A case series on the effect of dynamic neoprene orthosis on lower limb kinematic variables in children with cerebral palsy
Abstract
PURPOSE:
This study examined the effects of a dynamic neoprene orthosis on kinematic variables of gait in children with spastic bilateral cerebral palsy (CP).
METHODS:
Five children (whose median age was 9.6 years and who ranged in age from six to 12) with spastic bilateral CP and flexed knee at levels I-III of the Gross Motor Function Classification System were examined using kinematic gait analysis in three different conditions: 1) with dynamic neoprene orthosis; 2) without dynamic neoprene orthosis (immediate effect); and 3) without orthosis after six weeks of intervention.
RESULTS:
The comparison between condition one (with dynamic neoprene orthosis) and condition two (without dynamic neoprene orthosis) demonstrated the existence of improvements in minimum hip and knee flexion variables. Moreover, the results indicated that the improvements remained constant in several key gait variables after six weeks.
CONCLUSION:
The results varied from subject to subject, and there were signs of improvement in some of the subjects. Therefore, it was not possible to draw conclusions at a group level. Nonetheless, a number of individuals may benefit from this type of orthosis.
1Introduction
Cerebral palsy (CP) is a group of disorders that affect movement and posture and is associated with limitations in activities such as walking [1, 2]. Around 22% of children with CP are categorized as spastic bilateral CP with various levels of severity, which often impacts their gait [3]. One of the most common pathologic gait patterns in children with CP is crouch gait [4, 5]. This gait pattern is accompanied by increased hip and knee flexion and the dorsiflexion of the ankle joint in the stance phase. Walking with this pattern may be associated with high energy expenditure, joint pain, and joint deformity; it may also require them to be dependent upon caregivers for assistance with activities of daily living [6, 7]. Some interventions that reduce crouch posture include postural training, surgery, and orthotic management [8, 9].
A variety of rigid and dynamic orthoses can be prescribed to improve gait in children with CP [1]. New technologies have been introduced into rehabilitation programs to promote and/or enhance the engagement of children with CP in a variety of physical activities and tasks. Since the 1990 s, different types of therapeutic suits, such as TheraTogs (TT) and TheraSuit (TS), have been used for children with CP. TT is an orthotic undergarment suit and strapping system that is suitable for children with sensorimotor impairment. It is a new modality used for improving postural alignment and stability, movement skill and precision, and joint stability [22]. TS is a soft dynamic proprioceptive orthosis consisting of vest, shorts, knee pads, and specially adapted shoes that aligns the body by placing pressure on specific areas using a system of interconnected elastic cords [11].
Furthermore, Lycra garments known as dynamic orthoses have also been used for this population [10] as well as adults who suffer from neurologic and rheumatologic conditions [11, 12]. These tight, custom-made garments [13] exert a compressive or tensile force on the body, which can reduce high muscle tone, soft-tissue contracture, and involuntary movement [10]. In addition, they can improve postural alignment [2, 13]. Recent studies have suggested that dynamic Lycra garments provide patients with an immediate and continuous improvement in balance, proximal joint stability, and postural readiness for movement [2].
For example, Martins et al. [11] evaluated the immediate effect of a dynamic orthosis on gait patterns in children with unilateral spastic CP (US-CP). Seven participants with US-CP, level I-II on the Gross Motor Function Classification System (GMFCS), were examined using kinematic gait analysis in three different conditions: (A) at baseline; (B) TS without elastics; and (C) TS with elastics. Significant improvements were observed at the hip and knee joint during most of the gait cycle in participants who wore a TS. This kind of suit seemed to provide the patients with a more functional and safer gait pattern [11]. Mathew et al. showed that dynamic elastomeric fabric orthoses (DEFO) were able to positively affect the walking patterns of some children with spastic bilateral CP [12]. Bahramizadeh et al. reported that a dynamic neoprene orthosis could reduce crouch posture without causing adverse effects on postural stability in children with CP; however, postural control did not improve in a six-week time frame [13]. Despite increasing interest in dynamic elastomeric orthoses, there are few studies in this area, and a number of the studies that have been published have not used appropriate outcome measures. Moreover, most of the pertinent studies have focused on the problems of this suit, such as the difficulties that children with CP experience in wearing orthotic garments [10]. The present study aimed to investigate the effects of dynamic neoprene orthoses encompassing the lower limb and pelvis on kinematic gait variables in children with CP.
2Materials and methods
In this study, a case-series analysis approach was adopted based on the hypothesis that the identification of individual variation in treatment responses is an essential step in advancing evidence-based practice in the rehabilitation of children with CP [14]. The researchers adopted this approach because the use of mean group differences does not reveal the effectiveness of the intervention for each participant. Furthermore, the case-series analysis approach ensures that positive outcomes are produced for all of the children with CP [14].
2.1Subjects
Five children with spastic bilateral CP, who ranged in age from six to 12, were selected from two non-profit rehabilitation centers in Tehran, namely the Valiasr Rehabilitation Center and the Sadra Center. These centers offer specialized rehabilitation services to children with CP. In this study, the inclusion criteria involved: 1) a diagnosis of spastic bilateral CP with crouch gait or flexed knee, 2) 1 to 1 + spasticity level of the triceps surae and hamstrings based on the Modified Ashworth Scale [15], 3) GMFCS level I, II or III [16], 4) the ability to walk without support for at least five meters, 5) patient cooperation, and 6) patient and parental consent. The exclusion criteria were: 1) fixed flexion contracture in hip and/or knee joints, 2) knee flexion > 30° throughout the stance phase of gait (severe crouch gait) [13, 17, 18], 3) treatment injections of botulinum toxin, and 4) a history of neurosurgical and orthopedic surgeries in the past 1.5 years. The Human Research Ethics Committee of the University of Social Welfare and Rehabilitation Sciences approved the study protocol. In addition, the subjects’ parents read and signed the consent form before the onset of the study.
2.2Intervention
The orthoses were developed using neoprene fabrics and custom-made for each patient; each participant was measured individually by a certified orthotist (Fig. 1). There were not any reinforcements with additional plastic boning at any point. The fabricated elastic pants completely covered the lower limb, encompassed the pelvis, stretched distally below the malleolus and approximately above the iliac crests [12], and were zipped on the lateral side of each extremity.
Fig. 1
Posterior and lateral views of the dynamic neoprene orthosis used in this study showing the position and mounting arrangement of the markers. (Two markers of 17 markers on iliac crests are not visible in these photos.) The parents’ consent has been received to use these photographs.
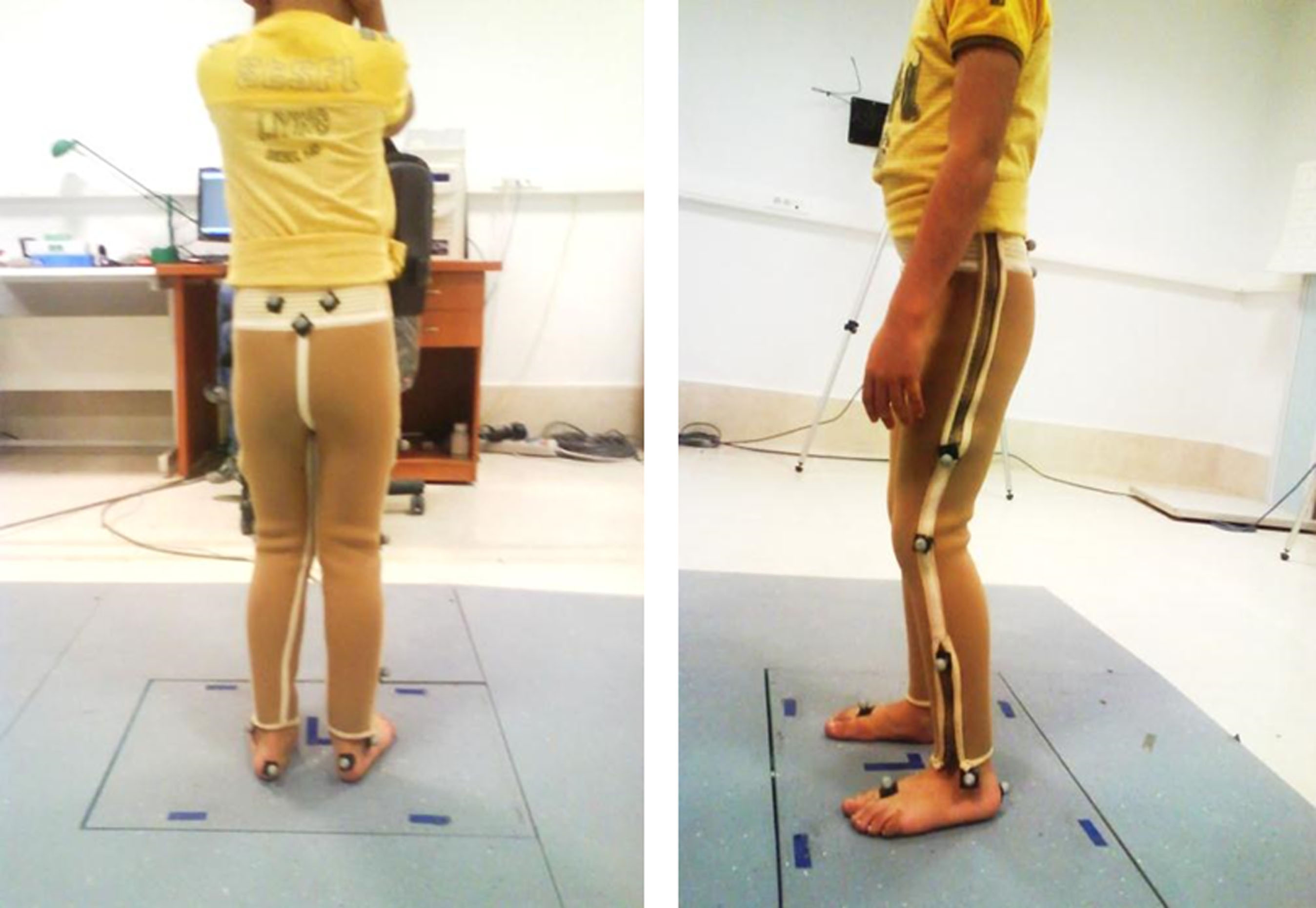
2.3Procedure
Each participant was evaluated in two sessions with a six-week interval (baseline and after six weeks). Three-dimensional (3D) data of lower limb movements were collected using a motion analysis system (Vicon 460 motion analysis system, Oxford, UK) at a frequency of 100 Hertz (Hz). In the baseline session, anthropometric measures (height, body weight, tibial length, distance between the femoral condyles or diameter of the knee, distance between the malleoli or diameter of the ankle, distance between the anterior iliac spines, and thickness of the pelvis) were determined first without dynamic orthosis and then with the garment on. Then, 17 retro-reflective markers were fixed directly on the skin using double-sided adhesive tape at anatomical landmarks of the pelvis and the lower extremities, which were identified by palpation. Marker attachments followed the Plug-in-Gait protocol (Fig. 1). The reference position was determined after collecting the marker data for five seconds (s) with and without the orthosis. Before beginning to capture data while walking, the subjects took practice walks wearing the dynamic orthosis. Next, the participants were instructed to walk up and down at a comfortable walking speed and then asked to stop after about 20 minutes (min); this procedure was repeated three times with 20 min rest breaks between each trial. Kinematic variables were recorded for each lower limb both with and without the orthosis. In the same session, lower extremity markers were placed on the garments as closely as possible to the given points. The participants walked along a six-meter walkway and data was captured with and without the orthosis.
Following the baseline session, the children were asked to wear the dynamic neoprene orthoses for six weeks for approximately 4-6 hours per day [12, 19]. In the second session, after six weeks, the data gathering procedures were repeated.
Kinematic variables were examined under three test conditions: 1) walking without orthoses in baseline, 2) walking with orthoses in baseline, and 3) walking without orthoses after six weeks.
2.4Statistical analysis
Acquisition and processing of the biomechanical data were performed with the Vicon system by using Nexus Software, version 1.8.5. The kinematic data were analyzed with the Plug-in- Gait model [20], which is widely used in clinical gait analysis [21]. All of the data used in this analysis were previously archived in folders and exported to Microsoft Excel files. Each file stored the task and evaluation data that were collected on one of the participants. The gait cycles of each trial were identified by observing the data and determining the moment of initial contact and toe off. The mean and standard deviation values of kinematic data, including the minimum and maximum flexion of the hip and knee joints, cadence, and stride length were reported.
3Results
Five children with spastic bilateral CP (two females and three males) participated in this study. The subjects’ mean weight, mean age, and mean height values were 27.1 kilograms, 9.6 years, and 120 centimeters respectively (Table 1). Spatiotemporal variables including cadence and stride length are provided in Table 2. Kinematic data in the sagittal plane of the right lower limb, including the maximum and minimum angles of flexion in the hip and knee joints, are shown in Table 3. Figures 2 and 3 show hip and knee patterns in the sagittal plane in five participants with and without dynamic neoprene orthosis.
Table 1
Characteristics of the patients who participated in this study. Kg = kilograms; cm = centimeters; BMI = body mass index; GMFCS = Gross Motor Function Classification System
Participants | Gender | Age, in years | Weight (kg) | Height (cm) | BMI | GMFCS |
1 | Female | 11 | 28.9 | 122 | 19.4 | 3 |
2 | Male | 10 | 23.2 | 118 | 16.7 | 3 |
3 | Male | 15 | 46.8 | 170 | 16.2 | 2 |
4 | Male | 7 | 21.3 | 116 | 15.8 | 1 |
5 | Female | 5 | 15.2 | 103 | 20.7 | 2 |
Table 2
Spatiotemporal variables. The mean and standard deviation (SD) of cadence and stride length in three trials in each condition
Variable | Cadence (steps/minute) (SD) | Stride length (meter) (SD) | ||||
A | B | C | A | B | C | |
Case 1 | 47 (5.5) | 61 (3.6) | 62 (6.1) | 0.44 (0.05) | 0.42 (0.23) | 0.42 (0.08) |
Case 2 | 52 (2.8) | 57 (2.8) | 57 (7.9) | 0.50 (0.06) | 0.64 (0.15) | 0.52 (0.06) |
Case 3 | 30.2 (2.8) | 29 (5.3) | 37 (3.5) | 0.57 (0.14) | 0.72 (0.08) | 0.65 (0.08) |
Case 4 | 34 (3.1) | 33.01 (3.6) | 59 (11.1) | 0.46 (0.07) | 0.36 (0.07) | 0.59 (0.08) |
Case 5 | 42 (7.8) | 43 (6.4) | 58 (8) | 0.15 (0.01) | 0.17 (0.06) | 0.34 (0.06) |
A: Without orthoses (baseline) B: With orthoses (baseline) C: After six weeks (without orthoses).
Table 3
Kinematic variables of hip and knee joints. The mean and standard deviation (SD) of three trials in each condition. Max = maximum; min = minimum
Mean max hip | Mean min hip | Mean max knee | Mean min knee | |||||||||
flexion (SD) | flexion (SD) | flexion (SD) | flexion (SD) | |||||||||
A | B | C | A | B | C | A | B | C | A | B | C | |
Case 1 | 43 (2) | 38 (0) | 41 (1) | 9 (5) | 13 (5) | 15 (2) | 65 (7) | 52 (2) | 57 (3) | 37 (1) | 28 (1) | 35 (1) |
Case 2 | 55 (5) | 51 (3) | 63 (4) | 15 (4) | 9 (2) | 9 (7) | 64 (6) | 49 (5) | 70 (5) | 21 (2) | 19 (7) | 15 (2) |
Case 3 | 35 (2) | 38 (3) | 29 (5) | 5 (3) | 0 (3) | -7 (2) | 63 (1) | 52 (4) | 55 (2) | -3 (1) | 8 (2) | 5 (0) |
Case 4 | 19 (3) | 17 (1) | 22 (0) | -10 (3) | -3 (0) | -12 (2) | 47 (1) | 37 2 | 50 (3) | 10 (1) | 4 (0) | 5 (3) |
Case 5 | 48 (1) | 33 (2) | 58 (8) | 34 (4) | 37 (0) | 21 (5) | 57 (6) | 51 (2) | 69 (4) | 36 (8) | 29 (1) | 23 (4) |
A: Without orthoses (Base line) B: With orthoses (Base line) C: After 6 weeks (without orthoses).
Fig. 2
Hip pattern in sagittal plane without and with dynamic neoprene orthosis. Flex = flexion; ext = extension.
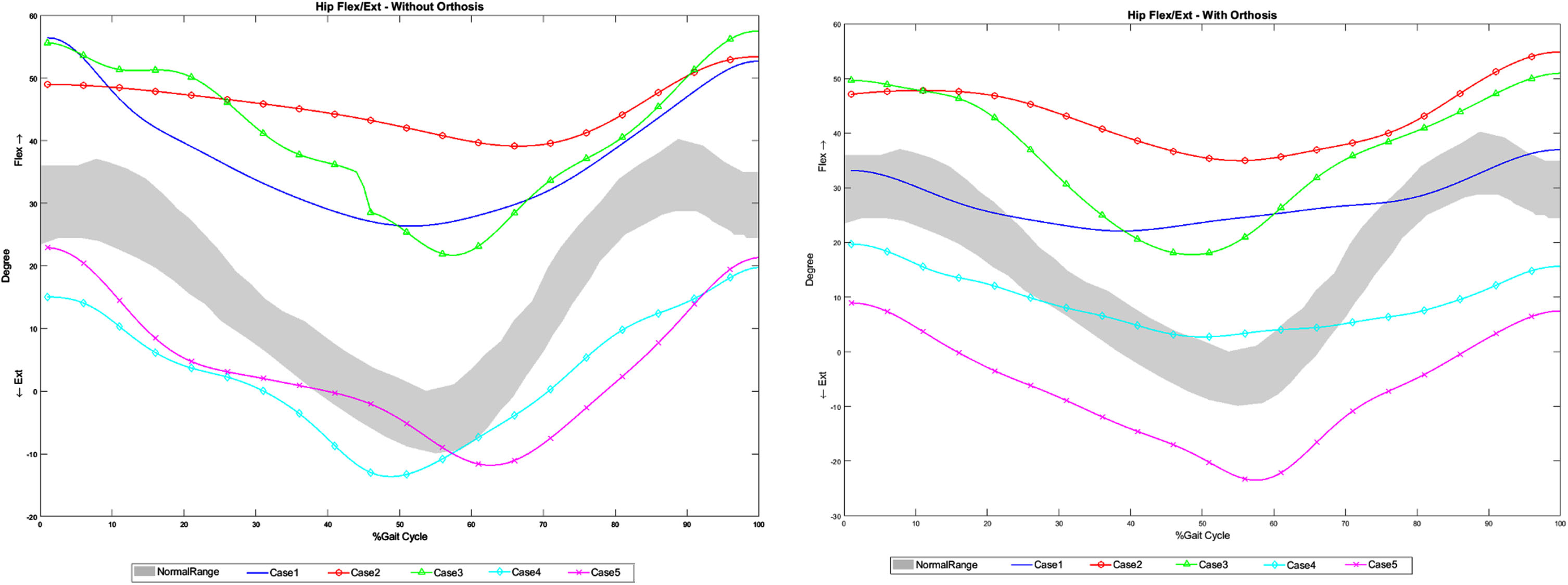
Fig. 3
Knee pattern without and with dynamic neoprene orthosis Flex = flexion; ext = extension.
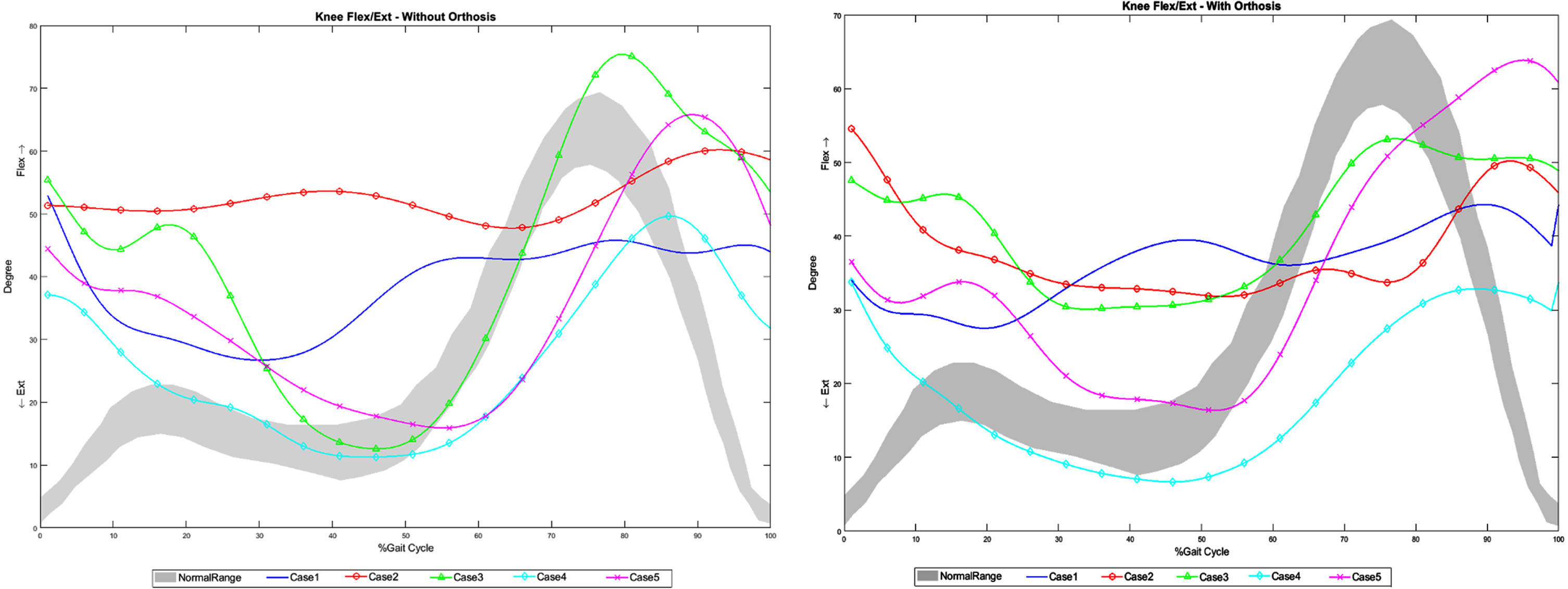
In case one, cadence increased both immediately and after six weeks of intervention, and there was no change in stride length. Moreover, considerable decreases were observed in the mean maximum and minimum knee flexion with the neoprene dynamic orthosis immediately after the intervention.
In case two, spatiotemporal variables, cadence, and stride length increased with the dynamic orthosis immediately after the intervention. Nonetheless, there were not any changes after six weeks of intervention. Indeed, all of the kinematic variables decreased immediately after the intervention. In this case, the decreased mean minimum hip and knee flexion remained constant after six weeks of intervention.
In case three, cadence increased only after six weeks of intervention, and stride length increased immediately and after six weeks. All of the kinematic variables decreased in this case.
In case four, the spatiotemporal variables increased noticeably after six weeks of intervention. Moreover, the mean minimum knee flexion decreased with dynamic orthosis immediately and after six weeks (Table 3).
In case five, increases in cadence and stride length were observed, but the kinematic variables decreased in the knee and hip joints.
Figure 2 shows the hip joint sagittal plane angular displacements in the five children during a gait cycle. Most of the participants showed a tendency to have a lower hip flexion pattern while wearing the dynamic neoprene orthosis. Figure 2 also shows the smoothness in hip joint movement with dynamic neoprene orthosis, especially in case three. Jerking was not observed (Fig. 3).
4Discussion
One of the gait patterns common to children with spastic bilateral CP is the crouch gait pattern, which involves hip and knee flexion and foot dorsiflexion. The present study explored the effects of custom-fabricated neoprene dynamic orthoses on the gait variables of five children with spastic bilateral CP.
Cadence increased immediately and after six weeks in three of the cases, but not in case three or four, in which cadence increased only after six weeks. One study that used full-body Lycra garments worn for at least six hours per day for six weeks reported improvements in proximal stability, which resulted in improvements in self-help and mobility [2]. Considering the effects of dynamic orthosis on most of the variables after six weeks in the current study, the duration of time a dynamic orthosis is worn may improve its effectiveness. Nonetheless, the ideal duration of wearing this type of orthosis has not been determined [22].
A few previous studies have evaluated spatiotemporal variables in Lycra dynamic orthoses: Flanagan et al. examined the kinematics of the lower limb joints, and Rennie et al. focused on the gait patterns of children with CP [2, 22]. In the current study, stride length increased in all of the cases except for case one, in which there was a very small increase in stride length after six weeks of intervention.
The minimum and maximum flexion were analyzed in hip and knee joints (Table 3). The mean of the maximum flexion of the hip in a cycle of gait in the normal kinematics of the hip joint is 40 degrees. On the other hand, the maximum extension (or minimum flexion) of the hip joint is zero and 10 degrees in 38% and 50% of the gait cycle respectively [23]. In case two and case five, the mean of the maximum flexion of the hip joint decreased with dynamic orthosis immediately after the intervention. However, there were not notable changes in the other cases. Moreover, the mean of the minimum hip flexion decreased immediately and after six weeks in all of the cases except for case one. This decreasing trend moved towards normative data, which supports the results of two previous studies. One of these studies examined walking in children with CP using TT garments and strapping, which ensured that the participants’ pelvic and hip kinematic gait data were similar to the data of children who developed typically [22]. The other study suggested that TS, which is similar to the dynamic orthosis used in the current study, reduced the greater hip flexion pattern during a gait cycle [11]; it is believed that this result may stem from the abdominal and back extensor strapping that attempts to facilitate the core trunk stabilizers.
The range of motion of the normal knee joint in a gait cycle is 0-70 degrees [23] but may vary according to the characteristics. The mean of the maximum knee flexion in a gait cycle is 65 degrees and occurs in 70% of the normalized gait cycle. On the other hand, the mean of the minimum knee flexion is about three degrees and occurs in 40% of the phase of gait cycle [23]. In this study, there were decreases in the maximum knee flexion with dynamic orthosis in all of the cases immediately after the intervention. However, there were not any significant changes in some of the cases after six weeks of intervention. This result shows that dynamic orthosis can impose a number of limitations on the movement of the knee joint, which controls knee flexion in the stance phase. In all of the cases, the mean of the minimum knee flexion decreased immediately and after six weeks of intervention. This type of dynamic orthosis decreased the mean of the minimum hip and knee flexion in the gait cycle of patients with a crouch gait pattern, confirming the immediate effects of this type of orthosis on gait kinematics, as well as after six weeks of wearing the orthosis in some of the cases. Likewise, Martins et al. [11] reported that using TS improved the knee and hip joints and decreased flexion patterns. Another previous study showed that dynamic Lycra garments were able to provide patients with an immediate and continuous improvement in balance, proximal joint stability, and postural readiness for movement [2].
The results of this study revealed that wearing dynamic neoprene orthoses could decrease knee flexion in walking. DEFO is similar to an external extension assist and can provide knee and hip extension moments. Consequently, it may decrease the crouch position in some children with spastic diplegia. Based on the findings of this study, the change in the ranges of motion of joints with dynamic orthoses may be related to the controlling and restriction effects of orthoses during gait along with the correction of biomechanical alignments.
The results of the current study support previous studies that demonstrated that Lycra can be considered as a possible mechanism to achieve functional improvements. In a previous study, it was reported that using neoprene stabilizing pressure input orthoses (SPIO) resulted in improvement in movement control and balance [24]. This study by Hylton et al. focused on the direct deep pressure on the skin and the increased internal soft-tissue pressure [24], the latter of which affects both mechanical stability and pressure receptors. Limb position and body awareness can improve when deep pressure receptors provide usable information and provide it to the proprioceptive feedback system. Consequently, the individual wearing the orthosis can precisely control their movement and activate specific muscles. Another previous study suggested that motor learning may occur when children wear the garments. This study argued that this kind of learning might stem from the lingering effects of dynamic orthoses after their use is discontinued [22].
The design of the current study enabled a relatively in-depth evaluation of the changes in each of the individual subjects. This study was limited because data was collected from a small, homogeneous sample of children with similar medical diagnoses (spastic bilateral CP with crouch gait or flexed knee pattern). Nonetheless, the results can pave the way for further structured studies with acceptable statistical power. Another limitation of this study was its focus on the sagittal plane to the exclusion of other body parts, which prevented the generalization of the obtained results.
5Conclusion
The elastic legging orthoses were able to compress the pelvic girdle and the knee joint, which suggests that this type of dynamic orthosis can decrease the flexion angle of the knee and the hip joints in the stance phase of the gait cycle in some children with crouch gait, although this study was limited to children with flexed knee at 1 to 1 + spasticity levels and I-III GMFCS levels. This orthosis caused reduction in the flexion of the knee and hip joints in the swing phase due to its mechanism of creating pressure. Nonetheless, it was dynamic and did not stop the movement in the joints. Moreover, the results varied from subject to subject, and there were signs of improvement in some of the subjects. While it was not possible to draw conclusions at the group level, some individuals may benefit from the garment. Future studies should carry out a functional assessment of this orthosis.
Acknowledgments
The authors express their gratitude to the children, parents, caregivers and clinicians who agreed to participate in this study. Also they would like to acknowledge Dr Mehdi Rassafiani for scientific support. The research leading to these results has not received any funding.
Conflict of interest
The authors declare that there is no conflict of interest.
References
[1] | Rosenbaum P , Paneth N , Leviton A , et al. A report: the definition and classification of cerebral palsy April 2006. Dev Med Child Neurol Suppl. (2007) ;109: :8–14. |
[2] | Rennie DJ , Attfield SF , Morton RE , Polak FJ , Nicholson J . An evaluation of lycra garments in the lower limb using 3-D gait analysis and functional assessment (PEDI), Gait Posture. (2000) ;12: (1):1–6. doi: 10.1016/S0966-6362(00)00066-7. |
[3] | Watson MJ , Crosby P , Matthews M . An evaluation of the effects of a dynamic Lycra® orthosis on arm function in a late stage patient with acquired brain injury, Brain Inj. (2007) ;21: (7):753–61. doi: 10.1080/02699050701481613. |
[4] | Wren TAL , Rethlefsen S , Kay RM . Prevalence of specific gait abnormalities in children with cerebral palsy: influence of cerebral palsy subtype, age, and previous surgery, J Pediatr Ortho. (2005) :25: (1):79–83. doi: 10.1097/00004694-200501000-00018. |
[5] | Abbaskhanian A , Rashedi V , Delpak A , Vameghi R , Gharib M . Rehabilitation Interventions for Children With Cerebral Palsy: A Systematic Review, J Pediatr Rev. (2015) ;3: (1):e361. doi: 10.5812/jpr.361. |
[6] | Hicks JL , Schwartz MH , Arnold AS , Delp SL . Crouched postures reduce the capacity of muscles to extend the hip and knee during the single-limb stance phase of gait, J Biomech. (2008) ;41: (5):960–7. doi: 10.1016/j.jbiomech.2008.01.002. |
[7] | Steele KM , Seth A , Hicks JL , Schwartz MS , Delp SL . Muscle contributions to support and progression during single-limb stance in crouch gait, J Biomech. (2010) ;43: (11):2099–105. doi: 10.1016/j.jbiomech.2010.04.003. |
[8] | Hsu JD , Michael JW , Fisk JR. AAOS atlas of orthoses and assistive devices. 4th ed. Mosby Elsevier: Philadelphia; (2008) . |
[9] | Wheater M . Cerebral palsy, principles and management, Archives of Disease in Childhood. (2005) ;90: :657. doi: 10.1136/adc.2004.065797. |
[10] | Coghill JE , Simkiss DE . Question 1 Do lycra garments improve function and movement in children with cerebral palsy? Arch Dis Child. (2010) ;95: (5):393–5. doi: 10.1136/adc.2009.178624. |
[11] | Martins E , Cordovil R , Oliveira R , Pinho J , Diniz A , Vaz JR . The immediate effects of a dynamic orthosis on gait patterns in children with unilateral spastic cerebral palsy: A kinematic analysis. Front Pediatr. (2019) ;7: :42. doi: 10.3389/fped.2019.00042. |
[12] | Matthews MJ , Watson M , Richardson B . Effects of dynamic elastomeric fabric orthoses on children with cerebral palsy, Prosthet Orthot Int. (2009) ;33: (4):339–47. doi: 10.3109/03093640903150287. |
[13] | Bahramizadeh M , Mousavi ME , Rassafiani M , et al. The effect of floor reaction ankle foot orthosis on postural control in children with spastic cerebral palsy, Prosthet Orthot Int. (2012) ;36: (1):71–6. doi: 10.1177/0309364611429855. |
[14] | Damiano DL . Meaningfulness of mean group results for determining the optimal motor rehabilitation program for an individual child with cerebral palsy, Dev Med Child Neurol. (2014) ;56: (12):1141–1146. doi: 10.1111/dmcn.12505. |
[15] | Clopton N , Dutton J , Featherston T , Grigsby A , Mobley J , Melvin J . Interrater and intrarater reliability of the Modified Ashworth Scale in children with hypertonia, Pediatr Phys Ther. (2005) ;17: (4):268–74. doi: 10.1097/01.pe0000186509.41238.1a. |
[16] | Palisano R , Rosenbaum P , Walter S , Russell D , Wood E , Galuppi B . Development and reliability of a system to classify gross motor function in children with cerebral palsy, Dev Med Child Neurol. (1997) ;39: (4):214–23. doi: 10.1111/j.1469-8749.1997.tb07414.x. |
[17] | Rha D-W , Kim DJ , Park ES . Effect of hinged ankle-foot orthoses on standing balance control in children with bilateral spastic cerebral palsy, Yonsei Med J. (2010) ;51: (5):746–52. doi: 10.3349/ymj.2010.51.5.746. |
[18] | Vuillermin C , Rodda J , Rutz E , Shore BJ , Smith K , Graham HK . Severe crouch gait in spastic diplegia can be prevented: a population-based study, J Bone Joint Surg Br. (2011) ;93: (12):1670–5. doi: 10.1302/0301-620X.93B12.27332. |
[19] | Knox V . The use of lycra garments in children with cerebral palsy: a report of a descriptive clinical trial, British Journal of Occupational Therapy. (2003) ;66: (2):71–77. doi: 10.1177/030802260306600205. |
[20] | Kadaba MP , Ramakrishnan HK , Wootten ME . Measurement of lower extremity kinematics during level walking, J Orthop Res. (1990) ;8: (3):383–92. doi: 10.1002/jor.1100080310. |
[21] | Nieuwenhuys A , Papageorgiou E , Pataky T , De Laet T , Molenaers G , Desloovere K . Literature review and comparison of two statistical methods to evaluate the effect of botulinum toxin treatment on gait in children with cerebral palsy, PLoS One. (2016) ;11: (3):e0152697. doi: 10.1371/journal.pone.0152697. |
[22] | Flanagan A , Krzak J , Peer M , Johnson P , Urban M . Evaluation of short-term intensive orthotic garment use in children who have cerebral palsy, Pediatr Phys Ther. (2009) ;21: (2):201–4. doi: 10.1097/PEP.0b013e3181a347ab. |
[23] | Perry J , Burnfield JM . Gait analysis: normal and pathological function, 2nd ed. Thorofare, NJ: Slack Incorporated; (2010) . |
[24] | Hylton N , Allen C . The development and use of SPIO Lycra compression bracing in children with neuromotor deficits, Pediatr Rehabil. (1997) ;1: (2):109–16. doi: 10.3109/17518429709025853. |