Immunophenotyping Tracks Motor Progression in Parkinson’s Disease Associated with a TH Mutation
Abstract
Background:
Parkinson’s disease (PD) is the second most common neurodegenerative disorder, with genetic factors accounting for about 15% of cases. There is a significant challenge in tracking disease progression and treatment response, crucial for developing new therapies. Traditional methods like imaging, clinical monitoring, and biomarker analysis have not conclusively tracked disease progression or treatment response in PD. Our previous research indicated that PD patients with increased dopamine transporter (DAT) and tyrosine hydroxylase (TH) in peripheral blood mononuclear cells (PBMCs) might show disease progression and respond to levodopa treatment.
Objective:
This study evaluates whether DAT- and TH-expressing PBMCs can monitor motor progression in a PD patient with a heterozygous TH mutation.
Methods:
We conducted a longitudinal follow-up of a 46-year-old female PD patient with a TH mutation, assessing her clinical features over 18 months through DaT scans and PBMC immunophenotyping. This was compared with idiopathic PD patients (130 subjects) and healthy controls (80 age/sex-matched individuals).
Results:
We found an increase in DAT+ immune cells concurrent with worsening motor scores (UPDRS-III). Following levodopa therapy, unlike idiopathic PD patients, TH+ immune cell levels in this patient remained high even as her motor scores improved.
Conclusions:
Longitudinal immunophenotyping in this PD patient suggests DAT+ and TH+ PBMCs as potential biomarkers for tracking PD progression and treatment efficacy, supporting further exploration of this approach in PD research.
Plain Language Summary
Parkinson’s disease, the second most prevalent neurodegenerative disease, has traditionally been considered a disorder of the brain. However, recent studies have shown that it also involves changes in the immune cells in the blood. Our previous studies demonstrated that Parkinson’s disease patients have higher levels of immune cells expressing two proteins, dopamine transporter (DAT) and tyrosine hydroxylase (TH). In this study, we explored whether these markers could help monitor disease progression or treatment response over time in a patient with a TH mutation. By analyzing blood samples from this patient over several months, we observed that the levels of immune cells expressing DAT and TH increase with the worsening of symptoms. Interestingly, unlike in idiopathic Parkinson’s disease patients, where effective treatment reduces both DAT and TH expressing immune cells, in this patient with a TH mutation, only the number of DAT-expressing immune cells decreases. These findings indicate that measuring DAT and TH expression in blood immune cells could be a valuable blood marker for tracking disease progression and treatment response in Parkinson’s disease patients.
INTRODUCTION
Parkinson’s disease (PD) is the second most prevalent neurodegenerative syndrome and is the fastest growing [1]. PD is not one disease, and thus the pathophysiology across known causes is complex. Genetic factors account for ∼15% of PD cases [2, 3] with several known pathogenic gene mutations [4, 5]. Many of the symptoms and some of the progression patterns in PD are similar, whether idiopathic or genetic [6, 7]. Currently, tracking the progression of PD or its response to symptomatic treatments, such as levodopa, has been attempted using imaging modalities such as DAT and MRI, as well as skin biopsy and MIBG cardiac testing [8–14]. While recent research has highlighted several promising disease progression markers [15–17], their longitudinal applicability in monitoring progression for individual PD patients has not been clearly demonstrated.
We previously studied peripheral blood immunophenotyping as an approach to disease progression. We used a cross-sectional design with 130 PD patients and the data revealed that peripheral immune cells expressed both a marker for dopamine transporter (DAT+) and tyrosine hydroxylase (TH+) in idiopathic PD [17]. The levels of DAT and TH decreased following treatment with levodopa and coincident improvement in UPDRS-III (PD motor) scores [17]. Herein, we report the case of a single PD subject who carried a heterozygous TH mutation and we performed immunophenotyping of peripheral blood mononuclear cells (PBMCs) to determine the consistency of the findings with idiopathic PD.
RESULTS AND DISCUSSION
Patient information and diagnostic
The patient was a 46-year-old female who presented with a right-hand resting tremor, bradykinesia, and rigidity which slowly progressed over a period of two years. She was of Asian ancestry, born in south Korea and was adopted in the USA at the age of 4 years. She previously met her biological family and reported no family history of parkinsonism or other neurological conditions. She had a long history of constipation and hyposmia prior to the development of her motor symptoms. Her symptoms progressed with increased bradykinesia, shuffling gait, lower extremity pain, dystonia and impaired hand dexterity. She was initially treated with a dopamine agonist due to her age and risk of motor complications. Because of a lack of meaningful benefit and persistent nausea, carbidopa/levodopa was initiated. Her levodopa dose was titrated over time to 700 mg/day in three divided doses. She showed marked, clear and sustained response to levodopa and improvement in her motor symptoms. Her initial motor UPDRS score prior to initiation of therapy was 27, and this was reduced to a motor score of 10 at 24 months following optimization of her medical therapy. DaTscans were collected at baseline and at 11 months. The series of DaTscans revealed a pattern consistent with parkinsonism (Fig. 1). Methods are provided in Supplementary Material: DaTscan.
Fig. 1
DaTscan reveals left-side asymmetry in striatal DAT binding and is not qualitatively different 11 months after initial scan. Study showing reduced striatal DAT binding both at study initiation and ∼11 months (10 months and 28 days) afterwards. Despite worsened symptoms and clinical presentation, two scans 11 months apart do not reveal changes in asymmetry.
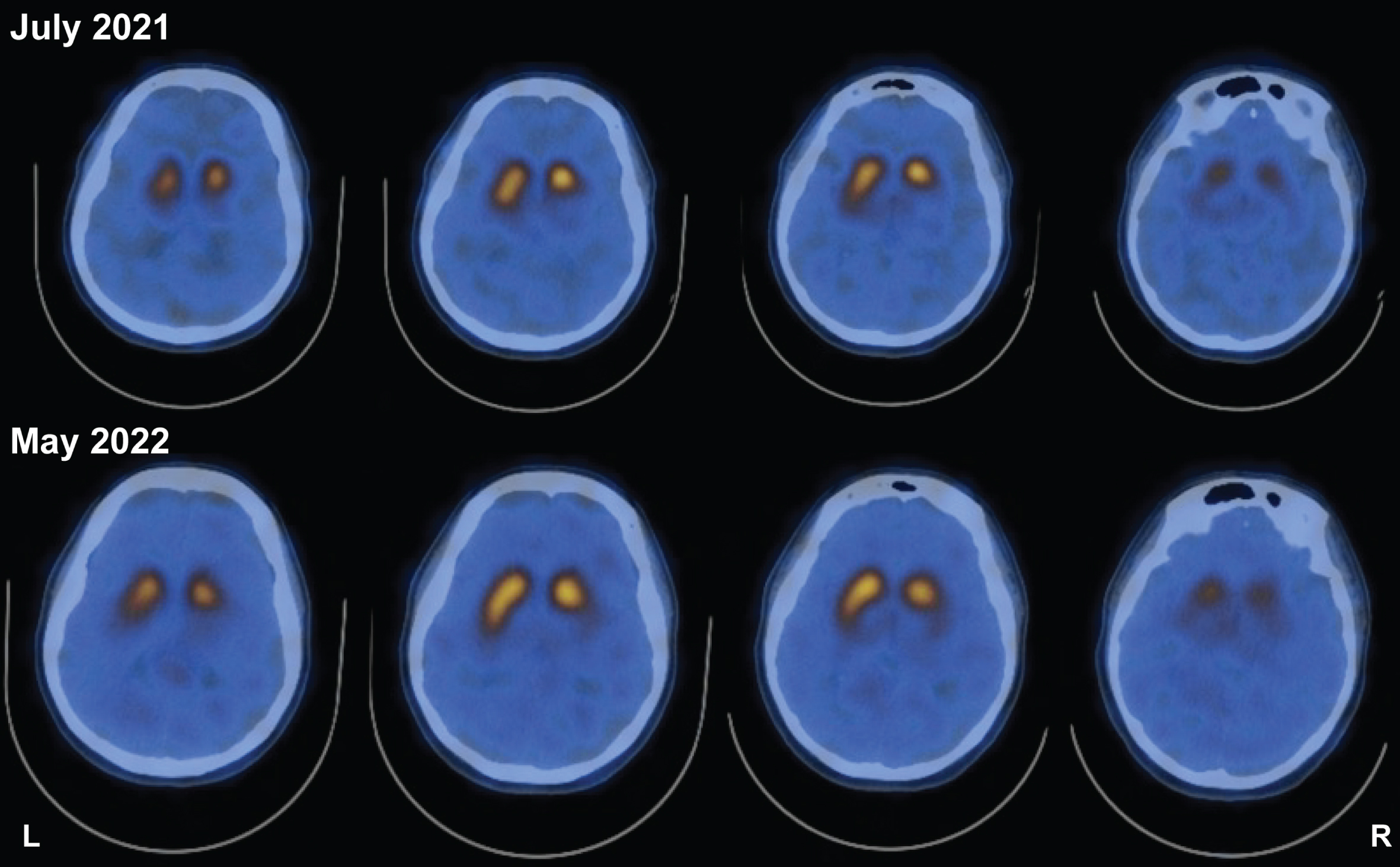
Sequencing
Whole genome sequencing was completed at a median 32X coverage (63% bases > 30X) by Illumina 150bp paired-end reads. All known genes for parkinsonism were examined. The patient was found to be a heterozygous carrier of a pathogenic variant in TH (GRCh37/hg19 chr11:2,186,975-6; ENST00000381178 c.1215_1216delCCinsTT; p.Glu406*; CADD v1.6 = 49). The sequencing depth at this position was 62 reads (Ref F:R 15:20, Alt F:R 18:9). The global minor allele frequency of this mutation in control individuals was 1.59E-06 (1/627,326 alleles) albeit with a frequency of 2.8E-05 in East Asia (1/36,048 alleles; gnomAD v4.0.0). A detailed inspection of read alignment, including all single nucleotide and structural variants in the locus, did not identify any other potentially damaging variants in the gene, and this was consistent with the patient possessing at least one normal TH allele. TH mutations have been associated with autosomal recessive Segawa syndrome, an infantile/juvenile onset dopa-responsive dystonia-parkinsonism disorder. Heterozygous TH carriers are not known to be symptomatic, however a single case documents a heterozygous pathogenic mutation in an individual with levodopa-responsive dystonia [18]. Methods are provided in the Supplementary Material: Genetics. Sequencing results in graphical form are provided in SupplementaryFigure 1.
In idiopathic PD and in this PD patient carrying a mutation in the gene encoding TH, changes in DAT+ PBMCs were coupled to changes in motor function: increases were coupled with worsening motor function prior to carbidopa:levodopa therapy, and decreases in DAT+ PBMCs were coupled with improved UPDRS-III scores following carbidopa/levodopa treatment. Unlike idiopathic PD cases which we previously described [17], changes in TH+ PBMCs and UPDRS-III scores were uncoupled. Consistent with previous reports, we found that in idiopathic PD, carbidopa/levodopa treatment reduced the levels of DAT+ and TH+ PBMCs (Fig. 2A, B). Therefore, in idiopathic PD, there was a direct correlation between changes in TH+ PBMCs, DAT+ PBMCs, carbidopa/levodopa and UPDRS-III scores. The immunophenotyping of a PD patient carrying a pathogenic TH mutation presented an opportunity to assess the association of the DAT and TH markers in this patient, and to compare them to an idiopathic PD cohort (n = 130) and to an age/sex matched healthy control cohort (n = 80). As described above, the patient presented classic PD symptoms, and we hypothesized increased DAT+ and TH+ PBMCs at the time of PD diagnosis (drug naïve) would be higher than age/sex matched healthy subjects and would be coupled to improvement of UPDRS-III scores following carbidopa/levodopa treatment.
Fig. 2
DAT+ and TH+ PBMCs are increased in idiopathic PD patients at the time of diagnosis and decreased following improved UPDRS-III scores as a consequence of L-DOPA/Carbidopa treatment. By contrast, unlike the DAT+ PBMCs, in a patient carrying a TH mutation, the TH+ PBMCs remains elevated even after successful pharmacotherapy, representing an uncoupled response to L-DOPA/Carbidopa treatment. Single cells, free from debris, were analyzed via flow cytometry for expression of DAT and TH (Supplementary Figure 2). A-B) DAT+ and TH+ PBMCs (grey dots) are increased in drug naïve idiopathic PD patients (n = 16) relative to healthy controls (n = 80, green dashed line). These markers are reduced significantly in drug treated idiopathic PD patients (n = 114), though remaining significantly higher than age/sex matched healthy control levels (***p < 0.001; t,df(3.763, 128), **p < 0.01; t,df(3.138, 128), unpaired Student’s t-test with Tukey’s correction, alpha = 0.05). In the PD patient carrying a single pathogenic TH mutation (red line), A) the pattern of DAT+ PBMCs change follows the pattern we identified in idiopathic PD patients, i.e., increased at the time of PD diagnosis (drug naïve condition) and then decreasing following successful L-DOPA/Carbidopa treatment. However, B) TH+ PBMCs of the PD patient carrying pathogenic TH mutation are uncoupled from both DAT+ PBMCs and the predicted reduction following L-DOPA/Carbidopa treatment, for instance, they are elevated in the drug naïve condition and continue to increase despite improved UPDRS-III, as a results of L-DOPA/Carbidopa treatment. C) Similar to idiopathic PD patients, the PD patient carrying pathogenic TH mutation, DAT+ PBMCs (red line) are elevated at the first visit (drug naïve) and remain elevated as a function of impaired motor function (UPDRS-III, black line). Once L-DOPA/Carbidopa treatment is initiated, both DAT+ PBMCs and UPDRS-III scores decrease, indicating positive response to pharmacotherapy. D) In the PD patient carrying pathogenic TH mutation, the TH+ PBMCs (blue line) are increased following worsening motor symptoms (UPDRS-III, black line), but TH+ PBMCs continue to rise despite positive response to L-DOPA/Carbidopa (indicated by decreasing UPDRS-III motor scores), suggesting in this patient, only changes in the DAT+ PBMCs are coupled to both worsening motor function and positive L-DOPA/Carbidopa treatment response.
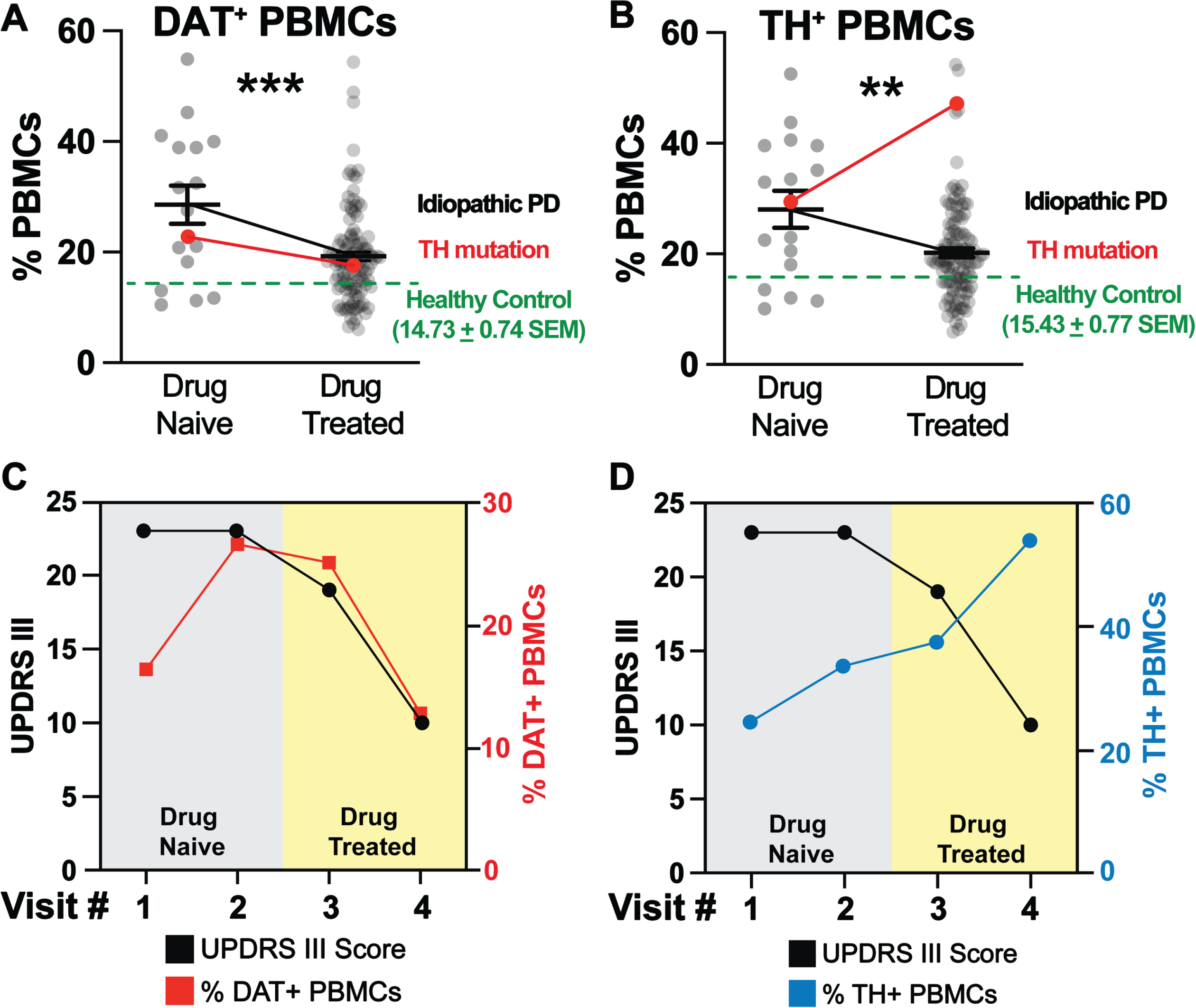
Consistent with our prior findings [17], at the time of diagnosis (drug naïve), idiopathic PD patients (n = 130) exhibited increased DAT+ and TH+ PBMCs (Fig. 2A, B, grey dots, black line) relative to age/sex matched healthy control levels (green dashed line, n = 80). Idiopathic PD patients show a significant decrease in DAT+ and TH+ PBMCs, and improved UPDRS-III scores following carbidopa/levodopa treatment (Fig. 2A, B, grey dots, black line, Fig. 2A, B, green). Similar to idiopathic PD patients, the patient with TH mutation revealed an increase in DAT+ PBMCs (Fig. 2A, red line). Consistent with our hypothesis, carbidopa/levodopa treatment provided symptom relief marked by reduced UPDRS III scores (Fig. 2C, black line) and was associated with a reduced frequency of DAT+ PBMCs (Fig. 2C, red line). Thus, the dynamic changes in DAT+ PBMCs followed a similar pattern in this subject as in idiopathic PD cohort. Consistent with our hypothesis, in the patient with TH mutation, TH+ PBMCs were increased at the time of diagnosis (drug Naïve), but contrary to our hypothesis they remained high and continued to rise despite carbidopa/levodopa and the improved UPDRS-III scores (Fig. 2B, red line, blue line, black line respectively). This finding was in contrast to that observed in idiopathic PD, where there was a direct correlation between decreased TH+ PBMC frequencies and improved UPDRS-III scores following carbidopa/levodopa treatment. The detailed methods are provided in the Supplementary Information: PBMC isolation and flow cytometry.
Unlike what is observed in a DaTscan, increases in DAT-expressing PBMC immunophenotype reflect PD progression. In the patient with a TH mutation, the asymmetric reduction in DaTscan (Fig. 1) at the time of PD diagnosis was reflected by an increase in DAT+ PBMCs (Fig. 2) and UPDRS-III scores. However, the longitudinal DaTscan signal, measured 11 months later, was unchanged with no qualitatively differences observed between scans, despite the worsening of UPDRS-III scores, and the increase in DAT+ PBMCs thus suggesting the peripheral immunophenotype may provide a more sensitive means to monitor disease progression.
Concluding remarks
The clinical manifestations, symptoms and progression of PD are continuous regardless of whether its origin is idiopathic or genetic [6, 19, 20]. The use of immune system-based approaches for diagnosing and monitoring PD is a relatively new and evolving field. There has been a considerable amount of large-scale and population-based research in this area, yet few longitudinal studies have systematically examined immunophenotyping in longitudinal PD cohorts [21–23]. Here we present a case of a PD patient with a heterozygous TH mutation. Longitudinal within subject sampling suggests that DAT+ PBMCs could serve as an indicator of disease progression, treatment response and as a potential diagnostic tool. We show that a TH gene variant can modulate TH-expression in peripheral immune cells, and we posit that this may be causal or a consequence of PD in this specific patient. As studies of other PD-associated gene variants have suggested [24–27], genotype may affect the immunological profile in PD and should be taken into consideration when evaluating immunological tools for PD assessment.
Consistent with our previous study [17] we documented that as UPDRS-III scores worsened, there was an elevation in DAT+ immune cells that was reduced after treatment with carbidopa/levodopa and there was subsequent improvement in UPDRS-III scores. The dynamic nature of DAT+ peripheral immune cells in the current study which was also conducted in idiopathic PD patients corroborate previous cross-sectional findings in idiopathic patients on this marker [17]. Intriguingly, in this PD patient harboring a heterozygous TH mutation, unlike DAT+ immune cells, the increase in TH+ immune cells persisted even following the positive response to carbidopa/levodopa. The current study marks the first instance of longitudinal immunophenotyping of a PD patient with TH mutation, highlighting DAT+ and TH+ PBMCs as potential indicators of PD, its progression, and the evolution of changes with treatment. Notably, the discernable disease progression and increased PD symptom severity, which was evident clinically, along with the changes of the DAT+ immunophenotype, were not reflected in two separate qualitative DaTscan’s collected 11 months apart. Prior studies have suggested an estimated annual decrease in striatal DAT binding of 13% during the first year [28].
The limitations of the present study include the absence of longitudinal immunophenotyping and longitudinal DaTscan data for the idiopathic PD patient cohort allowing disease progression identification and treatment response across various patient subgroups. Another limitation is the absence of quantitative DaTscan data -due to technical limitation with the type of scan performed- on the patient with TH mutation. While the data presented in this study suggest the identified PBMC immunophenotype can be used for disease detection, tracking progression of and response to pharmacotherapy in idiopathic PD patients, the inclusion of other genetic PD such as LRRK2 or GBA mutations may identify additional immunophenotypic differences. In conclusion, our findings underscore the notion that peripheral immunophenotype with regards to PBMCs expressing DAT offers a sensitive and robust tool for tracking disease onset, progression and therapeutic response in idiopathic PD and in a single case of a PD patient with TH mutation.
ACKNOWLEDGMENTS
We acknowledge the University of Florida ICBR Flow Cytometry core and the Center for Immunology and Transplantation, for technical assistance.
FUNDING
This study acknowledges support from the Evelyn F. and William L. McKnight Brain Institute’s Gator NeuroScholar’s program (to A.G.), T32-NS082169 (to A.G.) and the Karen Toffler Charitable Trust (to A.G.). In addition, these studies were accomplished using instrumentation and technical assistance from the University of Florida Center for Immunology and Transplantation.
CONFLICT OF INTEREST
Matthew Farrer is an editorial board member but was not involved in the peer-review process of this article, nor received any information regarding its peer-review. All other authors declare no conflicts of interest.
SUPPLEMENTARY MATERIAL
[1] The supplementary material is available in the electronic version of this article: https://dx.doi.org/10.3233/JPD-240030.
REFERENCES
[1] | Ou Z , Pan J , Tang S , Duan D , Yu D , Nong H , Wang Z ((2021) ) Global trends in the incidence, prevalence, and years lived with disability of Parkinson’s disease in 204 countries/territories from 1990 to 2019. Front Public Health 9: , 776847. |
[2] | Deng H , Wang P , Jankovic J ((2018) ) The genetics of Parkinson disease. Ageing Res Rev 42: , 72–85. |
[3] | Blauwendraat C , Nalls MA , Singleton AB ((2020) ) The genetic architecture of Parkinson’s disease. Lancet Neurol 19: , 170–178. |
[4] | Jia F , Fellner A , Kumar KR ((2022) ) Monogenic Parkinson’s disease: Genotype, phenotype, pathophysiology, and genetic testing. Genes (Basel) 13: , 471. |
[5] | Guadagnolo D , Piane M , Torrisi MR , Pizzuti A , Petrucci S ((2021) ) Genotype-phenotype correlations in monogenic Parkinson disease: A review on clinical and molecular findings. Front Neurol 12: , 648588. |
[6] | Correia Guedes L , Mestre T , Outeiro TF , Ferreira JJ ((2020) ) Are genetic and idiopathic forms of Parkinson’s disease the same disease? J Neurochem 152: , 515–522. |
[7] | Tolosa E , Garrido A , Scholz SW , Poewe W ((2021) ) Challenges in the diagnosis of Parkinson’s disease. Lancet Neurol 20: , 385–397. |
[8] | Heim B , Krismer F , De Marzi R , Seppi K ((2017) ) Magnetic resonance imaging for the diagnosis of Parkinson’s disease. J Neural Transm (Vienna) 124: , 915–964. |
[9] | Doppler K ((2021) ) Detection of dermal alpha-synuclein deposits as a biomarker for Parkinson’s disease. J Parkinsons Dis 11: , 937–947. |
[10] | Gibbons C , Wang N , Rajan S , Kern D , Palma JA , Kaufmann H , Freeman R ((2023) ) Cutaneous α-synucleinsignatures in patients with multiple system atrophy and Parkinson disease. Neurology 100: , e1529–e1539. |
[11] | Pitton Rissardo J , Fornari Caprara AL ((2023) ) Cardiac 123I-metaiodobenzylguanidine (MIBG) scintigraphy in Parkinson’s disease: A comprehensive review. Brain Sci 13: , 1471. |
[12] | Skowronek C , Zange L , Lipp A ((2019) ) Cardiac 123I-MIBG scintigraphy in neurodegenerative Parkinson syndromes: Performance and pitfalls in clinical practice. Front Neurol 10: , 152. |
[13] | Catalan M , Dore F , Polverino P , Bertolotti C , Sartori A , Antonutti L , Cucca A , Furlanis G , Capitanio S , Manganotti P ((2021) ) 123I-metaiodobenzylguanidine myocardial scintigraphy in discriminating degenerative parkinsonisms. Mov Disord Clin Pract 8: , 717–724. |
[14] | Lee JY , Martin-Bastida A , Murueta-Goyena A , Gabilondo I , Cuenca N , Piccini P , Jeon B ((2022) ) Multimodal brain and retinal imaging of dopaminergic degeneration in Parkinson disease. Nat Rev Neurol 18: , 203–220. |
[15] | Qi R , Sammler E , Gonzalez-Hunt CP , Barraza I , Pena N , Rouanet JP , Naaldijk Y , Goodson S , Fuzzati M , Blandini F , Erickson KI , Weinstein AM , Lutz MW , Kwok JB , Halliday GM , Dzamko N , Padmanabhan S , Alcalay RN , Waters C , Hogarth P , Simuni T , Smith D , Marras C , Tonelli F , Alessi DR , West AB , Shiva S , Hilfiker S , Sanders LH ((2023) ) A blood-based marker of mitochondrial DNA damage in Parkinson’s disease. Sci Transl Med 15: , eabo1557. |
[16] | Di Maio R , Hoffman EK , Rocha EM , Keeney MT , Sanders LH , De Miranda BR , Zharikov A , Van Laar A , Stepan AF , Lanz TA , Kofler JK , Burton EA , Alessi DR , Hastings TG , Greenamyre JT ((2018) ) LRRK2 activation in idiopathic Parkinson’s disease. Sci Transl Med 10: , eaar5429. |
[17] | Gopinath A , Mackie P , Hashimi B , Buchanan AM , Smith AR , Bouchard R , Shaw G , Badov M , Saadatpour L , Gittis A , Ramirez-Zamora A , Okun MS , Streit WJ , Hashemi P , Khoshbouei H ((2022) ) DAT and TH expression marks human Parkinson’s disease in peripheral immune cells. NPJ Parkinsons Dis 8: , 72. |
[18] | Bally JF , Breen DP , Schaake S , Trinh J , Rakovic A , Klein C , Lang AE ((2020) ) Mild dopa-responsive dystonia inheterozygous tyrosine hydroxylase mutation carrier: Evidence of symptomatic enzyme deficiency?}. ParkinsonismRelat Disord 71: , 44–45. |
[19] | Jankovic J , Tan EK ((2020) ) Parkinson’s disease: Etiopathogenesis and treatment. J Neurol Neurosurg Psychiatry 91: , 795–808. |
[20] | Schiesling C , Kieper N , Seidel K , Krüger R ((2008) ) Review: Familial Parkinson’s disease–genetics, clinical phenotype and neuropathology in relation to the common sporadic form of the disease. Neuropathol Appl Neurobiol 34: , 255–271. |
[21] | Espinosa-Cárdenas R , Arce-Sillas A , Álvarez-Luquin D , Leyva-Hernández J , Montes-Moratilla E , González-Saavedra I , Boll MC , Garcia-Garcia E , Ángeles-Perea S , Fragoso G , Sciutto E , Adalid-Peralta L ((2020) ) Immunomodulatory effect and clinical outcome in Parkinson’s disease patients on levodopa-pramipexole combotherapy: A two-year prospective study. J Neuroimmunol 347: , 577328. |
[22] | Lindestam Arlehamn CS , Dhanwani R , Pham J , Kuan R , Frazier A , Rezende Dutra J , Phillips E , Mallal S , Roederer M , Marder KS , Amara AW , Standaert DG , Goldman JG , Litvan I , Peters B , Sulzer D , Sette A ((2020) ) α-Synuclein-specific T cell reactivity is associated with preclinical and early Parkinson’s disease. Nat Commun 11: , 1875. |
[23] | Williams-Gray CH , Wijeyekoon R , Yarnall AJ , Lawson RA , Breen DP , Evans JR , Cummins GA , Duncan GW , Khoo TK , Burn DJ , Barker RA ((2016) ) Serum immune markers and disease progression in an incident Parkinson’s disease cohort (ICICLE-PD). Mov Disord 31: , 995–1003. |
[24] | Al-Azzawi ZAM , Arfaie S , Gan-Or Z ((2022) ) GBA1 and the immune system: A potential role in Parkinson’s disease? J Parkinsons Dis 12: , S53–s64. |
[25] | Kung PJ , Elsayed I , Reyes-Pérez P , Bandres-Ciga S ((2022) ) Immunogenetic determinants of Parkinson’s disease etiology. J Parkinsons Dis 12: , S13–s27. |
[26] | Tansey MG , Wallings RL , Houser MC , Herrick MK , Keating CE , Joers V ((2022) ) Inflammation and immune dysfunction in Parkinson disease. Nat Rev Immunol 22: , 657–673. |
[27] | Gate D ((2022) ) New perspectives on immune involvement in Parkinson’s disease pathogenesis. J Parkinsons Dis 12: , S5–s11. |
[28] | Ikeda K , Ebina J , Kawabe K , Iwasaki Y ((2019) ) Dopamine transporter imaging in Parkinson disease: Progressive changes and therapeutic modification after anti-parkinsonian medications. Intern Med 58: , 1665–1672. |