Effects of a 4-week pecan-enriched diet on cognitive function in healthy older adults
Abstract
BACKGROUND:
Pecans are rich in nutrients known to benefit cognition.
OBJECTIVE:
To examine the effect of daily pecan consumption on cognitive function in older adults.
METHODS:
In this randomized controlled trial, 42 adults (50–75y) either consumed 68 g of pecans/day for 4-weeks (pecan; n = 21) or avoided all nuts (control; n = 21). At pre- (V1) and post-intervention (V2) visits, cognitive function was assessed using a fluid composite score and four subtests from the NIH Toolbox-Cognitive Battery (NIHTB-CB) (Flanker Test, Digital Change Card Sort Test (DCCS), Picture Sequence Memory Test (PSMT), NIHTB Auditory Verbal Learning Test (RAVLT)) at fasting, 30- and 210-min after a high-saturated fat meal void of pecans.
RESULTS:
From V1 to V2, fasting and postprandial cognitive performance did not differ between groups. There were improvements in both groups for fasting fluid composite score (p < 0.001) and performance on RAVLT, PSMT, DCCS, and Flanker tests from V1 to V2 (p < 0.001 for all), with no differences between groups. Additionally, postprandial performance on RAVLT, PSMT, and Flanker tests improved at V2 (p < 0.01 for each), with no differences between groups.
CONCLUSIONS:
A short-term pecan-enriched diet did not provide additional cognitive benefits in healthy older adults in the fasted or postprandial state.
1Introduction
Age-related cognitive decline is linked with natural occurring elevations in health risk seen in normal aging. Implications of cognitive impairment can be debilitating as it affects cognitive domains essential to the activities of daily living such as executive function, memory, and attention. The direct link between aging and cognitive impairment has not yet been established; however, elevations in oxidative stress have been noted in the acceleration of cognitive decline in aging populations [3]. Recent evidence demonstrating the relationship between nutrition and cognition has noted dietary interventions efficacious in reducing oxidative stress further extend associated health benefits to cognitive function [4]. The Mediterranean diet [5, 6] and Dietary Approach to Stop Hypertension (DASH) diets [7], dietary sources of antioxidants [8], B vitamins [9], and mono- (MUFA) and poly unsaturated fatty acids (PUFA) [10–12] have all been associated with improved or preserved cognitive function in older adults.
Diets high in saturated fatty acids (SFA) can disrupt cognitive performance, affecting processing speed, attention, executive function, and memory [11, 13–17]. Interestingly, high fat (HF) meals consisting of MUFA (high oleic acid (OA)), or HF meals delivered with almonds (high in OA and the PUFA linoleic acid (LA)), may limit such post-meal deficits [11, 13, 18]. Further, there is evidence suggesting acute consumption polyphenol-rich blueberries may lead to cognitive improvements 2, 4, and 8 h postprandially [19, 20]. Its hypothesized that such post-meal protection is the result of substituting SFAs with MUFAs, and other nutrients comprising tree nuts, namely, vitamin E and other antioxidants [21]. As most Americans are in the postprandial state for approximately two-thirds of the day, the potential protection afforded by tree nuts 30 minutes and 2.5 hours postprandially may be critical for the prevention or deceleration of age-related cognitive decline.
Tree nuts are a rich source of previously noted nutrients associated with cognition. In particular, pecans contain the highest total phenolic content of all tree nuts and are a good dietary source of B vitamins, MUFA (OA), PUFA (LA), anthocyanins, proanthocyanidins, flavan-3-ols and γ-tocopherol (Supplementary Table 1 and 3) [22, 23]. Previous research in healthy and at-risk populations has shown an improvement in antioxidant status and blood lipids, and reduced oxidative stress associated with both acute [24, 25] and long-term consumption of pecans [26, 27]. Despite evidence that pecans are a principal source of antioxidants and phytonutrients, there is no literature investigating the effect of pecans on cognitive function. The purpose of this study was to examine the impact of daily pecan consumption for 4-weeks on cognitive performance in healthy older adults. We hypothesized that daily pecan consumption would result in better performance on tasks assessing executive function, episodic memory, and fluid composite score, compared to control. We also considered potential influences on cognitive performance such as age, anxiety, and sleep quality, hypothesizing that increased age, heightened anxiety, and negative changes in sleep quality could lead to worsened performance at the post-diet visit.
2Methods
2.1Study design
This study was a randomized, parallel controlled trial involving a 4-week intervention conducted at the University of Georgia. Recruitment spanned from August 2020 to final testing in May 2022. The study protocol included a phone screening, screening visit, and 2 testing visits (pre- and post-intervention). Participants were randomly assigned (balanced blocks stratified by age, sex, and body mass index (BMI)) to either a control group or a pecan group. Those randomized to the pecan group were instructed to consume 68 g of pecans per day for 4-weeks while those in the control group abstained from all nut consumption. All participants were also instructed to avoid a prescribed list of foods high in antioxidants and nitrates starting 2 days prior to the first visit and extending the duration of the intervention period (Supplementary Table 2). This study (PROJECT00001978) was approved by the Institutional Review Board for human subjects and informed written consent was obtained from each participant prior to testing.
2.2Participants
Sixty-two sedentary men and women between the ages of 50 and 75y were assessed for eligibility. Participants were recruited through flyers, paid advertisements on social media, campus emails, local listservs, radio ads, and word of mouth. Individuals with dementia or cognitive deterioration with a score < 24 on the Telephone Interview for Cognitive Status [28] were excluded. Additionally, individuals with significant head trauma, brain surgery, or severe major depression with a score > 26 on the Beck’s Depression Inventory-II [29] were excluded. Other exclusion criteria included habitual nut consumption (>2 servings/week), nut allergies, special diets (i.e., ketogenic diet, intermittent fasting), excessive alcohol use (>3 drinks/d for men and > 2 drinks/d for women), tobacco or nicotine use, exercise > 3 h/week, weight loss or gain > 5% of body weight in the past 3 months, plans to begin a weight loss or exercise program, history or medical events or medication use, gastrointestinal surgery, or chronic or metabolic diseases. Individuals taking fish oil or omega-3 fatty acid supplements were also excluded from the study. The use of nitrate- or arginine-based supplements or other supplements (vitamins and minerals) was allowed if supplementation was stopped 2 weeks prior to the study and for the duration of the study. Finally, individuals with the following biomarkers were excluded: fasting glucose > 126 mg/dL or blood pressure > 180/120mmHg. Eligibility based on blood pressure and blood glucose were determined at the screening visit.
2.3Protocol
2.3.1Screening visit
Individuals arrived at the lab after an 8–12 h overnight fast and 24 h without exercise or alcohol. A fasting blood draw for a glucose measurement was obtained and anthropometrics including height, weight, blood pressure, and waist and hip circumference were measured. Next, individuals were familiarized with the cognitive battery by completing the full battery as practice, which consisted of four subtests from the National Institute of Health Toolbox-Cognitive Battery (NIHTB-CB) (HealthMeasures; Northwestern University, CA) completed in the following order: 1) Picture Sequence Memory Test (PSMT), 2) Flanker Inhibitory Control and Attention Test (Flanker), 3) Dimensional Change Card Sort Test (DCCS), and 4) Rey’s Auditory and Verbal Learning Test (RAVLT) [30]. Additionally, alcohol consumption habits were assessed using the Alcohol Use Disorders Identification Test (AUDIT) to confirm habitual consumption of < 3 drinks/d for men or < 2 drinks/d for women [31]. If individuals qualified for the study, participants were randomized to one of two treatment groups by a researcher that had no interactions with participants and was not involved in data collection or analysis. With an allocation ratio of 1:1, a permuted block design (balanced for age, sex, and BMI) was used to randomize participants.
2.3.2Experimental protocol
Participants completed a two-day food diary containing one weekend day and one weekday between the screening visit and the pre-intervention visit (V1). One of the food diaries was completed the day before V1. The night before V1, participants consumed a lead-in dinner meal and snack (provided by research personnel) that contained 16% fat, 65% carbohydrate, and 19% protein. Additionally, questionnaires assessing pre-intervention state and trait anxiety levels, obtained through the State-Trait Anxiety Inventory (STAI) [32], sleep quality by the Pittsburg Sleep Quality Index (PSQI) [33], and physical activity, assessed by calculating total Metabolic Equivalent Task (MET) minutes/week with the International Physical Activity Questionnaire (IPAQ) [34], were delivered electronically 2 days prior to V1.
For V1 and the post-intervention visit (V2), participants arrived at the lab following an 8–12 h overnight fast, 24 h without exercise, alcohol, or over-the-counter medications, and 15 h without caffeine. Height, weight, waist-hip circumference, blood pressure, and body composition were measured. Body composition was measured by Dual-Energy X-ray (DXA) (Discovery A; Hologic Inc., Waltham, MA). The research personnel then administered the baseline cognitive assessment.
Following the completion of all baseline measures, participants were instructed to consume a SFA-rich breakfast shake within 5 min. This SFA meal provided 35% of total daily energy needs based on Mifflin St. Jeor [35] calculation using measurements from the screening visit and was made from an original milk chocolate ready-to-drink shake (Ensure, Abbott Nutrition, Abbott Laboratories, Inc., Columbus, Ohio, USA), unsalted butter, red palm oil, coconut oil, soy lecithin granules, and powdered chocolate drink mix. The SFA meal was 69.5% fat, 5.0% protein, and 25.0% energy from carbohydrates. Saturated fatty acids contributed 46.9% of total energy, with 15.7% and 6.9% from MUFAs and PUFAs, respectively [27]. Four ounces of water was used to rinse the container and then ingested to ensure that the entire liquid meal was consumed. A high-SFA meal (not including pecans) was specifically chosen since daily pecan consumption has been shown to protect against oxidative stress following a high SFA meal devoid of pecans [27], and because there is evidence suggesting high SFA meals or diets may be detrimental to cognitive performance [13]. After the high SFA meal, postprandial cognitive assessments occurred at 30- and 210-min postprandially. Four ounces of water was provided at fasting and hourly postprandially.
2.3.34-Week diet intervention
The day after V1, all participants began the 4-week intervention. Diet instructions were provided to all participants. Participants in both treatment groups were instructed to avoid a written list of antioxidant or nitrate-rich foods, to avoid consuming > 3 alcoholic beverages/d (men) or > 2/d (women), to consume < 2 servings of red wine/week, and < 2 servings of nut butter/week. Supplementary Table 2 shows the complete list of foods that were avoided during the intervention period. The list of excluded foods was limited to 38 items including processed meats, heavily spiced dishes, and foods rich in antioxidants, defined by total-ORAC values > 1001μmolTE/100 g, or rich in nitrate, defined as > 100 mg of nitrate/100 g [36, 37]. The prescribed list of antioxidant and nitrate-rich foods were excluded to separate the effect of a pecan-enriched diet from other foods rich in nutrients also hypothesized to impact cognition [38–40]. Participants in the control group were also asked to avoid all forms of nuts while those in the pecan group were asked to avoid all nuts apart from pecans. Participants in the pecan group received 68 g (∼0.5 cup or 2.25 ounces) portions of pecans, packaged in individual Ziploc bags, to consume as part of their free-living diet daily. A daily dose of 68 g was selected due to previous tree nut literature suggesting doses≥60 g/d have a larger impact on measures of health [41]. Further, a high dose of pecans was chosen to match other antioxidant-rich intervention studies, in which high doses may be necessary to observe an effect of the intervention [39, 40]. Complete nutritional information for the provided portion of pecans is shown in Supplementary Table 3. All pecans were provided to participants in the pecan group at the end of V1. Participants in the pecan group were instructed to eat the pecans in their raw form (no roasting, cooking, or baking) with or without other foods (i.e., as a snack or with cereal). Lastly, all participants were asked not to make any other changes to their diet or physical activity levels.
Participants in the pecan group completed daily nut compliance logs that described the time of day that pecans were consumed. Nut compliance logs were submitted to research personnel weekly. Poor compliance was considered to be consumption of < 75% of pecans throughout the 4-week intervention. All participants completed a food diary once per week alternating between weekdays and weekend days. In the weekly food diaries, participants recorded what, and how much, they consumed including foods and beverages during a 24-hour period. Participants were asked to be as specific as possible to improve the accuracy of nutrient intake data derived from the food diaries. Nutrient intake based on food diaries was assessed using the Food Processor SQL software (version 10.12.0). Two food diaries that were completed prior to V1 were averaged, and food diaries completed during weeks 1–4 were also averaged before analysis. During week 2, a link to an electronic version of the IPAQ was sent to the participants to complete.
2.4National Institutes of Health Toolbox-Cognitive Battery (NIHTB-CB)
All participants completed the same four subtests selected from the NIHTB-CB at each time point. The four subtests included in the battery assessed memory, executive function, processing speed, and attention within a 20–25 min assessment period. The battery was limited to four assessments to minimize undue participant burden during pre- and post-intervention visits. A visual analog scale (VAS) using a standard 100 mm line was used to assess motivation anchored by “Not at all motivated” and “Extremely motivated” prior to the initiation of the first subtest. We obtained an uncorrected standard score and computed scores for respective episodic memory, executive function, and processing speed subtests. A description of these tasks is detailed elsewhere [42] and briefly described below. Additionally, the fluid composite was derived from averaging standard scores from fasting Flanker, DCCS, and PSMT and using the calculated standard score to evaluate changes in overall fluid ability [43].
2.4.1Executive function and attention measures
Two NIHTB-CB subtests were selected to measure aspects of executive function (set-shifting, inhibition, and working memory). The Dimensional Change Card Sort Test (DCCS) assessed switching and set-shifting ability. During this task, a target stimulus was displayed while two different stimuli appeared at the bottom of the screen. Participants were asked to match one of the two new stimuli to the target stimulus based on the dimension presented. Each assessment begins with rule learning, in which you are asked to identify new stimuli based one dimension (e.g., color), and then after several trials, according to the other dimension (e.g., shape). “Switch” trials are then introduced, in which the participant must change the dimension being matched. The Flanker Inhibitory Control and Attention Test (Flanker) assessed inhibition and attention. In this task, participants were asked to inhibit automatic responses and select the direction of the central stimulus (arrow symbol) with similar stimuli on either side pointing the same or different directions. Twenty randomly generated trials with arrows are completed per assessment and each assessment takes approximately 3 minutes to complete. Computed scores for DCCS and Flanker tests factor both accuracy and reaction time with scores ranging between 0–10, with 10 indicating high levels of accuracy and quick reaction time. Computed scores were used to track changes from V1 to V2.
2.4.2Episodic memory measures
Selected subtests measured episodic memory or one’s ability to acquire, store and recall information. The Picture Sequence Memory Test (PSMT) asked participants to recall a sequence of 18 pictures immediately following their presentation. Performance on two sets of picture sequences was evaluated based on the number of correctly identified sequences. To prevent sequence learning, postprandial picture sequences were not repeated within a visit. To track changes in performance on this task, an uncorrected standard score was used. Uncorrected standard score is determined using NIHTB nationally representative normative sample, with higher scores indicating better performance. The NIHTB Auditory Verbal Learning Test (RAVLT) also assessed episodic memory. For this test, participants were asked to recall 15 unrelated words immediately after their auditory presentation. The same list is presented on three learning occasions per assessment. The number of words recalled correctly per assessment was recorded as a raw score, ranging from 0–45.
2.5Statistical analysis
SAS version 9.2 statistical package (SAS Institute Inc., Cary, NC, USA) was used for statistical analyses. All values were reported as mean±SEM unless otherwise indicated. Statistical significance was set at p≤0.05. A sample size of 32 (16 per group) was estimated to detect a large effect size for cognitive assessments based on the previous study conducted by Rakic et al. [44] using G*power 3.19.7 assuming at least 80% power and an α of 0.05. The decision to use per-protocol analyses was made a priori. A two-factor repeated measures linear mixed model for treatment and visit was used to test for differences within and between groups for anthropometrics, STAI, PSQI, total MET minutes, dietary intake, and calculated fasting fluid composite scores. For fasting and postprandial differences in motivation and cognitive data, a 3-factor (treatment×visit×time point) repeated measures linear mixed model was used. When significance was found, post hoc analyzes were performed using the Tukey’s test for multiple comparisons. Finally, an exploratory analysis was performed using Pearson’s linear correlation tests to determine whether changes in cognition were associated with anxiety, sleep quality, or age. Correlations were run between pre-intervention and change values for fasting subtest performance, postprandial area under the curve (AUC) subtest values, anxiety, sleep quality, and age.
3Results
3.1Participants
Forty-nine participants were randomly assigned to a group (n = 25 control, n = 24 pecan); however, seven participants were not included in the final analyses (Fig. 1). Therefore, forty-two participants completed the intervention (15 women and 6 men for control, 15 women and 6 men for pecan). Pre-intervention characteristics of the participants are presented in Table 1. There were no differences between groups at V1 for anthropometrics or cognitive function measures. Furthermore, there were no between group differences in the change from V1 to V2 for weight, BMI, waist circumference, hip circumference, systolic blood pressure, or diastolic blood pressure (data not shown).
Fig. 1
CONSORT flow diagram selection of participants.
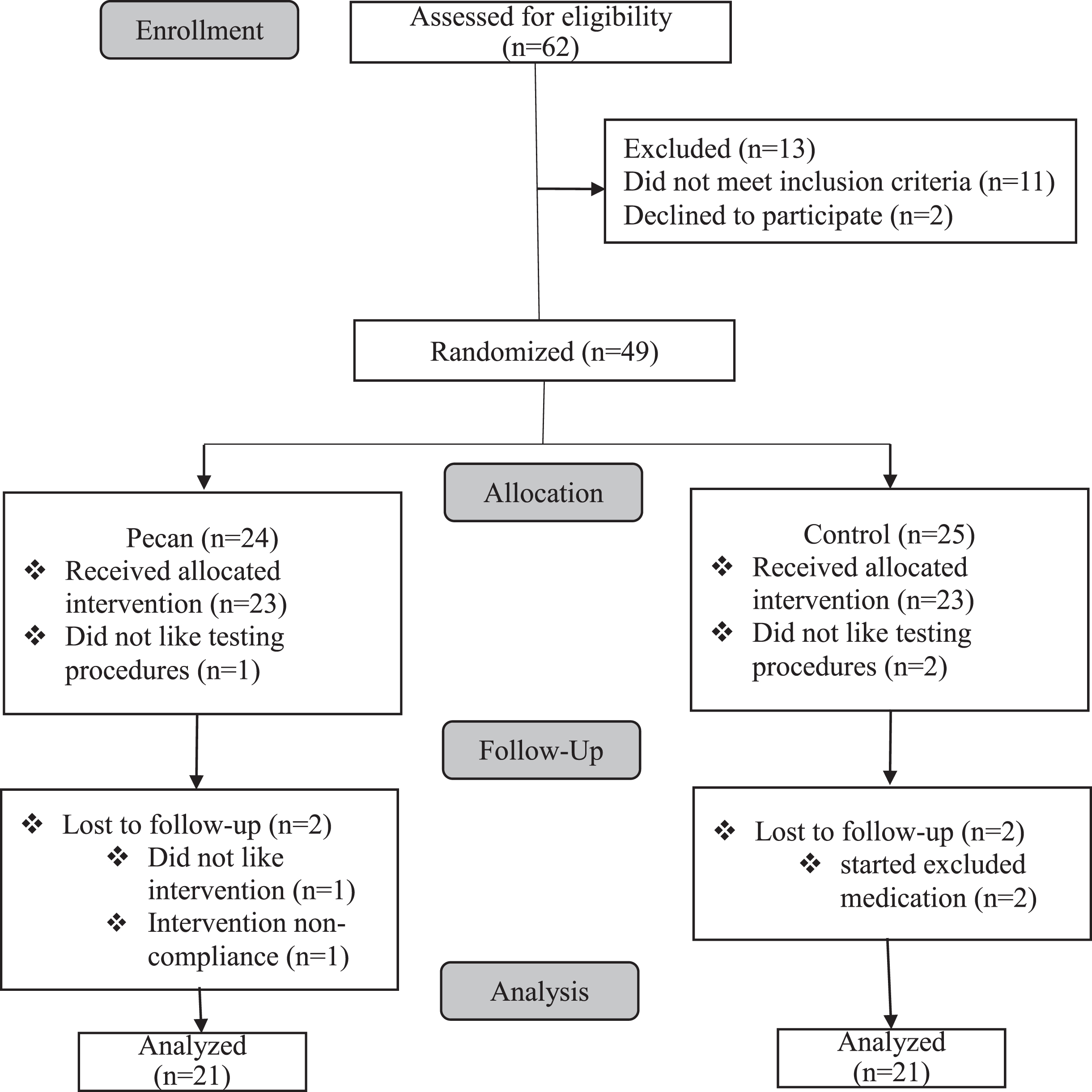
Table 1
Participant pre-intervention characteristics
Pecan | Control | P-value | |
(n = 21) | (n = 21) | ||
Female (%) | 71 | 71 | |
Age (years) | 59±1 | 59±1 | 0.66 |
BMI (kg/m2) | 25±1 | 25±1 | 0.99 |
Waist circumference (mm) | 85±14 | 84±12 | 0.86 |
Hip circumference (mm) | 104±11 | 103±10 | 0.92 |
SBP (mmHg) | 122±17 | 122±19 | 0.99 |
DBP (mmHg) | 75±10 | 77±11 | 0.94 |
Total MET (min/wk) | 1,339±710 | 1,185±858 | 0.97 |
STAI-state | 49±4 | 49±4 | 0.96 |
STAI-trait | 45±4 | 46±3 | 0.50 |
PSQI | 8±2 | 7±3 | 1.0 |
Baseline characteristics of participants by intervention group. Values are mean±SD. There were no significant differences between groups at baseline for any outcome. MET, metabolic equivalent task; STAI, state-trait anxiety inventory; PSQI, Pittsburg sleep quality index; SBP, systolic blood pressure; DBP, diastolic blood pressure.
On average, participants in the pecan group consumed 98±0.8% of the pecans provided. One participant reported poor compliance (defined as < 75% of pecans consumed) and was excluded from the analyses. Based on analyses of the weekly food diaries, self-reported intakes prior to the intervention and during the intervention are presented in Table 2. The only reported difference between groups prior to the intervention was for higher sugar intake in pecan vs. control (p = 0.01). As expected with the daily pecan consumption during the intervention, there was a treatment×visit interaction (p < 0.05) for an increase in energy (p = 0.01), percent of energy from carbohydrates (p < 0.001) and fat (p < 0.001), MUFA (p < 0.001), PUFA (p < 0.001), omega-3 (p < 0.001), omega-6 (p < 0.001), and copper (p = 0.001) for pecan vs. control. There were no main or interaction effects for self-reported intake of protein, saturated fat, trans fat, caffeine, cholesterol, sodium, or other micronutrients. Further, there were no differences in physical activity (total MET minutes), ratings of anxiety, or sleep quality from V1 to V2 within either group, and the change was not different between groups.
Table 2
Self-reported daily nutrient intake for diets enriched with pecans or diets void of all nuts
Control, n = 21 | Pecan, n = 21 | P values | |||||
Nutrient | Baseline | Intervention | Baseline | Intervention | Treatment | Visit | Interaction |
Energy, kcal | 1,850±167 | 1,798±101 | 1,928±124 | 2,526±171∧ | 0.01 | 0.09 | 0.04 |
Energy from protein (%) | 16±1 | 17±1 | 15±1 | 13±1 | 0.03 | 0.92 | 0.12 |
Energy from carbohydrate (%) | 50±2 | 50±2 | 51±2 | 40±1∧ | 0.03 | <0.001 | <0.001 |
Fiber (g) | 17.8±3.4 | 17.7±2.8 | 17.8±2.3 | 24.9±3.2 | 0.28 | 0.16 | 0.15 |
Sugar (g) | 55±5* | 68±7 | 97±14 | 88±13 | 0.02 | 0.72 | 0.09 |
Energy from fat (%) | 33±2 | 32±2 | 33±1 | 45±1∧ | <0.001 | <0.001 | <0.001 |
SFA (g) | 22±2.5 | 24±2.3 | 28±4 | 36±5 | 0.01 | 0.16 | 0.31 |
Trans-FA (g) | 0.7±0.2 | 0.6±0.1 | 0.6±0.3 | 0.6±0.2 | 0.82 | 0.66 | 0.80 |
MUFA (g) | 29.4±3.7 | 26±2.8 | 30±3 | 64±12∧ | 0.004 | 0.02 | 0.005 |
PUFA (g) | 14±1.9 | 16±2 | 16±1.6 | 35±6∧ | 0.003 | 0.002 | 0.01 |
Omega 3-FA (g) | 0.4±0.1 | 0.6±0.1 | 0.6±0.1 | 1.6±0.3∧ | 0.002 | 0.001 | 0.02 |
Omega 6-FA (g) | 4.0±0.5 | 5.8±0.7 | 5.7±0.9 | 23±5.1∧ | 0.001 | 0.001 | 0.01 |
Energy from alcohol (%) | 1±0.6 | 1±0.4 | 1±0.6 | 1±0.6 | 0.85 | 0.09 | 0.63 |
Cholesterol (mg) | 222±39 | 245±35 | 203±37 | 280±32 | 0.81 | 0.16 | 0.44 |
Sodium (mg) | 2,551±309 | 2,579±198 | 2,791±324 | 3,081±332 | 0.23 | 0.56 | 0.63 |
Caffeine (mg) | 441±353 | 244±63 | 156±28 | 150±26 | 0.32 | 0.49 | 0.54 |
All values are mean±SEM. Baseline values represent averages of the 2 food diaries before the intervention. Intervention values represent averages of all food diaries kept during the 4-week intervention. Main and interaction effects were analyzed using a linear mixed model for treatment and visit. SFA, saturated fatty acid; FA, fatty acid; MUFA, mono-unsaturated fatty acid; PUFA, polyunsaturated fatty acid. *indicates a significant difference between groups at baseline (p < 0.05). ∧indicates a significant difference from baseline within the pecan group only (p < 0.05).
3.2Fasting cognitive measures
Fasting motivation, Flanker, DCCS, PSMT, RAVLT, and calculated fluid cognition scores are presented in Table 3. There were no significant changes in motivation ratings. There were no significant differences in fasting fluid composite scores (V1: p = 0.98; V2: p = 0.99), and for fasting subtest performance by treatment at visit 1 or visit 2: RAVLT (V1: p = 0.99; V2: p = 0.99), PSMT (V1: p = 0.99; V2: p = 1.0), Flanker (V1: p = 0.99; V2: p = 0.98), and DCCS (V1: p = 0.99; V2: p = 0.99). There was a significant change in fasting fluid composite scores (pecan: p = 0.03; control: p = 0.01) and fasting RAVLT performance (pecan: p = 0.002; control: p = 0.02) within both pecan and control treatments. However, there were no other significant differences within pecan or control treatments detected for any of the other subtests.
Table 3
Fasting cognitive function measures
Pecan (n = 21) | Control (n = 21) | |||
Visit 1 | Visit 2 | Visit 1 | Visit 2 | |
Motivation (mm) | 57.3±25.3 | 64.6±26.5 | 64.2±23.4 | 66.8±29.9 |
Fluid composite | 104±7 | 109±6* | 104±7 | 109±7* |
NIHTB subtest scores | ||||
Flanker | 8.2±0.7 | 8.5±0.6 | 8.4±0.6 | 8.7±0.7 |
DCCS | 8.3±0.7 | 8.8±0.8 | 8.6±0.8 | 8.9±0.7 |
PSMT | 107±15 | 114±13 | 104±14 | 113±16 |
RAVLT | 28±8 | 33±10* | 30±8 | 35±7* |
Fasting motivation, performance on cognitive battery subtests, and fluid composite percentile rank for pecan (n = 21) and control (n = 21) at visit 1 (pre-intervention) and visit 2 (post-intervention). Data was analyzed using a linear mixed model. Posthoc differences within and between groups were determined using a Tukey’s test. All values are mean±SD. NIHTB, National Institute of Health Toolbox; Flanker, flanker inhibitory control and attention; DCCS, digital change card sort test; PSMT, picture sequence memory test; RAVLT, NIH toolbox audiotry verbal learning test. *Denotes within treatment differences in fasting performance at p < 0.05.
3.3Postprandial cognitive measures
The time course meal response data for Flanker, DCCS, PSMT, and RAVLT are presented in Fig. 2. There was no effect of time (p = 0.53), visit (p = 0.78), treatment (p = 0.47), or treatment×visit interaction (p = 0.12) for postprandial motivation ratings. For postprandial subtest performance, there were no treatment effects or treatment×visit interactions (p > 0.05), but there were significant effects of time for Flanker (p < 0.01) (Fig. 2A) and RAVLT (p < 0.01) (Fig. 2D), and significant visit effects for Flanker (p < 0.01), PSMT (p < 0.01), and RALVT (p < 0.01) (Fig. 1A, 2C, and 2D, respectively). The visit effects were for improvements in those measures from V1 to V2 across both pecan and control groups.
Fig. 2
Time course of meal response for (A) Flanker, (B) DCCS, (C) PSMT, (D) RAVLT at pre- (V1) and post- (V2) intervention visits for control (n = 21) in black and pecan (n = 21) in grey, with solid lines representing V1 and dashed lines representing V2. Participants consumed a high-saturated fat meal immediately after time 0. Data were analyzed using a linear mixed model for treatment, visit, and time. All values are presented as means±SEM. Flanker, flanker inhibitory control and attention; DCCS, digital change card sort test; PSMT, picture sequence memory test; RAVLT, NIH toolbox auditory verbal learning test.
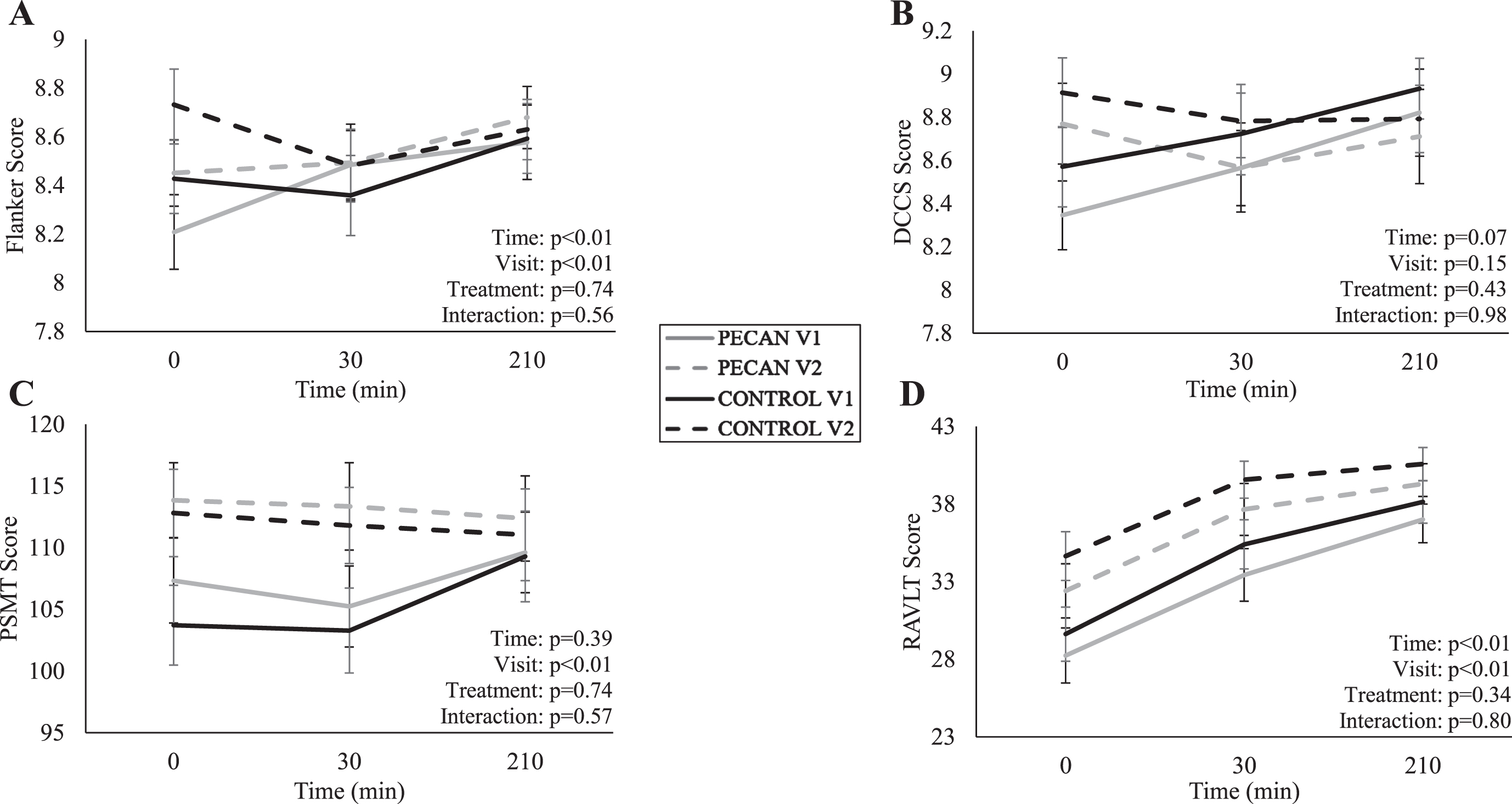
3.4Exploratory analysis
Correlations between age, anxiety, sleep quality and subtest performance (fasting, area under the curve (AUC)) were determined at V1, and for the change from V1 to V2 in all participants. There was a significant negative correlation between age and fasting performance on Flanker (r = –0.31; p = 0.05) and PSMT (r = –0.35; p = 0.02) but not with DCCS (r = –0.22; p = 0.16) or RAVLT (p = –0.24; p = 0.13) at V1. Age was not correlated with changes in any cognitive measures from V1 to V2 nor AUC data. Pre-intervention sleep quality (PSQI), and state and trait anxiety were not correlated with any fasting cognitive measures at V1. However, there was a trend for a negative correlation between PSQI (lower scores indicate better sleep quality) and fasting DCCS performance at V1 (r = –0.28, p = 0.08). Trait anxiety at V1 was negatively correlated with the change in fasting Flanker scores (r = –0.33, p = 0.05), while changes in state anxiety were negatively correlated with changes in fasting DCCS performance (r = –0.38; p = 0.02) and DCCS AUC (r = –0.35; p = 0.04) from V1 to V2. No other correlations with state anxiety were detected. Finally, change in sleep quality from V1 to V2 did not correlate with changes in performance for any of the subtests.
4Discussion
In this randomized control study, fluid cognition, executive function, and episodic memory improved following a four-week intervention in all participants. Postprandial performance on the Flanker test, a test of executive function, and both episodic memory subtests, RAVLT and PSMT, improved at the post-intervention visit for all participants, and scores on both the Flanker and RAVLT tests progressively increased following the high SFA meal challenge. Despite daily consumption of pecans rich in nutrients previously associated with cognitive improvements, there were no differences between pecan and control on fasting or postprandial fluid composite or subtest scores. As participant fluid composite scores and fasting RAVLT performance improved at the post-diet visit, and performance on RAVLT and Flanker tests improved at postprandial time points with no differences between control and pecan treatments, we demonstrated that with repeated exposure or practice to the same version of a task, performance on certain cognitive tasks improved. It is possible that the practice effect overshadowed any potential treatment effect if one existed. Finally, changes in anxiety and characteristic anxiousness correlated with executive function task performance showing that lower anxiety, either in the moment or a reduction at the post-intervention visit, was associated with improvements in executive function performance.
To our knowledge, this is the first study assessing the effect of pecans on cognitive function; however, investigations examining the effect of antioxidant-rich foods on cognitive decline are not novel [39]. Previously, a preservation effect on cognitive function in healthy older adults was noted following supplementation with antioxidant-rich vitamin E [45, 46], tree nuts [21, 44, 47–49], and fruit juices [50, 51] when consumed consistently over longer periods of time ranging from 3 months to 3 years. In those analyses, improvement or no change in the intervention group, along with impairment in control groups, was a marker of preserved cognitive function. Interestingly, our findings may mirror the existing evidence regarding tree nuts and aspects of cognitive function, in which almonds [18, 44, 47, 48], walnuts [47, 52], peanuts [53], or mixed nuts [5, 8, 54, 55] did not lead to significant improvements in one or all included measures of cognitive function.
Pecans contain more antioxidants than any other tree nut, approximately 873-1,370 mg total polyphenols per 68 g [23]. However, in the present study, change in cognitive function was not better with daily pecan consumption when compared to control. In a recent meta-analysis and systematic review, Ammar et al. [39, 40] suggested that cognitive benefits may be restricted to highly bioavailable sources at large doses (>500 mg total polyphenol) and in young or middle-aged populations. Although pecans exceed this dose, not having pecans in the HF meal challenge may have diminished potential cognitive benefits. It is also possible that our 4-week intervention was too short to detect cognitive changes. Previous studies with daily pecan consumption have shown changes in other physiologic markers within a 4-week period [26, 27, 56]; however, the lack of change between groups here suggests longer intervention studies may be warranted.
Despite the demonstrated antioxidant capacity of pecans [24, 25, 27, 57], and the effect of pecan-enriched diet on postprandial oxidative stress [27], post-meal cognitive performance on executive function and episodic memory tests was not different between pecan vs. control groups. However, the acute impact of a meal high in SFA on cognitive function is not well known. To date, only one study has examined the postprandial implications of a single meal high in SFA [13]. In their analysis, performance on attention tasks were impaired 1 h following a SFA-rich meal in a middle-aged women [13]. In contrast to those findings, postprandial cognitive performance in our study was unaffected or improved in both pecan and control treatments. As the benefits of daily pecan consumption on oxidative stress following a meal high in SFA has been noted after 8 weeks, the shorter intervention period and absence of pecans in the meal challenge in this study may have reduced the potent antioxidant effects previously noted [25–27, 58]. While this study, to our knowledge, is the first to investigate the effect of a dietary intervention on post SFA meal cognitive function, more research is needed to determine the long-term benefits of diet modifications, such as daily pecan consumption, on slowing age-related changes in cognition.
Anxiety and sleep quality are also factors known to impact performance on cognitive tests. In Wightman et al. [38], improved cognitive function following a 4-week intervention period was attributed to reductions in anxiety more so than the tea extract intervention being assessed. Exploratory correlation analyses from our study revealed a negative correlation between the change in state anxiety, or anxiety felt in the present moment, and the change from pre- to post-intervention in DCCS performance at fasting and postprandially. Although exploratory, this negative correlation aligns with Wightman et al.’s [38] findings suggesting participant comfort or familiarity with the testing environment following the initial visit may lead to reductions in anxiety that result in better cognitive performance. In the present analysis, we attempted to reduce the impact of anxiety and practice effects on cognitive outcomes by exposing participants to the cognitive battery at the screening visit. However, other aspects of the pre- and post-intervention visits such as IV placement, meal challenge, and 4 h of postprandial measurements were not practiced. Therefore, the novelty of the intervention visits may still have led to increased state anxiety at the first test visit. Previously, sleep quality has also been found to be an independent predictor of cognitive performance on memory and executive function tests [59]. However, we did not observe any significant changes in sleep quality during the intervention, nor was sleep quality correlated with changes in any of our cognitive performance outcomes.
There are a few limitations to this study that could possibly explain our lack of between group differences. The NIHTB-CB was chosen for this present analysis because it is well-accepted by older adult participants and its validity has been demonstrated in several studies [30, 42, 60–62]. However, few intervention studies have utilized this tool to examine changes in cognitive function over time [63]. Validation studies have noted practice effects comparable to other cognitive batteries in studies examining test-retest reliability over intervals spanning 7–28 days [30, 42, 60–62]. As practice effects are apparent, repeated exposure to subtests during each visit may have further masked true differences in cognitive performance that may have occurred, especially since this toolkit is designed to provide the same version of tasks at each session. Another limitation may have been the design of the study and the baseline cognitive health of our participants. As it was not possible for this to be a double-blinded study, the conclusions should be interpreted with some degree of caution. Moreover, fasting performance at baseline suggested that participants included in the present analysis had average to above average cognitive abilities possibly preventing further improvements with pecan consumption [43]. Further, the prescribed list of excluded food items was not a comprehensive list. Other sources of antioxidants and/or unsaturated fatty acids such as certain teas, fruit juices, dried fruits, fish, or avocados were allowed during the 4-week intervention; however, participants were instructed avoid introducing new foods or beverages to their diet during the intervention. Additionally, the application of our findings to everyday life may be limited due to the dose of pecans being higher than the U.S. Food and Drug Administration 2003 health claim, recommending just 1.5 ounce (42 g) of nuts per day [64]. Finally, in our analysis we did not account for the impact of COVID-19 on study outcomes. To our knowledge, none of our participants were diagnosed with COVID-19 during the intervention and per University guidelines both participants and research personnel were masked at pre- and post-intervention visits. Previous history of COVID-19 infections were not recorded, so that impact on our results are unknown.
Although this study, like every study, had some limitations, the current study design is a key feature with one unique aspect that deserves consideration. Few studies have assessed cognitive function postprandially, and even fewer following a HF meal challenge. Dhillon et al. [18] noted preserved performance on memory tasks 35 min following a HF meal containing almonds. In this study, the meal challenge was high in SFAs, known to increase oxidative stress and influence cognitive performance. Much like HF meals would be in real life, the meal challenge in our study was void of pecans. This is important for generalizability, as most individuals would not normally consume pecans in conjunction with the occasional unhealthy (high SFA) meal. Further, as the effect of a single meal high in SFA on cognitive function is not well understood, we wanted to determine whether daily pecan consumption could be protective from a cognitive standpoint against an occasional unhealthy meal. Unfortunately, the results of this present study did not support that notion.
In conclusion, short-term adherence to a pecan-enriched diet did not exert additional cognitive benefits in a group of cognitively healthy older adults in either the fasting or postprandial state compared to control. With the majority of the day spent in the postprandial state, investigating the effect of pecans on cognitive performance following a meal could provide applicable insight into the consequence of the habitual diet on age-related cognitive decline. As memory deficits are debilitating consequences of the aging process, future studies examining the longer-term effects of pecan consumption on age-related cognitive decline are needed to discern potential benefits of the inclusion of pecan as we age.
Acknowledgments
We would like to thank Dr. Patrick J. O’Connor (University of Georgia) for his assistance with cognitive test selection and the NIH toolbox.
Funding
This research was funded by the American Pecan Council (R-APEC0001190901). The sponsors had no role in the study design, in the collection, analysis, or interpretation of data, in the writing of the report, or in the decision to submit the article for publication.
Conflict of interest
The authors have no conflict of interest to report.
Supplementary material
[1] The supplementary material is available in the electronic version of this article: https://dx.doi.org/10.3233/NHA-220181.
References
[1] | Kornsteiner-Krenn M , Wagner K-H , Elmadfa I . Phytosterol content and fatty acid pattern of ten different nut types, Int J Vitam Nutr Res (2013) ;83: (5):263–70. |
[2] | Bolling BW , McKay DL , Blumberg JB . The phytochemical composition and antioxidant actions of tree nuts, Asia Pacific Journal of Clinical Nutrition (2010) ;19: (1):117. |
[3] | Von Bernhardi R , Eugenín J . Alzheimer’s disease: redoxdysregulation as a common denominator for diverse pathogenicmechanisms, Antioxidants & Redox Signaling (2012) ;16: (9):974–1031. |
[4] | Scarmeas N , Anastasiou CA , Yannakoulia M . Nutrition and prevention of cognitive impairment, The Lancet Neurology (2018) ;17: (11):1006–15. |
[5] | Martínez-Lapiscina EH , Clavero P , Toledo E , Estruch R , Salas-Salvadó J , San Julian B , et al., Mediterranean dietimproves cognition: the PREDIMED-NAVARRA randomised trial, Journalof Neurology, Neurosurgery & Psychiatry (2013) ;84: (12):1318–25. |
[6] | Valls-Pedret C , Sala-Vila A , Serra-Mir M , Corella D , de la Torre R , Martínez-González MÁ , et al., Mediterranean Diet andAge-Related Cognitive Decline: A Randomized Clinical Trial, JAMAInternal Medicine (2015) ;175: (7):1094–103. |
[7] | Daniel GD , Chen H , Bertoni AG , Rapp SR , Fitzpatrick AL , Luchsinger JA , et al., DASH diet adherence and cognitive function: Multi-ethnic study of atherosclerosis, Clinical Nutrition ESPEN (2021) ;46: :223–31. |
[8] | Valls-Pedret C , Lamuela-Raventós RM , Medina-Remón A , Quintana M , Corella D , Pintó X , et al., Polyphenol-rich foods in the Mediterranean diet are associated with better cognitive function in elderly subjects at high cardiovascular risk, Journal of Alzheimer’s Disease (2012) ;29: (4):773–82. |
[9] | Ford TC , Downey LA , Simpson TA-O , McPhee G , Oliver C , Stough CA-O . The Effect of a High-Dose Vitamin B Multivitamin Supplement on theRelationship between Brain Metabolism and Blood Biomarkers ofOxidative Stress: A Randomized Control Trial, Nutrients (2018) ;10: (12):1860. |
[10] | Stavrinou PS , Andreou E , Aphamis G , Pantzaris M , Ioannou M , Patrikios IS , et al. The Effects of a 6-Month High Dose Omega-3 and Omega-6 Polyunsaturated Fatty Acids and Antioxidant Vitamins Supplementation on Cognitive Function and Functional Capacity in Older Adults with Mild Cognitive Impairment, Nutrients (2020) ;12: (2):325. |
[11] | Cao G-Y , Li M , Han L , Tayie F , Yao S-S , Huang Z , et al. Dietary fat intake and cognitive function among older populations: A systematic review and meta-analysis, The Journal of Prevention of Alzheimer’s Disease (2019) ;6: (3):204–11. |
[12] | Assmann KE , Adjibade M , Hercberg S , Galan P , Kesse-Guyot E . Unsaturated Fatty Acid Intakes During Midlife Are Positively Associated with Later Cognitive Function in Older Adults with Modulating Effects of Antioxidant Supplementation, The Journal of Nutrition (2018) ;148: (12):1938–45. |
[13] | Madison AA , Belury MA , Andridge R , Shrout MR , Renna ME , Malarkey WB , et al. Afternoon distraction: a high-saturated-fat meal and endotoxemia impact postmeal attention in a randomized crossover trial, The American Journal of Clinical Nutrition (2020) ;111: (6):1150–8. |
[14] | Eskelinen MH , Ngandu T , Helkala EL , Tuomilehto J , Nissinen A , Soininen H , et al. Fat intake at midlife and cognitive impairment later in life: a population-based CAIDE study, International Journal of Geriatric Psychiatry: A Journal of the Psychiatry of Late Life and Allied Sciences (2008) ;23: (7):741–7. |
[15] | Kalmijn S , Launer LJ , Ott A , Witteman JC , Hofman A , Breteler MM . Dietary fat intake and the risk of incident dementia in the Rotterdam Study, Annals of Neurology (1997) ;42: (5):776–82. |
[16] | Holloway CJ , Cochlin LE , Emmanuel Y , Murray A , Codreanu I , Edwards LM , et al. A high-fat diet impairs cardiac high-energy phosphate metabolism and cognitive function in healthy human subjects, The American Journal of Clinical Nutrition (2011) ;93: (4):748–55. |
[17] | Winocur G , Greenwood CE . Studies of the effects of high fat diets on cognitive function in a rat model, Neurobiology of Aging (2005) ;26: (1):46–9. |
[18] | Dhillon J , Tan S-Y , Mattes RD . Effects of almond consumption on the post-lunch dip and long-term cognitive function in energy-restricted overweight and obese adults, British Journal of Nutrition (2017) ;117: (3):395–402. |
[19] | Dodd GF , Williams CM , Butler LT , Spencer JPE . Acute effects of flavonoid-rich blueberry on cognitive and vascular function in healthy older adults, Nutrition and Healthy Aging (2019) ;5: :119–32. |
[20] | Whyte AR , Rahman S , Bell L , Edirisinghe I , Krikorian R , Williams CM , et al. Improved metabolic function and cognitive performance in middle-aged adults following a single dose of wild blueberry, European Journal of Nutrition (2021) ;60: (3):1521–36. |
[21] | Gervasi T , Barreca D , Laganà G , Mandalari G . Health BenefitsRelated to Tree Nut Consumption and Their Bioactive Compounds, Int JMol Sci (2021) ;22: (11). |
[22] | Alvarez-Parrilla E . Bioactive components and health effects of pecan nuts and their by-products: a review. Instituto de Ciencias Biomédicas. 2018. |
[23] | Alasalvar C , Bolling BW . Review of nut phytochemicals, fat-soluble bioactives, antioxidant components and health effects, British Journal of Nutrition (2015) ;113: (S2):S68–S78. |
[24] | Hudthagosol C , Haddad EH , McCarthy K , Wang P , Oda K , Sabaté J . Pecans Acutely Increase Plasma Postprandial Antioxidant Capacity andCatechins and Decrease LDL Oxidation in Humans, The Journal ofNutrition (2010) ;141: (1):56–62. |
[25] | Marquardt AR , Lewandowski KR , Paton CM , Cooper JA . Comparison of metabolic and antioxidant responses to a breakfast meal with and without pecans, Journal of Functional Foods (2019) ;62: , 103559. |
[26] | Haddad E , Jambazian P , Karunia M , Tanzman J , Sabaté J . Apecan-enriched diet increases γ-tocopherol/cholesterol anddecreases thiobarbituric acid reactive substances in plasma ofadults, Nutrition Research (2006) ;26: (8):397–402. |
[27] | Guarneiri LL , Paton CM , Cooper JA . Pecan-enriched diets decrease postprandial lipid peroxidation and increase total antioxidant capacity in adults at-risk for cardiovascular disease, Nutrition Research (2021) ;93: :69–78. |
[28] | Brandt J , Spencer M , Folstein M . The telephone interview for cognitive status, Neuropsychiatry Neuropsychol Behav Neurol (1988) ;1: (2):111–7. |
[29] | Beck AT , Steer RA , Brown GK . Manual for the beck depression inventory-II, San Antonio, TX: Psychological Corporation (1996) ;1: (82):10.1037. |
[30] | Parsey CM , Bagger JE , Trittschuh EH , Hanson AJ . Utility of the iPad NIH Toolbox Cognition Battery in a clinical trial of older adults, J Am Geriatr Soc (2021) ;69: (12):3519–28. |
[31] | Bohn MJ , Babor TF , Kranzler HR . The Alcohol Use Disorders Identification Test (AUDIT): validation of a screening instrument for use in medical settings, Journal of Studies on Alcohol (1995) ;56: (4):423–32. |
[32] | Spielberger CD , Gonzalez-Reigosa F , Martinez-Urrutia A , Natalicio LF , Natalicio DS . The state-trait anxiety inventory, Revista Interamericana de Psicologia/Interamerican Journal of Psychology (1971) ;5: (3 & 4). |
[33] | Buysse DJ , Reynolds III CF , Monk TH , Berman SR , Kupfer DJ . The Pittsburgh Sleep Quality Index: a new instrument for psychiatric practice and research, Psychiatry Research (1989) ;28: (2):193–213. |
[34] | Geiselman PJ , Anderson AM , Dowdy ML , West DB , Redmann SM , Smith SR , Reliability and validity of a macronutrient self-selection paradigm and a food preference questionnaire, . Physiology & Behavior (1998) ;63: (5):919–28. |
[35] | Mifflin MD , St Jeor ST , Hill LA , Scott BJ , Daugherty SA , Koh YO . A new predictive equation for resting energy expenditure in healthy individuals, The American Journal of Clinical Nutrition (1990) ;51: (2):241–7. |
[36] | Bahadoran Z , Mirmiran P , Jeddi S , Azizi F , Ghasemi A , Hadaegh F . Nitrate and nitrite content of vegetables, fruits, grains, legumes,dairy products, meats and processed meats, Journal of FoodComposition and Analysis (2016) ;51: :93–105. |
[37] | Nutrient Data L. USDA database for the oxygen radical absorbance capacity (ORAC) of selected foods. 2010:USDA. |
[38] | Wightman EL , Jackson PA , Khan J , Forster J , Heiner F , Feistel B , et al. The acute and chronic cognitive and cerebral blood flow effects of a Sideritis scardica (Greek mountain tea) extract: A double blind, randomized, placebo controlled, parallel groups study in healthy humans, Nutrients (2018) ;10: (8):955. |
[39] | Ammar A , Trabelsi K , Boukhris O , Bouaziz B , Müller P , MGlenn J , et al. Effects of polyphenol-rich interventions on cognition and brain health in healthy young and middle-aged adults: systematic review and meta-analysis, Journal of Clinical Medicine (2020) ;9: (5):1598. |
[40] | Ammar A , Trabelsi K , Müller P , Bouaziz B , Boukhris O , Glenn JM , et al. The effect of (poly) phenol-rich interventions on cognitive functions and neuroprotective measures in healthy aging adults: A systematic review and meta-analysis, Journal of Clinical Medicine (2020) ;9: (3):835. |
[41] | Del Gobbo LC , Falk MC , Feldman R , Lewis K , Mozaffarian D . Effects of tree nuts on blood lipids, apolipoproteins, and blood pressure: systematic review, meta-analysis, and dose-response of 61 controlled intervention trials, The American Journal of Clinical Nutrition (2015) ;102: (6):1347–56. |
[42] | Heaton RK , Akshoomoff N , Tulsky D , Mungas D , Weintraub S , Dikmen S , et al. Reliability and validity of composite scores from the NIH Toolbox Cognition Battery in adults, Journal of the International Neuropsychological Society (2014) ;20: (6):588–98. |
[43] | NIH Toolbox® Scoring and Interpretation Guide. NIH Toolbox®. 2006-2016. NIH Toolbox and PROMIS iPad apps: National Institutes of Health and Northwestern University; 2021. |
[44] | Mustra Rakic J , Tanprasertsuk J , Scott TM , Rasmussen HM , Mohn ES , Chen C-YO , et al. Effects of daily almond consumption for six months on cognitive measures in healthy middle-aged to older adults: a randomized control trial. Nutritional Neuroscience. 2021:1-11. |
[45] | Kalmijn S , Feskens E , Launer LJ , Kromhout D . Polyunsaturated fatty acids, antioxidants, and cognitive function in very old men, American Journal of Epidemiology (1997) ;145: (1):33–41. |
[46] | Morris MC , Evans DA , Bienias JL , Tangney CC , Wilson RS . Vitamin E and cognitive decline in older persons, Archives of Neurology (2002) ;59: (7):1125–32. |
[47] | Sala-Vila A , Valls-Pedret C , Rajaram S , Coll-Padrós N , CofánM , Serra-Mir M , et al. Effect of a 2-year diet intervention withwalnuts on cognitive decline, The Walnuts And Healthy Aging (WAHA)study: a randomized controlled trial. The American Journal ofClinical Nutrition (2020) ;111: (3):590–600. |
[48] | Coates AA-O , Morgillo S , Yandell C , Scholey AA-O , Buckley JA-O , Dyer KA , et al. Effect of a 12-Week Almond-Enriched Diet on Biomarkers of Cognitive Performance, Mood, and Cardiometabolic Health in Older Overweight Adults, Nutrients (2020) ;12: (4):1180. |
[49] | Parilli-Moser I , Domínguez-López I , Trius-Soler M , Castellví M , Bosch B , Castro-Barquero S , et al. Consumption ofpeanut products improves memory and stress response in healthyadults from the ARISTOTLE study: A 6-month randomized controlledtrial, Clinical Nutrition (2021) ;40: (11):5556–67. |
[50] | Siddarth P , Li Z , Miller KJ , Ercoli LM , Merril DA , Henning SM , et al. Randomized placebo-controlled study of the memory effects of pomegranate juice in middle-aged and older adults. (1938-3207 (Electronic)). |
[51] | Chai SA-O , Jerusik J , Davis K , Wright RS , Zhang Z . Effect of Montmorency tart cherry juice on cognitive performance in older adults: a randomized controlled trial, Food & Function (2019) ;10: (7):4423–31. |
[52] | Pribis P , Bailey RN , Russell AA , Kilsby MA , Hernandez M , Craig WJ , et al. Effects of walnut consumption on cognitive performance in young adults, British Journal of Nutrition (2012) ;107: (9):1393–401. |
[53] | Barbour JA , Howe PR , Buckley JD , Bryan J , Coates AM . Cerebrovascular and cognitive benefits of high-oleic peanut consumption in healthy overweight middle-aged adults, Nutritional Neuroscience (2017) ;20: (10):555–62. |
[54] | Dong L , Xiao R , Cai C , Xu Z , Wang S , Pan L , et al. Diet, lifestyle and cognitive function in old Chinese adults, Archives of Gerontology and Geriatrics (2016) ;63: :36–42. |
[55] | Samieri C , Grodstein F , Rosner BA , Kang JH , Cook NR , Manson JE , et al. Mediterranean diet and cognitive function in older age, Epidemiology (2013) ;24: (4):490–9. |
[56] | Guarneiri LL , Paton CM , Cooper JA . Pecan-enriched diets alter cholesterol profiles and triglycerides in adults at risk for cardiovascular disease in a randomized, controlled trial, The Journal of Nutrition (2021) ;151: (10):3091–101. |
[57] | Robbins KS , Gong Y , Wells ML , Greenspan P , Pegg RB . Investigation of the antioxidant capacity and phenolic constituents of US pecans, Journal of Functional Foods (2015) ;15: :11–22. |
[58] | Hudthagosol C , Haddad EH , McCarthy K , Wang P , Oda K , Sabate J . Pecans acutely increase plasma postprandial antioxidant capacity and catechins and decrease LDL oxidation in humans, J Nutr (2011) ;141: (1):56–62. |
[59] | Falck RS , Best JR , Davis JC , Liu-Ambrose T . The independent associations of physical activity and sleep with cognitive function in older adults, Journal of Alzheimer’s Disease (2018) ;63: (4):1469–84. |
[60] | Scott EP , Sorrell A , Benitez A . Psychometric properties of the NIH toolbox cognition battery in healthy older adults: Reliability, validity, and agreement with standard neuropsychological tests, Journal of the International Neuropsychological Society (2019) ;25: (8):857–67. |
[61] | Zelazo PD , Anderson JE , Richler J , Wallner-Allen K , Beaumont JL , Conway KP , et al. NIH Toolbox Cognition Battery (CB): validation of executive function measures in adults, J Int Neuropsychol Soc (2014) ;20: (6):620–9. |
[62] | Weintraub S , Dikmen SS , Heaton RK , Tulsky DS , Zelazo PD , Bauer PJ , et al. Cognition assessment using the NIH Toolbox, Neurology (2013) ;80: (11 Supplement 3):S54–S64. |
[63] | Martin RC , Gaston TE , Thompson M , Ampah SB , Cutter G , Bebin EM , et al. Cognitive functioning following long-term cannabidiol use in adults with treatment-resistant epilepsy, Epilepsy & Behavior (2019) ;97: :105–10. |
[64] | Brown D . FDA considers health claim for nuts, (Beyond the Headlines). Journal of the American Dietetic Association (2003) ;103: (4):426–7. |