Disruption of self-motion perception without vestibular reflex alteration in Ménière’s disease
Abstract
BACKGROUND:
Self-motion misperception has been observed in vestibular patients during asymmetric body oscillations. This misperception is correlated with the patient’s vestibular discomfort.
OBJECTIVE:
To investigate whether or not self-motion misperception persists in post-ictal patients with Ménière’s disease (MD).
METHODS:
Twenty-eight MD patients were investigated while in the post-ictal interval. Self-motion perception was studied by examining the displacement of a memorized visual target after sequences of opposite directed fast-slow asymmetric whole body rotations in the dark. The difference in target representation was analyzed and correlated with the Dizziness Handicap Inventory (DHI) score. The vestibulo-ocular reflex (VOR) and clinical tests for ocular reflex were also evaluated.
RESULTS:
All MD patients showed a noticeable difference in target representation after asymmetric rotation depending on the direction of the fast/slow rotations. This side difference suggests disruption of motion perception. The DHI score was correlated with the amount of motion misperception. In contrast, VOR and clinical trials were altered in only half of these patients.
CONCLUSIONS:
Asymmetric rotation reveals disruption of self-motion perception in MD patients during the post-ictal interval, even in the absence of ocular reflex impairment. Motion misperception may cause persistent vestibular discomfort in these patients.
1Introduction
Clinical vestibular examination routinely consists of testing reflexes and not motion perception, although dizziness or imbalance are perceived by many individuals, even in the absence of any vestibular reflex impairment [20]. This perceptual and reflex dissociation can be explained by considering that vestibular perception and reflexes encompass different pathways [2, 14, 16] and central processing [5, 22, 25–27, 29–32, 34, 35]. Therefore, it is conceivable that distinct perceptual and reflex adaptive mechanisms can occur after labyrinthine deficit, inducing imbalance in the vestibular circuitry [3, 4, 23]. The lack of perception examination may represent a relevant limitation when evaluating all of the patient’s symptoms.
To demonstrate deficit in perceptual function, some methods have recently been described that include responses to rotation and translation [6] and also response to asymmetric rotation [23]. Asymmetric vestibular stimulation has been found to be very successful in revealing perceptual imbalance and differentiating it from the vestibulo-ocular reflex (VOR). This method is based on examining motion perception during asymmetric vestibular stimulation. It has been observed that, in healthy individuals, repetitive asymmetric rotation (fast rotation toward one side and slow rotation in the opposite direction) induces opposite effects in self-motion perception and VOR. Clearly, at the beginning of the stimulation, both perceptual and reflex responses are largely asymmetric, but adaptive mechanisms tend to cause increased asymmetry in the perceptual responses and reduce that of the VOR [26]. By reverting the direction of the asymmetric stimulation, the perceptual asymmetries are similar in healthy individuals, while in the presence of unilateral vestibular deficit they are remarkably different, suggesting that this stimulus can be very effective in revealing imbalance in the vestibular perceptual circuit. Indeed, using this particular stimulation in patients with vestibular neuritis, a residual imbalance in the perceptual circuit has been seen, even in the absence of any VOR asymmetry [23]. In contrast, perceptual imbalance is not observed after symmetric vestibular stimulation. Interestingly, the amount of the difference in perceptual asymmetry is well correlated with the subjective perception of dizziness as shown by the Dizziness Handicap Inventory (DHI) tests.
This evidence prompted us to investigate whether perceptual dysfunction may be present in other vestibular pathologic conditions, where reflex alterations may be absent, such as after transient vestibular damage. A typical condition in which vestibular reflex impairment is acutely altered occurs in the early stage of Ménière’s disease (MD) during post-ictal periods before permanent sensorineural damage occurs [17–19].
This study aims to compare the VOR and other clinical tests for ocular reflex with the perceptual responses to asymmetric and symmetric rotation during the post-ictal period.
2Methods
Twenty-eight consecutive patients with Ménière’s disease were enrolled in a prospective study. The diagnosis was made before this study between 1 September 2015 and 31 December 2018. The patients were evaluated for vestibular perception and reflex in the subsequent period between 1 April 2019 and 30 June 2019, during clinical control sessions which were performed every 2 months after MD diagnosis. Any symptoms or Ménière’s crisis occurring during the previous 2 months were reported. In general, the interval between the last vertiginous crisis and the patient’s examination varied from a minimum of 1 week to a maximum of 60 days. All of the procedures performed in studies involving human participants followed the ethical standards of the institutional research committee (the 1964 Helsinki declaration and its later amendments or comparable ethical standards). The ethics committee of the University of Perugia approved this study. Informed consent was obtained from all individual participants included in the work.
There were 16 women (20–45 years) and 12 men (25–50 years) (mean age 38±14.5 years) in the study. Inclusion criteria were based on the signs and symptoms of actual MD according to the Classification Committee of the Bárány Society (2015) [17]. Patients over the age of 50 years were not included because the test was very demanding (the imaginary pursuit of a visual target, see below) that required prolonged training sessions which older patients were less able to execute. We chose to verify the efficacy of the asymmetric perceptual test in younger individuals who could easily perform the test, before extending the study to the elderly.
All patients enrolled in the study were under the same medical therapy with betahistine (48 mg/die). Individuals experiencing migraine or previous/concomitant vestibular disorders of other types (e.g. vestibular neuritis) or different therapies (e.g. gentamicin) were excluded. In addition, we examined the perceptual responses to vestibular rotation in 28 unaffected individuals (controls) matched for age and sex (20–50 years old; mean 39±11.3 years), to establish the reference level for the new protocol of the motion perception test.
2.1Vestibular testing
Vestibular stimulation. The patients sat on a computer-controlled chair (fixed on a platform) rotating in the horizontal plane in an acoustically isolated cabin of 150 cm radius. One hand was placed on their chest and the other hand on the pointer used for tracking a memorized earth-fixed visual target. A vertical holder with a horizontal extension secured the individual’s head to the chair in the primary position.
We used a camera recording two pairs of reflective infrared markers placed on the midline scalp, and left and right acromion, to measure head yaw rotation. When the head markers revealed displacements unrelated to the stimulus, the trial was discarded. A plastic collar prevented roll and pitch head displacements.
The chair oscillated sinusoidally in the dark with a half cycle at different velocities (Fig. 1). The patient was rotated rapidly to one side and then returned slowly to the original position [same amplitude (40°) but different frequencies: fast half cycle (FHC) = 0.38 Hz, peak acceleration 120°/s2, peak velocity 47°/s; slow half cycle (SHC) = 0.09 Hz, peak acceleration 7°/s2, peak velocity 11°/s above thresholds for vestibular activation]. We used the asymmetric rotation protocol since we have shown in earlier studies [22, 23, 26, 27] that it was efficacious to reveal side difference in self-motion perception, whereas symmetric rotation was not able to show perceptual imbalance [8, 29]. A sequence of four cycles of asymmetric rotation was delivered with fast rotation toward one side and, thereafter, in a successive sequence, with fast rotation toward the other side. Depending on whether the fast rotation was delivered toward the lesion or unaffected side, the motion perception was remarkably different [8, 29] (Fig. 2). Symmetric stimulation was also delivered to patients [amplitude 40°, frequencies of 0.38 Hz and 0.09 Hz] to show the effectiveness of the asymmetric rotation protocol in revealing self-motion perceptual imbalance.
Fig. 1
Experimental setting and motion perception recording. A: Schematic drawing of the experimental setting. Acoustically isolated cabin and rotating chair: P: pointer, T: visual target, presented just before rotation in the dark, H: head holder. The solid arrows indicate the chair asymmetric rotation (solid arrow: fast rotation; dashed arrow: slow rotation). The representation of the memorized visual target at the end of four cycles of asymmetric rotation is shifted (dashed arrow) in the opposite direction to the fast rotation. B: Motion perception tracking in response to four cycles of asymmetric rotation. Upper trace: Tracking of the memorized visual target during rotation revealed by the pointer. The filled circle indicates the target position representation at the end of rotation. Lower trace: chair asymmetric rotation. Note the error (TPE) induced by asymmetric stimulation with respect to the real position of the target (vertical dashed line).
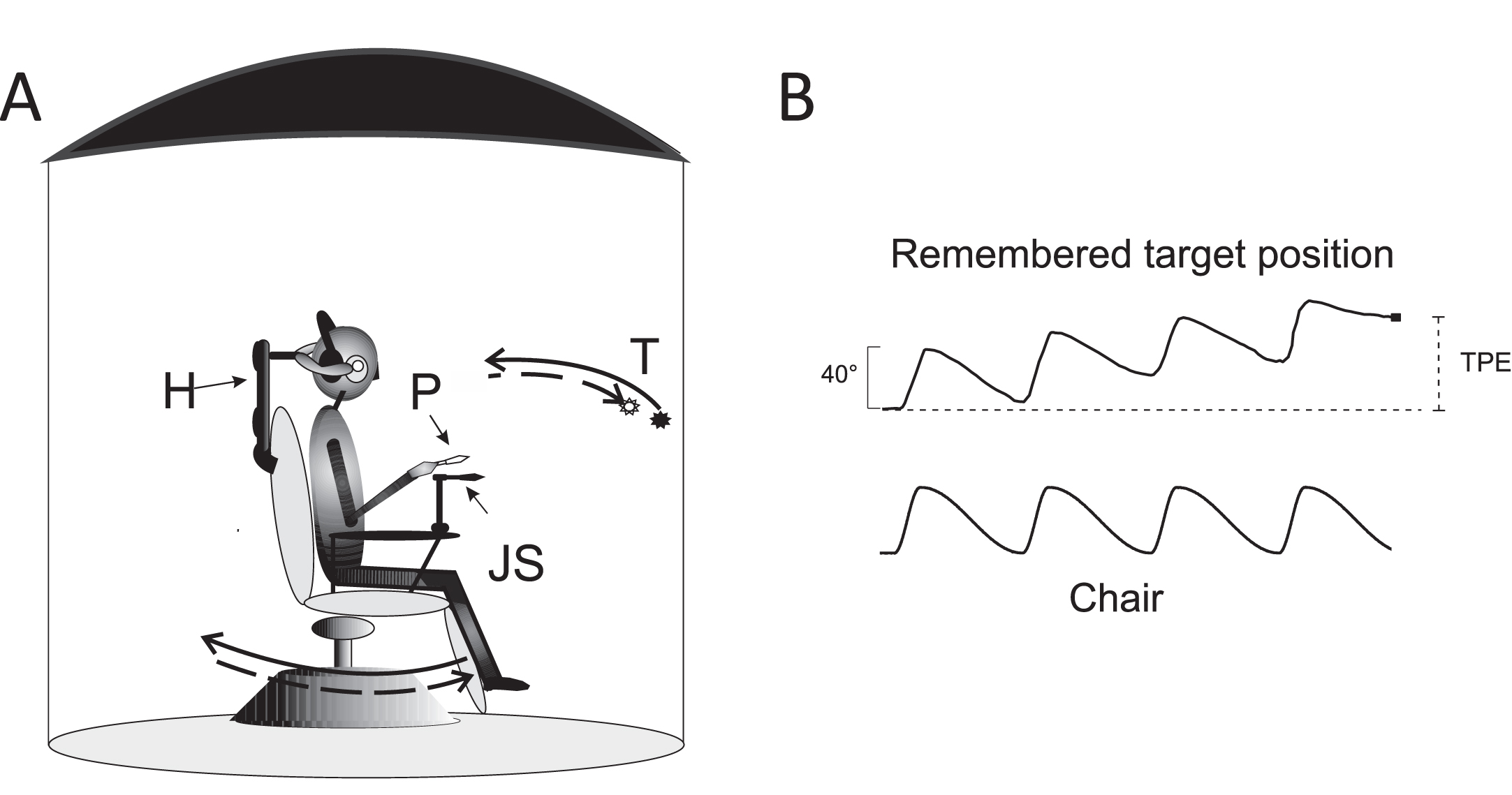
Fig. 2
Tracking position error after opposite directed asymmetric rotation and asymmetric index in unaffected and MD patients. A,B: tracking of memorized visual target during asymmetric rotation. Upper trace: chair rotation; lower trace: tracking of memorized target of unaffected (dashed line) and MD patients with vestibular functional deficit (solid line). The filled circles and vertical bars indicate the final tracking position error (TPE) on the right (solid line: MD patients; dashed line: controls). In A, the fast rotation was directed toward the lesion side; in B, the fast rotation was toward the healthy side. C: TPE (mean and SD) of 28 unaffected (control, filled circles) and 28 MD patients (open circles) in response to asymmetric rotation. The TPE values induced by a fast half cycle to the healthy or irritated side (left side) were greater than those in response to a fast half cycle to the lesion or non-irritated side (right side). D: Perceptual asymmetric index (mean and SD) of control (filled circles) and MD patients (open circles) in response to asymmetric rotation.
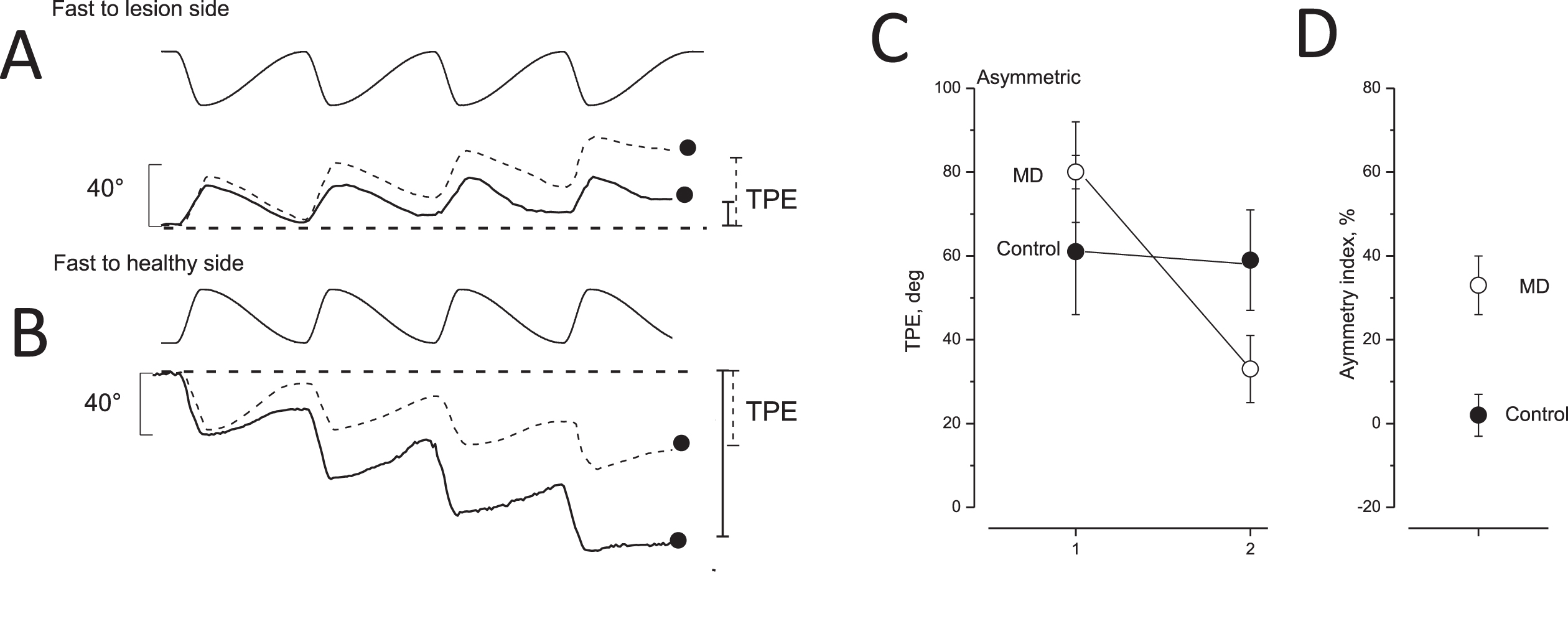
2.2Test for self-motion perception
We used a psychophysical tracking procedure to assess self-motion perception [33, 34]. Before starting the rotation, patients were asked to look at a target placed in front of them, and they had to imagine the target throughout the rotations executed in the dark and with eyes closed. The target was represented by a light spot (1 cm diameter) projected onto a wall of the cabin at the level of the patients’ eyes (Fig. 1). The spot was switched off just before rotation onset. Patients were instructed to continuously track the memorized spot in the dark by rotating the hand pointer toward that spot. The pointer, connected to a precision potentiometer pivoted on a chair-fixed support, allowed the continuous recording of the angular displacement. Moreover, the pointer had a laser beam that was turned on at the end of rotation to measure the angular distance between the projection of the beam on the wall and the light that was switched on.
Signals from the pointer and chair motion were digitized using a 12-bit analog-to-digital card (LabVIEW, National Instruments, Austin, TX, USA) at a sampling rate of 500 Hz and stored for offline analysis. The memorized target position was evaluated directly by measuring the position of the laser beam on the wall and the real target position, while the manual tracking was recorded using the pointer position signal to verify the quality of self-motion during asymmetric rotation. The tracking response was accepted when it resembled the sinusoidal stimulus profile. Patients were trained to track the memorized target with their forearm during asymmetric rotation. Training was stopped when visual inspection of the tracking showed no further improvement and good matching of the stimulus waveform. Depending on the participant, 5–10 trials were required. On the following day, the patients underwent the test for self-motion perception. We discarded responses when arm movements during rotation showed discontinuity, jerks, or pauses; inadequate tracking occurred in four patients and in 1–2 trials. After a 1-day interval, the VOR was examined during asymmetric rotation.
2.3Self-motion perception in response to asymmetric rotation
Due to the characteristic transfer function of the vestibular system and additional adaptive responses, patients perceived the FHC more vividly than the SHC [26]. Therefore, the different motion perceptions during fast and slow half cycles induced an erroneous representation of the memorized target position at the end of the session of four asymmetric cycles (tracking position error, TPE). The target was represented laterally with respect to the real target position in the opposite direction to that of the FHC (Fig. 1). In unaffected individuals, the TPE observed at the end of four asymmetric rotations was similar when the fast half cycle was directed to one side or toward the other side. In contrast, in vestibular-damaged patients, the TPE was different depending on the direction of the fast half cycle (FHC) (Fig. 2). Due to the effect of the direction of the intense activation within an unbalanced vestibular circuit, FHC to the healthy side (healthy-FHC) induced a greater TPE compared to that observed with FHC to the damaged side (damaged-FHC). The difference in the amplitude of TPEs was reported as the asymmetry index [(healthy-FHC TPE –damaged-FHC TPE/sum of the two TPEs) * 100]. This formula allows the perceptual side disparity to be calculated accurately with respect to the total observations [23]. On the other hand, in the case of the irritated condition, the formula was: (irritated-FHC TPE –healthy-FHC TPE/sum of two TPEs) * 100, with the irritated-FHC TPE as minuend. In this way, all values were positive.
2.4Test for VOR
In the same patients, we also recorded eye movements during asymmetric rotation in the dark, with bitemporal direct current electrooculography (DC-EOG) in a separate session 1 day later (Fig. 2). Signals from the EOG were digitized using a 12-bit A/D card (Labview, National Instruments) at a sampling rate of 500 Hz and stored for offline analysis. Quick nystagmic components were removed offline and the cumulative amplitude of the four half cycles of slow phase eye movement (cumulative slow phase eye position, SPEP) [23, 26] were computed for four cycles of asymmetric rotation (Fig. 3). Asymmetric rotation induced a large cumulative SPEP. The cumulative SPEP of the VOR was also evaluated after two opposite directed rounds of “four-cycle asymmetric rotations” to compute the asymmetry index that depended on the symmetry of the vestibular responsiveness of the two sides. To examine the VOR asymmetry, we used the cumulative SPEP: in the case of lesion: (healthy-FHC cumulative SPEP –damaged-FHC cumulative SPEP/sum of two cumulative SPEP) *100; in the case of irritation: (irritated-FHC cumulative SPEP –healthy-FHC cumulative SPEP/sum of two cumulative SPEP) * 100. Unaffected individuals had almost null side difference in the cumulative SPEP, while patients with vestibular imbalance had a marked difference.
Fig. 3
Eye movement recording during asymmetric rotation in response to opposite directed asymmetric rotation and asymmetric index in unaffected and MD patients. A, B: Slow phase eye position (SPEP) in response to opposite directed sequence of four cycles of asymmetric rotation with a fast half cycle toward the lesion side (A) and the healthy side (B). Upper traces: asymmetric rotation; and lower traces: SPEP (solid line: MD patients, dashed line: controls). Cumulative eye position at the end of rotation is indicated by vertical bars on the right (solid line: MD patients; dashed line: controls). Note that the cumulative SPEP of MD patients was smaller in A and greater in B compared with the controls. C: Cumulative SPEP (cSPEP) (mean and SD) of unaffected (control, filled circle) and MD patients (open circles) in response to opposite directed asymmetric rotation: fast half cycle to healthy or irritated side (left) versus fast half cycle to lesion or side not irritated (right). Note that controls did not show a different cumulative SPEP in contrast to MD patients. D: cSPEP asymmetry index in unaffected (control, filled circle) and in MD patients (open circles) in response to the opposite directed asymmetric rotation.
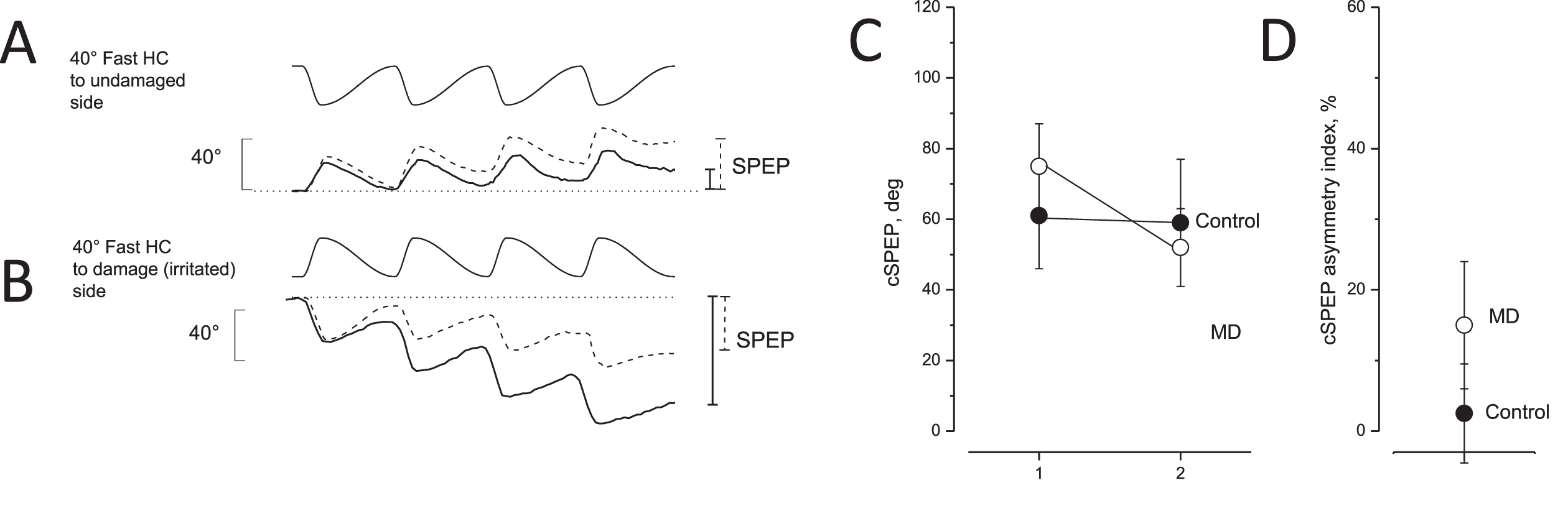
2.5Clinical vestibular tests
All patients underwent a bedside examination of vestibular function to reveal the presence of spontaneous and/or positional nystagmus and an audiometric study to verify the presence of hypoacusia which allowed the affected side to be established. Based on the directionality of response of each clinical test, the dysfunction was considered to be irritative for a functional prevalence of the affected side response or defective for a prevalence of healthy side response.
All patients underwent the following conventional tests: caloric test (CT), head shaking test (HST), v-head impulse test (vHIT) and vibration-induced nystagmus (VIN).
Caloric test: We followed the Fitzgerald-Hallpike method [7], and measured the peak slow phase eye velocity 60–90 seconds after irritation onset using EOG. The canal paresis values were evaluated by applying the Jongkees formula [11, 12]. The caloric test was considered to be pathological in the presence of canal paresis > 30 values.
Head shaking nystagmus test: We verified the presence of nystagmus after passive rotation of the head (±45° at 1 Hz for 20 seconds). The Head Shaking Test was considered to be positive if at least two evident post-rotation nystagmic beats with a peak slow phase eye velocity (SPEV) > 5 deg/s were observed [13].
v-head impulse test: We examined the asymmetry of the ocular responses using the GN-Otometrics vHIT system in response to 20 head impulses for stimulation on each side (10°–20° amplitude, velocity peak 150°/s and 250°/s). The v-HIT outcome was considered to be pathologic in the presence of 30%asymmetry values [1].
Vibration-induced nystagmus: The vibration (100 Hz for 10 seconds) was applied by a battery-powered device to one side of the mastoid process, and subsequently to the other side and to the vertex. The VIN test result was considered to be pathologic when per-stimulatory nystagmus was evoked during mastoid stimulation [21, 28].
2.6Audiometry
Pure tone audiometry was also performed to evaluate the acoustic threshold by delivering tones generated by a system for computer-controlled standard audiometry examination (P-A 1000, Inventis srl, Padova, Italy), driven by the interface software for the P-A 1000 (frequency range: 125 to 8000 Hz, signal/noise ratio > 70 dB, distortion 2.5%, accuracy of frequency > 1%). This device was assembled in combination with an Amplifon AM-13 calibrated clinical audiometer (Amplifon S.P.A, Milan, Italy). The intensity was increased in steps of 5 dB from the initial intensity of 0 dB SPL to 40 dB SPL maximum measured relative to the standard reference sound pressure level of 20μPa. The pattern was attributed to irritation of the labyrinthine receptors when the directional preponderance of the vestibular symptoms during an acute episode reflected a preponderant excitability on the side where the acoustic deficit was present, while the pattern was considered deficient when preponderant excitability was on the opposite side.
2.7Dizziness handicap inventory (DHI)
The DHI [10] was used to evaluate the functional, emotional and physical subjective perception of dizziness. The DHI comprises 25 items (questions) and three possible replies: “yes” (four points), “sometimes” (two points) and “no” (zero points) designed to assess a patient’s functional (nine questions), emotional (nine questions) and physical (seven questions) limitations. A score < 10 points indicated no handicap, scores between 16 and 34 points mild handicap, 36–52 moderate handicap, and 54–100 points severe handicap.
2.8Protocol
First, patients were examined using conventional clinical vestibular tests. Subsequently, all patients underwent a session for self-motion perception testing and 1 day later a session for VOR testing.
2.9Data evaluation and statistical analyses
Investigators who performed the data analysis were not aware of the research questions and were not the same investigators who tested the patients.
The control value for the asymmetry index was that measured in unaffected individuals in this study and in an earlier study on vestibular neuritis [23]. The responses were analyzed statistically using a Generalised Mixed Model Analysis (GLM) and a Bonferroni post-hoc test. P-values < 0.05 were considered statistically significant. Before GLM, the Shaphiro-Wilk W test) assessed normality and Levene’s homogeneity test assessed the variances. The confidence interval (CI) of the vestibular test values was defined as 95%of the values in our control group. After the last dizziness crisis, the linear fit and exponential decay of the asymmetry index were examined, and R and X2 values were reported for the goodness of exponential fit.
All statistical evaluations and fittings were performed using OriginPro software (Origin Lab Corp., Northampton, MA, USA) and SPSS 16.0 (IBM Corp., Armonk, NY, USA).
2.10Data availability
All published and unpublished anonymized data from this study can be shared by request from a qualified investigator.
3Results
3.1Analysis of self-motion perception and VOR during the post-ictal period in all MD patients
Self-motion rotation evaluated by asymmetric rotation in all MD patients. The error in remembering target representation (tracking position error, TPE) was measured to examine self-motion perception. The asymmetric rotation was performed with two opposite directed sequences of fast rotation toward the lesion (irritated or defective) side and toward the healthy side. In the presence of vestibular imbalance, the TPE was different in response to the two rotation sequence conditions. When the fast rotation of the asymmetric rotation sequence was directed toward the healthy side in the case of labyrinthine deficit or to the irritated side in the case of labyrinthine irritation, the TPE was much greater (70±11°). Conversely, it was smaller when the fast rotation of the asymmetric rotation was toward the lesion (in the presence of functional deficit) or the healthy side (in the presence of functional irritation) (29±12°). This difference was reported as the “asymmetric index” (Fig. 2). This index was minimal in unaffected individuals (7.5±4.9) while in the MD patients, it was significantly greater (33.73±7.14) (F(1,54) = 157.40, P < 0.001, η= 0.97). Interestingly, there was a correlation between the frequency of occurrence of the MD attack and the amplitude of the asymmetric index (R = 0.71, F(1,26) = 26.75 P < 0.001).
Self-motion rotation evaluated by symmetric rotation in all MD patients. Self-motion perception of patients was also tested with symmetric sinusoidal rotation at frequencies of rotation of 0.09 and 0.38 Hz. We measured the TPE observed after four cycles of symmetric rotation. Comparison of the TPE with the control group showed a statistically significant difference in values between control and MD patients (for 0.09 Hz: F(2,53) = 3.71, P < 0.05, η= 0.91; for 0.38 Hz: F(2,53) = 3.92, P < 0.05, η= 0.82).
VOR evaluated by asymmetric rotation during the post-ictal period in all MD patients. The VOR of MD patients was examined in response to asymmetric rotation by evaluating the cumulative slow phase eye position (SPEP) after the two opposite directed asymmetric sequences of rotation. The difference in SPEPs was reported as the asymmetric index and compared with the VOR of the control group (Fig. 3).
The comparison showed that the VOR asymmetric index for all MD patients was 14.8±9.2, significantly higher than that of our control group (8.1±7.5) (F(1,54) = 80, P < 0.001, η= 0.91).
3.2Analysis of self-motion perception and VOR in different subgroups of MD patients characterized by the presence or absence of ocular reflex alteration during the post-ictal period
The MD patients were also examined by routine vestibular tests (Caloric, v-HIT, HST, VIN) and for the presence of spontaneous or positional nystagmus during the post-ictal period. Two subgroups of patients were found: subgroup A with patients showing unaffected ocular responses to all vestibular tests (10 women and 6 men) (data in Table 1) and subgroup B with patients with at least one abnormal response (6 women and 6 men) (data in Table 2). Patients in subgroup A reported a few preceding acute MD episodes (1–3), and showed more functional irritation than functional deficit (11 vs 5), whereas subgroup B reported several preceding acute MD episodes (>3) and showed functional irritation in half of the cases. According to these different subgroups of patients, we performed a separate analysis of the perceptual and reflex responses to asymmetric rotation.
Table 1
Perceptual and reflex responses of group A MD patients to conventional vestibular test and to asymmetric rotation
Patients: age, sex, M/F | Spontaneous or positional nystagmus | C.P. normal < 30% | HST SPEV normal < 2°/s | vHIT normal < 30% | VIN | Asymmetry index Motion Perception | Asymmetry index VOR |
43, F | No | 11 | No | 1 | No | 35 | 6 |
21, F | No | 10 | No | 9 | No | 30 | 8 |
48, F | No | 6 | No | 6 | No | 28 | 1 |
50, M | No | 10 | No | 3 | No | 23 | 8 |
40, F | No | 1 | No | 13 | No | 44 | 7 |
55, M | No | 3 | No | 4 | No | 45 | 13 |
45, M | No | 15 | No | 5 | No | 32 | 15 |
38, F | No | 7 | No | 2 | No | 28 | 10 |
43, F | No | 8 | No | 11 | No | 27 | 9 |
50, F | No | 10 | No | 3 | No | 21 | 11 |
32, M | No | 12 | No | 14 | No | 35 | 12 |
35, F | No | 6 | No | 3 | No | 28 | 4 |
46, M | No | 5 | No | 15 | No | 40 | 13 |
48, F | No | 13 | No | 2 | No | 22 | 3 |
42, M | No | 7 | No | 13 | No | 36 | 10 |
41, F | No | 11 | No | 4 | No | 26 | 5 |
Each column is headed by the test which the patient has undergone. Presence of spontaneous or positional nystagmus, responses to caloric stimulation evaluated such as canal paresis (C.P.), the head shaking test (HST), the video head impulse test (vHIT), nystagmus induced by vibration (VIN), asymmetry value of tracking position error (Asymmetry index of motion perception) and asymmetry of cumulative SPEP (Asymmetry index of vestibulo-ocular reflex (VOR)) in response to asymmetric stimulation. Normal values, as reported in the literature, are indicated below the heading. The directionality of the responses (irritation or deficit) is indicated in parentheses.SPEV = slow phase eye velocity.
Table 2
Perceptual and reflex responses of group B MD patients to conventional vestibular test and to asymmetric rotation
Patients age sex | Spontaneous or positional nystagmus | C.P. normal < 30% | HST normal SPEV < 2°/s | vHIT normal < 30% | VIN | Asymmetry index Perception | Asymmetry index VOR |
29, F | No | 7 | 8.14° | 2 | Yes | 35 | 18 |
35, F | No | 5 | 10.15° | 7 | No | 37 | 19 |
48, M | No | 35 | 5.10° | 45 | Yes | 31 | 25 |
44, F | No | 37 | 8.10° | 36 | Yes | 45 | 35 |
49, F | No | 10 | 5.12° | 8 | No | 29 | 17 |
25, M | No | 18 | 13.16° | 8 | Yes | 35 | 19 |
45, M | No | 8 | 6.12° | 6 | Yes | 32 | 17 |
40,M | Yes | 38 | 7.10° | 51 | Yes | 44 | 38 |
39, M | Yes | 40 | 8.35 | 38 | Yes | 38 | 25 |
47, M | No | 16 | 9.56 | 15 | No | 45 | 20 |
26, F | No | 16 | 7.87 | 13 | No | 33 | 20 |
39, F | No | 10 | 10.11 | 36 | Yes | 40 | 27 |
All data explanations as in Table. 1.
Analysis of self-motion perception in response to asymmetric rotation in the A and B subgroups of MD patients. Comparison of the asymmetric index among A and B subgroups and the control group in response to asymmetric rotation showed values of 31.3±7.3 in subgroup A and 37.8±5.5 in subgroup B. Both sets of values were significantly higher (F(2,53) = 54, P < 0.001, η= 0.93) than those in the control group (P < 0.001) (Fig. 4A)
Fig. 4
Asymmetry in the self-motion perception and VOR in MD patients with and without ocular reflex symptoms. A: Asymmetric index (mean and SD) of tracking position error in control, patients with ocular symptoms (group A) and without ocular symptoms (group B) in response to opposite directed asymmetric rotation. B: TPE at the end of symmetric rotation at frequencies of 0.09 Hz and 0.38 Hz. Significant difference: * (p < 0.05), ** (p < 0.001), non-significant difference: ns. C: Asymmetric index (mean and SD) of cumulative SPEP (cSPEP) of VOR in response to opposite directed asymmetric rotation in controls, and group A and B of MD patients. Significant difference: ** (p < 0.001), non-significant: ns.
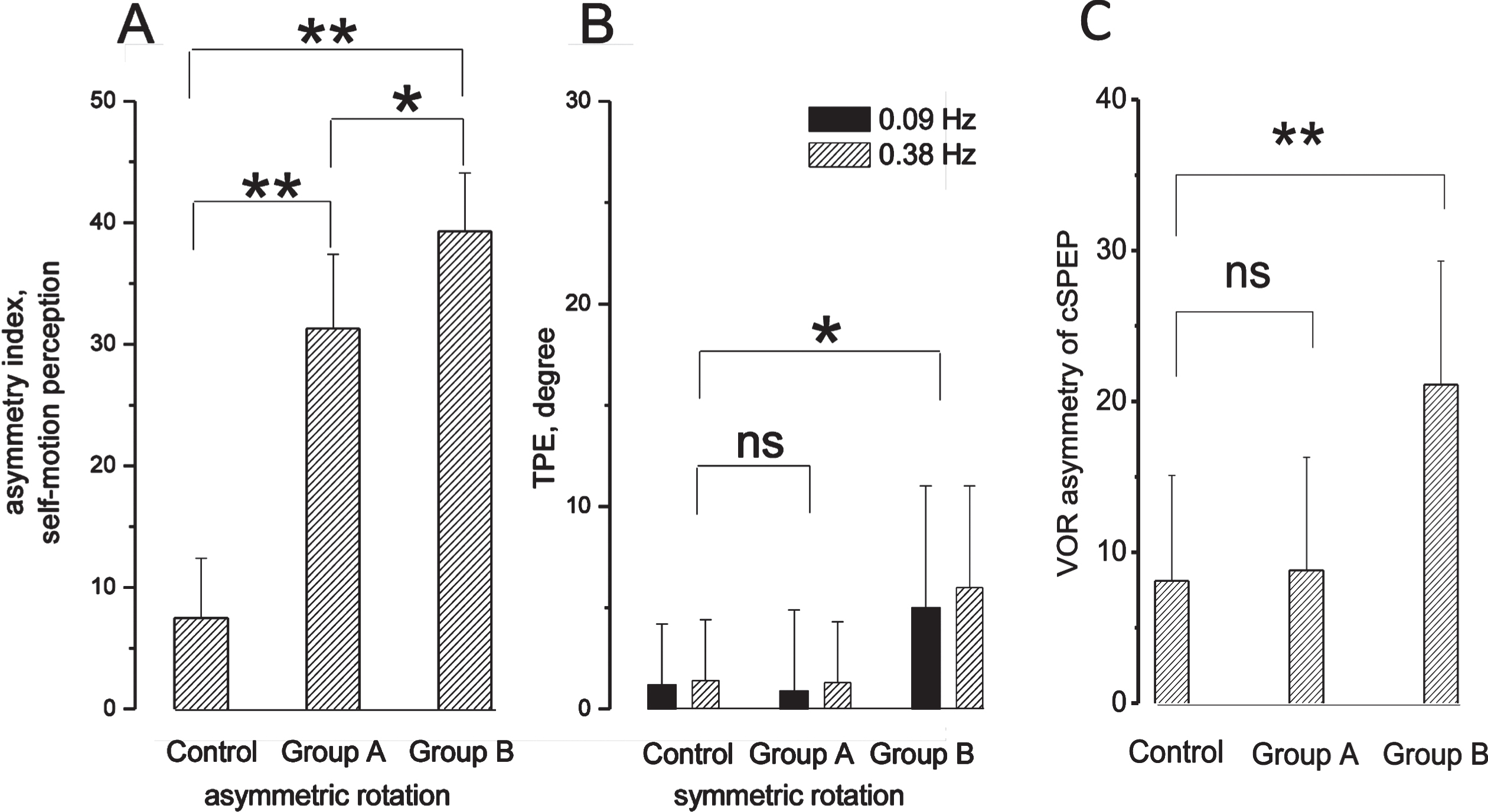
Analysis of self-motion perception in response to symmetric rotation in the A and B subgroups of MD patients. Comparison of the values in the two groups with the control group showed a statistically significant difference (for 0.09 Hz: F(2,53) = 3.71, P < 0.05, η= 0.91; for 0.38 Hz: F(2,53) = 3.92, P < 0.05, η= 0.83). However, post-hoc analysis showed that only the values in subgroup B presented a statistically significant difference with the control group (0.09, 0.38 Hz: P < 0.05), while subgroup A did not show a significant difference with the control group (0.09 Hz: P = 0.22; 0.38 Hz: P = 0.25). (Fig. 4B)
Analysis of VOR in response to asymmetric rotation in the subgroups of MD patients. Analysis of the VOR asymmetry has been performed in subgroups A and B versus control (F(2,53) = 60, P < 0.001, η= 0.85). The asymmetric index of subgroup A (8.4±3.9) was not different from the control group (P = 0.43), Fig. 4C and the individual values were within the CI of the control group, while the asymmetric index of subgroup B (23.5±6.9) (P < 0.001) was different from the control group.
On the whole, subgroup A without VOR asymmetry showed side asymmetry in the perceptual test, not in reflex responses and the side perceptual preponderance was evident only by asymmetric rotation. In contrast, subgroup B with VOR alteration showed perceptual side asymmetry in response to asymmetric as well as to symmetric rotation.
The time course of the side asymmetry of subgroup A after the last acute episode was also examined by repeated tests at increasing intervals (days). The index decreased exponentially with the increasing interval, but it was always higher than the CI of the control group, even after 60 days (Fig. 5).
Fig. 5
Decay of the perceptual asymmetry index in MD patients after the acute episode. Values of the asymmetry index (filled squares) during the post-ictal interval and the exponential fit (dashed line) of data (Tc: 28.1, and R square = 0.85). Below this is the mean value of the asymmetry index (entire line) and the CI (gray area) in unaffected individuals. Note the progressive reduction in the index value that remained above the CI of unaffected individuals after 60 days. CI = confidence interval.
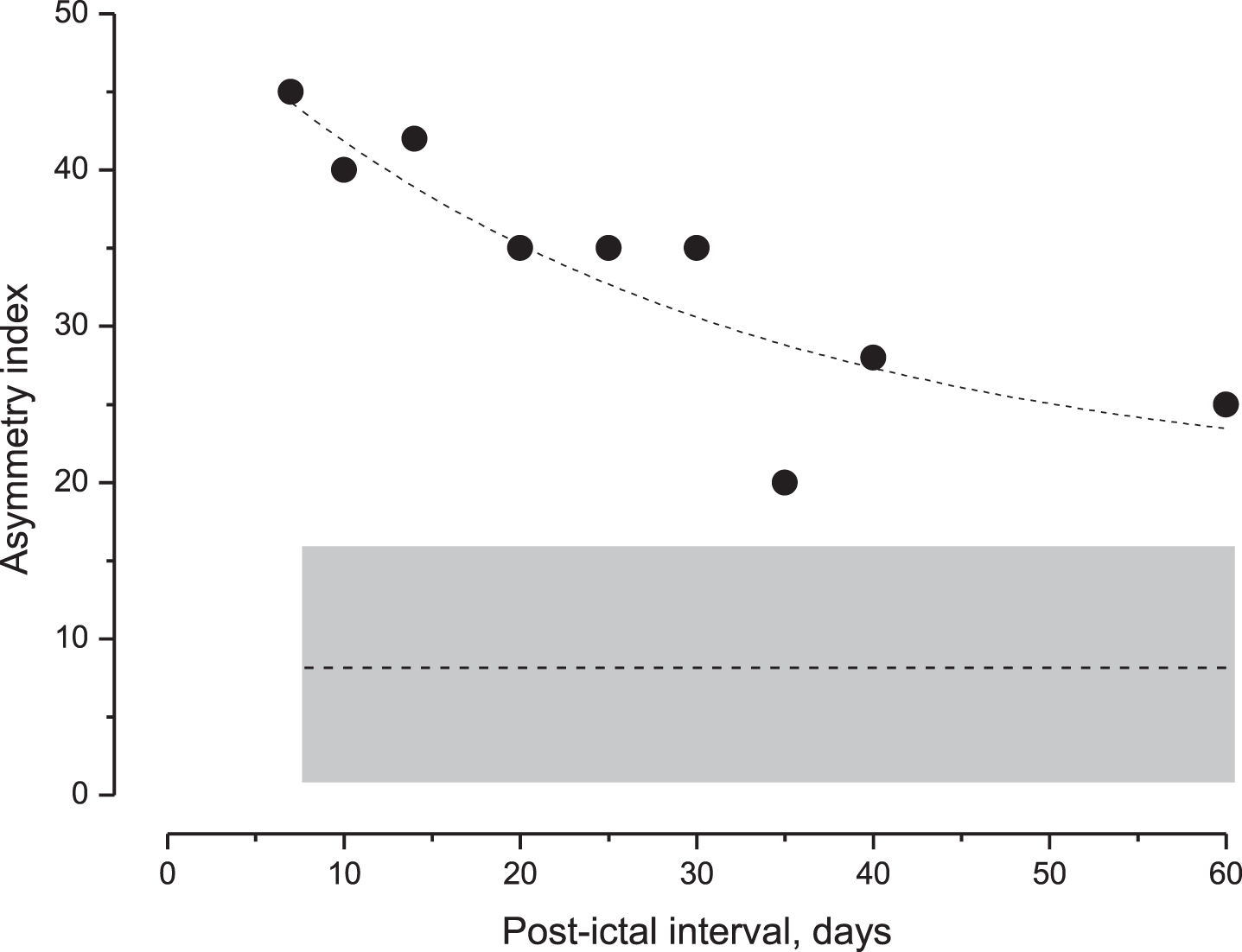
3.3DHI compared with perceptual and VOR asymmetry index
The relationship between DHI score with the asymmetry index of motion perception and that of VOR in all MD patients was different (Fig. 6). In fact, the DHI values were strictly correlated with the degree of perceptual asymmetry index, with R = 0.74 (F(1,26) = 36.99, P < 0.001), while the relationship of the DHI score with the VOR asymmetry was less evident, with R = 0.57 (F(1,26) = 13.11, P < 0.05). In particular, there was a lack of correlation between the DHI and asymmetry of VOR in subgroup A (R = 0.21, F(1,14) = 1.11, P = 0.55).
Fig. 6
Correlation of perceptual and VOR asymmetry indexes in response to asymmetric rotation with DHI score in MD patients. Correlation of perceptual (filled circles) and VOR (open circles) asymmetry indexes with DHI score. Vertical line separates values of patients without VOR imbalance (left side) from values of patients with VOR imbalance (right side). Linear fit of perceptual asymmetry index (entire line), linear fit of VOR asymmetry index (dashed line) of all patients and linear fit of VOR asymmetry values of patients without reflex alteration (thick dashed line). Correlation coefficient (R) and probability (P). Note the strict correlation between DHI score and perceptual asymmetry.
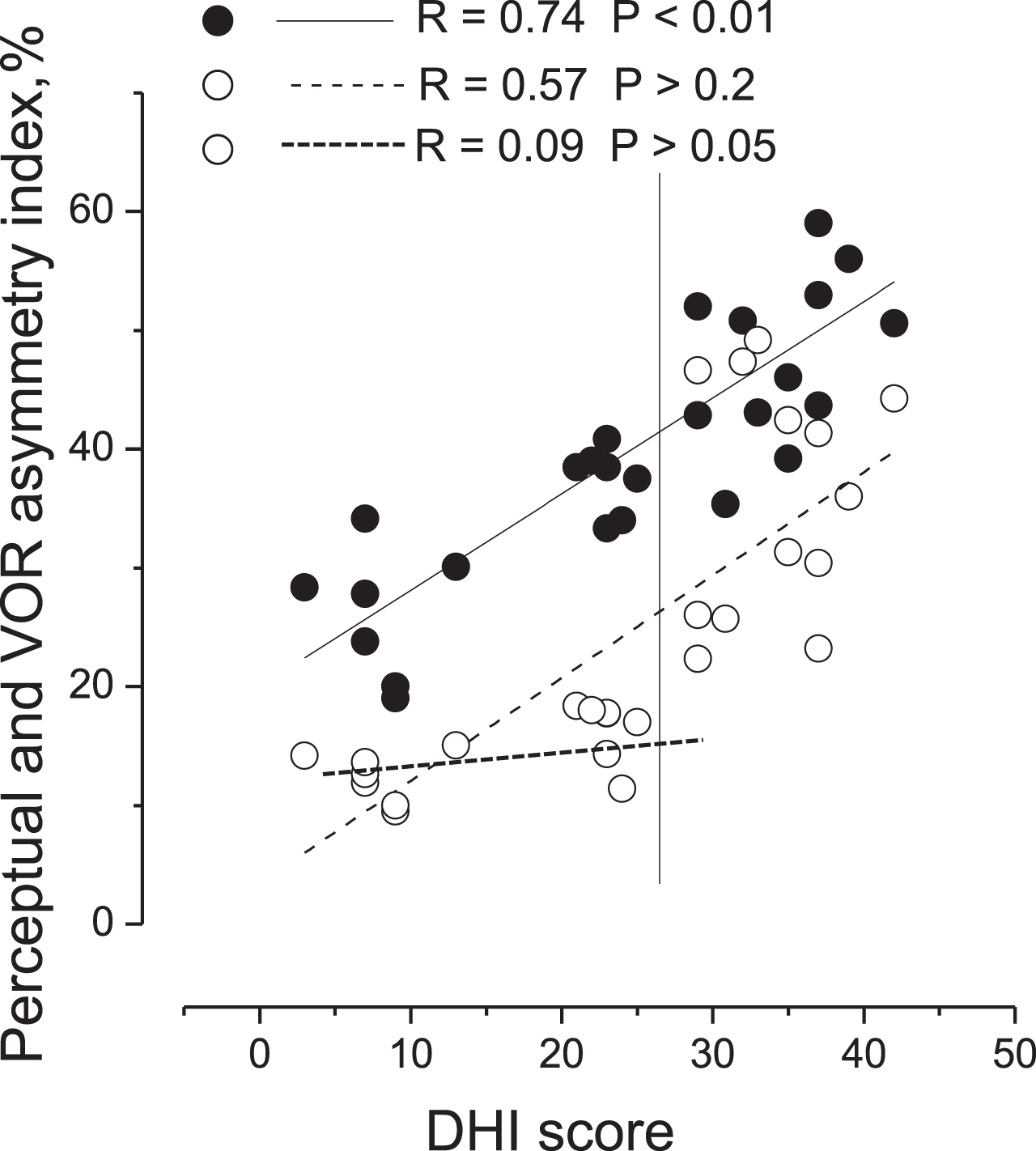
4Discussion
Our study shows that side imbalance in self-motion perception persisted during the post-ictal period in all MD patients and reflected the functional alteration expressed during the ictal episode, so that the perceptual asymmetric index could be used reliably to establish the laterality of the disease in cases where laterality was less clear. The alteration consisted of a directional preponderance as shown by the increased value of the perceptual asymmetry index. It persisted throughout the period of observation after the acute episode even if it tended to diminish after a longer time interval. In all patients, we found that this abnormality was greater in patients who underwent several acute episodes and that it was correlated with the number of these episodes.
The responses to both asymmetric and symmetric rotation showed a perceptual imbalance in all patients, but, when we examined the subgroups of MD patients in whom the ocular reflex was normal and the responses to clinical vestibular tests were in the normal range, only the asymmetric rotation detected perceptual imbalance. Therefore, the application of asymmetric rotation turns out to be a decisive method for revealing persistent motion perception abnormality in individuals with a normal ocular reflex response. The perceptual and reflex disparity has already been observed in an earlier study on vestibular neuritis [23] and can be explained by considering that the circuit elaborating motion perception adapts to maintain the internal perceptual imbalance increasing the responses to fast rotation and decreasing those to slow rotation [23, 26, 27]. The opposite occurs in the VOR circuit which adapts to reach a circuital balance earlier. The mechanism of vestibular adaptation may take place at the level of the vestibular nuclei since it has been shown in in vitro experiments that LTP and LTD can be induced depending on the intensity of stimulation and depending on the vestibular circuit [9, 24]. Therefore, asymmetric stimulation enhances the perceptual side difference, revealing even small disparities. In contrast to motion perception, asymmetric stimulation does not show any imbalance of the VOR. This may be the reason that explains why asymmetric rotation can show a functional residual deficit in the perceptual circuit during inter-critical periods of MD.
As in previous work on patients with unilateral vestibular lesions (UVL) [23], we examined the correlation between the degree of perceptual asymmetry and the degree of disability, assessed by the DHI. We found a strict correlation between DHI score and perceptual asymmetry, while the same correlation was not observed in the VOR asymmetry.
The findings confirm the relevance of motion perception in determining the subjective perception of disability. Indeed, the degree of perceptual asymmetry varied in parallel with the value of the DHI score. It is possible that the subjective evaluation of quality of life and psychic disorders which MD patients often complain of, could be related to persistence of a minor state of vestibular irritation or deficit where the nervous system compensates for eye movements, but not for self-motion perception. The evaluation of motion perception could be relevant in MD, especially because of its extreme variability of symptoms, and its significant impact on quality of life exacerbated by psychiatric comorbidity.
In addition, perceptual asymmetry may be at the core of the discomfort experienced by MD patients; therefore, an appropriate rehabilitation focused on motion perception may significantly improve the quality of life of MD patients. Evaluation of vestibular perceptual asymmetry can be important for assessing the effects of conventional rehabilitation techniques by observing their effects both at the level of reflexes, and of motion perception. Furthermore, this perceptual evaluation can activate specific rehabilitation programs for restoring the perceptual balance, possibly enhancing motion perception towards the side where it is less responsive or reducing the perception towards the side where it is excessive.
4.1Methodological limitation
This method presents some limitations when administered to patients since, even though this test is directed to find a measurable and objective perceptual error, there is a large variability between patients. Furthermore, the test also requires time for training patients to execute the trials correctly and to remember the visual target position during the rotation.
In conclusion, we suggest that the test for asymmetric rotation may be useful to demonstrate persistent perceptual disorder in MD patients and to guide them towards a specific rehabilitation program. This study also supports the idea that diagnostics involving the vestibular system should rely on evaluating reflexes such as the VOR and perceptual responses.
Acknowledgments
The authors thank Colin Woodham, a professional, native English-speaking editor for the linguistic review of the manuscript.
References
[1] | Bloomberg J. , Melvill Jones G. and Segal B. , Adaptive plasticity in the gaze stabilizing synergy of slow and saccadic eye movements, Exp Brain Res 84: ((1991) ), 35–46. |
[2] | Brandt T. and Dieterich M. , The vestibular cortex. Its locations, functions, and disorders, Ann N Y Acad Sci 871: ((1999) ), 293–312. |
[3] | Bronstein A.M. , Grunfeld E.A. , Faldon M. and Okada T. , Reduced self-motion perception in patients with midline cerebellar lesions, Neuroreport 19: ((2008) ), 691–693. |
[4] | Cousins S.E. , Kaski D. , Cutfield N. , Seemungal B. , Golding J.F. , Gresty M.A. , Glasauer S. and Bronstein A.M. , Vestibular perception after acute unilateral vestibular lesions, PLoS One 8: ((2013) ), e61862. |
[5] | Cullen K.E. , The vestibular system: multimodal integration and encoding of self-motion for motor control, Trends Neurosci 35: ((2012) ), 185–196. |
[6] | Dupuits B. , Pleshkov M. , Lucieer F. , Guinand N. and Pérez Fornos A. , Guyot J.P. , Pleshkov M. , Kingma H. and van de Berg R. , A new and faster test to assess vestibular perception, Front Neurol 10: ((2019) ), 707. |
[7] | Fitzgerald G. and Hallpike C.S. , Studies on human vestibular function: I. Observations on the directional preponderance of nystagmus resulting from cerebral lesions, Brain 65: (1942), 115. |
[8] | Grabherr L. , Nicoucar K. , Mast F.W. and Merfeld D.M. , Vestibular thresholds for yaw rotation about an earth-vertical axis as a function of frequency, Exp Brain Res 186: ((2008) ), 677–681. |
[9] | Grassi S. and Della Torre G. , Capocchi G. , Zampolini M. and Pettorossi V.E. , The role of GABA in NMDA-dependent long term depression (LTD) of rat medial vestibular nuclei, Brain Res 699: ((1995) ), 183–191. |
[10] | Jacobson G.P. and Newman C.W. , The development of the Dizziness Handicap Inventory, Arch Otolaryngol Head Neck Surg 116: ((1990) ), 424–427. |
[11] | Jongkees L.B.W. , Thermic test and electronystagmography, Acta Otorhinolaryngol Belg 19: ((1965) ), 455–464. |
[12] | Jongkees L.B.W. , Maas J.P.M. and Philipszoon A.J. , Clinical nystagmography. A detailed study of electro-nystagmography in 341 patients with vertigo, Pract Otorhinolaryngol (Basel) 24: ((1962) ), 65–93. |
[13] | Kamei T. , Kimura K. , Kaneko H. and Noro H. , Revaluation of the head shaking test as a method of nystagmus provocation. 1. Its nystagmus-eliciting effect, Nihon Jibiinkoka Gakkai Kaiho 67: ((1964) ), 1530–1534. |
[14] | Kaski D. , Quadir S. , Nigmatullina Y. , Malhotra P.A. , Bronstein A.M. and Seemungal B.M. , Temporoparietal encoding of space and time during vestibular-guided orientation, Brain 139: ((2016) ), 392–403. |
[15] | Leigh R.J. and Zee D.S. , The Neurology of Eye Movements, Oxford University Press, Detroit, (2015) . |
[16] | Lopez C. and Blanke O. , The thalamocortical vestibular system in animals and humans, Brain Res Rev 67: ((2011) ), 119–146. |
[17] | Lopez-Escamez J.A. , Carey J. , Chung W.H. , Goebel J.A. , Magnusson M. , Mandalá M. , Newman-Toker D.E. , Strupp M. , Suzuki M. , Trabalzini F. and Bisdorff A. , Diagnostic criteria for Menière’s disease, J Vestib Res 25: ((2015) ), 1–7. |
[18] | Maire R. and van G. , Melle, Vestibulo-ocular reflex characteristics in patients with unilateral Meniere’s disease, Otol Neurotol 29: ((2008) ), 693–698. |
[19] | Manzari L. , Burgess A.M. , MacDougall H.G. , Bradshaw A.P. and Curthoys I.S. , Rapid fluctuations in dynamic semicircular canal function in early Meniere’s disease, Eur Arch Otorhinolaryngol 268: ((2011) ), 637–639. |
[20] | Merfeld D.M. , Priesol A. , Lee D. and Lewis R.F. , Potential solutions to several vestibular challenges facing clinicians, J Vestib Res 20: ((2010) ), 71–77. |
[21] | Ohki M. , Murofushi T. , Nakahara H. and Sugasawa K. , Vibration-induced nystagmus in patients with vestibular disorders, Otolaryngol Head Neck Surg 129: ((2003) ), 255–258. |
[22] | Panichi R. , Botti F.M. , Ferraresi A. and Faralli M. , A Kyriakareli, M. Schieppati and V.E. Pettorossi, Self-motion perception and vestibulo-ocular reflex during whole body yaw rotation in standing subjects: the role of head position and neck proprioception, Hum Mov Sci 30: ((2011) ), 314–332. |
[23] | Panichi R. , Faralli M. , Bruni R. , Kiriakarely A. , Occhigrossi C. , Ferraresi A. , Bronstein A.M. and Pettorossi V.E. , Asymmetric vestibular stimulation reveals persistent disruption of motion perception in unilateral vestibular lesions, J Neurophysiol 118: ((2017) ), 2819–2832. |
[24] | Pettorossi V.E. , Dieni C.V. , Scarduzio M. and Grassi S. , Long-term potentiation of synaptic response and intrinsic excitability in neurons of the rat medial vestibular nuclei, Neuroscience 187: ((2011) ), 1–14. |
[25] | Pettorossi V.E. , Panichi R. , Bambagioni D. , Grassi S. and Botti. F.M. , Contribution of eye position to movement perception, Acta Otolaryngol 124: ((2004) ), 471–474. |
[26] | Pettorossi V.E. , Panichi R. , Botti F.M. , Kyriakareli A. , Ferraresi A. , Faralli M. , Schieppati M. and Bronstein A.M. , Prolonged asymmetric vestibular stimulation induces opposite, long-term effects on self-motion perception and ocular responses, J Physiol 591: (2013), 1907–1920. |
[27] | Pettorossi V.E. and Schieppati M. , Neck proprioception shapes body orientation and perception of motion, Front Hum Neurosci 8: ((2014) ), 895. |
[28] | Popov K.E. , Lekhel H. , Faldon M. , Bronstein A.M. and Gresty M.A. , Visual and oculomotor responses induced by neck vibration in normal subjects and labyrinthine-defective patients, Exp Brain Res 128: ((1999) ), 343–352. |
[29] | Seemungal B.M. , Gunaratne I.A. , Fleming I.O. , Gresty M.A. and Bronstein A.M. , Perceptual and nystagmic thresholds of vestibular function in yaw, J Vestib Res 14: ((2004) ), 461–466. |
[30] | Seemungal B.M. , Rizzo V. , Gresty M.A. , Rothwell J.C. and Bronstein A.M. , Posterior parietal rTMS disrupts human Path Integration during a vestibular navigation task, Neurosci Lett 437: ((2008) ), 88–92. |
[31] | Shaikh A.G. , Meng H. and Angelaki D.E. , Multiple reference frames for motion in the primate cerebellum, J Neurosci 24: ((2004) ), 4491–4497. |
[32] | Sharp P.E. , Blair H.T. , Etkin D. and Tzanetos D.B. , Influences of vestibular and visual motion information on the spatial firing patterns of hippocampal place cells, J Neurosci 15: ((1995) ), 173–189. |
[33] | Siegle J.H. , Campos J.L. , Mohler B.J. , Loomis J.M. and Bülthoff H.H. , Measurement of instantaneous perceived self-motion using continuous pointing, Exp Brain Res 195: ((2009) ), 429–444. |
[34] | Sinha N. , Zaher N. , Shaikh A.G. , Lasker A.G. , Zee D.S. and Tarnutzer A.A. , Perception of self motion during and after passive rotation of the body around an earth vertical axis, Prog Brain Res 171: ((2008) ), 277–281. |
[35] | Valko Y. , Lewis R.F. , Priesol A.J. and Merfeld D.M. , Vestibular labyrinth contributions to human whole-body motion discrimination, J Neurosci 32: ((2012) ), 13537–13542. |