The vertical computerized rotational head impulse test
Abstract
The computerized rotational head impulse test (crHIT) uses a computer-controlled rotational chair to deliver whole-body rotational impulses to assess the semicircular canals. The crHIT has only been described for horizontal head plane rotations. The purpose of this study was to describe the crHIT for vertical head plane rotations. In this preliminary study, we assessed four patients with surgically confirmed unilateral peripheral vestibular abnormalities and two control subjects. Results indicated that the crHIT was well-tolerated for both horizontal head plane and vertical head plane stimuli. The crHIT successfully assessed each of the six semicircular canals. This study suggests that the crHIT has the potential to become a new laboratory-based vestibular test for both the horizontal and vertical semicircular canals.
1Introduction
The video head impulse test (vHIT) uses manually delivered horizontal or vertical plane head rotations while both head and eye movements are recorded by a computer [12]. Horizontal head rotations are used to assess the horizontal semicircular canals. Vertical plane impulses have been advocated as a means of assessing the four vertical semicircular canals [3]. A recent study of vertical vHIT reports variability in how impulses are delivered [19] and suggests that additional development is required for vertical vHIT to achieve consistent results. Using the crHIT for testing the vertical semicircular canals may be helpful in the further development of vHIT.
An alternative to manually delivered head-only impulses is computerized whole-body rotational impulses. Our previous results suggest that horizontal computerized rotational head impulse testing (the crHIT-horizontal) is a feasible test of unilateral horizontal semicircular canal function that improves upon the reliability of manually delivered head-only vHIT testing [6]. The purpose of this study was to assess the early stage of development of whole-body computerized rotational impulse testing to assess the vertical semicircular canals. This test, the crHIT-vertical, in much the same way as the crHIT-horizontal, obviates the requirement for an examiner who is expert in delivering head impulses, eliminates any contribution from the neck, and improves patient comfort because there is no head-on-neck movement. Moreover, by aligning the plane of rotation precisely with either the right anterior-left posterior (RALP) or left anterior-right posterior (LARP) plane, the contribution to the vestibular ocular reflex (VOR) from the opposite vertical canal plane can be minimized. Alignment of the rotational stimulus to the canal plane being evaluated may be critical for an accurate assessment of the vertical semicircular canals as the contribution from the opposite plane vertical semicircular canals follows a sine rule such that small errors in aligning the axis of rotation of the head perpendicular to the plane of the vertical semicircular canals can have a non-negligible effect on eye movement [4, 17]. Note that this adverse effect of misalignment of the stimulus is thought to be mitigated if the subject’s gaze is directed toward the plane of the vertical canals being assessed and vertical eye movement is used as the response parameter [3, 7]. Another important advantage of whole-body rotational impulses is that the position excursion of the impulses is not constrained by anatomical considerations of the head-on-neck or neck-on-torso thereby allowing a longer duration and a higher amplitude impulse leading to a longer duration of usable data per impulse. Furthermore, computer-controlled whole-body impulses are precise and repeatable and can easily be randomized in both direction and duration to minimize predictability. The obvious disadvantage of computerized whole-body rotational impulses is the need for a large, costly, fixed-location, rotational chair in a dedicated clinical laboratory space.
In this preliminary study, we assessed four patients with surgically confirmed unilateral peripheral vestibular abnormalities and two control subjects. One of our patients had a surgically confirmed selective superior vestibular nerve lesion and was an ideal subject in whom to assess the effect of loss of one of the four vestibular nerves. We used the crHIT to assess all six semicircular canals. We found that the crHIT was well-tolerated for both horizontal plane and vertical plane stimuli and appeared to assess each vertical semicircular canal separately. Our aim was to develop the crHIT for both the horizontal and vertical semicircular canals as a new laboratory test based on decades of research by other investigators who discovered and developed the vHIT based on using manually delivered head impulse testing [8, 9].
2Methods
This study was designed to assess the feasibility of using a clinical computer-controlled earth-vertical axis rotational chair to assess the vertical semicircular canals using whole-body rotational impulses. The angular motion during the impulsive portion of the stimulus was comparable to that used for manual head impulse testing. The overall chair motion was the same as that used for horizontal semicircular canal testing using the crHIT-horizontal [6]. Subjects were oriented such that one parallel set of vertical semicircular canals was in the earth-horizontal plane. That is, the right anterior – left posterior (RALP) or the left anterior – right posterior (LARP) plane was oriented perpendicular to the earth-vertical axis of rotation, while the plane of the opposite plane vertical semicircular canals was parallel to the axis of rotation. We evaluated two control subjects and four patients with a surgically confirmed peripheral vestibular abnormality. This study was approved by the Institution Review Board of the University of Pittsburgh. All participants provided written informed consent before being enrolled in the study.
2.1Subjects
The subjects for this preliminary study of the crHIT-vertical consisted of two normal control subjects, one female aged 24 years and one male aged 25 years with no history of dizziness or disequilibrium and normal vestibular laboratory testing including ocular motor testing, caloric testing, positional testing, earth-vertical axis rotational testing, and cervical vestibular evoked myogenic positional testing. Pertinent details regarding the four patients are given in Table 1.
Table 1
Patient demographics
Patient demographics | ||||
Patient # | Age | Sex | Vestibular Abnormality | Time between Surgery and crHIT (years) |
1 | 52 | F | Left Superior Vestibular Nerve Section for Vestibular Schwannoma | 2.9 |
2 | 68 | M | Left Labyrinthectomy for Meniere’s Disease | 4.1 |
3 | 50 | F | Left Intracanalicular Vestibular Schwannoma Resection | 1.2 |
4 | 51 | F | Left Labyrinthectomy for Meniere’s Disease | 6.3 |
2.2Device
Whole-body rotational impulses were delivered using a Neuro-Otologic Test Center (NOTC) device supplied by Neurolign USA (Pittsburgh, PA), formerly Neuro-Kinetics Inc. (Pittsburgh PA). The rotational chair was computer-controlled and had a peak torque of 185 foot-pounds. Eye position was measured using head-mounted binocular infrared video-oculography with a resolution of 0.1° and a frame rate of 250 frames per second. The video-oculography goggles included an embedded 6-degree of freedom (DOF) sensor array to record angular head velocity and linear head acceleration. The rotational chair was surrounded by a light-proof and sound-reducing enclosure. The video-oculography goggles also contained a laser to display a visual target directly in front of the subject. Participants were secured using a 4-point shoulder belt system. For vertical semicircular canal testing each participant’s head was comfortably secured with either the RALP or the LARP plane aligned with the earth-horizontal plane using a molded foam pad that was preformed to include the occiput and cervical regions and a pad to secure the forehead (see Fig. 1). Details of the alignment process are described below.
Fig. 1
Subject seated in the computerized rotational head impulse test (crHIT) device. The subject is seated in the LARP testing position, which requires the LARP plane to be earth-horizontal because the axis of rotation of the crHIT device is earth-vertical.
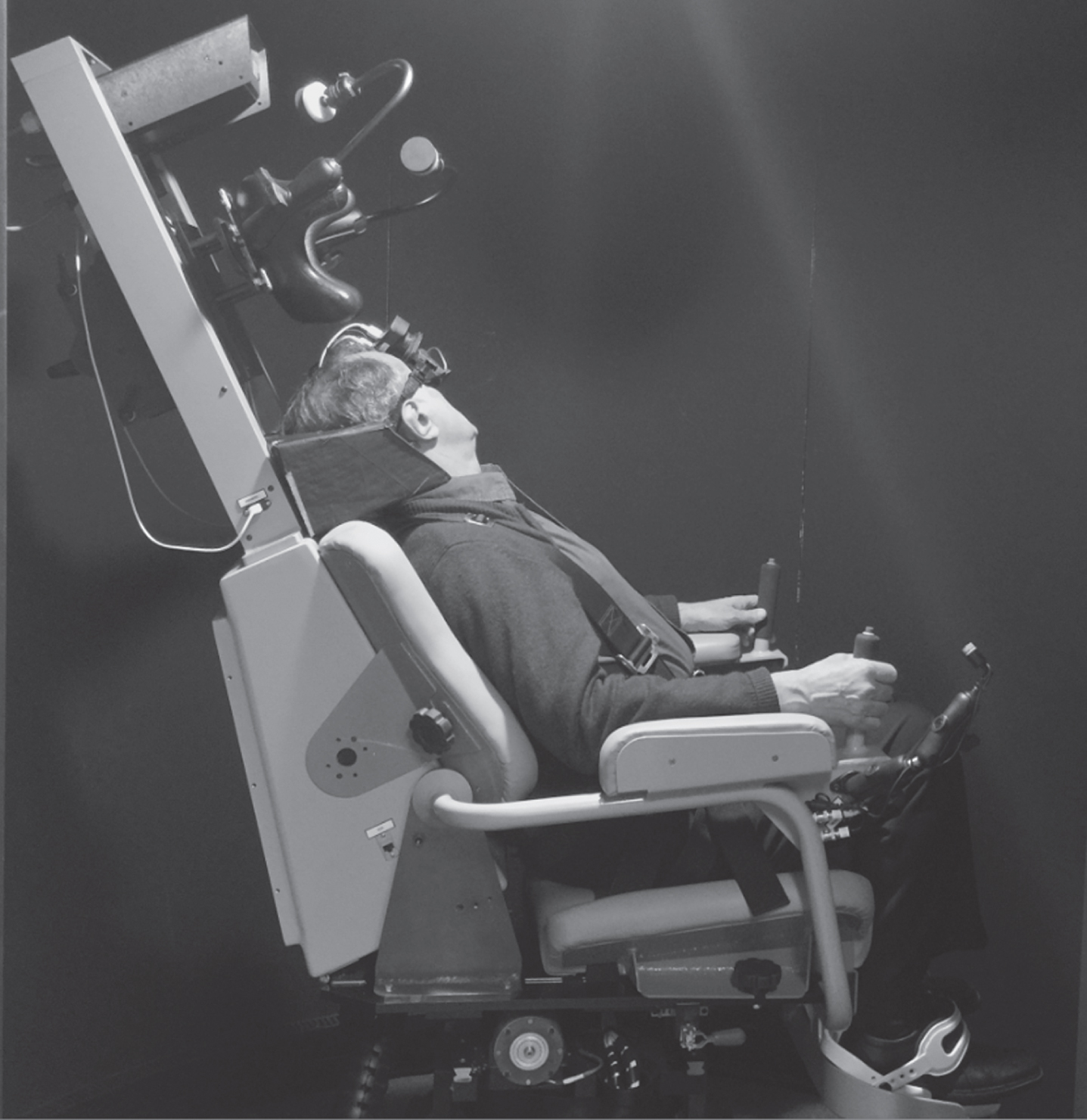
2.3Procedure
Each participant was first seated upright in the rotational chair and securely fitted with the infrared video goggles containing the 6-DOF sensor array. The orientation of the sensor array with respect to the subject’s anatomical skull landmarks was determined as follows. To determine the pitch axis orientation, the examiner used a level to orient the subject’s head such that Reid’s plane was earth-horizontal. Readings were taken of the pitch angle of the sensor array with respect to gravity and thus to Reid’s plane. Then, to determine the roll and yaw axes orientations, the subject was asked to pitch their head up and down at a frequency of about 0.1 Hz and approximately plus and minus 30°. This pitch plane head movement was monitored by the examiner administering the test to assure that the subject moved their head in the pitch plane. These data were then used to determine the orientation of the embedded 6-DOF sensor array to the subject’s sagittal plane. Note that the physical orientation of the goggles on the head was not altered based on data obtained from this procedure but rather, this procedure was used to process the data obtained during testing. Then, using published data regarding the orientation of the vertical semicircular canals with respect to Reid’s plane [4], the sensor array outputs were transformed into a nominal semicircular canal plane coordinate system.
Next, each subject’s head was oriented such that the horizontal semicircular canal plane was earth-horizontal. Testing for the horizontal semicircular canals followed the same protocol used in our earlier study [6]. Then, testing for the vertical semicircular canals began by reorienting the subject’s head such that either the RALP or LARP plane was earth-horizontal (see Fig. 1). This was achieved by pitching the subject’s torso back by about 30 degrees with respect to earth-vertical, then pitching the head back about 40 degrees with respect to the torso and rotating the head in the yaw plane by about 45°. To expedite this process, the examiner was provided with continuous data regarding the orientation of the subject’s RALP or LARP plane with respect to the earth-horizontal plane. When the subject’ s RALP or LARP plane was within 4.0 degrees of earth-vertical, the subject’s head was comfortably and securely restrained. When the subject was in this position, the axis of rotation of the rotational chair was approximately 30 centimeters anterior to the inter-aural line.
Then, the video-oculography goggles were calibrated separately for RALP and LARP rotations as follows. A stationary LED target was affixed to the interior wall of the enclosure in the subject’s primary position at approximately 1 m from the head. The alignment was confirmed using a laser projected from the video goggles that was switched off after being used to locate primary position. The subject was then rotated sinusoidally about an earth-vertical axis at a frequency of 0.3 Hz with an amplitude of +/–9° while the earth-fixed LED target remained illuminated, and the subject looked at the target. This frequency was selected so that the gain of the visually enhanced VOR gain would be nearly 1.0. This position excursion was selected to be comparable to the amount of vertical eye movement elicited during vertical semicircular canal head impulses, i.e., about+/–6°. In this way, the eye velocity calibration was valid for the vestibular testing in this study. The subject’s eye movement was a complex combination of horizontal, vertical, and torsional eye movement because of the angular orientation and linear location of the axis of rotation with respect to the head, the angular orientation and linear location of the eyes with respect to the labyrinth, and the use of 2-dimensional video-oculography to measure 3-dimensional rotation of the eyes. Assuming a visual-VOR gain of 1.0 for this low-frequency rotation, the head and eye movement data obtained during the calibration procedure were used to compute the relationship between the velocity of vertical eye movement and the velocity of the head in the head pitch plane. This computation was based on best-fit sinusoids using least squares for both vertical eye velocity, which was determined by digital differentiation of the eye position data, and pitch head velocity, determined by the adjusted data from the 6-DOF head movement sensor. Figure 2 illustrates an example of eye and head movement during the video-oculography calibration procedure. For the small position excursions used in this calibration procedure, the vertical eye position recorded by the video goggles was nearly sinusoidal with a harmonic distortion of only 2.7 %.
Fig. 2
Eye movement and head movement during vertical video-oculography calibration. A. Vertical eye position (solid line) and pitch head position (dotted line) during vertical eye movement calibration. Note that the scaling on the ordinate is nominal for vertical eye position assuming a visual-vestibulo-ocular reflex gain of 1.0 such that vertical eye position matches the pitch component of head rotation, which was based on the integral of pitch head velocity recorded by the 6-DOF sensor array embedded in the video goggles shown in panel B. B. Vertical eye velocity (solid line) derived from the eye position data illustrated in panel A and pitch head velocity (dotted line) recorded by the 6-DOF sensor embedded in the video googles. Note that the scaling on the ordinate is nominal for vertical eye velocity assuming a visual-vestibulo-ocular reflex gain of 1.0 such that vertical eye velocity matches the pitch component of head rotational velocity. The eye and head movement data shown in this calibration were recorded from the right eye during a RALP plane calibration.
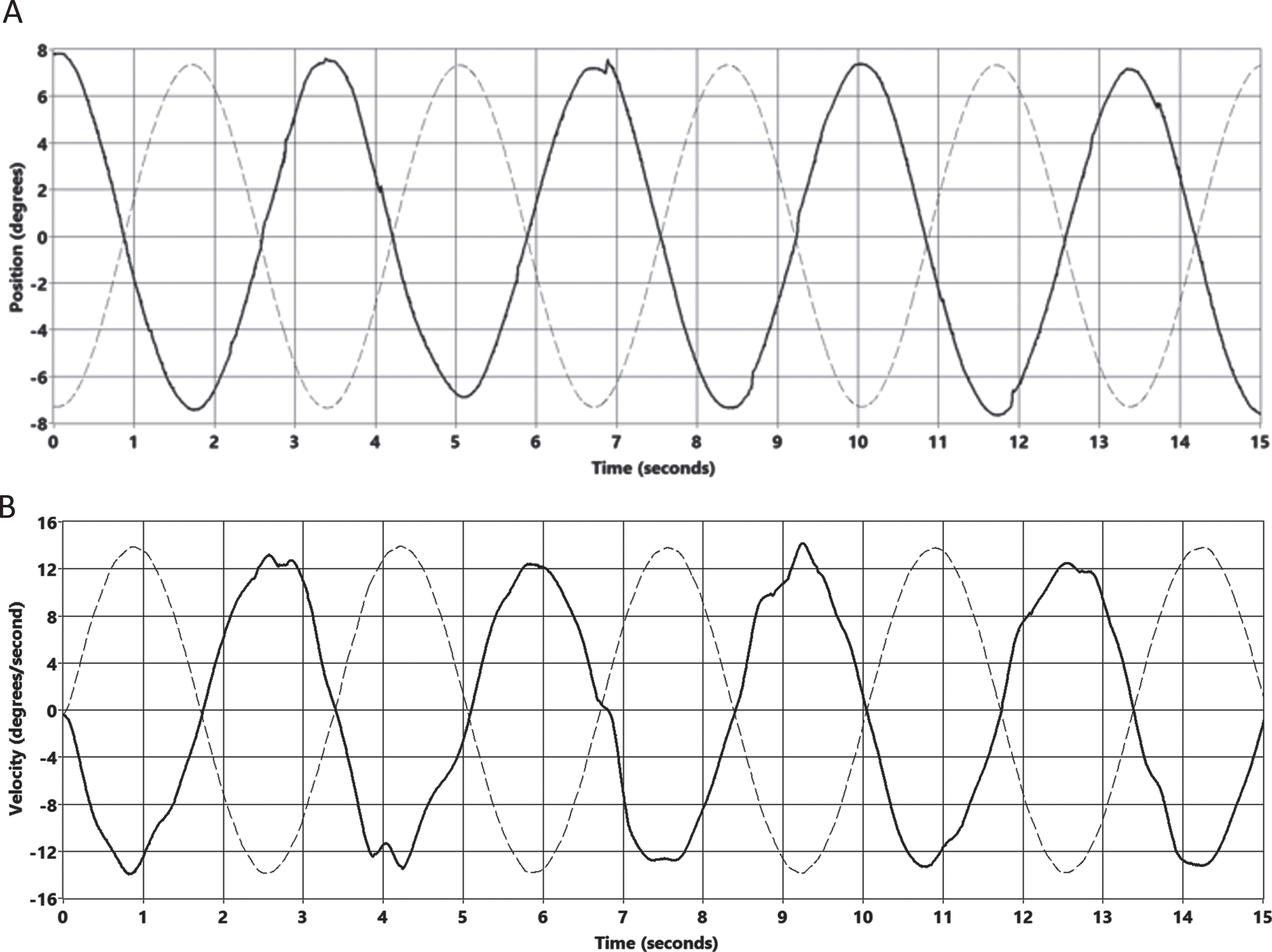
Then, each subject underwent RALP (and then LARP) plane whole-body rotational impulses. Each impulse consisted of an “S” shaped velocity profile (see Fig. 3) based on the built-in LabView (National Instruments, Austin, TX HQ. United States.) “error function”. Based on our preliminary data regarding the minimum acceleration required to identify a unilateral peripheral reduction [5] and typical peak head velocity for vHIT [10], the stimulus trajectory achieved a desired peak velocity of about 150°/s and average peak acceleration of about 750°/s/s. The nominal peak acceleration of the movement was calculated as the slope of a best fit line through the center 25% of the calculated velocity “S-curve”. This velocity profile achieved the desired stimulus intensity while minimizing subject discomfort caused by excessive jerk, i.e., change in acceleration, associated with the onset of chair rotation. The rotational chair remained at peak velocity for about one second after which the chair was gradually decelerated until it stopped (See Fig. 3).
Fig. 3
Position velocity, and acceleration profiles during and after a whole-body rotational impulse in the LARP plane. For this impulse, the peak velocity was 150 deg/sec, and the peak acceleration was 425 deg2. Note the gradual deceleration of the rotational motion following the impulse. The total position excursion for this trial was about 280 degrees. The portion of the impulse used for data analysis began at time 0.18 seconds, when the head velocity reached 40 deg/sec, and ended at or before (if there was a saccade) the time at which velocity reached 90% of peak velocity, which are denoted by vertical dotted lines. For this impulse, the data available for processing were between time 0.18. sec and 0.43 secs.
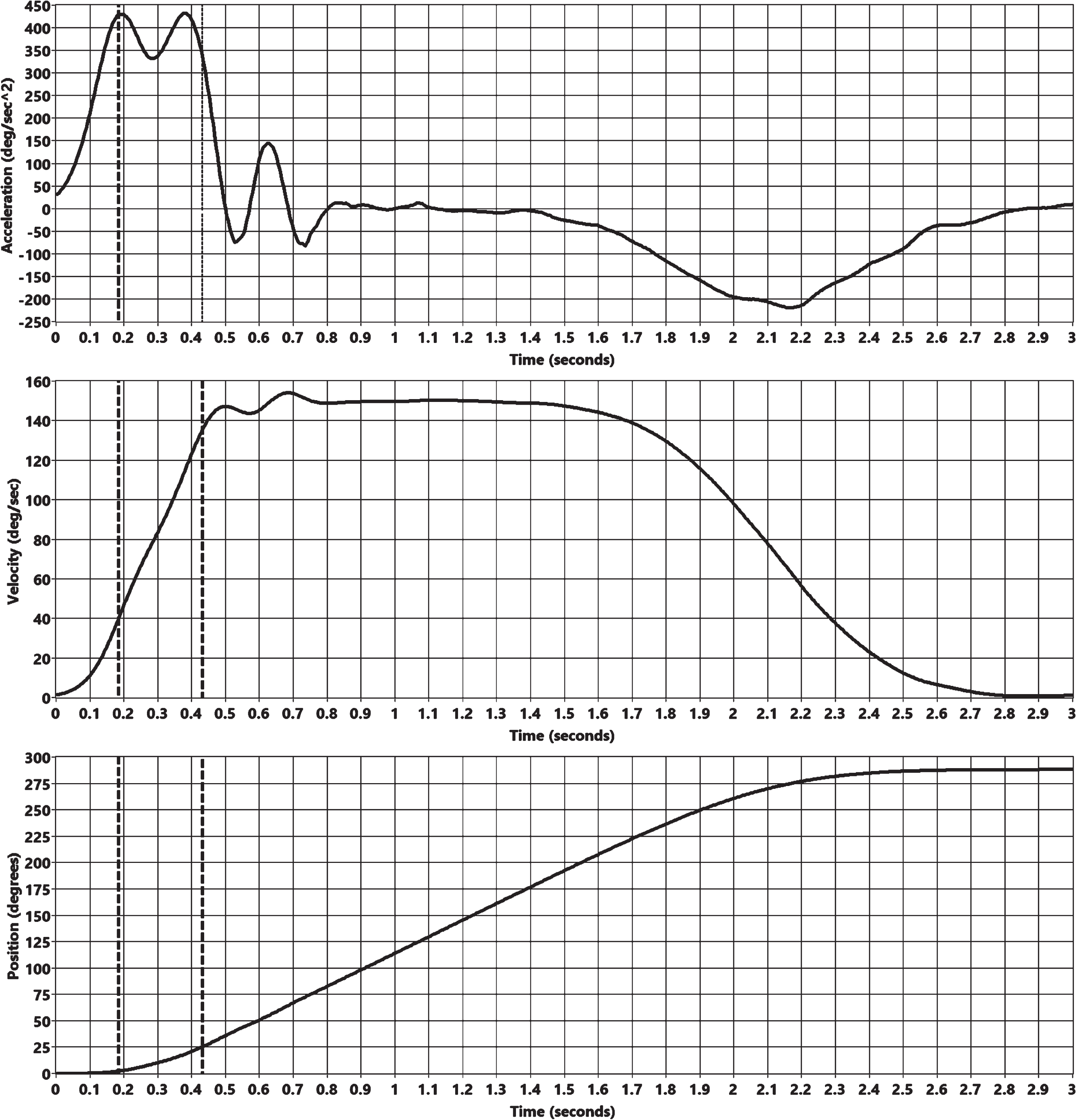
Fig. 4
Representative vertical semicircular canal plane impulses and their eye movement responses using crHIT. Pitch head velocity and vertical eye velocity data are shown for impulses in each of the vertical semicircular canal planes for two patients with surgically confirmed unilateral peripheral vestibular lesions and for one control subject. Head velocity is shown as a black line. Eye velocity is shown as a gray line. The vertical dotted line for each impulse indicates the end of the region of the impulse that was used for the computation of vestibular sensitivity. Data from Patient #1 are shown in panels A, D, G, and J. Data from Patient #2 are shown in panels B, E, H, and K. Data from Control subject #1 are shown in panels C, F, I and L.
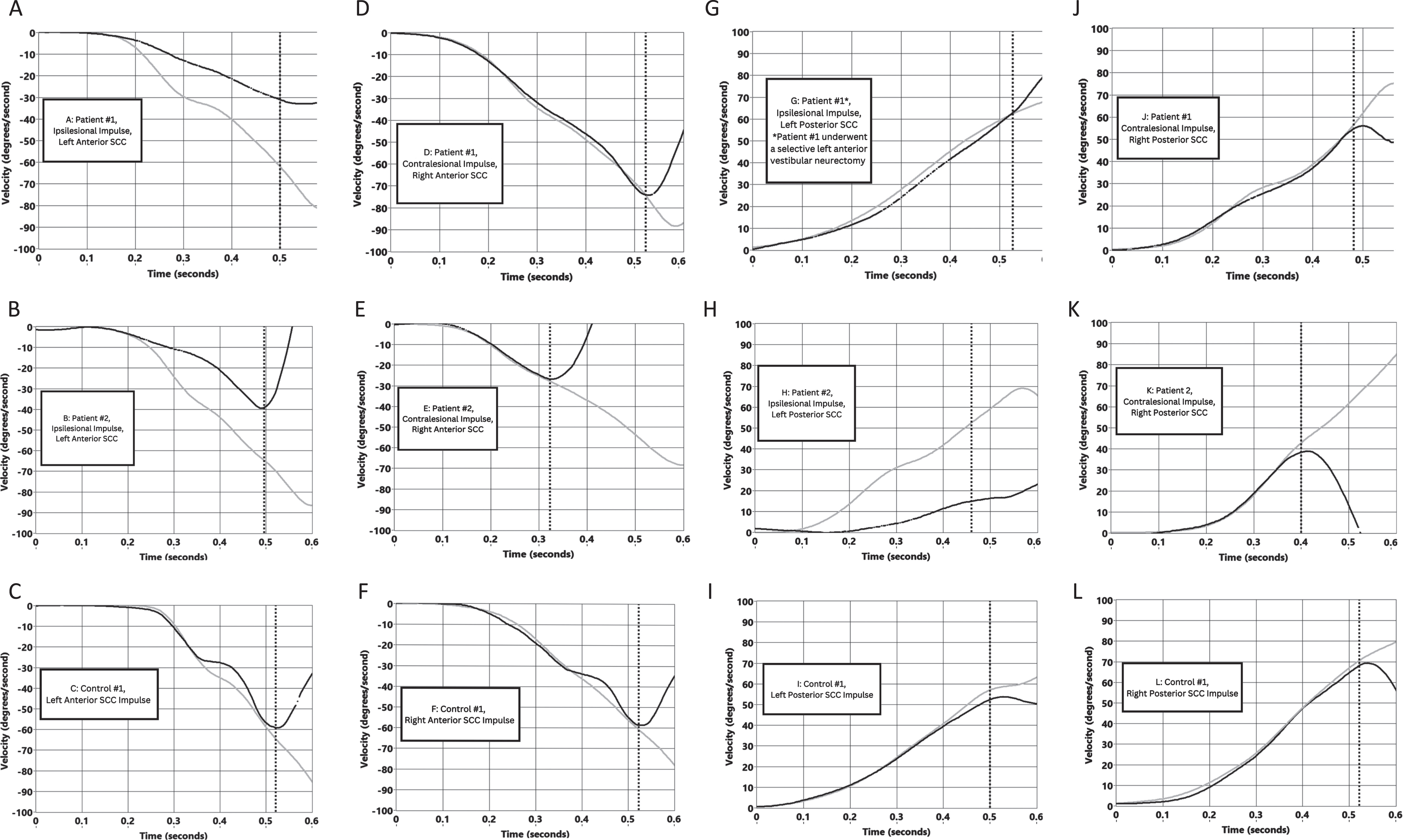
Prior to and during each impulse, the subject was asked to look at the same earth-stationary LED target used for calibration, which was positioned initially in the primary position. For each impulse, the rotational chair was slowly positioned 15 degrees in the opposite direction of the planned impulse. With the LED target illuminated, the subject was rotated using the trajectory shown in Fig. 3 for each rotational impulse. Impulses were delivered in a series of 18 impulses. The time of onset of the stimulus following the pre-positioning was randomized from 300 to 500 milliseconds. The magnitude of the constant velocity portion of the rotational impulse varied from 140 to 160 deg/sec. The peak acceleration varied from 425 to 500 deg/sec2. Also, there were two types of catch trials interspersed in the series of rotations including 1) rotation in the expected direction but of much lower acceleration and velocity, not suitable for analysis and 2) rotations in the “wrong” direction, i.e., rotation in the same direction as the pre-positioning movement. For each of the RALP and LARP planes, there were 2 catch trials.
2.4Data analysis
The eye movements recorded by the video oculography goggles, and the head movements measured by the 6-DOF sensor array were processed to yield a vestibular sensitivity value for each vertical semicircular canal. This sensitivity value was not true VOR gain as only the vertical component of the eye movement and only the pitch component of the head movement were used for data analysis. The eye and head movement data were analyzed during the impulse, beginning when the chair velocity exceeded zero and ending when the chair velocity reached 90% of the peak velocity for that trial or when a saccade occurred based on a vertical eye acceleration of 3000 deg/sec2. Vertical semicircular canal sensitivities were computed for each of the four vertical semicircular canals using the ratio of vertical eye position excursion to pitch head position excursion. We also evaluated VOR gain for the horizontal semicircular canals. This testing used the methods described in Furman et al. 2016 [6]. Asymmetries for the sensitivities of the vertical semicircular canals and for the gains of the horizontal semicircular canals were computed using the ratio of the left minus the right sensitivity or gain difference and the left plus the right sensitivity or gain sum.
3Results
The rotational impulses were well-tolerated by all of the subjects for testing both the vertical and horizontal semicircular canals. VOR gains and sensitivities for four patients and the two control subjects are shown in Table 1. The vertical semicircular canal asymmetries for the patients ranged from 15% to 53% excluding the asymmetry for the posterior semicircular canals for patient #1, who had a selective superior vestibular nerve section. Gain asymmetries for the horizontal semicircular canals ranged from 23% to 34%. Sensitivities for unaffected vertical semicircular canals ranged from 0.69 to 1.12 and for affected vertical semicircular canals ranged from 0.26 to 0.62. Gain asymmetries for horizontal semicircular canals ranged from 23% to 31%.
4Discussion
This report describes a new method for evaluating the vertical semicircular canals, the crHIT-vertical, based on the well-established head impulse test (HIT) [8], the video head impulse test (vHIT) [12], and the crHIT for horizontal semicircular canals [6, 15]. The bedside HIT was described by Halmagyi and Curthoys [8] as a means of assessing horizontal semicircular canal function unilaterally using yaw head accelerations of 2000–5000°/s/s, which were presumed to silence the contralateral semicircular canal afferents. Subsequently, the vHIT [12] has become routinely available taking advantage of computer-monitored head movement and video-oculography. The crHIT for assessing the vertical semicircular canals (crHIT-vertical) described in this report uses methodology comparable to that of the crHIT for testing the horizontal semicircular canals [6, 15] i.e., using whole-body rotation in a computer-controlled rotational chair while eye position is recorded with video-oculography. Whereas testing of the horizontal semicircular canals with the crHIT yields a measure of VOR gain, testing for the vertical semicircular canals with the crHIT-vertical yields measures of vestibular sensitivities, which are comparable to but not the same as true VOR gain.
Table 2
Semicircular Canal Vestibular Sensitivities and % Left-Right Asymmetries
Subject | Side of | Semicircular Canal Vestibular Sensitivities and % Left-Right Asymmetries | ||||||||
Peripheral Vestibular Abnormality | Left Horizontal | Right Horizontal | % Left-Right Asymmetry | Left Anterior | Right Anterior | % Left-Right Asymmetry | Left Posterior | Right Posterior | % Left- Right Asymmetry | |
Patient #1 | Left* | 0.62 | 0.98 | –23% | 0.62 | 0.94 | –21% | 0.92 | 1.09 | –8% |
Patient #2 | Left | 0.52 | 0.98 | –31% | 0.5‘ | 0.69 | –15% | 0.35 | 0.81 | –40% |
Patient #3 | Left | 0.52 | 0.87 | –25% | 0.53 | 0.88 | –25% | 0.29 | 0.80 | –51% |
Patient #4 | Left | 0.62 | 1.05 | –26% | 0.57 | 0.80 | –17% | 0.26 | 0.80 | –51% |
Control #1 | N/A | 1.01 | 1.00 | 0% | 0.91 | 1.00 | –5% | 1.00 | 1.03 | –1% |
Control #2 | N/A | 1.00 | 0.97 | 2% | 1.01 | 0.98 | 2% | 0.98 | 1.12 | –7% |
*Selective Left Superior Vestibular Nerve Section.
Several studies have advocated the vHIT as a means of assessing the vertical semicircular canals [1, 3, 11]. Despite these favorable reports, the use of the vHIT for assessing the vertical semicircular canals has not achieved widespread acceptance. A recent paper by Wittmeyer Cedervall et al. [19] suggests using the vHIT to assess the vertical semicircular canals presents several challenges and further development is required for using the vHIT for assessing the vertical semicircular canals reliably. We developed the crHIT-vertical to provide a laboratory-based method of reliably assessing individual vertical semicircular canal function clinically. Testing with the crHIT has the obvious disadvantage of requiring non-portable equipment housed in a dedicated laboratory space. Using the crHIT for assessing the vertical semicircular canals, like using the crHIT for testing the horizontal semicircular canals, overcomes many of the challenges associated with the manual vHIT [6] including 1) obviating the need for a highly trained examiner, 2) reducing the number of impulses necessary as each impulse is precisely defined, 3) reducing patient discomfort associated with head-on-torso rotation, especially in older patients and those with limited range of motion of the neck, 4) reducing the abruptness of the movement, thus reducing unpleasant jerk, and 5) avoiding the small position limitation of head-on-torso rotation, which is necessarily associated with an abrupt acceleration and deceleration of the head and limited data for each impulse. In addition to these advantages, which pertain to the crHIT for testing both the horizontal and vertical semicircular canals, for vertical semicircular canal testing in particular, the crHIT overcomes the problems of misalignment of the plane of rotation with the RALP and LARP planes.
The eye movement response to vertical semicircular canal plane stimulation is a gaze-dependent 3-dimensional combination of vertical, torsional, and horizontal rotations [11]. Thus, accurately measuring eye position with 2-dimensional video-oculography is challenging. Performing vertical semicircular canal testing during far lateral gaze to nearly align the plane of eye movement with that of the stimulated vertical semicircular canal plane can drastically reduce the contribution of torsional and horizontal movement. However, end-gaze nystagmus and interference by the eyelids with the video recording can degrade the images used for analysis of eye position. For this study, we chose to maintain the subjects’ eye position during the crHIT-vertical to a region around the primary position and used a visual-VOR calibration method to allow us to measure vertical semicircular canal sensitivities.
We chose a rotational velocity trajectory that was tolerable for the subjects and that likely reduced or eliminated the afferent vestibular nerve activity of the coplanar semicircular canal inhibited by the stimulus [7, 8, 16, 18, 20] Note that the peak acceleration of about 750°/sec/sec used for this study is considerably lower than that used for the bedside manual head-only vHIT. The absence of a head position constraint for the crHIT allowed us to use whole-body rotational impulses with a reduced jerk, i.e., with a reduced rate of change of acceleration, while maintaining a peak velocity magnitude comparable to that used for bedside head-only impulse testing, i.e., a peak velocity of about 150°/s. Although the crHIT is not constrained by head-on-torso limitations, the crHIT is still constrained by limitations of eye-in-head movement. We found that a peak acceleration of about 750°/sec/sec was comfortable for our subjects and, enabled us to identify the laterality of the patients’ abnormalities. An acceleration of 750°/s/s was previously found to be tolerable for assessing the horizontal semicircular canals using the crHIT [6].
For this study, we used the position gain analysis method described for the vHIT [2, 21]. Because we only used the vertical component of eye position and the pitch component of head position for assessing the vertical semicircular canals, our analysis yielded measures of semicircular canal sensitivities rather than actual VOR gain. Our calibration method was designed such that a sensitivity of 1.0 was directly comparable to a VOR gain of 1.0. The crHIT provides about 100–150 ms of eye and head movement data suitable for analysis. This duration is between two and five times greater than that obtained during the head-only vHIT. This preliminary study with a small number of subjects does not allow a reliable estimate of standard deviation or lower limits of normal for vertical semicircular canal sensitivity. Although the lower limit of normal for horizontal VOR gain using the crHIT is considerably higher than that found for the bedside vHIT [6, 12], further research will be required to determine normal limits for the crHIT when assessing the vertical semicircular canals and how these normal limits compare with those used when using the vHIT to assess the vertical semicircular canals.
As a component of this preliminary validation of the crHIT for assessing the vertical semicircular canals, we tested a single subject with a surgical unilateral superior vestibular nerve resection (patient #1). Our data clearly indicated a reduced gain for the ipsilesional horizontal semicircular canal and a reduced sensitivity for the ipsilesional anterior semicircular canal, both innervated by the superior vestibular nerve but a normal sensitivity for the ipsilesional posterior semicircular canal, which is innervated by the inferior vestibular nerve. For the three other patients in this study, each of whom had a surgical unilateral peripheral vestibular loss, ipsilesional gain for the horizontal semicircular canals and sensitivities for the vertical semicircular canals clearly identified the unilateral peripheral vestibular loss.
This preliminary evaluation of the crHIT for assessing the vertical semicircular canals suggests that the crHIT, despite its cost and requirement for a dedicated clinical laboratory space, is worthy of further development as a laboratory-based method of assessing the vertical semicircular canals. In addition to becoming a useful laboratory-based test, the crHIT could be used to help improve the testing technique and response analysis of the vHIT when assessing the vertical semicircular canals.
References
[1] | Abrahamsen E.R. , Christensen A.E. and Hougaard D.D. , Intra- and interexaminer variability of two separate video head impulse test systems assessing all six semicircular canals, Otol Neurotol 39: (2) ((2018) ), e113–e122. https://doi.org/10.1097/MAO.0000000000001665 |
[2] | Cleworth T.W. , Carpenter M.G. , Honegger F. and Allum J.H.J. , Differences in head impulse test results due to analysis techniques, J Vestib Res 27: (2-3) ((2017) ), 163–172. https://doi.org/10.3233/VES-170614 |
[3] | Cremer P.D. , Halmagyi G.M. , Aw S.T. , Curthoys I.S. , McGarvie L.A. , Todd M.J. , Black R.A. and Hannigan I.P. , Semicircular canal plane head impulses detect absent function of individual semicircular canals, Brain 121: (Pt 4) ((1998) ), 699–716. https://doi.org/10.1093/brain/121.4.699 |
[4] | Santina Della C.C., Potyagaylo V., Migliaccio A.A., Minor L.B. and Carey J.P., Orientation of human semicircular canals measured by three-dimensional multiplanar CT reconstruction, J Assoc Res Otolaryngol 6: (3) ((2005) ), 191–206. https://doi.org/10.1007/s10162-005-0003-x |
[5] | Furman J.M. , Roxberg J. , Shirey I. and Kiderman A. , The vertical computerized roational head impulse test (crHIT-vertical) Vestibular-Oriented Research meeting The Ohio State University May 20–22, (2019) . |
[6] | Furman J.M. , Shirey I. , Roxberg J. and Kiderman A. , The horizontal computerized rotational impulse test, J Vestib Res 26: (5-6) ((2016) ), 447–457. https://doi.org/10.3233/VES-160595 |
[7] | Halmagyi G.M. , Chen L. , MacDougall H.G. , Weber K.P. , McGarvie L.A. and Curthoys I.S. , The video head impulse test, Front Neurol 8: ((2017) ), 258. https://doi.org/10.3389/fneur.2017.00258 |
[8] | Halmagyi G.M. and Curthoys I.S. , A clinical sign of canal paresis, Arch Neurol 45: (7) ((1988) ), 737–739. https://doi.org/10.1001/archneur.1988.00520310043015 |
[9] | Halmagyi G.M. , Curthoys I.S. , Cremer P.D. , Henderson C.J. and Staples M. , Head impulses after unilateral vestibular deafferentation validate Ewald’s second law, J Vestib Res 1: (2) ((1990) ), 187–197. https://www.ncbi.nlm.nih.gov/pubmed/1670152 |
[10] | Halmagyi G.M. , Curthoys I.S. , Cremer P.D. , Henderson C.J. , Todd M.J. , Staples M.J. and D’Cruz D.M. , The human horizontal vestibulo-ocular reflex in response to high-acceleration stimulation before and after unilateral vestibular neurectomy, Exp Brain Res 81: (3) ((1990) ), 479–490. https://doi.org/10.1007/BF02423496 |
[11] | Macdougall H.G. , McGarvie L.A. , Halmagyi G.M. , Curthoys I.S. and Weber K.P. , The video Head Impulse Test (vHIT) detects vertical semicircular canal dysfunction,, PLoS One 8: (4) ((2013) ), e61488. https://doi.org/10.1371/journal.pone.0061488 |
[12] | MacDougall H.G. , Weber K.P. , McGarvie L.A. , Halmagyi G.M. and Curthoys I.S. , The video head impulse test: diagnostic accuracy in peripheral vestibulopathy, Neurology 73: (14) ((2009) ), 1134–1141. https://doi.org/10.1212/WNL.0b013e3181bacf85 |
[13] | McGarvie L.A. , Lopez Martinez-M. , Burgess A.M. , MacDougall H.G. and Curthoys I.S. , Horizontal eye position affects measured vertical VOR gain on the video head impulse test, Front Neurol 6: ((2015) ), 58. https://doi.org/10.3389/fneur.2015.00058 |
[14] | Migliaccio A.A. , Schubert M.C. , Clendaniel R.A. , Carey J.P. , Santina Della C.C., Minor L.B. and Zee D.S., Axis of eye rotation changes with head-pitch orientation during head impulses about earth-vertical, J Assoc Res Otolaryngol 7: (2) ((2006) ), 140–150. https://doi.org/10.1007/s10162-006-0029-8 |
[15] | Mittendorf N. , Reddy T.M. , Heinze B. , Kiderman A. and Gonzelez J. , Test-retest reliability of the computerized rotational head impulse test in the pediatric population, Internaional Journal of Otorhinolaryngology and Head and Neck Surgery 9: (1) ((2023) ), 5–11. |
[16] | Peterka R.J. , Pulse-step-sine rotation test for the identification of abnormal vestibular function, J Vestib Res 15: (5-6) ((2005) ), 291–311. https://www.ncbi.nlm.nih.gov/pubmed/16614475 |
[17] | Seo Y.J. , Park Y.A. , Kong T.H. , Bae M.R. and Kim S.H. , Head position and increased head velocity to optimize video head impulse test sensitivity, Eur Arch Otorhinolaryngol 273: (11) ((2016) ), 3595–3602. https://doi.org/10.1007/s00405-016-3979-4 |
[18] | Weber K.P. , Aw S.T. , Todd M.J. , McGarvie L.A. , Curthoys I.S. and Halmagyi G.M. , Head impulse test in unilateral vestibular loss: vestibulo-ocular reflex and catch-up saccades, Neurology 70: (6) ((2008) ), 454–463. https://doi.org/10.1212/01.wnl.0000299117.48935.2e |
[19] | Wittmeyer Cedervall L. , Magnusson M., Karlberg M., Fransson P.A., Nystrom A. and Tjernstrom F., vHIT testing of vertical semicircular canals with goggles yield different results depending on which canal plane being tested, Front Neurol 12: ((2021) ), 692196. https://doi.org/10.3389/fneur.2021.692196 |
[20] | Wuyts F. , Principle of the head impulse (thrust) test of Halmagyi Head Thrust Test (HHTT), B-ENT 4: (Suppl8) ((2008) ), 23–25. |
[21] | Zamaro E. , Saber Tehrani A.S. , KattahJ.C., EibenbergerK., GuedeC.I., ArmandoL., CaversaccioM.D., Newman-TokerD.E. and MantokoudisG., VOR gain calculation methods in video head impulse recordings, J Vestib Res 30: (4) ((2020) ), 225–234. https://doi.org/10.3233/VES-200708 |