Emerging Antibody-Drug Conjugate Therapies and Targets for Metastatic Renal Cell Carcinoma
Abstract
Background:
Approximately 30% of renal cell carcinoma (RCC) cases present with de novo metastatic disease, while 20% to 30% of those with localized disease will develop metastases following surgical resection. Various drug classes have been investigated to treat RCC, including cytokine-based therapies, small molecule Vascular Endothelial Growth Factor (VEGF) tyrosine kinase inhibitors (TKIs) and antibody-based therapies. Up to 58% of patients fail to respond to primary immune checkpoint inhibitor (ICI) therapy, and nearly all initial responders experience disease progression due to the development of secondary resistance. Consequently, novel treatment options are being investigated.
Objective:
Review the rapidly evolving ADC therapeutic landscape in metastatic RCC including recent trials, emerging ADCs targets, and future directions for ADCs in the treatment of advanced RCC.
Methods:
Literature review using the MEDLINE database on important trials and presentations from the American Society of Clinical Oncology (ASCO), and the European Society for Medical Oncology (ESMO) conferences. Key words used included “renal cell carcinoma,” “RCC,” “metastatic RCC,” “advanced RCC,” “antibody-based therapies,” “immunotherapy,” “clinical trials,” and “emerging drugs.” Specifically for review of ADCs in RCC, the following search string was used with additional review of bibliographies from retrieved papers: “((antibody drug conjugate) OR (antibody-dependent cellular cytotoxicity) OR (chimeric antigen receptor)) AND ((kidney cancer) OR (renal cell carcinoma))”.
Results:
Several promising targets including MMP14, EGFR, MCT4, CA9, MET, CDH13, B7-H3, and PSMA were identified with relevant preclinical and clinical studies reviewed.
Conclusions:
While ADCs therapeutics have not shown benefit to date for renal cell carcinoma, there are ample promising candidates and targets for future research.
INTRODUCTION
Renal cell carcinoma (RCC) ranks among the top ten types of cancer in the United States with 76,080 new cases and 13,780 deaths recorded in 2021. This equates to a lifetime risk of developing invasive RCC of approximately 1 in 46 for males and 1 in 80 for females [1]. Of new cases, de novo metastatic disease comprises nearly 30% of RCC patients and another 20% of patients with localized disease develop disease recurrence following surgical treatment [2].
The research landscape for treating advanced RCC has continually explored new drug classes, ranging from small molecule-based to antibody-based therapeutics. Recent clinical trials have demonstrated improved outcomes using immune checkpoint inhibitors (ICIs) in metastatic RCC. As a result, the American Society of Clinical Oncology (ASCO) and the European Society for Medical Oncology (ESMO) have updated their recommendation for first-line treatment of metastatic clear cell RCC (ccRCC) from monotherapy with vascular endothelial growth factor receptor (VEGF) tyrosine kinase inhibitors (TKIs), like sunitinib, to combination therapy involving a single ICI and TKI or dual-ICI therapy depending on a patient’s risk stratification [3, 4]. Only patients with coexisting medical problems that preclude combination treatment are recommended to receive monotherapy with either a VEGF TKI or an ICI [5]. Despite improved response rates to ICIs, up to 58% of patients do not respond to first-line therapy and eventual disease progression reaches 100% due to the development of secondary resistance [6]. In addition, three other issues that have been identified in relation with ICIs relate to their increase in cost, side effects, and lack of a prognostic biomarker to select between ICI therapies [7–9].
In response to the limitation of ICI therapy, antibody-drug conjugates (ADCs) are a new class of drugs that are being explored. ADCs use an antibody to specifically target proteins overexpressed on the surface of tumor cells and deliver linked cytotoxic drug payloads. As of December 2021, 14 ADCs have been approved worldwide for the treatment of hematological malignancies and solid tumors including breast cancer and urothelial cancer [10]. Despite this promise, ADCs to date have not been shown to be particularly effective in the treatment of RCC.
The objective of our review is to provide a clinically relevant overview of completed and ongoing clinical trials of ADCs for metastatic RCC, including potential future ADC candidates and targets for metastatic RCC treatment. In addition, we review future directions in ADC research to address clinical challenges.
ADC overview
Antibody drug conjugates consist of three distinct components: an antibody, a linker, and a cytotoxic payload. There are several different strategies to select a protein for the antibody to target including through the use of large expression databases like GTEx [11]. According to a review by Chia, the most common linker-toxin used in approved ADCs today is vedotin (patented in 2003 by Seattle Genetics), while valine-citrulline-based linkers are also popularly used [12]. While the cytotoxic payload is the mechanism of ADC toxicity, the specificity of the targeting antibody and stability of the linker is just as important to prevent systemic distribution of the payload. Akin to traditional chemotherapy agents, off-target cytotoxic payloads tend to most affect rapidly dividing tissues, like those in the GI tract and myeloid cells. However, different payloads have been associated with key side effects. For example, monomethyl auristatin F (MMAF), the payload in the drug Enfortumab vedotin (EV), is specifically associated with ocular and neurologic toxicity in addition to more general side effects [13].
Regarding ADCs used today in clinical practice, Gogia et al reviewed eleven FDA-approved therapies and the over 100 different compounds in various stages of clinical development. The first ADC to receive FDA accelerated approval in 2000 was Gemtuzumab ozogamicin, which was temporarily withdrawn in 2010 due to safety concerns based on a randomized clinical trial and then re-approved in 2017, but the first ADC for solid tumors was Ado-Trastuzumab Emtansine (TDM1) approved in 2013 for metastatic HER2-positive breast cancer after taxane and trastuzumab therapy. Later in 2019 this approval was extended for single agent adjuvant therapy for patients with residual breast cancer disease after neoadjuvant trastuzumab therapy based on the phase III KATHERINE trial [14].
In the field of Urologic Oncology, EV, a Nectin-4 targeting compound with a vedotin linker delivering a monomethyl auristatin E (MMAE) payload, was approved in 2019 for locally advanced or metastatic urothelial carcinoma after PD-1 or PD-L1 inhibitor and platinum-based chemotherapy. This initial approval was based on results from a phase II trial demonstrating an objective response rate of 44% (12% complete response rate and 32% partial response rate) with median response duration of 7.6 months [15]. EV’s approval was extended in 2021 to be used in cisplatin-ineligible patients treated with at least one other prior therapy. This approval was further extended in April 2023 for use of first-line combination EV and pembrolizumab for cisplatin-ineligible patients. Results from distinct cohorts in two trials, the combined dose-escalation and phase 1b/2EV-103/Keynote-869 trial, demonstrated objective response rates up to 68% (95% CI, 59–76%) [16, 17]. Results of the phase 3 EV-302 trial were recently released with impressive overall survival improvements [18]. Additionally, Sacituzumab govitecan is an ADC previously approved in 2020 for metastatic triple negative breast cancer that was approved in 2021 for metastatic urothelial cancer based on results from the phase II TROPHY trial, which demonstrated an objective response rate of 27.7% and median PFS of 5.4 months (95% CI, 3.5 to 7.2 months) [19]. Other approved ADCs for solid tumors include Mirvetuximab soravtansine-gynx for advanced ovarian cancer, Tisotumab vedotin-tftv for advanced cervical cancer, and Fam-Trastuzumab deruxtecan-nxki for advanced HER2-positive gastric cancer. These different therapies highlight the versatility and promise of ADC-based therapy [20].
MATERIALS AND METHODS
Literature was searched in the MEDLINE database on important trials and presentations from the ASCO and ESMO conferences. Our search was restricted to English language sources that were published since 2010. Key words used included: “renal cell carcinoma,” “RCC,” “metastatic RCC,” “advanced RCC,” “antibody-based therapies,” “immunotherapy,” “clinical trials,” and “emerging drugs.” Specifically for review of ADCs in RCC, the following search string was used with additional review of bibliographies from retrieved papers: “((antibody drug conjugate) OR (antibody-dependent cellular cytotoxicity) OR (chimeric antigen receptor)) AND ((kidney cancer) OR (renal cell carcinoma))”.
To identify promising ADC therapeutic targets, we compiled existing proteogenomic datasets for cc RCC [21, 22]. We generated volcano plots from normalized protein counts in both datasets to display differentially expressed proteins (DEPs) in tumors vs adjacent tissues. We applied thresholds of fold change≥2 and adjusted p value≤0.05 to classify significant DEPs. We used our in-house surfaceome database to select proteins with confirmed subcellular location at the cell surface [23]. The DEPs enriched in ccRCC from each study were compared using a Venn diagram to highlight the overlap of proteins identified in tumors. Finally, the shared surface proteins were ranked based on their maximal protein abundance in normal tissues using the publicly available GTEX proteome to highlight putative ADC targets that have minimal expression in normal tissues [24].
ADC THERAPIES FOR METASTATIC RCC
Unsuccessful ADC RCC Therapies
No ADCs drugs have shown effectiveness to date in treating RCC (Table 1). Toxicity has been observed to be a major limitation in ADC trials for RCC. One example is CDX-014, an ADC targeting the T cell Immunoglobulin Mucin-1 protein with a valine-citrulline linker delivering a MMAE payload. The T cell Immunoglobulin Mucin-1 protein is expressed in renal ischemia and can be a result of RCC pathogenesis [25, 26]. The phase I first-in-human study showed that of the 16 studied patients that 5 had sustained stable disease. However, the drug was significantly limited by toxic side-effects, including 3 episodes of hyperglycemia, 1 episode of urosepsis, and 1 episode of multiple organ failure resulting in death [27].
Table 1
Failed ADC trials to treat metastatic RCC to date
ADC | Target | Linker | Cytotoxic agent | Trial Phase | Trial concern |
CDX-014 | T-cell Immunoglobulin Mucin-1 protein | Valine-citrulline | MMAE | 1 | Toxicity (of 16 patients, 3 episodes of hyperglycemia, 1 episode of urosepsis, and one episode of multiple organ failure resulting in death) |
SGN-CD70A | CD-70 | Peptide-based | Pyrrolobenzodiazepine dimer | 1 | Toxicity (15/18 patients at least Grade 3 TEAE) |
HKT288 | CDH6 | Sulfur-based | DM4-based | 1 | Toxicity (2/9 patients with significant neurotoxicity) |
AGS-16C3F | ENPP3 | Maleimidocaproyl | MMAF | 2 | Lack of PFS (3 of 67 patients remained in treatment group) |
The ADC drug SGN-CD70A was also limited by its toxicity profile. It was investigated to treat RCC in a phase 1 first-in-human study by Pal et al. The authors report that the target protein CD-70 was overexpressed in 72% of a 283 patient RCC series, leading to an interest in targeting the protein with SGN-CD70A, consisting of a peptide-based linker and the DNA cross-linking Pyrrolobenzodiazepine dimer compound. However, of the 18 patients with metastatic RCC in the study, 15 (83%) were found to have at least a Grade 3 treatment emergent adverse event (TEAE), most commonly thrombocytopenia, anemia, and fatigue. In addition to the high rate of TEAEs, the clinical benefit of SGN-CD70A was modest with 1 patient having a partial response and 13 having stable disease over the course of the study. The authors discuss how the TEAEs found in relation to SGN-CD70A were unexpected given that the protein is not expressed in megakaryocytes and that CD70 itself may be involved in platelet destruction [28], potentially signifying that the TEAEs are related to ongoing primary metastatic RCC disease [29].
HKT288, a CDH6-targeting sulfur-based linker delivering the tubulin binder DM4 compound was investigated after the cell-cell adhesion molecule cadherin-6 (CDH6) was discovered to be enriched in ovarian and renal cancers from genome-wide differential gene expression analysis. The ADC induced specific and durable tumor regression in ovarian and renal cancer patient-derived xenograft (PDX) models [30]. However, the preclinical toxicology did not predict the neurotoxicity observed in patients in a phase 1 study of HKT288. Of the 9 patients enrolled in this study, 2 patients developed neurologic adverse effects, including seizures, aphasia, and encephalopathy which resulted in the premature termination of the study [31].
While toxicity has been a key limitation for ADCs in clinical trial, efficacy concerns have also been shown in a phase II trials of an ADC targeting the cell-surface marker ectonucleotide pyrophosphatase/phosphodiesterase 3 (ENPP3). This marker initially showed great promise due to its high surface expression in ccRCC. Comparing ccRCC, hepatocellular carcinoma (HCC), and colon cancers samples, elevated ENPP3 expression was most consistently found in 285 ccRCC samples (93.7% ENPP3 positive, 83.9% high ENPP3 expression) [32]. The authors of this study went on to investigate the ADC-AGS16F consisting of an anti-ENPP3 antibody conjugated with a maleimidocaproyl linker to the cytotoxic microtuble-disrupting MMAF agent in a RCC xenograft model. In this preclinical study, Donate et al. showed that AGS16F localized to tumors, formed its active metabolite, and induced apoptosis as indicated by an increase in blood levels of a marker of epithelial cell death [33]. A phase II study by Kollmannsberger et al. went on to compare the anti-ENPP3 ADC AGS-16C3F to axitinib in previously treated metastatic RCC patients. 133 patients were randomized 1:1 to each treatment group with the primary endpoint of PFS. 26% of patients in the AFS-16C3F arm had TEAEs of grade three or higher compared to 45% in the axitinib group. At time of study cutoff, only 3 of 67 (4.5%) of patients remained in the ADC treatment group compared to 5 of 66 (7.6%) axitinib group most related to lack of PFS. Mean PFS was 2.9 months in the ADC group compared to 5.7 in the axitinib group (Hazard Ratio, 1.7 95% Confidence Interval (1.1–2.5)). Key qualifiers to the study were that it included less common RCC subtypes in both arms, though, the response to axitinib was equivocal to past studies investigating its use in exclusively ccRCC cohorts. More troubling for the ADC, ENPP3 expression was not a limitation as most tissue samples expressed high level of the antigen. Based on the results of the clinical trial, further development of AGS-16C3F is not being pursued [32].
Potential RCC Targets for Future Therapies
In an effort to find a suitable RCC target antigen, two proteogenomic studies in ccRCC have been performed on a total of 342 ccRCC tumors and 315 normal adjacent tissue [21, 22]. In-house analysis of both studies revealed 64 cell-surface proteins significantly upregulated in ccRCC compared to normal adjacent tissue (Fold change≥2 and adjusted p value≤0.05) (Fig. 1A-1B). Ranking of cell-surface proteins based on their expression in normal human tissue using the GTEx Proteome revealed MMP14, EGFR, MCT4 and CDH13 as the top 4 surface proteins most expressed in RCC with minimal expression in normal tissue, thereby representing promising targets for new antibody-based therapeutics (Fig. 1C) [24]. Table 2 summarizes the targets reviewed below.
Fig.1
RCC targets for antibody-based therapeutics. A) Volcano plots showing differentially expressed surface proteins from two proteogenomic studies (Qu, Y., et al., Nature Communications, 2022. Clark, D., et al., Cell, 2019) B) Venn diagram showing commonly upregulated surface proteins in both studies C) Ranking of surface proteins based on their maximal protein abundance in normal tissue using GTEx proteome with top candidates indicated in bold.
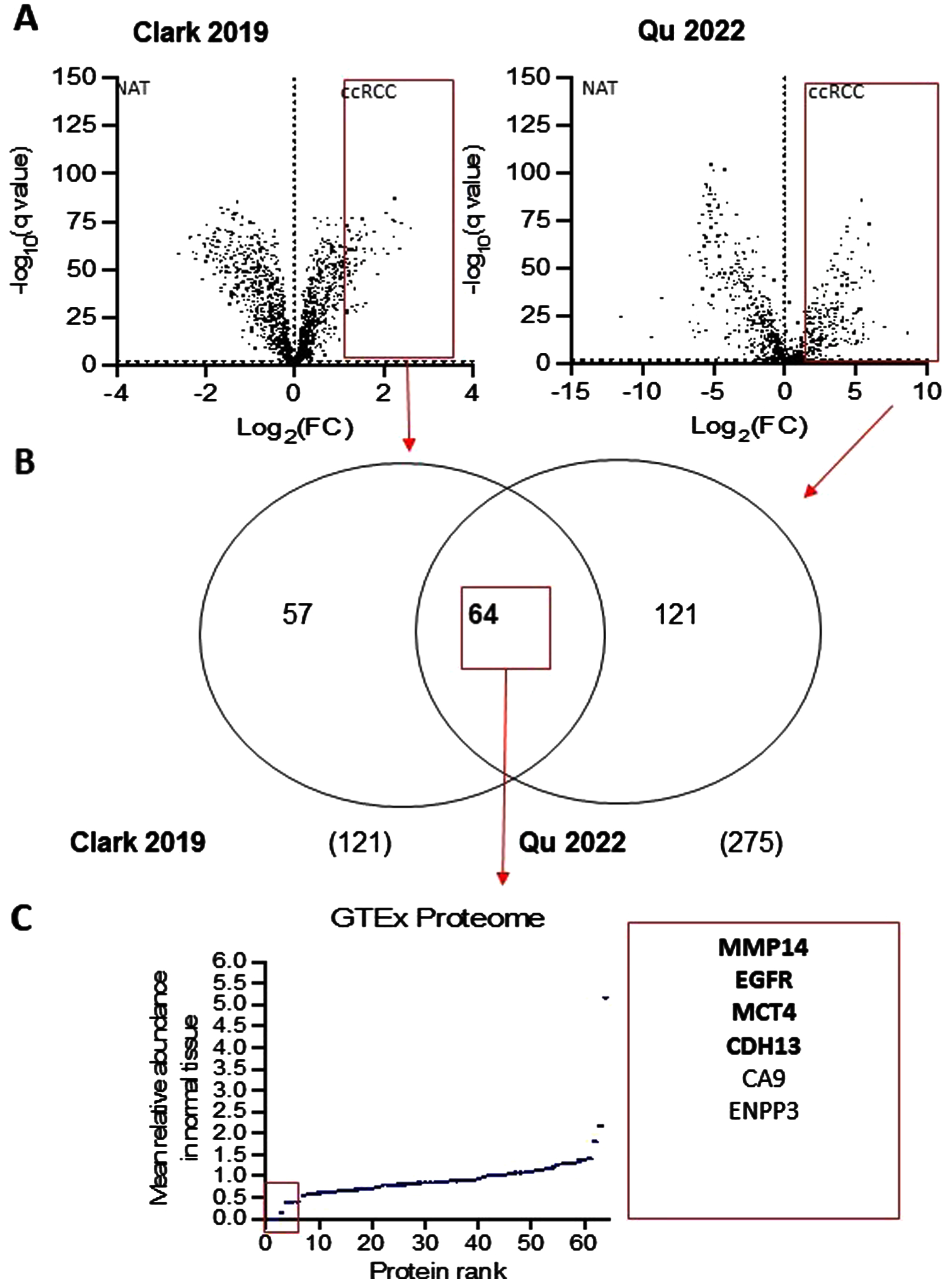
Table 2
Potential RCC Targets for future therapies
Targets | Preclinical development | Association to RCC |
MMP14 | BT1718 drug conjugate that binds to MMP14 and is covalently linked to DM1 | Upregulation of MMP14 was correlated with poor survival prognosis in RCC |
EGFR | Various for different cancers | Demonstrated effect in targeted CAR-T Cell therapy |
MCT4 | AZD0095 is a potent inhibitor of MCT4. | MCT4 mediates lactate export from glycolysis, an energy pathway highly active in RCC |
CA9 | Anti-CA9 ADC BAY 79-4620 | Highly expressed in ccRCCs |
MET | Telisotuzumab-vedotin | Same target as established RCC drug cabozantinib |
CDH13 | None to date | Relation between CDH13 expression in low grade tumors and PFS and OS |
B7-H3 (CD276) | MGCO18 | Demonstrated expression and antitumor activity in vitro and in xenografts by targeted monoclonal antibody |
PSMA | Lutetium-177–PSMA-617 | Demonstrated expression with validated safety profiles in treatment of prostate cancer |
MMP14
MMP14 (MT1-MMP) is a cell surface metalloprotease involved in extracellular matrix remodeling, cancer cell invasion and metastasis formation [34]. Upregulation of MMP14 was significantly positively correlated with poor survival prognosis in renal clear cell carcinoma [35]. Further supporting the importance of MMP14 in RCC pathogenesis is a study by Petrella et al. which linked the loss of the VHL tumor suppressor, a causative mechanism for RCC, with increased MMP14 gene and protein expression [36]. More recently a bicyclic drug conjugate that binds to MMP14 and is covalently linked to the anti-tubulin agent DM1 called BT1718 has been developed. Preclinical testing of BT1718 demonstrated effective killing of NSCLC tumors in vitro and in a PDX model, resulting in the progression of BT1718 to clinical trials [37].
EGFR
Several members of the Human Epidermal Growth Factor Receptor (EGFR) family have been identified as therapeutic targets in urologic cancers. A recent meta-analysis by Sheng et al. pooled 10 studies of EGFR expression in renal cell carcinoma and showed a statistical difference between EGFR expression in RCC tissue compared to controls as well as a higher EGFR level in patient with metastases [38]. A phase 1 nonrandomized clinical trial investigating an EGFR-targeting valine-citrulline linker MMAE ADC, MRG003, in EGFR-positive advanced refractory squamous cell carcinomas of the head and neck, nasopharyngeal cancer, and colorectal cancer showed 19 of the total 61 patients (39%) reported adverse events grade 3 or greater with objective response rates of 40%, 55%, and 0%, respectively. While the drug showed less skin toxic effects compared to US FDA EGFR-targeting monoclonal antibodies cetuximab and panitumumab, two deaths linked to febrile neutropenia related to MMAE were reported demonstrating the need for careful hematological monitoring with the drug [39]. Further supporting the role of EGFR as a target in RCC, Zhang et al. showed in a mouse model the potential of CAR-T cells targeting EGFR in combination with an established NK cell line (NK-92) and the TKI cabozantinib. Treatment with cabozantinib promoted increased EGFR and decreased PD-L1 membrane surface expression in RCC cells, further enhancing the efficacy of CAR-NK-92 cells against RCC in vitro and perhaps suggesting an interesting option to explore in EGFR-targeting ADCs for RCC [40].
MCT4
MCT4 is a monocarboxylate transporter (MCT) that complexes with the chaperone molecule CD147 to be expressed on cell surfaces [41]. MCT4 mediates the export of lactate produced from glycolysis, an energy pathway highly active in RCC [42, 43]. In line with this, there is significant interest in targeting MCT4 to disrupt glycolysis and induce apoptosis of RCC cells. MCT4 and MCT1, another member of the MCT family, were investigated as part of a study analyzing 180 ccRCC tissue samples resected at a single hospital between 1996 and 2013. The researchers found high expression levels in the samples of MCT1 (89/180, 49.4%), MCT4 (143/180, 79.4%), and CD147 (143/180, 79.4%). High expression of each protein demonstrated a significant correlation with PFS, and MCT1 was found to be an independent factor predicting poorer PFS in a multivariate survival analysis [44]. In support of the focus on MCT4 as a clinical target, a selective and potent inhibitor of MCT4, AZD0095, was recently discovered through a phenotypic drug screen [45]. In a panel of cancer cell lines expressing MCT4, AZD0095 had antiproliferative activity as a single agent. Furthermore, AZD0095 had strong antitumor efficacy in a mouse xenograft model when combined with VEGF inhibitors and in an immune competent murine model when combined with immune checkpoint inhibitors. To our knowledge, no specific MCT-ADC are currently being investigated, though, the MCT isoforms represent an interesting target for advanced RCC treatment [45].
CDH13
CDH13 is a member of the cadherin family involved in cell-cell adhesion and cellular signaling [46]. A group led by Shao et al. investigated whether CDH13 might serve as a target for ccRCC. The group analyzed 553 ccRCC and 72 normal kidney control samples from The Cancer Genome Atlas database. The researchers investigated the predictive value of CDH13 staining and determined in their dataset that its expression had an AUC of 0.822 (95% CI: 0.784–0.861) for diagnosing RCC. Interestingly, researchers found an association between elevated CDH13 expression with lower grade tumors and better PFS and OS. This relationship suggests that CDH13 expression may serve as good prognostic indicator and perhaps as a target in treating ccRCC disease [47].
A few other cell surface proteins have been recently described as emerging therapeutic targets for RCC which include Carbonic anhydrase 9 (CA9), MET, B7-H3 and PSMA.
CA9
CA9 is a well-studied protein associated with ccRCC [48]. It is upregulated in ccRCC cells and functions to help maintain a neutral pH in spite of excess lactic acid production as a by-product of ccRCC’s reliance on the glycolytic. Tostain et al. reviewed studies of the CA9 antigen and reported that it is expressed in 94–97% of ccRCCs samples with limited expression in other RCC sub-types and no expression in healthy renal tissue [49–52]. Girentuximab is an antibody against CA9 that holds promise for diagnostic imaging of ccRCC using molecular PET/CT and theranostics [53, 54].
In 2012, an anti-CA9 valine-citrulline MMAE-linked ADC (BAY 79-4620) developed by Bayer HealthCare showed efficacy in in vitro and in vivo studies with complete responses in several types of tumors [55]. A safety and tolerability phase I trial evaluating BAY 79-4620 in patients with advanced solid tumors was discontinued due to grade 5 adverse events in two patients (NCT01065623). No other ADC targeting CA9 is currently being evaluated in clinical trials.
MET
As mentioned previously when discussing the oral TKI cabozantinib, the overexpression of the MET receptor has been implicated in types of advanced RCC. Telisotuzumab-vedotin (TV) is an ADC currently being investigated that also targets tissues overexpressing the MET receptor and delivers the same linker-microtubule disruptor as EV (vedotin-MMAE). In the 2018 First-in-Human Phase 1, Dose Escalation and -Expansion study of TV, 48 patients with advanced solid tumors were enrolled into eight cohorts. 8 Patients (17%) experience a TEAEs (grade > = 3), most commonly fatigue, anemia, neutropenia, and hyperalbuminemia [56]. While the results of this study were designed to evaluate toxicity and response for non-small cell lung cancer, the shared pathway of targeting the MET receptor may lead TV to be investigated for use specifically in advanced RCC.
B7-H3 (CD276)
MGCO18 is a preclinical ADC targeting B7-H3 with a valine-citrulline link DNA alkylating duocarmycin agent, a protein overexpressed in many cancers [57]. Specifically in RCC, B7-H3 expression was found in only 17.4% of 743 tumors but in 95.1% of associated tumor vasculature samples. There was an association between increasing B7-H3 expression and adverse clinical outcomes with a retrospective finding that its expression independently predicted disease progression and cancer specific deaths [58]. Another study demonstrated in vitro and xenograft anti-RCC activity by B7-H3 monoclonal antibodies with no adverse effects noted in a safety assessment with 5 administrations over 4 weeks in cynomolgus monkeys [59]. These studies support the role of investigating B7-H3 targeting ADCs for possible treatment of advanced RCC.
PSMA
Prostate-specific membrane antigen (PSMA) is a transmembrane glycoprotein highly expressed on the surface of prostate cancer epithelial cells. Despite the specificity implied by its nomenclature, PSMA is also expressed in the vasculature of other types of tumors, including RCC. More specifically Baccala et al. reported positive PSMA staining in tumour-associated neovascular cells in 76.2% of ccRCC, 31.2% of chromophobe RCC, 52.6% of renal oncocytoma, and 21.4 % of transitional RCC [60, 61]. To date, there have been more than a dozen antibodies that target PSMA developed for the treatment of prostate cancer, such as the Lutetium-177-PSMA theranostic [62]. With validation of safety in trials for prostate cancer, this theranostic may be explored in the future for advanced RCC.
FUTURE DIRECTIONS IN ADC RESEARCH
A recent review by Mahmoud et al. discussed four key challenges to the use of antibody-based therapeutics: On-Target Off-Tumor Cytotoxicity, Tumor Heterogeneity, Circulating Molecular Decoys, and Tumor Uptake [63]. These concepts also apply to ADCs with some specific considerations.
It is estimated that approximately 0.1% of ADC dose administered accumulates at the target site, leaving the vast majority of drug in circulation [64]. Limited tumor uptake can lead to longer retention in blood resulting in antigen-independent uptake of ADC by blood cells and healthy tissues, such as lungs, and premature release of cytotoxic payloads or inflammatory responses. When considering toxicity, the main benefit of ADCs is the ability to deliver higher doses of cytotoxic compounds directly to pathologic tissues as opposed to standard chemotherapy. However, side effects observed in trials show that whether due to linkage dissolution or “on-target off-tumor toxicity”, some of the cytoxic payload is being delivered to non-pathologic tissues. The evidence of this is important to consider for individual drugs as well as when evaluating whether certain ADCs can be used together given the profile of their cytotoxic payloads. For example, administering two ADCs with MMAF or MMAE payloads at their standard dosing may significantly increase neurologic side effects [65, 66]. Regarding combinations with ICI therapy, data from early breast cancer trials have not shown significant interactions between the two therapies, owing to the different mechanisms of how the drug classes operate [67]. Efforts to improve toxicity of ADCs include research into miniaturized ADCs formed with payloads coupled to single chain region fragment (scfv) or monomeric antibody fragments to improve payload delivery within tumors and reduce retention in blood [68, 69].
Another important concept discussed by Mahalingaiah et al. is ADC payload release and the bystander effect. This concept describes how antigen-negative cells can be affected by residual cytotoxic payloads accumulating from premature release or from spilling of killed antigen-positive cells that have internalized an ADC. This feature enables the destruction of surrounding antigen-negative tumor cells and is especially beneficial in the treatment of tumors with heterogeneous expression of the target. Supporting the importance of the bystander effect, Mahmoud et al. summarized the phenotypical differences that can occur in a primary tumor (intratumoral heterogeneity) as well as primary and metastatic tumors (intertumoral heterogeneity) due to different environmental and treatment related interactions [63, 70–73]. Another important consideration of the bystander effect is payload cell membrane permeability. Ogitani et al. compared the association between ADCs with different membrane permeability and the effects on in vitro heterogenous tumors and found that payloads need to be able to cross the cell membrane for the bystander effect to occur [74, 75]. Thus, non-polar payloads that can be released from ADCs by a cleavable linker are better equipped to produce a bystander effect than their polar counterparts. An important secondary finding to their study regarding the safety concerns of the Bystander effect was that antigen-negative killing was limited to tumor adjacent cells rather than diffusely throughout the body [75]. While the bystander effect can possibly lead to toxic side effects for antigen-negative normal tissue, it represents an opportunity that should be leveraged in vivo to combat tumor heterogeneity.
Recent evidence highlights the therapeutic potential of ADCs with multiple payloads to overcome drug resistance. Levengood et al. demonstrated the efficacy of dual-payload ADCs in vivo, and Yamazaki et al. extended on this work by comparing the use of a dual-drug ADC against co-administered single-drug ADCs in the treatment of breast cancer [76, 77]. They first used in vitro and PDX studies to investigate the toxicity profile and to tune their dual-payload ADC. Then, they investigated its use in in vivo using mice models. They found that the dual-payload ADC resulted in complete remission at some doses, while the co-administered ADCs did not demonstrate similar efficacy. Two important findings suggested by their results are: 1) co-administration of ADCs targeting the same antigen may be ineffective due to receptor saturation and 2) payloads may demonstrate better efficacy working in concert with each other. These results represent further areas of research on how to leverage the remarkable flexibility of ADCs to improve efficacy and minimize toxicity.
CONCLUSIONS
Medical therapy for metastatic RCC has undergone several paradigm shifts beginning with cytokine therapy to now consisting of mainly combination checkpoint inhibitor-based therapies. Primary and secondary treatment resistance are key obstacles for current treatments with several promising breakthroughs being researched that may further change the therapeutic landscape. While ADCs therapeutics have not shown benefit to date for renal cell carcinoma compared to other solid organ tumors, there are ample promising candidates and targets for future research.
ACKNOWLEDGMENTS
The authors have no acknowledgments.
FUNDING
The authors report no funding.
AUTHOR CONTRIBUTIONS
Conception and design: FL, BAC and JC; Collection of data: HCG, MGD, AMM, JO, IG, and FL; Manuscript writing: HCG, MGD, RZ, RRP, IG, JO, BAC and FL; Final approval of the manuscript: all authors; Accountable for all aspects of the work: all authors.
CONFLICTS OF INTEREST
HCG, RN, MD, RRP, AMM, IG, JO, BAC, JC and FL have no conflicts of interest to report.
ABBREVIATIONS
ADC | Antibody Drug Conjugate |
ASCO | American Society of Clinical Oncology |
DEPs | Differentially Expressed Proteins |
DFS | Disease Free Survival |
ESMO | European Society for Medical Oncology |
EV | Enfortumab Vedotin |
OS | Overall Survival |
MMAE | Monomethyl auristatin E |
MMAF | Monomethyl auristatin F |
PDX | Patient Derived Xenograft |
PFS | Progression Free Survival |
RCC | Renal Cell Carcinoma |
REFERENCES
[1] | Siegel R , Miller K , Fuchs H , Jemal A . Cancer statistics, 2021 CA: Cancer Journal for Clinicians. (2021) ;71: (4):359. |
[2] | Ljungberg B , Campbell SC , Cho HY , Jacqmin D , Lee JE , Weikert S , et al. The epidemiology of renal cell carcinoma. European Urology. (2011) ;60: (4):615–21. |
[3] | Albiges L , Powles T , Staehler M , Bensalah K , Giles RH , Hora M , et al. Updated European Association of Urology guidelines on renal cell carcinoma: immune checkpoint inhibition is the new backbone in first-line treatment of metastatic clear-cell renal cell carcinoma. European Urology. (2019) ;76: (2):151–6. |
[4] | Powles T . Recent eUpdate to the ESMO Clinical Practice Guidelines on renal cell carcinoma on cabozantinib and nivolumab for first-line clear cell renal cancer: Renal cell carcinoma: ESMO Clinical Practice Guidelines for diagnosis, treatment and follow-up1. Annals of Oncology. (2021) ;32: (3):422–3. |
[5] | Rathmell WK , Rumble RB , Van Veldhuizen PJ , Al-Ahmadie H , Emamekhoo H , Hauke RJ , et al. Management of metastatic clear cell renal cell carcinoma: ASCO guideline. Journal of Clinical Oncology.. (2022) ;40: (25):2957–95. |
[6] | Moreira M , Pobel C , Epaillard N , Simonaggio A , Oudard S , Vano Y-A . Resistance to cancer immunotherapy in metastatic renal cell carcinoma. Cancer Drug Resistance. (2020) ;3: (3):454. |
[7] | Li S , Li J , Peng L , Li Y , Wan X . Cost-effectiveness of frontline treatment for advanced renal cell carcinoma in the era of immunotherapies. Frontiers in Pharmacology. (2021) ;12: :718014. |
[8] | Postow MA , Sidlow R , Hellmann MD . Immune-related adverse events associated with immune checkpoint blockade. New England Journal of Medicine. (2018) ;378: (2):158–68. |
[9] | Lu G , Fakurnejad S , Martin BA , van den Berg NS , van Keulen S , Nishio N , et al. Predicting therapeutic antibody delivery into human head and neck cancers. Clinical Cancer Research. (2020) ;26: (11):2582–94. |
[10] | Fu Z , Li S , Han S , Shi C , Zhang Y . Antibody drug conjugate: the “biological missile” for targeted cancer therapy. Signal Transduction and Targeted Therapy. (2022) ;7: (1):93. |
[11] | Lonsdale J , Thomas J , Salvatore M , Phillips R , Lo E , Shad S , et al. The genotype-tissue expression (GTEx) project. Nature genetics. (2013) ;45: (6):580–5. |
[12] | Chia CB . A patent review on FDA-approved antibody-drug conjugates, their linkers and drug payloads. ChemMedChem.e. (2022) ;17: (11):202200032. |
[13] | Donaghy H editor Effects of antibody, drug and linker on the preclinical and clinical toxicities of antibody-drug conjugates. MAbs; 2016: Taylor & Francis. |
[14] | Von Minckwitz G , Huang C-S , Mano MS , Loibl S , Mamounas EP , Untch M , et al. Trastuzumab emtansine for residual invasive HER2-positive breast cancer. New England Journal of Medicine. (2019) ;380: (7):617–28. |
[15] | Rosenberg JE , O’Donnell PH , Balar AV , McGregor BA , Heath EI , Evan YY , et al. Pivotal trial of enfortumab vedotin in urothelial carcinoma after platinum and anti-programmed death 1/programmed death ligand 1 therapy. Journal of Clinical Oncology. (2019) ;37: (29):2592. |
[16] | Hoimes CJ , Flaig TW , Milowsky MI , Friedlander TW , Bilen MA , Gupta S , et al. Enfortumab vedotin plus pembrolizumab in previously untreated advanced urothelial cancer. Journal of clinical oncology.. (2023) ;41: (1):22–31. |
[17] | Rosenberg J , Milowsky M , Ramamurthy C , Mar N , Mckay R , Friedlander T , et al. editors. Study EV-103 Cohort K: Antitumor activity of enfortumab vedotin (EV) monotherapy or in combination with pembrolizumab (P) in previously untreated cisplatin-ineligible patients (pts) with locally advanced or metastatic urothelial cancer (la/mUC). Annals of Oncology; 2022: ELSEVIERRADARWEG29, 1043NX AMSTERDAM, NETHERLANDS. |
[18] | Ayanambakkam, Adanma. Updates on advanced bladder cancer treatment. Urology (2023). |
[19] | Tagawa ST , Balar AV , Petrylak DP , Kalebasty AR , Loriot Y , Fléchon A , et al. TROPHY-U-01: a phase II open-label study of sacituzumab govitecan in patients with metastatic urothelial carcinoma progressing after platinum-based chemotherapy and checkpoint inhibitors. Journal of Clinical Oncology. (2021) ;39: (22):2474. |
[20] | Gogia P , Ashraf H , Bhasin S , Xu Y . Antibody–drug conjugates: a review of approved drugs and their clinical level of evidence. Cancers. (2023) ;15: (15):3886. |
[21] | Clark DJ , Dhanasekaran SM , Petralia F , Pan J , Song X , Hu Y , et al. Integrated proteogenomic characterization of clear cell renal cell carcinoma. Cell. (2019) ;179: (4):964–83. e31. |
[22] | Qu Y , Feng J , Wu X , Bai L , Xu W , Zhu L , et al. A proteogenomic analysis of clear cell renal cell carcinoma in a Chinese population. Nature communications. (2022) ;13: (1):1–21. |
[23] | Lavoie RR , Gargollo PC , Ahmed ME , Kim Y , Baer E , Phelps DA , et al. Surfaceome profiling of rhabdomyosarcoma reveals B7-H3 as a mediator of immune evasion. Cancers. (2021) ;13: (18):4528. |
[24] | Jiang L , Wang M , Lin S , Jian R , Li X , Chan J , et al. A quantitative proteome map of the human body. Cell. (2020) ;183: (1):269–83. e19. |
[25] | Han WK , Alinani A , Wu C-L , Michaelson D , Loda M , McGovern FJ , et al. Human kidney injury molecule-1 is a tissue and urinary tumor marker of renal cell carcinoma. Journal of the American Society of Nephrology. (2005) ;16: (4):1126–34. |
[26] | Lin F , Zhang PL , Yang XJ , Shi J , Blasick T , Han WK , et al. Human kidney injury molecule-1 (hKIM-1): a useful immunohistochemical marker for diagnosing renal cell carcinoma and ovarian clear cell carcinoma. The American Journal of Surgical Pathology. (2007) ;31: (3):371–81. |
[27] | McGregor BA , Gordon M , Flippot R , Agarwal N , George S , Quinn DI , et al. Safety and efficacy of CDX-014, an antibody-drug conjugate directed against T cell immunoglobulin mucin-1 in advanced renal cell carcinoma. Investigational New Drugs. (2020) ;38: (6):1807–14. |
[28] | Ma L , Zhou Z , Jia H , Zhou H , Qi A , Li H , et al. Effects of CD70 and CD11a in immune thrombocytopenia patients. Journal of Clinical Immunology. (2011) ;31: :632–42. |
[29] | Pal SK , Forero-Torres A , Thompson JA , Morris JC , Chhabra S , Hoimes CJ , et al. A phase 1 trial of SGN-CD70A in patients with CD70-positive, metastatic renal cell carcinoma. Cancer. (2019) ;125: (7):1124–32. |
[30] | Bialucha CU , Collins SD , Li X , Saxena P , Zhang X , Dürr C , et al. Discovery and Optimization of HKT288, a Cadherin-6–Targeting ADC for the Treatment of Ovarian and Renal CancersCDH6-ADC for the Treatment of Ovarian and Renal Cancer. Cancer Discovery. (2017) ;7: (9):1030–45. |
[31] | Schöffski P , Concin N , Suarez C , Subbiah V , Ando Y , Ruan S , et al. A phase 1 study of a CDH6-Targeting antibody-drug conjugate in patients with advanced solid tumors with evaluation of inflammatory and neurological adverse events. Oncology Research and Treatment. (2021) ;44: (10):547–56. |
[32] | Doñate F , Raitano A , Morrison K , An Z , Capo L , Aviña H , et al. AGS16F Is a Novel Antibody Drug Conjugate Directed against ENPP3 for the Treatment of Renal Cell CarcinomaADC Cancer Therapeutic Targeting ENPP3. Clinical Cancer Research. (2016) ;22: (8):1989–99. |
[33] | Kollmannsberger C , Choueiri TK , Heng DY , George S , Jie F , Croitoru R , et al. A Randomized Phase II Study of AGS-16C3F Versus Axitinib in Previously Treated Patients with Metastatic Renal Cell Carcinoma. The Oncologist. (2021) ;26: (3):182–e361. |
[34] | Castro-Castro A , Marchesin V , Monteiro P , Lodillinsky C , Rossé C , Chavrier P . Cellular and molecular mechanisms of MT1-MMP-dependent cancer cell invasion. Annual Review of Cell and Developmental Biology. (2016) ;32: :555–76. |
[35] | Zhao Y-W , Ma W , Jiang F , Xie Y , Tang L . Upregulation of matrix metalloproteinase 14 (MMP14) is associated with poor prognosis in renal clear cell carcinoma— a bioinformatics analysis. Translational Andrology and Urology. (2022) ;11: (11):1523. |
[36] | Petrella BL , Lohi J , Brinckerhoff CE . Identification of membrane type-1 matrix metalloproteinase as a target of hypoxia-inducible factor-2α in von Hippel–Lindau renal cell carcinoma. Oncogene. (2005) ;24: (6):1043–52. |
[37] | Bennett G , Lutz R , Park P , Harrison H , Lee K . Development of BT1718, a novel Bicycle Drug Conjugate for the treatment of lung cancer. Cancer Research. (2017) ;77: (13_Supplement):1167. |
[38] | Wang S , Yu Z-h , Chai K-q Identification of EGFR as a novel key gene in clear cell renal cell carcinoma (ccRCC) through bioinformatics analysis and meta-analysis. BioMed research International. 2019;2019. |
[39] | Qiu M-Z , Zhang Y , Guo Y , Guo W , Nian W , Liao W , et al. Evaluation of Safety of Treatment With Anti–Epidermal Growth Factor Receptor Antibody Drug Conjugate MRG003 in Patients With Advanced Solid Tumors: A Phase 1 Nonrandomized Clinical Trial. JAMA Oncology. (2022) ;8: (7):1042–6. |
[40] | Zhang Q , Tian K , Xu J , Zhang H , Li L , Fu Q , et al. Synergistic effects of cabozantinib and EGFR-specific CAR-NK-92 cells in renal cell carcinoma. Journal of Immunology Research. 2017;2017. |
[41] | Le Floch R , Chiche J , Marchiq I , Naiken T , Ilc K , Murray CM , et al. CD147 subunit of lactate/H+ symporters MCT1 and hypoxia-inducible MCT4 is critical for energetics and growth of glycolytic tumors. Proceedings of the National Academy of Sciences. (2011) ;108: (40):16663–8. |
[42] | Cao Y-W , Liu Y , Dong Z , Guo L , Kang E-H , Wang Y-H , et al. editors. Monocarboxylate transporters MCT1 and MCT4 are independent prognostic biomarkers for the survival of patients with clear cell renal cell carcinoma and those receiving therapy targeting angiogenesis. Urologic Oncology: Seminars and Original Investigations; 2018: Elsevier. |
[43] | Semenza GL . HIF-1 mediates the Warburg effect in clear cell renal carcinoma. Journal of Bioenergetics and Biomembranes. (2007) ;39: (3):231. |
[44] | Kim Y , Choi J-W , Lee J-H , Kim Y-S . Expression of lactate/H+ symporters MCT1 and MCT4 and their chaperone CD147 predicts tumor progression in clear cell renal cell carcinoma: immunohistochemical and The Cancer Genome Atlas data analyses. Human Pathology.. (2015) ;46: (1):104–12. |
[45] | Goldberg FW , Kettle JG , Lamont GM , Buttar D , Ting AK , McGuire TM , et al. Discovery of clinical candidate AZD0095, a selective inhibitor of monocarboxylate transporter 4 (MCT4) for oncology. Journal of Medicinal Chemistry. (2022) ;66: (1):384–97. |
[46] | Andreeva AV , Kutuzov MA . Cadherin 13 in cancer. Genes, Chromosomes and Cancer. (2010) ;49: (9):775–90. |
[47] | Shao Y , Li W , Zhang L , Xue B , Chen Y , Zhang Z , et al. CDH13 is a prognostic biomarker and a potential therapeutic target for patients with clear cell renal cell carcinoma. American Journal of Cancer Research.. (2022) ;12: (10):4520. |
[48] | Oosterwijk E , Ruiter DJ , Hoedemaeker PJ , Pauwels EK , Jonas U , Zwartendijk J , et al. Monoclonal antibody G 250 recognizes a determinant present in renal-cell carcinoma and absent from normal kidney. Int J Cancer. (1986) ;38: (4):489–94. |
[49] | Tostain J , Li G , Gentil-Perret A , Gigante M . Carbonic anhydrase 9 in clear cell renal cell carcinoma: a marker for diagnosis, prognosis and treatment. European Journal of Cancer. (2010) ;46: (18):3141–8. |
[50] | Patard JJ , Fergelot P , Karakiewicz PI , Klatte T , Trinh QD , Rioux-Leclercq N , et al. Low CAIX expression and absence of VHL gene mutation are associated with tumor aggressiveness and poor survival of clear cell renal cell carcinoma. International Journal of Cancer. (2008) ;123: (2):395–400. |
[51] | Bui MH , Seligson D , Han K-r , Pantuck AJ , Dorey FJ , Huang Y , et al. Carbonic anhydrase IX is an independent predictor of survival in advanced renal clear cell carcinoma: implications for prognosis and therapy. Clinical Cancer Research. (2003) ;9: (2):802–11. |
[52] | Sandlund J , Oosterwijk E , Grankvist K , Oosterwijk-Wakka J , Ljungberg B , Rasmuson T . Prognostic impact of carbonic anhydrase IX expression in human renal cell carcinoma. BJU International. (2007) ;100: (3):556–60. |
[53] | Feldman DR , Motzer RJ , Knezevic A , Lee C-H , Voss MH , Lyashchenko SK , et al. STARLITE 2: Phase 2 study of nivolumab plus 177Lutetium-labeled anti-carbonic anhydrase IX (CAIX) monoclonal antibody girentuximab (177Lu-girentuximab) in patients (pts) with advanced clear cell renal cell carcinoma (ccRCC). American Society of Clinical Oncology 2: :2022. |
[54] | Shuch BM , Pantuck AJ , Bernhard J-C , Morris MA , Master VA , Scott AM , et al. Results from phase 3 study of 89Zr- DFO-girentuximab for PET/CT imaging of clear cell renal cell carcinoma (ZIRCON). American Society of Clinical Oncology; 2023. |
[55] | Petrul HM , Schatz CA , Kopitz CC , Adnane L , McCabe TJ , Trail P , et al. Therapeutic mechanism and efficacy of the antibody-drug conjugate BAY 79-4620 targeting human carbonic anhydrase 9. Mol Cancer Ther. (2012) ;11: (2):340–9. |
[56] | Strickler JH , Weekes CD , Nemunaitis J , Ramanathan RK , Heist RS , Morgensztern D , et al. First-in-Human Phase I, Dose-Escalation and-Expansion Study of Telisotuzumab Vedotin, an Antibody-Drug Conjugate Targeting c-Met, in Patients With Advanced Solid Tumors. Journal of clinical oncology: official journal of the American Society of Clinical Oncology. (2018) ;36: (33):3298–306. |
[57] | Scribner JA , Brown JG , Son T , Chiechi M , Li P , Sharma S , et al. Preclinical Development of MGC018, a Duocarmycin-based Antibody–drug Conjugate Targeting B7-H3 for Solid CancerMGC018, a Duocarmycin-based ADC Targeting B7-H3 for Cancer. Molecular Cancer Therapeutics. (2020) ;19: (11):2235–44. |
[58] | Crispen PL , Sheinin Y , Roth TJ , Lohse CM , Kuntz SM , Frigola X , et al. Tumor cell and tumor vasculature expression of B7-H3 predict survival in clear cell renal cell carcinoma (2008) ;14: (16):5150–7. |
[59] | Loo D , Alderson RF , Chen FZ , Huang L , Zhang W , Gorlatov S , et al. Development of an Fc-Enhanced Anti–B7-H3 Monoclonal Antibody with Potent Antitumor ActivityDevelopment of Fc-Enhanced Anti–B7-H3 Monoclonal Antibody. Clinical Cancer Research.. (2012) ;18: (14):3834–45. |
[60] | Baccala A , Sercia L , Li J , Heston W , Zhou M . Expression of prostate-specific membrane antigen in tumor-associated neovasculature of renal neoplasms. Urology. (2007) ;70: (2):385–90. |
[61] | Ahn T , Roberts MJ , Abduljabar A , Joshi A , Perera M , Rhee H , et al. A review of prostate-specific membrane antigen (PSMA) positron emission tomography (PET) in renal cell carcinoma (RCC). Molecular Imaging Biology. (2019) ;21: (5):799–807. |
[62] | Sartor O , De Bono J , Chi KN , Fizazi K , Herrmann K , Rahbar K , et al. Lutetium-177–PSMA-617 for metastatic castration-resistant prostate cancer. New England Journal of Medicine. (2021) ;385: (12):1091–103. |
[63] | Mahmoud AM , Nabavizadeh R , Rodrigues Pessoa R , Garg I , Orme J , Costello BA , et al. Antibody-Based Therapeutics for the Treatment of Renal Cell Carcinoma: Challenges and Opportunities. The Oncologist.. (2023) ;28: (4):297–308. |
[64] | Casi G , Neri D . Antibody–Drug Conjugates and Small Molecule–Drug Conjugates: Opportunities and Challenges for the Development of Selective Anticancer Cytotoxic Agents: Miniperspective. Journal of Medicinal Chemistry. (2015) ;58: (22):8751–61. |
[65] | Marei HE , Cenciarelli C , Hasan A . Potential of antibody–drug conjugates (ADCs) for cancer therapy. Cancer Cell International. (2022) ;22: (1):1–12. |
[66] | de Padua TC , Moschini M , Martini A , Pederzoli F , Nocera L , Marandino L , et al. editors. Efficacy and toxicity of antibody-drug conjugates in the treatment of metastatic urothelial cancer: A scoping review. Urologic Oncology: Seminars and Original Investigations; 2022: Elsevier. |
[67] | Zhang L , Yan Y , Chen Y , Lu J , Wang B , Ren N , et al. Will antibody-drug conjugate and immune checkpoint inhibitorbased combination therapy close the gap between benefit and toxicity in the treatment of cancers?Asystematic review and meta-analysis. 2023. |
[68] | Wu Y , Li Q , Kong Y , Wang Z , Lei C , Li J , et al. A highly stable human single-domain antibody-drug conjugate exhibits superior penetration and treatment of solid tumors. Molecular Therapy. (2022) ;30: (8):2785–99. |
[69] | Panikar SS , Banu N , Haramati J , del Toro-Arreola S , Leal AR , Salas P . Nanobodies as efficient drug-carriers: progress and trends in chemotherapy. Journal of Controlled Release. (2021) ;334: :389–412. |
[70] | Dagogo-Jack I , Shaw AT . Tumour heterogeneity and resistance to cancer therapies. Nature Reviews Clinical oncology. (2018) ;15: (2):81–94. |
[71] | Beksac AT , Paulucci DJ , Blum KA , Yadav SS , Sfakianos JP , Badani KK . Heterogeneity in renal cell carcinoma. Urologic Oncology: Seminars and Original Investigations. (2017) ;35: (8):507–15. |
[72] | Lee C , Park J-W , Suh JH , Nam KH , Moon KC . Histologic variations and immunohistochemical features of metastatic clear cell renal cell carcinoma. Korean Journal of Pathology. (2013) ;47: (5):426. |
[73] | Bissig H , Richter J , Desper R , Meier V , Schraml P , Schäffer AA , et al. Evaluation of the clonal relationship between primary and metastatic renal cell carcinoma by comparative genomic hybridization. The American Journal of Pathology. (1999) ;155: (1):267–74. |
[74] | Singh AP , Shah DK . A “dual” cell-level systems PK-PD model to characterize the bystander effect of ADC. Journal of Pharmaceutical Sciences. (2019) ;108: (7):2465–75. |
[75] | Ogitani Y , Hagihara K , Oitate M , Naito H , Agatsuma T . Bystander killing effect of DS-8201a, a novel anti-human epidermal growth factor receptor 2 antibody-drug conjugate, in tumors with human epidermal growth factor receptor 2 heterogeneity. Cancer Science. (2016) ;107: (7):1039–46. |
[76] | Levengood MR , Zhang X , Hunter JH , Emmerton KK , Miyamoto JB , Lewis TS , et al. Orthogonal cysteine protection enables homogeneous multi-drug antibody–drug conjugates. Angewandte Chemie International Edition. (2017) ;56: (3):733–7. |
[77] | Yamazaki CM , Yamaguchi A , Anami Y , Xiong W , Otani Y , Lee J , et al. Antibody-drug conjugates with dual payloads for combating breast tumor heterogeneity and drug resistance. Nature Communications. (2021) ;12: (1):3528. |