Omega-3 long-chain polyunsaturated fatty acids and their bioactive lipids: A strategy to improve resistance to respiratory tract infectious diseases in the elderly?
Abstract
Age-related changes in organ function, immune dysregulation, and the effects of senescence explain in large part the high prevalence of infections, including respiratory tract infections in older persons. Poor nutritional status in many older persons increases susceptibility to infection and worsens prognosis. Interestingly, there is an association between the amount of saturated fats in the diet and the rate of community-acquired pneumonia. Polyunsaturated fatty acids, particularly omega-3 long chain polyunsaturated fatty acids (ω-3 LC-PUFAs) including eicosapentaenoic acid (EPA) and docosahexaenoic acid (DHA), have well-known anti-inflammatory, immunomodulatory, and antimicrobial effects, which may, in theory, be largely induced by PUFAs-derived lipids such as specialized pro-resolving mediators (SPMs). In adults, preliminary results of studies show that ω-3 LC-PUFAs supplementation can lead to SPM generation. SPMs have a crucial role in the resolution of inflammation, a factor relevant to survival from infection independent of the pathogen’s virulence. Moreover, the immune system of older adults appears to be more sensitive to ω-3 PUFAs. This review explores the effects of ω-3 LC-PUFAs, and PUFA bioactive lipid-derived SPMs in respiratory tract infections and the possible relevance of these data to infectious disease outcomes in the older population. The hypothesis that PUFAs have beneficial effects via SPM generation will need to be confirmed by animal experiments and patient-derived data.
1Introduction
Excess mortality and morbidity secondary to respiratory tract infections (RTIs) are mainly observed in persons aged 65 years and older [1, 2]. In Europe, influenza is the disease with the highest burden (81.8 disability-adjusted life years per 100,000 population), followed fourth place by invasive pneumococcal disease (53.5 disability-adjusted life years per 100,000 population) [1]. Of 13 368 cases in the European dataset of patients admitted to intensive care units with influenza, 46 % were 60 years and older, of which 25,6% were fatal [3]. These two diseases were among the top 10 leading causes of death in the United States in 2020 [4]. Multiple interrelated factors explain the greater susceptibility to infectious diseases with age. For example, swallowing disorder, a clinical condition prevalent among older persons, represents a risk factor for RTIs [5]. Older adults are not only exposed to a higher rate of RTIs but also to more adverse outcomes after community-acquired pneumonia, such as cardiovascular events [6]. In addition, the strength of the respiratory muscles and chest-wall compliance gradually decrease with aging resulting in reduced ventilatory response in case of infection [7]. Thereby, patients aged 80 years and older have been disproportionally affected by coronavirus disease 2019 (COVID-19) [8]. Moreover, disease severity and progression to acute respiratory distress syndrome in COVID-19 could be predicted by levels of pro-inflammatory cytokines and chemokines, particularly in older people [9, 10]. RTIs in older adults thus represent an important healthcare burden and new impetus to developing therapeutic strategies is needed.
This review explores the effects of ω-3 LC-PUFAs, and SPMs in RTIs, highlights some promising results of pre-clinical studies using various pathogens and the possible relevance of these data to infectious disease outcomes in the older population.
2Older subjects and nutritional status
There is considerable heterogeneity among available publications in this field, and the reported prevalence of malnutrition in nursing homes or long-term and rehabilitation care facilities varies between 17.5 % to 29.4% [11]. The interplay of numerous factors, including sensory (e.g., decreased senses of smell and taste), functional (e.g., dental problems, higher level of dependency), socio-economic, psychological (e.g., depression), and endogenous (e.g., increased pro-inflammatory cytokines, impaired production of peptides and hormones regulating appetite), contributes to lower nutritional intake [12–18]. In addition, a low intake of nutrient-dense foods leads to nutritional deficiencies associated with an impaired immune response [19, 20]. Moreover, nutritional status can be altered if the diet is unbalanced, even if the caloric intake is sufficient or even high.
Dietary lipids are fundamental, energy-providing nutrients, structural components of cells that significantly impact immune cell function [21]. In this context, a regular diet high in saturated-fats led to an impaired capacity to resist acute induced infections in mice [22]. In humans, a relationship between fatty acid consummation and RTIs has been observed in two cohorts. First, in a cohort of 83,165 women in the Nurses’ Health Study II, subjects with the highest quintile of palmitic acid intake (saturated fatty acid) had a greater risk of community-acquired pneumonia than those in the lowest quintile [23]. Second, in 38,378 male U.S. health professionals, Merchand et al. noted that those with the highest quintile intake of linoleic and alpha-linolenic acid (polyunsaturated fatty acids ω-6 et -3 respectively), present in vegetable oils, had fewer cases of pneumonia than those in the lowest quintile [24]. Despite these considerations, the average daily intake of ω-3 PUFAs in nursing-home residents is 100 mg, which is below recommended values [25, 26].
3Older subjects and immunity
Various changes in innate and adaptive immunity with age enhance the risk of infection in animals and humans [27]. Altered production of cytokines and reactive nitrogen species upon stimulation of monocyte-derived macrophages and alveolar macrophages from aged subjects weakens the capacity to kill some pathogens, such as Streptococcus pneumoniae (S. pneumoniae) [28, 29]. A reduced population of naïve T-cells because of slower output from the bone marrow and involution of the thymus also limits the antibody response to foreign antigens [30, 31]. Fewer antigen-specific B cells associated with impaired CD4 + T cell responses strongly reduced antibody titers in the elderly during vaccine administration [32]. Moreover, the expression of bacterial ligands of S. pneumoniae, the leading cause of death from RTIs, increases in lung senescent cells, particularly in older adults [33, 34]. Age-associated inflammation also primes the lungs for infection through an increased surface expression of proteins regulated by NF-kB and Toll-like receptor (TLR) dysfunction [35]. Even never-smoking healthy older individuals showed low-grade inflammation in bronchoalveolar lavage fluid (BALF) as illustrated by increased concentrations of interleukin (IL)-8 and expression of CD+4 T cell activation markers [36, 37]. Crucially, survival after infection will depend not only on the pathogen’s virulence and subsequently on the extent of the host’s immune response, but also on the ability of that response to be self-limited [38, 39]. However, a decreased anti-inflammatory cytokine IL-10 response in the lungs of aged rats exposed to a carrageenan challenge (a proinflammatory polysaccharide used to induce inflammation) negatively impacted the resolution of inflammation, resulting in increased parenchymal lung damage and dysfunction as assessed by nitrosative stress and lipid peroxidation [40]. Resolution of inflammation, called “catabasis”, is biologically represented by a switch from pro-inflammatory mediators, such as prostaglandins and leukotrienes, to anti-inflammatory mediators [41]. Arnardottir et al. demonstrated, using lipid mediator quantification in a model of peritonitis, that aged mice had an increased acute polymorphonuclear (PMN) influx and a temporal dysregulated lipid mediator level compared to young mice [42]. The delayed resolution of acute inflammation was associated with lower levels of mediators derived from endogenous PUFAs, like lipoxins, resolvins, protectins, and maresins, collectively termed “specialized pro-resolving mediators” (SPMs) [43]. Of interest, age influences resolvin D1 production in mice, as demonstrated by Dufour et al. [44].
It is now well-established that SPMs are largely responsible for the immunomodulatory actions of PUFAs, even in older subjects [42, 45]. Functions of cells that are part of the innate and adaptive immune response are either promoted or attenuated after exposure to ω-3 PUFAs [46]. Supplementation with even small doses of ω-3 LC-PUFAs, equivalent to consumption of two portions of fatty fish weekly (0.4 g /day), results in gene expression changes in the polymorphonuclear cells (PBMC) involved in inflammation among healthy elderly subjects [47]. These aspects deserve further exploration of the relationship between ω-3 LC-PUFAs, mediator-derived ω-3 LC-PUFAs, and resistance to RTIs, especially in older subjects.
4Older subjects and ω-3 LC-PUFAs
PUFAs are lipids distinguished by the presence of a double bond located at the third carbon atom from the methyl end group (ω-3 PUFA) or a double bond at the sixth carbon atom (ω-6 PUFA) [48]. The ω-3 PUFA family comprises alpha-linolenic acid (ALA), docosahexaenoic acid (DHA), and eicosapentaenoic acid (EPA), and the ω-6 PUFA family, linoleic acid (LA), gamma-linolenic acid (GLA), and arachidonic acid (AA) [48] (Fig. 1). This review will focus on EPA and DHA (ω-3 LC-PUFAs) rather than ALA.
Fig. 1
The anabolic processing of the principal polyunsaturated fatty acids. LA and ALA are essential fatty acids obtained from the diet. LA and ALA are converted to long chain PUFA sharing the same enzymatic pathway but this conversion is not complete and also variable with individuals. Stearidonic acid, eicosatetraenoic acid, GLA, and DGLA are intermediates to synthesize EPA, DHA and AA. AA and EPA are substrates for the synthesis of eicosanoid products including prostaglandins, thromboxanes, and leukotrienes that mediate inflammatory processes. EPA and DHA are metabolized to resolvins, protectins and maresins that promote the resolution of inflammation.
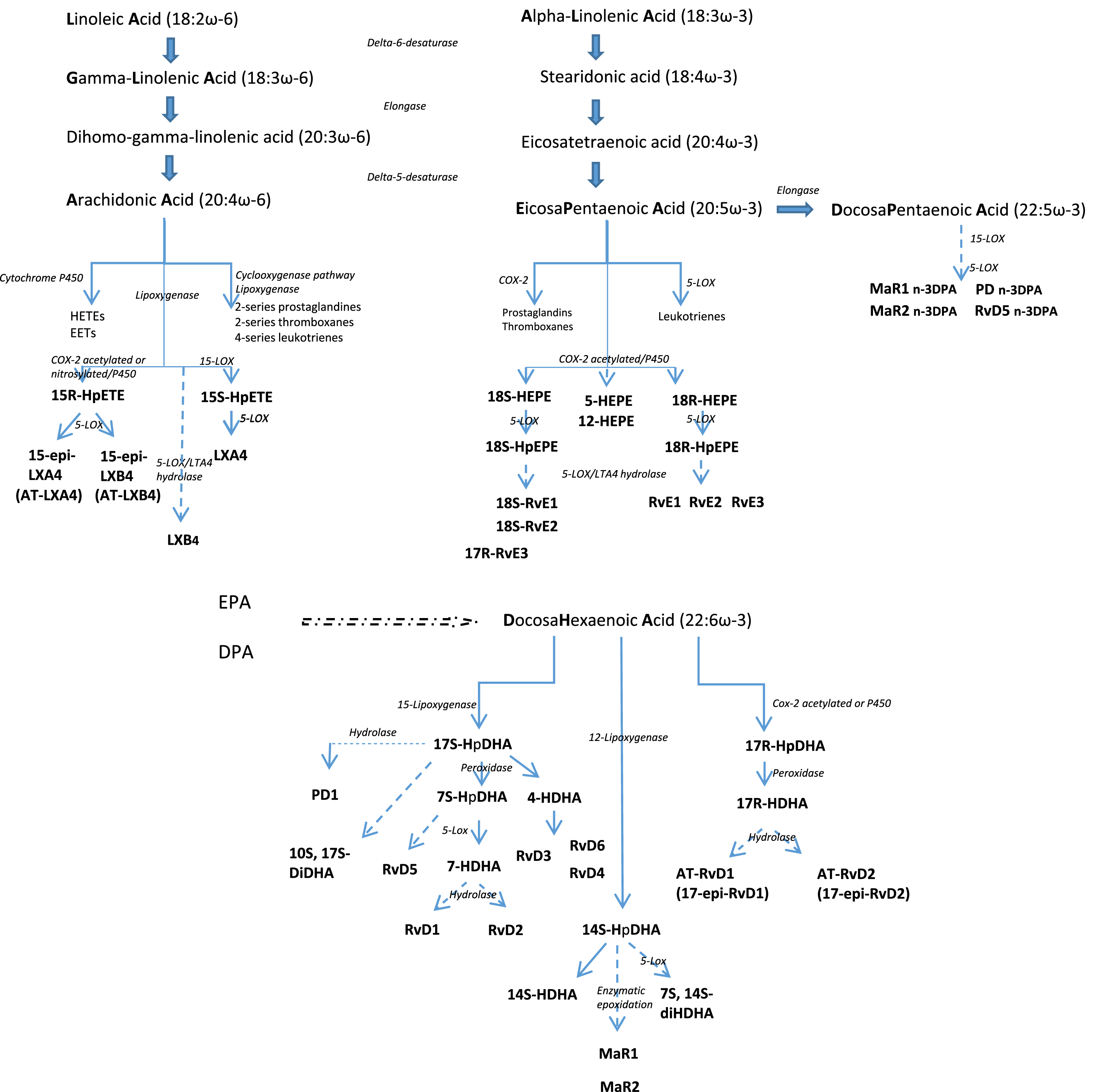
After administration, EPA and DHA rapidly appear in local inflammatory exudates within two hours [49]. By activating various enzymes, such as phospholipase A2, cyclooxygenase-2, and 5-,12-, and 15-lipoxygenase, SPMs are generated from the PUFAs and represent the leading players in the resolution phase of infection [50]. Lower concentrations of SPMs have been observed in pathological conditions, such as Alzheimer’s disease, peripheral artery disease and aspirin intolerant asthma [51–53]. Concentration of SPMs could be significantly improved with fish oil or ω-3 LC-PUFAs supplementation in adults, opening a field of exploration of new therapeutic strategies. Of interest, the ability of older subjects to incorporate EPA into plasma and peripheral blood mononuclear cell phospholipids after EPA-rich oil supplementation is significantly greater than that of younger people [54]. In addition, EPA metabolism is different in older than in younger men, inducing higher concentrations of DHA after EPA administration by increased conversion of EPA into DHA [55]. The significance and impact of this distinct metabolic functioning between older and younger participants has yet to be explained.
In the presence of invading microbes, pathogen-associated molecular patterns, such as TLR-4, induce signal transduction of cytokine expression initiated in membrane platforms called lipid rafts [56]. However, modification of lipid raft structure and subsequently of activated T cell functions with age may suggest a higher immune sensitivity of older subjects to ω-3 PUFAs than of younger ones through the actions of this molecule on lipid rafts [57, 58]. Taking these considerations into account, we will develop in this review the interplay between fatty acids and lipid-derived mediators, and their impact on lung pathogens and infectious disease.
5 ω-3 LC-PUFAs in RTIs: Pre-clinical studies
5.1Animal models
Some studies have shown positive results of ω-3 LC-PUFAs on pathogen clearance and host survival, enabling resolution of inflammation caused by infection. Table 1 resume effects of ω-3 LC-PUFAs administration in animal models. Sharma et al. compared infection induced by Klebsiella pneumoniae (K. pneumoniae) in mice supplemented with cod liver oil, flaxseed oil, and olive oil and mice fed with standard chow [59]. Six weeks of ω-3 PUFA administration decreased the lung bacterial load. The severity of the acute pneumonia was also reduced. Moreover, survival among the mice fed fish oil for 6 weeks was better than among those fed a diet supplemented with corn oil [60]. Saini et al. tested sea-cod oil containing ω-3 LC-PUFAs on the course of S. pneumoniae D39 serotype 2 in mice and showed a favorable immunomodulatory effect of ω-3 PUFAs against bacterial colonization of the lungs [61, 62]. Finally, ω-3 PUFA and ω-6 PUFA enriched diets were recently compared by Hinojosa et al.. Mice fed with an ω-3 LC-PUFAs diet (ratio 18:12 for EPA:DHA) had reduced bacterial titers in their lungs and blood associated with lower concentrations of pro-inflammatory cytokines and lower numbers of lung apoptotic cells [63]. In summary, long-term administration of ω-3 LC-PUFAs improves survival by reducing histopathological lesions of lung tissue and by modulating pro- and anti-inflammatory cytokines.
Table 1
In vivo experimental studies on animal model of effects of ω-3 LC-PUFAs enriched diet on the outcome of respiratory infectious diseases
Authors, year (References) | Model type, challenge | Interventions Daily dose of ω-3 LC-PUFAs | Effects |
Sharma et al., 2013 [59] | BALB/c mice K. pneumoniae induced lung infection | cod liver oil (150 mg EPA/DHA), Maxigard (150 mg EPA/DHA), flaxseed oil (0.5 ml), versus standard chow for 6 weeks | ↓ lung bacterial load ↓ severity of tissue damage with cod liver oil, Maxigard, and flaxseed |
Thors et al., 2004 [60] | Mice K. pneumoniae and S. pneumoniae. induced lung infection | fish oil enriched diet or corn oil enriched diet (10% w/w of the diet daily) for 6 week | ↓ mortality in mice infected with K. pneumoniae in group fish oil (ns S. pneumoniae group) |
Saini et al., 2011 [62] | 6–8 weeks old BALB/c mice, intra-tracheal S. pneumoniae D39 serotype 2 | sea-cod oil (0,5 ml) versus standard chow for 60 days | ↓ pulmonary bacterial load ↓ tissue injury ↓ mortality |
Hinojosa et al., 2020 [63] | Three-month old C57BL/6J mice, S. pneumoniae serotype 4 | 5% corn oil (5%) or corn oil (1%) combined with fish oil (4%) for 2 months. Fish oil contain EPA/DHA at a ratio of 18/12. | ↓ mortality ↓ lung bacterial titers ↓ tissue injury ↓ lung apoptotic cells ↓ pro-inflammatory cytokines |
Freedman et al., 2002 [66] | 30 days of age Cftr -/- mice and WT mice, repeated administration of aerosolized Pseudomona LPS | Peptamen containing 40 mg/day of DHA daily for 7 days | ↓ neutrophils in bronchoalveolar lavage in mice cftr -/- treated with DHA, |
Pierre et al., 2007 [67] | 5 weeks of age Male C57BL/6 mice, bacterial inoculum of P. aeruginosa | 3 isocaloric fatty acid mixtures: ω-3 PUFA (containing 10.5 and 5.1 g/100 g lipids of EPA and DHA respectively), ω-6 PUFA, or a control diet for 5 weeks | no change in lung bacterial load, ↑ distal alveolar fluid clearance ↓ mortality in ω-3 LC-PUFA group |
Tiesset et al., 2009[68] | 5 weeks of age male C57BL/6 mice, P. aeruginosa | EPA and DHA (DHA constituted 4.7% of the total fat content and EPA 11.4%) versus control diet for 5 weeks | ↓ alveolar-capillary barrier permeability (↓ pulmonary injury) ↑ bacterial clearance in the late stage of infection ↓ mortality during the first 24 h in ω-3 LC-PUFA group |
Caron et al., 2015 [69] | 5 weeks of age male C57BL/6 mice, P. aeruginosa | control, DHA/EPA (2:1) and alkylglycerol diets for 5 weeks | ↑ bacterial clearance ↓ lung injury ↓ mortality in ω-3 LC-PUFA group |
McFarland et al., 2008 [71] | Male and female guinea pigs, aerosolized virulent M. tuberculosis | Corn oil (CO) and Menhaden FO (9.5 g and 10 g/100 g lipids of EPA and DHA respectively) for 3 weeks | ↑ bacterial load in the lungs in ω-3 LC-PUFA group ↓ tuberculin skin test |
Jordao et al., 2008 [72] | 10 weeks old female BALB/c mice, intranasally M. tuberculosis | 3 groups: - 0% safflower oil (ω-6, linoleic acid content 7.50%) - 10% Ropufa (ω-3, EPA content 1.5% and DHA 1.1%) - control for 2 weeks | ↓ bacterial numbers in ω-3 LC-PUFA group no change in mortality |
Seki et al., 2010 [91] | Six-to-8-wk old male C57BL/6J mice, Aspiration pneumonia wit sequential administration of hydrochloric acid followed by bacterial instillation of E. Coli | EPA (100 ng) compared to vehicule | ↑ clearance of E. coli from the lung (↓ Bacterial Growth Index below 0.1) |
Byleveld et al., 1999 [77] | 6 weeks old BALB/c mice, Influenza A virus, H3N2 administered into the nostril | Fish oil for 2 weeks | ↑ pulmonary viral load |
Schwerbrock et al., 2009 [78] | 6 weeks old male C57BL/6J, Influenza A virus Puerto Rico/8/34 | Fish oil 4 g or corn oil 1 g for 2 weeks | ↑ pulmonary viral load ↓ lung neutrophils ↓ degree of lung pathology (reduced inflammation) ↑ mortality in group with fish oil |
Pseudomonas aeruginosa (P. aeruginosa) is an opportunistic pathogen involved in respiratory tract infections. This Gram-negative aerobe/facultative anaerobe is responsible for many chronic infections commonly found in patients with chronic obstructive pulmonary disease and plays a significant role in the pathogenesis of cystic fibrosis-induced lung disease [64]. Human subjects with cystic fibrosis, independent of pancreatic insufficiency and acute lung infection, showed an imbalance in essential fatty acid membrane composition compared to healthy control subjects [65]. The lower DHA and higher AA tissue concentrations observed in cystic fibrosis stimulated exploration of the effects of ω-3 LC-PUFAs in animal cystic fibrosis models. Indeed, oral administration of high doses of DHA suppressed the increase in bronchoalveolar neutrophil and eicosanoid concentration after pseudomonas lipopolysaccharide-induced pneumonia in CFTR knockout (CFTR-/-) mice but not in wild-type mice [66]. In addition to effects in cystic fibrosis, ω-3 LC-PUFAs have been shown to have a beneficial effect in wild-type animal models. In this context, Pierre et al. worked on a murine model of chronic P. aeruginosa infection [67]. An ω-3 LC-PUFAs enriched diet (EPA/DHA 2:1 ratio) given prior to P.aeruginosa infection improved distal alveolar fluid clearance (DAFC) and was associated with higher survival of animals compared with isocaloric control ω-6 PUFA diets. Using the same EPA/DHA ratio, Tiesset et al. observed the impact of ω-3 LC-PUFAs on kinetic modulation of inflammation, and showed heightened and overlapped pro and anti-inflammatory responses after P.aeruginosa-induced lung infection [68]. Finally, Caron et al. tested the impact of a mixture enriched with a reverse EPA/DHA ratio extracted from rays and chimeras given for five weeks [69]. EPA/DHA (1:2 ratio) supplementation was associated with faster bacterial clearance than in control mice and improved survival rate. In addition, a lower neutrophil count was detected in the lung 36 hours after the onset of infection in parallel with higher levels of the pro-inflammatory cytokine, tumor necrosis factor (TNF)-α, and the anti-inflammatory cytokine, Il-10. These observations suggest an accelerated resolution of infection with ω-3 PUFAs, predominantly DHA.
Intracellular pathogens such as Mycobacterium tuberculosis (M. tuberculosis) are also a part of the infectious disease burden in older individuals [70]. Unlike actions on the pathogens mentioned earlier, administration of high doses of ω-3 LC-PUFAs was associated with a larger bacterial load in mice lungs compared with an ω-6 PUFA diet rich in AA [71]. However, other experiments in mice showed that an EPA/DHA-enriched diet increased pathogen killing [72]. Despite the lower organ M. tuberculosis load in ω-3 LC-PUFA groups, there was no difference in survival between the interventions. In the context of intracellular infections, some authors have recommended caution with long-term, high-dose supplementation ω-3 LC-PUFA [73]. The Inuit population, well known for its high intake of ω-3 LC-PUFAs, despite signs of a nutritional transition [74], has the highest incidence rate of active tuberculosis among Canadian-born Aboriginals [75]. Nevertheless, epidemiologic data from a prospective study in Singapore showed a reduced risk of active tuberculosis with PUFA dietary intake [76]. The inconsistencies among pre-clinical and population studies evaluating the association between ω-3 LC-PUFAs and tuberculosis infection makes it difficult to draw definitive conclusions.
The effects of ω-3 LC-PUFA supplementation on influenza infections are also conflicting. Indeed, a fish oil-enriched diet for two weeks increased the susceptibility of mice to influenza viral infection and led to delayed viral clearance and an impaired antibody response and lung interferon-γ production [77]. In another experiment, an ω-3 LC-PUFA diet increased the severity of influenza-induced infection [78]. By contrast, administration of a bioactive lipid derived from DHA, protectin D1, improved survival of influenza-infected mice [79] (see later).
5.2Ex vivo studies
Sharma et al. demonstrated that the phagocytic ability of murine alveolar macrophages, evaluated by lung myeloperoxidase production, against K. pneumoniae was increased after six weeks of ω-3 PUFA administration [59]. However, reduced lymphoproliferative response in splenocyte cultures from guinea pigs was observed after enriched ω-3 LC-PUFAs [71].
According to these studies whatever the type of model used, the effects of ω-3 LC-PUFAs in preventing infectious disease require further studies.
6SPMs in RTI: Pre-clinical studies
The active process of resolution of inflammation mediated by bioactive pro-resolving molecules or SPMs works in concert and in an ordered manner with other anti-inflammatory processes to limit lung injury [80]. In cell cultures and ex vivo studies, SPMs counter-regulate the chemoattraction of polymorphonuclear cells to an inflamed site, stimulating monocyte recruitment and activation of macrophage efferocytosis of apoptotic granulocytes, pathogen, and tissue debris [81–83]. Since the discovery of the first SPM, studies have been conducted to evaluate the mechanisms of action of these molecules, including receptor expression, signalization, and intercellular communication in murine lungs [84]. Moreover, in response to inflammation, SPMs are produced during interactions between neutrophils and lung cells, including bronchial epithelial cells, alveolar epithelial type II cells, alveolar macrophages, and mesenchymal stem cells [85–87]. In murine model, SPMs enhance bacterial clearance such that lower antibiotic doses are required [88]. SPM concentrations are positively correlated with increased phagocytosis in whole blood, whether endogenously produced or generated after PUFA supplementation. Interestingly, various SPMs have been shown to have a marked effect on the evolution of lung infection (e.g. resolvin E1, aspirin-triggered resolvin D1, resolvin D1, maresin 1, protectin D1). The major pre-clinical studies in this field are summarized in Fig. 2 and Table 2.
Fig. 2
Pre-clinical studies using and derived bioactive lipids ω-3 LC-PUFAs. Summary of results from experimental studies in mice using bacterial induced respiratory tract infections and interventions with fish oil (FO), EPA, DHA or SPM: aSharma et al.; bSaini et al.; cHinojosa et al.; dPierre et al.; eSeki et al.; fTiesset el al.; gCaron et al.; hAbdoulnour et al.; iCodagnogne et al.
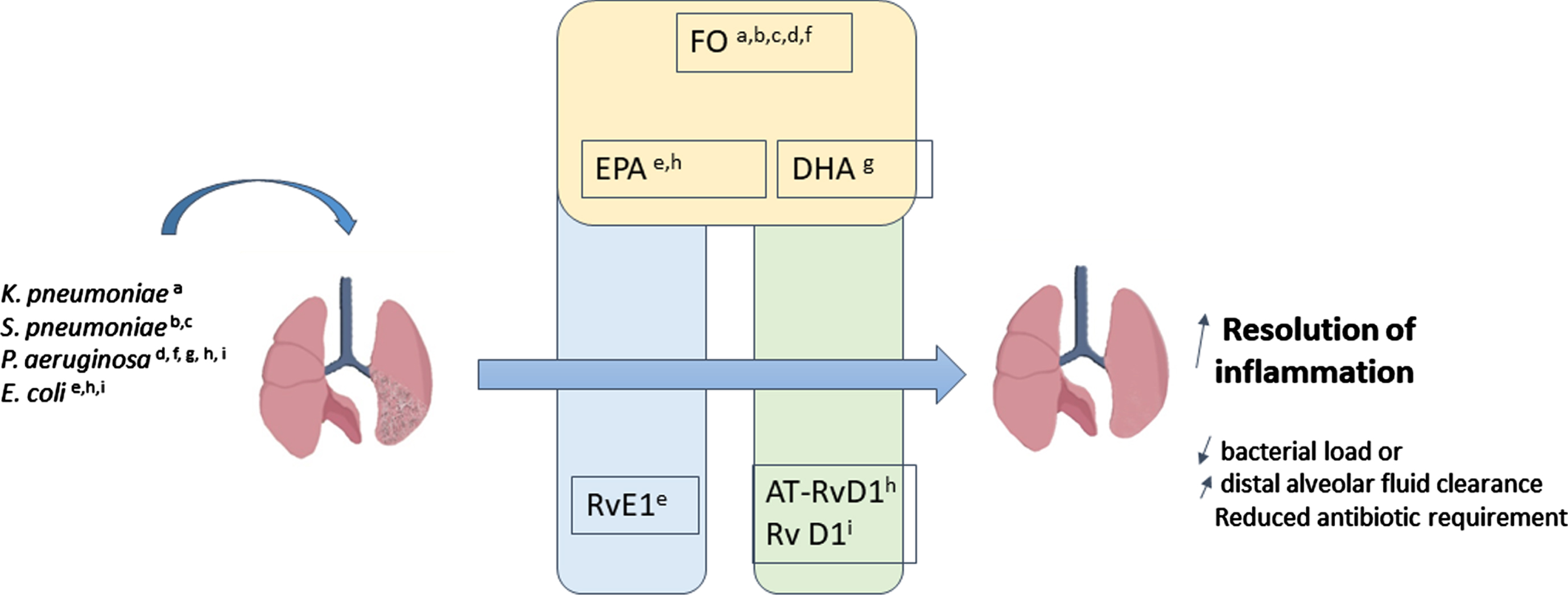
Table 2
In vivo experimental studies on animal model of effects of SPM derived from ω-3 LC-PUFA on the outcome of respiratory infectious diseases
Authors, year (References) | Model type, challenge | Interventions | Effects |
Seki et al., 2010 [91] | 6-to-8-wk old male C57BL/6J mice, Aspiration pneumonia wit sequential administration of hydrochloric acid followed by bacterial instillation of E. Coli | administration of RvE1 (EPA-derived resolving at 100 ng) prior to acid injury compared to vehicle | ↓ lung neutrophil accumulation by 55% ↑ clearance of E. coli (↓ Bacterial Growth Index) |
Abdulnour et al., 2016 [93] | 8–10 wk old C57BL/6 male mice, high dose of intra-bronchial E.coli or P.aeruginosa | AT-RvD1 (100 ng, IB) or vehicle via a repeat tracheostomy surgery 24 and 36 h after challenge | ↓ lung E.coli at 6 h and 24 h ↑ lung P.aeruginosa bacterial clearance |
Sekheri et al., 2020 [95] | Female C57BL/6 mice, intratracheal instillation of E. coli | Bolus injection 17-Epi-RvD1 (DHA-derived resolvin) 6 h post challenge | ↑ bacterial clearance, ↓ in BAL fluid total leukocyte and PMN numbers |
Codagnone et al., 2018 [96] | Mice, intratracheal instillation of P. aeruginosa | single oral administration of RvD1, a DHA-derived resolvin (100 ng/ mouse) or RvE1, a EPA-derived resolvin (100 ng/mouse) by intragastric gavage 3 h post intratracheal inoculum and every 48 h starting from day 5 post inoculum | ↓ bacterial load and leukocyte infiltration in acute and chronic lung infection ↓ mortality with RvD1 but not RvE1 additional benefit of RvD1 in combination with ciprofloxacin (200 mg, ip) |
Morita et al., 2013 [79] | wild-type mice subjected to intratracheal instillation of influenza 2009 H1N1 | PD1 (Protectin D1, a bioactive lipid derived from DHA) 1 mg/mouse i.v. 12 h before and immediately after challenge (prophylactic model) or 48 h after challenge (therapeutic model) RvD1 or RvD2 (Resolvin derived from DHA) | ↓ Mortality (additional benefit with PD1 plus peramivir treatment) ↓ nuclear export of viral mRNA from lung cells ↓ influenza virus replication no change in macrophage and neutrophil numbers in bronchioalveolar lavage (BAL) fluid 48 h after infection (completely rescued) no change with RvD1 |
Walker et al., 2021 [100] | 6–8 week old C57BL/6 male mice, Respiratory Syncytial Virus (RSV) pneumonia | Intranasal PD1 or PCTR1 (bioactive lipids derived from DHA) 100 ng or vehicle on days 3, 4, 5 post infection (p.i.) | ↓ lung eosinophils and lung macrophage ↓ lung neutrophils and Natural Killer (NK) with PCTR1 ↓ RSV N gene RNA 6 days after challenge with PD1 and PCTR1 |
Wang et al., 2017[94] | 8–10 weeks old C57BL/6J male mice, Coinfected intranasally with Influenza A virus and S. pneumoniae | Intranasal AT-RvD1 (bioactive lipid derived from DHA) 100 ng or vehicle once daily on day 4 and 6 | ↓ Pneumococcal load in BALF no change in viral titer ↓ lung neutrophil numbers ↓ myeloperoxidase activity ↓ antimicrobial activity |
6.1Animal models
In baboon, pneumonia influences the level of SPM production as demonstrated by Dalli et al [89]. These authors reported that inoculation of S. pneumoniae into baboons significantly reduced levels of plasma D-series, E-series, and lipoxins at 48 and 168 hours, demonstrating a temporal shift in the plasma lipid mediator profiles after infection.
Aspiration of gastric acid is a frequent clinical event that can lead to increased susceptibility to pneumonia and acute lung injury/acute respiratory distress syndrome (ALI/ARDS) [90]. In line with these data, Seki et al. used an experimental murine model of aspiration pneumonia with sequential administration of hydrochloric acid followed by bacterial instillation of E. coli to study the effects of SPMs and their precursors, ω-3 LC-PUFAs, on infection and inflammation [91]. EPA-derived resolvin E1 (RvE1) was injected prior to the insult and was associated with a dramatic reduction in pro-inflammatory cytokines and chemokines in the lung (i.e., IL-1β, IL-6, high-mobility group box [HMGB]-1 protein, macrophage inflammatory protein [MIP]-1α, MIP-1β, keratinocyte-derived chemokine, and monocyte chemoattractant protein [MCP]-1). Moreover, RvE1 administration also decreased neutrophil infiltration by 55% compared to vehicle. This experiment showed the anti-inflammatory effect of RvE1 combined with maintained clearance of E. coli pneumonia. By contrast, EPA decreased the Bacterial Growth Index (BGI) of E. coli in lung homogenate of animals and dampened the severity of bacterial pneumonia. However, RvE1 displayed more potent action during resolution of the infectious challenge than EPA.
Aspirin-triggered resolvin D1 (AT-RvD1), also known as 17-epi-RvD1, is a DHA-derived lipid mediator generated from an intermediate 17R-HDHA by COX-2 ASA and by oxygenation by 5-LOX [92]. This compound, 17R-HDHA, resists rapid degradation by oxidoreductase and has a longer in vivo half-life than RvD1 because of the conformation change of a hydrogen molecule from (S) to (R) around the seventeenth carbon. E. coli infection induced AT-RvD1 release in mouse lung [93]. AT-RvD1 significantly reduced bacterial lung load and severity of pneumonia in coinfected mice with influenza A virus and S. pneumoniae. Moreover, administration of AT-RvD1 enhanced macrophage phagocytosis of E. coli particles and efferocytosis in the lung, promoting bacterial clearance [93]. In contrast to combined use of ciprofloxacin and AT-RvD1, antibiotic administration alone did not decrease BALF neutrophils despite a lower concentration of E. coli. These results indicate a pivotal role of AT-RvD1 in bacterial clearance and pathogen-initiated lung inflammation. The same investigators observed increased bacterial clearance in vivo and lung macrophage phagocytosis ex vivo by early treatment with exogenous AT-RvD1 in P. aeruginosa-induced pneumonia. AT-RvD1 may restore impaired phagocytosis and facilitate resolution of acute inflammation via the receptor ALX/FPR2, a potential therapeutic target [95]. Similarly, RvD1 administration enhances bacterial clearance by an additive effect with ciprofloxacin, suggesting that RvD1 may potentiate the efficiency of antibiotics [96].
P. aeruginosa represented 20.8% of isolated microorganisms in ICU-acquired pneumonia in 2016 and has a high level of resistance to multiple antibiotics in Europe [97]. P. aeruginosa is found in the airways of patients with cystic fibrosis, bronchiectasis, and chronic bronchitis [98]. Non-antibiotic strategies have therefore been evaluated in mice using resolvin D1 and an endogenous SPM enzymatically synthesized from DHA [41]. Recently, Codagnone et al. examined different actions of RvD1 in acute and chronic lung infection induced by a multi-drug resistant clinical strain of P. aeruginosa [96]. The authors first analyzed lipid mediators and precursors in lung homogenates from mice with long-term infection with P. aeruginosa RP73 at 0, 5, and 21 days after inoculation and determined defects in SPM production using metabololipidomics and proteomics. An intragastric dose of RvD1 reduced lung bacterial titer and BALF total leukocyte accumulation compared to vehicle and increased survival.
Severe influenza infection dramatically decreases the bioactive lipid-derived DHA or protectin D1 (PD1) levels in infected lung [99]. However, the administration of PD1 restores levels to pre-infection values and was associated with increased survival of virus-infected mice [79]. In addition, PD1 inhibits the nuclear export of viral mRNA from lung cells and thus reduces replication of the influenza virus. A decrease in genomic viral load in lung tissue secondary to respiratory syncytial virus (RSV) infection was also observed after intranasal PD1 administration [100].
6.2Ex-vivo human studies
A pilot study analyzing plasma from patients with newly-diagnosed active tuberculosis infection showed higher levels of D-series resolvins (i.e., RvD1 and RvD2) and aspirin-triggered resolvin D1 (AT-RvD1) compared to asymptomatic and presumably uninfected controls, indicating that these resolvins are likely involved in the host response to infection [101]. The authors suggested the use of high-resolution metabolomic profiling of plasma to evaluate general response to TB by dosing D-series resolvins [101]. Ruiz et al. evaluated the effects of exogenous administration of SPMs on human monocytes-macrophages infected with M. tuberculosis [102]. All SPMs tested reduced tuberculosis-induced TNF-α production, but RvD1 and another DHA lipid-derived mediator, maresin R1 (MaR1), specifically limited M. tuberculosis growth. This study thus showed a potential therapeutic effect of pro-resolving lipids against M. tuberculosis [103].
Taken together, pre-clinical studies using SPMs seem to offer more encouraging results for infection outcomes than their parent molecules, the ω-3 LC-PUFAs [104].
7Older subjects and severity of RTI
The mortality rate from sepsis, an infectious condition caused by a dysregulated immune system response associated with organ dysfunction, is 25–30% and is higher in older patients [105]. About half of sepsis cases have a respiratory site origin [105]. The hyperinflammatory response present in the initial stage of sepsis often leads to progressive development of immunosuppression responsible for late mortality [38]. Sepsis is characterized by modified metabolism of essential fatty acids, which is associated with the severity of sepsis and clinical outcome [106]. Some argue that the essential fatty acid deficiency seen in sepsis may be partly due to TNF-α in the same way as in chronic malnourished states [107].
Considering this imbalance in fatty acid levels, 146 patients with ARDS as a result of pneumonia, trauma, or aspiration injury were randomized to a high-fat enteral diet combining EPA and GLA or a standard diet for at least 4–7 days in a interventional multicenter trial [108]. The group receiving the high-fat diet required significantly fewer days of ventilatory support and had a decreased ICU length of stay compared with controls. Similarly, in a systematic review, Dushianthan et al. reported a significant improvement in blood oxygenation, reduced ventilator days, and shorter ICU length of stay in patients with ARDS who received nutrition enriched with EPA and DHA [109]. Moreover, in a pooled analysis of 40 randomized controlled trials, PUFAs had some beneficial effects on survival in sepsis-induced ARDS in the enteral nutrition subgroup [110]. Conflicting data on pulmonary outcomes have been observed in some randomized controlled trials, suggesting possibly harm with PUFA administration [111]. Among these studies, daily combined enteral doses of EPA and DHA in a study by Rice et al. were more than 10 g, which is higher than doses considered safe [112]. Globally, trials that have evaluated PUFA in ALI or ARDS have been of low-quality [109].
By contrast with the population studies using PUFA, pre-clinical investigations with SPMs support their action in host immune response in severe inflammatory states, as suggested by a review of Fullerton et al. [113]. During lung injury, MaR1, a member of the SPM family, had a protective effect and may represent a potential new therapeutic approach for the treatment of ALI/ARDS [114]. This SPM, generated from DHA, is known to enhance macrophage phagocytosis [115]. Of interest, high doses of MaR1 mitigated LPS-induced lung injury by limiting neutrophil influx and pro-inflammatory cytokine/chemokine (TNF-α, IL-1β, IL-6, keratinocyte chemokine, monocyte chemoattractant protein-5, macrophage inflammatory protein MIP-1α and MIP-1γ) release [114]. In addition, MaR1 promoted generation of the anti-inflammatory cytokine, IL-10 [114]. RvE1 facilitates the resolution of inflammation and could prevent ALI/ARDS by promoting phagocytosis-induced neutrophil apoptosis [116]. These experiments highlight the evident link between neutrophil apoptosis in enhancing the resolution of inflammation and, conversely, the numbers of neutrophils in sustained deleterious inflammatory response [117, 118].
8 ω-3 LC-PUFAs intake and secondary SPM levels
The biosynthesis of SPMs partly depends on the availability of their precursor, the ω-3 LC-PUFAs. To analyze this relation, we reviewed all interventional studies combining ω-3 LC-PUFAs administration and detecting SPMs in blood samples of adult participants. We searched the Medline databases from May 2021 to March 2022 to identify relevant studies. The search terms were “Fatty Acids Omega-3, n3 PUFA, n3 Polyunsaturated Fatty acid, docosahexaenoic, eicosapentaenoic” and other related combined with the terms “resolvin, specialized pro-resolving lipid mediator.” The search identified 818 articles that assessed SPMs after ω-3 PUFAs administration, and 26 studies were included in the table following the screening process. The details of search strategies and the flow chart of studies selected are described in Fig. 3. Various doses of ω-3 LC-PUFAs and durations of administration were tested in different populations. Concentrations of intermediate precursors of SPMs, such as 18-HEPE, 15-HEPE, 14-HDHA, 17-HDHA, were often increased after combined of EPA and DHA administration. Concentration of resolvin D1, resolvin E1, and maresin 1 were higher with intakes of ω-3 LC-PUFAs greater than 1 g. Interventions included single administration of ω-3 LC-PUFAs [119–121] or administration for a short period of time such as one week [122–124]. Population samples from healthy and chronically ill individuals older than 65 years were studied [124–131]. Methods of SPM measurement differed, with for example two studies assessing SPMs in stimulated neutrophils [132, 133]. It is difficult to interpret the results of studies that do not provide baseline levels of SPMs, no comparator control group or no correction of the results for multiple tests. Although the effect of age on SPM production deserves further investigation and evaluation of duration and dose-response relationships, these preliminary results are encouraging.
Fig. 3
PRISMA chart: Search strategy for studies evaluating plasma production of specialized pro-resolving mediators (SPM) from interventional studies using ω-3 PUFAs in adults and search terms.
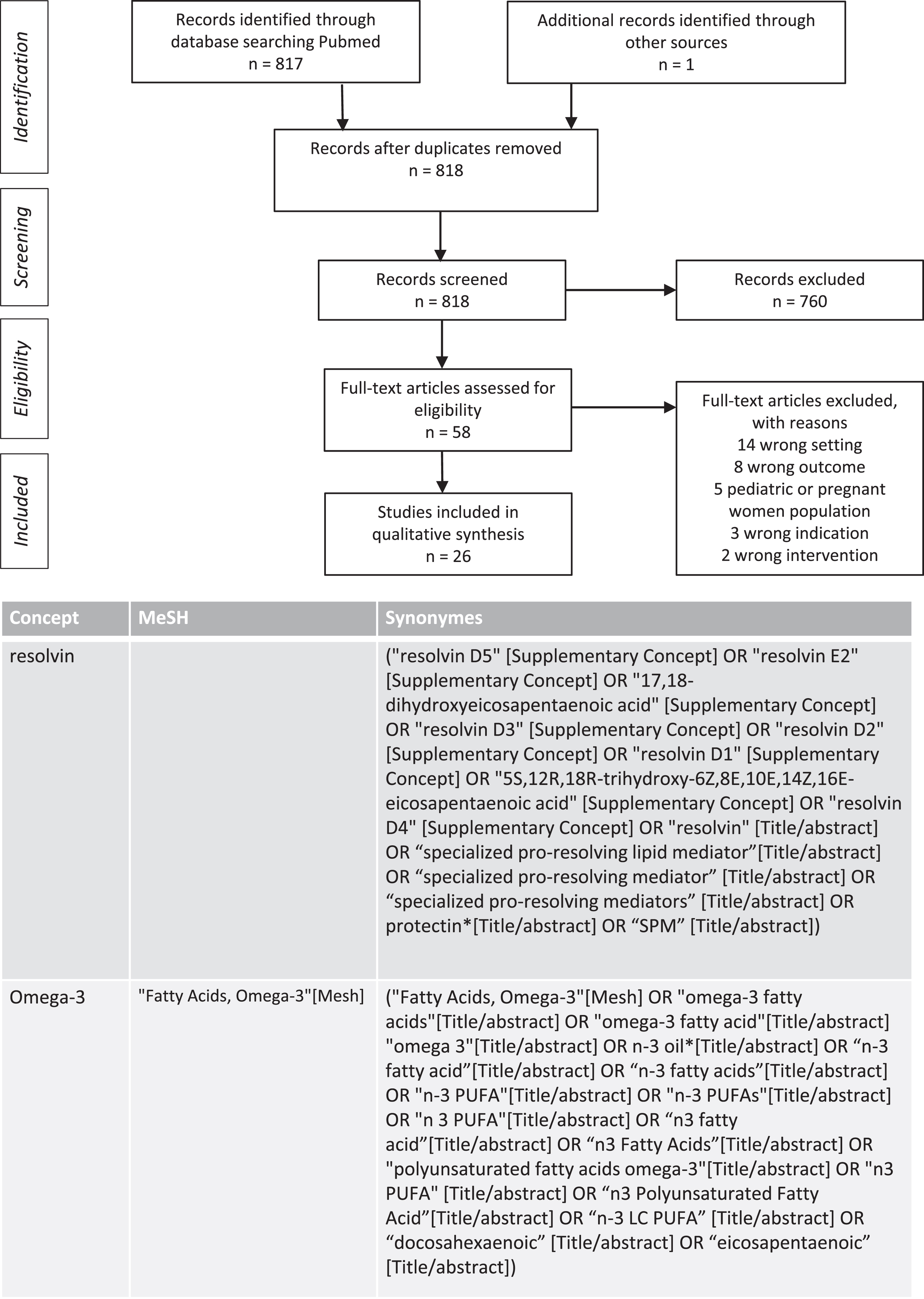
9 ω-3 LC-PUFAs and SPMs: A matter of debate
Despite some data showing plasma and local generation of SPM from EPA and DHA, interventional pre-clinical studies have mainly used direct bioactive lipid-derived SPMs instead of analyzing SPM production by the parent molecules. In addition, ω-3 LC-PUFAs are considered by some authors as immunosuppressive, describing absent or deleterious effects on the proliferation of certain pathogens in vitro [134]. Heterogeneity of experimental design in terms of ω-3 LC-PUFA doses, infectious challenge pathways, and inflammation-dampening strategies explain this mixed evidence base. Additionally, intake of ω-3 LC-PUFAs represents a considerable percentage of the total energy provided in animal studies, whereas in humans, the total amount of ω-3 LC-PUFAs rarely exceeds 3% [135]. In some cases, mice fed fish oil had less total food intake than controls, possibly secondary to a less palatable diet [77]. These discrepancies in studies concerning the immunomodulatory effects of ω-3 LC-PUFAs are challenging and should be explored in light of secondary SPM production.
Table 3
Interventional studies evaluating ω-3 LC-PUFA supplementation in adults and older participants on ω-3 LC-PUFA derived mediators production with related immunomodulatory effects
Authors, year, study design (References) | Total participants | Intervention Daily dose of ω-3 LC-PUFAs | Plasma SPMs, EPA-derived, n-3 DPA, DHA-derived precursors | Additional laboratory analysis, ex-vivo results and clinical outcome |
Barden et al., 2014, one arm [122] | 21 healthy participants, 53.7 (6.9) years old, | 1.4 g EPA and 1 g DHA, for 5 days | ↑ 18-HEPE ↑ 14-HDHA ↑ 17-HDHA ↑ RvE1 | No additional benefit of aspirin in SPM production |
Barden et al., 2015, two arms [142] | 43 participants in total, 57.3 (2.1) in healthy group and 59.9 (1.1) years old in metabolic syndrome group | 1.4 g EPA and 1 g DHA for 21 days | ↑ 18-HEPE ↑ 14-HDHA ↑ 17-HDHA ↑ RvE1, RvE2, ↑ RvE3 ↑17R-RvE3 | Concentration of 18-HEPE, 17-HDHA and 14-HDHA higher in women |
Barden et al., 2016, single arm [143] | 72 participants, 57.3 (2.4) years old, in arthritis patients | 1.4 g EPA and 1 g DHA for 28 days | * ↑ 10 S,17S-diHDHA, ↑ RvE2, ↑ AT-RvD1, ↑ RvD1, ↑ RvD2, ↑ PD1 ↑ 14-HDHA, ↑ MaR1 adjusted p-value for comparison between healthy and arthritis patients | Level of plasma SPMs more elevated in arthritis patients |
Barden et al., 2018, RCT [132] | 74 participants with chronic renal impairment, 56.5 (1.4) years old | 1.84 g EPA + 1.52 g DHA + 0.152 DPA g (ethyl ester formulation) for 56 days, | * ↑ 18-HEPE ↑ 17 HDHA ↑ RvE1, ↑ RvE2, ↑ RvE3, ↑ RvD5 (in stimulated neutrophils) | ↓ plasma myeloperoxidase No effect of Coenzyme Q10 |
Capo et al., 2016, controlled [133] | 15 healthy participants (male footballers), 19.7 (0.4) | 1.4 g DHA (drink beverage) for 56 days | ↑ RvD1 (LPS-stimulated PBMC) post-exercise | Synergistic effects of acute exercise and DHA supplementation in induction of anti-inflammatory response, ↓inflammatory response induced by PAMPs as LPS. |
Colas et al., 2014, single arm [120] | 10 healthy participants, 34 (2) years old | 0.5 g EPA and 0.2 g DHA, one oral administration with ASA 81 mg 2 hours later | ↑ RvE2, ↑ RvE3, ↑ RvD1, ↑ RvD2, ↑ 17-epi-PD1 (4 h after n-3 PUFA intake) | Whole blood phagocyte ingestion of E.Coli positively correlated with increased levels of Rv D1, Rv D2, Rv E2, Rv E3 and 17-epi-PD1 |
Dawczynski et al., 2013, RCT [144] | 47 mildly hypertriglyceridemic participants, 61.6 (11.8) years old | 3 groups: Yoghurt enriched with - 0.8 g ω-3 LC-PUFAs - 3 g ω-3 LC-PUFAs - control for 10 weeks | 12 HEPE 15 HEPE 18 HEPE Not significantly elevated | ↓ production of IL-2 by PMA/ionomycin-stimulated T cells in ω-3 LC-PUFAs groups |
Elajami et al., 2016, controlled [145] | 6 participants, 62.9 (11.8) years old, coronary artery disease | 1.86 g EPA and 1.5 DHA for 1 year | ↑ 17R-RvD3, ↑ RvD6, ↑ 17R-PD1 | ↑ macrophage phagocytosis of blood clots |
Fischer et al., 2014, one arm [146] | 20 healthy participants, 31.2 (2.5) years old for men and 38 (1.8) for women | 460 mg EPA and 380 mg DHA (ethyl esters) for 28 days followed by 980 mg and 760 mg per day for 28 additional days | * ↑ 5-HEPE, ↑ 4-HDHA, ↑ 7-HDHA, ↑ 12-HEPE, ↑ 14-HDHA, ↑ 18-HEPE | Whole blood sample treated with calcium ionophore stimulated monohydroxy-metabolite formation via LOX enzymes |
Grenon et al., 2015 [125] | 80 participants, 68 (7) years old, with peripheral artery disease | 2.6 g EPA and 1.8 g DHA for 30 days | ↑ 5-HEPE, ↑ 15-HEPE, ↑18-HEPE ↑ 4-HDHA, ↑ 17-HDHA | |
Lamon-Fava et al., 2021, RCT [147] | 42 participants, adults, obese/overweight with major depressive disorder and chronic inflammation, 52 (13) years old in placebo group | 3 groups tested, daily: - 1 g ω-3 LC-PUFAs - 2 g ω-3 LC-PUFAs - 3 g ω-3 LC-PUFAs | * ↑18-HEPE, ↑12-HEPE, 15-HEPE, ↑ 5-HEPE after 28 days of supplementation, ↑17-HDHA and ↑14-HDHA at 12 weeks | |
Markworth et al., 2016, RCT crossover [123] | 10 healthy participants (women), 25.5 (3.3) years old | 8 g ω-3 LC-PUFAs for 7 days, 14 days | ↑RvD1, ↑RvD2, ↑ RvD6, ↑ PD1, ↑ MaR1 | divergent effects of EPA and n-3 DPA on human lipid mediator profile |
Mas et al., 2012, one arm [148] | 20 healthy participants, 59 (5) years old | 1.4 g EPA and 1 g DHA with 81 mg ASA for 21 days | 17 HDHA, RvD1, RvD2 detected (tests of significance not found) | |
Mas et al., 2016, RCT [149] | 85 participants with chronic kidney disease, 56.5 (1.4) years old | 1.8 g EPA and 1.52 g DHA for 56 days with CoQ | ↑ 18-HEPE, ↑ 17 HDHA, ↑ RvD1 | Changes in 18-HEPE et 17-HDHA levels predicted by change in platelet EPA and DHA level. No effect of Coenzyme Q10 |
Norris et al., 2018, RCT cross over [150] | 9 healthy participants, 24 (0.3) years old | Study A: 2.7 g of ω-3 LC-PUFAs for 5 months (controlled design) Study B: 3.4 g of ω-3 LC-PUFAs for 8–12 weeks (crossover) | Study A (serum) and Study B (plasma): ↑RvD1, ↑RvE1, ↑17-HDHA, ↑18-HEPE after LPS challenge | |
Oh et al., 2011, two arm [119] | 3 healthy participants | 1 g EPA + 80 mg aspirin, single administration | ↑ 18-HEPE (serum) 3 h after administration | |
Polus et al., 2016, controlled [151] | 59 participants, 46.6 years old, women’s moderate obese | 0.45 g EPA and 1.29 g DHA for 3 months | * ↑ RvD1, ↑ RvD2, ↑ PDX | ↓ hs-CRP, ↓ MCP-1, ↓ s- VCAM-1 |
Poreba et al., 2017, RCT [126] | 74 participants, 65.6 (6.8) years old with type 2 diabetes, history of coronary artery disease or peripheral artery disease | 1 g EPA and 1 g DHA (in a drink) for 3 months | No change in Rv D1 | No change in inflammatory markers |
Ramirez et al., 2019, RCT [127] | 24 participants, 69 (8) years old with peripheral artery disease | 2.6 g EPA and 1.8 g DHA for 3 months | ↑ 18-HEPE, ↑ 15-HEPE, ↑ 5-HEPE, ↑ 12-HEPE, ↑ RvE3 | No change in hs-CRP, IL-6, ICAM-1 |
Schaller et al., 2017, RCT [124] | 80 participants, 68 (7) years old with peripheral artery disease | 2.6 g EPA and 1.8 g DHA for 1 month | ↑ 18-HEPE, ↑ 15-HEPE, ↑ 5-HEPE, ↑ 4-HDHA | |
Schaller et al., 2020, arm for healthy and arm for patients with peripheral artery disease [128] | 20 participants, 69.5 year old healthy and with peripheral artery disease | 3 different groups tested: - 0.69 g EPA and 0.5 g DHA - 3 g EPA and DHA - 4.14 g EPA and 3 g DHA for 7 days with monohydroxylated SPM precursors and n-3 DPA | ↑ 18-HEPE, ↑ 15-HEPE, ↑ 5-HEPE ↑ 17-HDHA, ↑ 14-HDHA, ↑ 7-HDHA, ↑ 4-HDHA 10 S,17S-diHDHA ↑ MaR2 n-3 DPA | ↑ phagocytic activity of peripheral blood monocytes and neutrophils |
Skarke et al., 2015, RCT [152] | 6 healthy participants, 24.3 (4.3) years old | 5.58 g EPA and 4.5 g DHA for 50 days followed by intravenous injection of 0.6 ng/kg endotoxin | ↑ PD1, ↑ RvE1 (tests of significance not found) | ↑ cytokines and CRP after endotoxin challenge |
12 healthy participants, 30.8 (11.6) years old | 9.7 g EPA and 7.9 g DHA for 24 days | PD1 (tests of significance not found) | ||
So et al., 2021, RCT crossover [129] | 21 participants, between 50–75 years old, with chronic inflammatory disease | 2 groups tested: 3 g of DHA and 3 g of EPA (ethyl ester) for 34 weeks | ↑18-HEPE,↑ 15S- HEPE,↑ 12-HEPE, ↑11-HEPE, ↑ DPA-derived SPM ↑ 17-HDHA, ↑ 13-HDHA, ↑ 14-HDHA, ↑ 7-HDHA, ↑ 4-HDHA | Modulation of LPS-stimulated monocyte cytokine expression |
Souza et al., 2020, RCT [121] | 22 healthy volunteers aged between 19 and 37 years | 3 different groups tested - 1.5 g ω-3 LC-PUFAs - 3 g ω-3 LC-PUFAs - 4.5 g ω-3 LC-PUFAs Analysis after 2 h, 4 h, 6 h, 24h | * ↑ 14-HDHA, ↑ 18-HEPE, ↑ 17-HDHA, ↑4 S,14S-diHDHA, ↑ Rv E3, ↑ Rv D5, ↑ Ma R1, ↑ Ma R2, ↑ DPA-SPM derived, for de two groups of higher dose received of ω-3 LC-PUFAs | ↑ dose-dependent in neutrophil and monocyte phagocytosis of bacteria |
Uno et al., 2016, control [130] | 40 participants, 65.5 (1.9) years old in patients undergoing major hepatobiliary resection | oral supplementation containing EPA (immunonutrition) | ↑ RvE1 (after surgery) | ↓ rate of infectious complications and severity of complication in group receiving immunonutrition |
Wang et al., 2015, RCT [131] | 15 participants with Alzheimer disease | 0.6 g EPA and 1.7 g DHA for 6 months | ↓ Rv D1 in controlled group (released from PBMCs after Aβ40 exposure) | |
Welty et al., 2021, RCT [136] | 31 participants with stable coronary artery disease | 1.86 g EPA and 1.5 g DHA for 30 months | ↑ 18-HEPE, ↑ MaR1, ↑ Rv E1 |
*Results adjusted for multiple tests. SPMs studies evaluated in animal model in Table 2 have been highlighted in bold in this Table.
Profiling of lipid mediators and their derivatives has recently been considered for its prognostic value [136, 137]. In healthy volunteers, early and delayed “resolvers” were described as two phenotypes in the resolution of inflammation [138]. Studies mentioned earlier suggest an association between lower levels of ω-3 LC-PUFAs and their derivatives in case of infection [89]. However, in the recent DO-HEALTH randomized clinical trial, no significant effects of ω-3 LC-PUFAs on infections rate was found [139]. To explore the effect of SPM precursor on infectious disease burden, the choice of a more sensitive population, such as older patients at risk of immune dysregulation, seems important. Nevertheless, the use of ω-3 LC-PUFAs should take into account the impact of the aging process on their metabolism and age-related plasma metabolomic profile changes [55, 140]. ω-3 LC-PUFAs supplementation has diverse kinetic profiles depending on the formulation [141]. A growing understanding of the immunomodulatory effects of nutritional components and immunosenescence opens the field to complementary therapy to antibiotics. If generation of SPMs is a crucial mechanism of action of EPA and DHA in the prevention and treatment of inflammatory conditions, then it is important to understand more about the most effective strategies by which EPA and DHA can increase SPM concentrations.
10Conclusions
Considering the impaired immune answer, increased risk of RTI, and low ω-3 LC-PUFAs intake of older subjects, there is an urgent need to examine the potential effects of these molecules on infectious processes in light of secondary SPMs production. Indeed, outcomes from bioactive lipids derived seem to mitigate bacterial-induced pneumonia injury. However, the results of studies using ω-3 LC-PUFAs in the case of influenza and mycobacterial infections are conflicting.
Abbreviations
AA: Arachidonic Acid
ALA: Alpha linolenic acid
ALI: Acute lung injury
ARDS: Acute respiratory distress syndrome
AT-RvD1: Aspirin triggered- Resolvin D1
BGI: Bacterial Growth Index
BALF: Bronchoalveolar lavage fluid
CFTR: Cystic fibrosis transmembrane conductance regulator
CoQ: Coenzyme Q10
COVID-19: Coronavirus disease 20–19
COX-2 ASA: Cyclooxygenase-2 aspirin-acetylated
DHA: Docosahexaenoic acid
10S, 17S-di HDHA: 10S, 17S -dihydroxy hydroxydocosahexaenoic acid
EPA: Eicosapentaenoic acid
GLA: Gamma linolenic acid
HDHA: Hydroxydocosahexaenoic acid
HEPE: Hydroxyeicosapentaenoic acid
HETE: Hydroxyeicosatetraenoic acid
HMGB-1: High-mobility group box 1
ICU: Intensive care unit
IFN-γ: Interferon-γ
IL-1β: Interleukin -1β
IL-10: Interleukin 10
IL-6: Interleukin 6
KC: keratinocyte-derived chemokine
LA: Linolenic acid
LC-PUFA: Long chain polyunsaturated fatty acid
LOX: Lipoxygenase
LPS: Lipopolysaccharide
LTA4 hydrolase: leukotriene A4 hydrolase
LXA4: Lipoxin A4
LXB5: Lipoxin B5
MaR1: Maresin 1
MCP-1: Monocyte chemoattractant protein 1
MIP-1α: Macrophage inflammatory protein-1α
MIP-1β: Macrophage inflammatory protein-1β
MPO: Myeloperoxidase
NF-kB: Nuclear factor-kB
PAMP: pathogen-associated molecular pattern
PCTR1, Protectin conjugates in tissue regeneration 1
PD1: Protectin D1
PGE2: Prostaglandin E2
PMN: Polymorphonuclear neutrophil
PUFA: Polyunsaturated fatty acids
ω -3 PUFAs: n-3 polyunsaturated fatty acids
ω-6 PUFAs: n-6 polyunsaturated fatty acids
RCT: Randomized controlled trial
Ri: Resolution interval
RTI: Respiratory tract infection
RvD1: Resolvin D1
RvE1: Resolvin E1
SPMs: Specialized pro-resolving mediators
TB: Tuberculosis
TLR-4: Toll-like receptor-4
TNF-α: Tumor Necrosis Factor- α
Acknowledgments
The author has no acknowledgments
Funding
The author reports no funding
Author contributions
All authors had a substantial contribution to the work, approve the final version to be published and agreed to be accountable for the accuracy and integrity of this review.
Name | Location | Role | Contribution |
Kéziah Korpak | Department of Geriatric Medicine, CHU de Charleroi, Université libre de Bruxelles (ULB), Charleroi, Belgium | Author | Drafting and revision of manuscript for important intellectual content |
Maxime Rossi | Department of Urology, CHU de Charleroi, A. Vésale Hospital, Université libre de Bruxelles (ULB), Montigny-le-Tilleul, Belgium. | Author | Drafting and/or revision of manuscript for important intellectual content |
Alain Van Meerhaeghe | Laboratory of Experimental Medicine (ULB 222 Unit), CHU de Charleroi, A. Vésale Hospital, Université libre de Bruxelles (ULB), Montigny-le-Tilleul, Belgium | Author | Drafting and/or revision of manuscript for important intellectual content |
Karim Zouaoui Boudjeltia | Laboratory of Experimental Medicine (ULB 222 Unit), CHU de Charleroi, A. Vésale Hospital, Université libre de Bruxelles (ULB), Montigny-le-Tilleul, Belgium | Author | Drafting and/or revision of manuscript for important intellectual content |
Michele Compagnie | Department of Geriatric Medicine, CHU de Charleroi, Université libre de Bruxelles (ULB), Charleroi, Belgium | Author | Drafting and/or revision of manuscript for important intellectual content |
Conflict of interest
The author has no conflict of interest to report
References
[1] | Cassini A , Colzani E , Pini A , Mangen MJ , Plass D , McDonald SA , Maringhini G , van Lier A , Haagsma JA , Havelaar AH , Kramarz P , Kretzschmar ME On Behalf Of The BCoDE Consortium Impact of infectious diseases on population health using incidence-based disability-adjusted life years (DALYs): results from the Burden of Communicable Diseases in Europe study, European Union and European Economic Area countries, 2009 to 2013. Euro Surveill. (2018) ;23: (16):17–00454. |
[2] | European Centre for Disease Prevention and Control. Seasonal influenza 2019-2020. In: ECDC. Annual epidemiological report for 2019. Stockholm: ECDC; 2020. Available from: https://www.ecdc.europa.eu/sites/default/files/documents/AER_for_2019_influenza-seasonal.pdf. |
[3] | Adlhoch C , Gomes Dias J , Bonmarin I , Hubert B , Larrauri A , Oliva Domínguez JA , Delgado-Sanz C , Brytting M , Carnahan A , Popovici O , Lupulescu E , O’Donnell J , Domegan L , Van Gageldonk-Lafeber AB , Meijer A , Kyncl J , Slezák P , Guiomar R , Orta Gomes CM , Popow-Kraupp T , Mikas J , Staronová E , Melillo JM , Melillo T , Ikonen N , Lyytikäinen O , Snacken R , Penttinen P . Determinants of Fatal Outcome in Patients Admitted to Intensive Care Units With Influenza, European Union 2009-2017. Open Forum Infect Dis. (2019) ;6: (11):462. |
[4] | Murphy SL , Kochanek KD , Xu JQ , Arias E Mortality in the United States, 2020. NCHS Data Brief, no 427. Hyattsville, MD: National Center for Health Statistics. 2021. Available from: https://www.cdc.gov/nchs/data/databriefs/db427.pdf |
[5] | Serra-Prat M , Palomera M , Gomez C , Sar-Shalom D , Saiz A , Montoya JG , Navajas M , Palomera E , Clavé P . Oropharyngeal dysphagia as a risk factor for malnutrition and lower respiratory tract infection in independently living older persons: a population-based prospective study. Age Ageing. (2012) ;41: (3):376–81. |
[6] | Corrales-Medina VF , Alvarez KN , Weissfeld LA , Angus DC , Chirinos JA , Chang CC , Newman A , Loehr L , Folsom AR , Elkind MS , Lyles MF , Kronmal RA , Yende S . Association between hospitalization for pneumonia and subsequent risk of cardiovascular disease. JAMA. (2015) ;313: (3):264–74. |
[7] | Janssens JP , Pache JC , Nicod LP . Physiological changes in respiratory function associated with ageing. Eur Respir J. (1999) ;13: (1):197–205. |
[8] | Williamson EJ , Walker AJ , Bhaskaran K , Bacon S , Bates C , Morton CE , Curtis HJ , Mehrkar A , Evans D , Inglesby P , Cockburn J , McDonald HI , MacKenna B , Tomlinson L , Douglas IJ , Rentsch CT , Mathur R , Wong AYS , Grieve R , Harrison D , Forbes H , Schultze A , Croker R , Parry J , Hester F , Harper S , Perera R , Evans SJW , Smeeth L , Goldacre B . Factors associated with COVID-19-related death using OpenSAFELY. Nature. (2020) ;584: (7821):430–436. |
[9] | Yang Y , Shen C , Li J , Yuan J , Wei J , Huang F , Wang F , Li G , Li Y , Xing L , Peng L , Yang M , Cao M , Zheng H , Wu W , Zou R , Li D , Xu Z , Wang H , Zhang M , Zhang Z , Gao GF , Jiang C , Liu L , Liu Y . Plasma IP-10 and MCP-3 levels are highly associated with disease severity and predict the progression of COVID-19. J Allergy Clin Immunol. (2020) ;146: (1):119–127.e4. |
[10] | Chi Y , Ge Y , Wu B , Zhang W , Wu T , Wen T , Liu J , Guo X , Huang C , Jiao Y , Zhu F , Zhu B , Cui L . Serum Cytokine and Chemokine Profile in Relation to the Severity of Coronavirus Disease 2019 in China. J Infect Dis. (2020) ;222: (5):746–54. |
[11] | Cereda E , Pedrolli C , Klersy C , Bonardi C , Quarleri L , Cappello S , Turri A , Rondanelli M , Caccialanza R . Nutritional status in older persons according to healthcare setting: A systematic review and meta-analysis of prevalence data using MNA®. Clin Nutr. (2016) ;35: (6):1282–90. |
[12] | Schiffman SS , Graham BG . Taste and smell perception affect appetite and immunity in the elderly. Eur J Clin Nutr. (2000) ;54: (Suppl 3):S54–63. |
[13] | van Bokhorst-de van der Schueren MA , Lonterman-Monasch S , de Vries OJ , Danner SA , Kramer MH , Muller M . Prevalence and determinants for malnutrition in geriatric outpatients. Clin Nutr. (2013) ;32: (6):1007–11. |
[14] | Pedersen AML , Dynesen AW , Heitmann BL . Older age, smoking, tooth loss and denture-wearing but neither xerostomia nor salivary gland hypofunction are associated with low intakes of fruit and vegetables in older Danish adults. J Nutr Sci. (2021) ;10: :e47. |
[15] | Besora-Moreno M , Llauradó E , Tarro L , Solà R . Social and Economic Factors and Malnutrition or the Risk of Malnutrition in the Elderly: A Systematic Review and Meta-Analysis of Observational Studies. Nutrients. (2020) ;12: (3):737. |
[16] | Smoliner C , Norman K , Wagner KH , Hartig W , Lochs H , Pirlich M . Malnutrition and depression in the institutionalised elderly. Br J Nutr. (2009) ;102: (11):1663–7. |
[17] | Pourhassan M , Babel N , Sieske L , Westhoff TH , Wirth R . Longitudinal Changes of Cytokines and Appetite in Older Hospitalized Patients. Nutrients. (2021) ;13: (8):2508. |
[18] | MacIntosh CG , Morley JE , Wishart J , Morris H , Jansen JB , Horowitz M , Chapman IM . Effect of exogenous cholecystokinin (CCK)-8 on food intake and plasma CCK, leptin, and insulin concentrations in older and young adults: evidence for increased CCK activity as a cause of the anorexia of aging. J Clin Endocrinol Metab. (2001) ;86: (12):5830–7. |
[19] | Bernstein M , Munoz N Academy of Nutrition and Dietetics Position of the Academy of Nutrition and Dietetics: food and nutrition for older adults: promoting health and wellness. J Acad Nutr Diet. (2012) ;112: (8):1255–77. |
[20] | Chandra RK . Nutritional regulation of immunity and risk of infection in old age. Immunology. (1989) ;67: (2):141–7. |
[21] | Radzikowska U , Rinaldi AO , Celebi Sözener Z , Karaguzel D , Wojcik M , Cypryk K , Akdis M , Akdis CA , Sokolowska M . The Influence of Dietary Fatty Acids on Immune Responses. Nutrients. (2019) ;11: (12):2990. |
[22] | Strandberg L , Verdrengh M , Enge M , Andersson N , Amu S , Onnheim K , Benrick A , Brisslert M , Bylund J , Bokarewa M , Nilsson S , Jansson JO . Mice chronically fed high-fat diet have increased mortality and disturbed immune response in sepsis. PLoS One. (2009) ;4: (10):e7605. |
[23] | Alperovich M , Neuman MI , Willett WC , Curhan GC . Fatty acid intake and the risk of community-acquired pneumonia in U. S. women. Nutrition. (2007) ;23: (3):196–202. |
[24] | Merchant AT , Curhan GC , Rimm EB , Willett WC , Fawzi WW . Intake of n-6 and n-3 fatty acids and fish and risk of community-acquired pneumonia in US men. Am J Clin Nutr. (2005) ;82: (3):668–74. |
[25] | Keser I , Cvijetić S , Ilić A , Colić Barić I , Boschiero D , Ilich JZ . Assessment of Body Composition and Dietary Intake in Nursing-Home Residents: Could Lessons Learned from the COVID-19 Pandemic Be Used to Prevent Future Casualties in Older Individuals? Nutrients (2021) ;13: (5):1510. |
[26] | European Food Safety Authority. Scientific opinion on dietary reference values for fats, including saturated fatty acids, polyunsaturated fatty acids, monounsaturated fatty acids, trans fatty acids and cholesterol. EFSA J. 2010, 8, 1461.Available from: https://www.efsa.europa.eu/fr/efsajournal/pub/1461 |
[27] | Gavazzi G , Krause KH . Ageing and infection. Lancet Infect Dis. (2002) ;2: (11):659–66. |
[28] | Verschoor CP , Johnstone J , Loeb M , Bramson JL , Bowdish DM . Anti-pneumococcal deficits of monocyte-derived macrophages from the advanced-age, frail elderly and related impairments in PI3K-AKT signaling. Hum Immunol. (2014) ;75: (12):1192–6. |
[29] | Koike E , Kobayashi T , Mochitate K , Murakami M . Effect of aging on nitric oxide production by rat alveolar macrophages. Exp Gerontol. (1999) ;34: (7):889–94. |
[30] | Fagnoni FF , Vescovini R , Passeri G , Bologna G , Pedrazzoni M , Lavagetto G , Casti A , Franceschi C , Passeri M , Sansoni P . Shortage of circulating naive CD8(+) T cells provides new insights on immunodeficiency in aging. Blood. (2000) ;95: (9):2860–8. |
[31] | Nasi M , Troiano L , Lugli E , Pinti M , Ferraresi R , Monterastelli E , Mussi C , Salvioli G , Franceschi C , Cossarizza A . Thymic output and functionality of the IL-7/IL-7 receptor system in centenarians: implications for the neolymphogenesis at the limit of human life. Aging Cell. (2006) ;5: (2):167–75. |
[32] | Aberle JH , Stiasny K , Kundi M , Heinz FX . Mechanistic insights into the impairment of memory B cells and antibody production in the elderly. Age (Dordr). (2013) ;35: (2):371–81. |
[33] | GBD 2016 Lower Respiratory Infections Collaborators Estimates of the global, regional, and national morbidity, mortality, and aetiologies of lower respiratory infections in 195 countries, 1990-2016: a systematic analysis for the Global Burden of Disease Study 2016. Lancet Infect Dis. (2018) ;18: (11):1191–210. |
[34] | Shivshankar P , Boyd AR , Le Saux CJ , Yeh IT , Orihuela CJ . Cellular senescence increases expression of bacterial ligands in the lungs and is positively correlated with increased susceptibility to pneumococcal pneumonia. Aging Cell. (2011) ;10: (5):798–806. |
[35] | Hinojosa E , Boyd AR , Orihuela CJ . Age-associated inflammation and toll-like receptor dysfunction prime the lungs for pneumococcal pneumonia. J Infect Dis. (2009) ;200: (4):546–54. |
[36] | Meyer KC , Rosenthal NS , Soergel P , Peterson K . Neutrophils and low-grade inflammation in the seemingly normal aging human lung. Mech Ageing Dev. (1998) ;104: (2):169–81. |
[37] | Meyer KC , Soergel P . Variation of bronchoalveolar lymphocyte phenotypes with age in the physiologically normal human lung. Thorax. (1999) ;54: (8):697–700. |
[38] | Angus DC , van der Poll T . Severe sepsis and septic shock. N Engl J Med. (2013) ;369: (9):840–51. |
[39] | Cilloniz C , Pantin-Jackwood MJ , Ni C , Goodman AG , Peng X , Proll SC , Carter VS , Rosenzweig ER , Szretter KJ , Katz JM , Korth MJ , Swayne DE , Tumpey TM , Katze MG . Lethal dissemination of H5N1 influenza virus is associated with dysregulation of inflammation and lipoxin signaling in a mouse model of infection. J Virol.. (2010) ;84: (15):7613–24. |
[40] | Corsini E , Di Paola R , Viviani B , Genovese T , Mazzon E , Lucchi L , Marinovich M , Galli CL , Cuzzocrea S . Increased carrageenan-induced acute lung inflammation in old rats. Immunology. (2005) ;115: (2):253–61. |
[41] | Serhan CN , Hong S , Gronert K , Colgan SP , Devchand PR , Mirick G , Moussignac RL . Resolvins: a family of bioactive products of omega-3 fatty acid transformation circuits initiated by aspirin treatment that counter pro-inflammation signals. J Exp Med. (2002) ;196: :1025–37. |
[42] | Arnardottir HH , Dalli J , Colas RA , Shinohara M , Serhan CN . Aging delays resolution of acute inflammation in mice: reprogramming the host response with novel nano-proresolving medicines. J Immunol. (2014) ;193: (8):4235–44. |
[43] | Chiang N , Serhan CN . Specialized pro-resolving mediator network: an update on production and actions. Essays Biochem. (2020) ;64: (3):443–62. |
[44] | Dufour D , Khalil A , Nuyens V , Rousseau A , Delporte C , Noyon C , Cortese M , Reyé F , Pireaux V , Nève J , Vanhamme L , Robaye B , Lelubre C , Desmet JM , Raes M , Boudjeltia KZ , Van Antwerpen P . Native and myeloperoxidase-oxidized low-density lipoproteins act in synergy to induce release of resolvin-D1 from endothelial cells. Atherosclerosis. (2018) ;272: :108–17. |
[45] | Sobrino A , Walker ME , Colas RA , Dalli J . Protective activities of distinct omega-3 enriched oils are linked to their ability to upregulate specialized pro-resolving mediators. PLoS One. (2020) ;15: (12):e0242543. |
[46] | Gutiérrez S , Svahn SL , Johansson ME . Effects of Omega-3 Fatty Acids on Immune Cells. Int J Mol Sci. (2019) ;20: (20):5028. |
[47] | Bouwens M , van de Rest O , Dellschaft N , Bromhaar MG , de Groot LC , Geleijnse JM , Müller M , Afman LA . Fish-oil supplementation induces antiinflammatory gene expression profiles in human blood mononuclear cells. Am J Clin Nutr. (2009) ;90: (2):415–24. |
[48] | Zárate R , El Jaber-Vazdekis N , Tejera N , Pérez JA , Rodríguez C . Significance of long chain polyunsaturated fatty acids in human health. Clin Transl Med. (2017) ;6: (1):25. |
[49] | Kasuga K , Yang R , Porter TF , Agrawal N , Petasis NA , Irimia D , Toner M , Serhan CN . Rapid appearance of resolvin precursors in inflammatory exudates: novel mechanisms in resolution. J Immunol. (2008) ;181: (12):8677–87. |
[50] | Das U Bioactive Lipids in Age-Related Disorders: Springer Nature Switzerland AG; 2020. pp. 33-84. |
[51] | Wang X , Zhu M , Hjorth E , Cortés-Toro V , Eyjolfsdottir H , Graff C , Nennesmo I , Palmblad J , Eriksdotter M , Sambamurti K , Fitzgerald JM , Serhan CN , Granholm AC , Schultzberg M . Resolution of inflammation is altered in Alzheimer’s disease. Alzheimers Dement. (2015) ;11: (1):40–50.e1-2. |
[52] | Ho KJ , Spite M , Owens CD , Lancero H , Kroemer AH , Pande R , Creager MA , Serhan CN , Conte MS . Aspirin-triggered lipoxin and resolvin E1 modulate vascular smooth muscle phenotype and correlate with peripheral atherosclerosis. Am J Pathol. (2010) ;177: (4):2116–23. |
[53] | Sanak M , Levy BD , Clish CB , Chiang N , Gronert K , Mastalerz L , Serhan CN , Szczeklik A . Aspirin-tolerant asthmatics generate more lipoxins than aspirin-intolerant asthmatics. Eur Respir J. (2000) ;16: (1):44–9. |
[54] | Rees D , Rees D , Miles EA , Banerjee T , Wells SJ , Roynette CE , Wahle KW , Calder PC . Dose-related effects of eicosapentaenoic acid on innate immune function in healthy humans: a comparison of young and older men. Am J Clin Nutr.331-42. (2006) ;83: (2). doi: 10.1093/ajcn/83.2.331. |
[55] | Léveillé P , Chouinard-Watkins R , Windust A , Lawrence P , Cunnane SC , Brenna JT , Plourde M . Metabolism of uniformly labeled 13C-eicosapentaenoic acid and 13C-arachidonic acid in young and old men. Am J Clin Nutr. (2017) ;106: (2):467–74. |
[56] | Simons K , Toomre D . Lipid rafts and signal transduction. Nat Rev Mol Cell Biol. (2000) ;1: (1):31–9. |
[57] | Larbi A , Douziech N , Dupuis G et al. Age-associated alterations in the recruitment of signaltransduction proteins to lipid rafts in human T lymphocytes. J Leukoc Biol. (2004) ;75: :373–81. |
[58] | Wong SW , Kwon MJ , Choi AM , Kim HP , Nakahira K , Hwang DH . Fatty acids modulate Toll-like receptor 4 activation through regulation of receptor dimerization and recruitment into lipid rafts in a reactive oxygen species-dependent manner. J Biol Chem. (2009) ;284: (40):27384–92. |
[59] | Sharma S , Chhibber S , Mohan H , Sharma S . Dietary supplementation with omega-3 polyunsaturated fatty acids ameliorates acute pneumonia induced by Klebsiella pneumoniae in BALB/c mice. Can J Microbiol. (2013) ;59: (7):503–10. |
[60] | Thors VS , Thórisdóttir A , Erlendsdóttir H , Einarsson I , Gudmundsson S , Gunnarsson E , Haraldsson A . The effect of dietary fish oil on survival after infection with Klebsiella pneumoniae or Streptococcus pneumoniae. Scand J Infect Dis. (2004) ;36: (2):102–5. |
[61] | Saini A , Harjai K , Mohan H , Punia RP , Chhibber S . Long-term flaxseed oil supplementation diet protects BALB/c mice against Streptococcus pneumoniae infection. Med Microbiol Immunol. (2010) ;199: (1):27–34. |
[62] | Saini A , Harjai K , Chhibber S . Sea-cod oil supplementation alters the course of Streptococcus pneumoniae infection in BALB/c mice. Eur J Clin Microbiol Infect Dis. (2011) ;30: (3):393–400. |
[63] | Hinojosa CA , Gonzalez-Juarbe N , Rahman MM , Fernandes G , Orihuela CJ , Restrepo MI . Omega-3 fatty acids in contrast to omega-6 protect against pneumococcal pneumonia. Microb Pathog. (2020) ;141: :103979. |
[64] | Malhotra S , Hayes D Jr , Wozniak DJ . Cystic Fibrosis and Pseudomonas aeruginosa: the Host-Microbe Interface. Clin Microbiol Rev. (2019) ;32: (3):e00138–18. |
[65] | Freedman SD , Blanco PG , Zaman MM , Shea JC , Ollero M , Hopper IK , Weed DA , Gelrud A , Regan MM , Laposata M , Alvarez JG , O’Sullivan BP . Association of cystic fibrosis with abnormalities in fatty acid metabolism. N Engl J Med. (2004) ;350: (6):560–9. |
[66] | Freedman SD , Weinstein D , Blanco PG , Martinez-Clark P , Urman S , Zaman M , Morrow JD , Alvarez JG . Characterization of LPS-induced lung inflammation in cftr-/- mice and the effect of docosahexaenoic acid. J Appl Physiol. (2002) ;92: (5):2169–76. |
[67] | Pierre M , Husson MO , Le Berre R , Desseyn JL , Galabert C , Béghin L , Beermann C , Dagenais A , Berthiaume Y , Cardinaud B , Barbry P , Gottrand F , Guery BP . Omega-3 polyunsaturated fatty acids improve host response in chronic Pseudomonas aeruginosa lung infection in mice. Am J Physiol Lung Cell Mol Physiol. (2007) ;292: (6):L1422–31. |
[68] | Tiesset H , Pierre M , Desseyn JL , Guéry B , Beermann C , Galabert C , Gottrand F , Husson MO . Dietary (n-3) polyunsaturated fatty acids affect the kinetics of pro- and antiinflammatory responses in mice with Pseudomonas aeruginosa lung infection. J Nutr.. (2009) ;139: (1):82–9. |
[69] | Caron E , Desseyn JL , Sergent L , Bartke N , Husson MO , Duhamel A , Gottrand F . Impact of fish oils on the outcomes of a mouse model of acute Pseudomonas aeruginosa pulmonary infection. Br J Nutr. (2015) ;113: (2):191–9. |
[70] | Xin H , Zhang H , Liu J , Pan S , Li X , Cao X , Feng B , Guan L , Shen F , Liu Z , Wang D , Yan J , Zhang M , Yang Q , Jin Q , Gao L . Mycobacterium Tuberculosis infection among the elderly in 20 486 rural residents aged 50-70 years in Zhongmu County, China. Clin Microbiol Infect. (2019) ;25: (9):1120–6. |
[71] | McFarland CT , Fan YY , Chapkin RS , Weeks BR , McMurray DN . Dietary polyunsaturated fatty acids modulate resistance to Mycobacterium tuberculosis in guinea pigs. J Nutr. (2008) ;138: (11):2123–8. |
[72] | Jordao L , Lengeling A , Bordat Y , Boudou F , Gicquel B , Neyrolles O , Becker PD , Guzman CA , Griffiths G , Anes E . Effects of omega-3 and -6 fatty acids on Mycobacterium tuberculosis in macrophages and in mice. Microbes Infect. (2008) ;10: (12-13):1379–86. |
[73] | Husson MO , Ley D , Portal C , Gottrand M , Hueso T , Desseyn JL , Gottrand F . Modulation of host defence against bacterial and viral infections by omega-3 polyunsaturated fatty acids. J Infect. (2016) ;73: (6):523–535.d |
[74] | Proust F , Lucas M , Dewailly E . Fatty acid profiles among the Inuit of Nunavik: current status and temporal change. Prostaglandins Leukot Essent Fatty Acids. (2014) ;90: (5):159–67. |
[75] | Vachon J , Gallant V , Siu W . Tuberculosis in Canada, 2016. Can Commun Dis Rep. (2018) ;44: (3-4):75–81. |
[76] | Soh AZ , Chee CB , Wang YT , Yuan JM , Koh WP . Dietary Cholesterol Increases the Risk whereas PUFAs Reduce the Risk of Active Tuberculosis in Singapore Chinese. J Nutr. (2016) ;146: (5):1093–100. |
[77] | Byleveld PM , Pang GT , Clancy RL , Roberts DC . Fish oil feeding delays influenza virus clearance and impairs production of interferon-gamma and virus-specific immunoglobulin A in the lungs of mice. J Nutr. (1999) ;129: (2):328–35. |
[78] | Schwerbrock NM , Karlsson EA , Shi Q , Sheridan PA , Beck MA . Fish oil-fed mice have impaired resistance to influenza infection. J Nutr. (2009) ;139: (8):1588–94. |
[79] | Morita M , Kuba K , Ichikawa A , Nakayama M , Katahira J , Iwamoto R , Watanebe T , Sakabe S , Daidoji T , Nakamura S , Kadowaki A , Ohto T , Nakanishi H , Taguchi R , Nakaya T , Murakami M , Yoneda Y , Arai H , Kawaoka Y , Penninger JM , Arita M , Imai Y . The lipid mediator protectin D1 inhibits influenza virus replication and improves severe influenza. Cell. (2013) ;153: (1):112–25. |
[80] | Levy BD , Serhan CN . Resolution of acute inflammation in the lung. Annu Rev Physiol. (2014) ;76: :467–92. |
[81] | Arita M , Ohira T , Sun YP , Elangovan S , Chiang N , Serhan CN . Resolvin E1 selectively interacts with leukotriene B4 receptor BLT1 and ChemR23 to regulate inflammation. J Immunol. (2007) ;178: (6):3912–7. |
[82] | Maddox JF , Hachicha M , Takano T , Petasis NA , Fokin VV , Serhan CN . Lipoxin A4 stable analogs are potent mimetics that stimulate human monocytes and THP-1 cells via a G-protein-linked lipoxin A4 receptor. J Biol Chem. (1997) ;272: (11):6972–8. |
[83] | Mitchell S , Thomas G , Harvey K , Cottell D , Reville K , Berlasconi G , Petasis NA , Erwig L , Rees AJ , Savill J , Brady HR , Godson C . Lipoxins, aspirin-triggered epi-lipoxins, lipoxin stable analogues, and the resolution of inflammation: stimulation of macrophage phagocytosis of apoptotic neutrophils in vivo. J Am Soc Nephrol. (2002) ;13: (10):2497–507. |
[84] | Krishnamoorthy N , Abdulnour RE , Walker KH , Engstrom BD , Levy BD . Specialized Proresolving Mediators in Innate and Adaptive Immune Responses in Airway Diseases. Physiol Rev. (2018) ;98: (3):1335–70. |
[85] | Bonnans C , Fukunaga K , Levy MA , Levy BD . Lipoxin A(4) regulates bronchial epithelial cell responses to acid injury. Am J Pathol. (2006) ;168: (4):1064–72. |
[86] | Fang X , Abbott J , Cheng L , Colby JK , Lee JW , Levy BD , Matthay MA . Human Mesenchymal Stem (Stromal) Cells Promote the Resolution of Acute Lung Injury in Part through Lipoxin A4. J Immunol.. (2015) ;195: (3):875–81. |
[87] | Levy BD , Romano M , Chapman HA , Reilly JJ , Drazen J , Serhan CN . Human alveolar macrophages have 15-lipoxygenase and generate S)-hydroxy-5,8,11-cis-13-trans-eicosatetraenoic acid and lipoxins. J Clin Invest. (1993) ;92: (3):1572–9. |
[88] | Chiang N , Fredman G , Bäckhed F , Oh SF , Vickery T , Schmidt BA , Serhan CN . Infection regulates pro-resolving mediators that lower antibiotic requirements. Nature. (2012) ;484: (7395):524–8. |
[89] | Dalli J , Kraft BD , Colas RA , Shinohara M , Fredenburgh LE , Hess DR , Chiang N , Welty-Wolf K , Choi AM , Piantadosi CA , Serhan CN . The Regulation of Proresolving Lipid Mediator Profiles in Baboon Pneumonia by Inhaled Carbon Monoxide. Am J Respir Cell Mol Biol.. (2015) ;53: (3):314–25. |
[90] | Marik PE . Aspiration pneumonitis and aspiration pneumonia. N Engl J Med. (2001) ;344: (9):665–71. |
[91] | Seki H , Fukunaga K , Arita M , Arai H , Nakanishi H , Taguchi R , Miyasho T , Takamiya R , Asano K , Ishizaka A , Takeda J , Levy BD . The anti-inflammatory and proresolving mediator resolvin E1 protects mice from bacterial pneumonia and acute lung injury. J Immunol. (2010) ;184: (2), 836–43.d |
[92] | Sun YP , Oh SF , Uddin J , Yang R , Gotlinger K , Campbell E , Colgan SP , Petasis NA , Serhan CN . Resolvin D1 and its aspirin-triggered 17R epimer. Stereochemical assignments, anti-inflammatory properties, and enzymatic inactivation. J Biol Chem. (2007) ;282: (13):9323–34. |
[93] | Abdulnour RE , Sham HP , Douda DN , Colas RA , Dalli J , Bai Y , Ai X , Serhan CN , Levy BD . Aspirin-triggered resolvin D1 is produced during self-resolving gram-negative bacterial pneumonia and regulates host immune responses for the resolution of lung inflammation. Mucosal Immunol. (2016) ;9: (5):1278–87. |
[94] | Wang H , Anthony D , Yatmaz S , Wijburg O , Satzke C , Levy B , Vlahos R , Bozinovski S . Aspirin-triggered resolvin D1 reduces pneumococcal lung infection and inflammation in a viral and bacterial coinfection pneumonia model. Clin Sci (Lond). (2017) ;131: (18):2347–62. |
[95] | Sekheri M , Sekheri M , El Kebir D , Edner N , Filep JG . 15-Epi-LXA4 and 17-epi-RvD1 restore TLR9-mediated impaired neutrophil phagocytosis and accelerate resolution of lung inflammation. Proc Natl Acad Sci U S A. (2020) ;117: (14):7971–80. |
[96] | Codagnone M , Cianci E , Lamolinara A , Mari VC , Nespoli A , Isopi E , Mattoscio D , Arita M , Bragonzi A , Iezzi M , Romano M , Recchiuti A . Resolvin D1 enhances the resolution of lung inflammation caused by long-term Pseudomonas aeruginosa infection. Mucosal Immunol. (2018) ;11: (1):35–49. |
[97] | European Centre for Disease Prevention and Control. Healthcare-associated infections in intensive care units - annual epidemiological report for 2016. Stockholm: ECDC. Available from: https://ecdc.europa.eu/sites/portal/files/documents/AER_for_2016-HAI 0.pdf-n.d. |
[98] | Garcia-Clemente M , de la Rosa D , Máiz L , Girón R , Blanco M , Olveira C , Canton R , Martinez-García MA . Impact of Pseudomonas aeruginosa Infection on Patients with Chronic Inflammatory Airway Diseases. J Clin Med. (2020) ;9: (12):3800. |
[99] | Imai Y . Role of omega-3 PUFA-derived mediators, the protectins, in influenza virus infection. Biochim Biophys Acta. (2015) ;1851: (4):496–502. |
[100] | Walker KH , Krishnamoorthy N , Brüggemann TR , Shay AE , Serhan CN , Levy BD . Protectins PCTR1 and PD1 Reduce Viral Load and Lung Inflammation During Respiratory Syncytial Virus Infection in Mice. Front Immunol. (2021) ;12: :704427. |
[101] | Frediani JK , Jones DP , Tukvadze N , Uppal K , Sanikidze E , Kipiani M , Tran VT , Hebbar G , Walker DI , Kempker RR , Kurani SS , Colas RA , Dalli J , Tangpricha V , Serhan CN , Blumberg HM , Ziegler TR . Plasma metabolomics in human pulmonary tuberculosis disease: a pilot study. PLoS One. (2014) ;9: (10):e108854. |
[102] | Ruiz A , Sarabia C , Torres M , Juárez E . Resolvin D1 (RvD1) and maresin 1 (Mar1) contribute to human macrophage control of M. tuberculosis infection while resolving inflammation. Int Immunopharmacol. (2019) ;74: :105694. |
[103] | Bafica A , Scanga CA , Serhan C , Machado F , White S , Sher A , Aliberti J . Host control of Mycobacterium tuberculosis is regulated by 5-lipoxygenase-dependent lipoxin production. J Clin Invest. (2005) ;115: (6):1601–6. |
[104] | Sandhaus S , Swick AG . Specialized proresolving mediators in infection and lung injury. Biofactors. (2021) ;47: (1):6–18. |
[105] | Angus DC , Linde-Zwirble WT , Lidicker J , Clermont G , Carcillo J , Pinsky MR . Epidemiology of severe sepsis in the United States: analysis of incidence, outcome, and associated costs of care. Crit Care Med.. (2001) ;29: (7):1303–10. |
[106] | Bruegel M , Ludwig U , Kleinhempel A , Petros S , Kortz L , Ceglarek U , Holdt LM , Thiery J , Fiedler GM . Sepsis-associated changes of the arachidonic acid metabolism and their diagnostic potential in septic patients. Crit Care Med. (2012) ;40: (5):1478–86. |
[107] | Mayer K , Schmidt R , Muhly-Reinholz M , Bögeholz T , Gokorsch S , Grimminger F , Seeger W . In vitro mimicry of essential fatty acid deficiency in human endothelial cells by TNFalpha impact of omega-3 versus omega-6 fatty acids. J Lipid Res. (2002) ;43: (6):944–51. |
[108] | Gadek JE , DeMichele SJ , Karlstad MD , Pacht ER , Donahoe M , Albertson TE , Van Hoozen C , Wennberg AK , Nelson JL , Noursalehi M . Effect of enteral feeding with eicosapentaenoic acid, gamma-linolenic acid, and antioxidants in patients with acute respiratory distress syndrome. Enteral Nutrition in ARDS Study GrouCrit Care Med. (1999) ;27: (8):1409–20. |
[109] | Dushianthan A , Cusack R , Burgess VA , Grocott MP , Calder PC . Immunonutrition for acute respiratory distress syndrome (ARDS) in adults. Cochrane Database Syst Rev.. (2019) ;1: (1):CD012041. |
[110] | Chen H , Wang S , Zhao Y , Luo Y , Tong H , Su L . Correlation analysis of omega-3 fatty acids and mortality of sepsis and sepsis-induced ARDS in adults: data from previous randomized controlled trials. Nutr J. (2018) ;17: (1):57. |
[111] | Rice TW , Wheeler AP , Thompson BT , deBoisblanc BP , Steingrub J , Rock P NIH NHLBI Acute Respiratory Distress Syndrome Network of Investigators Enteral omega-3 fatty acid, gamma-linolenic acid, and antioxidant supplementation in acute lung injury. JAMA. (2011) ;306: (14):1574–81. |
[112] | European Food Safety Authority (EFSA) Scientific Opinion on the Tolerable Upper Intake Level of eicosapentaenoic acid (EPA), docosahexaenoic acid (DHA) and docosapentaenoic acid (DPA). EFSA JournalAvailable from. (2815) ;10: (7). https://www.efsa.europa.eu/fr/efsajournal/pub/2815. |
[113] | Fullerton JN , O’Brien AJ , Gilroy DW . Lipid mediators in immune dysfunction after severe inflammation. Trends Immunol. (2014) ;35: (1):12–21. |
[114] | Gong J , Wu ZY , Qi H , Chen L , Li HB , Li B , Yao CY , Wang YX , Wu J , Yuan SY , Yao SL , Shang Y . Maresin 1 mitigates LPS-induced acute lung injury in mice. Br J Pharmacol. (2014) ;171: (14):3539–50. |
[115] | Serhan CN , Yang R , Martinod K , Kasuga K , Pillai PS , Porter TF , Oh SF , Spite M . Maresins: novel macrophage mediators with potent antiinflammatory and proresolving actions. J Exp Med. (2009) ;206: (1):15–23. |
[116] | El Kebir D , Gjorstrup P , Filep JG . Resolvin E1 promotes phagocytosis-induced neutrophil apoptosis and accelerates resolution of pulmonary inflammation. Proc Natl Acad Sci U S A. (2012) ;109: (37):14983–8. |
[117] | Sawatzky DA , Willoughby DA , Colville-Nash PR , Rossi AG . The involvement of the apoptosis-modulating proteins ERK 1/2, Bcl-xL and Bax in the resolution of acute inflammation in vivo. Am J Pathol. (2006) ;168: (1):33–41. |
[118] | Lucas CD , Dorward DA , Tait MA , Fox S , Marwick JA , Allen KC , Robb CT , Hirani N , Haslett C , Duffin R , Rossi AG . Downregulation of Mcl-1 has anti-inflammatory pro-resolution effects and enhances bacterial clearance from the lung. Mucosal Immunol. (2014) ;7: (4):857–68. |
[119] | Oh SF , Pillai PS , Recchiuti A , Yang R , Serhan CN . Pro-resolving actions and stereoselective biosynthesis of 18S E-series resolvins in human leukocytes and murine inflammation. J Clin Invest. (2011) ;121: (2):569–81. |
[120] | Colas RA , Shinohara M , Dalli J , Chiang N , Serhan CN . Identification and signature profiles for pro-resolving and inflammatory lipid mediators in human tissue. Am J Physiol Cell Physiol. (2014) ;307: (1):C39–54. |
[121] | Souza PR , Marques RM , Gomez EA , Colas RA , De Matteis R , Zak A , Patel M , Collier DJ , Dalli J . Enriched Marine Oil Supplements Increase Peripheral Blood Specialized Pro-Resolving Mediators Concentrations and Reprogram Host Immune Responses: A Randomized Double-Blind Placebo-Controlled Study. Circ Res. (2020) ;126: (1):75–90. |
[122] | Barden A , Mas E , Croft KD , Phillips M , Mori TA . Short-term n-3 fatty acid supplementation but not aspirin increases plasma proresolving mediators of inflammation, J. Lipid Res. (2014) ;55: (11):2401–7. |
[123] | Markworth JF , Kaur G , Miller EG , Larsen AE , Sinclair AJ , Maddipati KR , Cameron-Smith D . Divergent shifts in lipid mediator profile following supplementation with n-3 docosapentaenoic acid and eicosapentaenoic acid. FASEB J. (2016) ;30: (11):3714–25. |
[124] | Schaller MS , Zahner GJ , Gasper WJ , Harris WS , Conte MS , Hills NK , Grenon SM . Relationship between the omega-3 index and specialized pro-resolving lipid mediators in patients with peripheral arterial disease taking fish oil supplements. J Clin Lipidol. (2017) ;11: (5):1289–95. |
[125] | Grenon SM , Owens CD , Nosova EV , Hughes-Fulford M , Alley HF , Chong K , Perez S , Yen PK , Boscardin J , Hellmann J , Spite M , Conte MS . Short-Term, High-Dose Fish Oil Supplementation Increases the Production of Omega-3 Fatty Acid-Derived Mediators in Patients With Peripheral Artery Disease (the OMEGA-PAD I Trial). J Am Heart Assoc. (2015) ;4: (8):e002034. |
[126] | Poreba M , Mostowik M , Siniarski A , Golebiowska-Wiatrak R , Malinowski KP , Haberka M , Konduracka E , Nessler J , Undas A , Gajos G . Treatment with high-dose n-3 PUFAs has no effect on platelet function, coagulation, metabolic status or inflammation in patients with atherosclerosis and type 2 diabetes. Cardiovasc Diabetol. (2017) ;16: (1):50. |
[127] | Ramirez JL , Gasper WJ , Khetani SA , Zahner GJ , Hills NK , Mitchell PT , Sansbury BE , Conte MS , Spite M , Grenon SM . Fish Oil Increases Specialized Pro-resolving Lipid Mediators in PAD (The OMEGA-PAD II Trial). J Surg Res. (2019) ;238: :164–74. |
[128] | Schaller MS , Chen M , Colas RA , Sorrentino TA , Lazar AA , Grenon SM , Dalli J , Conte MS . Treatment With a Marine Oil Supplement Alters Lipid Mediators and Leukocyte Phenotype in Healthy Patients and Those With Peripheral Artery Disease. J Am Heart Assoc. (2020) ;9: (15):e016113. |
[129] | So J , Wu D , Lichtenstein AH , Tai AK , Matthan NR , Maddipati KR , Lamon-Fava S . EPA and DHA differentially modulate monocyte inflammatory response in subjects with chronic inflammation in part via plasma specialized pro-resolving lipid mediators: A randomized, double-blind, crossover study. Atherosclerosis. (2021) ;316: :90–98. |
[130] | Uno H , Furukawa K , Suzuki D , Shimizu H , Ohtsuka M , Kato A , Yoshitomi H , Miyazaki M . Immunonutrition suppresses acute inflammatory responses through modulation of resolvin E1 in patients undergoing major hepatobiliary resection. Surgery. (2016) ;160: (1):228–36. |
[131] | Wang X , Hjorth E , Vedin I et al. Effects of n-3 FA supplementation on the release of proresolving lipid mediators by blood mononuclear cells: the OmegAD study. J Lipid Res. (2015) ;56: (3):674–81. |
[132] | Barden AE , Shinde S , Burke V , Puddey IB , Beilin LJ , Irish AB , Watts GF , Mori TA . The effect of n-3 fatty acids and coenzyme Q10 supplementation on neutrophil leukotrienes, mediators of inflammation resolution and myeloperoxidase in chronic kidney disease. Prostaglandins Other Lipid Mediat. (2018) ;136: :1–8. |
[133] | Capó X , Martorell M , Sureda A , Tur JA , Pons A . Effects of dietary Docosahexaenoic, training and acute exercise on lipid mediators. J Int Soc Sports Nutr. (2016) ;13: :16. |
[134] | Fenton JI , Hord NG , Ghosh S , Gurzell EA . Immunomodulation by dietary long chain omega-3 fatty acids and the potential for adverse health outcomes. Prostaglandins Leukot Essent Fatty Acids. (2013) ;89: (6):379–90. |
[135] | Fritsche K . Important differences exist in the dose-response relationship between diet and immune cell fatty acids in humans and rodents. Lipids. (2007) ;42: (11):961–79. |
[136] | Welty FK , Schulte F , Alfaddagh A , Elajami TK , Bistrian BR , Hardt M . Regression of human coronary artery plaque is associated with a high ratio of (18-hydroxy-eicosapentaenoic acid + resolvin E1) to leukotriene B4. FASEB J. (2021) ;35: (4):e21448. |
[137] | Banoei MM , Vogel HJ , Weljie AM , Yende S , Angus DC , Winston BW . Plasma lipid profiling for the prognosis of 90-day mortality, in-hospital mortality, ICU admission, and severity in bacterial community-acquired pneumonia (CAP). Crit Care. (2020) ;24: (1):461. |
[138] | Morris T , Stables M , Colville-Nash P , Newson J , Bellingan G , de Souza PM , Gilroy DW . Dichotomy in duration and severity of acute inflammatory responses in humans arising from differentially expressed proresolution pathways. Proc Natl Acad Sci U S A. (2010) ;107: (19):8842–7. |
[139] | Bischoff-Ferrari HA , Vellas B , Rizzoli R , Kressig RW , da Silva JAP , Blauth M , Felson DT , McCloskey EV , Watzl B , Hofbauer LC , Felsenberg D , Willett WC , Dawson-Hughes B , Manson JE , Siebert U , Theiler R , Staehelin HB , de Godoi Rezende Costa Molino C , Chocano-Bedoya PO , Abderhalden LA , Egli A , Kanis JA , Orav EJ . DO-HEALTH Research GrouEffect of Vitamin D Supplementation, Omega-3 Fatty Acid Supplementation, or a Strength-Training Exercise Program on Clinical Outcomes in Older Adults: The DO-HEALTH Randomized Clinical Trial. JAMA. (2020) ;324: (18)::1855–68. |
[140] | Johnson LC , Martens CR , Santos-Parker JR , Bassett CJ , Strahler TR , Cruickshank-Quinn C , Reisdorph N , McQueen MB , Seals DR . Amino acid and lipid associated plasma metabolomic patterns are related to healthspan indicators with ageing. Clin Sci (Lond). (2018) ;132: (16):1765–77. |
[141] | Chevalier L , Plourde M . Comparison of pharmacokinetics of omega-3 fatty acid supplements in monoacylglycerol or ethyl ester in humans: a randomized controlled trial. Eur J Clin Nutr. (2021) ;75: (4):680–8. |
[142] | Barden AE , Mas E , Croft KD , Phillips M , Mori TA . Specialized proresolving lipid mediators in humans with the metabolic syndrome after n-3 fatty acids and aspirin. Am J Clin Nutr. (2015) ;102: (6):1357–64. |
[143] | Barden AE , Moghaddami M , Mas E , Phillips M , Cleland LG , Mori TA . Specialised pro-resolving mediators of inflammation in inflammatory arthritis. Prostaglandins Leukot Essent Fatty Acids. (2016) ;107: :24–9. |
[144] | Dawczynski C , Massey KA , Ness C , Kiehntopf M , Stepanow S , Platzer M , Grün M , Nicolaou A , Jahreis G . Randomized placebo-controlled intervention with n-3 LC-PUFA-supplemented yoghurt: effects on circulating eicosanoids and cardiovascular risk factors. Clin Nutr. (2013) ;32: (5):686–96. |
[145] | Elajami TK , Colas RA , Dalli J , Chiang N , Serhan CN , Welty FK . Specialized proresolving lipid mediators in patients with coronary artery disease and their potential for clot remodeling. FASEB J. (2016) ;30: (8):2792–801. |
[146] | Fischer R , Konkel A , Mehling H , Blossey K , Gapelyuk A , Wessel N , von Schacky C , Dechend R , Muller DN , Rothe M , Luft FC , Weylandt K , Schunck WH . Dietary omega-3 fatty acids modulate the eicosanoid profile in man primarily via the CYP-epoxygenase pathway. J Lipid Res. (2014) ;55: (6):1150–64. |
[147] | Lamon-Fava S , So J , Mischoulon D , Ziegler TR , Dunlop BW , Kinkead B , Schettler PJ , Nierenberg AA , Felger JC , Maddipati KR , Fava M , Rapaport MH . Dose- and time-dependent increase in circulating anti-inflammatory and pro-resolving lipid mediators following eicosapentaenoic acid supplementation in patients with major depressive disorder and chronic inflammation. Prostaglandins Leukot Essent Fatty Acids. (2021) ;164: :102219. |
[148] | Mas E , Croft KD , Zahra P , Barden A , Mori TA . Resolvins D1, D2, and other mediators of self-limited resolution of inflammation in human blood following n-3 fatty acid supplementation. Clin Chem. (2012) ;58: (10):1476–84. |
[149] | Mas E , Barden A , Burke V , Beilin LJ , Watts GF , Huang RC , Puddey IB , Irish AB , Mori TA . A randomized controlled trial of the effects of n-3 fatty acids on resolvins in chronic kidney disease. Clin Nutr. (2016) ;35: (2):331–6. |
[150] | Norris PC , Skulas-Ray AC , Riley I , Richter CK , Kris-Etherton PM , Jensen GL , Serhan CN , Maddipati KR . Identification of specialized pro-resolving mediator clusters from healthy adults after intravenous low-dose endotoxin and omega-3 supplementation: a methodological validation. Sci Rep. (2018) ;8: (1):18050. |
[151] | Polus A , Zapala B , Razny U , Gielicz A , Kiec-Wilk B , Malczewska-Malec M , Sanak M , Childs CE , Calder PC , Dembinska-Kiec A . Omega-3 fatty acid supplementation influences the whole blood transcriptome in women with obesity, associated with pro-resolving lipid mediator production. Biochim Biophys Acta. (2016) ;1861: (11):1746–55. |
[152] | Skarke C , Alamuddin N , Lawson JA , Li X , Ferguson JF , Reilly MP , FitzGerald GA . Bioactive products formed in humans from fish oils. J Lipid Res. (2015) ;56: (9):1808–20. |