs and untreated discourse genres. |
Cognitive-linguistic approaches | |||||
Cognitive Flexibility in Aphasia Therapy (CFAT) | Everyday communication | Verbal cognitive flexibility and semantic fluency | Integrates characteristics of cognitive flexibility, including response to change of topic and misunderstandings and use of nonverbal communication. | Spitzer et al. (2021) | CFAT promotes verbal cognitive flexibility and semantic fluency. Individuals with impaired verbal cognitive flexibility may benefit from this specific cognitive and linguistic training than linguistic approaches alone. |
Language-specific attention treatment (L-SAT) | Attention | Language-specific attention | Language tasks and stimuli that impose increasing attentional demands on lexical and sentence processing. For example, dual processing tasks for lexical items to promote attention allocation, and sentence tasks that focus attention during language processing using non-canonical sentence structure, grounding elements, event windowing, and anaphoric references, among others. | Peach et al. (2019) | L-SAT effects are larger than DAT for standardized WAB-AQ assessments, functional language, and patient-reported outcomes. |
Auditory-verbal WM and short-term memory (STM) | Word retrieval | WM and phonological processing | WM training provided with phonological component analysis (PCA) | Simic et al. (2022); Case study N = 1 | Larger gains in naming accuracy and generalization to verbal communication with WM and PCA training |
WM training | – | WM/STM | N-back tasks with pictures or words/letters; | Salis et al. (2017); Zakariás et al. (2018) | Improvements on sentence comprehension and functional communication; large variability across patients exists. |
Repetition and span treatment | Verbal STM | Maintenance of activation of representations | STM treatments include repetition of phrases or sentences, words or nonwords, or matching listening span tasks with no spoken output. Treatment included delay between hearing and repeating stimuli of varying durations (up to 10 s) the delay was filled (e.g., unison counting) or unfilled. One study provided cuing hierarchical feedback. | Martin et al. (2018); Kalinyak-Fliszar et al. (2011); Martin et al. (2020) | Generalization to measures of WM and STM load and verbal STM span; large variability found across patients. |
Most strategies focus on response generalization, specifically generalization within a linguistic level. For example, many therapies involve production, targeting noun or verb retrieval at the single word level, followed by an assessment of transfer to untreated words. Strategies based on the spreading activation theory (Collins & Loftus, 1975) are applied most often, where generalization is expected to untrained items that share some linguistic properties (semantic or phonological) with the items trained during therapy. The best example of this strategy is the semantic feature analysis (SFA) treatment, which is hypothesized to promote generalization first by lateral spreading of activation within the semantic network from one concept to related concepts. Activating concepts by cueing semantic properties or features related to a target item is expected to increase activation of semantically related concepts. Downstream effects to phoneme-level lexical representations are also expected whereby strongly activated phonological representations are selected. Thus, retrieval of the target item is thought to strengthen representations and access to its semantic neighbors that are not directly treated but share semantic features with the treated items, facilitating within-level generalization (Gilmore et al., 2020; Quique et al., 2019). A recent meta-analysis of SFA outcomes, including 35 PWA from 12 studies, found that SFA promotes generalization to untreated stimuli, albeit the treatment gains are smaller on untreated than treated stimuli. Consistent with the hypothesized mechanism of SFA, generalization was found to be larger for related than unrelated items (Quique et al., 2019). Another recent meta-analysis on SFA also reported group-level evidence of response generalization for naming to untreated items in a relatively large sample of patients (Oh et al., 2016). Reduction in aphasia severity on standardized aphasia assessment (WAB-AQ) was also reported in this study.
A strategy targeting phonology to promote generalization is the phonomotor treatment (PMT) approach. PMT is derived from the Lindamood Phoneme Sequencing program for developmental dyslexia (Lindamood & Lindamood, 1998) and is based on the assumption rooted in some connectionist models (Nadeau, 2001) that phonology is a fundamental building block of all language processing. Within the connectionist architecture, representations are thought to emerge from co-activation patterns of elements across primary domains of language processing: concept representations (semantics), phonemic, phonological and orthographic representations. Following damage to this massively interconnected network, the goal of PMT is to rebuild and strengthen phonological representations and the ability to manipulate them across perception and production and thus improve the efficiency and accuracy across all levels of language processing (Kendall et al., 2015). This is accomplished by therapeutic tasks that engage phonology through a variety of visual, acoustic, orthographic, motor and tactile representations. Sixty hours of PMT was associated with medium to large gains in naming of trained nouns, and small to medium gains in naming of untrained nouns immediately and 3 months after treatment (Kendall et al., 2015). The benefits also generalized across levels to discourse production (Silkes et al., 2019). While most PMT research has focused on word retrieval outcomes, several studies reported benefits of PMT to reading ability (Brookshire, Conway, et al., 2014; Madden et al., 2021).
Another treatment that focuses on strengthening central phonological processing skills, including sublexical letter-to-sound mapping and phonological manipulation, has been shown to induce positive outcomes in PWA. This treatment implements therapeutic tasks that retrain transcoding from letters to sounds and vice versa. More complex phonology is trained using tasks, such as deleting and replacing phonemes in words and blending individual phonemes into words (Beeson et al., 2010). Following this treatment sequence, PWA have shown improvement in transcoding and phonological manipulation skills (DeMarco et al., 2018). More importantly, several studies have demonstrated generalized gains to written language production, including reading and spelling of words and non-words as well as increased accuracy of written narratives (Nickels et al., 2023), with greater inclusion of morphological markers and functors.
Another interesting strategy to increase generalization, irrespective of the linguistic domain (as in semantic, phonological, syntactic), is grounded in complexity-based theories as it pertains to the language material used during therapy. The hypothesis is that more complex linguistic materials can be used to promote generalization to not only complex but down to simpler structures as well. Overall language learning is increased when planned therapies incorporate more complex than simple language material, which is counterintuitive to most treatment approaches that begin with simple structures and build up to more complex structures. This approach has been tested with treatment focused on phonological, semantic, and syntactic domains. One important aspect proposed by Thompson and colleagues (2003) under this Complexity Account of Treatment Efficacy (CATE) is that complexity effect only emerges “when untreated structures encompass processes relevant to (i.e., are in a subset relation to) treated ones” (Thompson et al., 2003). The trained and untrained material must be linguistically related and organized in a hierarchical fashion, by carefully considering relevant psycholinguistic factors within the domain of interest. For example, in studies by Kiran et al. (2003, 2007), complexity of training items during the SFA was modulated using only the atypical items within a semantic category (e.g., penguin in the category bird) (Gilmore et al., 2020; Kiran, 2007; Kiran & Thompson, 2003). Atypical items are more complex in that they contain distinctive features or share fewer features with other exemplars within the category. Atypical items are accessed slower and less accurately than typical items. Training features related to atypical items is hypothesized to activate analysis of both atypical and typical items, thus strengthening access to both atypical and typical items within the same therapy modality.
Similarly, CATE is applied to increase generalization of SFA treatment by modulating concreteness/imageability of language material used during training (Sandberg & Kiran, 2014). In patients with agrammatic aphasia, Thompson and Shapiro (2007) adopted CATE in the syntactic domain by training complex sentence structures such as object relative structures with wh-movement, which resulted in generalization to simpler structures (Thompson & Shapiro, 2007). A recent meta-analysis with aggregated data from 46 PWA from 13 studies reported significant generalization of treatment of underlying forms (TUF) to less complex sentence structures from more complex, treated sentences. Consistent with CATE, generalization was limited to sentences that belonged to the same structural family as the treated sentences (Swiderski et al., 2021). While evidence related to CATE is compelling, more research is needed to characterize the impact of variables such as type of treatment, frequency of treatment delivery, individual variability in impairment profiles, stroke-related factors and demographics, and how these may interact with complexity (Thompson, 2007). In addition, effects of this approach on stimulus generalization (across levels or in different contexts) remain largely unexplored.
One strategy that is shown to promote stimulus generalization is Verb Network Strengthening treatment (VNeST) with evidence indicating improved single-word naming and sentence production in untrained contexts (Edmonds et al., 2015). The “verb network” in VNeST relates to the semantic relationships between verbs and their thematic roles, which manifest as priming or facilitation effects. VNeST requires (1) generating agent (doer of action or thematic roles e.g., carpenter, chef) and patient (receiver of action e.g., lumber, sugar) pairs related to a given transitive verb (e.g., measure), (2) reading out loud the different subject-verb-object scenarios (to strengthen semantic-phonological connections and practice producing subjects, verbs and objects as a cohesive utterance, and (3) building up on these scenarios using where, when and why questions (Edmonds et al., 2009). Generalization is thought to occur because retrieval of nouns that represent plausible agent-patient thematic roles of target verbs promotes activation across different sets of semantic concepts, resulting in increased retrieval of not only trained items but also untrained items (Edmonds, 2016). Generalization to related verbs that share features with target verbs can also occur. Thus, large-scale engagement of semantic networks during therapy is proposed to increase lexical retrieval in different sentence and discourse contexts (Edmonds et al., 2009). In an initial Phase 1 study of VNeST in 4 PWA, generalization was demonstrated as improved retrieval of single nouns, trained and semantically related untrained verbs, and improved agent-verb-patient retrieval in sentences. Across a variety of tasks and stimuli not directly related to the treatment itself, generalization was observed on single verb and noun naming and retrieval in sentence production. Importantly, generalization to retrieval of nouns and verbs in constrained connected speech was found in 3 out of 4 PWA, which reflects stimulus generalization (Edmonds et al., 2009). Recent evidence, albeit also small, from 11 PWA who received 35 hours of VNeST over 10 weeks, indicated generalization to untrained sentence and discourse contexts. The underlying mechanism is thought to be increased integration of lexical retrieval during sentence construction, and generalization to complex or simpler sentences was shown to depend on individual patients’ pretreatment sentence construction abilities (Edmonds et al., 2015). The efficacy of VNeST was also demonstrated in a recent review with 19 PWA revealing increased naming of nouns and verbs and retrieval in sentence and discourse contexts on untrained items and tasks in more than half of the participants (Edmonds, 2016). Thus, VNeST that takes advantage of the “centrality of verbs to semantics and syntax within a sentence” (Edmonds, 2016) seems to promote cross-level generalization, but larger-scale clinical trials are needed to further confirm this evidence in heterogenous populations (Edmonds, 2016).
Another protocol that incorporates treatment of verbs with lexical verb retrieval as well as training of verbal morphology is the ACTION protocol (de Aguiar, Bastiaanse, et al., 2015), originally developed for Dutch (Bastiaanse et al., 1997). This treatment approach addresses grammatical properties of verbs (infinitive, finite) and is focused on verb retrieval in the sentence context, the ingredients that are hypothesized to be important for achieving generalization to untreated verbs. Links et al. (2010; N = 11 PWA) reported improvement on untrained infinitives and finite verbs, indicating response generalization. Stimulus generalization to verbal communication reflected in measures of spontaneous speech such as mean length of utterances, proportion of finite verbs and verb diversity was also observed (Links et al., 2010).
Final linguistic strategy that we will discuss focuses on integrated or multilevel treatments targeting more than one linguistic level during therapy, with a goal to maximize cross-linguistic generalization to discourse in everyday communication. A recent systematic review (Dipper et al., 2021) extensively covers the topic on discourse treatments, including multilevel treatments, of which we discuss two approaches. One approach, referred to as integrated discourse treatment for aphasia (Milman, 2016) or IDTA, applies principles of problem-based learning. The core elements of IDTA consist of solving a real-world problem, active role of participants in treatment planning and therapy materials, and training of specific linguistic components and their usage in connected speech. Evidence from case series analysis indicates that this approach can lead to generalization to related linguistic structures, to standardized measures of language and cognition, and measures of discourse production. But the evidence can be considered preliminary because of small sample sizes tested, and relatively limited changes observed on functional communication measures (observed in 2 out 5 mildly impaired individuals). Additionally, considerable variability in linguistic outcomes has been noted across individuals, reducing the overall effect sizes.
A second multilevel approach, referred to as Novel Approach to Real-life communication: Narrative Intervention in Aphasia or NARNIA (Whitworth et al., 2015), was developed under a metalinguistic premise of teaching and practicing sentence structures at the word and sentence levels as well as the macrostructure of discourse (Whitworth et al., 2015). In a pilot randomized controlled trial and between-group design, effects of NARNIA (N = 8) were compared against usual care (N = 6). Usual care consisted of routine clinical therapies planned around goal setting and aimed to improve word and sentence production, reading or writing, functional activities, and discourse. Discourse training during usual care did not follow any organization principles as NARNIA. Pertinent to within- and cross-level generalization, significant group differences favoring NARNIA were reported but only on a single measure of discourse production (orientation). Evaluating changes within groups, significant gains were reported in the NARNIA group related to the amount of output, verb usage, production of argument structures, and discourse macrostructure with medium to large effect sizes. Importantly, within-level generalization at the discourse level was found in the NARNIA group with changes noted in untreated discourse genres. No such changes were found in the usual care group. Significant gains in untreated nouns and verbs were found in the NARNIA group with large effect sizes. Generalization to untreated constrained sentences was not found in either group. Thus, multilevel training that explicitly targets discourse can lead to increased improvements at the discourse level.
While the evidence from pilot exploratory studies involving multilevel treatments is promising, larger clinical trials will need to substantiate the group-level evidence to assess the effectiveness of this approach. In addition to effectiveness, a better understanding of the interaction between levels of treatment and identification of patients most responsive to treatment will also benefit planning of future applications of this approach.
4.3Cognitive-linguistic approaches
Prior studies have confirmed a strong relationship between non-linguistic cognitive abilities on aphasia recovery (Murray, 2012; Seniów et al., 2009) and linguistic treatment success (Breitenstein et al., 2009; Dignam et al., 2017; Gilmore et al., 2019; Ralph et al., 2010; Schumacher et al., 2022; Simic et al., 2020). Impaired executive functions such as cognitive flexibility, goal-directed planning, working memory (WM) (Martin et al., 2012) and attention are reported in PWA. It has been suggested that cognitive impairments can influence verbal learning (Vallila-Rohter & Kiran, 2013) and can reduce generalization from linguistic therapies to everyday communication. Communication in real-life relies not only on intact linguistic abilities but also on nonlinguistic and cognitive skills such as attention, sequencing, flexible task shifting, and self-monitoring (Fridriksson et al., 2006; Purdy, 2002). Consequently, attention and executive skills can greatly influence functional communication abilities in aphasia (Frankel et al., 2007; Helm-Estabrooks, 2002; Purdy, 2002). Based on this evidence, one strategy to improve everyday communication abilities or generalization of linguistic treatments to everyday communication is using combination treatments that target linguistic as well as non-linguistic attention and executive functions (Ramsberger, 2005).
A recent case-series (N = 10) compared a conventional language therapy approach with a Cognitive Flexibility in Aphasia Therapy (CFAT) approach in a within-subject, cross-over design (Spitzer et al., 2021). Both therapies involved impairment-based and communicative exercises. The key difference was inclusion of cognitive flexibility training during these exercises as part of CFAT. CFAT integrated characteristics of cognitive flexibility including response to change of topic and misunderstandings and use of nonverbal communication, which were not trained during conventional therapy. Improved performance (mainly driven by 3/10 patients) after CFAT was reported on language measures and measures of verbal cognitive flexibility and semantic fluency tests. Generalization was also noted to different therapy settings after CFAT only while generalization to untrained topics was reported after both therapy methods and no additional gains were found with CFAT. Nonverbal cognitive flexibility was not improved after CFAT. Overall consistent with their hypothesis, verbal cognitive flexibility improved only after CFAT but not after conventional therapy. The authors suggested that individuals with impaired verbal cognitive flexibility may greatly benefit from this specific cognitive and linguistic training than linguistic approaches alone.
Another approach that a recent study tested is language-specific attention treatment (L-SAT) against domain-general treatment involving direct attention training (DAT) in 4 PWA. The hypothesis was that improving attention for language with a targeted treatment approach would be more beneficial than domain-general attentional approaches (Peach et al., 2019a). L-SAT consisted of language tasks and stimuli that impose increasing attentional demands on lexical and sentence processing (Peach et al., 2019b). DAT involved computer exercises from Attention Process Training-3 (Sohlberg & Mateer, 2010) for auditory and visual sustained attention, selective attention, suppression and alternating attention, and auditory WM. Mixed findings were reported with improvements noted after both programs. According to the authors’ evaluation, overall effects were larger after L-SAT on standardized WAB-AQ assessments, functional language, and patient-reported outcomes. However, there was significant variation across individuals and reported improvements after DAT. More research is needed to establish a strong claim for additional gains from an approach like L-SAT compared to domain-general attentional training.
Next approaches primarily target auditory-verbal WM and/or short-term memory (STM) during therapy. Recently a case study on WM training provided with phonological component analysis (PCA) resulted in larger gains in naming accuracy and appeared to generalize to communication measures in 1 PWA compared to matched control patient who did not receive WM training (Simic et al., 2022). Treatments that rely heavily on WM/STM but without explicit linguistic therapy have also been practiced with some success (Nikravesh et al., 2021; Zakariás, Salis, et al., 2018). The hypothesis is that STM/WM treatments will improve (or effects will generalize to) language functions that critically depend on STM/WM functions (Zakariás et al., 2019). Language processing in general, and aphasia as a disorder of language processing, relies strongly on cognitive abilities such as executive functions and verbal STM that enable activation of linguistic representations and maintenance of these representations for efficient language production and comprehension (Kalinyak-Fliszar et al., 2011). Treatments that directly target these abilities would impact how representations are retrieved and maintained as they are processed at a single unit level or as part of phrases and sentences over brief intervals of time. Thus, treatment effects could result in generalization beyond the context and conditions of treatment (Kalinyak-Fliszar et al., 2011). Thus far, evidence has been mixed and presents large variability across individuals.
WM training using N-back tasks with pictures or words/letters is shown to influence sentence comprehension (Salis et al., 2017; Zakariás, Keresztes, et al., 2018; Zakariás, Salis, et al., 2018), and the training impacts functional communication abilities (e.g. ANELT), albeit with large variability across individuals (Zakariás, Salis, et al., 2018). Other studies involving STM training that sought to improve sentence comprehension found no to minimal transfer to sentence comprehension (Salis et al., 2017). Across different studies, the specifics of STM treatments vary, and include repetition of phrases or sentences (Eom & Sung, 2016; Francis et al., 2003), words or nonwords (Harris et al., 2014), or matching listening span tasks with no spoken output (Salis, 2012). One case study that implemented word and nonword repetition treatment, specifically targeting STM or the ability to maintain activated linguistic representations, in a patient with conduction aphasia did not find response generalization to untrained stimuli. But generalization to (or improved performance on) measures of WM and STM load and verbal STM span from Temple Assessment of Language and Short-Term Memory in Aphasia or TALSA was reported (N. Martin et al., 2018). Another case study with repetition treatment with multisyllabic words and nonwords varied the timing between hearing and repeating stimuli (or the delay) and difficulty of stimuli. Improvements on repetition were reported on treated stimuli (3-syllable word, 2-syllable nonword at 5 second filled interval). While response generalization to untreated stimuli was not a clear finding from the data, post-treatment improvements were noted on several subtests of TALSA involving word pair repetition, synonym triplet judgements, rhyming triplet judgements and verbal STM span, overall reflecting an improved STM and WM (Kalinyak-Fliszar et al., 2011). More recently, N.Martin and colleagues (2020) tested whether repetition of word sequences with a delay could improve verbal span and in turn repetition and other language functions. For the planned therapy no feedback was provided, unlike prior studies which included hierarchical cueing (Kalinyak-Fliszar et al., 2011), and redundancy in treatment stimuli was removed. By eliminating repeated exposure to stimuli (unique items used during treatment), the authors rationalized that they could parse out the effects on short-term maintenance over and above simple practice effects. In a case series analysis, they found that 4 out of 8 PWA exhibited improvements on STM and other language functions, albeit with large variability across different outcome measures and with effect sizes ranging from small to large (Martin et al., 2021).
A recent review that critically evaluated the methodological quality of stroke aphasia studies implementing WM/STM treatments found several limitations including lack of experimental control across studies, lack of mechanistic understanding of STM/WM treatment effects and their relationship with language functions (Zakariás et al., 2019). Additionally, lack of standardized test batteries of WM/STM applicable to PWA with moderate to severe language impairments was also recognized as a limitation (Majerus, 2018). But a recent development of TALSA (N. Martin et al., 2018), a theoretically motivated comprehensive assessment of verbal STM and related aspects in functional communication, has filled this gap. Overall, while a very promising avenue for aphasia treatment, more research is needed to develop and test the efficacy of cognitive and cognitive-linguistic treatment approaches in stroke aphasia.
4.4Current evidence of tDCS effects on genera-lization and functional communication
In this section, we focus our discussion on studies that implemented one of the treatment strategies, or strategies that are similar to what we discussed in the earlier sections (Aphasia Treatment Strategies to Strengthen Generalization and Cognitive-Linguistic Approaches), paired with tDCS to facilitate and increase generalization (summarized in Table 4).
Table 4
Current evidence of conventional tDCS on generalization and functional communication
Treatment with tDCS | Broad impairment targets | Study | N | Behavioral treatment | tDCS brain targets | Other tDCS parameters | Outcomes | Findings |
VNeST | Word retrieval and discourse | Matar et al. (2018) | 6 | Over six weeks, VNeST was delivered for 45 minutes, once weekly, in both groups. | Left IFG (FC5 based on 10-10 EEG system). | Anodal tDCS at 2 mA intensity for 20 minutes in the active and sham groups | Language quantity and syntactic complexity from three discourse samples (picture description of a scene, description of a procedure such as making a cup of tea, Cinderella narrative) using Quantitative Production Analysis and Predicate Argument Structure procedures, respectively | Both active and sham groups showed improvements on lexical richness or diversity in verb production. Active tDCS group improved more on verb retrieval, total number of words and utterances, and Communicative Effectiveness Index. |
ACTION | Verb retrieval and verbal morphology | de Aguiar et al. (2015) | 9 | Sentence completions with infinitive and finite verbs of all tenses; Ten daily (over 2 weeks) treatment sessions lasted 1-hour per day | left perilesional frontal or temporal areas | Multiple-baseline, sham-controlled, double-blinded, crossover design, real tDCS at 1 mA or sham tDCS | Accuracy of treated and untreated infinitive and finite verbs | Improved retrieval for treated and untreated verbs and for both types of verbs. Improvements smaller for untreated verbs. Greater improvements after real than sham tDCS for both treated and untreated verbs. |
Ecologically-focused therapy | Sentence production, naming, and discourse | Galletta and Vogel-Eyny (2015) | 1 | Three treatment components, including Sentence Production Program for Aphasia, sentence-embedded production training, and focused discourse (on current events from newspaper articles) using supportive conversational strategies. | Left IFG (F7) | Active tDCS at 1 mA for 20 minutes; sham for 30 seconds. TDCS was delivered during the first 20 minutes of an hour long therapy session. | Sentence production, naming in sentence context and focused-discourse tasks | Increased verb retrieval for untrained verbs in a sentence context was reported after left IFG tDCS compared to sham-tDCS. |
Conversational Therapy | Discourse or conversational, and multi-level | Marangolo et al. (2013) | 12 | Natural conversation between patients and therapist using a series of videoclips reproducing common everyday situations (e.g., eating at a restaurant). Any form of communication, including gestures, drawings, orthographic or phonological cues, was allowed. 2 hours per day over 10 daily sessions | left IFG (Broca’s region; F5) or left posterior STG (Wernicke’s region; CP5) | Conventional anodal- (1 mA) or sham-tDCS for 20 minutes. Inter-condition interval was 14 days. | Correct content units, verbs and sentence production, | Significantly larger increases in correct content units, verbs and sentences produced were found on trained and untrained videoclips after active left IFG stimulation, compared to sham and left STG stimulation. |
Cognitive training | – | Pisano et al. (2022) | 20 | Cognitive training (ten 1-hour sessions over 2 weeks) involved computerized interactive exercises of alertness, visuo-spatial working memory, selective attention and planning | right DLPFC (F4); return electrode contralateral Fp1 | 2 mA, active for 20 minutes | Cognitive, linguistic and functional communication measures | Greater improvements with anodal tDCS across all cognitive domains except alerting, which persisted a month after training ended. Greater improvements reported on Communication Activities of Daily Living Scale, and linguistic measures such as noun and verb naming, and auditory and written language comprehension after anodal compared to sham-tDCS |
Word finding therapy | Word retrieval | Spielmann et al. (2016) | 58 subacute (< 3 m) | Word finding therapy 5 daily sessions of 45 minutes | Left IFG | 1 mA anodal tDCS for 20 minutes in experimental group vs. sham group | Boston naming test, naming performance on trained and untrained items, and verbal communication | No superior effect of anodal tDCS on naming, verbal communication, quality of life and participation. |
Speech and language therapy | – | Guillouët et al. 2020 | 10 (4 chronic; 6 subacute < 6 m) | Routine speech and language therapy 3 weeks; 2 to 5 days per week | Left IFG with anodal and right IFG with cathodal-tDCS; | Crossover design 2 mA active compared to sham tDCS; 1 week “washout” between conditions | Number of different nouns used in 2 minutes to answer the question: “what is your job”, and other flow and quality measures | No effects of active tDCS compared to sham-tDCS on number of nouns produced during job description, or verbs, adjectives, adverbs, pronouns or number of repetitions, utterance with and without novel ideas, grammatical errors, and paraphasias |
Computerized naming treatment | Word retrieval | Meinzer et al. (2016) | 26 | Intensive naming therapy over 2 weeks (twice daily; 4 days per week; 1.5 hours per day). | Left motor (M1 hand) region | 1 mA anodal tDCS group vs. sham tDCS group | Naming for trained and untrained items and generalization to everyday communication (CETI, PCQ ratings); Tested immediately after and at 6 months after the treatment | Trend toward larger gains on trained items after anodal compared to sham group. Treatment effects for trained items were better maintained in anodal group after 6 months. Generalization to untrained items and to functional communication larger for anodal group at both time points. |
Abbreviations: VNeST = Verb Network Strengthening Treatment; m = months; tDCS = transcranial direct current stimulation; IFG = inferior frontal gyrus; DLPFC = dorsolateral prefrontal cortex; STG. = superior temporal gyrus; CETI = Communicative Effectiveness Index; PCQ = Partner Communication Questionnaire.
A recent study tested the feasibility of anodal tDCS in combination with VNeST to improve discourse production in 6 PWA (Matar et al., 2022). A double-blinded, randomized design with an active tDCS and a control or sham tDCS group was implemented. Over six weeks, VNeST was delivered for 45 minutes, once weekly, in both groups. Conventional tDCS with anode placed over left IFG (FC5 based on 10-10 EEG system) was applied at 2 mA intensity for 20 minutes in the active group, whereas stimulation was turned off after 30 seconds in the sham group. Immediate and follow-up assessments at 6 months were conducted. Outcome measures included rates of screening (86%), eligibility (53%), consent (60%), retention (100%), completion (of treatment and follow-up; 100%) for feasibility. No adverse events were reported. Other measures included language quantity and syntactic complexity from three discourse samples (picture description of a scene, description of a procedure such as making a cup of tea, Cinderella narrative) using the Quantitative Production Analysis and Predicate Argument Structure (PAS) procedures, respectively. As expected from VNeST, both active and sham groups showed improvements on the measure of lexical richness or diversity in verb production (verb type token ratio or VTTR) at the discourse level. Large effect sizes were selectively reported in the active tDCS group for verb retrieval (verb type token and verb type total) across different discourse genres, reflecting increased stimulus generalization. Total number of words and utterances also increased in the active compared to sham groups. But no differences between groups were found in syntactic complexity, as measured with PAS. Out of several measures of functional communication, quality of life and psychological symptoms, significant improvements on Communicative Effectiveness Index (CETI), a validated measure that covers a wide range of everyday communication scenarios, were reported in the active compared to the sham group. Changes in cognitive skills measured using Montreal Cognitive Assessment (MoCA) were not significant. Overall, these results, while exploratory, are promising and suggest feasibility and early efficacy of anodal tDCS of left IFG as a potential tool to further enhance generalization of VNeST in stroke aphasia.
de Aguiar et al. (2015) paired tDCS with an Italian version of ACTION, which included sentence completions with infinitive and finite verbs of all tenses. In a multiple-baseline, sham-controlled, double-blinded, crossover design, real tDCS at 1 mA or sham tDCS was paired with ACTION and was delivered over left perilesional areas in 9 patients. To ensure that tDCS was delivered to spared tissue, stimulation targets were determined individually based on visual inspection of patients’ MRI scans and the extent of lesions covering the inferior frontal and parietal regions. The targets were different across patients with placement of anode over BA 44-45 (inferior frontal Broca’s area) in 3 patients, over BA 45-46 (superior to Broca’s area) in two patients, over BA 9-10 (superior and middle frontal gyri) in 3 patients with large anterior lesions, and over posterior middle and superior temporal gyri in 1 patient. Cathode was placed over the homologous region in the right hemisphere, except for 3 patients with anterior lesions, where cathode was placed over the right homolog of Broca’s area. Ten daily (over 2 weeks) treatment sessions lasted 1-hour per day. Important findings were that ACTION improved retrieval for both treated and untreated verbs and for both types of verbs (infinitive and finite) albeit improvement of untreated verbs was smaller than treated verbs. Greater improvements were observed after real than sham tDCS for both treated and untreated verbs, but the authors cautioned against conclusively interpreting these results in favor of tDCS. Because of differences in scores before and after real and sham-tDCS cycles, it was difficult to rule out the effects of order and/or ceiling effects during the second cycle of treatment. Replication of findings related to tDCS with ACTION in a larger sample is warranted (de Aguiar, Paolazzi, et al., 2015).
To our knowledge, there are no published studies that have applied tDCS for improved language learning and facilitating generalization based on CATE or PMT. We note this as a potentially promising yet uncharted future direction that could boost response generalization of these strategies. Additionally, the phonological treatment including sublexical letter-to-sound mapping and phonological manipulation has not been paired with NIBS in PWA. In an individual with primary progressive aphasia, this treatment combined with anodal tDCS to the left IFG demonstrated significant generalization to written language production, including written narratives (Nickels et al., 2023).
No published studies have paired tDCS with therapies closely following the principles of IDTA or NARNIA. But a few studies have implemented discourse or conversational therapies and other therapies with multi-level approaches paired with tDCS that we discuss in detail here. Marangolo et al. (2013) tested a Conversational Therapy approach with conventional anodal- (1 mA) or sham-tDCS delivered either over the left IFG (or Broca’s region; F5 from 10–20 EEG system; 5×7 cm2 sponge electrodes) or left posterior STG (or Wernicke’s region; CP5) (Marangolo et al., 2013). A within-subject, randomized, double-blinded design was implemented in 12 PWA. During sham-tDCS sessions, stimulation was turned off after 30 seconds. Active tDCS lasted 20 minutes. The therapy started at the beginning of tDCS and lasted a total of 2 hours per day over 10 daily sessions per tDCS condition. The inter-condition interval was 14 days. The Conversational Therapy consisted of natural conversation about salient information between patients and therapist using a series of videoclips reproducing common everyday situations (e.g., eating at the restaurant). Any form of communication, including gestures, drawings, orthographic or phonological cues, was allowed. This portion of the therapy deviates from what was previously described under IDTA or NARNIA, which consisted of a more structured hierarchical cuing approach and explicit training of discourse macrostructures. Nevertheless, significantly larger increases in correct content units, verbs and sentences produced were found on trained samples after left IFG stimulation at the end of 10 days of treatment, compared to sham- and left pSTG stimulation. Additionally, on untrained videoclips, significant generalization was reported on the same measures also after left IFG stimulation. No changes were found on any other standardized assessments of aphasia. The authors discussed that the use of informative speech as part of therapy together with a stimulation of a region involved in controlled lexical selection could have facilitated the flow of information during discourse, produced more informative chunks or content units, and resulted in improved verb production and production of grammatically correct sentences. In a subsequent study, anodal-tDCS over left IFG during the Conversational Therapy also significantly improved performance on picture description, and noun and verb naming tasks (Campana et al., 2015).
Galletta and Vogel-Eyny (2015) implemented an ecologically focused language therapy in a single case-study using training components at multiple levels involving sentence production, naming in sentence context and focused-discourse tasks paired with tDCS over left IFG (F7; 1 mA active for 20 minutes; sham for 30 seconds). They hypothesized that explicit treatment of nouns and verbs in a sentence context with left IFG stimulation would result in increased generalization to untrained sets of sentence-embedded nouns and verbs. TDCS was delivered during the first 20 minutes of an hour-long therapy session with 3 treatment components: Sentence Production Program for Aphasia (Helm-Estabrooks & Nicholas, 2000), sentence-embedded production training (Raymer & Kohen, 2006), and focused discourse (on current events from newspaper articles) using supportive conversational strategies. Increased verb retrieval for untrained verbs in a sentence context was reported after left IFG tDCS compared to sham-tDCS (Galletta & Vogel-Eyny, 2015).
For cognitive-linguistic approaches, we found one study by Pisano et al. (2022) that implemented cognitive training with tDCS in 20 PWA and reported outcomes related to generalization to linguistic measures and functional communication. Cognitive training (ten 1-hour sessions over 2 weeks) involved computerized interactive exercises of alertness, visuo-spatial working memory, selective attention, and planning paired with tDCS over the right DLPFC (F4; 2 mA, active for 20 minutes; return electrode contralateral Fp1). Compared to sham, greater improvements were found with anodal tDCS across all these cognitive domains except alerting, which persisted a month after the training ended. Significant generalization was noted with greater improvements reported on the Communication Activities of Daily Living Scale, and other linguistic measures such as noun and verb naming, and auditory and written language comprehension after anodal compared to sham-tDCS (Pisano et al., 2022).
A recent meta-analysis of tDCS randomized controlled trials found that while tDCS targeting IFG (left or bihemispheric IFG) improved specific linguistic outcome of naming nouns, its effect on functional communication was not superior to sham-tDCS. Sub-analysis related to communication included 112 PWA from studies that assessed communication abilities as secondary outcome measures. The impact of tDCS on communication was assessed using several different measures across these studies, involving spontaneous speech in response to open-ended questions (e.g., describe your job), everyday life settings using Amsterdam Nijmegen Everyday Language Test (ANELT) or using Aphasia Severity Rating Scale (ASRS), quality of everyday communication using CETI (Lomas et al., 1989) and Partner Communication Questionnaire (PCQ) (Blomert, 1993), and quality of life measures such as EuroQol-5D (Brooks, 1996), Stroke and Aphasia Quality of Life questionnaire (SAQOL) (Hilari et al., 2003), social participation using Community Integration Questionnaire (Dalemans et al., 2010), and cost-effectiveness using Cost Analysis Questionnaire (iMTA, n.d.).
One of the studies included subacute PWA (1 mA; anodal tDCS; left IFG; daily over 2-weeks) and did not find a superior effect of tDCS on verbal communication, quality of life and participation. The authors attributed the negative results to ongoing spontaneous recovery, which may preclude the effectiveness of left hemispheric tDCS and that targeting right hemispheric regions during this phase may be a more suitable approach (Spielmann et al., 2016). Another study in chronic PWA that targeted left IFG with anodal and right IFG with cathodal-tDCS during routine speech and language therapy (2 mA; bihemispheric IFG; 3 weeks; 2–5days/week) also did not find significant effects of tDCS compared to sham-tDCS. Outcomes used in this study to test the effects of tDCS were the number of nouns produced during job description, and other flow and quality measures such as verbs, adjectives, adverbs, pronouns or number of repetitions, utterance with and without novel ideas, grammatical errors, and paraphasias (Guillouët et al., 2020). In these studies, either the phase of stroke recovery (subacute) (Spielmann et al., 2018), or minimal constraints on therapy duration, frequency and type during routine clinical practice may have weakened the effects of tDCS.
Another study in chronic PWA that targeted left motor regions (1 mA; anodal tDCS; left M1 hand; 2-weeks; 4days/week) during a computerized naming treatment in a more controlled research setting did find significantly enhanced generalization of anodal tDCS to untrained items and to CETI and PCQ everyday communication ratings, which were maintained at 6-months after treatment termination (Meinzer et al., 2016).
Overall, the evidence related to tDCS on generalization/communication outcomes is mixed and appears to be influenced by several factors including the details of aphasia treatment protocol, stroke-related factors (e.g., chronicity) and tDCS parameters. Additionally, it is unclear how tDCS affects generalization. With variability across treatment protocols and outcomes, it is difficult to comment on tDCS parameters that are likely to facilitate generalization (e.g., individualized stimulation targets for a given therapy, or higher intensity or longer duration of stimulation or treatment).
It has been shown that factors governing effects of aphasia treatments on trained (acquisition) and untrained (generalization) items may be different. The brain regions associated with acquisition may be distinct from those associated with generalization (Meinzer et al., 2010). Additionally, the timing of treatment response between acquisition and generalization is also shown to be distinct; improvements for generalization stimuli emerge later than acquisition stimuli (Dickey et al., 2014). Interesting questions that remain unanswered are whether the patterns of treatment response for generalization with and without tDCS are qualitatively different. For example, as shown by Dickey, Hula and Yoo (2014), generalization responses with TUF are shifted in time compared to acquisition. They concluded that more sessions of TUF may be needed in some individuals to increase the likelihood of generalization. Can tDCS reduce the gap between acquisition and generalization in terms of timing, or in other words can tDCS induce generalization in fewer sessions? These questions remain largely unexplored.
Another important factor related to generalization is the timing of tDCS. It is not clear whether the timing of tDCS delivery in relation to aphasia treatments differentially affects language outcomes. The effectiveness of tDCS is potentially as “good” as the therapy that it is paired with. The pairing of tDCS can be done in multiple ways: concurrent to language therapy (online) or sequential to language therapy (offline). Both offline and online protocols would modulate the state of neuronal activation at the time of stimulation. Offline tDCS could either precondition neural activity or maintain or increase activation post-therapy. The question of timing has been raised recently in stroke-related motor recovery, and small sample trials provide proof of concept that while both concurrent and sequential tDCS with motor therapy result in improvements, sequential tDCS seems to be more effective for rehabilitating motor daily function and hand movement control (Liao et al., 2020). While we do not have direct evidence for post-stroke language recovery, an ongoing clinical trial aims to address this important question and promises to provide effect sizes related to three tDCS timing conditions: tDCS during language therapy, tDCS before therapy and tDCS after therapy in comparison with sham tDCS in a randomized, between-group, double-blinded study. The authors theorize that tDCS provided before therapy could “prime the language system” and tDCS after therapy could help in memory consolidation (Ashaie et al., 2022). Their main goal is to compare the efficacy between online and offline tDCS protocols. Relatedly, given the hypothesized mechanisms of offline tDCS on memory consolidation, it would be interesting to study differential effects of tDCS timing on treatment generalization and whether offline tDCS increases generalization.
4.5Real-time fMRI neurofeedback
Unlike tDCS, real-time fMRI NFB is an intrinsic neuromodulation method used to train individuals to increase or decrease activity in a specific brain region or network. It is a unique and innovative approach allowing individuals to regulate their own brain activity (Dewiputri & Auer, 2013; Renton et al., 2017; Thibault et al., 2018; T. Wang et al., 2018). FMRI NFB paradigm involves measurements of activity levels of a particular brain area relative to an appropriate baseline. This information is then given to the participant, who can employ mental strategies to modulate the signal. The choice and refinement of these mental regulation strategies are guided by neurofeedback given to the participant. Such repeated efforts to self-regulate brain activity are shown to result in learning-induced changes in cognitive as well as motor ability (Sitaram et al., 2017). Studies also show that individuals can learn to increase or decrease functional connectivity among targeted brain networks (Kim et al., 2015; Koush et al., 2013, 2015; Krylova et al., 2021; Megumi et al., 2015). Thus, fMRI neurofeedback can help individuals to learn to voluntarily modulate their brain activity, leading to changes in neural function, with the potential to improve symptoms of neurological or psychiatric disorders.
4.6Current evidence for efficacy
The number of published fMRI NFB studies has exponentially increased over recent years, with most studies in healthy individuals demonstrating efficacy (Watanabe et al., 2017). However, a review of 99 published fMRI NFB studies by Thibault and colleagues (2018) cautioned against over interpreting the positive evidence due to methodological variability (Thibault et al., 2018). While most of the studies included a control group and showed that participants were able to modulate their brain activity according to at least one statistical test, some did not compare the experimental and control groups directly. Moreover, although the primary goal of fMRI NFB interventions is to change behavior, the evidence for behavioral changes across the examined studies was modest. For example, out of the 59 studies that examined behavioral change from before to after the intervention, 69% showed an improvement, and of the studies that included a control group, 59% showed a greater behavioral improvement in the experimental than the control group. Thus, although it appears that individuals can regulate their brain activity using fMRI NFB, further research is needed to replicate clear behavioral improvements suggested by some studies, but not others.
Apart from its effectiveness in healthy volunteers, fMRI NFB has demonstrated potential for neurorehabilitation in various clinical applications, including stroke (Renton et al., 2017) and Parkinson’s Disease (Ruiz et al., 2014), to enhance motor function (Liew et al., 2016; Sitaram et al., 2012), language (Sreedharan et al., 2019, 2020), and other cognitive abilities (Krylova et al., 2021). It also shows promise as a treatment for psychiatric disorders (Taschereau-Dumouchel et al., 2018), including anxiety (Pindi et al., 2022), depression (Taylor et al., 2022; Young et al., 2017), and addiction (Subramanian et al., 2021). In cases of chronic pain, fMRI NFB has been utilized to help manage pain by modulating activity in pain-associated brain regions (Roy et al., 2020).
Additionally, fMRI NFB has proven valuable in studying neuroplasticity and the mechanisms underlying learning and memory (deBettencourt et al., 2019). By examining the role of specific brain regions and networks in various cognitive processes, fMRI NFB has contributed to a deeper understanding of the brain and its functions. For example, deBettencourt and colleagues (2015) used fMRI NFB to identify neural mechanisms that support sustained attention training (deBettencourt et al., 2015). In the study, participants performed a visual detection task on composite images containing a mixture of faces and scenes and were asked to respond when the composite contained a specific category of images (indoor scenes, female face, 90% of trials) or withhold a response when it did not (10% of trials). At the start of each block, participants were cued to attend to either faces or scenes. fMRI NFB was implemented using a whole brain multivariate classifier which detected participants’ current brain state and compared it to the pre-trained state for each category (faces vs. scenes). When the current state corresponded with the cued category, the composite image became easier to process as the proportion of task relevant image was increased. Such fMRI NFB training improved behavioral sensitivity in the NFB group but not in the control group who received NFB from other participants’ brains. Importantly, at the end of training, the NFB group showed altered brain dynamics, such that the ventral temporal cortex and the basal ganglia represented the two attentional states (attend to faces vs scenes) more distinctly. Furthermore, the degree of improved behavioral sensitivity depended critically on whether the fMRI NFB carried information from the frontoparietal attention network. The authors suggested that increased neural separation in the frontal cortex enabled stronger top-down modulation of category specific responses in the ventral temporal cortex and stronger irrelevant response inhibition in the basal ganglia. Thus, using fMRI NFB allowed to reveal the brain mechanisms supporting neuroplasticity of attention-related behavior. Compared to widely used EEG-based neurofeedback methods, fMRI NFB offers several advantages, including superior spatial resolution and signal specificity (Renton et al., 2017). It is also flexible with respect to the neurofeedback targets (single brain regions, and/or networks) and the nature of the neurofeedback signal (percent signal change, functional or effective connectivity, machine learning classifier weights) making it useful for a variety of clinical and research applications. One downside is the high cost and complexity of fMRI NFB procedures that currently precludes wide use in clinical practice, but this could change if future studies can demonstrate clear and unequivocal benefits to patients’ quality of life.
4.7Current evidence of efficacy in stroke and aphasia
In stroke rehabilitation, fMRI NFB shows considerable promise as a viable method for addressing dysfunction in structurally intact regions that may be directly or indirectly connected to the site of the infarction. In the context of aphasia rehabilitation, there are several advantages of treatments employing fMRI NFB compared to conventional rehabilitation methods or routine SLT. First, fMRI NFB affords personalized training that can target brain regions and networks associated with language processing in an individual, which is particularly beneficial for PWA who present with diverse and heterogeneous impairment profiles and lesion patterns. Second, fMRI NFB allows for real-time monitoring of brain activity and feedback, which can increase patient engagement and help them stay motivated to continue the treatment. Third, fMRI NFB can increase neuroplasticity by reinforcing the regional and language network activation, resulting in more effective language processing over time. Finally, fMRI NFB is non-invasive and safe as it does not involve exposure to ionizing radiation or use of pharmacological agents, making it an attractive adjunctive treatment option for PWA.
Results from several small-scale studies (N = 2–12) in stroke patients that employed real-time fMRI NFB (Liew et al., 2016; Robineau et al., 2019; Sitaram et al., 2012; Sreedharan et al., 2019), or combined fMRI and EEG NFB (Bezmaternykh et al., 2021; Giulia et al., 2021) indicate positive effects of this treatment. For example, Liew et al. (2016) found that in 3 out of 4 patients with severe hemiparesis ipsilesional connectivity between the thalamus and primary motor cortex was improved following two 2-hour sessions of connectivity-based NFB with small to medium effect size (ES) (Liew et al., 2016). Sitaram et al. (2012) reported increased activity in the ventral premotor area with large ES after 3 sessions of NFB training in 2 chronic stroke patients and 4 healthy controls. Visuomotor accuracy was improved in 4 participants (1 stroke participant and 3 healthy controls) (Sreedharan et al., 2019). Robineau et al. reported upregulated visual cortex activity in 6 stroke patients with spatial neglect after three 15-minute sessions of NFB (large ES) and reduced spatial neglect severity (Robineau et al., 2019).
Fig. 2
Parameter and design characteristics in fMRI NFB studies.
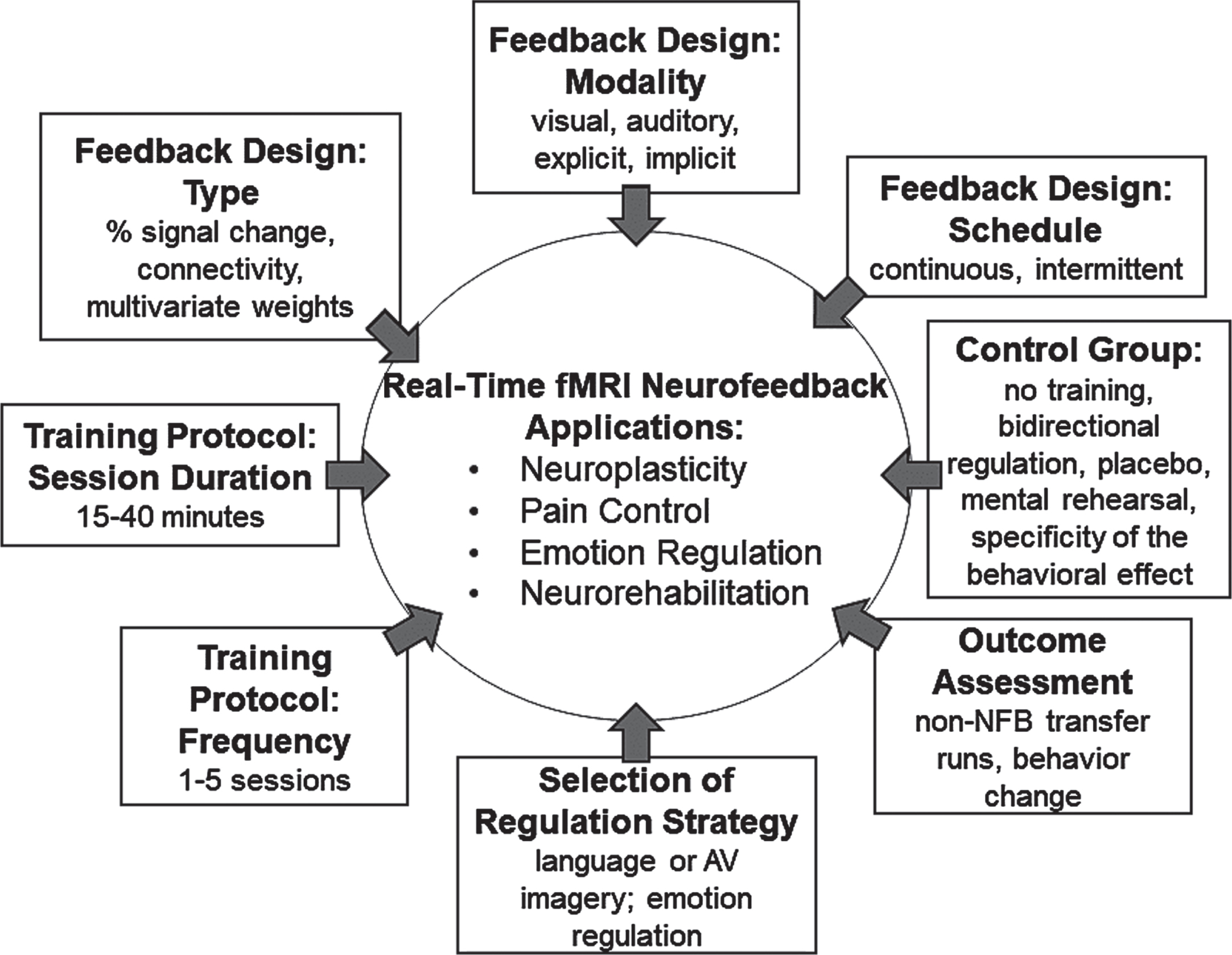
So far, two studies have evaluated the use of fMRI NFB in PWA. These studies compared effects of fMRI NFB training across 3 groups: 2 groups of PWA with a diagnosis of expressive and anomic aphasia and 1 group of healthy controls. The intervention PWA group (N = 4) and the healthy control group (N = 4) underwent 4 fMRI NFB training sessions and 2 pre-post test scans, while the control PWA group (N = 4) received routine clinical treatment similar to that received by the intervention PWA group and underwent 2 test scans but did not receive fMRI NFB training. During the fMRI NFB upregulation blocks, participants were instructed to engage in covert speech, recite a poem, have a conversation, or engage in another covert language task of their choice as a regulation strategy (Sreedharan et al., 2019). They received connectivity-based feedback such that greater connectivity between the Broca’s and Wernicke’s areas defined using a functional localizer resulted in higher feedback scores. Functionally defining the regions of interest ensured that lesioned areas were not considered for fMRI NFB training. The first study reported increased connectivity between Broca’s and Wernicke’s regions in patients with expressive aphasia who underwent fMRI NFB. The magnitude of connectivity after training was similar to that in healthy controls, suggesting normalization. In the second study (Sreedharan et al., 2020), activity in these regions during regulation compared to the baseline was compared across groups. Increased activation in the Broca’s area and its right homologue area was reported in the PWA group after fMRI NFB training, but this level of activity was still lower than that in healthy controls. No significant differences in language improvement (WAB and naming) were found between the fMRI NFB and the usual-care PWA groups. Taken together, emerging literature suggests that real-time fMRI NFB can induce changes in neural activation patterns and connectivity in patients with stroke, including PWA. But associated behavioral improvements are not consistently found. Small patient samples and lack of a sham/control group in some published studies overall limit the ability to drive concrete conclusions regarding the efficacy of fMRI NFB. Large-scale, properly controlled trials are needed to confirm the effects of fMRI NFB on neural function and ensuring behavioral changes.
In the next section, we provide some details and resources to implement fMRI NFB for interested researchers.
4.8Parameters important for implementation
Implementation of fMRI NFB requires specialized equipment configuration to enable a closed-loop system, wherein the MRI scanner is connected to a computer equipped with software that enables real-time neural data preprocessing and analysis for computing the neurofeedback signal. The specifics of such a system are extensively discussed in several high-quality reviews (e.g., Koush et al., 2017). Participant selection is crucial for successful fMRI NFB training. Participants should be able to withstand the MRI environment for extended periods of time and follow instructions during the session. Other important considerations are selection of the regulation strategy, feedback design, training protocol, outcome assessment and choice of an appropriate control group, which are highlighted in Fig. 2 and discussed below.
The selection of a successful regulation strategy is essential for the success of fMRI NFB training. The strategy should be engaging, meaningful, and tailored to the participant’s individual capabilities, while also designed to elicit the desired neural activity and remain feasible within fMRI paradigms. Many studies have opted for covert regulation strategies to prevent in-scanner motion. For example, Robineau and colleagues (2019) used visual imagery and covert attention to the left visual field to upregulate right visual cortex activity in patients with spatial neglect (Robineau et al., 2019). Rota and colleagues (2009) allowed participants to choose specific strategies to upregulate right IFG activation in the context of language processing, leading to strategies such as imagining lecturing before a class of students, arguing scenes, debates, singing or reciting poems, and recalling old conversations with friends (Rota et al., 2009). As discussed previously, Sreedharan and colleagues (2019, 2020) instructed participants to covertly engage in making a speech, having a conversation, reciting a poem, or another language activity of participants’ choice to upregulate connectivity between the Broca’s and Wernicke’s areas (Sreedharan et al., 2019, 2020). Lastly, Young and colleagues (2017) instructed patients with depression to retrieve positive memories to upregulate activity in the amygdala (Young et al., 2017). Thus, personalized and engaging regulation strategies have been used to maximize the effectiveness of fMRI NFB training across diverse clinical applications.
The nature of the feedback provided to participants is equally important. Feedback should be clear and meaningful and should prevent participants from getting overwhelmed as that can be counterproductive. While the optimal approach is likely dependent on the application, Stoeckel and colleagues (2014) noted that continuous feedback has the advantage of delivering immediate feedback but that can increase cognitive load. Intermittent feedback may be more effective as it reduces cognitive load (Stoeckel et al., 2014). Visually presented feedback has been reported to be more effective than auditory feedback, with various visual presentation methods employed across studies, including a continuously updated graph, smiling avatars, fluctuating levels of a thermometer, or fire (Dewiputri & Auer, 2013). Some studies implement implicit feedback by modulating task difficulty. For example, as discussed earlier, deBettencourt and colleagues (2015) trained participants to improve sustained attention wherein the feedback signal modulated the proportion of task relevant to task-irrelevant information presented on each trial (deBettencourt et al., 2015). Feedback can also vary based on whether the goal is to modulate signal within a single region or modulate coordinated activity from multiple regions. As abnormal brain states after stroke are thought to result from uncoordinated activation of distributed brain regions, fMRI NFB applications that enhance or modulate functional connectivity (Koush et al., 2013) across distributed regions can be particularly promising in stroke rehabilitation. With growing interest in pattern recognition, fMRI NFB studies have also begun to implement decoding of brain states in spatially distributed brain regions for providing feedback. Several pattern recognition techniques have been applied, with support vector machine (SVM) being the most widely used technique (Watanabe et al., 2017). But this presents a challenge in that it is difficult to build individualized pattern classifier in real-time. This issue was recently addressed in one study by developing a participant-independent pattern classifier from a larger set of group data, which represented a specific demographic population (Ruiz et al., 2014).
While single-group design studies have been used in clinical applications of fMRI NFB, efficacy cannot be established without appropriate control conditions. Sorger and colleagues (2019) provided a detailed review and critical analysis of different control groups and their suitability for fMRI NFB applications (Sorger et al., 2019). For instance, a no-training control, such as treatment as usual (TAU), may be used to reveal whether neurofeedback has a clinically significant benefit. However, TAU is a relatively inadequate control as there is no true “consistent background” practice against which a new intervention can be tested. Among the more robust alternatives are bidirectional regulation and placebo control conditions. In bidirectional regulation control, participants are trained to regulate the same brain region or network in opposite directions. This control condition ensures physiological specificity, controls for motivational and placebo effects, and rules out non-specific effects. However, it does not exclude that mental rehearsal alone might be sufficient to change brain activity and associated behavior. Additionally, alternating up- and down-regulation in a within-group design can induce order and carry-over effects. Various types of placebo control can be used in fMRI NFB, including feedback from an alternative brain region, non-neural feedback (e.g., respiration), and sham feedback, where participants receive unrelated or non-contingent feedback that does not reflect their actual brain signal. This type of control can match the experimental condition on all aspects except gaining control over the experimental region-of-interest signal. However, participant’s efforts to regulate a non-contingent NFB signal and the resulting frustration can become critical confounds, especially if participants detect the non-contingency. The mental-rehearsal control is another control condition where participants are instructed to self-regulate brain activity using a mental strategy but in the absence of neurofeedback. This allows to control for the effects of cognitive/behavioral strategy without the support of neurofeedback. While this approach can be easily applied, it cannot rule out motivational and placebo effects.
In a special case of implicit neurofeedback, where participants are not provided any information about the trained brain region, feedback signal, or potential mental strategies, the specificity of the behavioral effect can be used as a control by training only one specific brain state and testing for changes only in that state. An example of such paradigm is a study by Wang et al. (2021) where participants were trained to control the diameter of a visually presented disk to match the multivariate pattern of V1/V2 activity observed when they were viewing Sekular motion displays (Z. Wang et al., 2021). The study showed that participants improved their detection of local motion of dots in Sekular displays, but not of global motion, which may be subserved by higher order visual areas. Overall, while each type of control condition has clear advantages and disadvantages, the choice of the control condition should be tailored to the fMRI NFB implementation.
Other important design considerations include determining the number, optimal frequency, and duration of training sessions, and the number of neurofeedback runs per session. While some studies report successful regulation of brain activity after a single session, a more typical implementation requires 2–5 separate training sessions with 1 or 2 runs, each lasting 15–20 minutes (Ruiz et al., 2014; Sitaram et al., 2017; Thibault et al., 2018; Watanabe et al., 2017). The duration of neurofeedback training may vary in PWA, depending on stroke severity and the level of engagement with the training. However, at this point, there are no dose-response studies available for fMRI NFB training for neurorehabilitation applications. Such studies would inform the relative effectiveness of fMRI NFB compared to existing therapies or compared to biofeedback using more cost-effective neuroimaging tools such as EEG.
The success of fMRI NFB training can be assessed using various outcome measures, such as improved control of brain activity during NFB training runs and during non-NFB transfer runs, as well as changes in behavioral performance on the trained task, related tasks, or real-world outcomes. There is some support showing that fMRI NFB training can lead to behavioral changes, such as improved pain perception in patients with chronic pain, improved verbal working memory in healthy participants, and improved mood in individuals with major depressive disorder. However, there is limited evidence of behavioral change that has generalized to other tasks or real-world outcomes (Stoeckel et al., 2014). It is possible that longer and more intensive training sessions may be required to achieve generalization effects.
In conclusion, successful implementation of fMRI NFB depends on various factors, including appropriate equipment configuration, participant selection, personalized and engaging regulation strategies, and effective feedback design. More studies with suitable control conditions are needed to evaluate the efficacy of fMRI NFB by addressing potential confounds such as placebo effects. Other critical aspects that remain unclear are the optimal training frequency, duration, and assessment of outcomes. As fMRI NFB continues to be explored and refined, understanding these key parameters will contribute to its adoption across diverse clinical applications. While some studies have demonstrated behavioral change because of fMRI NFB training, further research is needed to determine the potential for generalization to functional and real-world outcomes. We anticipate that the ongoing development of fMRI data preprocessing and analysis methods, alongside the investigation of dose-response relationships, will help unlock new opportunities and expand the potential of fMRI NFB in various therapeutic settings, including in PWA after stroke.
4.9Challenges specific to aphasia in fMRI NFB implementation
Implementing fMRI neurofeedback treatments in PWA presents several challenges that must be addressed for the successful use of this method as a treatment tool. PWA could have difficulty following instructions, or attending to tasks that require linguistic ability, which can affect the quality of the neurofeedback signal. Careful design of task, instructions, and stimuli as well as selection of patients for whom this treatment may be appropriate is necessary when working with patients whose communication ability is compromised. Interpreting the fMRI NFB signal and providing meaningful feedback can also be challenging in PWA, as the signal from language-related brain regions may be affected by stroke. For successful implementation, the brain regions selected for neurofeedback training should be intact and relevant to the language or cognitive process being re-trained in PWA. The primary goal of biologically-based interventions, such as tDCS or fMRI NFB is to enhance the generalizability of treatment effects to other language tasks or real-world communication situations. To achieve this, we expect that fMRI NFB would need to be combined with speech therapies or cognitive therapies as part of an intensive aphasia treatment paradigm. Finally, as mentioned previously, fMRI NFB treatments can be time-consuming and require specialized equipment and expertise, which may limit their availability and accessibility to PWA.
While there is potential for fMRI NFB to become a treatment tool for aphasia, more research is needed to establish its efficacy and feasibility. The current literature on fMRI NFB in aphasia is limited to 2-3 studies with small to medium effect sizes. Larger randomized controlled trials with well-defined protocols are needed for validating this technique as a viable treatment option for PWA. If it is proven to be highly effective, the current barriers such as cost and accessibility of fMRI technology in clinical settings would need to be addressed for its widespread use in clinical practice. Additionally, before fMRI NFB transitions into mainstream clinical practice, RCTs must demonstrate its sustained benefits post-training, necessitating the inclusion of long-term follow-ups. The novelty of fMRI NFB means that methodologies across studies can differ significantly, precluding consistent conclusions. Checklists such as the Consensus on the Reporting and Experimental Design of Clinical and Cognitive-Behavioral Neurofeedback Studies (CRED-nf) outlining evidence-based best practices for conducting NFB research are a promising step in the right direction (Ros et al., 2020). Beyond standardizing fMRI NFB methods, it is also essential to evaluate the appropriateness and acceptability of the fMRI NFB procedures for the target population and understand their life-quality impact. These considerations will shape future research directions in this field.
5Future directions
After a thorough review of NIBS literature, and in particular literature related to tDCS, we have developed a following list of questions that remain largely unanswered and could be addressed in future studies involving PWA after stroke:
(1) We still do not fully understand the different patterns of neural activity changes during aphasia recovery. With an incomplete understanding of neural reorganization patterns, the selection of NIBS parameters to induce recovery remains a challenge. This is further complicated by observed variability in lesion, aphasia severity and language impairment characteristics. We posit that use of a single stimulation protocol across PWA based on hypothetical models of recovery may be an ineffective strategy and instead advocate for individualized protocols.
(2) Individualized targeting protocols that take structural and functional characteristics into account may optimize neuromodulatory effects. But empirical evidence to support this claim does not exist. In addition, several individualized targeting approaches have been implemented but there is no consensus on best practices.
(3) Targeting tDCS to restore cerebral perfusion in intact areas of the affected hemisphere may prove to be an effective treatment strategy for post-stroke aphasia and should be further explored in future studies.
(4) Individualized FE models using participant’s own head MRI should be generated to optimize tDCS montages to achieve maximal focality or intensity at the target, and in turn increase tDCS efficacy.
(5) Some argue that such precise targeting may be excessive for conventional and HD-tDCS. Future studies should quantify and compare the treatment effect sizes with and without targeting across neurological cohorts and applications of tDCS, including in PWA. We hypothesize that precise targeting with conventional or HD-tDCS would improve tDCS efficacy, particularly in patients with brain damage.
(6) Evidence for the implementation of HD-tDCS in PWA is lacking. Future studies should evaluate the relative effect sizes of conventional vs. HD-tDCS to determine whether focal stimulation provides any benefit over diffused stimulation modality.
(7) We rationalize in this review that for tDCS to be accepted clinically as routine practice, it must promote generalization with much larger effect sizes than aphasia therapy alone and make a significant impact on daily communicative needs. The evidence related to tDCS on generalization/communication outcomes is mixed and appears to be influenced by several factors including the details of aphasia treatment protocol, stroke-related factors (e.g., chronicity) and tDCS parameters. With variability across published treatment protocols and outcomes, it is difficult to comment on tDCS parameters that are likely to facilitate generalization (e.g., stimulation targets given a therapy, or higher intensity or longer duration of stimulation or treatment). We recommend that future tDCS protocols deviate from “train, [stimulate] and hope” (Stokes & Baer, 1977) for generalization to using theoretical understanding of changes induced by tDCS following aphasia therapies to form predictions for generalization (Webster et al., 2015a).
(8) Future studies should aim to disentangle how tDCS affects generalization. A few questions of interest to advance the use of tDCS for functional recovery are:
a. Given a therapy, are the brain regions associated with generalization distinct from acquisition (trained)?
b. For a given therapy, what brain regions should be targeted to promote generalization?
c. Can tDCS induce generalization in fewer treatment sessions?
d. When should tDCS be provided in relation to the treatment (concurrent or sequential) to increase generalization?
(9) Finally, to test the effectiveness of tDCS protocols on both acquisition and generalization of language therapies, there is little debate that we need large-scale, multi-site clinical trials in PWA. Given the heterogeneity in aphasia presentation combined with tDCS’ large parameter space, we think translation of research-based tDCS protocols to clinical practice cannot be accomplished using conventional randomized, double-blinded, parallel or cross-over trials. Conventional designs would need very large sample sizes, long study durations, and may still lack statistical power to run analysis in multiple subgroups to address questions related to heterogeneous samples. Coordinated effort across multiple stroke and aphasia groups using pragmatic clinical trial designs that allow more flexibility and greater efficiency such as cluster or adaptive designs will be needed to definitively enable selection of optimal tDCS protocols in a given sample of PWA and advance its application in routine clinical settings (Ajmera et al., 2021).
After a comprehensive review of the literature using fMRI NFB for stroke rehabilitation, we have identified several key objectives that are currently unaddressed and require further investigation in future studies:
(1) Investigate the optimal frequency and duration of fMRI NFB training in PWA necessary to establish efficacy, while also considering cost-effectiveness.
(2) Determine the long-term effects of fMRI NFB training on functional and real-world outcomes in PWA. This may require longitudinal studies that include long-term follow up and a variety of standardized and ecologically valid behavioral assessments.
(3) Explore ways to integrate fMRI NFB with other interventions, such as SLT or other neuromodulation techniques, to enhance treatment outcomes and promote generalization.
(4) Standardize methods to assess outcomes after fMRI NFB training.
(5) Develop personalized approaches to fMRI NFB training in PWA that can rapidly accommodate individual differences in stroke anatomy and the nature of language impairment.
Addressing these questions will contribute to the continued advancement and adoption of fMRI NFB as a potential therapeutic tool for aphasia.
Acknowledgments
The authors have no acknowledgments to report.
Funding
PSB was supported by the Cognitive Neuroscience Research Program and the Department of Neurology at the Medical College of Wisconsin during the writing of this manuscript. OB was supported in part by grant K01DC019178 from NIDCD and by pilot grants from the Bolger Trust and Mabel H. Flory Charitable Trust. The content is solely the responsibility of the authors and does not necessarily represent the official views of the National Institutes of Health. AK was supported by grants from the Arizona Alzheimer’s Consortium, the Data Science Academy, and the Innovations in Healthy Aging Grand Challenges.
References
1 | Abbott, N. T. , Baker, C. J. , Chen, C. , Liu, T. T. , Love, T. E. ((2021) ) Defining hypoperfusion in chronic aphasia: An individualized thresholding approach. Brain Sciences, 11: (4), 491. https://doi.org/10.3390/brainsci11040491 |
2 | Abo, M. , Kakuda, W. , Watanabe, M. , Morooka, A. , Kawakami, K. , Senoo, A. ((2012) ). Effectiveness of low-frequency rTMS and intensive speech therapy in poststroke patients with aphasia: A pilot study based on evaluation by fMRI in relation to type of aphasia. European Neurology, 68: (4), 199–208. https://doi.org/10.1159/000338773 |
3 | Abrams, G. (2017). Intensive speech therapy effective for chronic aphasia after stroke. NEJM Journal Watch. https://www.jwatch.org/na43611/2017/03/23/intensive-speech-therapy3036effective-chronic-aphasia-after |
4 | Agboada, D. , Mosayebi-Samani, M. , Kuo, M. -F. , Nitsche, M. A. ((2020) ). Induction of long-term potentiation-like plasticity in the primary motor cortex with repeated anodal transcranial direct current stimulation – Better effects with intensified protocols? Brain Stimulation, 13: (4), 987–997. https://doi.org/10.1016/j.brs.2020.04.009 |
5 | Ajmera, Y. , Singhal, S. , Dwivedi, S. N. , Dey, A. B. ((2021) ). The changing perspective of clinical trial designs. Perspectives in Clinical Research, 12: (2), 66–71. https://doi.org/10.4103/picr.PICR_138_20 |
6 | Alam, M. , Truong, D. Q. , Khadka, N. , Bikson, M. ((2016) ). Spatial and polarity precision of concentric highdefinition transcranial direct current stimulation (HD-tDCS). Physics in Medicine and Biology, 61: (12), 4506–4521. https://doi.org/10.1088/0031-9155/61/12/4506 |
7 | Ashaie, S. A. , Engel, S. , Cherney, L. R. ((2022) ). Timing of transcranial direct current stimulation (tDCS) combined with speech and language therapy (SLT) for aphasia: Study protocol for a randomized controlled trial. Trials, 23: (1), 668. https://doi.org/10.1186/s13063-022-06627-9 |
8 | Babbitt, E. M. , Worrall, L. , Cherney, L. R. ((2015) ). Structure, processes, and retrospective outcomes from an intensive comprehensive aphasia program. American Journal of Speech-Language Pathology, 24: (4), S854–S863. https://doi.org/10.1044/2015_AJSLP-14-0164 |
9 | Bahr-Hosseini, M. , Nael, K. , Unal, G. , Iacoboni, M. , Liebeskind, D. S. , Bikson, M. , Saver, J. L. ((2023) ). High-definition cathodal direct current stimulation for treatment of acute ischemic stroke. JAMA Network Open, 6: (6), e2319231. https://doi.org/10.1001/jamanetworkopen.2023.19231 |
10 | Baker, J. , Rorden, C. , Fridriksson, J. ((2010) ). Using transcranial direct current stimulation (tDCS) to treat stroke patients with aphasia. Stroke; a Journal of Cerebral Circulation, 41: (6), 1229–1236. https://doi.org/10.1161/STROKEAHA.109.576785 |
11 | Baron, J. -C. , Marchal, G. ((1999) ). Ischemic core and penumbra in human stroke. Stroke, 30: (5), 1150–1151. https://doi.org/10.1161/01.STR.29.9.1821 |
12 | Barwood, C. H. S. , Murdoch, B. E. , Riek, S. , O’Sullivan, J. D. , Wong, A. , Lloyd, D. , Coulthard, A. ((2013) ). Long term language recovery subsequent to low frequency rTMS in chronic non-fluent aphasia. NeuroRehabilitation, 32: (4), 915–928. https://doi.org/10.3233/NRE-130915 |
13 | Bastiaanse, Y. R. M. , Jonkers, R. , Quak, C. , Varela Put, M. (1997). Werkwoordproductie op woord- en zinsniveau. Swets & Zeitlinger. |
14 | Batsikadze, G. , Moliadze, V. , Paulus, W. , Kuo, M.-F. , Nitsche, M. A. ((2013) ). Partially non-linear stimulation intensitydependent effects of direct current stimulation on motor cortex excitability in humans. The Journal of Physiology, 591: (7), 1987–2000. https://doi.org/10.1113/jphysiol.2012.249730 |
15 | Beeson, P. M. , Rising, K. , DeMarco, A. T. , Foley, T. H. , Rapcsak, S. Z. ((2018) ). The nature and treatment of phonological text agraphia. Neuropsychological Rehabilitation, 28: (4), 568–588. https://doi.org/10.1080/09602011.2016.1199387 |
16 | Beeson, P. M. , Rising, K. , Kim, E. S. , Rapcsak, S. Z. ((2010) ). A treatment sequence for phonological alexia/agraphia. Journal of Speech, Language, and Hearing Research, 53: (2), 450–468. https://doi.org/10.1044/1092-4388(2009/08-0229) |
17 | Behroozmand, R. , Johari, K. , Bridwell, K. , Hayden, C. , Fahey, D. , den Ouden, D.-B. ((2020) ). Modulation of vocal pitch control through high-definition transcranial direct current stimulation of the left ventral motor cortex. Experimental Brain Research, 238: (6), 1525–1535. https://doi.org/10.1007/s00221-020-05832-9 |
18 | Berardelli, A. , Inghilleri, M. , Rothwell, J. C. , Romeo, S. , Currà, A. , Gilio, F. , Modugno, N. , Manfredi, M. ((1998) ). Facilitation of muscle evoked responses after repetitive cortical stimulation in man. Experimental Brain Research, 122: (1), 79–84. https://doi.org/10.1007/s002210050493 |
19 | Bezmaternykh, D. D. , Kalgin, K. V. , Maximova, P. E. , Mel’nikov, M. Ye. , Petrovskii, E. D. , Predtechenskaya, E. V. , Savelov, A. A. , Semenikhina, A. A. , Tsaplina, T. N. , Shtark, M. B. , Shurunova, A. V. ((2021) ). Application of fMRI and Simultaneous fMRI-EEG Neurofeedback in Post-Stroke Motor Rehabilitation. Bulletin of Experimental Biology and Medicine, 171: (3), 379–383. https://doi.org/10.1007/s10517-021-05232-1 |
20 | Blomert, L. (1993). Partner Communication Questionnaire. Dutch Aphasia Association. |
21 | Boukrina, O. , Barrett, A. M. , Graves, W. W. ((2019) ). Cerebral perfusion of the left reading network predicts recovery of reading in subacute to chronic stroke. Human Brain Mapping, 40: (18), 5301–5314. https://doi.org/10.1002/hbm.24773 |
22 | Breitenstein, C. , Kramer, K. , Meinzer, M. , Baumgaertner, A. , Floeel, A. , Knecht, S. ((2009) ). Intensives Sprachtraining bei Aphasie. Einfluss kognitiver Faktoren [Intense language training for aphasia. Contribution of cognitive factors]. Der Nervenarzt, 80: (2), 149–154. https://doi.org/10.1007/s00115-008-2571-6 |
23 | Brooks, R. ((1996) ). EuroQol: The current state of play. Health Policy, 37: (1), 53–72. https://doi.org/10.1016/0168-8510(96)00822-6 |
24 | Brookshire, C. E. , Conway, T. , Pompon, R. H. , Oelke, M. , Kendall, D. L. ((2014) ). Effects of intensive phonomotor treatment on reading in eight individuals with aphasia and phonological alexia. American Journal of Speech-Language Pathology, 1: (23), 300–311. |
25 | Brookshire, C. E. , Wilson, J. P. , Nadeau, S. E. , Gonzalez Rothi, L. J. , Kendall, D. L. ((2014) ). Frequency, nature, and predictors of alexia in a convenience sample of individuals with chronic aphasia. Aphasiology, 28912: , 1464–1480. https://doi.org/10.1080/02687038.2014.945389 |
26 | Brumm, K. P. , Perthen, J. E. , Liu, T. T. , Haist, F. , Ayalon, L. , Love, T. ((2010) ). An arterial spin labeling investigation of cerebral blood flow deficits in chronic stroke survivors. NeuroImage, 51: (3), 995–1005. https://doi.org/10.1016/j.neuroimage.2010.03.008 |
27 | Campana, S. , Caltagirone, C. , Marangolo, P. ((2015) ). Combining voxel-based lesion-symptom mapping (VLSM) with A-tDCS language treatment: Predicting outcome of recovery in nonfluent chronic aphasia. Brain Stimulation, 8: (4), 769–776. https://doi.org/10.1016/j.brs.2015.01.413 |
28 | Chen, R. , Classen, J. , Gerloff, C. , Celnik, P. , Wassermann, E. M. , Hallett, M. , Cohen, L. G. ((1997) ). Depression of motor cortex excitability by low-frequency transcranial magnetic stimulation. Neurology, 48: (5), 1398–1403. https://doi.org/10.1212/wnl.48.5.1398 |
29 | Cherney, L. R. , Babbitt, E. M. , Wang, X. , Pitts, L. L. ((2021) ). Extended fMRI-guided anodal and cathodal transcranial direct current stimulation targeting perilesional areas in poststroke aphasia: A pilot randomized clinical trial. Brain Sciences, 11: (3), 306. https://doi.org/10.3390/brainsci11030306 |
30 | Chew, T. , Ho, K. -A. , Loo, C. K. ((2015) ). Interand intra-individual variability in response to transcranial direct current stimulation (tDCS) at varying current intensities. Brain Stimulation, 8: (6), 1130–1137. https://doi.org/10.1016/j.brs.2015.07.031 |
31 | Chieffo, R. , Ferrari, F. , Battista, P. , Houdayer, E. , Nuara, A. , Alemanno, F. , Abutalebi, J. , Zangen, A. , Comi, G. , Cappa, S. F. , Leocani, L. ((2014) ). Excitatory deep transcranial magnetic stimulation with H-coil over the right homologous Broca’s region improves naming in chronic post-stroke aphasia. Neurorehabilitation and Neural Repair, 28: (3), 291–298. https://doi.org/10.1177/1545968313508471 |
32 | Choi, J. Y. , Perrachione, T. K. ((2019) ). Noninvasive neurostimulation of left temporal lobe disrupts rapid talker adaptation in speech processing. Brain and Language, 196: , 104655. https://doi.org/10.1016/j.bandl.2019.104655 |
33 | Chu, R. K. O. , Braun, A. R. , Meltzer, J. A. ((2015) ). MEG-based detection and localization of perilesional dysfunction in chronic stroke. NeuroImage. Clinical, 8: , 157–169. https://doi.org/10.1016/j.nicl.2015.03.019 |
34 | Cloutman, L. L. , Newhart, M. , Davis, C. L. , Heidler-Gary, J. , Hillis, A. E. ((2011) ). Neuroanatomical correlates of oral reading in actue left hemisphere stroke. Brain and Language, 116: (1), 14–21. https://doi.org/10.1523/JNEUROSCI.3593-07.2007.Omega-3 |
35 | Collins, A. M. , Loftus, E. F. ((1975) ). A spreading-activation theory of semantic processing. Psychological Review, 82: (6), 407–428. https://doi.org/10.1037/0033-295X.82.6.407 |
36 | Crosson, B. , Mcgregor, K. , Gopinath, K. S. , Conway, T. W. , Benjamin, M. , Chang, Y. , Moore, A. B. , Raymer, A. M. , Briggs, R. W. , Sherod, M. G. , Wierenga, C. E. , White, K. D. ((2007) ). Funcitonal MRI of language in aphasia: A review of the literature and the methodological challenges. Neuropsychology Review, 17: (2), 157–177. https://doi.org/10.1007/s11065-007-9024-z.Functional |
37 | Dalemans, R. J. , de Witte, L. P. , Beurskens, A. J. , van den Heuvel, W. J. , Wade, D. T. ((2010) ). Psychometric properties of the community integration questionnaire adjusted for people with aphasia. Archives of Physical Medicine and Rehabilitation, 91: (3), 395–399. https://doi.org/10.1016/j.apmr.2009.10.021 |
38 | Darkow, R. , Martin, A. , Würtz, A. , Flöel, A. , Meinzer, M. ((2017) ). Transcranial direct current stimulation effects on neuralprocessing in post-stroke aphasia. Human Brain Mapping, 38: (3), 1518–1531. https://doi.org/10.1002/hbm.23469 |
39 | Datta, A. , Baker, J. M. , Bikson, M. , Fridriksson, J. ((2011) ). Individualized model predicts brain current flow during transcranial direct-current stimulation treatment in responsive stroke patient. Brain Stimulation, 4: (3), 169–174. https://doi.org/10.1016/j.brs.2010.11.001 |
40 | Datta, A. , Bansal, V. , Diaz, J. , Patel, J. , Reato, D. , Bikson, M. ((2009) ). Gyri-precise head model of transcranial direct current stimulation: Improved spatial focality using a ring electrode versus conventional rectangular pad. Brain Stimulation, 2: (4), 201–207, 207.e1. https://doi.org/10.1016/j.brs.2009.03.005 |
41 | de Aguiar, V. , Bastiaanse, R. , Capasso, R. , Gandolfi, M. , Smania, N. , Rossi, G. , Miceli, G. ((2015) ). Can tDCS enhance item-specific effects and generalization after linguistically motivated aphasia therapy for verbs? Frontiers in Behavioral Neuroscience, 9: , 190. https://www.frontiersin.org/articles/10.3389/fnbeh.2015.00190 |
42 | de Aguiar, V. , Paolazzi, C. L. , Miceli, G. ((2015) ). tDCS in post-stroke aphasia: The role of stimulation parameters, behavioral treatment and patient characteristics. Cortex, 63: , 296–316. https://doi.org/10.1016/j.cortex.2014.08.015 |
43 | De Smet, H. J. , Paquier, P. , Verhoeven, J. , Mariën, P. ((2013) ). The cerebellum: Its role in language and related cognitive and affective functions. Brain and Language, 127: (3), 334–342. https://doi.org/10.1016/j.bandl.2012.11.001 |
44 | deBettencourt, M. T. , Cohen, J. D. , Lee, R. F. , Norman, K. A. , Turk-Browne, N. B. ((2015) ). Closed-loop training of attention with real-time brain imaging. Nature Neuroscience, 18: (3), 470–475. https://doi.org/10.1038/nn.3940 |
45 | deBettencourt, M. T. , Turk-Browne, N. B. , Norman, K. A. ((2019) ). Neurofeedback helps to reveal a relationship between context reinstatement and memory retrieval. NeuroImage, 200: , 292–301. https://doi.org/10.1016/j.neuroimage.2019.06.001 |
46 | DeMarco, A. T. , Dvorak, E. , Lacey, E. , Stoodley, C. J. , Turkeltaub, P. E. ((2021) ). An exploratory study of cerebellar transcranial direct current stimulation in individuals with chronic stroke aphasia. Cognitive and Behavioral Neurology: Official Journal of the Society for Behavioral and Cognitive Neurology, 34: (2), 96–106. https://doi.org/10.1097/WNN.0000000000000270 |
47 | DeMarco, A. T. , Wilson, S. M. , Rising, K. , Rapcsak, S. Z. , Beeson, P. M. ((2018) ). The neural substrates of improved phonological processing following successful treatment in a case of phonological alexia and agraphia. Neurocase, 24: (1), 31–40. https://doi.org/10.1080/13554794.2018.1428352 |
48 | Dewiputri, W. I. , Auer, T. ((2013) ). Functional magnetic resonance imaging (FMRI) neurofeedback: Implementations and applications. The Malaysian Journal of Medical Sciences: MJMS, 20: (5), 5–15. |
49 | Dickey, M. W. , Hula, W. , Yoo, H. (2014). Acquisition and generalization responses in aphasia treatment: Evidence from sentence-production treatment. Clinical Aphasiology Conference Paper. http://aphasiology.pitt.edu/2571/ |
50 | Dignam, J. , Copland, D. , O’Brien, K. , Burfein, P. , Khan, A. , Rodriguez, A. D. ((2017) ). Influence of cognitive ability on therapyoutcomes for anomia in adults with chronic poststroke aphasia. Journal of Speech Language and Hearing Research, 60: (2), 406–421. https://doi.org/10.1044/2016JSLHR-L-15-0384 |
51 | Dipper, L. , Marshall, J. , Boyle, M. , Botting, N. , Hersh, D. , Pritchard, M. , Cruice, M. ((2021) ). Treatment for improving discourse in aphasia: A systematic review and synthesis of the evidence base. Aphasiology, 35: (9), 1125–1167. https://doi.org/10.1080/02687038.2020.1765305 |
52 | D’Mello, A. M. , Turkeltaub, P. E. , Stoodley, C. J. ((2017) ). Cerebellar tDCS Modulates Neural Circuits during Semantic Prediction: A Combined tDCS-fMRI Study. Journal of Neuroscience, 37: (6), 1604–1613. https://doi.org/10.1523/JNEUROSCI.2818-16.2017 |
53 | Dmochowski, J. P. , Datta, A. , Huang, Y. , Richardson, J. , Bikson, M. , Fridriksson, J. , Parra, L. C. ((2013) ). Targeted transcranial direct current stimulation for rehabilitation after stroke. NeuroImage, 75: , 12–19. https://doi.org/10.1016/j.neuroimage.2013.02.049 |
54 | Dreyer, F. R. , Doppelbauer, L. , Buscher, V. , Arndt, V. , Stahl, B. , Lucchese, G. , Hauk, O. , Mohr, B. , Pulvermüller, F. ((2021) ). Increased recruitment of domain-general neural networks in language processing following intensive language-action therapy: FMRI evidence from people with chronic aphasia. American Journal of Speech-Language Pathology, 30: (1S), 455–465. |
55 | Edmonds, L. A. ((2016) ). A review of verb network strengthening treatment: Theory, methods, results, and clinical implications. Topics in Language Disorders, 36: (2), 123–135. https://doi.org/10.1097/TLD.0000000000000088 |
56 | Edmonds, L. A. , Nadeau, S. E. , Kiran, S. ((2009) ). Effect of verb network strengthening treatment (VNeST) on lexical retrieval of content words in sentences in persons with aphasia. Aphasiology, 23: (3), 402–424. https://doi.org/10.1080/02687030802291339 |
57 | Edmonds, L. A. , Obermeyer, J. , Kernan, B. ((2015) ). Investigation of pretreatment sentence production impairments in individuals with aphasia: Towards understanding the linguistic variables that impact generalisation in Verb Network Strengthening Treatment. Aphasiology, 29: (11), 1312–1344. https://doi.org/10.1080/02687038.2014.975180 |
58 | Elsner, B. , Kugler, J. , Mehrholz, J. ((2020) ). Transcranial direct current stimulation (tDCS) for improving aphasia after stroke: A systematic review with network meta-analysis of randomized controlled trials. Journal of Neuroengineering and Rehabilitation, 17: (1), 88. https://doi.org/10.1186/s12984-020-00708-z |
59 | Elsner, B. , Kugler, J. , Pohl, M. , Mehrholz, J. ((2019) ). Transcranial direct current stimulation (tDCS) for improving aphasia in adults with aphasia after stroke. The Cochrane Database of Systematic Reviews, 5: (5), CD009760. https://doi.org/10.1002/14651858.CD009760.pub4 |
60 | Eom, B. , Sung, J. E. ((2016) ). The effects of sentence repetition–based working memory treatment on sentence comprehension abilities in individuals with aphasia. American Journal of Speech-Language Pathology, 25: (4S). https://doi.org/10.1044/2016_AJSLP-15-0151 |
61 | Figeys, M. , Zeeman, M. , Kim, E. S. ((2021) ). Effects of transcranial direct current stimulation (tDCS) on cognitive performance and cerebral oxygen hemodynamics: A systematic review. Frontiers in Human Neuroscience, 15: , 623315. https://doi.org/10.3389/fnhum.2021.623315 |
62 | Flöel, A. , Meinzer, M. , Kirstein, R. , Nijhof, S. , Deppe, M. , Knecht, S. , Breitenstein, C. ((2011) ). Short-term anomia training and electrical brain stimulation. Stroke, 42: (7), 2065–2067. https://doi.org/10.1161/STROKEAHA.110.609032 |
63 | Flowers, H. L. , Skoretz, S. A. , Silver, F. L. , Rochon, E. , Fang, J. , Flamand-Roze, C. , Martino, R. ((2016) ). Poststroke aphasia frequency, recovery, and outcomes: A systematic review and meta-analysis. Archives of Physical Medicine and Rehabilitation, 97: (12), 2188–2201.e8. https://doi.org/10.1016/j.apmr.2016.03.006 |
64 | Francis, D. , Clark, N. , Humphreys, G. ((2003) ). The treatment of an auditory working memory deficit and the implications for sentence comprehension abilities in mild “receptive” aphasia. Aphasiology, 17: (8), 723–750. https://doi.org/10.1080/02687030344000201 |
65 | Frankel, T. , Penn, C. , Ormond-Brown, D. ((2007) ). Executive dysfunction as an explanatory basis for conversation symptoms of aphasia: A pilot study. Aphasiology, 21: (6–8), 814–828. https://doi.org/10.1080/02687030701192448 |
66 | Fridriksson, J. ((2010) ). Preservation and modulation of specific left hemisphere regions is vital for treated recovery from anomia in stroke. Journal of Neuroscience, 30: (35), 11558–11564. https://doi.org/10.1523/JNEUROSCI.2227-10.2010 |
67 | Fridriksson, J. , Bonilha, L. , Baker, J. M. , Moser, D. , Rorden, C. ((2010) ). Activity in preserved left hemisphere regions predicts anomia severity in aphasia. Cerebral Cortex, 20: (5), 1013–1019. https://doi.org/10.1093/cercor/bhp160 |
68 | Fridriksson, J. , Elm, J. , Stark, B. C. , Basilakos, A. , Rorden, C. , Sen, S. , George, M. S. , Gottfried, M. , Bonilha, L. ((2018) ). BDNF genotype and tDCS interaction in aphasia treatment. Brain Stimulation, 11: (6), 1276–1281. https://doi.org/10.1016/j.brs.2018.08.009 |
69 | Fridriksson, J. , Nettles, C. , Davis, M. , Morrow, L. , Montgomery, A. ((2006) ). Functional communication and executive function in aphasia. Clinical Linguistics & Phonetics, 20: (6), 401–410. https://doi.org/10.1080/02699200500075781 |
70 | Fridriksson, J. , Richardson, J. D. , Baker, J. M. , Rorden, C. ((2011) ). Transcranial direct current stimulation improves naming reaction time in fluent aphasia: A double-blind, sham-controlled study. Stroke, 42: (3), 819–821. https://doi.org/10.1161/STROKEAHA.110.600288 |
71 | Fridriksson, J. , Richardson, J. D. , Fillmore, P. , Cai, B. ((2012) ). Left hemisphere plasticity and aphasia recovery. Neuroimage, 60: (2), 854–863. https://doi.org/10.1016/j.neuroimage.2011.12.057 |
72 | Fridriksson, J. , Rorden, C. , Elm, J. , Sen, S. , George, M. S. , Bonilha, L. ((2018) ). Transcranial direct current stimulation vs sham stimulation to treat aphasia after stroke: A randomized clinical trial. JAMA Neurology, 75: (12), 1470–1476. https://doi.org/10.1001/jamaneurol.2018.2287 |
73 | Gainotti, G. ((1993) ). The riddle of the right hemisphere’s contribution to the recovery of language. European Journal of Disorders of Communication, 28: (3), 227–246. https://doi.org/10.3109/13682829309060038 |
74 | Galletta, E. E. , Vogel-Eyny, A. ((2015) ). Translational treatment of aphasia combining neuromodulation and behavioral intervention for lexical retrieval: Implications from a single case study. Frontiers in Human Neuroscience, 9: , 447. https://www.frontiersin.org/articles/10.3389/fnhum.2015.00447 |
75 | Georgiou, A. M. , Kambanaros, M. ((2022) ). The effectiveness of transcranial magnetic stimulation (TMS) paradigms as treatment options for recovery of language deficits in chronic poststroke aphasia. Behavioural Neurology, 2022: , 7274115. https://doi.org/10.1155/2022/7274115 |
76 | Geranmayeh, F. , Leech, R. , Wise, R. J. S. ((2016) ). Network dysfunction predicts speech production after left hemisphere stroke. Neurology, 86: (14), 1296–1305. https://doi.org/10.1212/WNL.0000000000002537 |
77 | Gersner, R. , Kravetz, E. , Feil, J. , Pell, G. , Zangen, A. ((2011) ). Long-term effects of repetitive transcranial magnetic stimulation on markers for neuroplasticity: Differential outcomes in anesthetized and awake animals. The Journal of Neuroscience, 31: (20), 7521–7526. https://doi.org/10.1523/JNEUROSCI.6751-10.2011 |
78 | Gholami, M. , Pourbaghi, N. , Taghvatalab, S. ((2022) ). Evaluation ofrTMS in patients with poststroke aphasia: A systematic review andfocused meta-analysis. Neurological Sciences: Official Journal of the Italian Neurological Society and of the Italian Society of Clinical Neurophysiology, 43: (8), 4685–4694. https://doi.org/10.1007/s10072-022-06092-x |
79 | Gill, J. , Shah-Basak, P. , Hamilton, R. ((2015) ). It’s the thought that counts: Examining the task-dependent effects of transcranial direct current stimulation on executive function. Brain Stimulation, 8: (2), 253–259. https://doi.org/10.1016/j.brs.2014.10.018 |
80 | Gilmore, N. , Meier, E. L. , Johnson, J. P. , Kiran, S. ((2019) ). Nonlinguistic cognitive factors predict treatment-induced recovery in chronic poststroke aphasia. Archives of Physical Medicine and Rehabilitation, 100: (7), 1251–1258. https://doi.org/10.1016/j.apmr.2018.12.024 |
81 | Gilmore, N. , Meier, E. L. , Johnson, J. P. , Kiran, S. ((2020) ). Typicality-based semantic treatment for anomia results in multiple levels of generalisation. Neuropsychological Rehabilitation, 30: (5), 802–828. https://doi.org/10.1080/09602011.2018.1499533 |
82 | Giulia, L. , Adolfo, V. , Julie, C. , Quentin, D. , Simon, B. , Fleury, M. , Leveque-Le Bars, E. , Bannier, E. , Lécuyer, A. , Barillot, C. , Bonan, I. ((2021) ). The impact of neurofeedback on effective connectivity networks in chronic stroke patients: An exploratory study. Journal of Neural Engineering, 18: (5), 056052. https://doi.org/10.1088/1741-2552/ac291e |
83 | Goodglass, H. , Kaplan, E. , Barresi, B. (2000). The Boston Diagnostic Aphasia Examination. Lippincott. |
84 | Green, A. E. , Spiegel, K. A. , Giangrande, E. J. , Weinberger, A. B. , Gallagher, N. M. , Turkeltaub, P. E. ((2017) ). Thinking cap plusthinking zap: TDCS of frontopolar cortex improves creativeanalogical reasoning and facilitates consciousaugmentation of state creativity in verbgeneration. Cerebral Cortex, 27: (4), 2628–2639. https://doi.org/10.1093/cercor/bhw080 |
85 | Griffis, J. C. , Nenert, R. , Allendorfer, J. B. , Szaflarski, J. P. ((2016) ). Interhemispheric plasticity following intermittent theta burst stimulation in chronic poststroke aphasia. Neural Plasticity, 2016: , 4796906. https://doi.org/10.1155/2016/4796906 |
86 | Grimaldi, G. , Argyropoulos, G. P. , Bastian, A. , Cortes, M. , Davis, N. J. , Edwards, D. J. , Ferrucci, R. , Fregni, F. , Galea, J. M. , Hamada, M. , Manto, M. , Miall, R. C. , Morales-Quezada, L. , Pope, P. A. , Priori, A. , Rothwell, J. , Tomlinson, S. P. , Celnik, P. ((2016) ). Cerebellar transcranial direct current stimulation (ctDCS). The Neuroscientist, 22: (1), 83–97. https://doi.org/10.1177/1073858414559409 |
87 | Guillouët, E. , Cogné, M. , Saverot, E. , Roche, N. , Pradat-Diehl, P. , Weill-Chounlamountry, A. , Ramel, V. , Taratte, C. , Lachasse, A.-G. , Haulot, J.-A. , Vaugier, I. , Barbot, F. , Azouvi, P. , Charveriat, S. ((2020) ). Impact of combined transcranial direct current stimulation and speech-language therapy on spontaneous speech in aphasia: A randomized controlled double-blind study. Journal of the International Neuropsychological Society: JINS, 26: (1), 7–18. https://doi.org/10.1017/S1355617719001036 |
88 | Hallett, M. ((2007) ). Transcranial magnetic stimulation: A primer. Neuron, 55: (2), 187–199. https://doi.org/10.1016/j.neuron.2007.06.026 |
89 | Hamilton, R. H. , Chrysikou, E. G. , Coslett, B. ((2011) ). Mechanisms of aphasia recovery after stroke and the role of noninvasive brain stimulation. Brain and Language, 118: (1–2), 40–50. https://doi.org/10.1016/j.bandl.2011.02.005 |
90 | Hamilton, R. H. , Sanders, L. , Benson, J. , Faseyitan, O. , Norise, C. , Naeser, M. , Martin, P. , Coslett, H. B. ((2010) ). Stimulatingconversation: Enhancement of elicited propositional speech in apatient with chronic non-fluent aphasia following transcranialmagnetic stimulation. Brain and Language, 113: (1), 45–50. https://doi.org/10.1016/j.bandl.2010.01.001 |
91 | Hara, T. , Abo, M. , Kakita, K. , Mori, Y. , Yoshida, M. , Sasaki, N. ((2017) ). The effect of selective transcranial magnetic stimulation with functional near-infrared spectroscopy and intensive speech therapy on individuals with post-stroke aphasia. European Neurology, 77: (3–4), 186–194. https://doi.org/10.1159/000457901 |
92 | Hara, T. , Abo, M. , Kobayashi, K. , Watanabe, M. , Kakuda, W. , Senoo, A. ((2015) ). Effects of low-frequency repetitive transcranial magnetic stimulation combined with intensive speech therapy on cerebral blood flow in post-stroke aphasia. Translational Stroke Research, 6: (5), 365–374. https://doi.org/10.1007/s12975-015-0417-7 |
93 | Harris, L. , Olson, A. , Humphreys, G. ((2014) ). The link between STM and sentence comprehension: A neuropsychological rehabilitation study. Neuropsychological Rehabilitation, 24: (5), 678–720. https://doi.org/10.1080/09602011.2014.892885 |
94 | Hartwigsen, G. , Saur, D. ((2019) ). Neuroimaging of stroke recoveryfrom aphasia –Insights into plasticity of the human languagenetwork. NeuroImage, 190: , 14–31. https://doi.org/10.1016/j.neuroimage.2017.11.056 |
95 | Harvey, D. Y. , Mass, J. A. , Shah-Basak, P. P. , Wurzman, R. , Faseyitan, O. , Sacchetti, D. L. , DeLoretta, L. , Hamilton, R. H. ((2019) ). Continuous theta burst stimulation overright pars triangularis facilitates naming abilities in chronicpost-stroke aphasia by enhancing phonological access. Brain and Language, 192: , 25–34. https://doi.org/10.1016/j.bandl.2019.02.005 |
96 | Harvey, D. Y. , Podell, J. , Turkeltaub, P. E. , Faseyitan, O. , Coslett, H. B. , Hamilton, R. H. ((2017) ). Functional reorganization of right prefrontal cortex underlies sustained naming improvements in chronic aphasia via repetitive transcranial magnetic stimulation. Cognitive and Behavioral Neurology: Official Journal of the Society for Behavioral and Cognitive Neurology, 30: (4), 133–144. https://doi.org/10.1097/WNN.0000000000000141 |
97 | Heath, S. , McMahon, K. L. , Nickels, L. , Angwin, A. , MacDonald, A. D. , van Hees, S. , Johnson, K. , McKinnon, E. , Copland, D. A. ((2012) ). Neural mechanisms underlying the facilitation of naming in aphasia using a semantic task: An fMRI study. BMC Neuroscience, 13: (1), 98. https://doi.org/10.1186/1471-2202-13-98 |
98 | Heikkinen, P. H. , Pulvermüller, F. , Mäkelä, J. P. , Ilmoniemi, R. J. , Lioumis, P. , Kujala, T. , Manninen, R.-L. , Ahvenainen, A. , Klippi, A. ((2019) ). Combining rTMS with intensive language-action therapy in chronic aphasia: A randomized controlled trial. Frontiers in Neuroscience, 12: , 1036. https://www.frontiersin.org/articles/10.3389/fnins.2018.01036 |
99 | Heiss, W.-D. , Hartmann, A. , Rubi-Fessen, I. , Anglade, C. , Kracht, L. , Kessler, J. , Weiduschat, N. , Rommel, T. , Thiel, A. ((2013) ). Noninvasive brain stimulation for treatment of right- and left-handed poststroke aphasics. Cerebrovascular Diseases (Basel, Switzerland), 36: (5–6), 363–372. https://doi.org/10.1159/000355499 |
100 | Helm-Estabrooks, N. ((2002) ). Cognition and aphasia: A discussion anda study. Journal of Communication Disorders, 35: (2), 171–186. https://doi.org/10.1016/S0021-9924(02)00063-1 |
101 | Helm-Estabrooks, N. , Nicholas, M. (2000). Sentence production program for aphasia. Pro-ed. |
102 | Hilari, K. , Byng, S. , Lamping, D. L. , Smith, S. C. ((2003) ). Stroke and aphasia quality of life scale-39 (SAQOL-39): Evaluation of acceptability, reliability, and validity. Stroke, 34: (8), 1944–1950. https://doi.org/10.1161/01.STR.0000081987.46660.ED |
103 | Hillis, A. E. ((2007) ). Pharmacological, surgical, and neurovascular interventions to augment acute aphasia recovery. Journal of Physical Medicine & Rehabilitation, 86: (6), 426–434. https://doi.org/10.1097/PHM.0b013e31805ba094 |
104 | Hillis, A. E. , Heidler, J. ((2002) ). Mechanisms of early aphasia recovery. Aphasiology, 16: (9), 885–895. https://doi.org/10.1080/0268703 |
105 | Hillis, A. E. , Vimal, S. , Newhart, M. , Aldrich, E. , Heidler, J. , Ken, L. ((2005) ). Reperfusion of selective areas is association with improved naming in acute stroke. Brain and Language, 95: (1), 100–101. |
106 | Hsu, T.-Y. , Juan, C.-H. , Tseng, P. ((2016) ). Individual differences and state-dependent responses in transcranial direct current stimulation. Frontiers in Human Neuroscience, 10: , 643. https://doi.org/10.3389/fnhum.2016.00643 |
107 | Hu, X. , Zhang, T. , Rajah, G. B. , Stone, C. , Liu, L. , He, J. , Shan, L. , Yang, L. , Liu, P. , Gao, F. , Yang, Y. , Wu, X. , Ye, C. , Chen, Y. ((2018) ). Effects of different frequencies of repetitive transcranial magnetic stimulation in stroke patients with non-fluent aphasia: A randomized, sham-controlled study. Neurological Research, 40: (6), 459–465. https://doi.org/10.1080/01616412.2018.1453980 |
108 | Huang, Y. -Z. , Edwards, M. J. , Rounis, E. , Bhatia, K. P. , Rothwell, J. C. ((2005) ). Theta burst stimulation of the human motor cortex. Neuron, 45: (2), 201–206. https://doi.org/10.1016/j.neuron.2004.12.033 |
109 | Huang, Y.-Z. , Rothwell, J. C. , Chen, R.-S. , Lu, C.-S. , Chuang, W.-L. ((2011) ). The theoretical model of theta burst form of repetitive transcranial magnetic stimulation. Clinical Neurophysiology: Official Journal of the International Federation of Clinical Neurophysiology, 122: (5), 1011–1018. https://doi.org/10.1016/j.clinph.2010.08.016 |
110 | Iadecola, C. , Yang, G. , Ebner, T. J. , Chen, G. ((1997) ). Local and propagated vascular responses evoked by focal synaptic activity in cerebellar cortex. Journal of Neurophysiology, 78: (2), 651–659. https://doi.org/10.1152/jn.1997.78.2.651 |
111 | iMTA. (n.d.). IMTA Medical Consumption Questionnaire. IMTA. Retrieved March 20, 2023, from https://www.imta.nl/questionnaires/imcq/ |
112 | Jacobson, L. , Koslowsky, M. , Lavidor, M. ((2012) ). tDCS polarity effects in motor and cognitive domains: A meta-analytical review. XXXX, 216: (1), 1–10. https://doi.org/10.1007/s00221-011-2891-9 |
113 | Johari, K. , Riccardi, N. , Malyutina, S. , Modi, M. , Desai, R. H. ((2021) ). HD-tDCS over motor cortex facilitates figurative and literal action sentence processing. Neuropsychologia, 159: , 107955. https://doi.org/10.1016/j.neuropsychologia.2021.107955 |
114 | Johnson, L. , Basilakos, A. , Yourganov, G. , Cai, B. , Bonilha, L. , Rorden, C. , Fridriksson, J. ((2019) ). Progression of aphasia severity in the chronic stages of stroke. American Journal of Speech-Language Pathology, 28: (2), 639–649. https://doi.org/10.1044/2018_AJSLP-18-0123 |
115 | Johnson, M. D. , Lim, H. H. , Netoff, T. I. , Connolly, A. T. , Johnson, N. , Roy, A. , Holt, A. , Lim, K. O. , Carey, J. R. , Vitek, J. L. , He, B. ((2013) ). Neuromodulation for brain disorders: Challenges and opportunities. IEEE Transactions on Bio-Medical Engineering, 60: (3), 610–624. https://doi.org/10.1109/TBME.2013.2244890 |
116 | Kalinyak-Fliszar, M. , Kohen, F. , Martin, N. ((2011) ). Remediation of language processing in aphasia: Improving activation and maintenance of linguistic representations in (verbal) short-term memory. Aphasiology, 25: (10), 1095–1131. https://doi.org/10.1080/02687038.2011.577284 |
117 | Kang, E. K. , Kim, Y. K. , Sohn, H. M. , Cohen, L. G. , Paik, N. -J. ((2011) ). Improved picture naming in aphasia patients treated with cathodal tDCS to inhibit the right Broca’s homologue area. Restorative Neurology and Neuroscience, 29: (3), 141–152. https://doi.org/10.3233/RNN-2011-0587 |
118 | Kendall, D. L. , Oelke, M. , Brookshire, C. E. , Nadeau, S. E. ((2015) ). The Influence of phonomotor treatment on word retrieval abilities in 26 individuals with chronic aphasia: An open trial. Journal of Speech, Language, and Hearing Research, 58: , 798–812. https://doi.org/10.1044/2015 |
119 | Kertesz, A. (2007). Western Aphasia Battery Revised. Pearson. |
120 | Kielar, A. , Deschamps, T. , Chu, R. K. O. , Jokel, R. , Khatamian, Y. B. , Chen, J. J. , Meltzer, J. A. ((2016) ). Identifying dysfunctional cortex: Dissociable effects of stroke and aging on resting state dynamics in MEG and fMRI. Frontiers in Aging Neuroscience, 8: , 40. https://www.frontiersin.org/articles/10.3389/fnagi.2016.00040 |
121 | Kielar, A. , Panamsky, L. , Links, K. A. , Meltzer, J. A. ((2015) ). Localization of electrophysiological responses to semantic and syntactic anomalies in language comprehension with MEG. NeuroImage, 105: , 507–524. https://doi.org/10.1016/j.neuroimage.2014.11.016 |
122 | Kielar, A. , Patterson, D. , Chou, Y.-H. ((2022) ). Efficacy of repetitive transcranial magnetic stimulation in treating stroke aphasia: Systematic review and meta-analysis. Clinical Neurophysiology: Official Journal of the International Federation of Clinical Neurophysiology, 140: , 196–227. https://doi.org/10.1016/j.clinph.2022.04.017 |
123 | Kim, D.-Y. , Yoo, S.-S. , Tegethoff, M. , Meinlschmidt, G. , Lee, J.-H. ((2015) ). The inclusion of functional connectivity information into fMRI-based neurofeedback improves its efficacy in the reduction of cigarette cravings. Journal of Cognitive Neuroscience, 27: (8), 1552–1572. https://doi.org/10.1162/jocn_a_00802 |
124 | Kindler, J. , Schumacher, R. , Cazzoli, D. , Gutbrod, K. , Koenig, M. , Nyffeler, T. , Dierks, T. , Müri, R. M. ((2012) ). Theta burst stimulation over the right broca’s homologue induces improvement of naming in aphasic patients. Stroke, 43: (8), 2175–2179. https://doi.org/10.1161/STROKEAHA.111.647503 |
125 | Kiran, S. ((2007) ). Complexity in the treatment of naming deficits. American Journal of Speech-Language Pathology / American Speech-Language-Hearing Association, 16: (1), 18–29. https://doi.org/10.1044/1058-0360(2007/004) |
126 | Kiran, S. , Thompson, C. K. ((2003) ). The role of semantic complexity in treatment of naming deficits: Training semantic categories in fluent aphasia by controlling exemplar typicality. Journal of Speech, Language, and Hearing Research: JSLHR, 46: (4), 773–787. https://doi.org/10.1044/1092-4388(2003/061) |
127 | Klomjai, W. , Katz, R. , Lackmy-Vallée, A. ((2015) ). Basic principles of transcranial magnetic stimulation (TMS) and repetitive TMS (rTMS). Annals of Physical and Rehabilitation Medicine, 58: (4), 208–213. https://doi.org/10.1016/j.rehab.2015.05.005 |
128 | Koush, Y. , Ashburner, J. , Prilepin, E. , Sladky, R. , Zeidman, P. , Bibikov, S. , Scharnowski, F. , Nikonorov, A. , De Ville, D. V. ((2017) ). OpenNFT: An open-source Python/Matlab framework for real-time fMRI neurofeedback training based on activity, connectivity and multivariate pattern analysis. NeuroImage, 156: , 489–503. https://doi.org/10.1016/j.neuroimage.2017.06.039 |
129 | Koush, Y. , Meskaldji, D. -E. , Pichon, S. , Rey, G. , Rieger, S. W. , Linden, D. E. J. , Van De Ville, D. , Vuilleumier, P. , Scharnowski, F. ((2015) ). Learning control over emotion networks through connectivity-based neurofeedback. Cerebral Cortex, 27: (2), 1193–1202. https://doi.org/10.1093/cercor/bhv311 |
130 | Koush, Y. , Rosa, M. J. , Robineau, F. , Heinen, K. , Rieger, W. S. , Weiskopf, N. , Vuilleumier, P. , Van De Ville, D. , Scharnowski, F. ((2013) ). Connectivity-based neurofeedback: Dynamic causal modeling for real-time fMRI. NeuroImage, 81: , 422–430. https://doi.org/10.1016/j.neuroimage.2013.05.010 |
131 | Krames, E. S. , Hunter Peckham, P. , Rezai, A. , Aboelsaad, F. (2009). Chapter 1—What Is Neuromodulation? In E. S. Krames, P. H. Peckham, & A. R. Rezai (Eds.), Neuromodulation, pp. 3–8. Academic Press. https://doi.org/10.1016/B978-0-12-374248-3.00002-1 |
132 | Krylova, M. , Skouras, S. , Razi, A. , Nicholson, A. A. , Karner, A. , Steyrl, D. , Boukrina, O. , Rees, G. , Scharnowski, F. , Koush, Y. ((2021) ). Progressive modulation of resting-state brain activity during neurofeedback of positive-social emotion regulation networks. Scientific Reports, 11: (1), 23363. https://doi.org/10.1038/s41598-021-02079-4 |
133 | Lee, S. Y. , Cheon, H.-J. , Yoon, K. J. , Chang, W. H. , Kim, Y.-H. ((2013) ). Effects of dual transcranial direct current stimulation for aphasia in chronic stroke patients. Annals of Rehabilitation Medicine, 37: (5), 603–610. https://doi.org/10.5535/arm.2013.37.5.603 |
134 | Li, L. M. , Uehara, K. , Hanakawa, T. ((2015) ). The contribution of interindividual factors to variability of response in transcranial direct current stimulation studies. Frontiers in Cellular Neuroscience, 9: , 181. https://www.frontiersin.org/articles/10.3389/fncel.2015.00181 |
135 | Liao, W. , Chiang, W. , Lin, K. , Wu, C. , Liu, C. , Hsieh, Y. , Lin, Y. , Chen, C. ((2020) ). Timing-dependent effects of transcranial direct current stimulation with mirror therapy on daily function and motor control in chronic stroke: A randomized controlled pilot study. Journal of NeuroEngineering and Rehabilitation, 17: , 101. https://doi.org/10.1186/s12984-020-00722-1 |
136 | Liew, S.-L. , Rana, M. , Cornelsen, S. , Fortunato de Barros Filho, M. , Birbaumer, N. , Sitaram, R. , Cohen, L. G. , Soekadar, S. R. ((2016) ). Improving motor corticothalamic communication after stroke using real-time fMRI connectivity-based neurofeedback. Neurorehabilitation and Neural Repair, 30: (7), 671–675. https://doi.org/10.1177/1545968315619699 |
137 | Lindamood, P. , Lindamood, P. (1998). The Lindamood phoneme sequencing program for reading, spelling, and speech: The LiPS program. [Multimedia Kit]. PRO-ED, Inc. |
138 | Links, P. , Hurkmans, J. , Bastiaanse, R. ((2010) ). Training verb and sentence production in agrammatic Broca’s aphasia. Aphasiology, 24: (11), 1303–1325. https://doi.org/10.1080/02687030903437666 |
139 | Lomas, J. , Pickard, L. , Bester, S. , Elbard, H. , Finlayson, A. , Zoghaib, C. ((1989) ). The communicative effectiveness index. Journal of Speech and Hearing Disorders, 54: (1), 113–124. https://doi.org/10.1044/jshd.5401.113 |
140 | Madden, E. B. , Torrence, J. , Kendall, D. L. ((2021) ). Cross-modal generalization of anomia treatment to reading in aphasia. In Aphasiology, 35: (7), 875–899. https://doi.org/10.1080/02687038.2020.1734529 |
141 | Majerus, S. ((2018) ). Working memory treatment in aphasia: Atheoretical and quantitative review. Journal of Neurolinguistics, 48: , 157–175. https://doi.org/10.1016/j.jneuroling.2017.12.001 |
142 | Malyutina, S. , Ouden den, D.-B. ((2015) ). High-definition tDCS ofnoun and verb retrieval in naming and lexical decision. NeuroRegulation, 2: (3), Article 3. https://doi.org/10.15540/nr.2.3.111 |
143 | Mangano, G. R. , Turriziani, P. , Bonnì, S. , Caltagirone, C. , Oliveri, M. ((2015) ). Processing past tense in the left cerebellum. Neurocase, 21: (2), 185–189. https://doi.org/10.1080/13554794.2013.878730 |
144 | Marangolo, P. , Fiori, V. , Calpagnano, M. A. , Campana, S. , Razzano, C. , Caltagirone, C. , Marini, A. ((2013) ). TDCS over the left inferior frontal cortex improves speech production in aphasia. Frontiers in Human Neuroscience, 7: , 539. https://doi.org/10.3389/fnhum.2013.00539 |
145 | Marangolo, P. , Fiori, V. , Caltagirone, C. , Pisano, F. , Priori, A. ((2018) ). Transcranial cerebellar direct current stimulation enhances verb generation but not verb naming in poststroke aphasia. Journal of Cognitive Neuroscience, 30: (2), 188–199. https://doi.org/10.1162/jocn_a_01201 |
146 | Mariën, P. , Ackermann, H. , Adamaszek, M. , Barwood, C. H. S. , Beaton, A. , Desmond, J. , De Witte, E. , Fawcett, A. J. , Hertrich, I. , Küper, M. , Leggio, M. , Marvel, C. , Molinari, M. , Murdoch, B. E. , Nicolson, R. I. , Schmahmann, J. D. , Stoodley, C. J. , Thürling, M. , Timmann, D. , Ziegler, W. ((2014) ). Consensus paper: Language and the cerebellum: an ongoing enigma. Cerebellum (London, England), 13: (3), 386–410. https://doi.org/10.1007/s12311-013-0540-5 |
147 | Martin, N. , Kohen, F. , Kalinyak-Fliszar, M. , Soveri, A. , Laine, M. ((2012) ). Effects of working memory load on processing of sounds and meanings of words in aphasia. Aphasiology, 26: (3–4), 462–493. https://doi.org/10.1080/02687038.2011.619516 |
148 | Martin, N. , Minkina, I. , Kohen, F. P. , Kalinyak-Fliszar, M. ((2018) ). Assessment of linguistic and verbal short-term memory components of language abilities in aphasia. Journal of Neurolinguistics, 48: , 199–225. https://doi.org/10.1016/j.jneuroling.2018.02.006 |
149 | Martin, N. , Schlesinger, J. , Obermeyer, J. , Minkina, I. , Rosenberg, S. ((2021) ). Treatment of verbal short-term memory abilities to improve language function in aphasia: A case series treatment study. Neuropsychological Rehabilitation, 31: (5), 731–772. https://doi.org/10.1080/09602011.2020.1731554 |
150 | Martin, P. , Naeser, M. , Theoret, H. , Tormos, J. M. , Nicholas, M. , Kurland, J. , Fregni, F. , Seekins, H. , Doron, K. , Pascual-Leone, A. ((2004) ). Transcranial magnetic stimulation as a complementary treatment for aphasia. Seminars in Speech and Language, 25: (02), 181–191. https://doi.org/10.1055/s-2004-825654 |
151 | Matar, S. J. , Newton, C. , Sorinola, I. O. , Pavlou, M. ((2022) ). Transcranial direct-current stimulation as an adjunct to verb network strengthening treatment in post-stroke chronic aphasia: A double-blinded randomized feasibility study. Frontiers in Neurology, 13: , 722402.https://www.frontiersin.org/articles/10.3389/fneur.2022.722402 |
152 | Medina, J. , Norise, C. , Faseyitan, O. , Coslett, H. B. , Turkeltaub, P. E. , Hamilton, R. H. ((2012) ). Finding the right words: Transcranial magnetic stimulation improves discourse productivity in non-fluent aphasia after stroke. Aphasiology, 26: (9), 1153–1168. https://doi.org/10.1080/02687038.2012.710316 |
153 | Megumi, F. , Yamashita, A. , Kawato, M. , Imamizu, H. ((2015) ). Functional MRI neurofeedback training on connectivity between two regions induces long-lasting changes in intrinsic functional network. Frontiers in Human Neuroscience, 9: , 160. https://doi.org/10.3389/fnhum.2015.00160 |
154 | Meinzer, M. , Darkow, R. , Lindenberg, R. , Flöel, A. ((2016) ). Electrical stimulation of the motor cortex enhances treatment outcome in post-stroke aphasia. Brain, 139: (4), 1152–1163. https://doi.org/10.1093/brain/aww002 |
155 | Meinzer, M. , Mohammadi, S. , Kugel, H. , Schiffbauer, H. , Flöel, A. , Albers, J. , Kramer, K. , Menke, R. , Baumgärtner, A. , Knecht, S. , Breitenstein, C. , Deppe, M. ((2010) ). Integrity of the hippocampus and surrounding white matter is correlated with language training success in aphasia. NeuroImage, 53: (1), 283–290. https://doi.org/10.1016/j.neuroimage.2010.06.004 |
156 | Mendonca, M. E. , Santana, M. B. , Baptista, A. F. , Datta, A. , Bikson, M. , Fregni, F. , Araujo, C. P. ((2011) ). Transcranial DC stimulation in fibromyalgia: Optimized cortical target supported by high-resolution computational models. The Journal of Pain, 12: (5), 610–617. https://doi.org/10.1016/j.jpain.2010.12.015 |
157 | Milman, L. ((2016) ). An integrated approach for treating discourse in aphasia bridging the gap between language impairment and functional communication. Topics in Language Disorders, 36: (1), 80–96. https://doi.org/10.1097/TLD.0000000000000076 |
158 | Mohr, B. , Difrancesco, S. , Harrington, K. , Evans, S. , Pulvermüller, F. ((2014) ). Changes of right-hemispheric activation after constraint-induced, intensive language action therapy in chronic aphasia: FMRI evidence from auditory semantic processing. Frontiers in Human Neuroscience, 8: , 919. https://www.frontiersin.org/articles/10.3389/fnhum.2014.00919. |
159 | Moisa, M. , Pohmann, R. , Uludag, K. , Thielscher, A. ((2010) ). Interleaved TMS/CASL: Comparison of different rTMS protocols. NeuroImage, 49: (1), 612–620. https://doi.org/10.1016/j.neuroimage.2009.07.010 |
160 | Monti, A. , Cogiamanian, F. , Marceglia, S. , Ferrucci, R. , Mameli, F. , Mrakic-Sposta, S. , Vergari, M. , Zago, S. , Priori, A. ((2008) ). Improved naming after transcranial direct current stimulation inaphasia. Journal of Neurology, Neurosurgery & Psychiatry, 79: (4), 451–453. https://doi.org/10.1136/jnn2007.135277 |
161 | Murray, L. L. ((2012) ). Attention and other cognitive deficits inaphasia: Presence and relation to language and communicationmeasures. American Journal of Speech-Language Pathology, 21: (2), S51–S64. https://doi.org/10.1044/1058-0360(2012/11-0067) |
162 | Nadeau, S. E. ((2001) ). Phonology: A review and proposals from a connectionist perspective. Brain and Language, 79: (3), 511–579. https://doi.org/10.1006/brln.2001.2566 |
163 | Naeser, M. A. , Martin, P. I. , Nicholas, M. , Baker, E. H. , Seekins, H. , Kobayashi, M. , Theoret, H. , Fregni, F. , Maria-Tormos, J. , Kurland, J. , Doron, K. W. , Pascual-Leone, A. ((2005) ). Improved picture naming in chronic aphasia after TMS to part of right Broca’s area: An open-protocol study. Brain and Language, 93: (1), 95–105. https://doi.org/10.1016/j.bandl.2004.08.004 |
164 | Nair, V. A. , Young, B. M. , La, C. , Reiter, P. , Nadkarni, T. N. , Song, J. , Vergun, S. , Addepally, N. S. , Mylavarapu, K. , Swartz, J. L. , Jensen, M. B. , Chacon, M. R. , Sattin, J. A. , Prabhakaran, V. ((2015) ). Functional connectivity changes in the language network during stroke recovery. Annals of Clinical and Translational Neurology, 2: (2), 185–195. https://doi.org/10.1002/acn3.165 |
165 | Neuling, T. , Wagner, S. , Wolters, C. , Zaehle, T. , Herrmann, C. ((2012) ). Finite-element model predicts current density distribution for clinical applications of tDCS and tACS. Frontiers in Psychiatry, 3: , 83. https://www.frontiersin.org/articles/10.3389/fpsyt.2012.00083 |
166 | Nickels, K. , Beeson, P. M. , Rising, K. , Jebahi, F. , Kielar, A. ((2023) ). Positive changes to written language following phonological treatment in logopenic variant primary progressive aphasia: Case report. Frontiers in Human Neuroscience, 16: , 1006350. https://doi.org/10.3389/fnhum.2022.1006350 |
167 | Nikravesh, M. , Aghajanzadeh, M. , Maroufizadeh, S. , Saffarian, A. , Jafari, Z. ((2021) ). Working memory training in post-stroke aphasia: Near and far transfer effects. Journal of Communication Disorders, 89: , 106077. https://doi.org/10.1016/j.jcomdis.2020.106077 |
168 | Nitsche, M. A. , Paulus, W. ((2001) ). Sustained excitability elevations induced by transcranial DC motor cortex stimulation in humans. Neurology, 57: (10), 1899–1901. https://doi.org/10.1212/wnl.57.10.1899 |
169 | Norise, C. , Hamilton, R. H. ((2016) ). Non-invasive brain stimulation in the treatment of post-stroke and neurodegenerative aphasia: Parallels, differences, and lessons learned. Frontiers in Human Neuroscience, 10: , 675. https://doi.org/10.3389/fnhum.2016.00675 |
170 | Norise, C. , Sacchetti, D. , Hamilton, R. ((2017) ). Transcranial direct current stimulation in post-stroke chronic aphasia: The impact of baseline severity and task specificity in a pilot sample. Frontiers in Human Neuroscience, 11: , 260. https://www.frontiersin.org/articles/10.3389/fnhum.2017.00260 |
171 | Nozari, N. , Woodard, K. , Thompson-Schill, S. L. ((2014) ). Consequences of cathodal stimulation for behavior: When does it help and when does it hurt performance? PLoS ONE, 9: (1), e84338. https://doi.org/10.1371/journal.pone.0084338 |
172 | Oh, S. J. , Eom, B. , Park, C. , Sung, J. E. , Oh, S. J. , Eom, B. , Park, C. , Sung, J. E. ((2016) ). Treatment efficacy of semantic feature analyses for persons with aphasia: Evidence from meta-analyses. Communication Sciences & Disorders, 21: (2), 310–323. https://doi.org/10.12963/csd.16312 |
173 | Otal, B. , Olma, M. C. , Flöel, A. , Wellwood, I. ((2015) ). Inhibitory non-invasive brain stimulation to homologous language regions as an adjunct to speech and language therapy in post-stroke aphasia: A meta-analysis. Frontiers in Human Neuroscience, 9: , 236. https://www.frontiersin.org/articles/10.3389/fnhum.2015.00236 |
174 | Peach, R. K. , Beck, K. M. , Gorman, M. , Fisher, C. ((2019) a). Clinical outcomes following language-specific attention treatment versus direct attention training for aphasia: A comparative effectiveness study. Journal of Speech, Language & Hearing Research, 62: (8), 2785–2811. https://doi.org/10.1044/2019_JSLHR-L-18-0504 |
175 | Peach, R. K. , Beck, K. M. , Gorman, M. , Fisher, C. (2019b). Language-specific attention treatment for aphasia. ASHA Journals Journal Contribution. https://doi.org/10.23641/asha.8986427.v1 |
176 | Pedersen, P. M. , Vinter, K. , Olsen, T. S. ((2004) ). Aphasia after stroke: Type, severity and prognosis: The Copenhagen aphasia study. Cerebrovascular Diseases, 17: (1), 35–43. https://doi.org/10.1159/000073896 |
177 | Perani, D. , Cappa, S. F. , Tettamanti, M. , Rosa, M. , Scifo, P. , Miozzo, A. , Basso, A. , Fazio, F. ((2003) ). A fMRI study of word retrieval in aphasia. Brain and Language, 85: (3), 357–368. https://doi.org/10.1016/s0093-934x(02)00561-8 |
178 | Perceval, G. , Martin, A. K. , Copland, D. A. , Laine, M. , Meinzer, M. ((2017) ). High-definition tDCS of the temporo-parietal cortex enhances access to newly learned words. Scientific Reports, 7: (1), 17023. https://doi.org/10.1038/s41598-017-17279-0 |
179 | Pindi, P. , Houenou, J. , Piguet, C. , Favre, P. ((2022) ). Real-time fMRI neurofeedback as a new treatment for psychiatric disorders: A meta-analysis. Progress in NeuroPsychopharmacology and Biological Psychiatry, 119: , 110605. https://doi.org/10.1016/j.pnpb2022.110605 |
180 | Pisano, F. , Manfredini, A. , Castellano, A. , Caltagirone, C. , Marangolo, P. ((2022) ). Does executive function training impact on communication? A randomized controlled tDCS study on post-stroke aphasia. Brain Sciences, 12: (9), 1265. https://doi.org/10.3390/brainsci12091265 |
181 | Pope, P. A. , Miall, R. C. ((2012) ). Task-specific facilitation of cognition by cathodal transcranial direct current stimulation of the cerebellum. Brain Stimulation, 5: (2), 84–94. https://doi.org/10.1016/j.brs.2012.03.006 |
182 | Pope, P. , Miall, R. ((2014) ). Restoring cognitive functions using non-invasive brain stimulation techniques in patients with cerebellar disorders. Frontiers in Psychiatry, 5: , 33. https://www.frontiersin.org/articles/10.3389/fpsyt.2014.00033 |
183 | Price, A. R. , Peelle, J. E. , Bonner, M. F. , Grossman, M. , Hamilton, R. H. ((2016) ). Causal evidence for a mechanism of semantic integration in the angular gyrus as revealed by high-definition transcranial direct current stimulation. The Journal of Neuroscience: The Official Journal of the Society for Neuroscience, 36: (13), 3829–3838. https://doi.org/10.1523/JNEUROSCI.3120-15.2016 |
184 | Price, C. J. , Crinion, J. ((2005) ). The latest on functional imaging studies of aphasic stroke. Current Opinion in Neurology, 18: (4), 429–434. https://doi.org/10.1097/01.wco.0000168081.76859.c1 |
185 | Pulvermüller, F. ((2005) ). Brain mechanisms linking language and action. Nature Reviews. Neuroscience, 6: (7), 576–582. https://doi.org/10.1038/nrn1706 |
186 | Purdy, M. ((2002) ). Executive function ability in persons with aphasia. Aphasiology, 16: (4–6), 549–557. https://doi.org/10.1080/02687030244000176 |
187 | Quique, Y. M. , Evans, W. S. , Dickey, M. W. ((2019) ). Acquisition and generalization responses in aphasia naming treatment: A meta-analysis of semantic feature analysis outcomes. American Journal of Speech-LanguagePathology, 28: (1S), 230–246. https://doi.org/10.1044/2018_AJSLP-17-0155 |
188 | Ralph, M. A. L. , Snell, C. , Fillingham, J. K. , Conroy, P. , Sage, K. ((2010) ). Predicting the outcome of anomia therapy for people with aphasia post CVA: Both language and cognitive status are key predictors. Neuropsychological Rehabilitation, 20: (2), 289–305. https://doi.org/10.1080/09602010903237875 |
189 | Ramsberger, G. ((2005) ). Achieving conversational success in aphasia by focusing on non-linguistic cognitive skills: A potentially promising new approach. Aphasiology, 19: (10–11), 1066–1073. https://doi.org/10.1080/02687030544000254 |
190 | Raymer, A. , Kohen, F. ((2006) ). Word-retrieval treatment in aphasia: Effects of sentence context. The Journal of Rehabilitation Research and Development, 43: (3), 367. https://doi.org/10.1682/JRRD.2005.01.0028 |
191 | Renton, T. , Tibbles, A. , Topolovec-Vranic, J. ((2017) ). Neurofeedback as a form of cognitive rehabilitation therapyfollowing stroke: A systematic review. PloS One, 12: (5), e0177290. https://doi.org/10.1371/journal.pone.0177290 |
192 | Richardson, J. , Datta, A. , Dmochowski, J. , Parra, L. C. , Fridriksson, J. ((2015) ). Feasibility of using high-definitiontranscranial direct current stimulation (HD-tDCS) to enhancetreatment outcomes in persons with aphasia. NeuroRehabilitation,, 36: (1), 115–126. https://doi.org/10.3233/NRE-141199 |
193 | Robineau, F. , Saj, A. , Neveu, R. , Van De Ville, D. , Scharnowski, F. , Vuilleumier, P. ((2019) ). Using real-time fMRI neurofeedback to restore right occipital cortex activity in patients with left visuo-spatial neglect: Proof-of-principle and preliminary results. Neuropsychological Rehabilitation, 29: (3), 339–360. https://doi.org/10.1080/09602011.2017.1301262 |
194 | Roc, A. C. , Wang, J. , Ances, B. M. , Liebeskind, D. S. , Kasner, S. E. , Detre, J. A. ((2006) ). Altered hemodynamics and regional cerebral blood flow in patients with hemodynamically significant stenoses. Stroke, 37: (2), 382–387. https://doi.org/10.1161/01.STR.0000198807.31299.43 |
195 | Ros, T. , Enriquez-Geppert, S. , Zotev, V. , Young, K. D. , Wood, G. , Whitfield-Gabrieli, S. , Wan, F. , Vuilleumier, P. , Vialatte, F. , Van De Ville, D. , Todder, D. , Surmeli, T. , Sulzer, J. S. , Strehl, U. , Sterman, M. B. , Steiner, N. J. , Sorger, B. , Soekadar, S. R. , Sitaram, R. , Thibault, R. T. ((2020) ). Consensus on the reporting and experimental design of clinical and cognitive-behavioural neurofeedback studies (CRED-nf checklist). Brain: A Journal of Neurology, 143: (6), 1674–1685. https://doi.org/10.1093/brain/awaa009 |
196 | Rota, G. , Sitaram, R. , Veit, R. , Erb, M. , Weiskopf, N. , Dogil, G. , Birbaumer, N. ((2009) ). Self-regulation of regional cortical activity using real-time fMRI: The right inferior frontal gyrus and linguistic processing. Human Brain Mapping, 30: (5), 1605–1614. https://doi.org/10.1002/hbm.20621 |
197 | Roy, R. , de la Vega, R. , Jensen, M. P. , Miró, J. ((2020) ). Neurofeedback for Pain Management: A Systematic Review. Frontiers in Neuroscience, 14: , 671. https://doi.org/10.3389/fnins.2020.00671 |
198 | Rubi-Fessen, I. , Hartmann, A. , Huber, W. , Fimm, B. , Rommel, T. , Thiel, A. , Heiss, W. D. ((2015) ). Add-on effects of repetitive transcranial magnetic stimulation on subacute aphasia therapy: Enhanced improvement of functional communication and basic linguistic skills. A randomized controlled study. Archives of Physical Medicine and Rehabilitation, 96: (11), 2. https://doi.org/10.1016/j.apmr.2015.06.017 |
199 | Ruiz, S. , Buyukturkoglu, K. , Rana, M. , Birbaumer, N. , Sitaram, R. ((2014) ). Real-time fMRI brain computer interfaces: Self-regulation of single brain regions to networks. Biological Psychology, 95: , 4–20. https://doi.org/10.1016/j.biopsycho.2013.04.010 |
200 | Salis, C. ((2012) ). Short-term memory treatment: Patterns of learning and generalisation to sentence comprehension in a person with aphasia. Neuropsychological Rehabilitation, 22: (3), 428–448. https://doi.org/10.1080/09602011.2012.656460 |
201 | Salis, C. , Hwang, F. , Howard, D. , Lallini, N. ((2017) ). Short-term and working memory treatments for improving sentence comprehension in aphasia: A review and a replication study. Seminars in Speech and Language, 38: (01), 029–039. https://doi.org/10.1055/s-0036-1597262 |
202 | Sandberg, C. , Kiran, S. ((2014) ). How justice can affect jury: Training abstract words promotes generalisation to concrete words in patients with aphasia. Neuropsychological Rehabilitation, 24: (5), 738–769. https://doi.org/10.1080/09602011.2014.899504 |
203 | Sandberg, C. W. ((2017) ). Hypoconnectivity of resting-state networks in persons with aphasia compared with healthy age-matched adults. Frontiers in Human Neuroscience, 11: , 91. https://doi.org/10.3389/fnhum.2017.00091 |
204 | Saturnino, G. B. , Antunes, A. , Thielscher, A. ((2015) ). On the importance of electrode parameters for shaping electric field patterns generated by tDCS. NeuroImage, 120: , 25–35. https://doi.org/10.1016/j.neuroimage.2015.06.067 |
205 | Saur, D. , Lange, R. , Baumgaertner, A. , Schraknepper, V. , Willmes, K. , Rijntjes, M. , Weiller, C. ((2006) ). Dynamics of language reorganization after stroke. Brain, 129: (6), 1371–1384. https://doi.org/10.1093/brain/awl090 |
206 | Schmahmann, J. D. ((2019) ). The cerebellum and cognition. Neuroscience Letters, 688: , 62–75. https://doi.org/10.1016/j.neulet.2018.07.005 |
207 | Schumacher, R. , Halai, A. D. , Lambon Ralph, M. A. ((2022) ). Assessing executive functions in post-stroke aphasia— Utility of verbally based tests. Brain Communications, 4: (3), fcac107. https://doi.org/10.1093/braincomms/fcac107 |
208 | Sebastian, R. , Kim, J. H. , Brenowitz, R. , Tippett, D. C. , Desmond, J. E. , Celnik, P. A. , Hillis, A. E. ((2020) ). Cerebellar neuromodulation improves naming in post-stroke aphasia. Brain Communications, 2: (2), fcaa179. https://doi.org/10.1093/braincomms/fcaa179 |
209 | Sebastian, R. , Saxena, S. , Tsapkini, K. , Faria, A. V. , Long, C. , Wright, A. , Davis, C. , Tippett, D. C. , Mourdoukoutas, A. P. , Bikson, M. , Celnik, P. , Hillis, A. E. ((2017) ). Cerebellar tDCS: A novel approach to augment language treatment post-stroke. Frontiers in Human Neuroscience, 10: , 695. https://doi.org/10.3389/fnhum.2016.00695 |
210 | Senaha, M. L. H. , Martin, M. G. M. , Amaro, E. , Campi, C. , Caramelli, P. ((2005) ). Patterns of cerebral activation during lexical and phonological reading in Portuguese. Brazilian Journal of Medical and Biological Research=Revista Brasileira De Pesquisas Medicas E Biologicas, 38: (12), 1847–1856. https://doi.org/10.1590/s0100-879x2005001200013 |
211 | Seniów, J. , Litwin, M. , Leśniak, M. ((2009) ). The relationship between non-linguistic cognitive deficits and language recovery in patients with aphasia. Journal of the Neurological Sciences, 283: (1), 91–94. https://doi.org/10.1016/j.jns.2009.02.315 |
212 | Shah, P. , Szaflarski, J. , Allendorfer, J. , Hamilton, R. ((2013) ). Induction of neuroplasticity and recovery in post-stroke aphasia by non-invasive brain stimulation. Frontiers in Human Neuroscience, 7: , 888. https://www.frontiersin.org/article/10.3389/fnhum.2013.00888 |
213 | Shah-Basak, P. , Fernandez, A. , Armstrong, S. E. M. , Hodzic-Santor, B. H. , Lavoie, M. , Jokel, R. , Meltzer, J. A. ((2021) ). Behavioural and neurophysiological responses to written naming treatment and high definition tDCS: A case study in advanced primary progressive aphasia. Aphasiology, 36: (10), 1182–1205. https://doi.org/10.1080/02687038.2021.1959015 |
214 | Shah-Basak, P. , Norise, C. , Garcia, G. , Torres, J. , Faseyitan, O. , Hamilton, R. H. ((2015) ). Individualized treatment with transcranial direct current stimulation in patients with chronic non-fluent aphasia due to stroke. Frontiers in Human Neuroscience,, 9: , 201. https://doi.org/10.3389/fnhum.2015.00201 |
215 | Shah-Basak, P. P. , Wurzman, R. , Purcell, J. B. , Gervits, F. , Hamilton, R. ((2016) ). Fields or flows? A comparative metaanalysis of transcranial magnetic and direct current stimulation to treat post-stroke aphasia. Restorative Neurology and Neuroscience, 34: (4), 537–558. https://doi.org/10.3233/RNN-150616 |
216 | Shah-Basak, P. , Sivaratnam, G. , Teti, S. , Francois-Nienaber, A. , Yossofzai, M. , Armstrong, S. , Nayar, S. , Jokel, R. , Meltzer, J. ((2020) ). High definition transcranial direct current stimulation modulates abnormal neurophysiological activity in post-stroke aphasia. Scientific Reports, 10: (1), 19625. https://doi.org/10.1038/s41598-020-76533-0 |
217 | Siegel, J. S. , Ramsey, L. E. , Snyder, A. Z. , Metcalf, N. V. , Chacko, R. V. , Weinberger, K. , Baldassarre, A. , Hacker, C. D. , Shulman, G. L. , Corbetta, M. ((2016) ). Disruptions of network connectivity predict impairment in multiple behavioral domains after stroke. Proceedings of the National Academy of Sciences, 113: (30), E4367–E4376. https://doi.org/10.1073/pnas.1521083113 |
218 | Silkes, J. A. P. , Fergadiotis, G. , Hunting Pompon, R. , Torrence, J. , Kendall, D. L. ((2019) ). Effects of phonomotor treatment on discourse production. Aphasiology, 33: (2), 125–139. https://doi.org/10.1080/02687038.2018.1512080 |
219 | Simic, T. , Bitan, T. , Turner, G. , Chambers, C. , Goldberg, D. , Leonard, C. , Rochon, E. ((2020) ). The role of executive control in post-stroke aphasia treatment. Neuropsychological Rehabilitation, 30: (10), 1853–1892. https://doi.org/10.1080/09602011.2019.1611607 |
220 | Simic, T. , Laird, L. , Brisson, N. , Moretti, K. , Théorêt, J.-L. , Black, S. E. , Eskes, G. A. , Leonard, C. , Rochon, E. ((2022) ). Cognitive training to enhance aphasia therapy (Co-TrEAT): A feasibility study. Frontiers in Rehabilitation Sciences, 3: , 815780. https://www.frontiersin.org/articles/10.3389/fresc.2022.815780 |
221 | Simmons-Mackie, N. , Cherney, L. R. ((2018) ). Aphasia in North America: Frequency, demographics, impact, communication access, services and service gaps. Archives of Physical Medicine and Rehabilitation, 99: (10), e117. |
222 | Sitaram, R. , Ros, T. , Stoeckel, L. , Haller, S. , Scharnowski, F. , Lewis-Peacock, J. , Weiskopf, N. , Blefari, M. L. , Rana, M. , Oblak, E. , Birbaumer, N. , Sulzer, J. ((2017) ). Closed-loop brain training: The science of neurofeedback. https://doi.org/10.1038/nrn.2016.164, 18: (2), 86–100. https://doi.org/10.1038/nrn.2016.164 |
223 | Sitaram, R. , Veit, R. , Stevens, B. , Caria, A. , Gerloff, C. , Birbaumer, N. , Hummel, F. ((2012) ). Acquired control of ventral premotor cortex activity by feedback training: An exploratory real-time FMRI and TMS study. Neurorehabilitation and Neural Repair, 26: (3), 256–265. https://doi.org/10.1177/1545968311418345 |
224 | Sohlberg, M. M. , Mateer, C. (2010). Attention Process Training—Attention Process Training 3—Lash & Associates Publishing. https://lapublishing.com/apt3-attention-processtraining/ |
225 | Sorger, B. , Scharnowski, F. , Linden, D. E. J. , Hampson, M. , Young, K. D. ((2019) ). Control freaks: Towards optimal selection of control conditions for fMRI neurofeedback studies. Neuro Image, 186: , 256–265. https://doi.org/10.1016/j.neuroimage.2018.11.004 |
226 | Spielmann, K. , van de Sandt-Koenderman, W. M. E. , Heijenbrok-Kal, M. H. , Ribbers, G. M. ((2016) ). Transcranial direct current stimulation in post-stroke sub-acute aphasia: Study protocol for a randomized controlled trial. Trials, 17: , 380. https://doi.org/10.1186/s13063-016-1505-z |
227 | Spielmann, K. , van de Sandt-Koenderman, W. M. E. , Heijenbrok-Kal, M. H. , Ribbers, G. M. ((2018) ). Transcranial direct current stimulation does not improve language outcome in subacute poststroke aphasia. Stroke, 49: (4), 1018–1020. https://doi.org/10.1161/STROKEAHA.117.020197 |
228 | Spitzer, L. , Binkofski, F. , Willmes, K. , Bruehl, S. ((2021) ). The novel cognitive flexibility in aphasia therapy (CFAT): A combined treatment of aphasia and executive functions to improve communicative success. International Journal of Speech-Language Pathology, 23: (2), 168–179. https://doi.org/10.1080/17549507.2020.1757152 |
229 | Sreedharan, S. , Arun, K. M. , Sylaja, P. N. , Kesavadas, C. , Sitaram, R. ((2019) ). Functional connectivity of language regions of stroke patients with expressive aphasia during real-time functional magnetic resonance imaging based neurofeedback. Brain Connectivity, 9: (8), 613–626. https://doi.org/10.1089/brain.2019.0674 |
230 | Sreedharan, S. , Chandran, A. , Yanamala, V. R. , Sylaja, P. N. , Kesavadas, C. , Sitaram, R. ((2020) ). Self-regulation of languageareas using real-time functional MRI in stroke patients withexpressive aphasia. Brain Imaging and Behavior, 14: (5), 1714–1730. https://doi.org/10.1007/s11682-019-00106-7 |
231 | Stagg, C. J. , Antal, A. , Nitsche, M. A. ((2018) ). Physiology of transcranial direct current stimulation. The Journal of ECT,, 34: (3), 144–152. https://doi.org/10.1097/YCT.0000000000000510 |
232 | Stagg, C. J. , Best, J. G. , Stephenson, M. C. , O’Shea, J. , Wylezinska, M. , Kineses, Z. T. , Morris, P. G. , Matthews, P. M. , Johansen-Berg, H. ((2009) ). Polarity-sensitive modulation of cortical neurotransmitters by transcranial stimulation. Journal of Neuroscience, 29: (16), 5202–5206. https://doi.org/10.1523/JNEUROSCI.4432-08.2009 |
233 | Stagg, C. J. , Lin, R. L. , Mezue, M. , Segerdahl, A. , Kong, Y. , Xie, J. , Tracey, I. ((2013) ). Widespread modulation of cerebral perfusion induced during and after transcranial direct current stimulation applied to the left dorsolateral prefrontal cortex. Journal of Neuroscience, 33: (28), 11425–11431. https://doi.org/10.1523/JNEUROSCI.3887-12.2013 |
234 | Stockert, A. , Kummerer, D. , Saur, D. ((2016) ). Insights into early language recovery: From basic principles to practical applications. Aphasiology, 39: (5), 517–541. |
235 | Stoeckel, L. E. , Garrison, K. A. , Ghosh, S. , Wighton, P. , Hanlon, C. A. , Gilman, J. M. , Greer, S. , Turk-Browne, N. B. , deBettencourt, M. T. , Scheinost, D. , Craddock, C. , Thompson, T. , Calderon, V. , Bauer, C. C. , George, M. , Breiter, H. C. , Whitfield-Gabrieli, S. , Gabrieli, J. D. , LaConte, S. M. , Evins, A. E. ((2014) ). Optimizing real time fMRI neurofeedback for therapeutic discovery and development. NeuroImage. Clinical, 5: , 245–255. https://doi.org/10.1016/j.nicl.2014.07.002 |
236 | Stokes, T. F. , Baer, D. M. ((1977) ). An implicit technology of generalization1. Journal of Applied Behavior Analysis, 10: (2), 349–367. https://doi.org/10.1901/jaba.1977.10-349 |
237 | Subramanian, L. , Skottnik, L. , Cox, W. M. , Lührs, M. , McNamara, R. , Hood, K. , Watson, G. , Whittaker, J. R. , Williams, A. N. , Sakhuja, R. , Ihssen, N. , Goebel, R. , Playle, R. , Linden, D. E. J. ((2021) ). Neurofeedback training versus treatment-as-usual for alcohol dependence: Results of an early-phase randomized controlled trial and neuroimaging correlates. European Addiction Research, 27: (5), 381–394. https://doi.org/10.1159/000513448 |
238 | Swiderski, A. M. , Quique, Y. M. , Dickey, M. W. , Hula, W. D. ((2021) ). Treatment of Underlying Forms: A Bayesian Meta-Analysis of the Effects of Treatment and Person-Related Variables on Treatment Response. Journal of Speech, Language, and Hearing Research: JSLHR, 64: (11), 4308–4328. https://doi.org/10.1044/2021_JSLHR-21-00131 |
239 | Szaflarski, J. P. , Griffis, J. , Vannest, J. , Allendorfer, J. B. , Nenert, R. , Amara, A. W. , Sung, V. , Walker, H. C. , Martin, A. N. , Mark, V. W. , Zhou, X. ((2018) ). A feasibility study of combined intermittent theta burst stimulation and modified constraint-induced aphasia therapy in chronic post-stroke aphasia. Restorative Neurology and Neuroscience, 36: (4), 503–518. https://doi.org/10.3233/RNN-180812 |
240 | Szaflarski, J. P. , Vannest, J. , Wu, S. W. , DiFrancesco, M. W. , Banks, C. , Gilbert, D. L. ((2011) ). Excitatory repetitive transcranial magnetic stimulation induces improvements in chronic post-stroke aphasia. Medical Science Monitor: International Medical Journal of Experimental and Clinical Research, 17: (3), CR132–CR139. https://doi.org/10.12659/msm.881446 |
241 | Taschereau-Dumouchel, V. , Cortese, A. , Chiba, T. , Knotts, J. D. , Kawato, M. , Lau, H. ((2018) ). Towards an unconscious neural reinforcement intervention for common fears. Proceedings of the National Academy of Sciences, 115: (13), 3470–3475. https://doi.org/10.1073/pnas.1721572115 |
242 | Taylor, J. E. , Yamada, T. , Kawashima, T. , Kobayashi, Y. , Yoshihara, Y. , Miyata, J. , Murai, T. , Kawato, M. , Motegi, T. ((2022) ). Depressive symptoms reduce when dorsolateral prefrontal cortex-precuneus connectivity normalizes after functional connectivity neurofeedback. Scientific Reports, 12: (1), 2581. https://doi.org/10.1038/s41598-022-05860-1 |
243 | Thibault, R. T. , MacPherson, A. , Lifshitz, M. , Roth, R. R. , Raz, A. ((2018) ). Neurofeedback with fMRI: A critical systematic review. NeuroImage,, 172: , 786–807. https://doi.org/10.1016/j.neuroimage.2017.12.071 |
244 | Thiel, A. , Hartmann, A. , Rubi-Fessen, I. , Anglade, C. , Kracht, L. , Weiduschat, N. , Kessler, J. , Rommel, T. , Heiss, W.-D. ((2013) ). Effects of noninvasive brain stimulation on language networks and recovery in early poststroke aphasia. Stroke, 44: (8), 2240–2246. https://doi.org/10.1161/STROKEAHA.111.000574 |
245 | Thompson, C. K. ((2007) ). Complexity in language learning and treatment. American Journal of Speech-Language Pathology, 16: (1), 3–5. https://doi.org/10.1044/1058-0360(2007/002) |
246 | Thompson, C. K. , den Ouden, D. -B. ((2008) ). Neuroimaging and recovery of language in aphasia. Current Neurology and Neuroscience Reports, 8: (6), 1–14. https://doi.org/10.1097/MPG.0b013e3181a15ae8.Screening |
247 | Thompson, C. K. , Shapiro, L. P. ((2007) ). Complexity in treatmentof syntactic deficits. American Journal of Speech-Language Pathology / American Speech-Language-Hearing Association,, 16: (1), 30–42. https://doi.org/10.1044/1058-0360(2007/005) |
248 | Thompson, C. K. , Shapiro, L. P. , Kiran, S. , Sobecks, J. ((2003) ). The role of syntactic complexity in treatment of sentence deficits in agrammatic aphasia: The complexity account of treatment efficacy (CATE). Journal of Speech, Language, and Hearing Research: JSLHR, 46: (3), 591–607. |
249 | Thompson, C. K. , Walenski, M. , Chen, Y. , Caplan, D. , Kiran, S. , Rapp, B. , Grunewald, K. , Nunez, M. , Zinbarg, R. , Parrish, T. B. ((2017) ). Intrahemispheric perfusion in chronic stroke-induced aphasia. Neural Plasticity, 236169: , 1–15. https://doi.org/10.1155/2017/2361691 |
250 | Treger, I. , Luzki, L. , Gil, M. , Ring, H. ((2007) ). Transcranial Doppler monitoring during language tasks in stroke patients with aphasia. Disability and Rehabilitation, 29: (15), 1177–1183. https://doi.org/10.1080/09638280600999493 |
251 | Tsai, P.-Y. , Wang, C.-P. , Ko, J. S. , Chung, Y.-M. , Chang, Y.-W. , Wang, J.-X. ((2014) ). The persistent and broadly modulating effect of inhibitory rTMS in nonfluent aphasic patients: A sham-controlled, double-blind study. Neurorehabilitation and Neural Repair, 28: (8), 779–787. https://doi.org/10.1177/1545968314522710 |
252 | Turkeltaub, P. E. ((2015) ). Brain stimulation and the role of the right hemisphere in aphasia recovery. Current Neurology and Neuroscience Reports, 15: (11), 72. https://doi.org/10.1007/s11910-015-0593-6 |
253 | Turkeltaub, P. E. , Coslett, H. B. , Thomas, A. L. , Faseyitan, O. , Benson, J. , Norise, C. , Hamilton, R. H. ((2012) ). The right hemisphere is not unitary in its role in aphasia recovery. Cortex, 48: (9), 1179–1186. https://doi.org/10.1016/j.cortex.2011.06.010 |
254 | Turkeltaub, P. E. , Messing, S. , Norise, C. , Hamilton, R. H. ((2011) ). Are networks for residual language function and recovery consistent across aphasic patients? Neurology, 76: (20), 1726–1734. https://doi.org/10.1212/WNL.0b013e31821a44c1 |
255 | Turkeltaub, P. E. , Swears, M. K. , D’Mello, A. M. , Stoodley, C. J. ((2016) ). Cerebellar tDCS as a novel treatment for aphasia? Evidence from behavioral and resting-state functional connectivity data in healthy adults. Restorative Neurology and Neuroscience, 34: (4), 491–505. https://doi.org/10.3233/RNN-150633 |
256 | Ulm, L. , McMahon, K. , Copland, D. , de Zubicaray, G. I. , Meinzer, M. ((2015) ). Neural mechanisms underlying perilesional transcranial direct current stimulation in aphasia: A feasibility study. Frontiers in Human Neuroscience, 9: , 550. https://doi.org/10.3389/fnhum.2015.00550 |
257 | Vallila-Rohter, S. , Kiran, S. ((2013) ). Non-linguistic learning and aphasia: Evidence from a paired associate and feedback-based task. Neuropsychologia, 51: (1), 79–90. https://doi.org/10.1016/j.neuropsychologia.2012.10.024 |
258 | van der Cruijsen, J. , Piastra, M. C. , Selles, R. W. , Oostendorp, T. F. ((2021) ). A method to experimentally estimate the conductivity of chronic stroke lesions: A tool to individualize transcranial electric stimulation. Frontiers in Human Neuroscience, 15: , 738200. https://www.frontiersin.org/articles/10.3389/fnhum.2021.738200 |
259 | Villamar, M. F. , Volz, M. S. , Bikson, M. , Datta, A. , Dasilva, A. F. , Fregni, F. ((2013) ). Technique and considerations in the use of 4x1 ring high-definition transcranial direct current stimulation (HD-tDCS). Journal of Visualized Experiments: JoVE, 77: , e50309. https://doi.org/10.3791/50309 |
260 | Vukovic, N. , Hansen, B. , Lund, T. E. , Jespersen, S. , Shtyrov, Y. ((2021) ). Rapid microstructural plasticity in the cortical semantic network following a short language learning session. PLOS Biology, 19: (6), e3001290. https://doi.org/10.1371/journal.pbio.3001290 |
261 | Walenski, M. , Chen, Y. , Litcofsky, K. A. , Caplan, D. , Kiran, S. , Rapp, B. , Parrish, T. B. , Thompson, C. K. ((2022) ). Perilesional perfusion in chronic stroke-induced aphasia and its response to behavioral treatment interventions. Neurobiology of Language, 3: (2), 345–363. https://doi.org/10.1162/nol_a_00068 |
262 | Wang, C. -P. , Hsieh, C. -Y. , Tsai, P. -Y. , Wang, C. -T. , Lin, F. -G. , Chan, R. -C. ((2014) ). Efficacy of synchronous verbal training during repetitive transcranial magnetic stimulation in patients with chronic aphasia. Stroke, 45: (12), 3656–3662. https://doi.org/10.1161/STROKEAHA.114.007058 |
263 | Wang, T. , Mantini, D. , Gillebert, C. R. ((2018) ). The potential of real-time fMRI neurofeedback for stroke rehabilitation: A systematic review. Cortex, 107: , 148–165. https://doi.org/10.1016/j.cortex.2017.09.006 |
264 | Wang, Z. , Tamaki, M. , Frank, S. M. , Shibata, K. , Worden, M. S. , Yamada, T. , Kawato, M. , Sasaki, Y. , Watanabe, T. ((2021) ). Visual perceptual learning of a primitive feature in human V1/V2 as a result of unconscious processing, revealed by decoded functional MRI neurofeedback (DecNef). Journal of Vision, 21: (8), 24. https://doi.org/10.1167/jov.21.8.24 |
265 | Watanabe, T. , Sasaki, Y. , Shibata, K. , Kawato, M. ((2017) ). Advances in fMRI real-time neurofeedback. Trends in Cognitive Sciences, 21: (12), 997–1010. https://doi.org/10.1016/j.tics.2017.09.010 |
266 | Webster, J. , Whitworth, A. , Morris, J. ((2015) a). Is it time to stop “fishing”? A review of generalisation following aphasia intervention. Aphasiology, 29: (11), 1240–1264. https://doi.org/10.1080/02687038.2015.1027169 |
267 | Webster, J. , Whitworth, A. , Morris, J. ((2015) b). Is it time to stop “fishing”? A review of generalisation following aphasia intervention. Aphasiology, 29: (11), 1240–1264. https://doi.org/10.1080/02687038.2015.1027169 |
268 | Whitworth, A. , Leitão, S. , Cartwright, J. , Webster, J. , Hankey, G. J. , Zach, J. , Howard, D. , Wolz, V. ((2015) ). NARNIA: A new twist to an old tale. A pilot RCT to evaluate a multilevel approach to improving discourse in aphasia. Aphasiology, 29: (11), 1345–1382. https://doi.org/10.1080/02687038.2015.1081143 |
269 | Wilson, S. M. , Schneck, S. M. ((2021) ). Neuroplasticity in post-stroke aphasia: A systematic review and meta-analysis of functional imaging studies of reorganization of language processing. Neurobiology of Language, 2: (1), 22–82. https://doi.org/10.1515/9781614518679-006 |
270 | Winhuisen, L. , Thiel, A. , Schumacher, B. , Kessler, J. , Rudolf, J. , Haupt, W. F. , Heiss, W. D. ((2005) ). Role of the contralateralinferior frontal gyrus in recovery of language function inpoststroke aphasia: A combined repetitive transcranial magneticstimulation and positron emission tomography study. Stroke, 36: (8), 1759–1763. https://doi.org/10.1161/01.STR.0000174487.81126.ef |
271 | Xu, S. , Yang, Q. , Chen, M. , Deng, P. , Zhuang, R. , Sun, Z. , Li, C. , Yan, Z. , Zhang, Y. , Jia, J. ((2021) ). Capturing neuroplastic changes after iTBS in patients with post-stroke aphasia: A pilot fMRI study. Brain Sciences, 11: (11), 1451. https://doi.org/10.3390/brainsci11111451 |
272 | Xue, H. , Zhao, L. , Wang, Y. , Dong, Q. , Chen, C. , Xue, G. ((2017) ). Anodal transcranial direct current stimulation over the left temporoparietal cortex facilitates assembled phonology. Trends in Neuroscience and Education, 8–9: , 10–17. https://doi.org/10.1016/j.tine.2017.08.001 |
273 | You, D. S. , Kim, D.-Y. , Chun, M. H. , Jung, S. E. , Park, S. J. ((2011) ). Cathodal transcranial direct current stimulation of the right Wernicke’s area improves comprehension in subacute stroke patients. Brain and Language, 119: (1), 1–5. https://doi.org/10.1016/j.bandl.2011.05.002 |
274 | Young, K. D. , Siegle, G. J. , Zotev, V. , Phillips, R. , Misaki, M. , Yuan, H. , Drevets, W. C. , Bodurka, J. ((2017) ). Randomized clinical trial of real-time fMRI amygdala neurofeedback for major depressive disorder: Effects on symptoms and autobiographical memory recall. The American Journal of Psychiatry, 174: (8), 748–755. https://doi.org/10.1176/appi.aj2017.16060637 |
275 | Zakariás, L. , Kelly, H. , Salis, C. , Code, C. ((2019) ). The methodological quality of short-term/working memory treatments in poststroke aphasia: A systematic review. Journal of Speech, Language & Hearing Research, 62: (6), 1979–2001. https://doi.org/10.1044/2018_JSLHR-L-18-0057 |
276 | Zakariás, L. , Keresztes, A. , Marton, K. , Wartenburger, I. ((2018) ). Positive effects of a computerised working memory and executive function training on sentence comprehension in aphasia. Neuropsychological Rehabilitation, 28: (3), 369–386. https://doi.org/10.1080/09602011.2016.1159579 |
277 | Zakariás, L. , Salis, C. , Wartenburger, I. ((2018) ). Transfer effects on spoken sentence comprehension and functional communication after working memory training in stroke aphasia. Journal of Neurolinguistics, 48: , 47–63. https://doi.org/10.1016/j.jneuroling.2017.12.002 |
278 | Zheng, X. , Alsop, D. C. , Schlaug, G. ((2011) ). Effects of transcranial direct current stimulation (tDCS) on human regional cerebral blood flow. NeuroImage, 58: (1), 26–33. https://doi.org/10.1016/j.neuroimage.2011.06.018 |