DAF-16 and SKN-1 mediate Anti-aging and Neuroprotective efficacies of “thai ginseng” Kaempferia parviflora Rhizome extract in Caenorhabditis elegans
Abstract
BACKGROUND:
The rhizomes of Kaempferia parviflora (KP), have been traditionally used for treating various ailments with 5,7-dimethoxyflavone (DMF) as a prominent compound.
OBJECTIVE:
To investigate the anti-aging and neuroprotective properties of KP and DMF in Caenorhabditis elegans.
METHODS:
C. elegans (wild-type (N2), transgenic and mutant strains) were treated with KP and DMF and were monitored for lifespan and neuroprotection through physiological assays, fluorescence microscopy and qPCR analysis. Molecular docking studies were employed to identify the interaction mode of DMF with DAF-16 and SKN-1.
RESULTS:
KP and DMF significantly increased the lifespan of N2 along with modulating pharyngeal pumping and lipofuscin accumulation. They also exhibited neuroprotection in Aβ transgenic strains by improving lifespan and delaying paralysis. Further, they reduced ROS accumulation significantly in worms exposed to UV-A, thereby exhibiting anti-photoaging potential. KP and DMF could activate SKN-1, DAF-16 which was evident from molecular docking and qPCR analysis. The DAF-2 and DAF-16 mutants did not exhibit any variations in lifespan upon treatment with KP and DMF suggesting the involvement of the DAF-16 mediated pathway in regulating the anti-aging and neuroprotective effects.
CONCLUSION:
Our findings suggest that KP with DMF as an active ingredient is a potential nutraceutical for aging and associated disorders.
1Introduction
Aging could be described as the periodic and progressive loss of molecular, cellular, physiological functioning of body leading to several chronic pathological conditions [1]. Alzheimer’s disease (AD) is one of the most recurrent neurodegenerative conditions linked to aging, and is challenging to diagnose. Also, the present treatment strategies could not effectively reduce the progression of the disease [2]. Plant based herbal medicine is on the rise against aging and age associated diseases and is considered to be safe with limited side effects, which could improve both the lifespan and healthspan of the host [2, 3].
The “Thai ginseng” Kaempferia parviflora, which was initially found in Thailand, belongs to the family Zingiberaceae. The rhizomes of this plant, commonly known as black ginger, has abundant medicinal properties and is traditionally known to be used for the treatment of inflammation, depression, ulcers, gout, colic disorder, abscesses, allergy, osteoarthritis and many more [4, 5]. The major constituent identified from the K. parviflora rhizome (KP) extract is methoxyflavone and so far, 16 different types of methoxyflavones have been identified, with 5,7-dimethoxyflavone (DMF) being the prominent phytoconstituent which imparts many of the health benefits [4].
KP rhizomes were reported to contain 5-Hydroxy-3,7-dimethoxyflavone, 5-hydroxy-7-methoxyflavone, 5-hydroxy-3,7,4’-trimethoxyflavone, 5-hydroxy-7,4’-dimethoxyflavone, 5-hydroxy-3,7,3’,4’-tetramethoxyflavone, 3,5,7-trimethoxyflavone, 3,5,7,4’-tetramethoxyflavone, 5,7,4’-trimethoxyflavone, 5,7,3’,4’-tetramethoxyflavone, 5,7-dimethoxyflavone, 3,5,7,3’,4’-pentamethoxyflavone, tectochrysin, 5,3’-dihydroxy-3,7,4’-trimethoxyflavone, 5-hydroxy-7,3’,4’-trimethoxyflavone and genkwanin as some of the major flavonoids and phytoconstituents [6–8].
The extract along with the methoxyflavones have been reported to exhibit positive effects on cellular metabolism regulation, anti-cancer, cardioprotection, sexual enhancement, neuroprotection, anti-allergic, anti-inflammatory, antioxidant, anti-osteoarthritis, and antimicrobial activity [4]. The KP extract has been analyzed for its effects in humans and a recent systemic review suggested positive correlation of KP in physical or exercise performance in improving hand grip, erectile response, pain indicators, and energy expenditure with no adverse effects reported [5]. However, the overall mechanism involved behind the KP mediated health benefits is still unclear.
Previously, our group has identified that KP extract can induce neuroprotection against glutamate induced toxicity by diminishing extracellular signal-regulated kinase (ERK) phosphorylation and reactive oxygen species (ROS) regulation, recovering brain-derived neurotrophic factor (BDNF) and modulating apoptosis [9]. The KP extract was able to improve the level of serotonin, norepinephrine, and dopamine in the rat hippocampus. Additionally, proteins related to the synaptic function, endocytosis, and ATP synthesis were regulated apart from improving the levels of mitochondria-targeted antioxidants enzymes [10].
The free-living soil nematode, Caenorhabditis elegans is being extensively used recently for the study of human and ecological toxicology, drug targets and drug development, as well as elucidating overall mechanism through high-throughput screening mechanisms. The model can typify the distinctive characteristics of various pathological conditions that occurs in human through physiological and molecular approach. Additionally, it can be employed to understand various stress response mechanisms that could be well correlated with the humans because of the existing homologs for nearly 80% of genes [11, 12].
C. elegans have been used to study the anti-aging, stress resistance and neuroprotective effects of various traditional medicinal plants including, Cleistocalyx nervosum [13, 14], Lignosus rhinoceros [15], Bacopa monnieri [16], Caesalpinia mimosoides [17] and Diplocyclos palmatus [18]. In the present study, the anti-aging and neuroprotective effects of KP and its active constituent DMF has been analyzed using C. elegans model.
2Materials and methods
2.1Chemicals, reagents, and equipments used
All the chemicals used in the study were obtained from Sigma-Aldrich (St. Louis, MO, USA) and HiMedia Laboratories (Mumbai, India), unless otherwise specified. UV-A exposure for C. elegans strains was done by exposing them (4 h) under a UV transilluminator, SANKYO DENKI (F20T10BL).
2.2Collection of KP and extraction
KP plant was collected with permissions obtained from College of Oriental Medicine, Rangsit University, Thailand in 2019 from Phu Ruea District, Loei province in Northeast of Thailand. The plant was authenticated by the pharmaceutical botanist, Asst. Prof. Nijsiri Ruangrungsi (Rangsit University, Thailand), recorded with the identification number ORMRSU2019001. The rhizomes were washed, shade-dried, powdered and extracted with 95% ethanol by using the Soxhlet extractor for 24 h. The extract was dissolved in dimethyl sulfoxide (100 mg/mL) and stored at –20 °C until further use [9].
2.3C. elegans strains used and culture conditions
All the C. elegans strain used in the study were purchased from Caenorhabditis Genetics Center, (University of Minnesota, USA) and were maintained under standard conditions [19].
2.4Lifespan assay
Young adult nematodes (first day adults) (wild type or mutants; 10 numbers) were treated with different concentrations of KP extract (100–1000μg/ml for N2 strain; 400 –600μg/ml for transgenic and mutant strains) and DMF (10 –100μM for N2 strain; 70 –90μM for transgenic and mutant strains) in 24-well plate containing M9 buffer. The live nematodes from five independent trials were counted every 24 h and calculated for their percentage of survival [14].
2.5Estimation of pharyngeal pumping
The young adult N2 worms (10 numbers) were treated with KP/DMF, as explained earlier in NGM plates and the pharyngeal contractions were monitored under a stereomicroscope and counted at every 24 h [14].
2.6Brood size assay
Treatment to young adult stage N2 nematodes (10 numbers) in NGM plates were done as explained earlier. The number of eggs laid was counted once in 24 h and the experimental worms were changed to new plates, until the egg laying was ceased [20].
2.7Fluorescence imaging
Wild type nematodes (for lipofuscin) and the LG333 strain (for skn-1 expression) were treated with varying concentrations of KP (400 –600μg/ml) and DMF (70 –90μM) consecutively for 5 days. After treatment period, the worms from three independent trials were washed thoroughly and imaged under ZEISS LSM 700 Confocal microscope (10X). The obtained images were processed using Image J and fluorescence intensity was denoted in arbitrary units (AU) [13].
2.8Paralysis assay
Synchronized eggs of CL4176 were maintained at 15 °C, on the NGM plates for 36 h followed by treatment of worms with different concentrations of KP (400 –600μg/ml) and DMF (70 –90μM) and were incubated at 25 °C to induce expression of Aβ. After 24 h of temperature shift, paralyzed nematodes (that did not respond to touch) were monitored every hour. The paralyzed worms were counted and expressed as percentage of paralysis [21].
2.9Analysis of photoaging effect
The wild type young adult nematodes were transferred to 24-well plate in two batches. In the first batch, the nematodes were treated (pre-treatment) with varying concentrations of KP extracts (400 –600μg/ml) and DMF (70 –90μM) individually and exposed to UV-A for 4 h. In the second batch, the nematodes were first exposed to UV-A for 4 h, which is followed with KP/DMF treatment individually (post-treatment). Lifespan was monitored for every 24 h and was represented as percentage of survival [22].
2.10Measurement of extracellular ROS using DCF
The worms were transferred to 24-well plate and treatment were carried out as explained in the previous section (2.9) followed by incubation at 15 °C for 5 days. After treatment period, the worms were exposed to DCFH-DA (5μg/ml) for 20 minutes, followed by washing and imaged under confocal microscope [14].
2.11Molecular docking analysis
The molecular docking analysis was done as previously reported with slight modifications [23]. In the absence of the protein structures, 3D structures of SKN-1 (Uniprot ID: P34707) and DAF-16 (Uniprot ID: 16850) were developed by homology modelling using the online platform server trROSETTA [24]. The chemical structure of DMF was obtained from PubChem and was considered for molecular docking with grid-based docking approach using DockThor and the binding energies of were calculated and compared with resveratrol as the reference compound [25].
2.12Total RNA isolation and real time PCR analysis
Wild type nematodes were treated with KP and DMF as explained earlier and total RNA was isolated using Trizol kit. Real-Time PCR analysis was done with the converted cDNA from the experimental groups according to manufacturer’s protocol (Bioneer, Korea) using specific primers (Table 1). The obtained data were normalized to actin as the internal control [14].
Table 1
List of primers used
Gene Name | Forward Primer | Reverse Primer |
act-2 | ATCGTCCTCGACTCTGGAGATG | TCACGTCCAGCCAAGTCAAG |
skn-1 | ATCCATTCGGTAGAGGACCA | GGCGCTACTGTCGATTTCTC |
daf-16 | TGGTGGAATTCAATCGTGAA | ATGAATATGCTGCCCTCCAG |
2.13Statistical analysis
All the experimental results were indicated as mean of independent trials. One-way ANOVA (SPSS 17) was employed for statistical investigation and (p < 0.05) was represented as significant upon comparing between control vs treated.
3Results
3.1KP and DMF prolonged the lifespan of C. elegans
Lifespan analysis of varying concentrations of KP and DMF tested showed that they were able to prolong the lifespan of wild type C. elegans. The doses ranging between 400 –600μg/ml of KP exhibited significant (p < 0.05) increase in lifespan which was up to 25, 27 and 25 days respectively. Likewise, DMF (70 –90μM) exhibited prolonged lifespan, which was up to 27, 27 and 25 days respectively, while the solvent control survived for 22 days similar to control (Fig. 1). The results indicate that there was no change induced by the solvent in the lifespan of the nematodes. The concentrations which showed the maximum survival rate (400 –600μg/ml of KP and 70 –90μM of DMF) were taken for further experiments.
Fig. 1
KP and DMF could extend the maximum lifespan in C. elegans. (A) Average maximum survival rate of KP extract (100 –1000μg/ml) in N2 worms, wherein 400 –600μg/ml exhibited significant (p < 0.05) extension of lifespan when compared to control (B) KP extract (400 –600μg/ml) significantly (p < 0.05) prolonged the lifespan of nematodes to 25, 27 and 25 days respectively (C) Average maximum survival rate of DMF (10 –100μM) in N2 worms, wherein 70 –90μM exhibited significant (p < 0.05) extension of lifespan when compared to control (D) DMF (70 –90μM) significantly (p < 0.05) prolonged the lifespan of nematodes to 27, 27 and 25 days respectively.
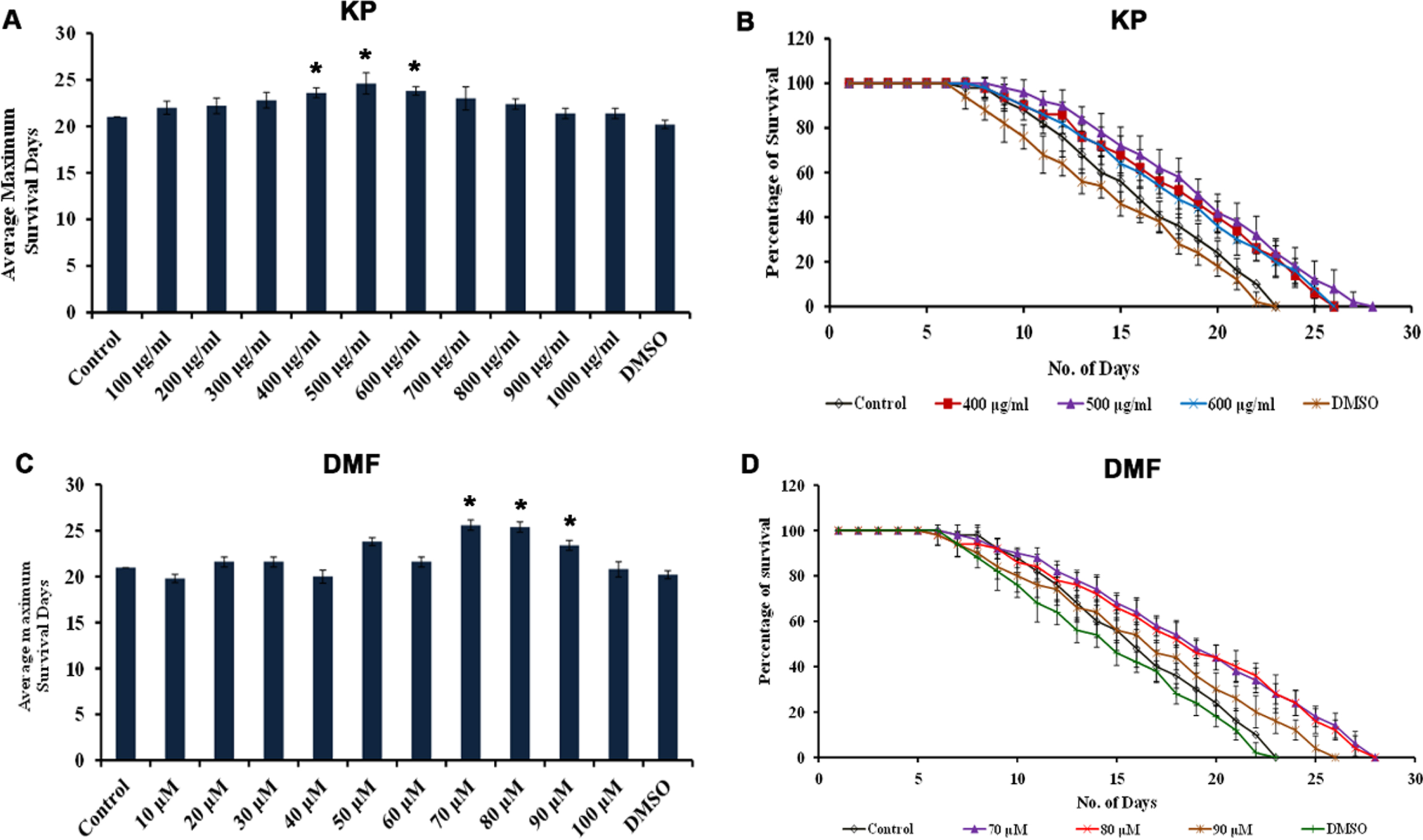
3.2KP and DMF augmented the healthspan of C. elegans
For pharyngeal pumping a single dose of KP (500μg/ml) and DMF (80μM) individually were analyzed. Upon treatment with KP/DMF, a significant improvement in the pharyngeal pumping were observed compared to control (Fig. 2A). The brood size of the nematodes also improved slightly after treatment with KP and DMF however, it was not significant (Fig. 2B).
Fig. 2
Influence of KP and DMF on (A) Pharyngeal pumping rate (B) Reproductive behavior. Pharyngeal pumping rate was monitored on Day 0, Day 3, Day 6 and Day 10. Both KP (D6 and D10) and DMF (D10) were able to significantly (p < 0.05) prolong the pumping rate.
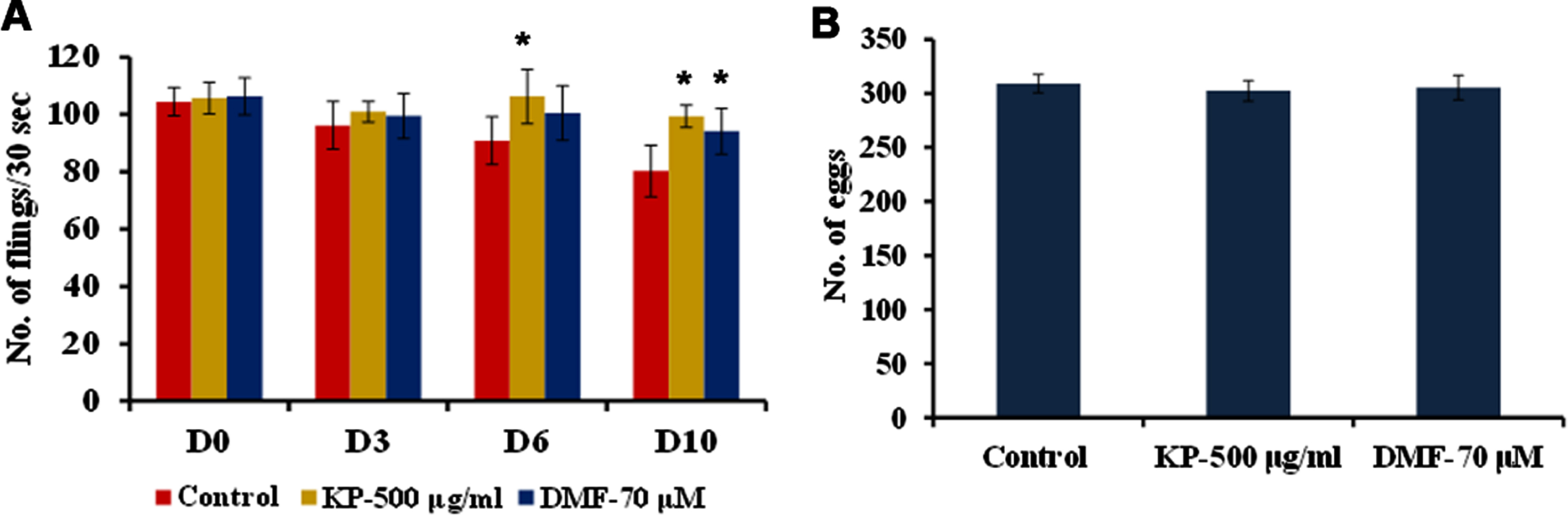
In addition, the expression of lipofuscin, which is a marker of aging in the nematodes was analyzed upon treatment with 400 –600μg/ml of KP and 70 –90μM of DMF. A significant attenuation in the fluorescent level of lipofuscin accumulation was observed in all the tested concentrations compared to control (Fig. 3), implying that both KP and DMF were successful in slowing down the aging process, as it could improve pharyngeal pumping and reduce the lipofuscin level without changing the brood size.
Fig. 3
Effect of KP and DMF on the aging marker lipofuscin in N2 nematodes. (A) Control (B) KP-400μg/ml (C) KP-500μg/ml (D) KP-600μg/ml (E) DMF-70μM (F) DMF-80μM (G) DMF-90μM (H) Quantification of lipofuscin fluorescence intensity in N2 worms treated with different concentrations of KP and DMF. All the tested concentrations exhibited significant (p < 0.05; control vs treated; n = 10) reduction in lipofuscin levels.
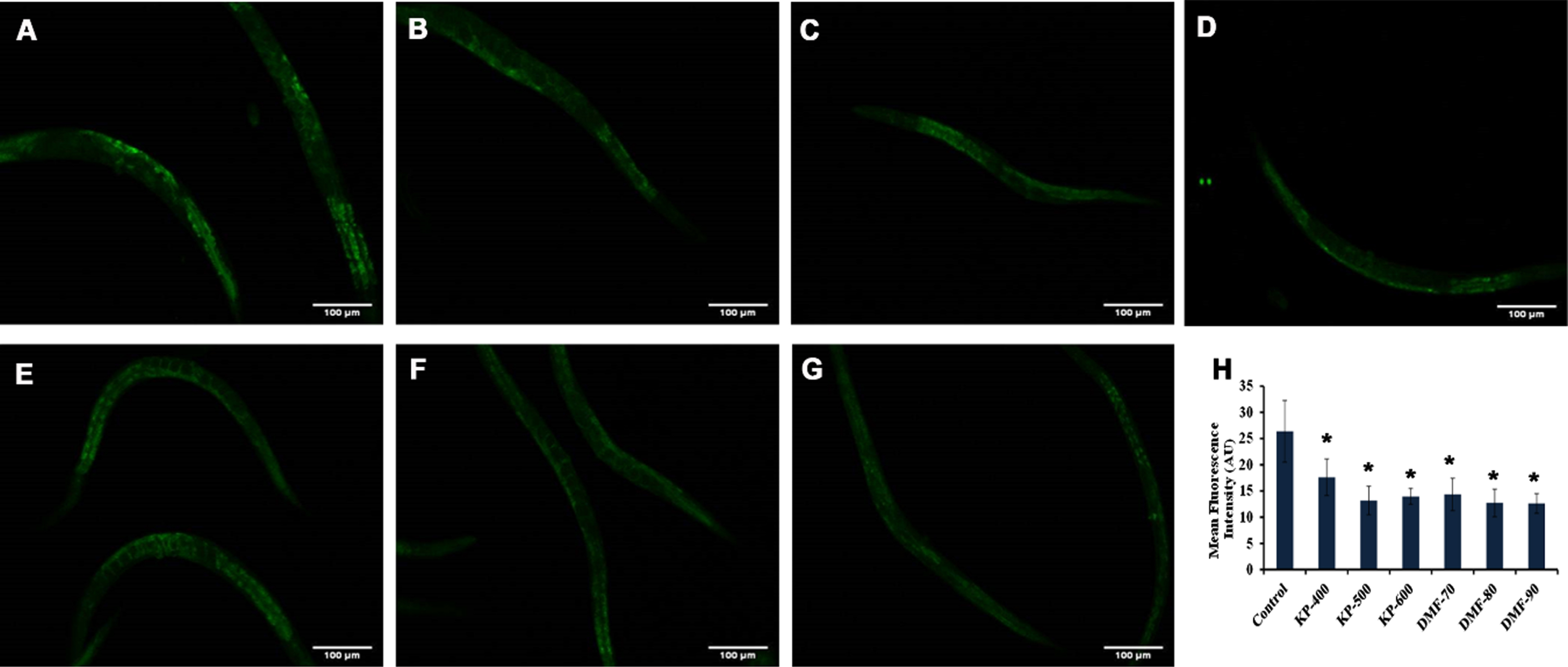
3.3KP and DMF imparts protection against Aβ in transgenic C. elegans
The transgenic strains of C. elegans CL2006 (constitutive expression) and CL4176 (upon temperature upshift) that expresses Aβ1–42 were used to study the neuroprotective effect of KP and DMF. Both KP and DMF were observed to prolong the lifespan of the CL2006 worms significantly as they survived up to 19, 20 and 19 days when treated with KP (400 –600μg/ml) and 20, 21 and 19 days when treated with DMF (70 –90μM) upon comparison with control which survived for 17 and 18 days respectively (Fig. 4) suggesting the neuroprotective potential of the extract.
Fig. 4
KP and DMF can impart neuroprotection in Aβ transgenic strain CL2006 (A) Maximum lifespan upon treatment with 400 –600μg/ml KP, wherein 500μg/ml exhibited significant (p < 0.05) extension of lifespan when compared to control (B) KP extract (400 –600μg/ml) prolonged the lifespan in CL2006 nematodes to offer neuroprotection against Aβ expression (C) Maximum lifespan upon treatment with 70 –90μM DMF, wherein 80 –90μM exhibited significant (p < 0.05) extension of lifespan when compared to control (D) DMF (70 –90μM) prolonged the lifespan in CL2006 nematodes.
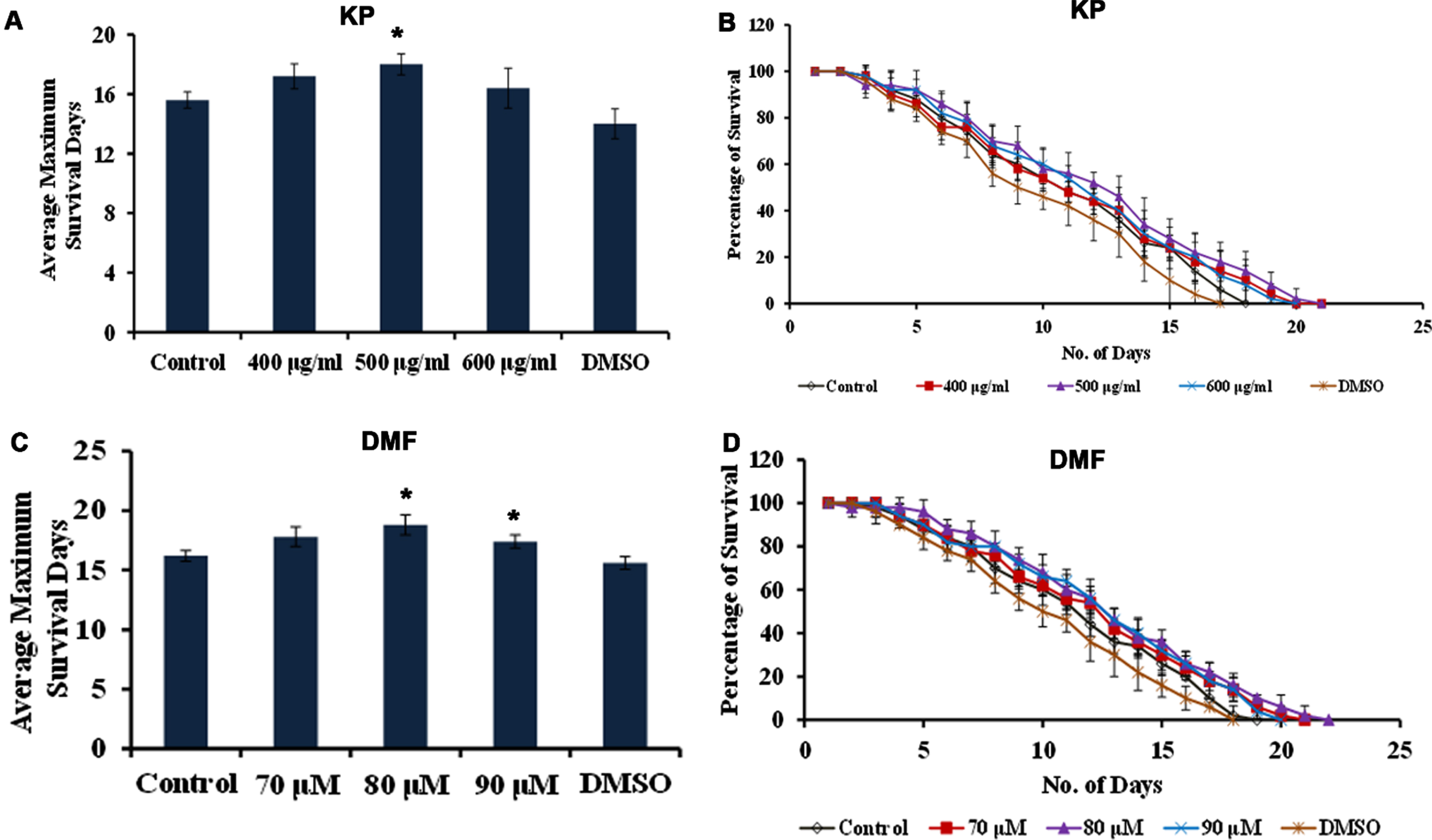
The transgenic strain CL4176 expresses Aβ1–42 in the body wall muscles when the temperature is upshifted resulting in paralysis of the nematodes. Both KP and DMF significantly (p < 0.05) delayed the paralysis, as the worms remained active until 34, 35 and 34 h when treated with KP (400 –600μg/ml) and 35, 35 and 34 h when treated with DMF (70 –90μM) when compared to controls which remained active until 32 h (Fig. 5) suggesting the neuroprotective potential of the extract.
Fig. 5
Effect of KP and DMF on paralysis in Aβ transgenic strain CL4176 (A) KP 400 –600μg/ml (B) DMF 70 –90μM. Treatment with KP and DMF significantly (p < 0.05) diminished Aβ-induced paralysis when compared to control.
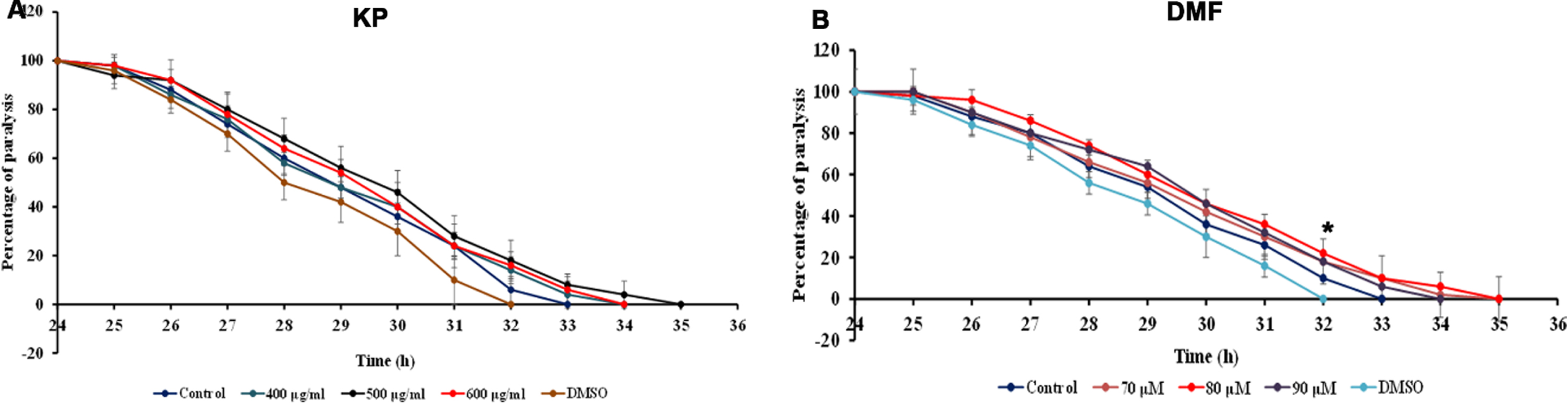
3.4KP and DMF exhibits anti-photoaging in C. elegans
The protective as well as repair effects of varying concentrations of KP/DMF was analyzed upon pre- and post- exposure to UV-A. A significant (p < 0.05) increase of lifespan in worms pre-treated with KP and DMF was observed upon UV-A exposure (Fig. 6). The post-treated worms also showed extended lifespan when compared to control, which was not as significant as the pre-treatment (Fig. 6).
Fig. 6
Pre-treatment with KP and DMF exhibit anti-photoaging potential against UV-A exposure. Graphs representing (A) Mean survival days by pre- and post-treatment of KP (400 –600μg/ml) upon exposure to UV-A (B) Lifespan extension by pre- and post-treatment of KP (400 –600μg/ml) during UV-A exposure (C) Mean survival days by pre- and post-treatment of DMF (70 –90μM) upon UV-A exposure (D) Lifespan extension by pre- and post-treatment of DMF (70 –90μM) during UV-A exposure. The pre-treatment of KP and DMF displayed significant (p < 0.05) anti-photo-aging effect compared to UV-A exposure.
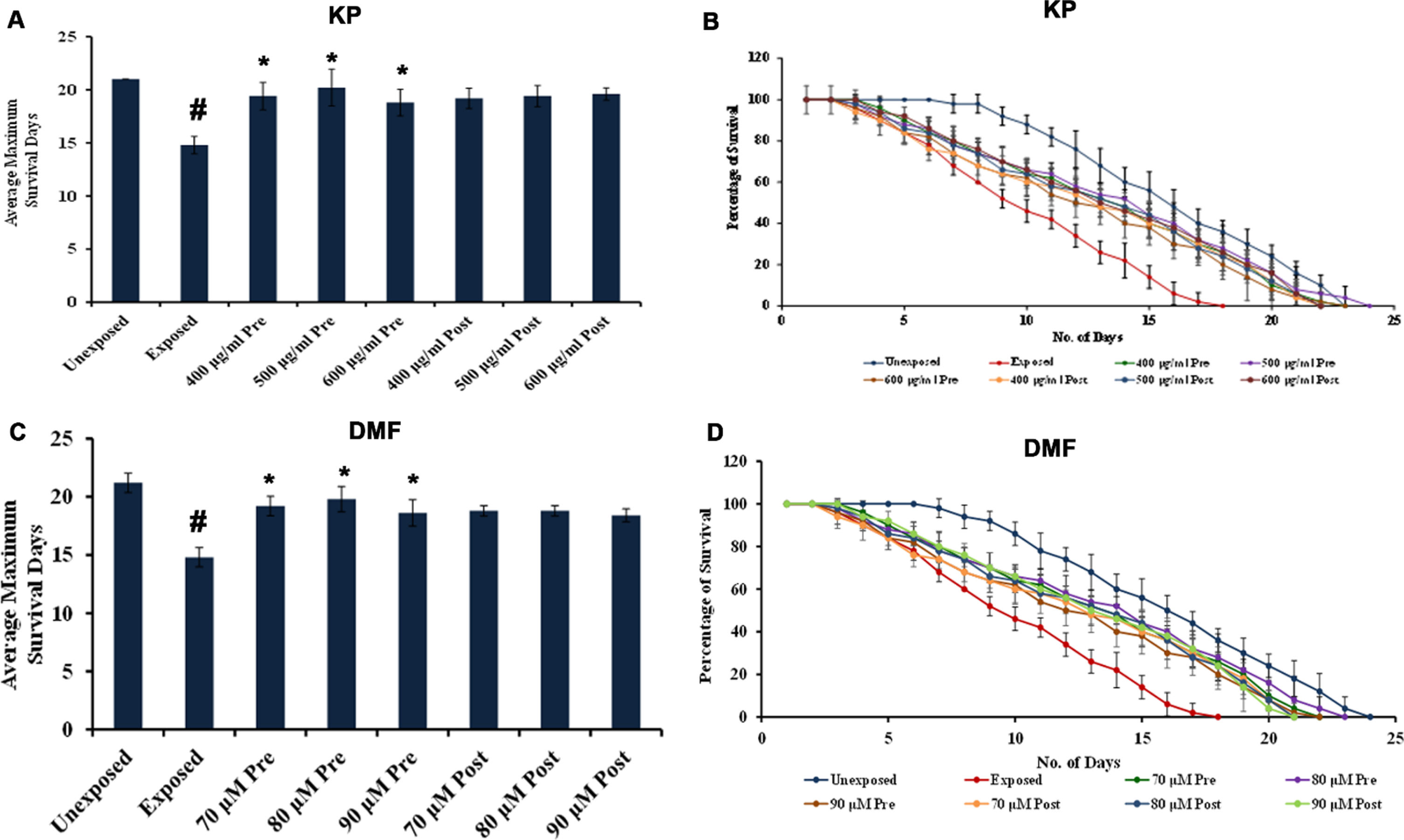
Exposure to UV-A lead to the formation of ROS in C. elegans. On that note, the antioxidative property of KP (400 –600μg/ml) and DMF (70 –90μM) was analyzed. Both KP and DMF treatment showed a significant (p < 0.05) reduction in ROS, which could be manifested from the diminution in fluorescence intensity indicating its protective effect against ROS formation (Fig. 7). The results indicate that KP/DMF can counteract ROS formation and could offer protection against UV-A induced photoaging to the nematodes.
Fig. 7
KP and DMF could reduce ROS formation (A) Quantification of ROS fluorescence intensity in N2 worms pre-treated with KP (400 –600μg/ml) and DMF (70 –90μM) (n = 10; significance at p < 0.05 # Control vs UV-A treated; *UV-A treated vs UV-A + KP/DMF treated Representative image of worm showing the level of ROS (B) Un-exposed control (C) UV-A exposed (D –F) KP (400 –600μg/ml) pre-treatment + UV-A exposure (G –I) DMF (70 –90μM) pre-treatment + UV-A exposure.
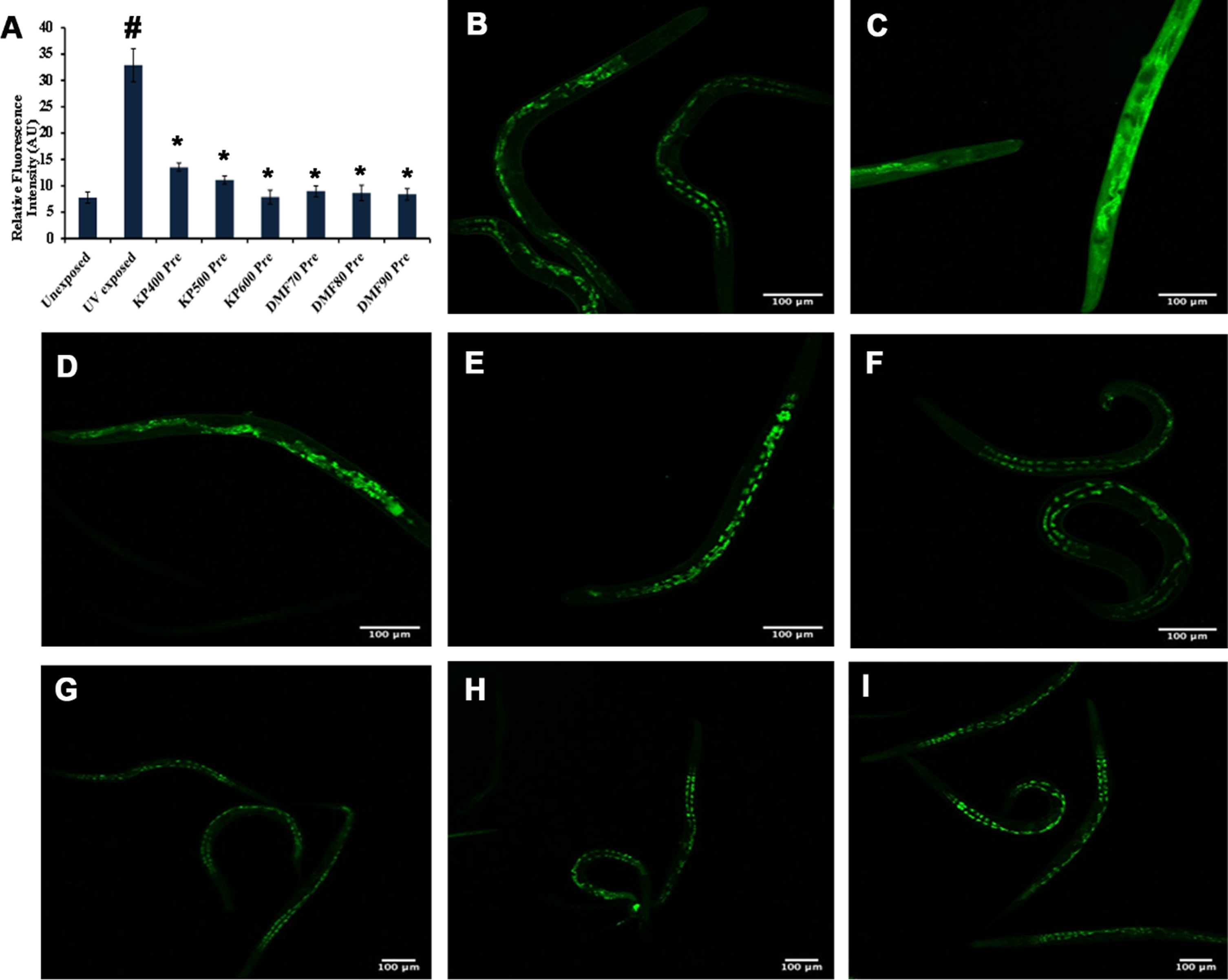
3.5KP and DMF induced SKN-1 in C. elegans
The interaction between SKN-1 and DMF was analyzed by docking approach and the binding affinity was compared with that of the one of the known positive regulators, resveratrol. DMF showed high binding affinity (–8.932 kcal/mol) towards SKN-1 with π-anion interaction, amide-π stacked and carbon-hydrogen interactions, when compared to resveratrol (–7.313 kcal/mol) indicating the phytochemical can activate SKN-1 (Fig. 8A, B). The data observed from in silico analysis was further confirmed using qPCR analysis of skn-1 in wild type nematodes (Fig. 8C) and localization of skn-1 in skn-1::GFP transgenic strain LG333. The qPCR analysis showed significant upregulation of skn-1 when treated with KP and DMF. Similarly, a significant (p < 0.05) increase in the fluorescence level was displayed in LG333 strain when treated with KP (600μg/ml) and DMF (90μM) implying the activation of skn-1 (Fig. 9). The results suggests that the activation of skn-1 by KP/DMF might be the reason for the activation of the antioxidant mechanism to offer protection against various insults.
Fig. 8
In silico analysis showed activation of SKN-1 (A) Resveratrol showing binding affinity of –7.313 kcal/mol towards SKN-1 (B) DMF showing binding affinity of –8.932 kcal/mol towards SKN-1 (C) KP and DMF significantly (p < 0.05) induced skn-1 gene expression in C. elegans.
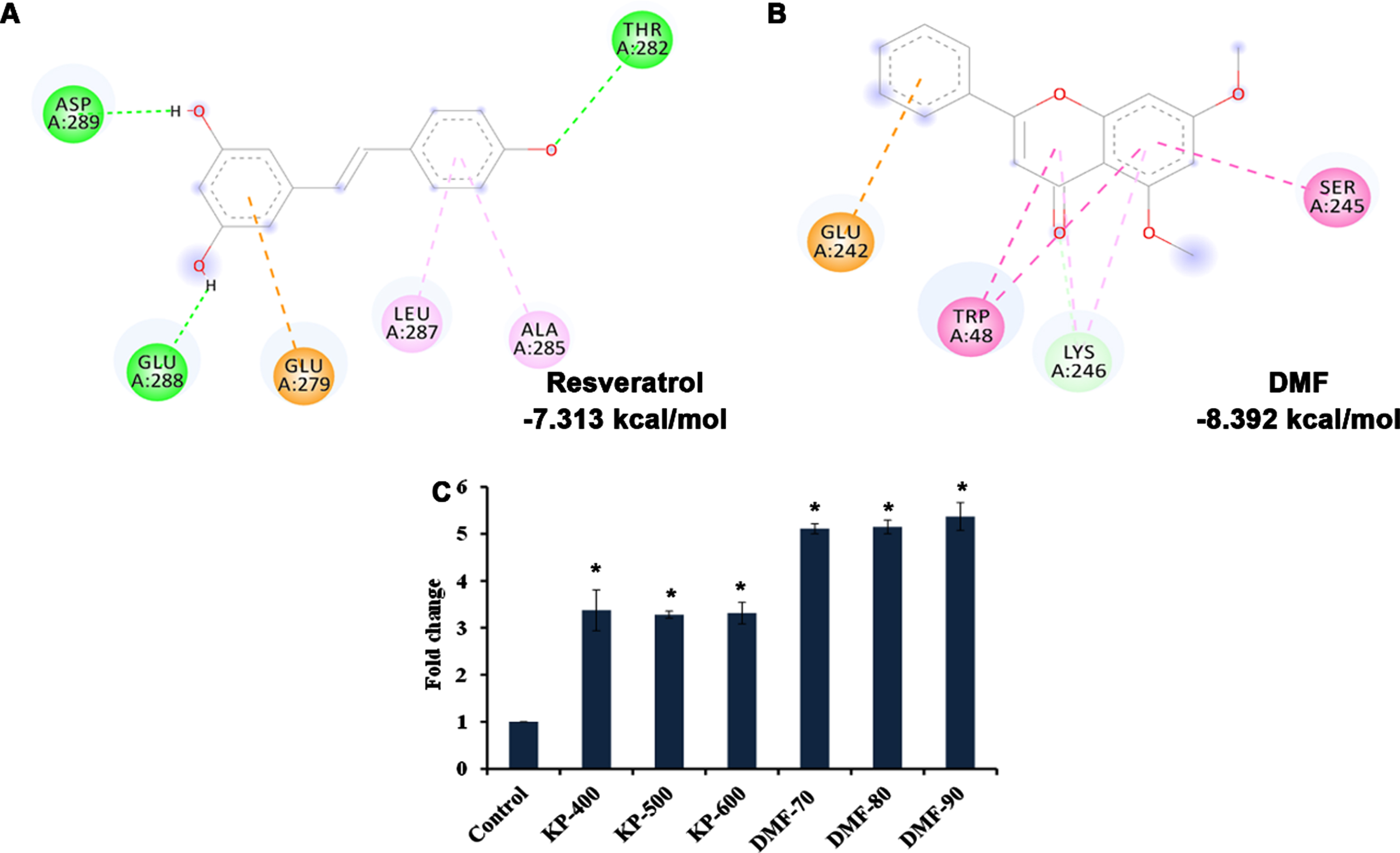
Fig. 9
KP and DMF could activate SKN-1 in LG333 transgenic strain (A) Control (B –D) KP (400 –600μg/ml) wherein 600μg/ml showing significance (p < 0.05) (E –G) DMF (70 –90μM) wherein 90μM showing significance (p < 0.05) (H) Quantification of SKN-1 fluorescence intensity of LG333 transgenic worms upon treatment with KP (400 –600μg/ml) and DMF (70 –90μM) (* represents significance at p < 0.05; n = 10).
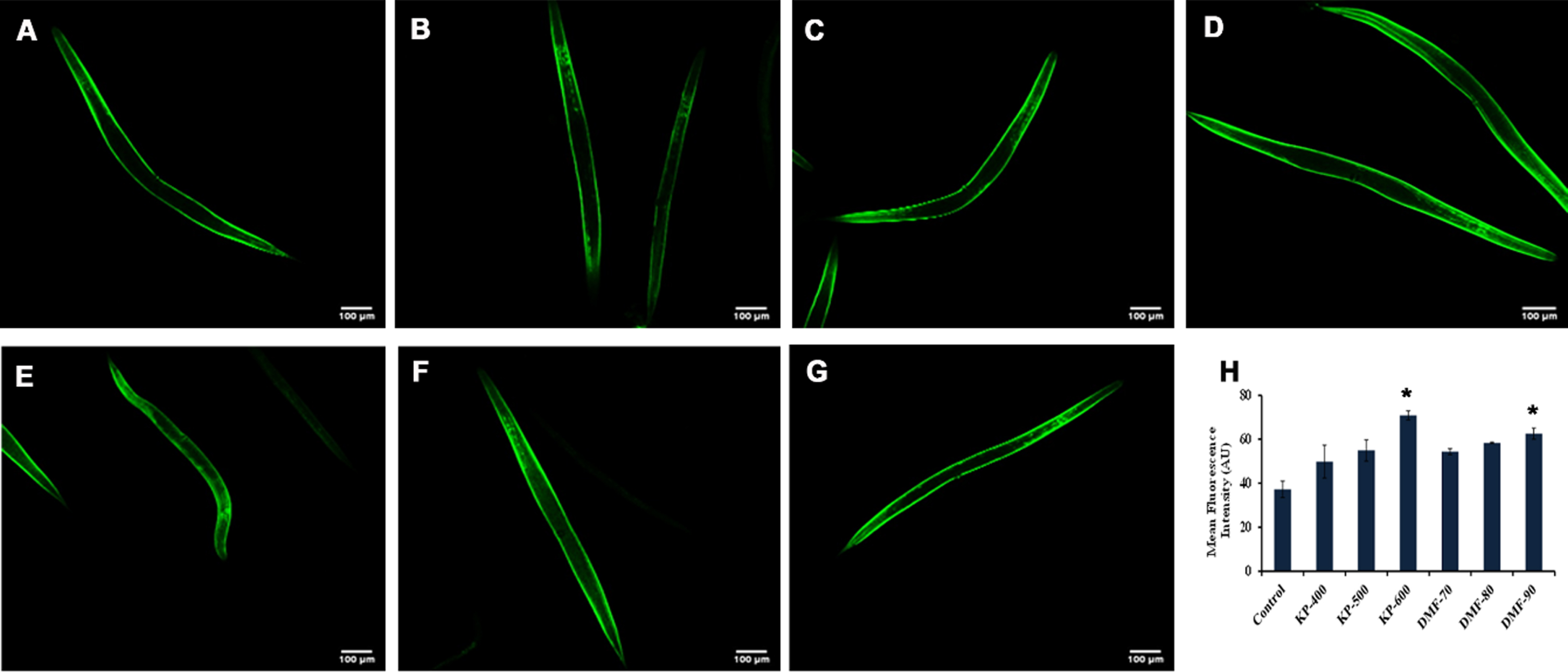
3.6KP and DMF mediated effects is dependent of DAF-16 pathway
Further, CF1038 strain (DAF-16 mutant) and CB1370 strain (DAF-2 mutant) were treated with both KP (400 –600μg/ml) and DMF (70 –90μM) and the survival rate was monitored. The DAF-2 mutants showed survival up to 50, 50 and 49 days when treated with KP (400 –600μg/ml) and 48, 48 and 48 days when treated with DMF (70 –90μM) wherein the untreated control survived up to 49 days and the DMSO treated worms survived up to 48 days respectively (Fig. 10). Meanwhile, DAF-16 mutants survived up to 16, 16 and 15 days when treated with KP (400 –600μg/ml) and 15, 16 and 16 days when treated with DMF (70 –90μM) wherein the untreated control survived up to 15 days (Fig. 11). There was no significant change in the lifespan of both the mutants upon treatment with KP and DMF indicating the importance of DAF-16 mediated pathway in the health benefits mediated by KP and DMF. The results are further substantiated with in silico studies. The mode of interaction of DMF with DAF-16 was analyzed with resveratrol as the reference compound. DMF showed interaction with the amino acid residues of DAF-16 through hydrogen bonding and carbon-hydrogen bonding with a higher binding energy of –8.032 kcal/mol when compared to that of resveratrol –7.668 kcal/mol indicating the compound can interact with the protein and activate the pathway (Fig. 12A, B). The qPCR analysis also showed upregulation of daf-16 upon treatment with KP and DMF, further substantiating the obtained results (Fig. 12C).
Fig. 10
KP and DMF displays DAF-2-dependent increase in lifespan (A) Average maximum lifespan of daf-2 mutants upon treatment with KP (400 –600μg/ml) (B) Lifespan extension effect of KP (400 –600μg/ml) in daf-2 mutants (C) Average maximum lifespan of daf-2 mutants upon treatment with DMF (70 –90μM) (D) Lifespan extension effect DMF (70 –90μM) in daf-2 mutants.
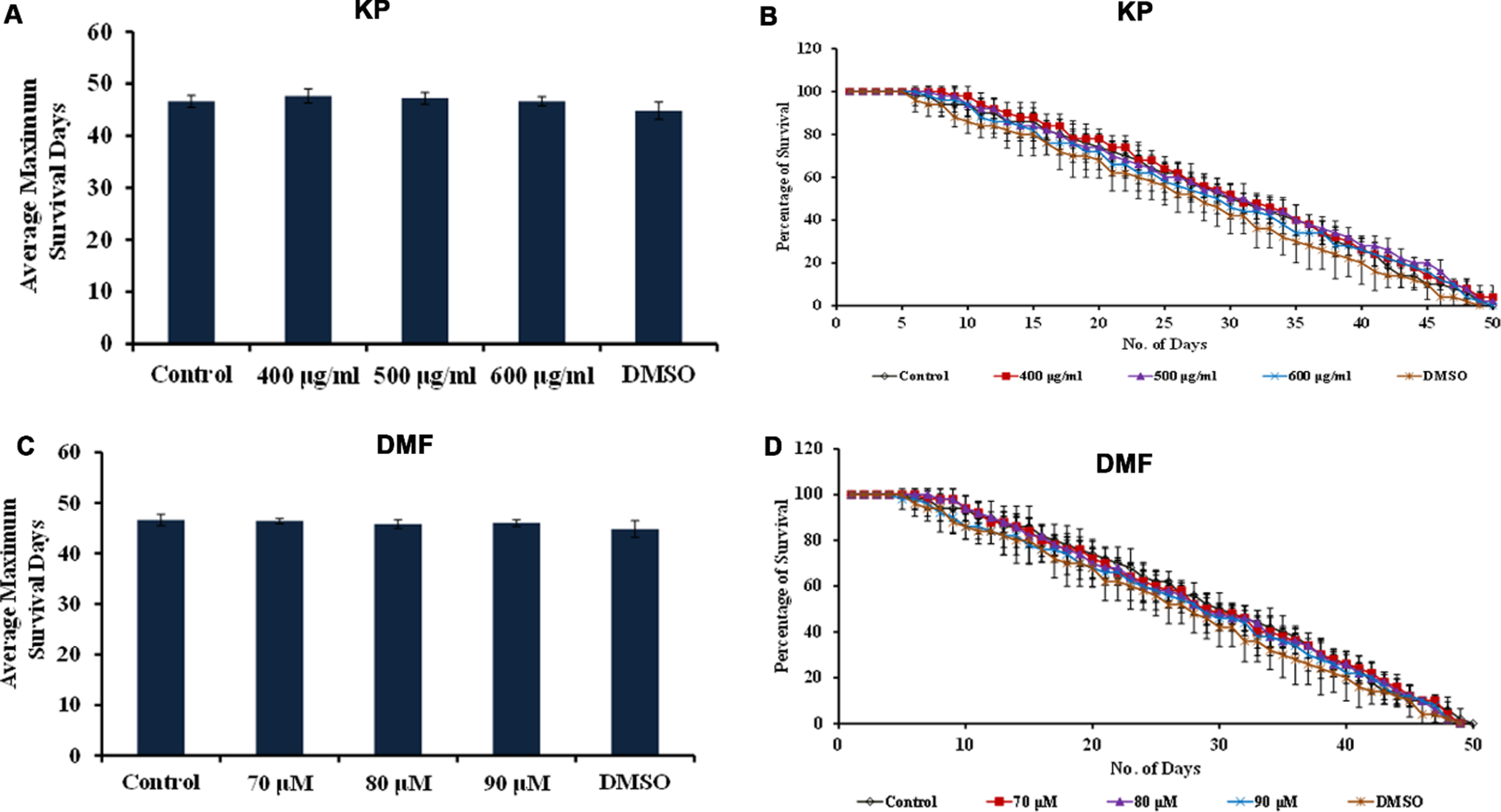
Fig. 11
KP and DMF displays DAF-16-dependent increase in lifespan (A) Average maximum lifespan of daf-16 mutants when treated with KP (400 –600μg/ml) (B) Lifespan extension effect of KP (400 –600μg/ml) in daf-16 mutants (C) Average maximum lifespan of daf-16 mutants when treated with DMF (70 –90μM) (D) Lifespan extension effect of KP (400 –600μg/ml) in daf-16 mutants.
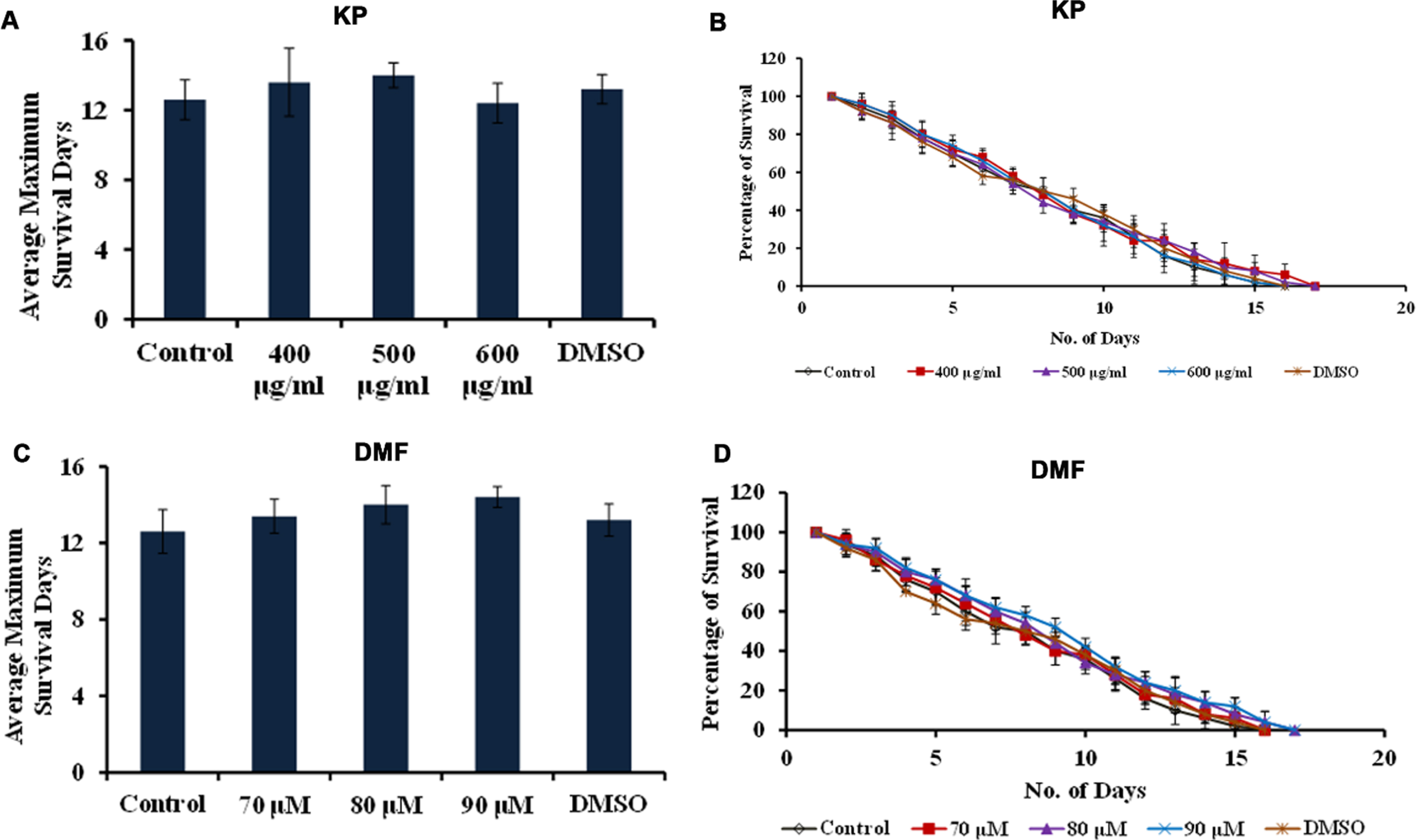
Fig. 12
In silico analysis showed activation of DAF-16 (A) Resveratrol showing binding affinity of –7.668 kcal/mol towards DAF-16 (B) DMF showing binding affinity of –8.032 kcal/mol towards DAF-16 (C) KP and DMF significantly (p < 0.05) induced the expression of daf-16 in C. elegans.
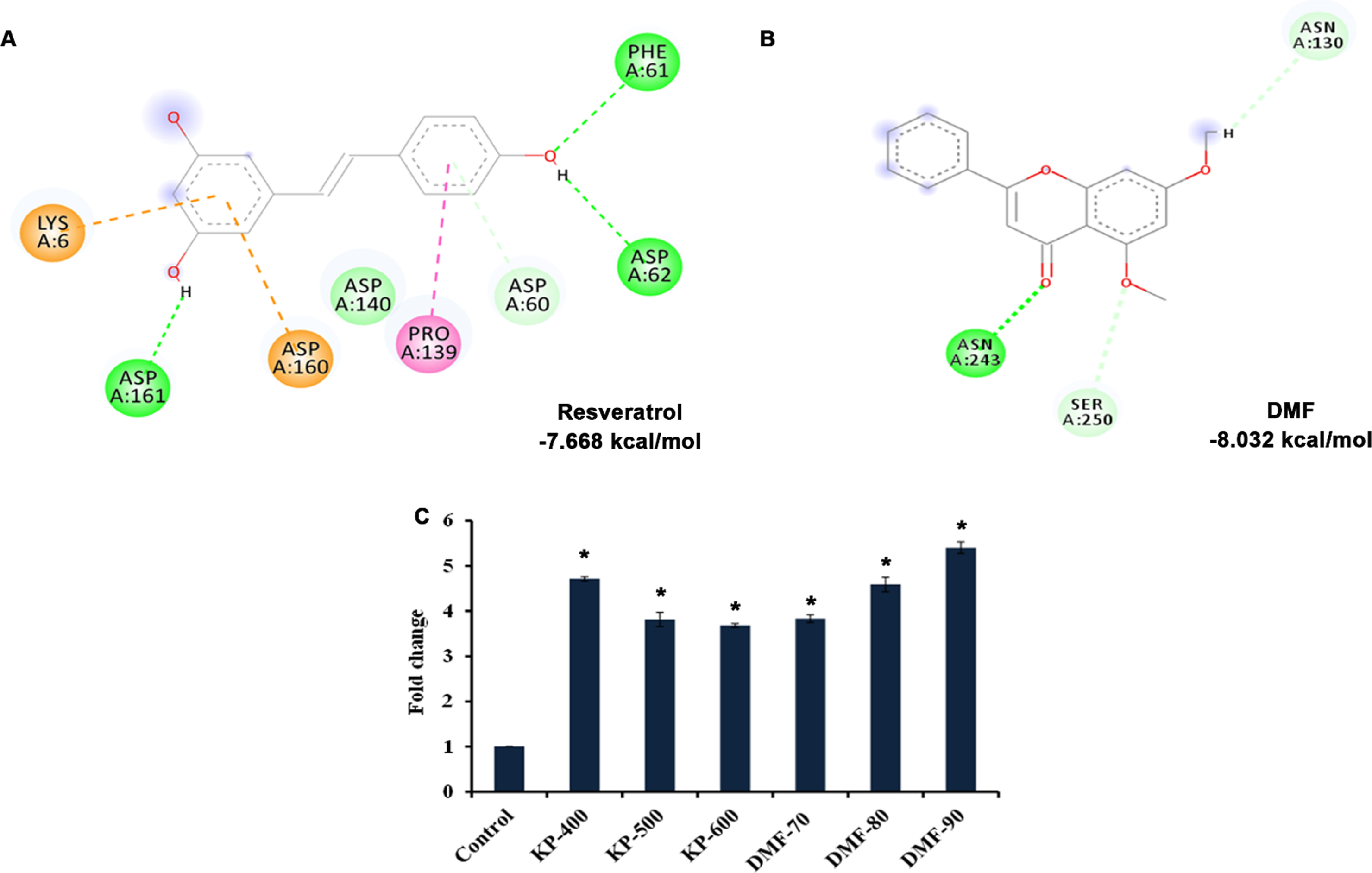
4Discussion
Aging and diseases or disorders associated with aging (dermatological, cardiovascular, neurodegenerative) were always prominent area of research in medicine. Many herbal plants known for their traditional health benefits have been used by researchers to slow down the rate of aging and to reduce the severity of age-related disorders. These plants act as antiaging and antioxidant factors, improve cognitive impairments, reduce oxidative stress and other age associated markers, and reduce cardiovascular and neurodegenerative risks, through physiological and molecular mechanisms. Different bioactive compounds in these plants makes it multi-faceted with limited or negligible side effects, making it one of the most preferred medication [26–28].
K. parviflora is one such herb, which has been pharmacologically proven to have health enhancing properties and the main effector components were methoxyflavones, which majorly includes DMF [29]. Pioneering studies have been done with KP to identify the anti-cancer activity by increasing apoptosis or inhibiting melanogenesis, neuroprotective activity by improving memory and related neurotransmitters such as serotonin and dopamine, and other metabolism related activities such as maintaining energy expenditure and physical fitness, improving antioxidant levels, increasing the blood flow to different organs, increasing the sperm and testosterone levels and reducing the impact of photoaging, among many more [4, 5]. However, the mode of action elicited by KP in incorporating these health benefits are still unclear. The current study was intended to decipher the anti-aging and neuroprotective effect of KP and DMF using C. elegans as the model.
The toxicological evaluation of KP rhizome extract through mutagenicity and sub-chronic toxicity analysis has revealed no genotoxic or histopathological effects and instead was proven safe for daily consumption, in humans as well [30, 31]. In the present study, the toxicity and anti-aging properties were evaluated in wild type C. elegans. KP (100 –1000μg/ml) and DMF (10 –100μM) were able to extend the lifespan at all doses without any specific toxicity at any doses, wherein selective doses were able to significantly extend lifespan (Fig. 1). KP extract was able to induce anti-aging activity in fibroblasts and experimental animals by attenuating senescence and activating other molecular mechanisms [32]. DMF was previously identified for its anti-aging activity, as it reduced sarcopenia (muscle aging) in mice by improving mitochondrial function and protein turnover [33].
Apart from mediating lifespan, KP and DMF were able to mediate the healthspan of the nematodes as well. Both the treatments improved pharyngeal pumping of the nematodes while reducing the accumulation of lipofuscin (Fig. 2, 3), which are known markers of healthspan [14, 15]. Both KP and DMF were found to enhance energy metabolism in myocytes by mediating the phosphorylation of AMP activated protein kinase [34]. KP was able to inhibit fat accumulation and muscle atrophy [35], improve physical fitness and muscular endurance [36], and improve energy metabolism by activating brown adipose tissue [37] in mice models. The KP extract was also able to improve physical fitness, heart rate and oxygen consumption in a randomized double-blind trial [38, 39], reduce body fat [40] and the polymethoxyflavones from KP were found to reduce visceral fat area and subcutaneous fat area in another randomised, double-blind, placebo-controlled trial [41]. These reports fall parallel to the point that both KP and DMF could improve the healthspan.
The neuroprotective efficacy of both KP (400 –600μg/ml) and DMF (70 –90μM) was monitored in the transgenic strain which could express Aβ. It was observed that, both KP and DMF could prolong the lifespan of the Aβ transgenic strain, CL2006 (Fig. 4), indicating neuroprotection [42]. Another Aβ transgenic strain, CL4176, expresses Aβ in the body wall muscle, which is accompanied by paralysis upon temperature upshift from 15 °C to 25 °C [21]. Both KP (400 –600μg/ml) and DMF (70 –90μM) were able to significantly delay the paralysis phenotype suggesting that it could induce neuroprotection in C. elegans (Fig. 5). The KP extract and the methoxyflavones present in it were able to increase the transcription of cAMP response element in PC12D cells, which is essential for long term memory thereby preventing cognitive decline [43]. Additionally, KP improved the learning and memory in mice and prevented the brain from valproic acid mediated toxicity by increasing the level of doublecortin in sub granular zone of brain [44]. KP also mediated different neurotransmitters by increasing serotonin, norepinephrine and dopamine in the rat hippocampus [10]. DMF exhibited butyrylcholinesterase inhibitory activity [45], whereas another methoxyflavone, 3,5,7,3’,4’-pentamethoxyflavone exhibited acetylcholinesterase inhibitory activity [46].
Exposure to ultraviolet (UV) radiation will induce photoaging by creating oxidative stress, which will disrupt the antioxidant mechanism of the host [47]. In this regard, the antioxidant potential of KP and DMF were monitored upon UV-A-induced oxidative stress, which has been reported to induce photoaging [20, 48]. The pre-treatment of KP/DMF was able to significantly prolong the lifespan of worms exposed to UV-A indicating the protective effect of KP and DMF against UV-A induced photoaging (Fig. 6).
One of the major markers in UV-A-induced photoaging in C. elegans is the elevation of ROS level [48]. ROS can be categorized into intracellular and extracellular ROS, wherein the former is generated due to the damaged mitochondrial function and the latter is induced by NADPH oxidase (NOX) [49]. In addition, the generation of either of the ROS can complement the induction of the other contributing to the oxidative microenvironment. Further, intra- and extracellular ROS can damage proteins, lipids, carbohydrates and DNA in the cells, oxidize lipoproteins and activate collagen degrading matrix metalloproteases, respectively which can have irreversible damages to the host cells [50]. Although low level of ROS can contribute to antioxidant defense mechanism, increase in ROS is associated with aging and associated diseases through epigenetic modulations [51–53]. It was observed that the worms exhibited significant reduction in fluorescence upon KP/DMF treatment indicating the inhibition of accumulation of ROS during UV-A exposure suggesting its antioxidative and anti-photoaging potential (Fig. 7). Both KP and DMF were reported to inhibit the production of cellular ROS in pyocyanin-stimulated HUVECs [54]. The antioxidant property of KP was instrumental in eliciting anti-inflammatory effect in mice skin tissue when exposed to solar UV (95% UV-A) radiation [55]. In another study wherein obese mice were exposed to UV-B, the KP extract reduced the harmful effects by reducing the level of subcutaneous adipocyte tissues and retaining the structure of collagenous fibers in the dermis [56]. The KP extract was also able to prevent the loss of collagen fibers and prevent wrinkle formation by mediating the expression of matrix metalloproteases and increasing the expression of catalase and other inflammatory mediators in hairless mice during UV-B induced photoaging [57].
The evolutionarily conserved mammalian Nrf-2 is responsible for antioxidant, anti-inflammatory and lifespan extending properties. The ortholog of Nrf-2 in C. elegans is SKN-1. SKN-1 plays diverse role in C. elegans that could promote longevity, indicating the importance of SKN-1 in mediating aging and other mechanisms [58, 59]. The molecular docking analysis revealed that DMF could bind effectively with the target SKN-1 with higher energy and activate the pathway when compared to that of the known SKN-1 activator resveratrol (Fig. 8) [22, 60]. The upregulation of skn-1 after the treatment with KP and DMF also supported this view. The activation of SKN-1 was further confirmed using the transgenic strain LG333 treated with KP and DMF. It was revealed that both KP and DMF could activate skn-1 which was proportional to the fluorescence intensity expressed by the nematodes (Fig. 9) [61, 62]. SKN-1 was already reported to mediate anti-aging and neuroprotection in C. elegans. Even though there are no direct reports suggesting the activation of SKN-1 by KP extract, several evidences suggest that quercetin present in KP has better binding affinity with sirtuins, and the extract could elevate sirtuin catalytic activity, suggesting the activation of this antioxidant enzyme [64, 65].
SKN-1 acts in parallel with DAF-16, the forkhead box transcription factor homolog in C. elegans, to mediate anti-aging and stress resistance. DAF-2, the ortholog of insulin/insulin-like growth factor, mediates the activation of DAF-16. In simple terms, reduction in DAF-2 causes the activation of DAF-16 leading to lifespan extension, stress resistance and other positive effects [59, 66]. The contribution of DAF-16 mediated pathway in KP and DMF initiated lifespan extension was monitored by analyzing the effect of both KP and DMF in the mutants of daf-2 and daf-16 individually. Both the mutants did not display any significant changes in the lifespan when treated with KP or DMF indicating the importance of DAF-16 mediated pathway in the anti-aging and neuroprotective efficacies (Fig. 10, 11) [48, 67]. The qPCR analysis also suggests the activation of daf-16 when treated with KP and DMF. This was further confirmed through molecular docking analysis wherein DMF exhibited better binding affinity with DAF-16 when compared to resveratrol indicating the activation of DAF-16 (Fig. 12).
The KP extract was previously reported to mediate the activity of phosphatidylinositol 3 kinase and thereby mediate the forkhead box O3a in human dermal fibroblasts leading to anti-aging activity [32] and the same effect was observed in obese mice, which lead to anti-obesity and anti-aging potential by KP extract [35]. DMF was reported to stimulate the phosphatidylinositol 3-kinase-Akt pathway and mediate the phosphorylation of Forkhead box O3 in mice thereby reducing sarcopenia [33]. Our results indicate that the anti-aging and neuroprotective properties of KP and DMF in C. elegans were mediated by DAF-16 pathway along with SKN-1.
5Conclusions
Aging and age-related diseases are associated with elevated oxidative stress and attenuation of antioxidant mechanisms in the host. Our study shows that traditionally important Kaempferia parviflora extract and its phytoconstituent DMF exerts anti-aging and neuroprotection in the model organism C. elegans via SKN-1 and DAF-16 pathway. With the epidemiological evidences pointing on the role of nutritional antioxidants against several pathological conditions, K. parviflora could be used as a nutraceutical as a booster against age-associated disorders.
Acknowledgments
M.I.P. and J.M.B. wish to thank the Ratchadaphiseksomphot Endowment Fund for Postdoctoral Fellowship, Chulalongkorn University, Thailand, for the support. D.S.M wishes to thank the Second Century Fund (C2F), Chulalongkorn University, Thailand, for the support. The authors would like to thank Natural Products for Neuroprotection and Anti-ageing Research Unit and Ratchadaphiseksomphot Endowment Fund for the support.
Funding
This study was supported by a Grant from the Ratchadaphiseksomphot Endowment Fund (T.T).
Conflicts of interest
The authors declare no conflicts of interest.
References
[1] | Dai X , Guo X , Decoding and rejuvenating human ageing genomes: Lessons from mosaic chromosomal alterations. Ageing Res Rev. (2021) ;68: :101342. |
[2] | El Gaamouch F , Liu K , Lin HY , Wu C , Wang J , Development of grape polyphenols as multi-targeting strategies for Alzheimer’s disease. Neurochem Int. (2021) ;147: :105046. |
[3] | Mehla J , Gupta P , Pahuja M , Diwan D , Diksha D , Indian Medicinal Herbs and Formulations for Alzheimer’s Disease, from Traditional Knowledge to Scientific Assessment. Brain Sci. (2020) ;10: (12):964. |
[4] | Chen D , Li H , Li W , Feng S , Deng D , Kaempferia parviflora and Its Methoxyflavones: Chemistry and Biological Activities. Evid Based Complement Alternat Med. 2018; 2018:4057456. |
[5] | Saokaew S , Wilairat P , Raktanyakan P , Dilokthornsakul P , Dhippayom T , Kongkaew C , Sruamsiri R , Chuthaputti A , Chaiyakunapruk N , Clinical Effects of Krachaidum (Kaempferia parviflora): A Systematic Review. J Evid Based Complementary Altern Med. (2017) ;22: (3):413–28. |
[6] | Yenjai C , Prasanphen K , Daodee S , Wongpanich V , Kittakoop P , Bioactive flavonoids from Kaempferia parviflora. Fitoterapia. (2004) ;75: (1):89–92. |
[7] | Sutthanut K , Sripanidkulchai B , Yenjai C , Jay M , Simultaneous identification and quantitation of 11 flavonoid constituents in Kaempferia parviflora by gas chromatography. J Chromatogr A. (2007) ;1143: (1-2):227–33. |
[8] | Sawasdee P , Sabphon C , Sitthiwongwanit D , Kokpol U , Anticholinesterase activity of 7-methoxyflavones isolated from Kaempferia parviflora. Phytother Res. (2009) ;23: (12):1792–4. |
[9] | Tonsomboon A , Prasanth MI , Plaingam W , Tencomnao T , Kaempferia parviflora Rhizome Extract Inhibits Glutamate-Induced Toxicity in HT-22 Mouse Hippocampal Neuronal Cells and Extends Longevity in Caenorhabditis elegans . Biology (Basel). (2021) ;10: (4):264. |
[10] | Plaingam W , Sangsuthum S , Angkhasirisap W , Tencomnao T , Kaempferia parviflora rhizome extract and Myristica fragrans volatile oil increase the levels of monoamine neurotransmitters and impact the proteomic profiles in the rat hippocampus: Mechanistic insights into their neuroprotective effects. J Tradit Complement Med. (2017) ;7: (4):538–52. |
[11] | Giunti S , Andersen N , Rayes D , De Rosa MJ , Drug discovery: Insights from the invertebrate Caenorhabditis elegans. Pharmacol Res Perspect. (2021) ;9: (2):e00721. |
[12] | Hartman JH , Widmayer SJ , Bergemann CM , King DE , Morton KS , Romersi RF , Jameson LE , Leung MCK , Andersen EC , Taubert S , Meyer JN , Xenobiotic metabolism and transport in Caenorhabditis elegans. J Toxicol Environ Health B Crit Rev. (2021) ;24: (2):51–94. |
[13] | Brimson JM , Prasanth MI , Isidoro C , Sukprasansap M , Tencomnao T , Cleistocalyx nervosum var. paniala seed extracts exhibit sigma-1 antagonist sensitive neuroprotective effects in PC12 cells and protect C. elegans from stress via the SKN-1/NRF-2 pathway. Nutr Healthy Aging. (2021) ;6: :131–46. |
[14] | Prasanth MI , Brimson JM , Chuchawankul S , Sukprasansap M , Tencomnao T , Antiaging, Stress Resistance, and Neuroprotective Efficacies of Cleistocalyx nervosum var. paniala Fruit Extracts Using Caenorhabditis elegans Model. Oxid Med Cell Longev. (2019) ;2019: :7024785. |
[15] | Kittimongkolsuk P , Roxo M , Li H , Chuchawankul S , Wink M , Tencomnao T , Extracts of the Tiger Milk Mushroom (Lignosus rhinocerus) Enhance Stress Resistance and Extend Lifespan in Caenorhabditis elegans via the DAF-16/FoxO Signaling Pathway. Pharmaceuticals (Basel). (2021) ;14: (2):93. |
[16] | Brimson JM , Prasanth MI , Plaingam W , Tencomnao T , Bacopa monnieri (L.) wettst. Extract protects against glutamate toxicity and increases the longevity of Caenorhabditis elegans. J Tradit Complement Med. (2019) ;10: (5):460–70. |
[17] | Rangsinth P , Prasansuklab A , Duangjan C , Gu X , Meemon K , Wink M , Tencomnao T , Leaf extract of Caesalpinia mimosoides enhances oxidative stress resistance and prolongs lifespan in Caenorhabditis elegans. BMC Complement Altern Med. (2019) ;19: (1):164. |
[18] | Alexpandi R , Prasanth MI , Ravi AV , Balamurugan K , Durgadevi R , Srinivasan R , De Mesquita JF , Pandian SK , Protective effect of neglected plant Diplocyclos palmatus on quorum sensing mediated infection of Serratia marcescens and UV-A induced photoaging in model Caenorhabditis elegans. J Photochem Photobiol B. (2019) ;201: :111637. |
[19] | Brenner S , The genetics of Caenorhabditis elegans . Genetics. (1974) ;77: (1):71–94. |
[20] | Prasanth MI , Santoshram GS , Bhaskar JP , Balamurugan K , Ultraviolet-A triggers photoaging in model nematode Caenorhabditis elegans in a DAF-16 dependent pathway. Age (Dordr). (2016) ;38: :27. |
[21] | DanQing L , YuJie G , ChengPeng Z , HongZhi D , Yi H , BiSheng H , Yan C , N-butanol extract of Hedyotis diffusa protects transgenic Caenorhabditis elegans from Aβ-induced toxicity. Phytother Res. (2021) ;35: (2):1048–61. |
[22] | Prasanth MI , Gayathri S , Bhaskar JP , Krishnan V , Balamurugan K , Analyzing the Synergistic Effects of Antioxidants in Combating Photoaging Using Model Nematode, Caenorhabditis elegans. Photochem Photobiol. (2020) ;96: :139–47. |
[23] | Pandey S , Phulara SC , Jha A , Chauhan PS , Gupta P , Shukla V , 3-Methyl-3-buten-1-ol (isoprenol) confers longevity and stress tolerance in Caenorhabditis elegans. Int J Food Sci Nutr. (2019) ;70: (5):595–602. |
[24] | Yang J , Anishchenko I , Park H , Peng Z , Ovchinnikov S , Baker D , Improved protein structure prediction using predicted interresidue orientations. Proc Natl Acad Sci U S A. (2020) ;117: (3):1496–503. |
[25] | Santos KB , Guedes IA , Karl ALM , Dardenne LE , Highly Flexible Ligand Docking: Benchmarking of the DockThor Program on the LEADS-PEP Protein-Peptide Data Set. J Chem Inf Model. (2020) ;60: :667–83. |
[26] | Alzobaidi N , Quasimi H , Emad NA , Alhalmi A , Naqvi M , Bioactive Compounds and Traditional Herbal Medicine: Promising Approaches for the Treatment of Dementia. Degener Neurol Neuromuscul Dis. (2021) ;11: :1–14. |
[27] | Ahuja A , Gupta J , Gupta R , Miracles of Herbal Phytomedicines in Treatment of Skin Disorders: Natural Healthcare Perspective. Infect Disord Drug Targets. (2021) ;21: (3):328–38. |
[28] | Phu HT , Thuan DTB , Nguyen THD , Posadino AM , Eid AH , Pintus G , Herbal Medicine for Slowing Aging and Aging-associated Conditions: Efficacy, Mechanisms and Safety. Curr Vasc Pharmacol. (2020) ;18: (4):369–93. |
[29] | Pitakpawasutthi Y , Palanuvej C , Ruangrungsi N , Quality evaluation of Kaempferia parviflora rhizome with reference to 5,7-dimethoxyflavone. J Adv Pharm Technol Res. (2018) ;9: (1):26–31. |
[30] | Yoshino S , Awa R , Miyake Y , Fukuhara I , Sato H , Endo Y , Tomita S , Kuwahara H , Evaluation of the Safety of Daily Consumption of Kaempferia parviflora Extract (KPFORCE): A Randomized Double-Blind Placebo-Controlled Trial. J Med Food. (2019) ;22: (11):1168–74. |
[31] | Yoshino S , Awa R , Ohto N , Miyake Y , Kuwahara H , Toxicological evaluation of standardized Kaempferia parviflora extract: Sub-chronic and mutagenicity studies. Toxicol Rep. (2019) ;6: :544–9. |
[32] | Park JE , Woo SW , Kim MB , Kim C , Hwang JK , Standardized Kaempferia parviflora Extract Inhibits Intrinsic Aging Process in Human Dermal Fibroblasts and Hairless Mice by Inhibiting Cellular Senescence and Mitochondrial Dysfunction. Evid Based Complement Alternat Med. (2017) ;2017: :6861085. |
[33] | Kim C , Hwang JK , The 5,7-Dimethoxyflavone Suppresses Sarcopenia by Regulating Protein Turnover and Mitochondria Biogenesis-Related Pathways. Nutrients. (2020) ;12: (4):1079. |
[34] | Toda K , Takeda S , Hitoe S , Nakamura S , Matsuda H , Shimoda H , Enhancement of energy production by black ginger extract containing polymethoxy flavonoids in myocytes through improving glucose, lactic acid and lipid metabolism. J Nat Med. (2016) ;70: (2):163–72. |
[35] | Lee S , Kim C , Kwon D , Kim MB , Hwang JK , Standardized Kaempferia parviflora Wall. ex Baker (Zingiberaceae) Extract Inhibits Fat Accumulation and Muscle Atrophy in ob/ob Mice. Evid Based Complement Alternat Med. (2018) ;2018: :8161042. |
[36] | Toda K , Hitoe S , Takeda S , Shimoda H , Black ginger extract increases physical fitness performance and muscular endurance by improving inflammation and energy metabolism. Heliyon. (2016) ;2: (5):e00115. |
[37] | Yoshino S , Kim M , Awa R , Kuwahara H , Kano Y , Kawada T , Kaempferia parviflora extract increases energy consumption through activation of BAT in mice. Food Sci Nutr. (2014) ;2: (6):634–7. |
[38] | Sripanidkulchai B , Promthep K , Tuntiyasawasdikul S , Tabboon P , Areemit R , Supplementation of Kaempferia parviflora Extract Enhances Physical Fitness and Modulates Parameters of Heart Rate Variability in Adolescent Student-Athletes: A Randomized, Double-Blind, Placebo-Controlled Clinical Study. J Diet Suppl. (2020) ;4: :1–18. |
[39] | Promthep K , Eungpinichpong W , Sripanidkulchai B , Chatchawan U , Effect of Kaempferia parviflora Extract on Physical Fitness of Soccer Players: A Randomized Double-Blind Placebo-Controlled Trial. Med Sci Monit Basic Res. (2015) ;21: :100–8. |
[40] | Matsushita M , Yoneshiro T , Aita S , Kamiya T , Kusaba N , Yamaguchi K , Takagaki K , Kameya T , Sugie H , Saito M , Kaempferia parviflora extract increases whole-body energy expenditure in humans: roles of brown adipose tissue. J Nutr Sci Vitaminol (Tokyo). (2015) ;61: (1):79–83. |
[41] | Yoshino S , Tagawa T , Awa R , Ogasawara J , Kuwahara H , Fukuhara I , Polymethoxyflavone purified from Kaempferia parviflora reduces visceral fat in Japanese overweight individuals: a randomised, double-blind, placebo-controlled study. Food Funct. (2021) ;12: (4):1603–13. |
[42] | Malar DS , Prasanth MI , Jeyakumar M , Balamurugan K , Devi KP , Vitexin prevents Aβ proteotoxicity in transgenic Caenorhabditis elegans model of Alzheimer’s disease by modulating unfolded protein response. J Biochem Mol Toxicol. (2021) ;35: (1):e22632. |
[43] | Natsume N , Yamano A , Watanabe A , Yonezawa T , Woo JT , Yamakuni T , Teruya T , Effect of methoxyflavones contained in Kaempferia parviflora on CRE-mediated transcription in PC12D cells. Bioorg Med Chem Lett. (2020) ;30: (23):127606. |
[44] | Welbat JU , Chaisawang P , Chaijaroonkhanarak W , Prachaney P , Pannangrong W , Sripanidkulchai B , Wigmore P , Kaempferia parviflora extract ameliorates the cognitive impairments and the reduction in cell proliferation induced by valproic acid treatment in rats. Ann Anat. (2016) ;206: :7–13. |
[45] | Songngam S , Sukwattanasinitt M , Siralertmukul K , Sawasdee P , A 5,7-dimethoxyflavone/hydroxypropyl-β-cyclodextrin inclusion complex with anti-butyrylcholinesterase activity. AAPS PharmSciTech. (2014) ;15: (5):1189–96. |
[46] | Seo SH , Lee YC , Moon HI , Acetyl-cholinesterase Inhibitory Activity of Methoxyflavones Isolated from Kaempferia parviflora. Nat Prod Commun. (2017) ;12: (1):21–22. |
[47] | Brand RM , Wipf P , Durham A , Epperly MW , Greenberger JS , Falo LD Jr . Targeting Mitochondrial Oxidative Stress to Mitigate UV-Induced Skin Damage. Front Pharmacol. (2018) ;9: :920. |
[48] | Prasanth MI , Venkatesh D , Murali D , Bhaskar JP , Krishnan V , Balamurugan K , Understanding the role of DAF-16 mediated pathway in Caenorhabditis elegans during UV-A mediated photoaging process. Arch Gerontol Geriatr. (2019) ;82: :279–85. |
[49] | Liu RM , Desai LP , Reciprocal regulation of TGF-β and reactive oxygen species: a perverse cycle for fibrosis. Redox Biol. (2015) ;6: :565–77. |
[50] | Ekstrand M , Trajkovska MG , Sundelin JP , Fogelstrand P , Adiels M , Johansson M , Hultén LM , Borén J , Levin M , Imaging of Intracellular and Extracellular ROS Levels in Atherosclerotic Mouse Aortas Ex Vivo: Effects of Lipid Lowering by Diet or Atorvastatin. PLoS One. (2015) ;10: (6):e0130898. |
[51] | Yan LJ , Positive oxidative stress in aging and aging-related disease tolerance. Redox Biol. (2014) ;2: :165–9. |
[52] | Cencioni C , Spallotta F , Martelli F , Valente S , Mai A , Zeiher AM , Gaetano C , Oxidative stress and epigenetic regulation in ageing and age-related diseases. Int J Mol Sci. (2013) ;14: (9):17643–63. |
[53] | Santos JH , Meyer JN , Skorvaga M , Annab LA , Van Houten B , Mitochondrial hTERT exacerbates free-radical-mediated mtDNA damage. Aging Cell. (2004) ;3: (6):399–411. |
[54] | Horigome S , Yoshida I , Ito S , Inohana S , Fushimi K , Nagai T , Yamaguchi A , Fujita K , Satoyama T , Katsuda SI , Suzuki S , Watai M , Hirose N , Mitsue T , Shirakawa H , Komai M , Inhibitory effects of Kaempferia parviflora extract on monocyte adhesion and cellular reactive oxygen species production in human umbilical vein endothelial cells. Eur J Nutr. (2017) ;56: (3):949–64. |
[55] | Lee MH , Han AR , Jang M , Choi HK , Lee SY , Kim KT , Lim TG , Antiskin Inflammatory Activity of Black Ginger (Kaempferia parviflora) through Antioxidative Activity. Oxid Med Cell Longev. (2018) ;2018: :5967150. |
[56] | Hidaka M , Horikawa K , Akase T , Makihara H , Ogami T , Tomozawa H , Tsubata M , Ibuki A , Matsumoto Y , Efficacy of Kaempferia parviflora in a mouse model of obesity-induced dermatopathy. J Nat Med. (2017) ;71: (1):59–67. |
[57] | Park JE , Pyun HB , Woo SW , Jeong JH , Hwang JK , The protective effect of Kaempferia parviflora extract on UVB-induced skin photoaging in hairless mice. Photodermatol Photoimmunol Photomed. (2014) ;30: (5):237–45. |
[58] | Loboda A , Damulewicz M , Pyza E , Jozkowicz A , Dulak J , Role of Nrf2/HO-1 system in development, oxidative stress response and diseases: an evolutionarily conserved mechanism. Cell Mol Life Sci. (2016) ;73: (17):3221–47. |
[59] | Blackwell TK , Steinbaugh MJ , Hourihan JM , Ewald CY , Isik M , SKN-1/Nrf, stress responses, and aging in Caenorhabditis elegans. Free Radic Biol Med. (2015) ;88: (Pt B):290–301. |
[60] | Yoon DS , Cha DS , Choi Y , Lee JW , Lee MH , MPK-1/ERK is required for the full activity of resveratrol in extended lifespan and reproduction. Aging Cell. (2019) ;18: (1):e12867. |
[61] | Song B , Zheng B , Li T , Liu RH , ((2020) ). Raspberry extract ameliorates oxidative stress in Caenorhabditis elegans via the SKN-1/Nrf2 pathway. J Func Foods. (2020) ;70: :103977. |
[62] | Prasanth MI , Gayathri S , Bhaskar JP , Krishnan V , Balamurugan K , Understanding the role of p38 and JNK mediated MAPK pathway in response to UV-A induced photoaging in Caenorhabditis elegans . J Photochem Photobiol B. (2020) ;205: :111844. |
[63] | Phulara SC , Pandey S , Jha A , Chauhan PS , Gupta P , Shukla V , Hemiterpene compound, 3,3-dimethylallyl alcohol promotes longevity and neuroprotection in Caenorhabditis elegans. Geroscience. (2021) ;43: (2):791–807. |
[64] | Zhang M , Lu P , Terada T , Sui M , Furuta H , Iida K , Katayama Y , Lu Y , Okamoto K , Suzuki M , Asakura T , Shimizu K , Hakuno F , Takahashi SI , Shimada N , Yang J , Ishikawa T , Tatsuzaki J , Nagata K , Quercetin 3,5,7,3’,4’-pentamethyl ether from Kaempferia parviflora directly and effectively activates human SIRT1. Commun Biol. (2021) ;4: (1):209. |
[65] | Nakata A , Koike Y , Matsui H , Shimadad T , Aburada M , Yang J , Potent SIRT1 enzyme-stimulating and anti-glycation activities of polymethoxyflavonoids from Kaempferia parviflora . Nat Prod Commun. (2014) ;9: (9):1291–4. |
[66] | Zečić A , Braeckman BP , DAF-16/FoxO in Caenorhabditis elegans and Its Role in Metabolic Remodeling. Cells. (2020) ;9: (1):109. |
[67] | Qi Z , Ji H , Le M , Li H , Wieland A , Bauer S , Liu L , Wink M , Herr I , Sulforaphane promotes C. elegans longevity and healthspan via DAF-16/DAF-2 insulin/IGF-1 signaling. Aging. (2021) ;13: (2):1649–70. |