Biofluid Detection of Pathological α-Synuclein in the Prodromal Phase of Synucleinopathies
Abstract
Synucleinopathies are disorders characterized by the aggregation and deposition of pathological α-synuclein conformers. The underlying neurodegenerative processes begin years or decades before the onset of cardinal motor symptoms. This prodromal phase may manifest with various signs or symptoms. However, there are no current standardized laboratory tests to ascertain the progression and conversion of prodromal conditions such as mild cognitive impairment, isolated REM sleep behavior disorder or pure autonomic failure. The aim of this systematic review was to evaluate the diagnostic possibilities using human biofluids as source material to detect pathological α-synuclein in the prodromal phase of synucleinopathies. Our review identified eight eligible studies, that investigated pathological α-synuclein conformers using cerebrospinal fluid from patients with prodromal signs of synulceinopathies to differentiate this patient group from non-synucleinopathies, while only one study investigated this aspect using blood as medium. While previous studies clearly demonstrated a high diagnostic performance of α-synuclein seed amplification assays for differentiating synucleinopathies with Lewy bodies from healthy controls, only few analyses were performed focussing on individuals with prodromal disease. Nevertheless, results for the early detection of α-synuclein seeds using α-synuclein seed amplification assays were promising and may be of particular relevance for future clinical trials and clinical practice.
INTRODUCTION
Synucleinopathies are neurodegenerative diseases that are characterized by abnormal misfolding and aggregation of α-synuclein (α-syn) and include Parkinson’s disease (PD), dementia with Lewy bodies (DLB) and multiple system atrophy (MSA) [1]. All of these diseases are known to be characterized by a long prodromal phase in which clinical manifest cognitive and motor symptoms have not yet emerged [2, 3]. Due to the slowly progressing neurodegenerative process, several phases can be defined [4]. In the beginning, already before the onset of neurodegeneration, a risk phase exists, in which different factors like genetic, environmental, or behavioral aspects are involved [5–8]. The following preclinical phase is determined by the initiation of progressive neurodegenerative pathology [9]. Clinical symptoms or potential signs are absent. The subsequent prodromal phase is characterized by the occurrence of first symptoms indicating ongoing neurodegeneration [10]. Symptoms of the prodromal phase of synucleinopathies may include mild motor signs, mild cognitive impairment, isolated rapid-eye-movement sleep behavior disorder (iRBD), olfactory loss, autonomic dysfunction, and depression [9, 11–14]. Transition to the clinical motor phase of PD is reached if manifest bradykinesia with rest tremor and/or rigidity are present. Transition from iRBD to dementia occurs when the patient has overt memory complaints, abnormal performance in cognitive tests plus dependencies in daily living activities. MSA clinically presents with autonomic failure, parkinsonism, and a cerebellar syndrome in various combinations [15]. Prodromal signs of MSA include iRBD, urogenital failure, subtle parkinsonian and/or cerebellar signs, stridor, and orthostatic hypotension. Central feature of DLB is the presence of dementia [16]. Fluctuating cognition with cognitive decline, visual hallucinations, and iRBD are known as core clinical features. Autonomic dysfunction, depression, hyposmia and constipation are only few potential prodromal symptoms. The onset and progression of motor/non-motor symptoms can differ enormously between individuals [3, 5].
The pathological hallmarks of synucleinopathies are intraneuronal (PD, DLB) or oligondendroglial (MSA) accumulation and aggregation of misfolded α-synuclein (α-syn) [17, 18]. α-syn is able to form monomers as well as soluble oligomers under physiological conditions. In the pathological state, however, toxic oligomers are formed, which can become insoluble and aggregate to amyloid fibrils— as they are found in brains of patients as Lewy bodies and Lewy neurites [18]. Pathological α-syn conformers (seeds) are able to recruit soluble monomers or soluble oligomers by a seeded polymerization mechanism to form larger oligomeric forms or aggregate into fibrillar structures [19, 20]. The ability to detect synucleinopathy-specific α-syn conformers in different biofluids or tissues by the amplification of α-syn seeds using seed amplification assay (SAA) protocols, which take advantage of the seeding properties of pathological α-syn conformers to amplify small quantities of α-syn seeds, is widely known. So far, SAA protocols have been tested in cerebrospinal fluid (CSF), saliva, skin biopsies, olfactory mucosa biopsies and blood plasma or serum including extracellular vesicles (EVs) derived from serum or plasma [21–23]. Prior studies have shown that α-syn SAA using CSF distinguish PD/DLB and MSA patients from healthy controls with high sensitivity and specificity [21, 23]. Moreover, it has recently been demonstrated that SAA from blood is also able to distinguish between PD/DLB, MSA and healthy control samples [24, 25].
In the prodromal phase, patients show specific or mild clinical symptoms that make an early and accurate diagnosis challenging. The ability of liquid biopsy-based SAA to detect synucleinopathies at the earliest clinical disease stages is promising and of particular interest. In this section, we provide an overview of the current findings regarding the detection of α-syn seeds in human biofluids (CSF/blood) in the earliest phase of synucleinopathies.
CSF-BASED DETECTION OF α-SYN AGGREGATION
Based on the knowledge about biochemical abnormalities in the central nervous system, total α-syn in CSF has been proposed as a biomarker for distinguishing PD patients from healthy controls and individuals with atypical parkinsonisms. Specifically, total α-syn CSF concentration was shown to be significantly decreased in individuals with PD and MSA compared to healthy controls and tauopathies such as progressive supranuclear palsy (PSP) and corticobasal degeneration (CBD) [26]. However, in 2017, Eusebi et al. demonstrated through a systematic review and meta-analysis that the diagnostic accuracy is low [27]. Prior studies have shown that SAAs performed on CSF distinguish patients with manifest PD and DLB with a sensitivity of 90–95% and specificity of 80–100% from healthy controls [23, 28–39]. Shahnawaz et al. demonstrated that CSF-SAA is able to discriminate between PD and MSA with an overall sensitivity of 95.4% [40]. He and his colleagues included 94 PD patients, 75 MSA patients and 56 healthy controls and observed consistently different maximum fluorescence signals and kinetics of aggregation for PD and MSA. Samples from MSA patients revealed faster aggregation but reached lower fluorescence plateaus than those from PD patients [40]. Due to calculated typical SAA profiles the sensitivity for diagnosis of PD and MSA compared to healthy controls was 93.6% and 84.6% and specificity was 100% in both cases [40]. Similar but not identical findings were reported by Poggiolini et al [41]. His group also described lower fluorescence plateaus for MSA patients but slower aggregation. Whereas 89% of PD patients showed positive CSF-SAA results, only 75% of MSA patients analyzed had positive tests. Fairfoul et al. first reported positive CSF-SAA signals from patients with iRBD [23]. All three tested patients with iRBD had a positive SAA response. In 2021 Iranzo et al. examined 52 polysomnographic proven iRBD patients and 40 healthy controls. 90% of iRBD patients and 10% of healthy controls had a positive CSF-SAA [42]. Mean follow-up time of the lumbar puncture to the end of the study was 7.1–7.8 years. During this time period, 32 (62%) patients were diagnosed with PD or DLB and 31 (97%) of these had a positive CSF-SAA at baseline. The Kaplan-Meier analysis showed that patients with iRBD who were α-syn negative had a lower risk of developing PD or DLB after up to 10 years of follow-up than patients with iRBD who were α-syn positive (log-rank test p = 0·028; hazard ratio 0.143, 95% CI 0.019–1.063). During the observation period, none of the healthy controls developed an α-synucleinopathy. In 2023 Iranzo and colleagues recruited 91 consecutive patients with polysomnographic-proven iRBD and 41 healthy controls for skin biopsies and lumbar puncture performed at the same day to detect pathological α-syn [43]. Sensitivity to detect pathological α-syn by SAA was 76.9% in skin and 75.0% in CSF (specificity in skin: 97.6%, specificity in CSF: 97.5%). Comparing results of both SAA techniques, CSF and skin, the samples showed 99.2% agreement. Compared to iRBD patients with negative SAA, SAA-positive patients had a higher likelihood ratio for prodromal PD (p < 0.001), more frequently hyposmia (p < 0.001), dopamine transporter imaging single-photon emission CT deficit (p = 0.002), and orthostatic hypotension (p = 0.014). In the control cohort 2.5% showed positive SAA results. Similar findings were confirmed by Siderowf et al. [44]. His group described α-syn SAA results on over 1,100 participants in the Parkinson’s Progression Markers Initiative (PPMI) study. They analyzed 545 patients with PD, 163 healthy controls and 51 prodromal participants (18 individuals with hyposmia, 33 individuals with iRBD). Sensitivity and specificity for differentiating between PD and healthy controls were 88% and 96%. Sensitivity in sporadic PD with the typical olfactory deficit was 99%. The proportion of positive SAA was lower in subgroups including LRRK2 PD (68%) and sporadic PD patients without olfactory deficit (78%). Participants with LRRK2 variant and normal olfaction had an lower SAA positivity rate (35%). Among at-risk groups, 86% of iRBD (28/33) and hyposmic individuals (16/18) had positive SAAs. The diagnostic value of CSF-SAA in early-disease cases was validated by another large cohort CSF study (n=81) that showed 95% sensitivity in detecting patients with mild cognitive impairment (MCI) due to probable Lewy body disease [45]. Next to iRBD and MCI patients, individuals with pure autonomic failure (PAF) are also known to phenoconvert to one of the synucleinopathies [46, 47]. Singer and colleagues enrolled 32 patients with PAF and followed them for a median of 3.9 years [48]. During this observation period five patients converted to MSA, two to PD, and two to DLB [48]. Only CSF samples at baseline were utilized in their study [48]. The CSF-SAA was positive in all but two cases [48]. Of the positive cases, five strongly separated from the other samples with lower ThT fluorescence maximum [48]. These patients later developed MSA [48]. ThT fluorescence of all other SAA-positive samples was markedly higher [48]. Two of those patients developed PD, two DLB, and none developed MSA during follow-up [48].
Fig. 1
Schematic representation of α-syn SAAs from biofluids for prodromal α-synucleinopathies. Illustration was created using Smart Servier Medical Art (http://smart.servier.com/), which is licensed under CC BY 3.0.
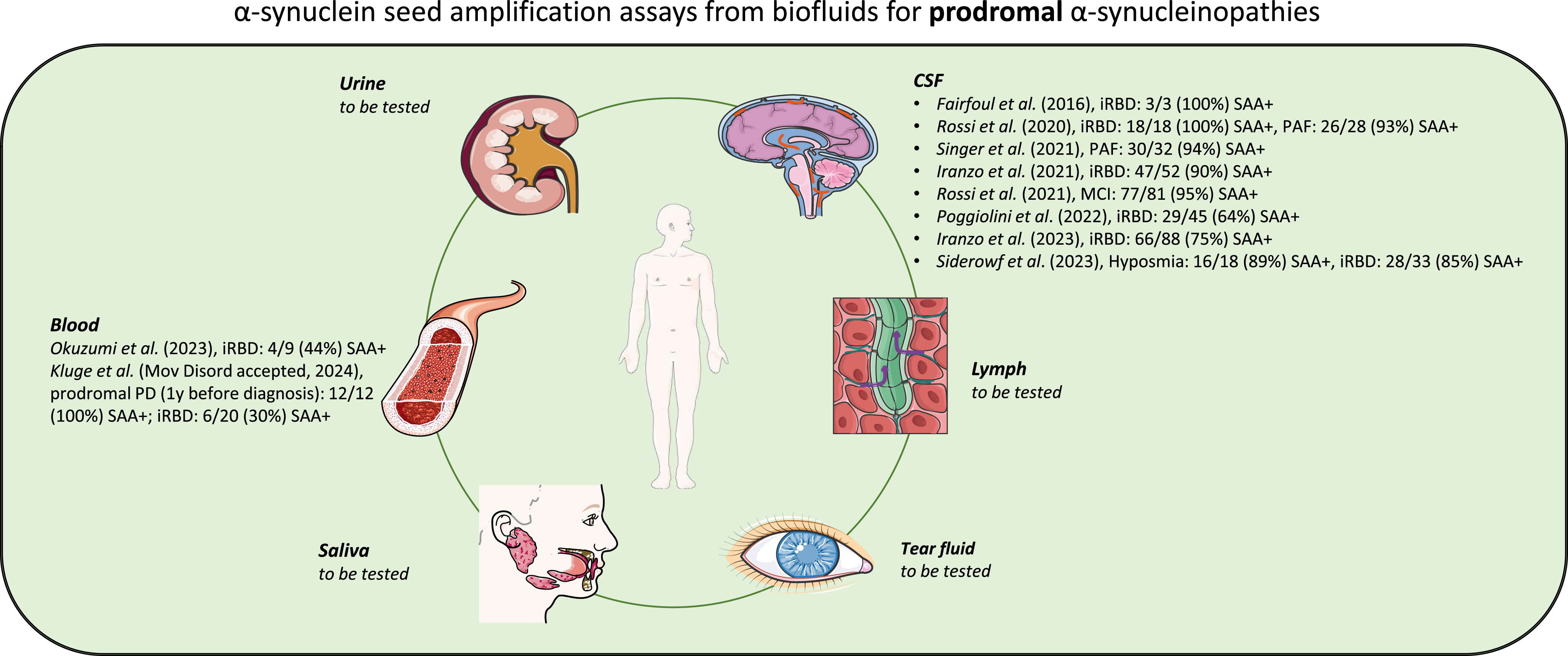
BLOOD-BASED DETECTION OF α-SYN AGGREGATION
Currently, there is no established or standardized blood-based test in clinical practice that can predict risk, can be used as diagnostic marker or distinguish patients with synucleinopathies from healthy controls. The blood concentration of α-syn is influenced by red blood cells, which represent the major source of peripheral α-syn [49]. For this reason, free, non-erythrocytic α-syn in synucleinopathy-patients and control individuals is limited and hemolysis significantly influences α-syn values [50, 51]. An alternative is the detection and measurement of α-syn released from neuronal cells in the form of neuron-derived extracellular vesicles (NEs). These vesicles are released by nearly all cell types and they carry selectively incorporated bioactive molecules [51]. The most important property of vesicles originating from the nervous system is that they can cross the blood– brain barrier and enter the bloodstream [52]. Shi et al. and Fiandaca et al. independently established 2014/2015 an immunoaffinity capturing protocol to isolate NCAM-L1-containing EVs from plasma [51, 53]. NCAM-L1 is known as cell adhesion molecule with high expression in neurons [51, 54]. Subsequently, several protocols were used to efficiently isolate these neuronal vesicles from blood plasma or serum. The majority of studies focused on investigating changes in α-syn protein levels in NEs. Several studies consistently reported a significant increase in total α-syn protein levels in PD-NEs compared to healthy controls [51, 55–60]. However, inconsistent α-syn concentrations were reported for MSA-NEs compared to PD-NEs. Dutta et al. detected significantly higher α-syn level in the MSA group compared to the PD group, whereas other studies observed lower concentrations of α-syn in MSA-NEs in comparison to PD-NEs [57, 60]. Only single studies have already focused on the detection of pathological α-syn conformers in blood plasma or serum. In 2021 Agliardi and her working group first measured the concentrations of oligomeric α-syn in NEs [61]. The concentration of oligomeric α-syn was significantly higher in PD-NEs (n = 32, mean: 0.573 ng/ml, SD = 0.154 ng/ml) compared to control-NEs (n = 40, mean: 0.389 ng/ml, SD = 0.148 ng/ml) (p < 0.001) using a sandwich enzyme-linked immunosorbent assay (ELISA) [61]. Similar findings were demonstrated by Zheng and his colleagues [62]. They measured the concentration of oligomeric and phosphorylated α-syn in blood plasma-derived NEs using immunoblots. The α-syn oligomer/total α-syn level in plasma exosomes of PD patients (n = 36) was significantly higher than the level in the healthy control group (n = 36, p = 0.0056). Next to concentration measurement studies of pathological α-syn conformers in blood, first groups demonstrated the possibility of SAA-based detection and amplification of α-syn seeds. Kluge et al. precipitated NEs from blood plasma and analyzed 50 healthy controls and 30 PD patients [25]. All PD patients tested showed a positive SAA, whereas none of the control subjects had a positive SAA-signal. Recently, Okuzumi and his colleagues developed a technique to combine SAA with immunoprecipitation to concentrate α-syn seeds from blood serum [24]. In contrast to Kluge and colleagues they targeted the free, not vesicle-associated α-syn seeds in serum. Positive SAA results were observed in 95% of patients with PD in their first cohort analyzed. In their second cohort 100% and in their third cohort 75% of PD patients had a positive SAA. Furthermore, 39 MSA patients and 10 patients with DLB were included. 65.4% of patients with MSA parkinsonian variant (MSA-P) and 61.5% of patients with MSA cerebellar variant (MSA-C) were IP/SAA positive, whereas 90% of all DLB individuals tested showed positive findings. 8.5% of healthy control and 16% of patients with Alzheimer’s disease were tested positive. In addition, 9 iRBD patients were recruited. In total 4 out of 9 samples had positive SAA signals [24]. Okuzumi also investigated serum of seven patients with pathologically confirmed synucleinopathies (four PD patients with dementia, three individuals with MSA), two pathologically verified patients with tauopathies and three age-matched control individuals. All PD patients tested had a positive SAA, one out of three MSA patients were SAA-positive and none of non-synucleinopathy patients and healthy controls had positive results. Additionally, Okuzumi et al. analyzed morphological characteristics of fibrils amplified by SAA from the serum seeds of patients with LB disease, MSA or iRBD [24]. Subsequent transmission electron microscopy (TEM) analysis of SAA end-products revealed paired or bundled multiple filaments of PD and DLB seeds, whereas, in MSA seeds twisted filaments and straight filaments were observed. Interestingly, all amplified fibrils derived from patients with iRBD with positive SAA results had the Lewy body diseases-type structure.
DETECTION OF α-SYN AGGREGATION IN OTHER BIOFLUIDS
Compared to CSF and blood saliva is easier to collect. Interestingly, several studies revealed that the α-syn oligomer/total α-syn ratio was significantly higher in PD patients compared to healthy controls [63, 64]. More recently, the working group of Luan et al. has shown positive α-syn SAA in saliva of patients with PD or MSA. Salivary SAA distinguished PD patients with 76.0% and MSA patients with 61.1% sensitivity [65]. Similar findings were observed by Vivacqua et al., 31 out of 37 PD salivary samples were SAA positive [66]. However, 22% of the control cohort also showed seeding activity. To date, no studies have focused on the detection of pathological α-syn in saliva in the prodromal phase of synucleinopathies. Another biofluid, that can be collected in a noninvasive way is the tear fluid. Hamm-Alvarez and her working group observed significant increases in oligomeric α-syn and in the oligomeric α-syn/total α-syn ratio in PD patients tears compared to healthy control tears [67, 68]. They showed that this discrimination was stronger in reflex tears than in basal tears [67]. So far, no tears from at-risk patients have been examined. Due to its convenient sampling process and the sufficiency of collected volumes is urine an excellent biofluid as source for potential biomarker [69]. In 2020 Nam et al. described significant higher oligomeric α-syn level in PD patients urine compared to healthy control urine samples [70]. Data on the use of lymph to analyze pathological α-syn are not yet available.
Details about SAA positive (+) results in cohorts tested in each included study are given in Table 1.
Table 1
Diagnostic SAA-results and medium used for all included studies
Study (first author, year) | Used | #PD +SAA | #DLB +SAA | #MSA +SAA | #at-risk individuals | #control |
medium | results | results | results | +SAA results | +SAA results | |
Shahnawaz et al., 2017 [21] | CSF | 67/76 | 4/65 | |||
Groveman et al., 2018 [28] | CSF | 11/12 | 16/17 | 1/12 | ||
Manne et al., 2019 [30] | CSF | 15/15 | 11/11 | |||
Bongianni et al., 2019 [31] | CSF | 7/7 | 1/1 | |||
Garrido et al., 2019 [32] | CSF | 9/10 | 2/10 | |||
Van Rumund et al., 2019 [33] | CSF | 43/53 | 1/1 | 6/17 | 1/52 | |
Rossi et al., 2020 [34] | CSF | 67/71 | 33/34 | 2/31 | iRBD: 18/18 PAF: 26/28 | 1/62 |
Brockmann et al., 2021 [37] | CSF | 200/235 | 42/49 | 2/26 | ||
Orrù et al., 2021 [38] | CSF | 105/108 | 11/85 | |||
Bargar et al., 2021 [39] | CSF | 86/88 | 57/58 | 0/23 | ||
Shahnawaz et al., 2020 [40] | CSF | 88/94 | 64/75 | 0/56 | ||
Poggiolini et al., 2022 [41] | CSF | 66/74 | 18/24 | iRBD: 29/45 | 2/55 | |
Fairfoul et al., 2016 [23] | CSF | 2/2 | 11/12 | iRBD: 3/3 | 0/20 | |
Iranzo et al., 2021 [42] | CSF | iRBD: 47/52 | 4/40 | |||
Iranzo et al., 2023 [43] | CSF | iRBD: 66/88 | 1/40 | |||
Siderowf et al., 2023 [44] | CSF | 478/545 | Hyposmia: 16/18 iRBD: 28/33 | 6/157 | ||
Rossi et al., 2021 [45] | CSF | MCI: 77/81 | 2/58 | |||
Singer et al., 2021 [48] | CSF | 16/16 | 11/13 | 60/62 | PAF: 30/32 | 0/29 |
Kluge et al., 2022 [25] | plasma-derived | 30/30 | 0/50 | |||
NEs | ||||||
Okuzumi et al. 2023 [24] | serum | 210/221 | 9/10 | 25/39 | iRBD: 4/9 | 11/128 |
CONCLUSIONS AND PERSPECTIVES
Since we know that misfolding and aggregation of α-syn precede clinical manifestations, the identification of these phenomena at the earliest, premotor phases would enable the transition from a clinical to a molecular diagnosis. In this review, we summarized the research progress in detecting α-syn seeds in biofluids, especially using SAAs, in CSF and blood in prodromal patients. SAAs exploit the self-propagation activity of disease-associated α-syn conformers to detect them with unprecedented diagnostic sensitivity and specificity for synucleinopathies. Thus, early prodromal synucleinopathy stages such as MCI or iRBD represent optimal cohorts to verify the potential of SAA techniques. To date, few blood-based SAA protocols have been described and only one study reported about SAA-findings in at-risk patients. Several studies have demonstrated the high sensitivity and specificity for CSF-SAA in detecting α-syn aggregations. Nevertheless, lumbar punctures are even more invasive than blood collection and therefore associated with more complications. Furthermore, several different protocols exist for blood-SAA as well as for CSF-SAA. Therefore, future studies should focus on more consistent, precise, and linearly responsive SAA methods. Additionally, larger cohorts of patients at-risk should be overserved longitudinally to understand seeding profiles/activities and to verify the time period from the onset of detectable seeding to the time of clinical conversion in specific prodromal cohorts (iRBD patients, patients with hyposmia, MCI individuals etc.). This might improve diagnostic and prognostic values of CSF- and blood-based SAAs and enables earliest diagnosis and therapy.
ACKNOWLEDGMENTS
The authors have no acknowledgments to report.
FUNDING
The authors have no funding to report.
CONFLICT OF INTEREST
The authors have no conflict of interest to report.
DATA AVAILABILITY
Data are available on request.
REFERENCES
[1] | Goedert M , Jakes R , Spillantini MG ((2017) ) The synucleinopathies: Twenty years on. J Parkinsons Dis 7: , S51–S69. |
[2] | Kalia LV , Lang AE ((2015) ) Parkinson’s disease. Lancet 386: , 896–912. |
[3] | Martí MJ , Tolosa E , Campdelacreu J ((2003) ) Clinical overview of the synucleinopathies. Mov Disord 18 Suppl 6: , S21–27. |
[4] | Mahlknecht P , Seppi K , Poewe W ((2015) ) The concept of prodromal Parkinson’s disease. J Parkinsons Dis 5: , 681–697. |
[5] | Berg D , Borghammer P , Fereshtehnejad SM , Heinzel S , Horsager J , Schaeffer E , Postuma RB ((2021) ) Prodromal Parkinson disease subtypes - key to understanding heterogeneity. Nat Rev Neurol 17: , 349–361. |
[6] | Blauwendraat C , Nalls MA , Singleton AB ((2020) ) The genetic architecture of Parkinson’s disease. Lancet Neurol 19: , 170–178. |
[7] | ((2019) ) Global, regional, and national burden of neurological disorders, 1990-2016: A systematic analysis for the Global Burden of Disease Study 2016. Lancet Neurol 18: , 459–480. |
[8] | Simuni T , Chahine LM , Poston K , Brumm M , Buracchio T , Campbell M , Chowdhury S , Coffey C , Concha-Marambio L , Dam T , DiBiaso P , Foroud T , Frasier M , Gochanour C , Jennings D , Kieburtz K , Kopil CM , Merchant K , Mollenhauer B , Montine T , Nudelman K , Pagano G , Seibyl J , Sherer T , Singleton A , Stephenson D , Stern M , Soto C , Tanner CM , Tolosa E , Weintraub D , Xiao Y , Siderowf A , Dunn B , Marek K ((2024) ) A biological definition of neuronal α-synuclein disease: Towards an integrated staging system for research. Lancet Neurol 23: , 178–190. |
[9] | Tolosa E , Garrido A , Scholz SW , Poewe W ((2021) ) Challenges in the diagnosis of Parkinson’s disease. Lancet Neurol 20: , 385–397. |
[10] | Heinzel S , Berg D , Gasser T , Chen H , Yao C , Postuma RB ((2019) ) Update of the MDS research criteria for prodromal Parkinson’s disease. Mov Disord 34: , 1464–1470. |
[11] | Schrag A , Horsfall L , Walters K , Noyce A , Petersen I ((2015) ) Prediagnostic presentations of Parkinson’s disease in primary care: A case-control study. Lancet Neurol 14: , 57–64. |
[12] | Fereshtehnejad SM , Yao C , Pelletier A , Montplaisir JY , Gagnon JF , Postuma RB ((2019) ) Evolution of prodromal Parkinson’s disease and dementia with Lewy bodies: A prospective study. Brain 142: , 2051–2067. |
[13] | Gustafsson H , Nordström A , Nordström P ((2015) ) Depression and subsequent risk of Parkinson disease: A nationwide cohort study. Neurology 84: , 2422–2429. |
[14] | Savica R , Carlin JM , Grossardt BR , Bower JH , Ahlskog JE , Maraganore DM , Bharucha AE , Rocca WA ((2009) ) Medical records documentation of constipation preceding Parkinson disease: A case-control study. Neurology 73: , 1752–1758. |
[15] | Wenning GK , Stankovic I , Vignatelli L , Fanciulli A , Calandra-Buonaura G , Seppi K , Palma JA , Meissner WG , Krismer F , Berg D , Cortelli P , Freeman R , Halliday G , Höglinger G , Lang A , Ling H , Litvan I , Low P , Miki Y , Panicker J , Pellecchia MT , Quinn N , Sakakibara R , Stamelou M , Tolosa E , Tsuji S , Warner T , Poewe W , Kaufmann H ((2022) ) The Movement Disorder Society criteria for the diagnosis of multiple system atrophy. Mov Disord 37: , 1131–1148. |
[16] | Yamada M , Komatsu J , Nakamura K , Sakai K , Samuraki-Yokohama M , Nakajima K , Yoshita M ((2020) ) Diagnostic criteria for dementia with Lewy bodies: Updates and future directions. J Mov Disord 13: , 1–10. |
[17] | Braak H , Del Tredici K , Rüb U , de Vos RA , Jansen Steur EN , Braak E ((2003) ) Staging of brain pathology related to sporadic Parkinson’s disease. Neurobiol Aging 24: , 197–211. |
[18] | Spillantini MG , Schmidt ML , Lee VM , Trojanowski JQ , Jakes R , Goedert M ((1997) ) Alpha-synuclein in Lewy bodies. Nature 388: , 839–840. |
[19] | Aulić S , Le TT , Moda F , Abounit S , Corvaglia S , Casalis L , Gustincich S , Zurzolo C , Tagliavini F , Legname G ((2014) ) Definedα-synuclein prion-like molecular assemblies spreading in cell culture. BMC Neurosci 15: , 69. |
[20] | Tarutani A , Arai T , Murayama S , Hisanaga SI , Hasegawa M ((2018) ) Potent prion-like behaviors of pathogenic α-synuclein and evaluation of inactivation methods. Acta Neuropathol Commun 6: , 29. |
[21] | Shahnawaz M , Tokuda T , Waragai M , Mendez N , Ishii R , Trenkwalder C , Mollenhauer B , Soto C ((2017) ) Development of a biochemical diagnosisof Parkinson disease by detection of α-synuclein misfolded aggregates in cerebrospinal fluid. JAMA Neurol 74: , 163–172. |
[22] | Srivastava A , Alam P , Caughey B ((2022) ) RT-QuIC and related assays for detecting and quantifying prion-like pathological seeds ofα-synuclein. Biomolecules 12: , 576. |
[23] | Fairfoul G , McGuire LI , Pal S , Ironside JW , Neumann J , Christie S , Joachim C , Esiri M , Evetts SG , Rolinski M , Baig F , Ruffmann C , Wade-Martins R , Hu MT , Parkkinen L , Green AJ ((2016) ) Alpha-synuclein RT-QuIC in the CSF of patients with alpha-synucleinopathies. Ann Clin Transl Neurol 3: , 812–818. |
[24] | Okuzumi A , Hatano T , Matsumoto G , Nojiri S , Ueno SI , Imamichi-Tatano Y , Kimura H , Kakuta S , Kondo A , Fukuhara T , Li Y , Funayama M , Saiki S , Taniguchi D , Tsunemi T , McIntyre D , Gérardy JJ , Mittelbronn M , Kruger R , Uchiyama Y , Nukina N , Hattori N ((2023) ) Propagativeα-synuclein seeds as serum biomarkers for synucleinopathies. Nat Med 29: , 1448–1455. |
[25] | Kluge A , Bunk J , Schaeffer E , Drobny A , Xiang W , Knacke H , Bub S , Lückstädt W , Arnold P , Lucius R , Berg D , Zunke F ((2022) ) Detection of neuron-derived pathological α-synuclein in blood. Brain 145: , 3058–3071. |
[26] | Mondello S , Constantinescu R , Zetterberg H , Andreasson U , Holmberg B , Jeromin A ((2014) ) CSF α-synuclein and UCH-L1 levels in Parkinson’s disease and atypical parkinsonian disorders. Parkinsonism Relat Disord 20: , 382–387. |
[27] | Eusebi P , Giannandrea D , Biscetti L , Abraha I , Chiasserini D , Orso M , Calabresi P , Parnetti L ((2017) ) Diagnostic utility of cerebrospinal fluid α-synuclein in Parkinson’s disease: A systematic review and meta-analysis. Mov Disord 32: , 1389–1400. |
[28] | Groveman BR , Orrù CD , Hughson AG , Raymond LD , Zanusso G , Ghetti B , Campbell KJ , Safar J , Galasko D , Caughey B ((2018) ) Rapid and ultra-sensitive quantitation of disease-associatedα-synuclein seeds in brain and cerebrospinal fluid by αSyn RT-QuIC. Acta Neuropathol Commun 6: , 7. |
[29] | Ma J , Gao J , Wang J , Xie A ((2019) ) Prion-like mechanisms in Parkinson’s disease. Front Neurosci 13: , 552. |
[30] | Manne S , Kondru N , Hepker M , Jin H , Anantharam V , Lewis M , Huang X , Kanthasamy A , Kanthasamy AG ((2019) ) Ultrasensitive detection of aggregated α-synuclein in glial cells, human cerebrospinal fluid, and brain tissue using the RT-QuIC assay: New high-throughput neuroimmune biomarker assay for parkinsonian disorders. J Neuroimmune Pharmacol 14: , 423–435. |
[31] | Bongianni M , Ladogana A , Capaldi S , Klotz S , Baiardi S , Cagnin A , Perra D , Fiorini M , Poleggi A , Legname G , Cattaruzza T , Janes F , Tabaton M , Ghetti B , Monaco S , Kovacs GG , Parchi P , Pocchiari M , Zanusso G ((2019) ) α-Synuclein RT-QuIC assay in cerebrospinal fluid of patients with dementia with Lewy bodies. Ann ClinTransl Neurol 6: , 2120–2126. |
[32] | Garrido A , Fairfoul G , Tolosa ES , Martí MJ , Green A ((2019) ) α-synuclein RT-QuIC in cerebrospinal fluid of LRRK2-linked Parkinson’s disease. Ann Clin Transl Neurol 6: , 1024–1032. |
[33] | van Rumund A , Green AJE , Fairfoul G , Esselink RAJ , Bloem BR , Verbeek MM ((2019) ) α-Synuclein real-time quaking-induced conversion in the cerebrospinal fluid of uncertain cases of parkinsonism. Ann Neurol 85: , 777–781. |
[34] | Rossi M , Candelise N , Baiardi S , Capellari S , Giannini G , Orrù CD , Antelmi E , Mammana A , Hughson AG , Calandra-Buonaura G , Ladogana A , Plazzi G , Cortelli P , Caughey B , Parchi P ((2020) ) Ultrasensitive RT-QuIC assay with high sensitivity and specificity for Lewy body-associated synucleinopathies. Acta Neuropathol 140: , 49–62. |
[35] | Candelise N , Schmitz M , Thüne K , Cramm M , Rabano A , Zafar S , Stoops E , Vanderstichele H , Villar-Pique A , Llorens F , Zerr I ((2020) ) Effect of the micro-environment on α-synuclein conversion and implication in seeded conversion assays. Transl Neurodegener 9: , 5. |
[36] | Russo MJ , Orru CD , Concha-Marambio L , Giaisi S , Groveman BR , Farris CM , Holguin B , Hughson AG , LaFontant DE , Caspell-Garcia C , Coffey CS , Mollon J , Hutten SJ , Merchant K , Heym RG , Soto C , Caughey B , Kang UJ ((2021) ) High diagnostic performance of independent alpha-synuclein seed amplification assays for detection of early Parkinson’s disease. Acta Neuropathol Commun 9: , 179. |
[37] | Brockmann K , Quadalti C , Lerche S , Rossi M , Wurster I , Baiardi S , Roeben B , Mammana A , Zimmermann M , Hauser AK , Deuschle C , Schulte C , Waniek K , Lachmann I , Sjödin S , Brinkmalm A , Blennow K , Zetterberg H , Gasser T , Parchi P ((2021) ) Association between CSF alpha-synuclein seeding activity and genetic status in Parkinson’s disease and dementia with Lewy bodies. Acta Neuropathol Commun 9: , 175. |
[38] | Orrù CD , Ma TC , Hughson AG , Groveman BR , Srivastava A , Galasko D , Angers R , Downey P , Crawford K , Hutten SJ , Kang UJ , Caughey B ((2021) ) A rapid α-synuclein seed assay of Parkinson’s disease CSF panel shows high diagnostic accuracy. Ann ClinTransl Neurol 8: , 374–384. |
[39] | Bargar C , Wang W , Gunzler SA , LeFevre A , Wang Z , Lerner AJ , Singh N , Tatsuoka C , Appleby B , Zhu X , Xu R , Haroutunian V , Zou WQ , Ma J , Chen SG ((2021) ) Streamlined alpha-synuclein RT-QuIC assay for various biospecimens in Parkinson’s disease and dementia with Lewy bodies. Acta Neuropathol Commun 9: , 62. |
[40] | Shahnawaz M , Mukherjee A , Pritzkow S , Mendez N , Rabadia P , Liu X , Hu B , Schmeichel A , Singer W , Wu G , Tsai AL , Shirani H , Nilsson KPR , Low PA , Soto C ((2020) ) Discriminating α-synuclein strains in Parkinson’s disease and multiple system atrophy. Nature 578: , 273–277. |
[41] | Poggiolini I , Gupta V , Lawton M , Lee S , El-Turabi A , Querejeta-Coma A , Trenkwalder C , Sixel-Döring F , Foubert-Samier A , Pavy-LeTraon A , Plazzi G , Biscarini F , Montplaisir J , Gagnon JF , Postuma RB , Antelmi E , Meissner WG , Mollenhauer B , Ben-Shlomo Y , Hu MT , Parkkinen L ((2022) ) Diagnostic value of cerebrospinal fluid alpha-synuclein seed quantification in synucleinopathies. Brain 145: , 584–595. |
[42] | Iranzo A , Fairfoul G , Ayudhaya ACN , Serradell M , Gelpi E , Vilaseca I , Sanchez-Valle R , Gaig C , Santamaria J , Tolosa E , Riha RL , Green AJE ((2021) ) Detection of α-synuclein in CSF by RT-QuIC in patients with isolated rapid-eye-movement sleep behaviour disorder: A longitudinal observational study. Lancet Neurol 20: , 203–212. |
[43] | Iranzo A , Mammana A , Muñoz-Lopetegi A , Dellavalle S , Mayà G , Rossi M , Serradell M , Baiardi S , Arqueros A , Quadalti C , Perissinotti A , Ruggeri E , Cano JS , Gaig C , Parchi P ((2023) ) Misfolded α-synuclein assessment in the skin and CSF by RT-QuIC in isolated REM sleep behavior disorder. Neurology 100: , e1944–e1954. |
[44] | Siderowf A , Concha-Marambio L , Lafontant D-E , Farris CM , Ma Y , Urenia PA , Nguyen H , Alcalay RN , Chahine LM , Foroud T , Galasko D , Kieburtz K , Merchant K , Mollenhauer B , Poston KL , Seibyl J , Simuni T , Tanner CM , Weintraub D , Videnovic A , Choi SH , Kurth R , Caspell-Garcia C , Coffey CS , Frasier M , Oliveira LMA , Hutten SJ , Sherer T , Marek K , Soto C ((2023) ) Assessment of heterogeneity among participants in the Parkinson’s Progression Markers Initiative cohort using α-synuclein seed amplification: A cross-sectional study. Lancet Neurol 22: , 407–417. |
[45] | Rossi M , Baiardi S , Teunissen CE , Quadalti C , van de Beek M , Mammana A , Stanzani-Maserati M , Van der Flier WM , Sambati L , Zenesini C , Caughey B , Capellari S , Lemstra AW , Parchi P ((2021) ) Diagnostic value of the CSF α-synuclein real-time quaking-induced conversion assay at the prodromal MCI Stage of dementia with Lewy bodies. Neurology 97: , e930–e940. |
[46] | Kaufmann H , Norcliffe-Kaufmann L , Palma JA , Biaggioni I , Low PA , Singer W , Goldstein DS , Peltier AC , Shibao CA , Gibbons CH , Freeman R , Robertson D ((2017) ) Natural history of pure autonomic failure: A United States prospective cohort. Ann Neurol 81: , 287–297. |
[47] | Coon EA , Mandrekar JN , Berini SE , Benarroch EE , Sandroni P , Low PA , Singer W ((2020) ) Predicting phenoconversion in pure autonomic failure. Neurology 95: , e889–e897. |
[48] | Singer W , Schmeichel AM , Shahnawaz M , Schmelzer JD , Sletten DM , Gehrking TL , Gehrking JA , Olson AD , Suarez MD , Misra PP , Soto C , Low PA ((2021) ) Alpha-synuclein oligomers and neurofilament light chain predict phenoconversion of pure autonomic failure. Ann Neurol 89: , 1212–1220. |
[49] | Barbour R , Kling K , Anderson JP , Banducci K , Cole T , Diep L , Fox M , Goldstein JM , Soriano F , Seubert P , Chilcote TJ ((2008) ) Red blood cells are the major source of alpha-synuclein in blood. Neurodegener Dis 5: , 55–59. |
[50] | Tian C , Liu G , Gao L , Soltys D , Pan C , Stewart T , Shi M , Xie Z , Liu N , Feng T , Zhang J ((2019) ) Erythrocytic α-synuclein as a potential biomarker for Parkinson’s disease. Transl Neurodegener 8: , 15. |
[51] | Shi M , Liu C , Cook TJ , Bullock KM , Zhao Y , Ginghina C , Li Y , Aro P , Dator R , He C , Hipp MJ , Zabetian CP , Peskind ER , Hu SC , Quinn JF , Galasko DR , Banks WA , Zhang J ((2014) ) Plasma exosomalα-synuclein is likely CNS-derived and increased in Parkinson’s disease. Acta Neuropathol 128: , 639–650. |
[52] | Goetzl EJ , Mustapic M , Kapogiannis D , Eitan E , Lobach IV , Goetzl L , Schwartz JB , Miller BL ((2016) ) Cargo proteins of plasma astrocyte-derived exosomes in Alzheimer’s disease. FASEB J 30: , 3853–3859. |
[53] | Fiandaca MS , Kapogiannis D , Mapstone M , Boxer A , Eitan E , Schwartz JB , Abner EL , Petersen RC , Federoff HJ , Miller BL , Goetzl EJ ((2015) ) Identification of preclinical Alzheimer’s disease by a profile of pathogenic proteins in neurally derived blood exosomes: A case-control study. Alzheimers Dement 11: , 600–607.e601. |
[54] | Fauré J , Lachenal G , Court M , Hirrlinger J , Chatellard-Causse C , Blot B , Grange J , Schoehn G , Goldberg Y , Boyer V , Kirchhoff F , Raposo G , Garin J , Sadoul R ((2006) ) Exosomes are released by cultured cortical neurones. Mol Cell Neurosci 31: , 642–648. |
[55] | Zhao ZH , Chen ZT , Zhou RL , Zhang X , Ye QY , Wang YZ ((2018) ) Increased DJ-1 and α-synuclein in plasma neural-derived exosomes as potential markers for Parkinson’s disease. Front Aging Neurosci 10: , 438. |
[56] | Fu Y , Jiang C , Tofaris GK , Davis JJ ((2020) ) Facile impedimetric analysis of neuronal exosome markers in Parkinson’s disease diagnostics. Anal Chem 92: , 13647–13651. |
[57] | Jiang C , Hopfner F , Katsikoudi A , Hein R , Catli C , Evetts S , Huang Y , Wang H , Ryder JW , Kuhlenbaeumer G , Deuschl G , Padovani A , Berg D , Borroni B , Hu MT , Davis JJ , Tofaris GK ((2020) ) Serum neuronal exosomes predict and differentiate Parkinson’s disease from atypical parkinsonism. J Neurol Neurosurg Psychiatry 91: , 720–729. |
[58] | Niu M , Li Y , Li G , Zhou L , Luo N , Yao M , Kang W , Liu J ((2020) ) A longitudinal study on α-synuclein in plasma neuronal exosomes as a biomarker for Parkinson’s disease development and progression. Eur J Neurol 27: , 967–974. |
[59] | Zou J , Guo Y , Wei L , Yu F , Yu B , Xu A ((2020) ) Long noncoding RNA POU3F3 and α-synuclein in plasma L1CAM exosomes combined with β-glucocerebrosidase activity: Potential predictors of Parkinson’s disease. Neurotherapeutics 17: , 1104–1119. |
[60] | Dutta S , Hornung S , Kruayatidee A , Maina KN , Del Rosario I , Paul KC , Wong DY , Duarte Folle A , Markovic D , Palma JA , Serrano GE , Adler CH , Perlman SL , Poon WW , Kang UJ , Alcalay RN , Sklerov M , Gylys KH , Kaufmann H , Fogel BL , Bronstein JM , Ritz B , Bitan G ((2021) ) α-Synuclein in blood exosomes immunoprecipitated using neuronal and oligodendroglial markers distinguishes Parkinson’s disease from multiple system atrophy. Acta Neuropathol 142: , 495–511. |
[61] | Agliardi C , Meloni M , Guerini FR , Zanzottera M , Bolognesi E , Baglio F , Clerici M ((2021) ) Oligomeric α-Syn and SNARE complex proteins in peripheral extracellular vesicles of neural origin are biomarkers for Parkinson’s disease. Neurobiol Dis 148: , 105185. |
[62] | Zheng H , Xie Z , Zhang X , Mao J , Wang M , Wei S , Fu Y , Zheng H , He Y , Chen H , Xu Y ((2021) ) Investigation of α-synuclein species in plasma exosomes and the oligomeric and phosphorylatedα-synuclein as potential peripheral biomarker of Parkinson’s disease. Neuroscience 469: , 79–90. |
[63] | Vivacqua G , Latorre A , Suppa A , Nardi M , Pietracupa S , Mancinelli R , Fabbrini G , Colosimo C , Gaudio E , Berardelli A ((2016) ) Abnormal salivary total and oligomeric alpha-synuclein in Parkinson’s disease. PLoS One 11: , e0151156. |
[64] | Kharel S , Ojha R , Bist A , Joshi SP , Rauniyar R , Yadav JK ((2022) ) Salivary alpha-synuclein as a potential fluid biomarker in Parkinson’s disease: A systematic review and meta-analysis. Aging Med (Milton) 5: , 53–62. |
[65] | Luan M , Sun Y , Chen J , Jiang Y , Li F , Wei L , Sun W , Ma J , Song L , Liu J , Liu B , Pei Y , Wang Z , Zhu L , Deng J ((2022) ) Diagnostic value of salivary real-time quaking-induced conversion in Parkinson’s disease and multiple system atrophy. Mov Disord 37: , 1059–1063. |
[66] | Vivacqua G , Mason M , De Bartolo MI , Węgrzynowicz M , Calò L , Belvisi D , Suppa A , Fabbrini G , Berardelli A , Spillantini M ((2023) ) Salivary α-synuclein RT-QuIC correlates with disease severity in de novo Parkinson’s disease. Mov Disord 38: , 153–155. |
[67] | Hamm-Alvarez SF , Janga SR , Edman MC , Feigenbaum D , Freire D , Mack WJ , Okamoto CT , Lew MF ((2019) ) Levels of oligomericα-synuclein in reflex tears distinguish Parkinson’s disease patients from healthy controls. Biomark Med 13: , 1447–1457. |
[68] | Hamm-Alvarez SF , Okamoto CT , Janga SR , Feigenbaum D , Edman MC , Freire D , Shah M , Ghanshani R , Mack WJ , Lew MF ((2019) ) Oligomericα-synuclein is increased in basal tears of Parkinson’s patients. Biomark Med 13: , 941–952. |
[69] | Jing J , Gao Y ((2018) ) Urine biomarkers in the early stages of diseases: Current status and perspective. Discov Med 25: , 57–65. |
[70] | Nam D , Lee JY , Lee M , Kim J , Seol W , Son I , Ho DH ((2020) ) Detection and assessment of α-synuclein oligomers in the urine of Parkinson’s disease patients. J Parkinsons Dis 10: , 981–991. |