Intermittent fasting - a potential approach to modulate the gut microbiota in humans? A systematic review
Abstract
Research on gut microbiota has increased in popularity over the past decade, with evidence associating different dietary habits with changes in the makeup of the rich ecosystem of microorganisms that performs a variety of functions and induces a range of health effects, within and well beyond the gastrointestinal tract. Similarly, intermittent fasting (IF), an umbrella term describing various regimens of periods of voluntary abstinence from food and drink, has classically been associated with favourable impacts on cardiovascular risk factors, body weight, circadian biology, and, more recently, the gut health. The objective of this PRISMA systematic review was to summarize the peer-reviewed literature of clinical trials related to the impact of IF regimens on the gut microbiota. A MEDLINE search was conducted using PubMed and the keywords “intermittent fasting”, “gut microbiota”, “microbes”, and others. Whilst the field is still in its infancy, an emerging body of evidence suggests beneficial effects of IF on the health of the gut through increasing the microbial diversity and abundance, with possible clinical implications related to improving the immune function and ameliorating the metabolic status. Further research in larger clinical trials is warranted before practical recommendations for the public health can be made.
1Background
Both human and animal studies demonstrate a beneficial impact of intermittent fasting (IF), a term describing several regimens of periods of voluntary abstinence from food and drink, on various aspects of health. IF regimens can be categorized into fasting for up to 24 hours once or twice a week with ad libitum food intake for the remaining days, known as periodic prolonged fasting (PF) or intermittent calorie restriction (ICR) [1]; eating for 8 hours then fasting for the other 16 hours of the day (time-restricted feeding, TRF); and alternating between feasting and fast days (alternate-day fasting, ADF) [2, 3] (Table 1). IF has classically been recognized to ameliorate obesity [2], insulin resistance [4], dyslipidemia [5], blood pressure [4] and inflammation [6]. More recently, IF has been shown to also benefit the gut microbiota [7], a term describing the trillions of microorganisms (bacteria, viruses, protozoa, and fungi),which are present in the human gut and are involved in virtually all aspects of health [8, 9]. This is a vast and complex microbial community, with over 1000 bacterial species identified and approximately 160 species found in the gut of any one person [10], and the most abundant bacterial phyla in the adult gastrointestinal tract are Firmicutes and Bacteroidetes [11, 12].
Table 1
Comparison of different types of intermittent fasting
Type of IF | Description | Metabolic states involved |
Alternate day fasting | Alternating feast (ad lib intake) and fast days (≤25% of energy needs) | Fed, post-absorptive, fasting (short duration, likely < 36 hours between meals) |
Time-restricted fasting | Eating only during certain time periods (i.e., 8 hours), then fasting for remaining hours of the day | Fed, post-absorptive (maximum duration between meals is usually < 16 hours) |
Periodic fasting | Fasting for up to 24 hours once or twice a week with ad lib intake on the remaining days | Fed, post-absorptive, fasting (up to 48 hours between meals depending on whether fast days are consecutive) |
The gut microbiota provides many benefits to the host, such as the biosynthesis of certain vitamins and essential amino acids, and the generation of short-chain fatty acids (SCFAs) as metabolic by-products from undigested food components [13]. SCFAs, including butyrate, propionate, and acetate are a major source of energy for the intestinal cells and may strengthen the intestinal barrier [14] and improve the gut integrity [15], which is paramount in promoting optimum colonic health and function; resulting in better immunity [16]. SCFA production is influenced by gut microbiota composition and diet, with primarily butyrate and acetate decreasing the inflammatory response, whilst increasing the anti-inflammatory response of the adaptive immune system [17]. In addition, butyrate methylates promoter regions, thus influencing gene expression in enterocytes, macrophages and immune cells; deficient SCFA can disrupt these processes, which can lead to an autoimmune response and disease [17]. Furthermore, SCFA and butyrate specifically controls the function and size of the regulatory T cell network by stimulating the induction and fitness of regulatory T cells in the gut [18–20]. In addition, the microbiota is involved in many critical functions to ensure that optimum immune responses can be produced, including aiding development and maturation of lymphoid structures and potentiation of the function of innate immune cells [21]. Whilst, the microbiota is critical for maturation of the immune system, in return, the latter determines the composition of the microbiota. As such, disrupted microbial composition has been associated with several diseases in humans. However, the intricate immune/microbial interactions make it difficult to determine whether dysbiosis, the imbalance of gut microbiota, is a cause and/or a consequence of immune dysregulation and disease initiation or progression [21].
Diet is reported as a major factor influencing gut microbiota and several studies have investigated the impact of different dietary components, including carbohydrates, predominantly fibre, and plant-based diets, on the gut microbiota [22–26]. Nuts and other plant-based foods that are abundant in polyunsaturated and monounsaturated fats and, occasionally, polyphenols and other phytochemicals have been shown to increase bacterial diversity, as well as the beneficial butyrate-producing bacteria revealing a positive metabolic effect [27–28].
With rapidly advancing screening used to analyse and differentiate complex ecosystems, the role of microbiota in a significant number of gastrointestinal diseases has become increasingly clear [29, 30]. Dysbiosis may contribute to the pathogenesis of a vast range of such diseases, including inflammatory bowel disease (IBD), celiac disease, colorectal cancer, Clostridium difficile infection, and obesity [31]. For instance, studies [32–35] have found IBD patients to have less bacterial diversity in the gut and reduced numbers of Bacteroidetes and Firmicutes, potentially leading to decreased concentrations of butyrate that is, along with other SCFAs, believed to have a direct anti-inflammatory effect [33, 36, 37]. Greater diversity in the microbial community has also been associated with a healthier gut microbiome [38–40]; a diverse array of bacteria promotes microbiome capability, and is imperative for a healthy host–microorganism balance to ensure optimal metabolic and immune function.
For this reason, the gut microbiome has become a promising target for prediction, prevention and treatment of diseases [8]. Given that it is evident that diet is a significant modulator of the gut health and microbiota diversity [22–26], and that dietary restrictions such as IF may also contribute to such effect [9], the objective of this review was to summarize the peer-reviewed literature of clinical trials related to the impact of IF regimens on the gut microbiota.
2Methods
The design of the study is a qualitative systematic review in line with the Preferred Reporting Items for Systematic Reviews and Meta-analyses (PRISMA) checklist (Fig. 1). A literature review was performed independently by two reviewers, KLW and MA using PubMed. Search criteria included clinical trials published in English between January 2000 and April 2020 with the keywords “intermittent fasting”, “periodic fasting”, “time-restricted”, “alternate-day fasting”, “whole day fasting”, “gut microbiota”, and “microbes”. References were reviewed from seminal papers to identify additional articles.
Fig. 1
PRISMA 2009 flow diagram.
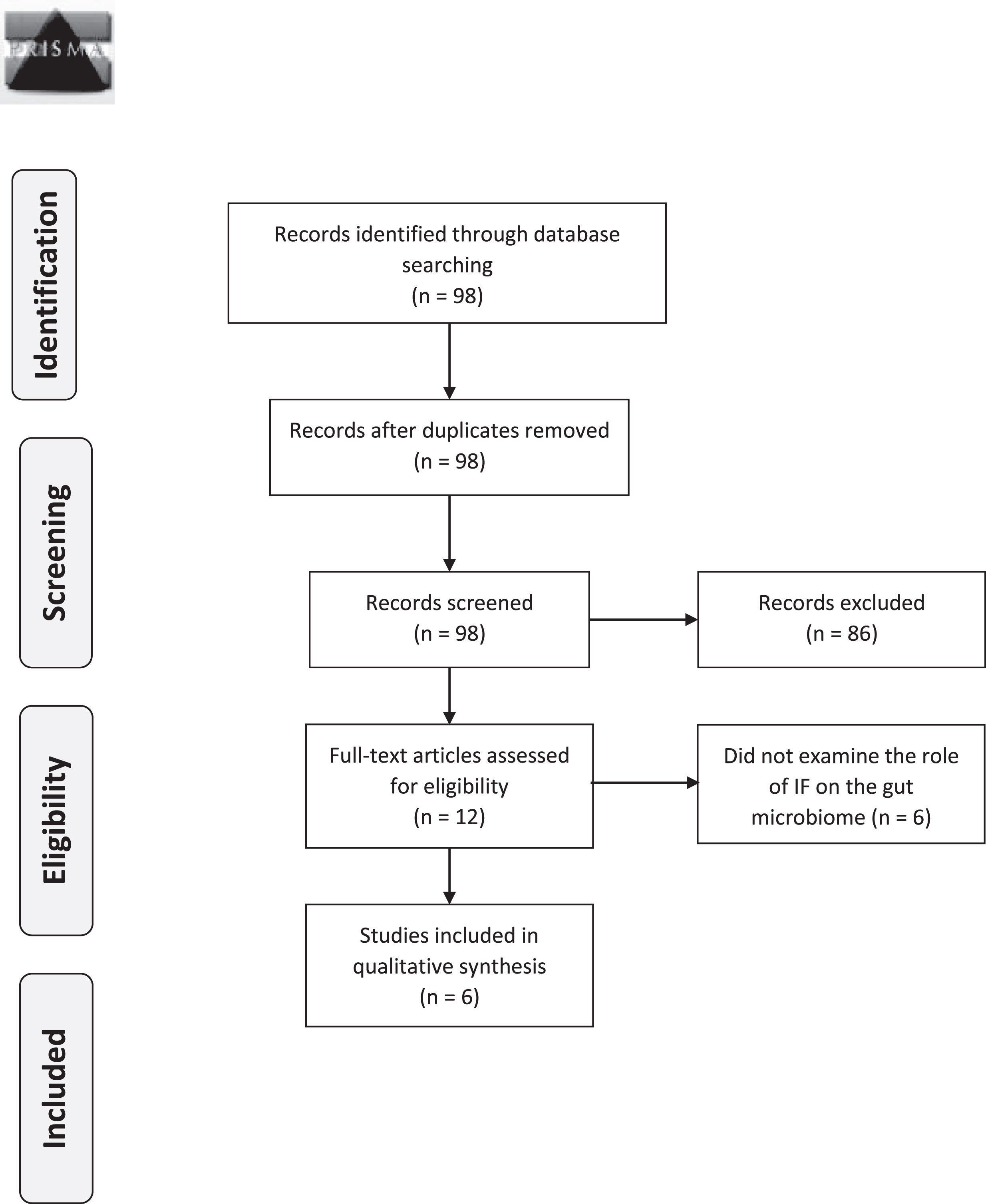
3Results and discussion
Based on the inclusion criteria, 98 clinical studies were identified and six articles were included in this review [8, 41–45] (Fig. 1). Other studies were excluded as they did not specifically examine the role of IF on the gut microbiota. The excluded articles investigated the effect of alternative nutrients’ or foods such as gluten, yogurt, high-fat and/or high-sugar diet on the gut microbiota. Main micro-biota-related findings of studies included in the present review are summarized in Table 2 and are discussed below.
Table 2
Summary of studies included in the present review
Reference | Participants (n) | Duration and type of fasting | Comparison group | Change in microbial Composition |
He et al. (2019) [8] | Healthy adults aged 18–40 years (n = 16) | 1 week: water-only fasting (n = 6) | 1 week: juice fasting (n = 10) | ↓ Fusobacterium water-only fasting |
↔ Akkermansia water-only fasting | ||||
↔ juice fasting | ||||
Remely et al. (2015) [41] | Overweight (n = 13) | CR and PF/TRF (non-traditional fasting regimen) (600–800 kcal) | None | ↑ Lactobacillus |
↑Enterobacteria | ||||
↑ Akkermansia | ||||
Cignarella et al. (2018) [42] | Adults with MS (n = 16) | 15 days: ADF | 15 days: ad libitum | ↑ Bacteroides |
↑ Lactobacillus | ||||
↑ Prevotella (fasting group) | ||||
Özkul et al. (2019) [43] | Healthy adults aged 31–56 years (n = 9) | 29 days: Ramadan fasting /TRF | None | ↑ Bacteroides |
↑ Akkermansia | ||||
Gabel et al. (2020) [44] | Obese adults (n = 14) | 12 weeks: TRF | None | ↔ |
Özkul et al. (2020) [45] | Healthy adults (n = 9) | 29 days: Ramadan fasting /TRF | None | ↑ Bacteroides |
↑ Butyricicoccus | ||||
↑ Faecalibacterium | ||||
↑ Roseburia | ||||
↑ Allobaculum | ||||
↑ Eubacterium | ||||
↑ Dialister | ||||
↑ Erysipelotrichi |
Abbreviations: ADF, alternate-day fasting; CR, calorie restriction; MS, multiple sclerosis; PF, periodic fasting; TRF, time-restricted feeding; ↑, statistically significant increase (p < 0.05); ↔, no change.
Cignarella et al. 2008 [42] initiated a 15-day randomized controlled pilot trial to multiple sclerosis (MS) subjects experiencing relapse; where seventeen subjects were equally randomized to ADF vs ad libitum diet. No bacteria were significantly different at day 15 between the two groups, but the abundance of Faecalibacterium, Lachnospiracea_incertae_sedis and Blautia showed an increasing trend after 15 days of IF [42]. Faecalibacterium and Blautia belong to the Clostridia clusters XIV and XIVa (in the Firmicutes phylum) and have been shown to increase regulatory T cell (Treg) accumulation in the colon [46]. These bacteria are important as they produce acetate and have been observed to be decreased in MS subjects [47]. As such, the increase in the Clostridia clusters XIV and XIVa with IF may function to counterbalance the dysbiosis usually observed in MS [42].
In a 2019 study of sixteen healthy subjects aged 18–40 years and have BMI >18.5 kg/m2, six individuals were allocated to a water-only fast and ten were assigned a juice fast for one week [8]. Daily stool sample collection, prior to and post fasting, started from two weeks before fasting until four weeks after. The authors hypothesized that water only fasting may be a potential therapeutic strategy in reducing Fusobacterium, which has been shown to promote colorectal cancer [8]. However, the differential abundance findings suggest that the impact of fasting on individual microbial taxa is unique and personalized. Despite this individualized effect, relative abundance of Fusobacterium was decreased across all participants in group 1 (P < 0.05) when compared with pre-fasting controls. This finding was not reported in group 2 (P > 0.05), however pre-fasting relative abundance of Fusobacterium was increased in group 1 compared with group 2 participants. In all participants, post water-only fasting Fusobacterium remained consistently reduced. In addition, eight out of ten subjects were not affected by juice fasting, with no increased homogeneity between subjects. These findings suggest that water-only fasting may have a long-lasting effect on the microbiota and a more homogenous microbial community; indicating increased homogeneity and alterations in microbiota demonstrated in water-only fasting may not be necessarily due to the absence of solid food [8]. The authors anticipated relative abundance of Akkermansia in the water-only fast participants since Akkermansia uses mucin as a sole substrate [48]. However, they observed no increase in the relative abundance of Akkermansia after fasting. This suggests that there could be other bacteria that utilize mucin, which compete with Akkermansia in the gut during water-only fasting.
There are a limited number of small-scale human studies that consider the role of Akkermansia mucini-phila, a species of bacteria reported to thrive when undergoing fasting conditions [43, 45], and which may represent 3–5% of the healthy gut microbiota [49-50]. One small intervention study of obese patients demonstrated significant improvement in microbiota diversity and showed a significant increase in A. muciniphila after a week of mild fasting (Remely et al, 2015) [41]. This pilot study [41] assigned thirteen overweight subjects to a non-traditional fasting regimen that involved a limited period of abstinence from solid food and natural stimulants. The fasting regimen was low in energy with an intake of 2.5 L/day of calorie-free liquid (water, herbal tea) or vegetable broth (600–800 calories/day) followed by a probiotic formula. Microbiota diversity was shown to increase due to fasting and probiotic intervention between the time points T1 (before fasting), T2 (during fasting) and T3 (after 6-weeks of probiotic intervention (P = 0.05), and between the time points T2–T3 (P = 0.02) [41]. In addition, the authors reported a significant increase in Akkermansia between the time points (T1–T3: P = 0.03, T1–T2: P = 0.47, T2–T3: P = 0.47).
In a pilot study by Özkul et al., [2019] [43], 9 subjects were included in a fasting protocol involving a 17 h fast/day for 29 days during the month of Ramadan. A significantly increased abundance of A. muciniphila and B. fragilis group was observed in all subjects after fasting when compared with baseline levels (P = 0.004 and 0.008, respectively). A similar Ramadan-based study involving nine subjects by Özkul et al., [2020] [45] demonstrated increased microbial richness (P = 0.016) and differing microbiota composition after 29 days vs before fasting (P = 0.025). Butyricicoccus pullicaecorum (P = 0.002), Faecalibacterium prausnitzii (P = 0.003), and Roseburia (P = 0.02) were the major species that showed a significant increase after the end of Ramadan fasting. A.muciniphila (P = 0.005) and Bacteroides spp (P = 0.02) were also significantly increased post fasting. This finding is similar to that of Remely et al., 2015 [41] in which the authors reported increased A.muciniphila in overweight subject post fasting. Roseburia has the ability to metabolise dietary components, generate SCFAs and influence the integrity of the intestinal epithelial barrier, whilst supporting immunity with its anti-inflammatory capabilities [51]. F. prausnitzii is an anti-inflammatory commensal bacterium that also produces SCFAs [52], whilst B. pullicaecorum has recently been shown to be one of the main butyrate-producing bacterial species with the ability to promote intestinal epithelial barrier integrity with its anti-inflammatory capabilities. Furthermore, in a 2018 study [53] in an antibiotic-disrupted microbiota, depleted B. pullicaecorum was observed.
Finally, in a 12-week pilot study by Gabel et al. 2020 [44], 14 obese adults were allocated to daily 8-hour feeding/16-hour fasting TRF intervention. At baseline, the two most common phyla were Firmicutes and Bacteroidetes, at 61.2% and 26.9%, respectively, of total abundance. The authors hypothesized that the proportion of Firmicutes would decrease and the proportion of Bacteroidetes would increase with TRF, and that these improvements would be associated with weight reduction. Whilst the results indicated that TRF reduced body weight (P < 0.05), TRF did not significantly alter the diversity or overall gut microbiota composition, with no significant changes in the abundance observed at the end of the trial [44]. These findings are contradictory to what has generally been observed with caloric restricted diets [54–56], which have all reported beneficial changes in gut microbiota composition and/or diversity. The authors concluded that in view of these previous findings, it is possible that the weight reduction (2%) and caloric restriction (20%) produced in their study was not sufficient and subsequently, did not impact the gut microbiota composition beneficially [44].
4Conclusion
Chronic calorie restriction (CR) has been reported to elicit metabolic changes, including shaping the gut microbial community in humans [57] and mice [58]. Fecal microbiota of subjects exercising long-term CR may also be more diverse and richer than in individuals consuming Western-style diets [59–61]. Data suggests that chronic CR is, however, difficult to adhere to [62] and thus IF could be a more feasible method for compliance. Although, it still needs to be established whether individuals can maintain IF for long terms or obtain the similar IF benefits observed in animal studies [63–65]. Furthermore, it is still not known which individuals would benefit the most from IF, which form of IF is the most effective, whether there are sex-based differences, or variations between healthy individuals and those present with certain disease. In addition, all the relevant studies have small sample sizes, a drawback that limits the generalizability of the observed effects. Therefore, future research should take these limitations into consideration for better understanding of the role of IF on gut health.
In conclusion, whilst current research is still in its infancy stage, findings of the available human studies, thus far, suggest that IF may play a potentially beneficial role in enhancing changes in gut microbiota composition and diversity. Fasting has been demonstrated to increase the abundance of protective, beneficial microbial families, such as Bifidobacteriaceae, Lactobacillaceae and Akkermanisaceae. The initial findings may be promising for the use of fasting to beneficially influence and alter the gut microbiota. However, further confirmation is warranted, with larger clinical trials with longer observation timeframes needed to replicate the available findings before clinical recommendations may be made on the role of IF in the gut health.
Author contributions
KLW conceived the review idea and wrote the first draft of the manuscript. MA contributed to the article search and revision of the manuscript.
Conflicts of interest
The authors have no proprietary, financial, professional or other personal interest of any nature in any product, service or company. The authors alone are responsible for the content and writing of the paper.
Funding
This work was not supported by any grant or other form of funding.
References
[1] | St-Onge M-P , Ard J , Baskin ML , Chiuve SE , Johnson HM , Kris-Etherton P , et al. Meal timing and frequency: implications for cardiovascular disease prevention: a scientific statement from the American Heart Association. Circulation [Internet]. (2017) ;135: :e96–121. |
[2] | Varady KA , Bhutani S , Church EC , Klempel MC . Short-term modified alternate-day fasting: a novel dietary strategy for weight loss and cardioprotection in obese adults. Am J Clin Nutr. (2009) ;90: :1138–43. |
[3] | Collier R . Intermittent fasting: The science of going without. CMAJ. (2013) ;185: :E363–4. |
[4] | Sutton E , Beyl R , Early K , Cefalu W , Ravussin E , Peterson C . Early Time-Restricted Feeding improves insulin sensitivity, blood pressure, and oxidative stress even without weight loss in men with prediabetes. Cell Metab. (2018) ;27: (6):1212–1221.e3. |
[5] | Anton SD , Moehl K , Donahoo WT , Marosi K , Lee SA , Mainous AG , et al. Flipping the metabolic switch: understanding and applying the health benefits of fasting. Obesity. (2018) ;26: :254. |
[6] | Moro T , Tinsley G , Bianco A , Marcolin G , Pacelli Q , Battaglia G , Palma A . Effects of eight weeks of time-rest-ricted feeding (16/8) on basal metabolism, maximal strength, body composition, inflammation, and cardiovascular risk factors in resistance-trained males. J Transl Med. (2016) ;14: :291. |
[7] | Lederberg J , McCray AT . ’Ome Sweet ’Omics—a genealogical treasury of words. Scientist. (2001) ;15: :8. |
[8] | He Y , Yin J , Lei J , Liu F , Zheng H , Wang S , Wu S , Sheng H , McGovern E , Zhou H . Fasting challenges human gut microbiome resilience and reduces Fusobacterium.Medicine in Microecology 1-2. 2019;100003. |
[9] | Johnson AJ , Vangay P , Al-Ghalith GA , Hillmann BM , Ward TL , Shields-Cutler RR , et al. Daily sampling reveals personalized diet-microbiome associations in humans. Cell Host Microbe. (2019) ;25: (6):789–802. |
[10] | Rajilić-Stojanović M , de Vos WM . The first cultured species of the human gastrointestinal microbiota. FEMS Microbiol Rev. (2014) ;38: :996–1047. |
[11] | Flint HJ , Duncan SH , Scott KP , Louis P . Interactions and competition within the microbial community of the human colon: links between diet and health. Environ Microbiol. (2007) ;9: :1101–11. |
[12] | Walker AW , Ince J , Duncan SH , Webster LM , Holtrop G , Ze X , et al. Dominant and diet-responsive groups of bacteria within the human colonic microbiota. ISME J.. (2011) ;5: :220–30. |
[13] | Bäckhed F , Ley RE , Sonnenburg JL , Peterson DA , Gordon JI . Host-bacterial mutualism in the human intestine. Science. (2005) ;307: :1915–20. |
[14] | Topping DL , Clifton PM . Short-chain fatty acids and human colonic function: roles of resistant starch and non-starch polysaccharides. Physiol Rev. (2001) ;81: :1031–64. |
[15] | Natividad JM , Verdu EF . Modulation of intestinal barrier by intestinal microbiota: Pathological and therapeutic implications. Pharmacol. Res. (2013) ;69: :42–51. |
[16] | Gensollen T , Iyer SS , Kasper DL , Blumberg RS . How colonization by microbiota in early life shapes the immune system. Science. (2016) ;352: :539–44. |
[17] | Theyab A , Almutairi T , Al-Suwaidi A , Bendriss G , McVeigh C , Chaari A . Epigenetic effects of gut metabolites: Exploring the path of dietary prevention of Type 1 diabetes. Front Nutr 7: :563605. |
[18] | Arpaia N , Campbell C , Fan X , Dikiy S , van der Veeken J , Deroos P , Liu H , Cross JR , Pfeffer K , Coffer PJ , et al. Metabolites produced by commensal bacteria promote peripheral regulatory T-cell generation. Nature. (2013) ;504: (7480):451–5. |
[19] | Furusawa Y , Obata Y , Fukuda S , Endo TA , Nakato G , Takahashi D , Nakanishi Y , Uetake C , Kato K , Kato T , et al. Commensal microbe-derived butyrate induces the differentiation of colonic regulatory T cells. Nature. (2013) ;504: (7480):446–50. |
[20] | Smith PM , Howitt MR , Panikov N , Michaud M , Gallini CA , Bohlooly YM , Glickman JN , Garrett WS . The microbial metabolites, short-chain fatty acids, regulate colonic Treg cell homeostasis. Science. (2013) ;341: :569–73. |
[21] | Alegre M , Mannon R , Mannon P . The microbiota, the immune system and the allograft. Am J Transplant. . (2014) ;14: (6):1236–48. |
[22] | Zimmer J , Lange B , Frick J-S , Sauer H , Zimmermann K , Schwiertz A , et al. A vegan or vegetarian diet substantially alters the human colonic faecal microbiota. Eur J Clin Nutr. (2012) ;66: :53–60. |
[23] | De Filippis F , Pellegrini N , Vannini L , Jeffery IB , La Storia A , Laghi L , et al. High-level adherence to a Mediterranean diet beneficially impacts the gut microbiota and associated metabolome. Gut. (2016) ;65: :1812–21. |
[24] | Klinder A , Shen Q , Heppel S , Lovegrove JA , Rowland I , Tuohy KM . Impact of increasing fruit and vegetables and flavonoid intake on the human gut microbiota. Food Funct. (2016) ;7: (4):1788–96. |
[25] | Tomova A , Bukovsky I , Rembert E , Yonas W , Alwarith J , Barnard M , Kahleova H . The effects of vegetarian and vegan diets on gut microbiota. Front Nutr. (2019) ;6: :47. |
[26] | Zmora N , Suez E and Elinav E . You are what you eat: diet, health and the gut microbiota. Nat Rev Gastroenterol Hepatol. (2019) ;16: (1):35–56. |
[27] | Ukhanova M , Wang X , Baer DJ , Novotny JA , Fredborg M , Mai V . Effects of almond and pistachio consumption on gut microbiota composition in a randomised cross-over human feeding study. Br J Nutr. (2014) ;111: :2146–52. |
[28] | Hernández-Alonso P , Cañueto D , Giardina S , Salas-Salvadó J , Cañellas N , Correig X , et al. Effect of pistachio consumption on the modulation of urinary gut microbiota-related metabolites in prediabetic subjects. J Nutr Biochem.. (2017) ;45: :48–53. |
[29] | Chang C , Lin H . Dysbiosis in gastrointestinal disorders. Best Pract. Res Clin Gastroenterol. (2016) ;30: :3–15. |
[30] | Schroeder SO , Bäckhed F . Signals from the gut microbiota to distant organs in physiology and disease. Nat Med. (2016) ;22: :1079–89. |
[31] | Kho Z , Lal S . The Human Gut Microbiome - A Potential Controller of Wellness and Disease. Front Microbiol. . (2018) ;9: :1835. |
[32] | Frank DN , Amand ALS , Feldman RA , Boedeker EC , Harpaz N , Pace NR . Molecular-phylogenetic characterization of microbial community imbalances in human inflammatory bowel diseases. Proc Natl Acad Sci USA. (2007) ;104: :13780–5. |
[33] | Mondot S , Kang S , Furet JP , Aguirre de Cárcer D , McSweeney C , Morrison M , Marteau P . et al. Highlighting new phylogenetic specificities of Crohn’s disease microbiota. Inflamm Bowel Dis. (2010) ;17: :185–92. |
[34] | Hedin CR , McCarthy NE , Louis P , Farquharson FM , McCartney S , Taylor K , Prescott NJ , et al. Altered intestinal microbiota and blood T cell phenotype are shared by patients with Crohn’s disease and their unaffected siblings. Gut.. (2014) ;63: :1578–86. |
[35] | Li J , Butcher J , Mack D , Stintzi A . Functional impacts of the intestinal microbiome in the pathogenesis of inflammatory bowel disease. Inflamm. Bowel Dis. (2014) ;21: :139–53. |
[36] | David LA , Maurice CF , Carmody RN , Gootenberg DB , Button JE , Wolfe BE , et al. Diet rapidly and reproducibly alters the human gut microbi—ome. Nature. (2014) ;505: :559–63. |
[37] | Zeng MY , Inohara N , Nuñez G . Mechanisms of inflamma-tion-driven bacterial dysbiosis in the gut. Mucosal Immunol. (2017) ;10: :18–26. |
[38] | Lozupone C , Stombaugh J , Gordon J , Jansson J , Knight R . Diversity, stability and resilience of the human gut microbiota. Nature. (2012) ;489: (7415):220–30. |
[39] | Le Chatelier E , Nielsen T , Qin J , Prifti E , Hildebrand F , Falony G , Almeida M , Arumugam M , Batto JM , Kennedy S , et al. Richness of human gut microbiome correlates with metabolic markers. Nature. (2013) ;500: :541–6. |
[40] | Heiman ML , Greenway FL . A healthy gastrointestinal microbiome is dependent on dietary diversity. Molecular Metabolism. (2016) ;5: :317–20. |
[41] | Remely M , Hippe B , Geretschlaeger I , Stegmayer S , Hoefinger I , Haslberger A . Increased gut microbiota diversity and abundance of Faecalibacterium prausnitzii and Akkermansia after fasting: a pilot study. Wien Klin Wochenschr. (2015) ;127: (9-10):394–8. |
[42] | Cignarella F , Cantoni C , Ghezzi L , Salter A , Dorsett Y , Chen L , Phillips D , Weinstock GM , Fontana L , Cross AH , Zhou Y , Piccio L . Intermittent Fasting Confers Protection in CNS Autoimmunity by Altering the Gut Microbiota. Cell Metab. (2018) ;27: (6):1222–35. |
[43] | Özkul C , Yalınay M , Karakan T . Islamic fasting leads to an increased abundance of Akkermansia muciniphila and Bacteroides fragilis group: A preliminary study on intermittent fasting. Turk J Gastroenterol. (2019) ;30: (12):1030–5. |
[44] | Gabel K , Marcell J , Cares K , Kalam F , Cienfuegos S , Ezpeleta M , Varady KA . Effect of time restricted feeding on the gut microbiome in adults with obesity:Apilot study. Nutr Health. 2020;260106020910907. |
[45] | Özkul C , Yalinay M , Karakan T . Structural changes in gut microbiome after Ramadan fasting: a pilot study. Benef Microbes. 2020:1-8. |
[46] | Atarashi K , Tanoue T , Shima T , Imaoka A , Kuwahara T , Momose Y , Cheng G , Yamasaki S , Saito T , Ohba Y , et al. Induction of colonic regulatory T cells by indigenous Clostridium species. Science. (2011) ;331: :337–41. |
[47] | Miyake S , Kim S , Suda W , Oshima K , Nakamura M , Matsuoka T , Chihara N , Tomita A , Sato W , Kim SW , et al. Dysbiosis in the Gut Microbiota of Patients with Multiple Sclerosis, with a Striking Depletion of Species Belonging to Clostridia XIVa and IV Clusters. PloS one. (2015) ;1: :e0137429. |
[48] | Geerlings S , Kostopoulos I , de Vos W , Belzer C . Akkermansia muciniphila in the Human Gastrointestinal Tract: When, Where, and How? Microorganisms. (2018) ;6: (3). |
[49] | Everard A , Belzer C , Guerts L , Ouwerkerk J , Druart J , Bindels L , Guiot Y , et al. Cross-talk between Akkermansia muciniphila and intestinal epithelium controls diet-induced obesity. Proceedings of the National Academy of Sciences of the USA 110: :9066–9071. |
[50] | Santacruz A , Collago M , Garcia-Valdes L , Segura M , Martin-Lagos J , Anjos T , Marti-Romero M . et al. Gut microbiota composition is associated with body weight, weight gain, and biochemical parameters in pregnant women. British Journal of Nutrition 104: :83–92. |
[51] | Tamanai-Shacori Z , Smida L , Bousarghin L , Loreal O , Meuric V , Fong S , Bonnaure-Mallet M , et al. Roseburia spp: a marker of health? Future Microbiology 12: :157–70. |
[52] | Sokol H , Pigneur B , Watterlot L , Lakhdari O , Bermudez-Humaran I , Gratadoux J , Blugeon S , et al. Faecalibacterium prausnitzii is an anti-inflammatory commensal bacterium identified by gut microbiota analysis of Crohn disease patients. Proceedings of the National Academy of Sciences of the USA 105: :16731–6. |
[53] | Le Bastard Q , Ward T , Sidiropoulos D , Hillman B , Chun C , Sadowsky M , Knights D . et al. Fecal microbiota transplantation reverses antibiotic and chemotherapy-induced gut dysbiosis in mice. Scientific Reports 8: :6219. |
[54] | Kim M , Hwang S , Park E , Bae J-W . Strict vegetarian diet improves the risk factors associated with metabolic diseases by modulating gut microbiota and reducing intestinal inflammation. Environmental Microbiology Reports. (2013) ;5: (5):765–75. |
[55] | Heinsen F , Fangmann D , Muller N , Schulte D , Ruhlemann M , Turk K , Settgast U . et al. Beneficial effects of a dietary weight loss intervention on human gut microbiome diversity and metabolism are not sustained during weight maintenance. Obesity Facts. (2016) ;9: (6):379–91. |
[56] | Frost F , Storck L , Kacprowski T , Gartner S , Ruhlemann M , Bang C , Franke A , et al. A structured weight loss program increases gut microbiota phylogenetic diversity and reduces levels of Collinsella in obese type 2 diabetics: A pilot study. PLoS One. (2019) ;14: (7):e0219489. |
[57] | Zhang C , Li S , Yang L , Huang P , Li W , Wang S , Zhao G , Zhang M , Pang X , Yan Z , et al. Structural modulation of gut microbiota in life-long calorie-restricted mice. Nature communications. (2013) ;4: :2163. |
[58] | Griffin NW , Ahern PP , Cheng J , Heath AC , Ilkayeva O , Newgard CB , Fontana L , and Gordon JI) . Prior Dietary Practices and Connections to a Human Gut Microbial Metacommunity Alter Responses to Diet Interventions. Cell Host Microbe. (2017) ;21: :84–96. |
[59] | Davis C . The gut microbiome and its role in obesity. Nutr. Today. (2016) ;51: (4):167–74. |
[60] | Hills R , Pontefract B , Mishcon H , Black C , Sutton S , Theberge C . Gut microbiome: Profound Implications for diet and disease. Nutrients. (2019) ;11: (7):1613. |
[61] | Klement R and Paziena V . Impact of different types of diet on gut microbiota profiles and cancer prevention and treatment. Medicine. (2019) ;55: (4):81. |
[62] | Carter S , Clifton P , Keogh J . Effect of Intermittent Compared With Continuous Energy Restricted Diet on Glycemic Control in Patients with Type 2 Diabetes A Randomized Noninferiority Trial. JAMA Netw Open. (2018) ;1: (3):e180756. |
[63] | Antoni R , Johnston KL , Collins AL , Robertson MD . Effects of intermittent fasting on glucose and lipid metabolism. Proc Nutr Soc. 2017:1-8. |
[64] | Harvie M , Howell A . Potential Benefits and Harms of Intermittent Energy Restriction and Intermittent Fasting Amongst Obese, Overweight and NormalWeight Subjects-A Narrative Review of Human and Animal Evidence. Behav Sci (Basel). 2017;7. |
[65] | Patterson RE , Sears DD . Metabolic Effects of Intermittent Fasting. Annu Rev Nutr. (2017) ;37: :371–93. |