Why do sexes differ in lifespan extension? Sex-specific pathways of aging and underlying mechanisms for dimorphic responses
Abstract
Males and females typically have different lifespans and frequently differ in their responses to anti-aging interventions. These sex-specific responses are documented in mice and Drosophila species, in addition to other organisms where interventions have been tested. While the prevalence of sex-specific responses to anti-aging interventions is now recognised, the underlying causes remain poorly understood. This review first summarises the main pathways and interventions that lead to sex-specific lifespan responses, including the growth-hormone/insulin-like growth factor 1 (GH-IGF1) axis, mechanistic target of rapamycin (mTOR) signalling, and nutritional and pharmacological interventions. After summarising current evidence, several different potential causes for sex-specific responses are discussed. These include sex-differences in xenobiotic metabolism, differing disease susceptibility, sex-specific hormone production and chromosomes, and the relative importance of different signalling pathways in the control of male and female life-history. Understanding why sex-differences in lifespan-extension occur should provide a greater understanding of the mechanisms that regulate the aging process in each sex, and will be crucial for understanding the full implications of these treatments if they are translated to humans.
1Introduction
Lifespan can now be extended through a variety of different interventions, across organisms as diverse as yeast — a single celled fungus — to mice. At least some of these interventions cause improvements to measures of health during aging [1], including in primates [2], and small scale clinical trials have been conducted to test for efficacy and potential health benefits in elderly humans [3, 4]. It is clear from the phylogenetic diversity of tested organisms that some signals controlling the aging process have been conserved across evolution. For example, treating laboratory mice (Mus musculus), nematode worms (Caenorhabditis elegans), fruit flies (Drosophila melanogaster) or yeast (Saccharomyces cerevisiae) with rapamycin – which inhibits target of rapamycin (mTOR) signalling – leads to lifespan extension [5], and on average the change in median lifespan is similar in each of these species [6]. In spite of these commonalities, there are factors within each species that influence the effectiveness of a particular intervention. Interventions can have different levels of effectiveness in different strains [7], and treatment effects can interact with environment conditions (e.g. temperature [8], diet [9]) to influence the degree of lifespan extension. It is becoming increasingly recognised that lifespan effects of many manipulations also differ according to sex [10]. Using rapamycin as an example, lifespan extension in mice usually occurs to a greater degree in female mice than males [11], an effect observed across different strains [12], and also mimicked in genetic mutant animals where signalling through the mTOR pathway has been dampened genetically [13]. However, life-extension is not always a female-dominant phenomena: treatment of mice with pharmacological compounds including acarbose and 17-α estradiol can lead to greater lifespan extension in males [14]. Sex-differences in lifespan extension are also frequently reported in fruit flies, which have a different sex-determination system and physiological control of sex-specific phenotypes. Given the clear influence of sex on human lifespan [15], understanding the mechanisms and degree to which males and females respond differently to anti-aging interventions will be crucial as these treatments are translated to a clinical context.
1.1How do we know a manipulation has a sex-specific effect?
A startling array of manipulations have been reported to have sex-specific effects on lifespan. One important statistical caveat to consider is that just because an intervention has a statistically significant effect on lifespan in one sex, but not in the other, does not automatically mean that males and females really respond differently to that manipulation. Random differences in variation between the populations, perhaps as a consequence of one or two outlier deaths, can influence whether a survival test reaches the criterion for statistical significance in one sex [16]. Consider a hypothetical statistical test that gives a P value of P = 0.049 for a treatment effect in one sex, versus P = 0.051 for a treatment effect in the other sex, as an extreme example of how a slight difference in variation could influence whether a test is statistically significant in either of the sexes [17]. If one sex shows more variability in lifespan then this would reduce the probability of detecting a significant difference between control and treatment groups in lifespan [18]. Comparisons of lifespan extension within each sex are sometimes conducted with a relatively small sample size, particularly given the small total sample sizes of many lifespan studies [19]. Therefore effects of one or two outliers will be felt more strongly in sex-specific comparisons. One way to test whether responsiveness to a manipulation is sex-specific is by conducting a test for a statistical interaction between sex and the treatment response. However, this requires extension from the typical Log-Rank Test, or more complicated survival models [20], which biomedical scientists are less familiar with and often do not use. Such tests are now more frequently used in genetic epistasis studies, and tests of treatment responses on different genetic backgrounds [21], and could be helpful in quantifying the sex-specific nature of lifespan extension in new studies.
In spite of the caveats in quantifying sex-differences in lifespan extension, there are now several different interventions that have been shown to have stronger effects in one sex than the other, where this has been replicated several times across different institutions and animal strains [10]. This review focuses on manipulations where this sex-specificity has been repeatedly documented, predominantly in mouse models with reference to Drosophila where applicable.
2Interventions with sex-specific aging effects
2.1GH-IGF1 signalling
Lifespan extension has been repeatedly documented in mice with mutations that cause developmental pituitary deficiencies, resulting in dwarf animals living approximately 30% longer than controls [22–24]. Ames and Snell dwarf mice do not develop functional pituitaries, and as a consequence have very low circulating levels of growth-hormone (GH), thyroxine and prolactin. Also notable, as a side product of this, they show various reproductive deficiencies, resulting in low circulating levels of gonadally-derived hormones including testosterone and estrogens [25]. Robust lifespan extension has been repeatedly shown in both male and female dwarf and GH deficient mouse models. Some variation in the degree of lifespan extension has been observed between the sexes. For example, the first study reporting lifespan extension in Ames dwarf mice noted an increase in average lifespan of 350 days for males and more that 470 days for females. However, this study had a relatively small sample size of approximately 15 mice per genotype per sex; while clearly sufficient to show the strong effect of dwarfism on aging, the particularly long lifespan of two female dwarfs contributed to the apparent difference in average lifespan increase between sexes [23]. A 2017 meta-analysis of lifespan extension in pituitary and GH-deficient mice reported no significant moderator effect of sex on the degree of lifespan-extension in these models [26]. This indicates that there is no consistent bias in the degree of lifespan extension between sexes when considering data from all available published studies. It should be noted that in meta-analysis the effect size observed in each study is weighed against an estimate of sampling variance. This means that effect sizes of larger more precise studies have greater weighting in the meta-analysis. However, studies conducted in differing environments (for example, SPF versus non-SPF environments) are typically weighed equally, and are not distinguished unless specifically accounted for by an additional term in the meta-analytic model. It is therefore possible that differences in the degree of lifespan extension between sexes with reduced pituitary function might arise in particular conditions, although this is not a consistent feature across all environments where these mice have been studied [26].
Lifespan extension in pituitary deficient mice is at least partly attributable to effects of low GH signalling, with GH receptor-deficient mice recapitulating many of the lifespan effects, including a consistent increase in lifespan in both sexes [9, 27]. Working downstream of GH signalling, there has been substantial investment in defining the role of insulin-like growth factor-1 (IGF1) signalling in mammalian lifespan, particularly since related insulin signals control aging in invertebrates [28]. This work has shown that the degree of life-extension, in terms of change in median lifespan [26], is smaller when IGF1 signalling is directly reduced compared to GH signalling. In additional to this smaller life-extension, reduced IGF1 signalling also appears to have a greater effect on female lifespan than that of males, an effect that has been observed across several different manipulations of the IGF1 pathway, including heterozygous deletion of the IGF1 receptor (IGF1R+/–) [29–31], conditional knockout of hepatic IGF1 production [32], mice with a hypomorphic IGF1 gene that have low expression levels of IGF1 [33], and pregnancy-associated plasma protein A knockout mice, which show a decrease in IGF1 availability [34]. In addition to observed lifespan effects, improvements in late-life function are observed in female mice treated with an antibody that antagonizes IGF1R [35], but not males, and female IGF1R+/– mice show increased paraquat resistance in adulthood but males do not [29, 30]. A sex-specificity in the GH-IGF1 pathway for lifespan responses may also be manifest by manipulations further downstream, since disrupted expression of insulin-receptor substrate 1, which transduces information from IGF1R, extends lifespan in females but not males [36] (although the authors acknowledge this may be a consequence of sample size). Although less well studied, there is also a hint that heterozygous loss of the insulin receptor has sex-specific lifespan effects in mice, although the changes in lifespan in either sex are weak [37].
Current evidence for the effects of reduced GH-IGF1 signalling on lifespan in mice leads to the interesting conclusion that general pituitary deficiencies and impaired GH signalling impact lifespan similarly in both sexes, while reducing IGF1 signalling directly increases lifespan to a greater degree in females. The underlying causes for this are unknown. It is interesting to note that GH signalling is a major controller of sexual dimorphism, with sex differences in GH pulsitility leading to sex differences in body size [38] and gene expression [39]. GH and pituitary deficiencies can remove these sex differences [40, 41], while reducing IGF1 signalling can leave these sex-differences intact [30]. This could hint that some sex-specific responses to anti-aging interventions may be linked to sexual dimorphism in size and function, as discussed further below.
Downstream of the insulin and IGF1 receptors, a cascade of signalling complexes transduce growth factor information, integrating further signals of cellular nutrient and energy status. One key target is the mechanistic target of rapamycin (mTOR) signalling complex, which has wide-ranging effects on cellular growth and stress resistance [42]. Manipulation of mTOR activity itself influences lifespan, in a range of organisms [5]. Where two sexes are present, differences in the degree of lifespan extension in males and females have been repeatedly noted after mTOR inhibition.
2.2Altered mTOR signalling
The mechanistic target of rapamycin represents two related complexes – mTORC1 and mTORC2 – each comprised of several protein sub-units [42]. Much of the research on the link between mTOR and aging has come from pharmacological studies using the drug rapamycin, which acutely inhibits mTORC1, although chronic rapamycin treatment can have inhibitory effects on mTORC2 signalling in certain tissues [43]. Rapamycin treatment extends lifespan in several organisms including mice, fruit flies, nematode worms and yeast, with genetic perturbation of mTORC1 activity – typically through inactivating a gene/genes encoding for one or several subunits that comprise this complex – recapitulating these effects on lifespan [5]. Effects of mTORC2 inhibition are more variable and species-specific. In worms and flies, depleted expression of different mTORC2 protein-subunits has led to lifespan extension in one study [44], but a reduction in lifespan in another [45]. In mice, the consequences of impaired mTORC2 signalling have been studied in models where the Rictor gene has been conditionally knocked out. This encodes the RICTOR protein, an essential component of the mTORC2 complex. Male mice with heterozygous deletion of Rictor globally, liver-specific deletion, or induced global deletion of Rictor in adulthood, all show a reduction in lifespan compared to controls, while the same manipulations have little effect on female lifespan [46].
Reduced mTOR signalling via rapamycin and impaired mTORC1 activity has been shown to robustly extend lifespan in both sexes in mice, although when relative life-extension is compared, effects are consistently greater in females than males [6, 11, 47]. Notably though, with rapamycin treatment in food there are strong dose-dependent effects, with lifespan increasing more greatly with higher doses in both sexes [48], at least at levels tested to date. Furthermore, there is evidence that at equivalent levels of rapamycin administration in diet females show higher circulating levels in blood when compared to males [48]. This indicates that part of the sex-specificity at a specific dose may be caused by different rates of drug metabolism between males and females [48]. However, relatively greater lifespan extension in females has also been observed when mTORC1 signalling is reduced genetically via heterozygous deletion of two mTORC1 subunits [13], where differences in drug metabolism would not be expected to factor. Mice with deletion of Ribosomal S6 Kinase 1, a key downstream target of mTORC1 that controls protein translation, also show lifespan extension in females only [49], suggesting this sexual dimorphism could be linked to sexually dimorphic consequences of reduced protein translation. It has also been suggested that reduced mTOR signalling may have sexually dimorphic effects on fruit fly survival, providing greater lifespan extension in females also [50]. However, a 2016 meta-analysis of survival effects of reduced mTOR signalling documented no consistent sexual-dimorphism in mTOR mediated fly life-extension, including with rapamycin treatment [6]. Studies of rapamycin treatment in fruit flies have documented variable effects on longevity including life-shortening [51, 52] and lifespan-extension, with a wide variety of relative effects on lifespan in males and females [53–56]. The lack of consistency may represent strain-specific effects, or sensitivity of treatment to environmental and nutrient conditions.
In the cases of rapamycin treatment in mice, sex-specific effects on lifespan may be partly attributable to suppression of mTORC2 signalling, which as highlighted above leads to a reduction in lifespan specifically in males. The mechanisms that lead to this life-shortening are not defined: it may be a consequence of earlier, or more deleterious effects on glucose tolerance in males than females, although this does not seem to be purely a consequence of mTORC2-regulation of hepatic AKT signalling, since mice with heterozygous deletion of Rictor also show male-specific lifespan reductions, but hepatic AKT signalling remains normal [46]. Genetic manipulations that impair the insulin signalling cascade can also have male-specific effects on survival and glucose tolerance. Male mice with deletion of insulin receptor substrate (IRS) II die quickly in adulthood, while females die much later, a sexual dimorphism linked to a much earlier onset of severe diabetes in male mice [57]. It’s notable that the sex-specific life-shortening effects of mTORC2 and IRS2 loss have been examined in C57BL/6 animals. The C57BL/6 strain has an established susceptibility to metabolic dysfunction, and the life-shortening effects of another insulin signalling deficient mouse strain appears greater in this strain than in some others [58]. It would be of interest to determine whether male mortality occurring with deficiencies in IRS2 and mTORC2 signalling also occurs on other strain backgrounds.
2.3Sex-specific responses to dietary restriction
The intervention most intensively studied with respect to lifespan is dietary restriction (DR). Effects of dietary restriction on lifespan have been studied in model organisms, but also a wide range of other species, including in a variety that have two sexes [59]. Reports in mice [7] and fruit flies [50] have indicated that lifespan extension with DR can be stronger/more robust in females than males. Nakawaga et al. [60] conducted a meta-analysis of the effects of DR on lifespan across 36 species, testing among other things whether sex moderates the lifespan response. Their study showed that females on average show greater lifespan extension with DR than males, an analysis that collated data from a range of different species, including mice, rats, dogs, several species of fly, redback spiders, and field crickets [60]. One interesting observation from this study is that sexual dimorphism in lifespan extension with DR occurs in a similar direction across species of widely varying taxa, with different sex determination systems.
Sex-specific responses to DR have been specifically investigated in fruit flies, where the underlying cause for this difference in response has been linked to sex-differences in the development of gut pathology [50]. In this study female fruit flies were found to develop greater gut pathology during aging than males, but this pathology was ameliorated with DR, which the authors postulated was the cause of sexual dimorphism in lifespan extension. In flies, sex-determination is cell autonomous and mediated by sex-chromosomes; by manipulating this system the authors were then able to generate male flies with a feminized mid-gut, producing males that showed gut physiology (increased cell-size) and gene expression typical of females. These gut-feminized males developed female specific gut pathology during aging, and importantly DR ameliorated this effect, leading to a greater lifespan extension in gut-feminised males compared to normal male controls [50]. This study provides a rare insight into a potential source from which sex-specific lifespan extension occurs. Interestingly, females in this study lived longer than males in control conditions, so this correction of gut pathology was not just rectifying a single pathology type that caused sexual dimorphism in lifespan. While gut pathology constrained female lifespan, it did not constrain that of males, meaning that there were likely other factors that limited male lifespan in their study.
2.4Sex-differences in response to pharmacological compounds
The examples documented above have generally reported lifespan extension with preferential benefits to females, but treatment with several pharmacological compounds in mice have shown survival benefits that are greater in males. The discovery of this male-specific lifespan extension has come mainly from the Interventions Testing Program (ITP), which tests suggested compounds to see whether they extend the lifespan of genetically heterogenous mice at three different US institutions (The University of Michigan, The University of Texas Health Science Center at San Antonio, The Jackson Laboratories) [61]. In addition to showing lifespan extension with rapamycin, which preferentially increases female lifespan [11, 47], the ITP have shown that lifespan extension with the compounds nordihydroguaiaretic acid (NDGA), aspirin, acarbose and 17-α estradiol occurs to a greater extent in male mice than females [14, 62–64]. The degree of lifespan-extension and sex-specificity differs with the compound: acarbose leads to lifespan extension of approximately 15–20% in males, but approximately 5% in females. Greater male lifespan extension has been observed at each institution, at several different dosing levels [62], and when starting the treatment either early or later in life [63]. Although acarbose still has a significant effect on female lifespan, albeit smaller than in males, NDGA and 17-α estradiol extend lifespan only in males, with no detectable lifespan effect in females. NDGA’s effects on male lifespan seem confined to changes in median lifespan, while 17-α estradiol extends median and maximal lifespan and provides an approximate 20% increase in lifespan in males [63]. Both have been tested at two different doses, and in neither instance was female lifespan detectably altered by treatment [14, 63, 64].
Fig.1
Several suggestions of hypothetical causes for sex-specific responses to anti-aging interventions. (A) sex-differences in xenobiotic metabolism as a cause for sex-specific life-extension; (B) sex-differences in disease susceptibility; (C) differential importance of signalling pathways in control of aging in each sex; (D) differences in activation status of aging pathways in each sex.
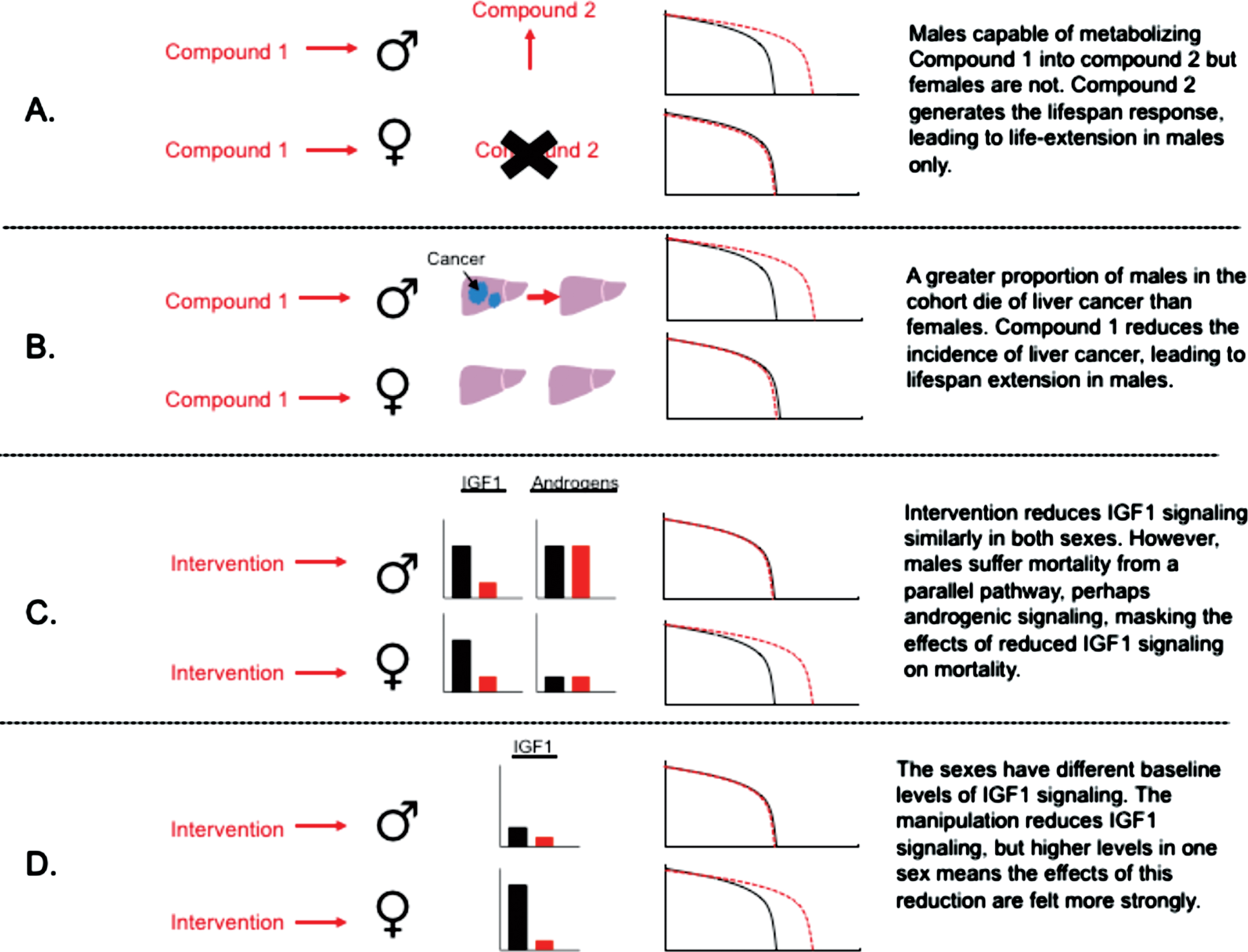
Table 1
Some interventions showing sexual dimorphism in lifespan extension and suggestions for why this sex-specificity occurs. Note these suggestions are usually acknowledged as speculative discussion, where authors highlight the need for further experiments. Arrows indicate lifespan extension. Two arrows versus one arrow indicates lifespan extension in both sexes, but a greater effect in the sex with two arrows
Intervention | Effect in females | Effect in males | Species | Suggested reason for sex-difference | Reference |
Rapamycin/mTOR inhibition | ↑↑ | ↑ | Mice | Sex-specific drug metabolism | Miller et al. 2014 [48] |
Interactions with sex hormones | Lamming 2014 [110] | ||||
Negative effects of mTORC2 suppression in males | Lamming et al. 2014 [46]/Austad &Bartke [10] | ||||
Differences in baseline signalling levels | Regan &Partridge 2013 [111]/ Barr et al. 2016 [98] | ||||
Acarbose | ↑ | ↑↑ | Mice | Improves male-specific metabolic dysfunction | Harrison et al. 2014 [14] |
Interactions with sex hormones | Garratt et al. 2017 [69] | ||||
Reduced IGF1 signalling | ↑ | – | Mice | Sex-differences in glucose metabolism/endocrinology | Holzenberger et al. 2003 [30] |
Interactions with sex-hormones/sex-specific drug metabolism | Mao et al. 2018 [35]/Austad &Bartke 2016 [10] | ||||
Dietary restriction | ↑↑ | ↑ | Various | Amelioration of sex-specific gut pathology | Regan et al. 2016 [50] |
Sex differences in level of reproductive investment | Nakagawa et al. 2012 [60] | ||||
Aspirin/nordihydroguaiaretic acid | – | ↑ | Mice | Differences in drug metabolism | Strong et al. 2008 [64]/ Austad &Bartke 2015 [10]/Austad &Fisher 2016 [15] |
17- α estradiol | – | ↑ | Mice | Interaction with sex hormones | Garratt et al. 2017 [26] |
Sex-specific drug metabolism | Garratt et al. 2018 [72] |
The three drugs showing a large male-specific lifespan-extension have fairly disparate postulated modes of action, although all have been reported to have anti-inflammatory effects in some instances [65–67]. Acarbose is an anti-diabetes drug that slows the breakdown of complex carbohydrates in the gut, leading to blunting of postprandial glucose peaks [68]. A recent study showed that acarbose is more effective at blunting postprandial glucose in males, with glucose levels in females less influenced by acarbose after feeding [62]. By contrast, the effects of acarbose on blood glucose levels after a prolonged period of fasting (18hrs) are similar in both sexes, increasing plasma glucose presumably due to slowed starch breakdown [69]. One notable observation in relation to acarbose is that while lifespan extension is greater in males, some markers of health during aging are affected similarly in both sexes [62]. This contrasts with the effects of 17-α estradiol, where effects on physical function during aging (rotarod capacity, grip strength) are improved specifically in males, with females unaffected by treatment [70].
The observation that three different drugs lead to a substantial increase in male lifespan, with minimal effects in females, demonstrates the presence of manipulations with preferential benefits for males too. Understanding whether these have similar or different effects on male physiology could provide an insight into pathways important in regulation of male lifespan. Lifespan extension with both acarbose and 17-α estradiol is associated with an enhancement of hepatic mTORC2 signalling, but this only occurs in male mice, matching the lifespan response and improvements in glucose tolerance [69]. As highlighted above, impaired hepatic mTORC2 functionality reduces lifespan in male mice but not females [46]. Thus, there appears to be a bidirectional relationship between mTORC2 signalling and lifespan in male mice, such that both increases and decreases in male mouse lifespan are matched with changes in mTORC2 signalling, in opposite directions. At least in the case of reduced mTORC2 signalling, the links have been causally established. It would be of interest to understand whether enhancements in aspects of mTORC2 signalling can directly improve male health, or whether functional mTORC2 signalling is required for the lifespan response to male-specific longevity interventions.
3Factors underlying sex-specific responses to interventions
There are a wide range of factors that could cause males and females to respond differently to anti-aging interventions, and in the following section I discuss several of these potential causes in light of current evidence (Table 1). One straight forward factor that could lead to sexual dimorphism in lifespan extension relates to sex differences in drug metabolism and bioavailability (Fig. 1a). Genes controlling xenobiotic metabolism are some of the most sexually dimorphic in expression in mice [71], and some compounds may differ in their resulting bioactivity as a consequence. There is good evidence that equivalent rapamycin treatment in diet leads to higher circulating levels in female blood, which could explain the greater female lifespan extension. Study of metabolomic responses to 17-α estradiol in mice, a drug that extends lifespan in males only, shows that dietary administration of 17-α estradiol leads to a highly male-specific increase in another estrogen in the liver – estriol – which can be metabolised from estradiol [72]. Females do not show an elevation in this second estrogen in response to the same treatment. Estriol itself has established immunological and metabolic effects [73–75], and this apparent sex-specific conversion could contribute to sexual dimorphism in response to 17-α estradiol. While sex-specific drug metabolism may explain sex-specific responses to certain pharmacological compounds, it does not explain sex-specific responses to genetic manipulations, where drug metabolism, directly, does not play a role. Here, sexual dimorphism in aging responses may be linked to the importance of different pathways in the control of aging in either sex. To understand why this may be the case, it is worth considering the definition of males and females, and how theory predicts the sexes may differ in their aging rates in natural contexts.
3.1Why do the sexes differ in aging?
There are various theories attempting to explain the often large differences in lifespan occurring between males and females. Life-history theories consider differences in the ways males and females reproduce, and how differences in optimal reproductive rates may lead to differences in aging, assuming there is a trade-off between reproduction and survival [76]. A classic example is the idea that males – which by definition produce more gametes than females – may have been selected to “live fast and die young”, growing fast, investing in reproduction heavily early in life, but consequently having a shorter lifespan relative to females [77]. Other ideas center around genetic differences between the sexes, often taxon specific, which may constrain the lifespan of one sex irrespective of trade-offs with reproduction [78]. For example, mammalian sex determination is controlled by sex chromosomes, with the presence of single X and Y chromosomes causing development of males, while two X chromosomes leads to females. It has been hypothesized that having a single Y chromosome may be bad for aging, for one reason because a lack of recombination allows deleterious mutations to accumulate on the Y chromosome [79]. Alternatively, the presence of two X chromosomes could itself have female-specific impacts on aging. One possible way this could occur is through loss of X inactivation: in most cells, gene expression from the X chromosome occurs from one chromosome only, with the expression of most genes from the other strongly repressed (inactivated). However, there is evidence that during aging this repression can be lost, leading to changes in relative gene expression as a consequence of transcription from both chromosomes, a potential cause of disease [79]. Recent evidence suggests that sex chromosome differences can influence mortality in mice [80], although the causes for this remain to be elucidated.
3.2Sex-differences in response to anti-aging interventions: Reducing sex-specific disease susceptibility
Given the variety of potential causes for sex differences in survival, there are a variety of different ways that these factors could interact with anti-aging interventions to cause sex-specific life-extension. Selection on life-history, or genetic differences between the sexes, can lead to the occurrence of sex-specific causes of death, for example cancers. An intervention curing a type of cancer that kill males only, assuming this is fairly prevalent in the population, as seen in many lab animal strains [81], would lead to lifespan extension in this sex without effects in females (Fig. 1b). Conversely, an intervention may promote a disease in one sex only, limiting lifespan extension in that sex. Actions on reproductive systems may be particularly important in this context given their sex-specificity. It has been shown that rapamycin can cause testicular degeneration in male mice [82] and while unlikely to be the cause of sexual dimorphism in degree of lifespan extension, illustrates how a drug can influence pathology of a reproductive system present in one sex only.
In situations of sex-specific disease susceptibility, even though sex-specific lifespan extension occurs, other markers of aging could be unaffected in either sex, or would change in a sex-independent manner. Furthermore, we might expect a different consequence for males and females if the intervention was tested in a different strain, or a different species, which has a different susceptibility to that disease. One example of an intervention causing sex-specificity in this context might be over-expression of Sirt6, which increased male lifespan without effects on females [83]. The authors highlight that more than half of the measured male mice died presenting lung cancer, and that Sirt6 overexpression males survived longer with lung cancer than wild-type males, which could contribute to the pro-longevity effect in this sex. In this situation sex-differences in cancer susceptibility, caused by one of many different factors, could be the apparent driver of the sex-specific survival effect. Testing the intervention on a different genetic background would likely help explain the nature of these interactions: if Sirt6 over-expression causes male-specific life-extension on a background without prevalence of lung cancer, this would indicate potential sex-specific actions outside of the disease context.
It has also been suggested that sex-differences in lifespan extension may occur as a consequence of altered insulin signalling (Table 1), potentially alleviating or leading to mortality in one sex as a consequence of a range of factors stemming from metabolic dysfunction. Males and females differ in their production of hormones that maintain metabolic homeostasis, and often differ in insulin sensitivity and glucose homeostasis [84]. As eluded to above, several models of sex-specific lifespan extension concomitantly show sex-specific changes in glucose tolerance. The general observation from metabolism research, although there are exceptions, is that male mice have impaired glucose tolerance when compared to females [85–88]. Treatments that improve glucose tolerance may therefore provide male-specific survival benefits, potentially stemming from the myriad of mortality sources that can arise from impaired glucose handling and associated metabolic dysfunction [89]. Lifespan extension with the type 2 diabetes drug acarbose fits this model, increasing lifespan in male mice by ∼20% while increasing female survival by only ∼5% [14]. These sex-specific survival benefits are matched by sex-specific improvements in glucose homeostasis, with acarbose improving male glucose tolerance without effects in females [69]. Similar male-specific metabolic improvements were observed in mice treated with 17-α estradiol, and in both causes these improvements could be inhibited if males were castrated prior to treatment onset [69].
The dependence of drug responses on gonadal hormones is of particular interest, because it points to a sex-specific endocrine pathway from which these sex differences originate. The production of gonadal testosterone in males in the noted study suppressed glucose tolerance [69], relative to females or castrated males, and these pharmacological treatments at least partly rectify this difference. In some respects this fits with the lifespan observations in UM-HET3 mice, where under control conditions males have a shorter lifespan than females [64]. Male castration has been shown to extend lifespan in rodents [90, 91], and therefore part of lifespan extension in these contexts could be attributable to drug responses that counteract negative effects of testosterone on physiology linked to aging. Testosterone is a potent anabolic regulator, favouring growth over stress resistance [92], and is involved in the diversification of male and female life-histories, promoting male-specific growth, and development of male-specific reproductive traits [93]. Part of these actions in mammals occur as a consequence of testosterone’s effects on GH-pulsatility, which is programmed during development and also responds to adult sex-hormone exposure [94, 95]. Anabolic actions of testosterone in control of growth versus stress resistance might also explain why reducing IGF1 and mTOR signalling is less effective for extension of male-lifespan: testosterone could represent an additional pathway axis that constrains male survival, and therefore reducing IGF1 or mTOR signalling may have less of an effect, because male survival is constrained by this additional axis (Fig. 1c). The study of anabolic actions by testosterone also suggests testosterone can have mTORC1 dependent actions [96, 97] on hypertrophy. In hypothetical situations of mTORC1 dependent testosterone action, androgens may drive up mTOR signalling, making it harder for mTOR signalling to be repressed in particular tissue types (Fig. 1d).
3.3Sex-differences in activation of pathways controlling aging
Males and females commonly show differences in pathway activation of GH-IGF1 and mTOR signalling, although this can vary strongly by tissue-type and age [94, 98]. Such differences in pathway activation may contribute to sexual dimorphism in aging, and may lead to sex differences in response to interventions through various postulated routes. The observation that lifespan extension with both reduced IGF1 and mTORC1 signalling is typically greater in females than males points to a sex-specific role of these pathways in control of aging. Taking as an example manipulations that reduce IGF1 signalling, assuming signalling intensity is reduced proportionally in both sexes, if lifespan extension occurs more in one sex, it could indicate greater lifespan dependence on that pathway for that sex. If females die of factors related to IGF1 signalling, but males do not, then no matter how much IGF1 signalling is reduced in males lifespan extension will not be achieved. An alternative explanation is that baseline levels of IGF1 signalling may differ between the sexes, and therefore reducing IGF1 signalling may lead sex-differences in the threshold at which reduced IGF1 signalling extends lifespan. Xu et al. [31] provided an interesting analysis of strain differences in lifespan extension with heterozygous loss of the IGF1 receptor. They show that the strain showing the greatest lifespan extension has the highest baseline levels circulating IGF1, and higher IGF1 signalling in several tissues, which they suggest could lead to this strain responding more greatly to the IGF1 manipulation. Analogous effects could occur in relation to sex. In the case of mTOR signalling, females have been shown to have higher levels of mTORC1 signalling at young ages than males [98], and it has been suggested that this could contribute to the greater lifepan extension observed in females treated with rapamycin. Unfortunately we still have little understanding of when and where IGF1/mTOR signalling is important for control of aging, limiting our ability to pinpoint where variation in signalling intensity may translate into variation in aging and lifespan responses.
3.4Understanding sex-differences in treatment responses will improve understanding of aging
At present these models for why sex-specific lifespan extension occurs are largely hypothetical and require experimental validation or refutation. The figure provided illustrates several of potentially many different causes for sex differences in lifespan extension. However, if we can tease apart the underlying driving factors it will likely provide substantial new insight into pathways that control the aging process, and particularly how these give rise to the substantial sexual dimorphism seen in lifespan in most species. Given the major role of gonadal hormones in control of sexual dimorphism in reproduction, body form and gene expression, it is surprisingly the role of these hormones in control of sexually dimorphic lifespan responses has been so little explored. For the pharmacological treatments acarbose and 17-α estradiol, there is evidence that some of the health benefits in males are dependent on the presence of intact gonads [69, 99]. An earlier study assessing sexually-dimorphic responses to insulin-receptor manipulation for protection against oxidative stress showed a role of estrogens and female ovarian hormones in mediating sex-specific responses in terms of stress resistance [100]. Estrogens are thought to play a beneficial role in the uptake and control of circulating glucose [101], particularly in metabolic disease states [102–104], and influence susceptibility to various cancers [84], highlighting a likely important role of female-specific hormones for mortality sources in this sex. Elucidating the location of steroid receptors through which these gonadal effects operate may provide good clues to where and when sex specific lifespan responses manifest and originate. It would also be of great interest to understand whether sex-specific responses to IGF1, IRS, mTORC1 or mTORC2 manipulation are dependent on gonadal hormones. Sex differences in lifespan responses to these manipulations have been repeatedly documented, but as yet there is little currently published data testing whether these responses are linked to gonadal hormone action. Understanding the ways that testosterone and estrogens feed into these pathways, and interact with manipulations such as mTOR signalling to influence lifespan, could inform us of additional inputs that act through these established signals to cause sex-specific aging.
The role of genetic differences between the sexes may also be important in causing these sex-specific responses. In fruit flies, where chromosomal differences between the sexes are more important for control of sexual dimorphism, chromosomal arrangement in the gut is important in lifespan responses to dietary restriction [50]. The increasing recognition that sex-chromosome composition can influence a range of metabolic endpoints in mice [105], and have direct effects on mortality [80], highlights the potential importance of sex-chromosomes in mammalian aging, which could occur as a consequence of Y or X chromosome effects. Additional genetic asymmetries between the sexes could also play roles. For example, inheritance of mitochondria almost entirely through the maternal line can have deleterious effects for male aging [106] — if these effects are mitigated by a specific intervention, this could lead to sex-specific lifespan responses.
4Conclusion
Ultimately, sex-differences in response to anti-aging interventions will be particularly important if they manifest in humans. There is much current discussion about the translation of anti-aging interventions for improving health during aging in humans [107], with particular reference to rapamycin and mTORC1 inhibitors [5], which in mice have sex-specific anti-aging effects. If we knew more about the causes for sex-specific responses to mTOR inhibition in animals, we could make inferences about the potential for sex-specific effects to occur in humans, or develop ways to harness or mitigate these effects. Extreme care will be required to ensure that sex-specific responses do not intensify the already large disparities in frailty, disease and survival seen between men and women in human populations [15]. A variety of models are now available for elucidating the underlying causes for a documented sex-specific response in mice or Drosophila; applying these systems may illuminate causes for sex-specific responses to anti-aging interventions, in addition to providing insight into reasons for why males and females often age in different ways.
Acknowledgments
I thank Michael Stout, Dudley Lamming and two anonymous referees for helpful comments on an earlier version of this manuscript.
References
[1] | Richardson A et al., Measures of healthspan as indices of aging in mice—a recommendation. Journals of Gerontology Series A: Biomedical Sciences and Medical Sciences. (2015) ;71: (4):427–430. |
[2] | Colman RJ et al., Caloric Restriction Delays Disease Onset and Mortality in Rhesus Monkeys. Science. 325. (2009) ;325: (5937):201–204. |
[3] | Kraig E et al., A randomized control trial to establish the feasibility and safety of rapamycin treatment in an older human cohort: Immunological, physical performance, and cognitive effects. Experimental Gerontology. (2018) ;105: :53–69. |
[4] | Mannick JB et al., TORC1 inhibition enhances immune function and reduces infections in the elderly. Science Translational Medicine. (2018) ;10: (449):eaaq1564. |
[5] | Johnson SC , Rabinovitch PS , Kaeberlein M . mTOR is a key modulator of ageing and age-related disease. Nature. (2013) ;493: (7432):338–345. |
[6] | Garratt M , Nakagawa SN , Simons MJ . Comparative idiosyncrasies in life extension by reduced mTOR signalling and its distinctiveness from dietary restriction. Aging Cell. (2016) ;15: (4):737–43. |
[7] | Liao C-Y et al., Genetic variation in the murine lifespan response to dietary restriction: from life extension to life shortening. Aging Cell. (2010) ;9: (1):92–95. |
[8] | Miller H et al., Genetic interaction with temperature is an important determinant of nematode longevity. Aging Cell. (2017) ;16: (6):1425–1429. |
[9] | Bonkowski MS et al., Targeted disruption of growth hormone receptor interferes with the beneficial actions of calorie restriction. Proceedings of the National Academy of Sciences of the United States of America. (2006) ;103: (20):7901–7905. |
[10] | Austad S , Bartke A . Sex Differences in Longevity and in Responses to Anti-Aging Interventions: A Mini-Review. Gerontology. (2015) )–10.1159/000381472. |
[11] | Miller RA et al., Rapamycin, But Not Resveratrol or Simvastatin, Extends Life Span of Genetically Heterogeneous Mice. The Journals of Gerontology Series A: Biological Sciences and Medical Sciences. (2011) ;66: (2):191–201. |
[12] | Zhang Y et al., Rapamycin Extends Life and Health in C57BL/6 Mice. The Journals of Gerontology Series A: Biological Sciences and Medical Sciences. (2014) ;69A: (2):119–130. |
[13] | Lamming DW et al., Rapamycin-Induced Insulin Resistance Is Mediated by mTORC2 Loss and Uncoupled from Longevity. Science. (2012) ;335: (6076):1638–1643. |
[14] | Harrison DE et al., Acarbose, 17-α-estradiol, and nordihydroguaiaretic acid extend mouse lifespan preferentially in males. Aging Cell. (2014) ;13: (2):273–282. |
[15] | Austad SN , Fischer KE . Sex Differences in Lifespan. Cell Metab. (2016) ;23: (6):1022–1033. |
[16] | Promislow DE et al., Below-threshold mortality: implications for studies in evolution, ecology and demography. Journal of Evolutionary Biology. (1999) ;12: (2):314–328. |
[17] | Nieuwenhuis S , Forstmann BU , Wagenmakers E-J . Erroneous analyses of interactions in neuroscience: A problem of significance. Nature Neuroscience. (2011) ;14: :1105. |
[18] | Kleinbaum DG , Klein M Kaplan-Meier Survival Curves and the Log-Rank Test, in Survival Analysis: A Self-Learning Text, Third Edition, Kleinbaum D.G. and Klein M. , Editors. (2012) , Springer New York: New York, NY. pp. 55–96. |
[19] | Ladiges W et al., Lifespan extension in genetically modified mice. Aging Cell. (2009) ;8: (4):346–352. |
[20] | Lee Johnson L , Shih JH . Chapter 23 – An Introduction to Survival Analysis, in Principles and Practice of Clinical Research (Third Edition), Gallin J.I. and Ognibene F.P. , Editors. (2012) , Academic Press: Boston. pp. 285–293. |
[21] | Zhu C-T , Ingelmo P , Rand DM . G×G×E for Lifespan in Drosophila: Mitochondrial, Nuclear, and Dietary Interactions that Modify Longevity. PLOS Genetics. (2014) ;10: (5):e1004354. |
[22] | Bartke A et al., Extending the lifespan of long-lived mice. Nature. (2001) ;414: (6862):412. |
[23] | Brown-Borg HM et al., Dwarf mice and the ageing process. Nature. (1996) ;384: (6604):33–33. |
[24] | Flurkey K , Papaconstantinou J , Harrison DE . The Snell dwarf mutation Pit1(dw) can increase life span in mice. Mech Ageing Dev. (2002) ;123: (2-3): 121–30. |
[25] | Bartke A , Sun LY , Longo V . Somatotropic signaling: trade-offs between growth, reproductive development, and longevity. Physiol Rev. (2013) ;93: (2):571–98. |
[26] | Garratt M , Nakagawa S , Simons MJP . Life-span Extension With Reduced Somatotrophic Signaling: Moderation of Aging Effect by Signal Type, Sex, and Experimental Cohort. J Gerontol A Biol Sci Med Sci.. (2017) ;72: (12):1620–1626. |
[27] | Coschigano KT et al., Deletion, but not antagonism, of the mouse growth hormone receptor results in severely decreased body weights, insulin, and insulin-like growth factor I levels and increased life span. Endocrinology. (2003) ;144: (9):3799–810. |
[28] | Tatar M , Bartke A , Antebi A . The endocrine regulation of aging by insulin-like signals. Science. (2003) ;299: (5611):1346–1351. |
[29] | Bokov AF et al., Does reduced IGF-1R signaling in Igf1r+/– mice alter aging? PLoS One. (2011) ;6: (11):e26891. |
[30] | Holzenberger M et al., IGF-1 receptor regulates lifespan and resistance to oxidative stress in mice. Nature. (2003) ;421: (6919):182–187. |
[31] | Xu J et al., Longevity effect of IGF-1R(+/–) mutation depends on genetic background-specific receptor activation. Aging Cell. (2014) ;13: (1):19–28. |
[32] | Svensson J et al., Liver-derived IGF-I regulates mean life span in mice. PLoS One. (2011) ;6: (7):e22640. |
[33] | Lorenzini A et al., Mice producing reduced levels of insulin-like growth factor type 1 display an increase in maximum, but not mean, life span. J Gerontol A Biol Sci Med Sci. (2014) ;69: (4):410–9. |
[34] | Conover CA , Bale LK . Loss of pregnancy-associated plasma protein A extends lifespan in mice. Aging Cell. (2007) ;6: (5):727–9. |
[35] | Mao K et al., Late-life targeting of the IGF-1 receptor improves healthspan and lifespan in female mice. Nat Commun. (2018) ;9: (1):2394. |
[36] | Selman C et al., Evidence for lifespan extension and delayed age-related biomarkers in insulin receptor substrate 1 null mice. Faseb j. (2008) ;22: (3):807–18. |
[37] | Nelson JF et al., Probing the relationship between insulin sensitivity and longevity using genetically modified mice. J Gerontol A Biol Sci Med Sci. (2012) ;67: (12):1332–8. |
[38] | Udy GB et al., Requirement of STAT5b for sexual dimorphism of body growth rates and liver gene expression. Proceedings of the National Academy of Sciences. (1997) ;94: (14):7239–7244. |
[39] | Clodfelter KH et al., Sex-dependent liver gene expression is extensive and largely dependent upon signal transducer and activator of transcription 5b (STAT5b): STAT5b-dependent activation of male genes and repression of female genes revealed by microarray analysis. Mol Endocrinol. (2006) ;20: (6):1333–51. |
[40] | Arum O et al., The growth hormone receptor gene-disrupted mouse fails to respond to an intermittent fasting diet. Aging Cell. (2009) ;8: (6):756–760. |
[41] | Li X et al., Direct and indirect effects of growth hormone receptor ablation on liver expression of xenobiotic metabolizing genes. American Journal of Physiology-Endocrinology and Metabolism. (2013) ;305: (8):E942-E950. |
[42] | Laplante M , Sabatini DM . mTOR signaling at a glance. Journal of Cell Science. (2009) ;122: (20):3589–3594. |
[43] | Kennedy BK , Lamming DW . The Mechanistic Target of Rapamycin: The Grand ConducTOR of Metabolism and Aging. Cell Metab. (2016) ;23: (6):990–1003. |
[44] | Robida-Stubbs S et al., TOR Signaling and Rapamycin Influence Longevity by Regulating SKN-1/Nrf and DAF-16/FoxO. Cell Metabolism. (2012) ;15: (5):713–724. |
[45] | Soukas AA et al., Rictor/TORC2 regulates fat metabolism, feeding, growth, and life span in Caenorhabditis elegans. Genes & Development. (2009) ;23: (4):496–511. |
[46] | Lamming DW et al., Depletion of Rictor, an essential protein component of mTORC2, decreases male lifespan. Aging Cell. (2014) ;13: (5):911–7. |
[47] | Harrison DE et al., Rapamycin fed late in life extends lifespan in genetically heterogeneous mice. Nature. (2009) ;460: (7253):392–395. |
[48] | Miller RA et al., Rapamycin-mediated lifespan increase in mice is dose and sex dependent and metabolically distinct from dietary restriction. Aging Cell. (2014) :10.1111/acel.12194. |
[49] | Selman C et al., Ribosomal Protein S6 Kinase 1 Signaling Regulates Mammalian Life Span. Science. (2009) ;326: (5949):140–144. |
[50] | Regan JC et al., Sex difference in pathology of the ageing gut mediates the greater response of female lifespan to dietary restriction. eLife. (2016) ;5: :e10956. |
[51] | Harrison B et al., Effect of rapamycin on lifespan in Drosophila. Geriatrics & Gerontology International. (2010) ;10: (1):110–112. |
[52] | Villa-Cuesta E , Fan F , Rand DM . Rapamycin reduces Drosophila longevity under low nutrition. Journal of Pharmacy. (2014) ;8: :43–51. |
[53] | Bjedov I et al., Mechanisms of Life Span Extension by Rapamycin in the Fruit Fly Drosophila melanogaster. Cell Metabolism. (2010) ;11: (1):35–46. |
[54] | Danilov A et al., Selective anticancer agents suppress aging in Drosophila. Oncotarget. (2013) ;4: (9):1507–26. |
[55] | Moskalev AA , Shaposhnikov MV . Pharmacological inhibition of phosphoinositide 3 and TOR kinases improves survival of Drosophila melanogaster. Rejuvenation Res. (2010) ;13: (2-3):246–7. |
[56] | Sun X et al., Nutrient-dependent requirement for SOD1 in lifespan extension by protein restriction in Drosophila melanogaster. Aging Cell. (2012) ;11: (5):783–93. |
[57] | Withers DJ et al., Disruption of IRS-2 causes type 2 diabetes in mice. Nature. (1998) ;391: (6670):900–4. |
[58] | Kulkarni RN et al., Impact of Genetic Background on Development of Hyperinsulinemia and Diabetes in Insulin Receptor/Insulin Receptor Substrate-1 Double Heterozygous Mice. Diabetes. (2003) ;52: (6):1528–1534. |
[59] | Heilbronn LK , Ravussin E . Calorie restriction and aging: review of the literature and implications for studies in humans. The American Journal of Clinical Nutrition. (2003) ;78: (3):361–369. |
[60] | Nakagawa S et al., Comparative and meta-analytic insights into life extension via dietary restriction. Aging Cell. (2012) ;11: (3):401–409. |
[61] | Miller RA et al., An Aging Interventions Testing Program: study design and interim report. Aging Cell. (2007) ;6: (4):565–75. |
[62] | Harrison DE. et al., Acarbose improves health and lifespan in aging HET3 mice. Aging Cell. 0(0), e12898. |
[63] | Strong R et al., Longer lifespan in male mice treated with a weakly estrogenic agonist, an antioxidant, an α-glucosidase inhibitor or a Nrf2-inducer. Aging Cell. (2016) ;15: :872–884. |
[64] | Strong R et al., Nordihydroguaiaretic acid and aspirin increase lifespan of genetically heterogeneous male mice. Aging Cell. (2008) ;7: (5):641–650. |
[65] | Kim SY et al., Regulation of pro-inflammatory responses by lipoxygenases via intracellular reactive oxygen species in vitro and in vivo. Experimental & Molecular Medicine. (2008) ;40: :461. |
[66] | Derosa G et al., Acarbose actions on insulin resistance and inflammatory parameters during an oral fat load. Eur J Pharmacol. (2011) ;651: (1-3):240–50. |
[67] | Stout MB et al., 17α-Estradiol alleviates age-related metabolic and inflammatory dysfunction in male mice without inducing feminization. The Journals of Gerontology Series A: Biological Sciences and Medical Sciences. (2016) :glv309. |
[68] | Balfour JA , McTavish D . Acarbose. An update of its pharmacology and therapeutic use in diabetes mellitus. Drugs. (1993) ;46: (6):1025–54. |
[69] | Garratt M et al., Sex differences in lifespan extension with acarbose and 17-alpha estradiol: gonadal hormones underlie male-specific improvements in glucose tolerance and mTORC2 signaling. Aging Cell. (2017) ;16: (6):1256–1266. |
[70] | Garratt M et al., 17-alpha estradiol ameliorates age-associated sarcopenia and improves late-life physical function in male mice but not in females or castrated males. Aging Cell. (2019) ;18: (2):e12920. |
[71] | Waxman DJ , Holloway MG . Sex differences in the expression of hepatic drug metabolizing enzymes. Mol Pharmacol. (2009) ;76: (2):215–28. |
[72] | Garratt M et al., Male lifespan extension with 17-α estradiol is linked to a sex-specific metabolomic response modulated by gonadal hormones in mice. Aging Cell. (2018) ;17: (4):e12786. |
[73] | Kim S et al., Estriol ameliorates autoimmune demyelinating disease: implications for multiple sclerosis. Neurology. (1999) ;52: (6):1230–8. |
[74] | Vermillion MS et al., Estriol Reduces Pulmonary Immune Cell Recruitment and Inflammation to Protect Female Mice From Severe Influenza. Endocrinology. (2018) ;159: (9):3306–3320. |
[75] | Yamabe N et al., Estriol blunts postprandial blood glucose rise in male rats through regulating intestinal glucose transporters. American Journal of Physiology – Endocrinology And Metabolism. (2015) ;308: (5):E370-E379. |
[76] | Brooks RC , Garratt MG . Life history evolution, reproduction, and the origins of sex-dependent aging and longevity. Annals of the New York Academy of Sciences. (2017) ;1389: (1):92–107. |
[77] | Bonduriansky R et al., Sexual selection, sexual conflict and the evolution of ageing and life span. Functional Ecology. (2008) ;22: (3):443–453. |
[78] | Maklakov AA , Lummaa V . Evolution of sex differences in lifespan and aging: Causes and constraints. Bioessays. (2013) ;35: (8):717–724. |
[79] | Marais GAB et al., Sex gap in aging and longevity: can sex chromosomes play a role? Biology of Sex Differences. (2018) ;9: (1):33–33. |
[80] | Davis EJ , Lobach I , Dubal DB . Female XX sex chromosomes increase survival and extend lifespan in aging mice. Aging Cell. (2019) ;18: (1):e12871. |
[81] | Green EL Biology of the Laboratory Mouse, New York: Dover Publication ((1966) ). |
[82] | Wilkinson JE et al., Rapamycin slows aging in mice. Aging Cell. (2012) ;11: (4):675–682. |
[83] | Kanfi Y et al., The sirtuin SIRT6 regulates lifespan in male mice. Nature. (2012) ;483: (7388):218–221. |
[84] | Legato MJ Principles of gender-specific medicine 2010, London, UK: Elsevier ((2010) ). |
[85] | Macotela Y et al., Sex and depot differences in adipocyte insulin sensitivity and glucose metabolism. Diabetes. (2009) ;58: (4):803–12. |
[86] | Bonaventura MM et al., Sex differences in insulin resistance in GABAB1 knockout mice. Life Sci. (2013) ;92: (3):175–82. |
[87] | Sadagurski M et al., Long-lived crowded-litter mice exhibit lasting effects on insulin sensitivity and energy homeostasis. Am J Physiol Endocrinol Metab. (2014) ;306: (11):E1305–14. |
[88] | Shivaswamy V et al., Tacrolimus and sirolimus have distinct effects on insulin signaling in male and female rats. Transl Res. (2014) ;163: (3):221–31. |
[89] | Zambon S et al., Metabolic syndrome and all-cause and cardiovascular mortality in an Italian elderly population: the Progetto Veneto Anziani (Pro.V.A.) Study. Diabetes Care. (2009) ;32: (1):153–159. |
[90] | Asdell SA et al., The effects of sex steroid hormones upon longevity in rats. J Reprod Fertil. (1967) ;14: (1):113–20. |
[91] | Muehlbock O . Factors influencing the life-span of inbred mice. Gerontologia. (1959) ;3: :177–83. |
[92] | Rossetti ML , Steiner JL , Gordon BS . Androgen-mediated regulation of skeletal muscle protein balance. Mol Cell Endocrinol. (2017) ;447: :35–44. |
[93] | Hau M . Regulation of male traits by testosterone: implications for the evolution of vertebrate life histories. Bioessays. (2007) ;29: (2):133–144. |
[94] | Meinhardt UJ , Ho KKY . Modulation of growth hormone action by sex steroids. Clinical Endocrinology. (2006) ;65: (4):413–422. |
[95] | Rinn JL , Snyder M . Sexual dimorphism in mammalian gene expression. Trends Genet. (2005) ;21: (5):298–305. |
[96] | Basualto-Alarcon C et al., Testosterone signals through mTOR and androgen receptor to induce muscle hypertrophy. Med Sci Sports Exerc. (2013) ;45: (9):1712–20. |
[97] | Altamirano F et al., Testosterone induces cardiomyocyte hypertrophy through mammalian target of rapamycin complex 1 pathway. J Endocrinol. (2009) ;202: (2):299–307. |
[98] | Baar EL et al., Sex- and tissue-specific changes in mTOR signaling with age in C57BL/6J mice. Aging Cell. (2016) ;15: (1):155–166. |
[99] | Garratt M. et al., 17-α estradiol ameliorates age-associated sarcopenia and improves late-life physical function in male mice but not in females or castrated males. Aging Cell. 0(0), e12920. |
[100] | Baba T et al., Estrogen, insulin, and dietary signals cooperatively regulate longevity signals to enhance resistance to oxidative stress in mice. J Biol Chem. (2005) ;280: (16):16417–26. |
[101] | Geer EB , Shen W . Gender Differences in Insulin Resistance, Body Composition, and Energy Balance. Gender Medicine. (2009) ;6: (Suppl 1):60–75. |
[102] | Stubbins RE et al., Estrogen modulates abdominal adiposity and protects female mice from obesity and impaired glucose tolerance. Eur J Nutr. (2012) ;51: (7):861–70. |
[103] | Stubbins RE et al., Oestrogen alters adipocyte biology and protects female mice from adipocyte inflammation and insulin resistance. Diabetes Obes Metab. (2012) ;14: (1):58–66. |
[104] | Zhu L et al., Estrogen treatment after ovariectomy protects against fatty liver and may improve pathway-selective insulin resistance. Diabetes. (2013) ;62: (2):424–34. |
[105] | Chen X , Arnold AP , Reue K . Metabolic impact of sex chromosomes AU – Link, Jenny C. Adipocyte. (2013) ;2: (2):74–79. |
[106] | Camus MF , Clancy DJ , Dowling DK . Mitochondria, maternal inheritance, and male aging. Current Biology. (2012) ;22: (18):1717–1721. |
[107] | Longo VD et al., Interventions to Slow Aging in Humans: Are We Ready? Aging Cell. (2015) ;14: (4):497–510. |
[108] | Becker JB et al., Strategies and methods for research on sex differences in brain and behavior. Endocrinology. (2005) ;146: (4):1650–1673. |
[109] | Flintham Ewan O et al., Interactions between the sexual identity of the nervous system and the social environment mediate lifespan in Drosophila melanogaster. Proceedings of the Royal Society B: Biological Sciences. (2018) ;285: (1892):20181450. |
[110] | Lamming DW . Diminished mTOR signaling: a common mode of action for endocrine longevity factors. SpringerPlus. (2014) ;3: :735–735. |
[111] | Regan JC , Partridge L . Gender and longevity: Why do men die earlier than women? Comparative and experimental evidence. Best Practice & Research Clinical Endocrinology & Metabolism. (2013) ;27: (4):467–479. |