Effect of goji berry on the formation of extracellular senile plaques of Alzheimer’s disease
Abstract
BACKGROUND:
Alzheimer’s disease (AD) is the most common neurodegenerative disease and a major source of morbidity and mortality. Currently, no therapy nor drug can cure or modify AD progression, but recent studies suggest that nutritional compounds in certain foods can delay or prevent the onset of AD. Diets with high antioxidants is one of the examples which is believed to influence AD pathogenesis through direct effect on amyloid beta levels. Compared to other fruits and vegetables, goji berry (GB) has high levels of polyphenolic substances with antioxidant activities which have shown some positive effects on cognitive function while its mechanism on neuroprotection is yet to be explored. We investigated whether GB would decrease the quantity of amyloid beta in cell culture model of AD.
OBJECTIVE:
To assess the protective effects of GB against amyloid beta toxicity in M17 cells using different techniques.
METHODS:
Goji berry powder (GBP) at different concentrations was treated with 20 μM amyloid beta-induced neuronal cells. MTS assay (3-(4,5-dimethylthiazol-2-yl)-5-(3-carboxymethoxy-phenyl)-2-(4-sulfophenyl)-2H-tetrazolium), bicinchoninic acid (BCA) assay, Western blot analysis, enzyme-linked immunosorbent assay (ELISA) and atomic force microscopy (AFM) were performed to identify how GB affected amyloid beta.
RESULTS:
MTS assay indicated that GBP significantly increased cell viability up to 105% when GBP was at 1.2 μg/ mL. Western blot showed significant reduction of amyloid beta up to 20% in cells treated with 1.5 μg/ mL GBP. GBP at 1.5 μg/ mL was the most effective concentration with 17% reduction of amyloid beta in amyloid beta-induced neuronal cells compared to control (amyloid beta only) based on ELISA results. AFM images further confirmed increasing GBP concentration led to decreased aggregation of amyloid beta.
CONCLUSION:
GB can be a promising anti-aging agent and warrants further investigating due to its effect on reduction of amyloid beta toxicity.
1Introduction
Alzheimer’s disease (AD) is a progressive neurodegenerative disease caused by nerve cell death resulting in loss of short-term and long-term memory, thinking and language skills, and behavioural changes. In 2016, the total cost of dementia in Australia was 14.25 billion AUD and it is estimated to reach 36.8 billion AUD which is more than two-fold increase in 2036. AD is characterised by the formation of extracellular senile plaques (amyloid beta) and intracellular neurofibrillary tangles in brain [1]. The production and deposition of the amyloid beta peptide in the aging brain are the main pathology of AD. The concentration of amyloid beta in an AD patient brain is significantly higher than that of a normal health person [2]. Amyloid beta causes neuronal loss and the clinical manifestation of AD is correlated with the degree of neuronal loss in brain [3]. Thus, it is believed that therapeutic strategies focusing on the modulation of amyloid beta toxicity is important to avoid the development and progression of AD. Therefore, it is essential to identify a drug that effectively reduce the loss of neurons due to amyloid beta toxicity to prevent the decline in cognitive abilities in AD. Currently, only neurotransmitter modulator is available as an approved drug against AD. Research has also indicated that food compounds with high levels of antioxidants is one of the preventative options for AD that has attracted a lot of attention. These compounds can attenuate the progression of the disease by reducing oxidative stress, neuronal death and inflammation due to the presence of antioxidants [4]. The use of GB as an antioxidant has shown promising neuroprotection. However, its therapeutic potential towards the reduction of amyloid toxicity has not been evaluated.
GB or wolfberry is the fruit of Lycium barbarum and Lycium chinense. It is a deciduous fruit of 1– 2 cm in diameter and produced by a perennial shrub. Usually, GB grows up to 1– 3 meters in height and produces ellipsoid, bright orange-red berries. The taste of fresh GB is sweet and sour. GB contains a wide range of nutrients and bioactive compounds including polysaccharides (5– 8% in dried GB), carotenoids (0.3– 0.5% in dried GB), amino acids, betaine, cerebroside, beta-sitosterol, p-coumaric acid, and various vitamins (B1, B2, B6 and E) [5– 7]. These compounds have antioxidation, anti-inflammatory, and anti-neoplastic properties which have been used for the treatment of various blood circulation disorders and diabetes [8]. GB has demonstrated its health benefits on improvement of metabolism, vision, blood circulation, kidney, liver and lungs function [5, 9, 10].
Recent work identified that GB could enhance cognitive function and reduce the risk factors related to AD via prevention of brain oxidative mitochondrial damage due to its polysaccharides including arabinose, rhamnose, mannose and galactose [5, 11]. For example, GB contains betaine which has a strong potential to scavenge free radicals and is important to reduce the oxidative stress in neuronal cells [12]. Oxidative stress causes more production of amyloid beta in the brain [12]. GB also can activate T cells, B cells, macrophages, NK cells and other major immune cells that are important to maintain cellular immune function and humoral immune function [13]. As a result, GB has shown to reduce the risk factors related to AD such as oxidative stress through initiating immune cells [10, 14]. The aim of our study was to identify the effect of GB on the physiological levels of amyloid beta which determines the rate of neuronal death under in vitro conditions.
2Materials and methods
Goji berry powder (GBP) was purchased from Bozhou Baofeng Biotech Co., Ltd. As advised by the manufacturer, GB was convective dried in 3 stages. Firstly, fresh GB was dried under 45°C for 36 h. Then further dried at 50°C for 48 h and 55°C for 24 h. After drying, GB was ground into GBP at 200 mesh.
2.1Degradation or clearance of amyloid beta
2.1.1Preparation of amyloid beta oligomers
Amyloid beta oligomer solution was prepared as per method described by Stine, Jungbauer [15]. The 1 mM amyloid beta solution was prepared by adding 2.217 mL hexafluoroisopropanol (HFIP) directly to the vial containing 10 mg amyloid beta 42 lyophilized powder (W. M. Keck Laboratory, US) through the rubber septum using a 2.5 mL glass Hamilton syringe with a Teflon plunger and sharp (not blunt end) needle. Once the amyloid beta peptides were completely dissolved, septum was pierced with a syringe needle to release the vacuum. The amyloid beta-HFIP solution was incubated at room temperature for 30 mins. Then the glass vial was decapped, and the rubber septum was removed (Note: HFIP should not be in contact with the septum). The amyloid beta-HFIP solution was aliquoted by Eppendorf positive-displacement pipetting 100 μL (containing 0.45 mg amyloid beta 42) into 1.7 mL microcentrifuge tubes using micropipette. The amyloid beta-HFIP solution was allowed to evaporate in the open tubes overnight in a fume hood. To remove any remaining traces of HFIP and moisture, tubes were transferred to a SpeedVac (PD131DDA, Thermo Scientific, US) and dried for 1 h without heating. A thin and clear film of peptides was observed at the bottom of the tubes once it was removed from the SpeedVac. These tubes with dried peptide films were stored over desiccant in a glass jar at – 20°C in a freezer. Prior to use, amyloid beta peptide film was taken from the freezer and allowed to return to room temperature.
The 5 mM amyloid beta dimethyl sulfoxide (DMSO) stock was prepared by adding 20 μL fresh dry DMSO into a tube with 0.451 mg amyloid beta 42 peptide (2 μL to 0.045 mg amyloid beta 42). A complete resuspension of peptide film in which the amyloid beta DMSO stock was clean and cloudless was achieved by pipetting thoroughly and scraping down the sides of the tube. Then vortexed (vortexer: PV-1, BIOLAB, US) the tube vigorously for 30 sec and used a microcentrifuge tube to collect the solution, followed by sonicating for 10 mins in a water-bath sonicator (ultrasonic cleaner: 250HD, SONICLEAN, Japan). This was used as the starting material for oligomeric amyloid beta preparation.
The 100 μM amyloid beta was prepared by pipetting 2 μL of 5 mM amyloid beta DMSO solution and 98 μL cold phenol-free F12 solution to a new tube. To prepare oligomers, solution was vortexed for 15 sec (vortexer: PV-1, BIOLAB, US) and incubated for 24 h at 4°C in a cold room.
2.1.2Culturing of cells
Culturing human neuroblastoma BE(2)-M17 cells (ATCC, Manassas, VA, USA) was carried out as per manufacturer’s guidelines (ATCC, USA). Cell lines were cultured with growth medium (Dulbecco’s modified Eagle medium (DMEM) supplemented with 10% fetal bovine serum (FBS) and 1% Pen-Strep; growth medium was approximately 5– 10 mL for 25 cm2) in culture flasks. Cells were checked daily by a microscope (BX51, Olympus, Japan) and ensured they were heathy and growing as expected during incubation at 37°C and 5% CO2 (incubator: Heracell Vios 160i, Thermo Scientific, US). The cells were attached to the bottom of the flask, as round and plump or elongated in shape. Cells grew until 70% confluence.
2.1.3Neurotoxicity of amyloid beta 42 determination
The neurotoxicity of amyloid beta 42 was first assessed by treating the cells with 100 μL treatment medium (prepared as 5 mL 1% fetal calf serum (FCS) + 45 mL DMEM/F-12 (Dulbecco’s Modified Eagle Medium/Ham’s F-12) supplemented with different concentrations of amyloid beta 42 (20, 30 and 40 μM) per well as per Table 1. Then the 96-well plate was incubated (incubator: Heracell Vios 160i, Thermo Scientific, US) at 37°C and 5% CO2 for 48 h and 72 h respectively. After incubation, 20 μL MTS solution was pipetted into each well and the 96-well plate was immediately wrapped with aluminium foil and placed into the incubator again for 4 h until purple precipitate was visible. The absorbance of each well at 570 nm was recorded by a Multimode Plate Reader (Enspire, US).
Table 1
Amyloid beta concentrations and culture time
Amyloid beta concentration (μM) | Incubation time (h) |
10 | 48 |
10 | 72 |
20 | 48 |
20 | 72 |
30 | 48 |
30 | 72 |
2.1.4Pre-screening of GBP
To pre-screen GBP, wells contained different concentrations (0.3– 1.8 μg/mL) of GBP with 100 μL treatment medium per well were incubated (incubator: Heracell Vios 160i, Thermo Scientific, US) at 37°C and 5% CO2 for 48 h. After incubation, 20 μL MTS solution was pipetted into each well and the 96-well plate was immediately wrapped with aluminium foil and placed into the incubator again for 4 h until purple precipitate was visible. The absorbance of each well at 570 nm was recorded by a Multimode Plate Reader (Enspire, US).
2.1.5MTS assay for cell viability
Cells were treated with 100 μL treatment medium and 20 μM amyloid beta 42 at different concentrations (0.6, 0.9, 1.2, 1.5 and 1.8 μg/mL) of GBP. The 96-well plate was incubated (incubator: Heracell Vios 160i, Thermo Scientific, US) at 37°C and 5% CO2 for 48 h, followed by adding 20 μL MTS solution into each well. Then the 96-well was wrapped with aluminium foil and incubated again for 4 h (at 37°C and 5% CO2). Cell viability was calculated based on the absorbance which was recorded by a Multimode Plate Reader (Enspire, US) at 570 nm using Equation 1.
(1)
Where:
N = number of cells,
At = absorbance of sample solution (sample solution + treatment media + cells + amyloid beta),
Ab = absorbance of the blank (treatment media only),
Ac = absorbance of the control (cells + treatment media) which was considered 100% viability,
1.2×104 = number of cells in control.
2.2Bicinchoninic acid (BCA) assay
The BCA protein assay is a colorimetric detection and used for quantification of total protein. Carefully pipetted 100 μL blank (1×PBS), 100 μL protein assay standard and 100 μL diluted cell supernatants (cell supernatants were diluted in 1×PBS at a ratio of 1:100, v/v) onto each well of 96-well plate. Then 100 μL BCA mix (5 mL reagent A, 4.8 mL reagent B and 0.2 mL reagent C) was added into each well. The 96-well plate was incubated at 69°C for 20 mins (incubator: UL30, MEMMERT, Germany). Absorbance was measured at 550 nm. The reduction of amyloid beta concentration was calculated between protein concentration of control and sample with amyloid beta and without amyloid beta as per Equation 2.
(2)
Where:
CR = reduction of amyloid beta concentration,
CA = protein concentration of amyloid beta only,
CB = protein concentration of blank,
CS = protein concentration of sample with amyloid beta,
CW = protein concentration of sample but no amyloid beta,
CA-CB = amyloid beta concentration of control.
2.3Western blot analysis
Western blot analysis was conducted as per protocols developed by the manufacturer (Bio-Rad, US).
2.3.1Preparation of cell lysate and media
A density of 1.2×105 cells per well was transferred into the 12-well plate. Then 800 μL treatment medium (prepared as 5 mL 1% FCS + 45 mL DMEM/ F12 (1:1)) and 200 μL amyloid beta (100 mM) were pipetted into each well.
Then 0.9, 1.2 and 1.5 μg/mL (these concentrations were selected according to MTS assay results) of GBP solution was pipetted respectively into each well. The 12-well plate was immediately placed into an incubator (Heracell Vios 160i, Thermo Scientific, US) for 24 h (condition: 37°C with 5% CO2). Then the plate was removed from the incubator and placed on ice. Cells were washed using 800 μL ice-cold 1 × Hanks’ Balanced Salt Solution (HBSS) after collecting the treatment medium. Then 33 μL ice-cold lysis buffer (formulation: 10% 10×phosphate buffered saline (PBS), 10% sodium dodecyl sulfate (SDS):Trix, 4% protease inhibitor and 76% purified water) was added into each well for 1-2 min after removal of HBSS. Cell lysate was then collected into a pre-cooled microcentrifuge tube using a cell scraper. Both cell lysate and cell medium were placed on ice for further analysis after a 10 min sonication (ultrasonic cleaner: 250HD, SONICLEAN, Japan).
2.3.2Loading and running the gel
Before starting Western blot analysis, the volume of cell lysate or cell medium pipetted into each tube was calculated as per Equation 3.
(3)
Where:
V = volume of cell supernatants (μL),
C = protein concentration (μL/ mL).
Then carefully pipetted required volume of cell lysate or cell medium, 18.80 μL 4×Sample Buffer, 7.50 μL 10×Reducing Agent into a new tube and topped up to 75 μL with purified water. The tube went through vortex for seconds (vortexer: N12504, VELP, Italy), sonication for 10 mins (ultrasonic cleaner: 250HD, SONICLEAN, Japan), and incubation for 5 mins at 95°C (dry block heater: DBH30D, Ratek, Australia).
Set up the gel tank and power pack including the gel (Bio-Rad TGX stain free 4– 15% gel). Then poured the running buffer (1×2- (N-morpholino) ethanesulfonic acid (MES) SDS Running Buffer) into the gap between the cassette and gel (ensured no running buffer was leaking from the tank). Carefully removed the comb of the gel once the wells were immerged. Immediately ran the gel at 110 V after loading 7 μL molecular-weight marker and 25 μL protein sample solution into each cassette well respectively. Stopped the gel-running once the dye front met gel foot. Then placed the gel carefully onto a tray which was pre-filled with running buffer since the gel was removed from the cassette.
Placed the gel on nitrocellulose membrane according to the manufacturer’s guidelines (Transfer Kit 1704270, Bio-Rad, US) and immediately transferred it to the Transfer System (Trans-Blot Turbo System, Bio-Rad, US) for 7 min run in which the protein was transferred to the membrane during this process. Then removed gel from the membrane and placed it onto a tray with 1×tris-buffered saline (TBS). Cut the membrane by a knife for a better shape in a glass slab.
2.3.3Antibody staining
The membranes were washed 3×5 min using 25 mL 1×TBS (pH adjusted to 7.4) in a lid (approximately 15 cm×15 cm) on the rocking platform, followed by being boiled for 40 seconds in pre-microwaved 25 mL 1×PBS (microwave oven: MS40J5133BT, Samsung, Korea). The membranes were rinsed twice by 1×PBS until it cooled down. The membranes were blocked in 25 mL 1×TBS with 5% skim milk for 1 h and then incubated by 25 mL 1×TBST (1×TBS added with 0.1% Tween 20) with 0.5% skim milk plus 10 μL primary antibody WO2 (Sigma-Aldrich, US) (1:2500 dilution) on a rocking platform overnight in a 4°C cold room. Then the membranes were washed by 25 mL 1×TBST for 3×10 mins and incubated with 5 μL secondary antibody anti-mouse HRP (Thermofisher, US) in TBST with 0.5% skim (1:5000 dilution) milk for 1 h at room temperature. The membranes were further washed in 1×TBST for 3×10 mins followed by being rinsed in 1×TBS for 2– 3 mins. Then the membranes were incubated for 2 mins with ECL reagent (a mixture of detection reagent 1 and 2 at 1:1 from the ECL Western blot Detection Reagents Kid, GE Healthcare UK, 50 mL ECL reagent for 4 membranes) and shake vigorously. Then exposed membranes were scanned using chemiluminescence imaging system (Fusion FX Spectra, Vilber Lourmat). The immune-reactive bands were quantified through Bio-Rad Quantity One 1-D analysis software. Amyloid beta ratio based on density was calculated to see the effect of GBP on the reduction of amyloid beta as per Equation 4.
(4)
Where:
DS = density of sample with amyloid beta,
DA = density of amyloid beta only,
DB = density of blank (no amyloid beta and sample).
2.4Enzyme-linked immunosorbent assay (ELISA)
ELISA was performed at 450 nm within 30 min after adding the Stop Solution as per manufacturer’s guidelines (Thermo Scientific, US). The standard curve was automatically drawn by the curve-fitting software (BMG OPTIMA Windows) based on readings of unknown samples and control concentrations through the microplate reader (BMG FLUOstar Optima, Germany). Dilution factor was considered for calculation of amyloid beta 42 concentration.
2.5AFM
AFM was conducted as per method described by Stine et al. [15]. Carefully pipetted 3 μL of 1 M HCl onto the mica surface and ensure it covered the full surface, followed by an incubation for 30 seconds at room temperature. Used two drops of ultrapure water from syringe filter (0.02 μm) at 45° angle to wash the mica surface. Then sample solution was delivered onto the mica surface in full via a syringe and followed by a 3 min room temperature incubation. Rinsed the mica surface with three drops of water by the syringe filter and followed by blow dry using several gentle pulses of compressed air. The sample solution was analysed for AFM once it was completely dried after room temperature incubation.
2.6Sample size
This study was carried out with and without amyloid beta for each concentration of GBP. Based on our previous in vitro work using short chain fatty acids, 6 cell culture plates (24 well) per group were used for statistical significance to be achieved. Total sample size was determined to be 32 for this investigation assuming a failure rate of 5%.
2.7Statistical analysis
All analyses were carried out in triplicate with data were presented as mean/standard deviation (SD). Statistical tests were conducted using the STATA statistical analysis software program version 12 (Stata Corp LLC, US). One-way analysis of variance (ANOVA) was used to determine the effect of GB on amyloid beta through different cell culture techniques. The p value of less than 0.05 (p < 0.05) was considered for significant difference.
3Results
3.1Effect of amyloid beta on cell viability
MTS assay results showed that compared with untreated cells, cell viability was significantly inhibited in cells treated with amyloid beta 42 alone, while 50 % or more of cell viability was significantly decreased after treatment with 20 μM (p < 0.01) or 30 μM but not for 10 μM (Fig. 1). Although decrease of cells were observed at 48 h and 72 h, 50% reduction was observed at 72 h when amyloid bate concentration was 20 μM. Therefore, based on MTS assay, the treatment with 20 μM amyloid beta 42 for 72 h was used in subsequent experiment [16].
Fig. 1
Cell number at different amyloid beta concentrations. Letters such as a, b, c and d are the superscripts of cell numbers based on mean±SD. Different letters indicate the cell numbers of each column are significantly different (p < 0.05).
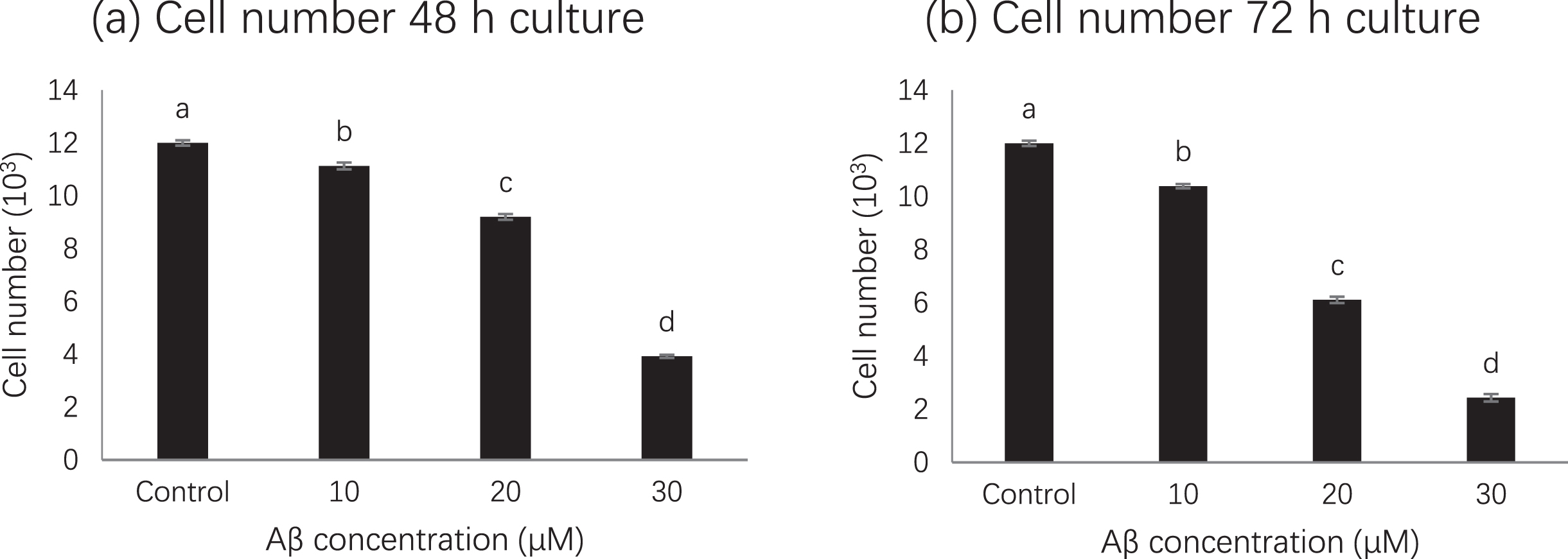
3.2Effect of GBP on cell viability
GBP inhibited amyloid beta induced cell death in a dose-dependent manner as per Fig. 2. Cell viability was significantly (p < 0.01) increased with increased GBP concentration from 0.6 to 1.2 μg/mL, whilst there was a significant decrease of cell viability in the range of 1.2– 1.8 μg/mL of GBP. The highest cell viability (105%) appeared when GBP was 1.2 μg/mL. As shown in Fig. 3, cells appeared more elongated when treated with GB.
Fig. 2
Effect of GBP concentrations on cell viability. Letters such as a, b, c and d are the superscripts of cell numbers based on Mean±SD. Different letters indicate the cell numbers of each column are significantly different (p < 0.05).
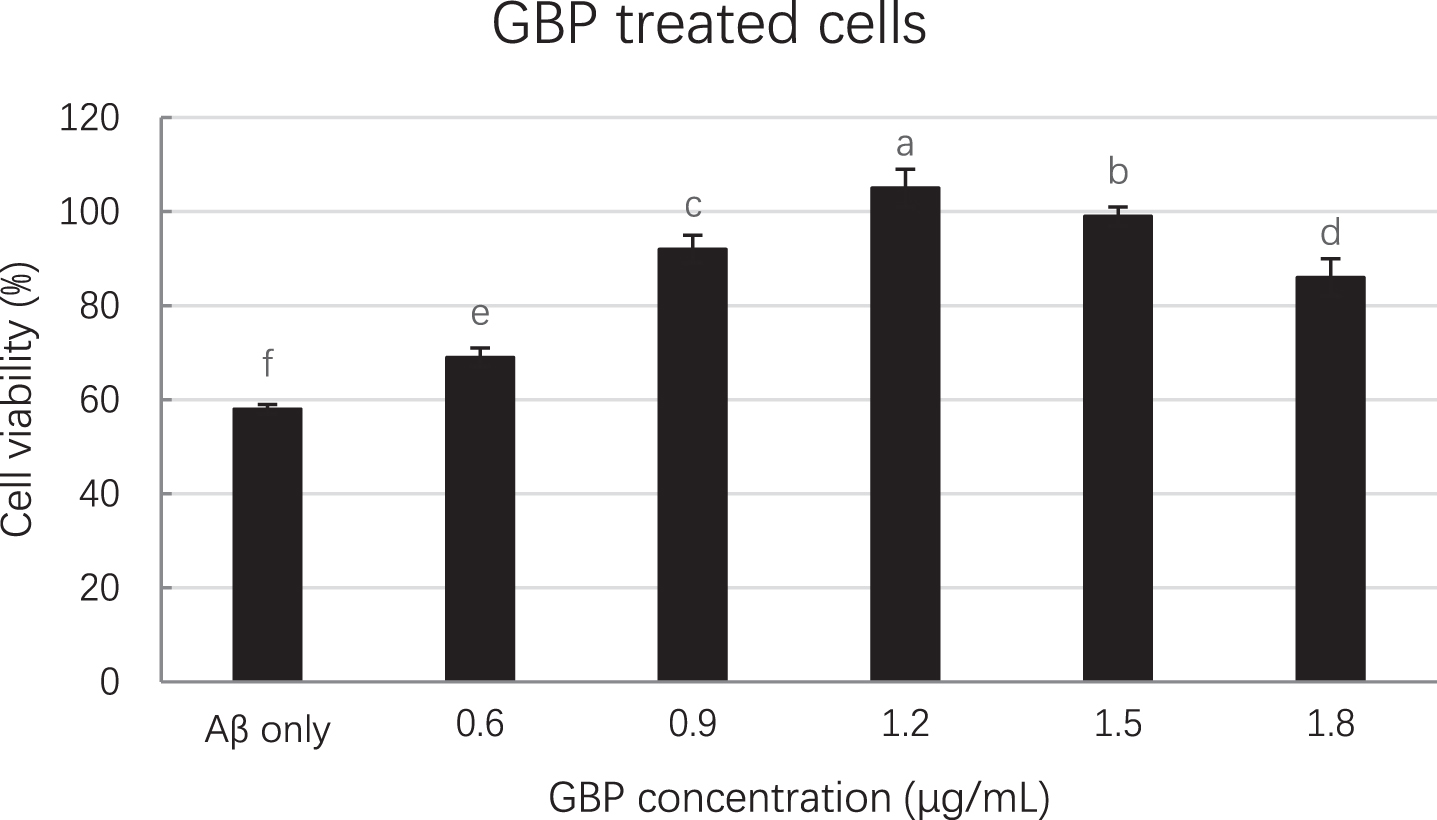
Fig. 3
Cell morphology of GBP treated with amyloid beta induced cells at 72 h culture. (a) Amyloid beta only (20 μM); (b) control (only cells without amyloid beta and GBP); (c) cells treated with GBP at 1.2 μg/mL.
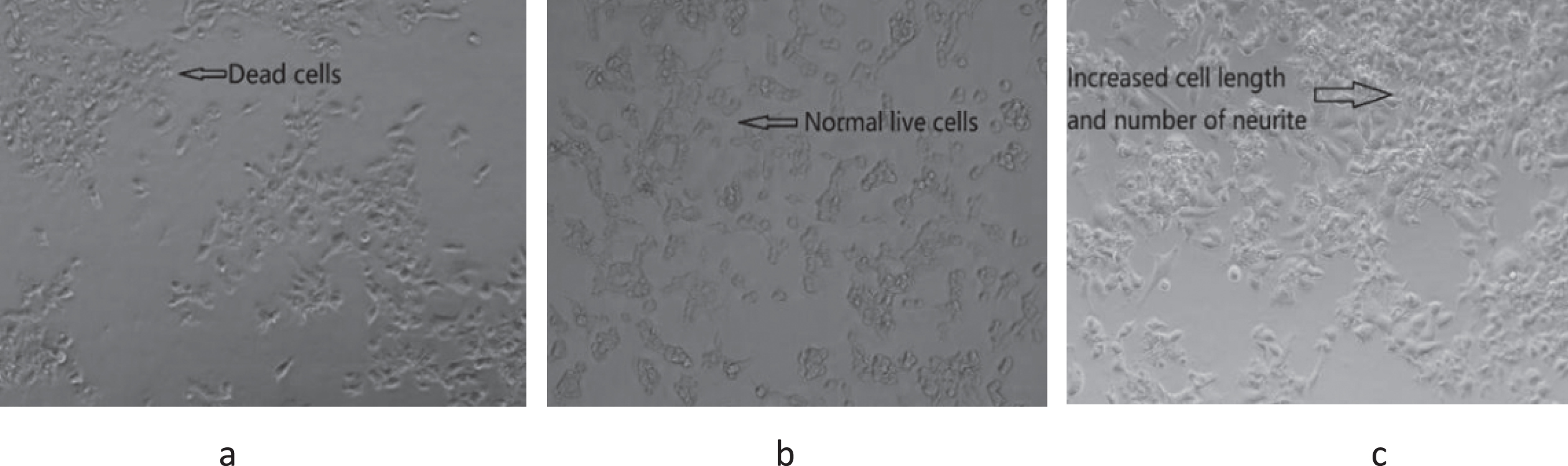
3.3Bicinchoninic acid (BCA) assay
The resulted protein concentration of sample based on cell lysate was dependent on the GB concentration. For example, protein concentrations of cells treated with three different GB concentrations were 24.67, 24.22 and 24.10 μg/mL respectively (Table 3). Results implies that higher concentration of GBP could decrease the concentration of amyloid beta in cells (Table 3). Proteins comes from cell itself and added amyloid beta. In the light of results, GB had reduced the concentration of protein in cell lysate by 22.5%. The highest protein concentration reduction (0.98 μg/mL) was reported at the concentration of 1.5 μg/mL of GBP (Table 4).
Table 2
Protein concentration of sample without amyloid beta
Sample treatment | Sample concentration (μg/ mL) | Cell lysate protein concentration (μg/ mL) | Cell medium protein concentration (μg/ mL) | |
1 | Amyloid beta (20 μM) only | N.A. | 18.60±0.03c | 6.67±0.06b |
2 | GBP | 0.9 | 17.52±0.06a | 6.26±0.05a |
3 | GBP | 1.2 | 17.16±0.17b | 6.52±0.05b |
4 | GBP | 1.5 | 17.12±0.08b | 6.42±0.02ab |
Letters such as a, b and c are the superscripts of protein concentrations based on mean±SD. Different letters indicate the protein concentrations of each column are significantly different (p < 0.05).
Table 3
Protein concentration of sample with amyloid beta
Sample treatment | Sample concentration (μg/ mL) | Cell lysate protein concentration (μg/ mL) | Cell medium protein concentration (μg/ mL) | |
1 | Amyloid beta (20 μM) only | N.A. | 18.60±0.03c | 6.67±0.06b |
2 | GBP | 0.9 | 24.67±0.11a | 9.56±0.09a |
3 | GBP | 1.2 | 24.22±0.17b | 9.58±0.02a |
4 | GBP | 1.5 | 24.10±0.07b | 9.44±0.00a |
Letters such as a, b and c are the superscripts of protein concentrations based on mean±SD. Different letters indicate the protein concentrations of each column are significantly different (p < 0.05).
Table 4
Reduced amyloid beta concentration
Sample treatment | Sample concentration (μg/mL) | Reduced amyloid beta concentration in cell lysate (μg/mL) | Reduced amyloid beta concentration in cell medium (μg/mL) | |
1 | GBP | 0.9 | 0.80±0.06b | 2.89±0.14b |
2 | GBP | 1.2 | 0.89±0.00ba | 3.14±0.03a |
3 | GBP | 1.5 | 0.98±0.01a | 3.18±0.02a |
Letters such as a, b and c are the superscripts of reduced amyloid beta concentrations based on mean±SD. Different letters indicate reduced amyloid beta concentrations of each column are significantly different (p < 0.05).
3.4Western blot analysis
The density of amyloid beta from Western blot is shown in Table 5. Cells treated with GBP at 1.5 μg/mL showed the lowest amyloid beta density (3.17×104) in cell lysate while GBP was at 1.5 μg/mL in cell medium indicated the highest amyloid beta inhibition effect (amyloid beta density was 1.38×104). Cell lysate and cell medium at 1.5 μg/mL GBP showed the lowest amyloid beta ratios (relative densities) indicating it was the most effective concentration of GB against amyloid beta (Fig. 4). Their blots in both cell lysate and cell medium are shown in Fig. 5.
Table 5
Results of western blot (density)
Sample treatment | Sample concentration (μg/ mL) | Amyloid beta density (104) in cell lysate | Amyloid beta density (104) in cell medium |
Amyloid beta (100 mM) only | N.A. | 4.08±0.01a | 3.19±0.01a |
Blank | N.A. | 0.10±0.00d | 0.10±0.00d |
GBP | 0.9 | 3.40±0.04b | 1.70±0.02b |
GBP | 1.2 | 3.40±0.04b | 1.67±0.05b |
GBP | 1.5 | 3.17±0.04c | 1.38±0.03c |
Letters such as a, b, c and d are the superscripts of amyloid beta densities based on mean±SD. Different letters indicate the amyloid beta density of each column are significantly different (p < 0.05).
Fig. 4
Amyloid beta ratios at different GBP concentrations from Western blot. Letters such as a and b are the superscripts of amyloid beta concentration ratios based on mean±SD. Different letters indicate amyloid beta concentrations of the same-coloured columns are significantly different (p < 0.05).
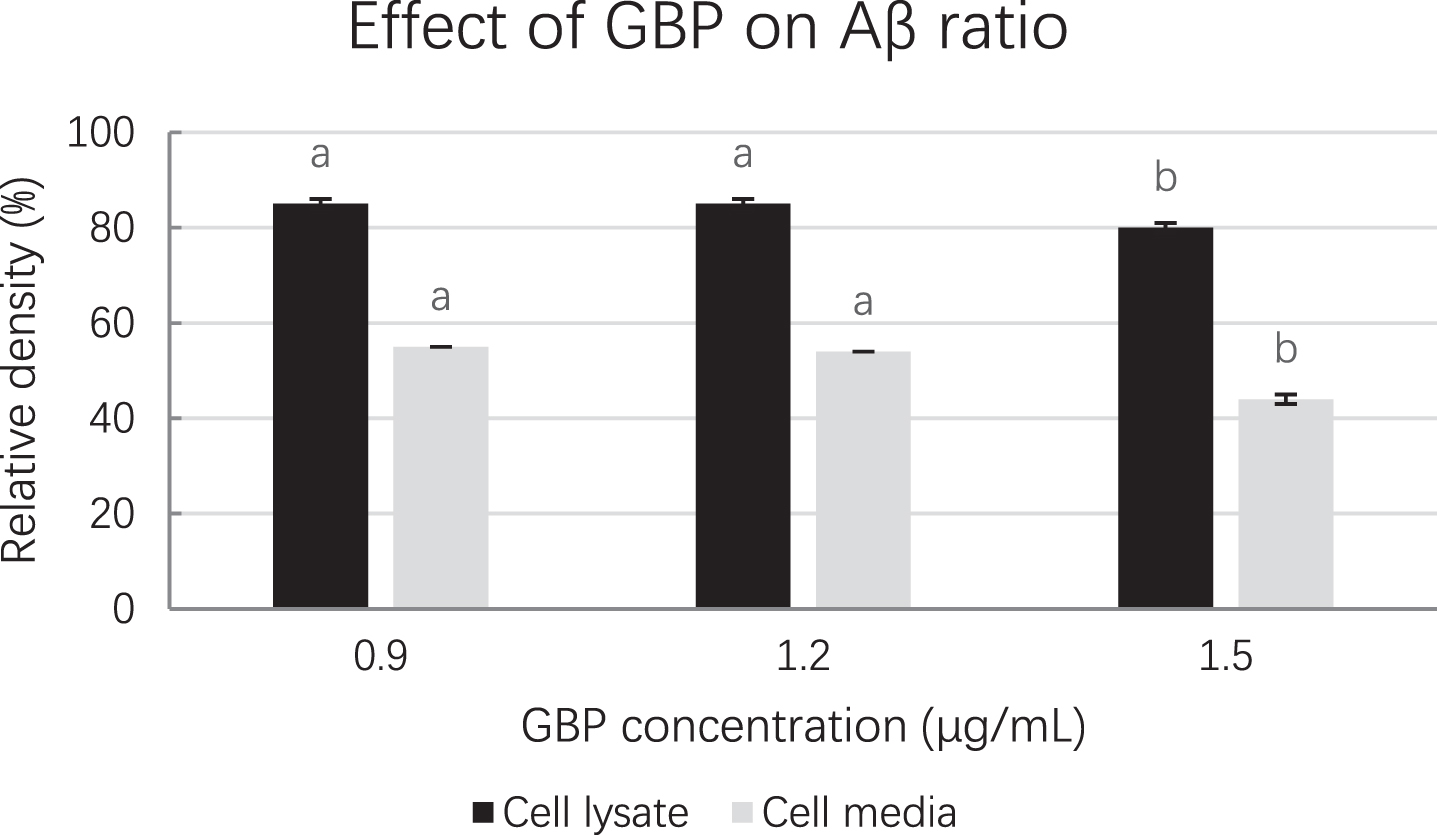
Fig. 5
Effect of GBP on amyloid beta blot. (1) Amyloid beta only; (2) GBP at 0.9 μg/mL; (3) GBP at 1.2 μg/mL; (4) GBP at 1.5 μg/mL.
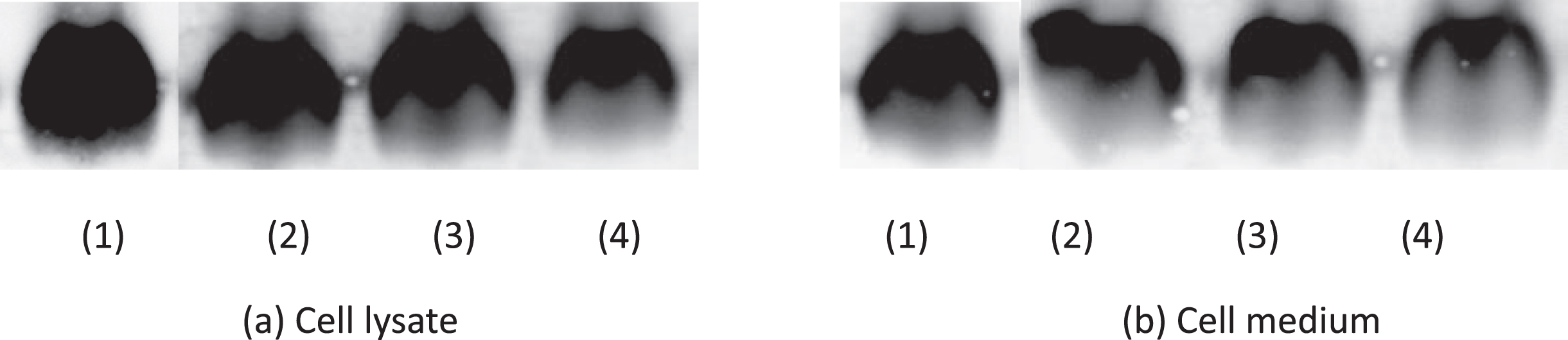
3.5ELISA
Each sample was diluted at 1/3, 1/10, 1/100, 1/1000 and 1/10000 to ensure the unknown sample’s absorbance fell into the range of the standard curve. The cell lysates at 0.9, 1.2 and 1.5 μg/mL GBP concentrations were selected for ELISA which was based on MTS assay and Western blot analysis results. When the concentration of GB was increased, the concentration of amyloid beta was decreased significantly (p < 0.01). The amyloid beta concentration at 0.9, 1.2 and 1.5 μg/mL of GBP was 9199, 8821 and 8499 pg/mL respectively while that in control (amyloid beta only) was 10189 pg/mL (Fig. 6). Therefore, GBP at 1.5 μg/mL was the concentration with the highest effect on amyloid beta reduction (17%) in amyloid beta-induced neuronal cells compared to the control (Fig. 6).
Fig. 6
The effect of GBP concentration on amyloid beta from ELISA. Letters such as a, b and c are the superscripts of amyloid beta concentrations based on mean±SD. Different letters indicate amyloid beta concentrations of the columns are significantly different (p < 0.05).
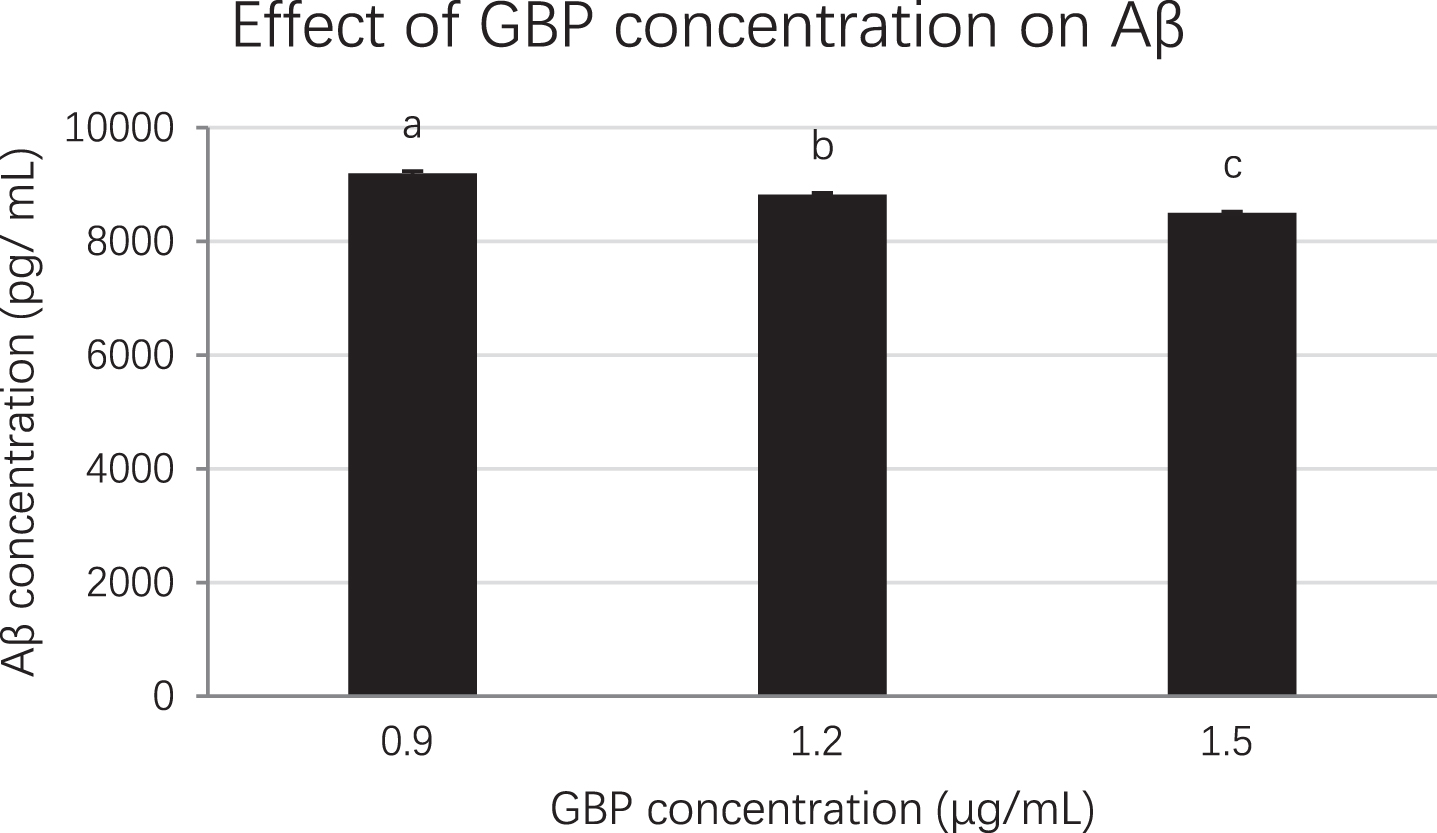
3.6AFM
AFM was applied to estimate the approximate molecular size distributions of amyloid beta (1– 42) oligomers after the treatment. The effect of GBP concentration on amyloid beta is shown in Fig. 7. The effect was dose dependent and lower aggregation or lower number of molecules were observed with increased GBP concentration. Size of the oligomers were reduced when treated with higher concentrations. For example, number and size of oligomers were reduced while GBP concentration was increased. There were some oligomeric amyloid beta that could be observed when GBP was at 0.9 μg/mL (Fig. 7 (a)). When GBP concentration was increased, amyloid beta was further inhibited as only a few small (in height) amyloid beta oligomers were identified in Fig. 7 (b) and (c).
Fig. 7
Effect of GBP at different concentrations on amyloid beta. Representative AFM images of adsorbed 20 μM amyloid beta (1– 42) oligomers prepared by HFIP protocol (Mica surfaces (3×3 μm) were visualized after 24 h incubation with preparations (images in 3×3 μm, 24 h incubation).
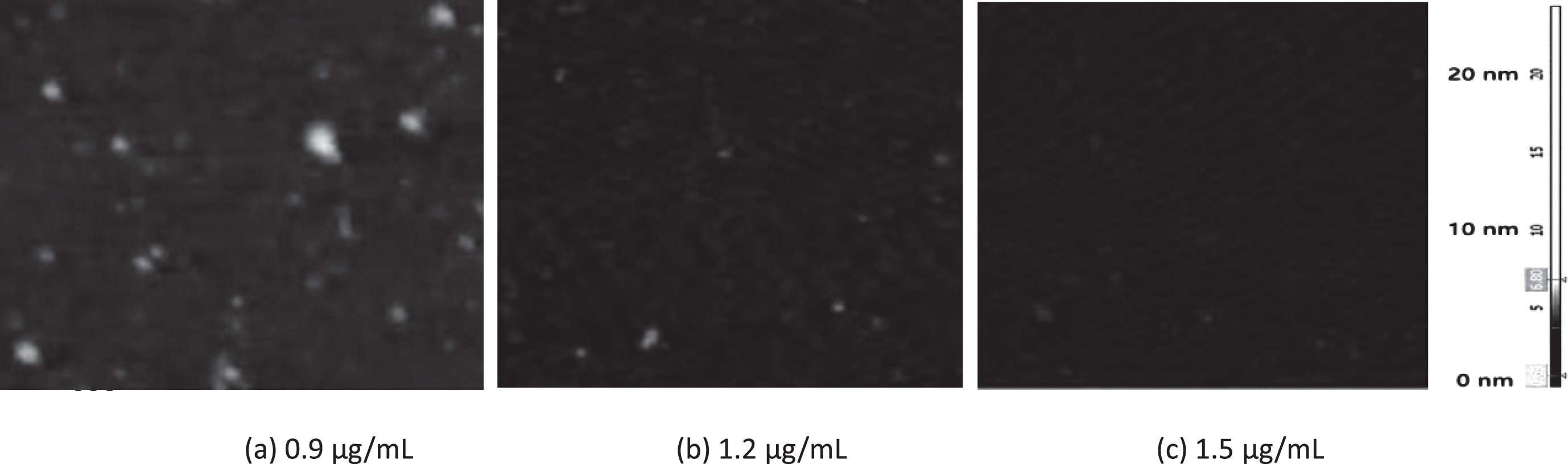
The average particle diameters for the oligomeric assemblies were 35 and 30 nm for amyloid beta only (20 μM) and that with GBP at 0.9 μg/mL respectively (Table 6). The GBP at 1.5 μg/mL showed the smallest diameter (25 nm) with the lowest particle number [45] which indicated the strongest inhibitive effect against amyloid beta.
Table 6
Comparison of particle number and diameter of amyloid beta under AFM
Sample | Concentration (μg/mL) | Average particle size (diameter, nm) | Number of particles (in 3×3μm) |
Amyloid beta only (20 μM) | N.A. | 35 | 69 |
GBP | 0.9 | 30 | 57 |
GBP | 1.2 | 27 | 50 |
GBP | 1.5 | 25 | 45 |
Averages were calculated using three different images from three trials.
4Discussion
Amyloid beta peptide is produced through the proteolytic cleavage from an integral transmembrane domain of the amyloid protein precursor (APP) by β- and γ-secretases. After cleavage, amyloid beta peptide becomes either toxic soluble/diffusible oligomers (amyloid-beta) or relatively inert fibrillary amyloid polymers within the extracellular interstitial compartments as plaques and perivascular deposits [17, 18]. In AD brain, it contains an average 6.5±5.0 mg amyloid beta compared to a 1.7±2.3 mg in healthy brain [2]. The estimated time frame of amyloid beta accumulation from threshold to onset of dementia is 19.2 years, and the rate of amyloid beta accumulation in brain is 28±27 ng/h [19].
Several studies have revealed that amyloid beta toxic properties in neurons are mediated by different mechanisms, like oxidative stress, mitochondrial diffusion, alterations in membrane permeability, inflammation and synaptic dysfunction [20– 22]. On the other hand, the deposition of amyloid beta is considered as a major driving force in the initial stages of AD. Therefore, reduction of amyloid beta toxicity in cells is important when identifying a therapeutic strategy for AD.
GB is a novel ingredient which has attracted a great deal of attention due to its high biological activities and health benefits. GB is rich in antioxidants such as phenolic acids and flavonoids. In addition, the polysaccharides in GB have various important biological activities, such as antioxidation, immunomodulation, and neuroprotection [11, 14, 23]. In the neurological area, the extract or fruit or powder of GB has been shown to have effects on the brain and neuronal function through mechanisms independent of its anti-oxidative effects. Although the underlining mechanism of neuroprotection of GB is not yet conclusive, the studies have shown that GB can exhibit protective effects against neurotoxicity by upregulating Nrf2/HO-1 signalling, reverse the H2O2-induced reactive oxygen species (ROS) levels, increase in caspase-3 and -9 activity and decrease in mitochondrial membrane potential [18]. GB also has demonstrated the signs of decreasing the infarct size, hemispheric swelling and water content in male mice after consumption of GB polysaccharides for 7 days [24]. Amyloid beta peptides in brain activate c-Jun N-terminal kinase (JNK) by phosphorylation [25]. However, treatment with GB had shown a marked reduction of phosphorylation of JNK-1 [Thr183/Tyr185] and its substrates c-Jun-I [Ser 73] and c-Jun-II [Ser 63] [26]. Recently, double-blind randomized control study with young healthy participants has shown the evidences of improved neurological performance after consumption of GB for 14 days [27]. These studies support the anti-aging properties as well as the neurological effects found in the present study.
The presence of low physiological concentrations of amyloid beta in brain (picomoles) plays important roles in the facilitation of synaptic plasticity, memory and learning, whereas the accumulation of high concentrations of amyloid beta (nanomoles or micromoles) results in loss of synaptic function as shown in the AD [28]. As shown in our previous work, reduction of amyloid beta even in picomoles may contribute to higher scores in memory improvement in AD patients [29]. The present study clearly demonstrated that GB significantly reduced the cell death by enhancing the cell viability of amyloid beta treated cells (20 micromoles) in the concentration of 0.6 to 1.2 μg/mL. The mechanism by which GB exerts the neuroprotection may be its strong antioxidative and anti-aging properties. GB could protect DNA, lipids and proteins from free radicals relating to damage and alleviate oxidative stress [14]. For example, GB polysaccharides could increase H2O2-induced cell viability and reduce CoCl2-treated brain tissue apoptosis [30]. The consumption of GB at recommended quantity (5– 12 g) could also reduce DNA damage and maintain cell functions appropriately [31]. However, our study identified that concentration of 1.2– 1.8 μg/mL GBP led to decrease in cell viability (Fig. 2). Higher concentration of GBP provides high sugar content which could cause apoptosis of the cells and the formation of reactive oxygen species (ROS) [32]. Alternatively, the reduction in cell death by GB at the tested concentrations may be explained as a consequence of the reduction in amyloid beta concentrations which occurred at the same concentrations (Table 4, Fig. 4 and Fig. 5)
Our work further proved the capacity of GB to reduce the quantity of amyloid beta in neuronal cells. Simultaneously, more elongated cells that were treated with GB indicated GB neuroprotective effect against amyloid beta [33]. This was further evident by the results of the Western blot, AFM and ELISA. The Western blot analysis has confirmed the presence of lower density of amyloid beta in treated cells than controlled ones. Amyloid beta density was reduced by 20% at the concertation of 1.5 μg/mL GBP. Western blot can separate smaller oligomeric forms of amyloid beta as well as monomeric amyloid beta but not the fibrillar aggregates of amyloid beta due to its size and the mass [34– 36]. The results of ELISA which quantify the total level of amyloid beta including fibrils further demonstrated that GB could reduce amyloid beta concentration in neuronal cells which was consistent to the outcomes of MTS assay and Western blot [37, 38]. GB potent cytoprotective effect may have inhibited amyloid beta which helped to decrease amyloid beta concentration [33, 39].
Aggregation of amyloid beta is toxic to neurons and may trigger apoptosis in AD [40]. The exposure of neurons to amyloid beta deposits increased in Par-4, Bax, and p53, and the release of cytochrome c result in caspase-3 activation then apoptosis [41]. Therefore, it is also plausible that GB may also reduce amyloid beta-induced cell death by inhibiting the aggregation of amyloid beta. The results of AFM showed the smaller particles of amyloid beta after the treatment indicating the inhibition of aggregation of oligomeric amyloid beta. The similar results were obtained with curcumin [42]. This may be attributed to reduction of ROS formed by amyloid beta since GB is a strong antioxidant.
In conclusion, MTS, BCA assay, Western blot analysis and ELISA have indicated that GBP at the concertation of 1.5 μg/mL could significantly impact on the AD amyloidogenesis process through reduction of amyloid beta. The effect of GBP against amyloid beta aggregation is further supported by AFM from imaging perspective. This indicates that GB could significantly affect the amyloidogenesis process of AD reducing its risk. GBP at 1.5 μg/ mL is the most effective concentration on amyloid beta reduction in amyloid beta-induced neuronal cells. However, permeability of GB to blood-brain barrier remained to be elusive to understand its effect in humans.
Acknowledgments
The authors thank Western Sydney University and CSIRO for their financial support. The present study was mainly conducted at the Australian Alzheimer’s Research Foundation, Ralph and Patricia Sarich Neuroscience Research Institute. The authors thank their support.
Funding
This work was supported by Western Sydney University PhD Scholarship and CSIRO Top-up Scholarship.
Conflict of interest
The authors have no conflict of interest to report.
References
[1] | Morris J , Galvin JE , Holtzman DM . Handbook of Dementing Illnesses. Boca Raton: CRC Press (2006) . |
[2] | Roberts BR , Lind M , Wagen AZ , Rembach A , Frugier T , Li Q , et al. Biochemically-defined pools of amyloid-β in sporadic Alzheimer’s disease: correlation with amyloid PET. Brain. (2017) ;140: (5):1486–98. |
[3] | Carter J , Lippa CF . Beta-amyloid, neuronal death and Alzheimer’s disease. Current Molecular Medicine. . (2002) ;1: (6):733–7. |
[4] | Luchsinger JA , Noble JM , Scarmeas N . Diet and Alzheimer’s disease. Current Neurology and Neuroscience Reports. (2007) ;7: (5):366–72. |
[5] | Amagase H , Farnsworth NR . A review of botanical characteristics, phytochemistry, clinical relevance in efficacy and safety of Lycium barbarum fruit (Goji). Food Research International. (2011) ;44: (7):1702–17. |
[6] | Zhang J . Antioxidant Properties of Goji Berries. Detroit: Wayne State University; 2013. |
[7] | Skenderidis P , Lampakis D , Giavasis I , Leontopoulos S , Petrotos K , Hadjichristodoulou C , et al. Chemical Properties, Fatty-Acid Composition, and Antioxidant Activity of Goji Berry (Lycium barbarum L.and Lycium chinense Mill.) Fruits. Antioxidants. (2019) ;8: (60):1–13. |
[8] | Soneji JR , Nageswara-Rao M . Breeding and Health Benefits of Fruit and Nut Crops. Chen J, C.T.C, X.W, editors. London: IntechOpen Limited; 2018. |
[9] | Peng Y , Ma C , Li Y , Leung K , Jiang Z , Zhao Z . Quantification of zeaxanthin dipalmitate and total carotenoids in Lycium fruits (Fructus lycii). Plant Foods For Human Nutrition.161-. (2005) ;60: (4):4. |
[10] | Shan X , Zhou J , Ma T , Chai Q . Lycium barbarum Polysaccharides Reduce Exercise-Induced Oxidative. International Journal of Molecular Sciences.. (2011) ;12: (2):1081–8. |
[11] | Shi Z , Zhu L , Li T , Tang X , Xiang Y , Han X , et al. Neuroprotective Mechanisms of Lycium barbarum Polysaccharides Against Ischemic Insults by Regulating NR2B and NR2A Containing NMDA Receptor Signaling Pathways. Frontiers in Cellular Neuroscience. (2017) ;11: :288. |
[12] | Miwa M , Tsuboi M , Noguchi Y , Enokishima A , Nabeshima T , Hiramatsu M . Effects of betaine on lipopolysaccharide-induced memory impairment in mice and the involvement of GABA transporter 2. Journal Of Neuroinflammation. . (2011) ;8: :153. |
[13] | Shah T , Bule M , Niaz K . Goji Berry Lycium barbarum–(superfood). Nonvitamin and Nonmineral Nutritional Supplements 1st Edition. Amsterdam: Academic Press Elsevier Publisher; (2018) . |
[14] | Ma ZF , Zhang H , Teh SS , Wang CW , Zhang Y , Hayford F , et al. Goji Berries as a Potential Natural Antioxidant Medicine: An Insight into Their Molecular Mechanisms of Action. Oxidative Medicine and Cellular Longevity. . (2019) ;2019: :2437397. |
[15] | Stine WB , Jungbauer L , Yu C , Ladu MJ . Preparing synthetic Aβ in different aggregation states. Methods in Molecular Biology. (2011) ;670: :13–32. |
[16] | Liu Y , Jia M , Xie Z , Liu X , Yang H , Zheng X , et al. Arrestins Contribute to Amyloid Beta-induced Cell Death via Modulation of Autophagy and The α7nAch Receptor in SH-SY5Y Cells. Scientific Reports. (2017) ;7: :3446. |
[17] | Lambert MP , Barlow AK , Chromy BA , Edwards C , Freed R , Liosatos M , et al. Diffusible, nonfibrillar ligands derived from Aβ1-42 are potent central nervous system neurotoxins. Proceedings of The National Academy of Sciences of The United States of America. (1998) ;95: (11):6448–53. |
[18] | McLean CA , Cherny RA , Fraser FW , Fuller SJ , Smith MJ , Beyreuther K , et al. Soluble pool of Abeta amyloid as a determinant of severity of neurodegeneration in Alzheimer’s disease. Annals of Neurology. (1999) ;46: (6):860–6. |
[19] | Villemagne VL , Burnham S , Bourgeat P , Brown B , Ellis KA , Salvado O , et al. Amyloid β deposition, neurodegeneration, and cognitive decline in sporadic Alzheimer’s disease: a prospective cohort study. The Lancet Neurology.. (2013) ;12: (4):357–67. |
[20] | Forner S , Baglietto-Vargas D , Martini AC , Trujillo-Estrada L , LaFerla FM . Synaptic Impairment in Alzheimer’s Disease: A Dysregulated Symphony. Trends in Neurosciences. (2016) ;40: (6). |
[21] | Moreira PI , Carvalho C , Zhu X , Smith MA , Perry G . Mitochondrial Dysfunction Is A Trigger of Alzheimer’s Disease Pathophysiology. Biochimica et Biophysica Acta. (2010) ;1802: (1):2–10. |
[22] | Onyango IG , Dennis J , Shaharyah MK . Mitochondrial Dysfunction in Alzheimer’s Disease and the Rationale for Bioenergetics Based Therapies. Aging and Disease.. (2016) ;7: (2):201–14. |
[23] | He N , Yang X , Jiao Y , Tian L , Zhao Y . Characterisation of Antioxidant and Antiproliferative Acidic Polysaccharides from Chinese Wolfberry Fruits. Food Chemistry. . (2012) ;133: (3):978–89. |
[24] | Yang D , Li S , Yeung C , Chang RC , So K , Wong D , et al. Lycium barbarum Extracts Protect the Brain from Blood-Brain Barrier Disruption and Cerebral Edema in Experimental Stroke. PLoS One. (2012) ;7: (3):e33596. |
[25] | Fogarty MP , Downer EJ , Campbell V . A role for c-Jun N-terminal kinase 1 (JNK1), but not JNK2, in the beta-amyloid-mediated stabilization of protein p53 and induction of the apoptotic cascade in cultured cortical neurons. Biochemical Journal. (2003) ;371: (Pt 3):789–98. |
[26] | Li L , Feng Z , Porter AG . JNK-dependent Phosphorylation of c-Jun on Serine 63 Mediates Nitric Oxide-induced Apoptosis of Neuroblastoma Cells. The American Society for Biochemistry and Molecular Biology. (2004) ;279: (6):4058–65. |
[27] | Amagase H , Nance DM . A Randomized, Double-Blind, Placebo-Controlled, Clinical Study of the General Effects of a Standardized Lycium barbarum (Goji) Juice, GoChi ™. The Journal of Alternative and Complementary Medicine. (2008) ;14: (4):403–12. |
[28] | Cárdenas-Aguayo MdC , Silva-Lucero MdC , Cortes-Ortiz M , Jiménez-Ramos B , Gómez-Virgilio L , Ramírez-Rodríguez G , et al. Physiological Role of Amyloid Beta in Neural Cells: The Cellular Trophic Activity. 2014. In: Neurochemistry [Internet]. IntechOpen. Available from: https://www.intechopen.com/books/neurochemistry/physiological-role-of-amyloid-beta-in-neural-cells-the-cellular-trophic-activity. |
[29] | Fernando WMADB , Martins IJ , Morici M , Bharadwaj P , Rainey-Smith SR , Lim WLF , et al. Sodium Butyrate Reduces Brain Amyloid-β Levels and Improves Cognitive Memory Performance in an Alzheimer’s Disease Transgenic Mouse Model at an Early Disease Stage. Journal of Alzheimer’s Disease. (2020) ;74: (1):91–9. |
[30] | Cheng J , Zhou Z , Sheng H , He L , Fan X , He Z , et al. An Evidence-Based Update on The Pharmacological Activities and Possible Molecular Targets of Lycium Barbarum Polysaccharides. Drug Design, Development and Therapy. (2015) ;9: :33–78. |
[31] | Ceccarini MR , Vannini S , Cataldi S , Moretti M , Villarini M , Fioretti B , et al. In Vitro Protective Effects of Lycium barbarum Berries Cultivated in Umbria (Italy) on Human Hepatocellular Carcinoma Cells. BioMed Research International. (2016) ;2016: :9. |
[32] | Li Q , Lin Y , Wang S , Zhang L , Guo L . GLP-1 Inhibits High-Glucose-Induced Oxidative Injury of Vascular Endothelial Cells. Scientific Reports. (2017) ;7: (8008):1–9. |
[33] | Yu M , Leung S , Lai S , Che C , Zee S , So K , et al. Neuroprotective Effects of Anti-Aging Oriental Medicine Lycium Barbarum against B-Amyloid Peptide Neurotoxicity. Experimental Gerontology. (2005) ;40: (8-9):716–27. |
[34] | Nagarajan S , Kirubagaran R , Rajadas J . Surfactant-induced Conformational Transition of Amyloid β-peptide. Biophysics of Structure and Mechanism. (2008) ;38: (4):355–67. |
[35] | Pryor NE , Moss MA , Hestekin CN . Unraveling the Early Events of Amyloid-β Protein (Aβ) Aggregation: Techniques for the Determination of Aβ Aggregate Size. International Journal of Molecular Sciences. (2012) ;13: :3038–72. |
[36] | Ghosh R , Gilda JE , Gomes AE . The Necessity of and Strategies For Improving Confidence in The Accuracy of Western Blots. Expert Review Proteomics. (2014) ;11: (5):549–60. |
[37] | Cao S , Du J , Hei Q . Lycium Barbarum Polysaccharide Protects Against Neurotoxicity via The Nrf2-HO-1 Pathway. Experimental and Therapeutic Medicine.. (2017) ;14: :4919–27. |
[38] | Gao J , Chen C , Liu Y , Li Y , Long Z , Wang H , et al. Lycium barbarum polysaccharide improves traumatic cognition via reversing imbalance of apoptosis/regeneration in hippocampal neurons after stress. Life Sciences. (2015) ;121: (15):124–34. |
[39] | Wawruszak A , Czerwonka A , Okła K , Rzeski W . Anticancer Effect of Ethanol Lycium Barbarum (Goji Berry) Extract on Human Breast Cancer T47D Cell Line. Natural Product Research. (2016) ;30: (17):1993–6. |
[40] | Kiko T , Nakagawa K , Satoh A , Tsuduki T , Furukawa K , Arai H , et al. Amyloid β Levels in Human Red Blood Cells. PLoS One. (2012) ;7: (11):49620. |
[41] | Mattson MP . Pathways towards and away from Alzheimer’s disease. Nature. (2004) ;430: (7000):631–9. |
[42] | Jiang J , Zhi XL , Zhang XH , Pan LF , Zhou P . Inhibitory Effect of Curcumin on The Al(Iii)-Induced Aβ 42 Aggregation and Neurotoxicity in Vitro. Biochimica et Biophysica Acta - Molecular Basis of Disease. (2012) ;1822: (8):1207–15. |