Respiratory-Swallow Coordination in Individuals with Parkinson’s Disease: A Systematic Review and Meta-Analysis
Abstract
Background:
Swallowing impairment, including altered physiology and aspiration, occur across the progression of Parkinson’s disease (PD). The phase of respiration during which a swallow is initiated has been linked to swallowing impairment and aspiration in cohorts with dysphagia following stroke and head and neck cancer treatment, but has been understudied in PD. If similar findings are shown in individuals with PD, the implications for swallowing assessment and treatment are significant.
Objective:
The aim of this systematic review and meta-analysis of literature was to examine respiratory-swallow coordination measures and potential implications on swallowing physiology in individuals with PD.
Methods:
An extensive search of 7 databases (PubMed, EMBASE, Central, Web of Science, ProQuest Dissertations & Theses, Scopus, and CINAHL) with predetermined search terms was conducted. Inclusion criteria were individuals with PD and the use of objective evaluations of respiratory-swallow coordination.
Results:
Of the 13,760 articles identified, 11 met the inclusion criteria. This review supports the presence of atypical respiratory swallow patterning, respiratory pause duration and lung volume at swallow initiation in individuals with PD. The meta-analysis estimated an occurrence of 60% of non-expiration-expiration and 40% of expiration-expiration respiratory phase patterns surrounding swallowing.
Conclusion:
Although this systematic review supports the occurrence of atypical respiratory-swallow coordination in individuals with PD, the evidence is limited by the variability in the methods of data acquisition, analysis, and reporting. Future research examining the impact of respiratory swallow coordination on swallowing impairment and airway protection using consistent, comparable, and reproducible methods and metrics in individuals with PD is warranted.
INTRODUCTION
Parkinson’s disease (PD) is one of the most common neurodegenerative disorders in the world [1, 2], and it is prevalent in approximately 1 million individuals in the United States [3]. Although the cause of PD is unknown, its pathological hallmark sign is the loss of dopamine producing or dopaminergic neurons in the substantia nigra pars compacta of the basal ganglia [4, 5]. Dopamine deficiency and the resultant inhibition of neural signals in the basal ganglia circuity [6] lead to insufficiency of movement in PD [7, 8], characterized by classic motor symptoms including rest tremors, bradykinesia, muscular rigidity, and postural instability [9]. Theoretical and empirical research supports that the neuropathology of PD disrupts not only motor control, but also has a negative impact on swallowing physiology [10–14] and respiratory systems [15–25], superimposed by the natural process of aging in this population [26]. Swallowing and respiratory impairments in individuals with PD are known to be “silent threats,” progressing undetected until the clinical complaints are severe in advanced stages of the disease [27], resulting in respiratory compromise, aspiration pneumonia, or both.
Dysphagia is a highly prevalent manifestation of PD [10, 11], characterized by altered swallow physiology and aspiration [12–14]. It is a primary factor contributing to aspiration pneumonia, the leading cause of hospitalization and death in individuals with PD [28–31]. The dopaminergic basal ganglia system, primarily impaired in PD, plays an important role in the neural control of swallowing. This occurs at the level of the putamen and globus pallidus, which are regions in the basal ganglia responsible for initiating motor activity and modulating swallowing [14, 32]. Deposition of pathologies of PD such as alpha-synuclein and Lewy bodies in this anatomical area may lead to medullary swallowing and respiratory center dysfunction [14, 33–35]. Evidence suggests that these anatomical changes give rise to physiological impairments in swallowing characterized by repetitive pumping lingual movements, piecemeal deglutition, somatosensory deficits, reduced rate of spontaneous swallows, spasms, hypomotility, etc. [14].
PD is frequently associated with respiratory impairments [15–25]. Parasympathetic hyperactivity, changes in the central ventilatory control, and occurrence of restrictive and obstructive pulmonary patterns are some mechanisms which may result in respiratory dysfunction [15, 36, 37]. These deficits can lead to abnormalities including reduced chest wall expansion, upper airway muscular dysfunction, reduced lung volume, and decreased respiratory muscular and coordination deficits, which are characteristic signs of PD [16, 36–38].
Swallowing and respiration are complex physiological events that share a tightly coordinated relationship [26, 39–41]. In healthy adults, swallowing occurs during a pause in the expiratory phase of quiet breathing with brief expiratory airflow following the offset of swallowing [26, 39–74]. This typical pattern has been described as “optimal” because of the beneficial impact on airway protection and bolus clearance [26, 39, 40, 60, 75–77]. However, this typical coordination can be negatively impacted in several disorders such as stroke and head and neck cancer, resulting in reduced swallowing safety and efficiency [78–80]. Atypical patterns differing from an expiration-swallow-expiration (EX-EX) pattern have potential biomechanical disadvantages, especially for airway protection. Research regarding typical lung volume at swallow initiation in healthy adults is mixed, influenced by data analysis methods, bolus volume and consistencies [74, 81]. While some research reports that swallowing initiation occurs at the mid to low lung volume relative to resting expiratory level [41, 74], other research has found that swallowing initiation may occur at mid or higher lung volumes [72, 73].
Dysphagia and respiratory dysfunction have been studied independently in individuals with PD, but limited research explores their coordination. Studies targeting respiratory swallow coordination (RSC) in individuals in PD used inconsistent methods for data acquisition, analysis, and reporting of results. Review studies focusing on the impact of PD on RSC are unavailable, despite the high prevalence of motor control deficits, abnormal respiratory patterns, and dysphagia in individuals with PD [82–100]. As such, the aim of this systematic review and meta-analysis of the literature was to examine RSC measures and potential implications on swallowing physiology in individuals with PD. Studies included in this systematic review contained small sample sizes, variable results, and limited data points. A meta-analysis was completed to ensure effective synthesis of the results, accounting for effect sizes and limited number of included articles. Our long-term goal is to enhance evidence-based dysphagia rehabilitation and improve quality of life of these patients.
METHODS
Protocol
PROSPERO, an international prospective register of systematic reviews, was examined to ensure a similar review does not exist. This review was registered with PROSPERO on May 15, 2021, to avoid duplication and limit reporting bias. The research question, rationale, and selection criteria were determined a-priori. The study selection process utilized the Preferred Reporting Items for Systematic Reviews and Meta-Analysis (PRISMA) standards [101].
Eligibility criteria
Studies that met the following criteria were included in the review: a) Participants were adults (18+ years of age) with a diagnosis of PD; b) Instrumental methods were used to assess RSC; c) Studies were randomized controlled trials, cohort studies, case-control studies, case series or reports, dissertations, and single-subject case studies; d) Articles published in the English language.
Studies that met the following criteria were excluded in the review: a) Participants were healthy individuals, individuals with progressive neurological disorders other than PD, animals, or children; b) Non-instrumental methods were used to assess RSC or analyzed respiration and swallowing abilities independently; c) Expert opinions, tutorials, background information, in vitro studies, and conference abstracts; d) Articles published in languages other than English.
Search strategy and information sources
The search strategy using the keywords “((dysphagia OR deglutition OR swallow*) AND (respirat* OR breath*)) AND (Parkinson*)” was the most inclusive and yielded several articles. We chose the following seven databases: PubMed, EMBASE, Central, Web of Science, ProQuest Dissertations & Theses, Scopus, and CINAHL. The search string utilized for PubMed was: ((“deglutition disorders"[MeSH Terms] OR (“deglutition"[All Fields] AND “disorders"[All Fields]) OR “deglutition disorders"[All Fields] OR “dysphagia"[All Fields] OR “dysphagias"[All Fields] OR (“deglutition"[MeSH Terms] OR “deglutition"[All Fields] OR “deglutitions"[All Fields] OR “deglutitive"[All Fields]) OR “swallow*"[All Fields]) AND (“respirat*"[All Fields] OR “breath*"[All Fields]) AND “parkinson*"[All Fields]); Translations: dysphagia: “deglutition disorders"[MeSH Terms] OR (“deglutition"[All Fields] AND “disorders"[All Fields]) OR “deglutition disorders"[All Fields] OR “dysphagia"[All Fields] OR “dysphagias"[All Fields]; deglutition: “deglutition"[MeSH Terms] OR “deglutition"[All Fields] OR “deglutitions"[All Fields] OR “deglutitive"[All Fields]. Using this search strategy and these databases, we completed an initial search. Repeat literature searches were completed in February 2022 and February 2023 in all databases to review for any additional articles.
We conducted an ancestral bibliography search to ensure that we did not miss any eligible articles. Using the online tool SnowGlobe [102] that assists with backward literature searching, we scanned the reference lists of all included papers [85, 87, 88, 91–93, 96]. We manually searched the back references of two included articles that were undetected by SnowGlobe [82, 83]. In February 2022, the references of the two new articles included in this systematic review were manually searched as well [99, 100]. No additional articles met the inclusion criteria.
Selection process
The first author (RR) screened all articles at the title, abstract, and full text stages. We utilized the Rayyan QCRI software, an online systematic review manager for orderly screening of articles [103]. Once duplicates were excluded from the initial search results, title screen was completed based on the following keywords: (“dysphagia”/ “deglutition”/ “swallowing”/ “swallow”/ “swallowing disorder” and/or “breathing”/ “respiration”/ “respiratory”/ “pulmonary” and/or “Parkinson”/ “Parkinson’s disease”). Studies that met this eligibility criteria were then reviewed for further inclusion via abstract screening or full text review. The full-text study selection process was completed using a brief code consisting of 5 criteria: the mention of the keyword “respiratory swallow coordination”, the participant diagnosis, evaluation type, intervention technique, and the outcome measures provided. Each code had a criterion of 1 or 0, as outlined in Supplementary Table 1.
Thereafter, a blinded rater (RS) independently screened 20% of the studies at the title, abstract, and full-text stage to ensure reliability, such that no eligible studies were missed in the study selection process. For the final inclusion of studies, complete agreement was required. Any disagreement was resolved through discussion between the two authors. If an agreement could not be reached, the senior author was consulted.
Data collection process and data items
Following study selection, data collection was completed utilizing a full codebook of 82 questions related to descriptive measures, outcome measures, and the risk of bias, as outlined in Supplementary Table 2.
Study risk of bias assessment
A modified version of the NIH Quality Assessment Tool for Observational Cohort and Cross-Sectional studies [104] was utilized to assess methodological quality of the studies included in this systematic review. This tool consisted of one question focusing on the research question, four questions targeting subject characteristics and recruitment, three questions targeting study methodology, and one question specifically answering blinding and reliability of the study. Ordinal and binary data were coded for each question and each study. Reliability was completed by two independent raters (RR, RS) for 20% of studies. Disagreements were discussed until a consensus was reached.
Meta-analysis
Raw data of respiratory phase patterns were extracted from all included studies. These patterns were categorized as: expiration-swallow-expiration (EX-EX), inspiration-swallow-expiration (IN-EX), expiration-swallow-inspiration (EX-IN), inspiration-swallow-inspiration (IN-IN), expiration before a swallow (EX-S), inspiration before a swallow (IN-S), expiration after a swallow (S-EX), and inspiration after a swallow (S-IN). Certain studies reported patterns other than EX-EX and grouped them as non-expiration-swallow-expiration (non-EX-EX).
Raw frequency and percentages of respiratory phase patterns (EX-EX, non-EX-EX, IN-EX, EX-IN, IN-IN, EX-S, IN-S, S-EX, S-IN) were transformed into logit event rates to compute effect sizes for analysis. The robust variance estimation (RVE) method [105] was employed to account for the dependent structure of the correlated effect sizes because effect sizes were not from an independent sample of participants. RVE method assumes the covariance structure between dependent data by accounting for the different correlation strengths between effect sizes from the same group of participants. All analyses were performed using the Robumeta package in R [106]. Three models were created to determine if there were a predominance of atypical respiratory phase patterns in individuals with PD and healthy controls. The three respiratory-swallow phase patterns used for purposes of this meta-analysis were EX-EX patterns, non-EX-EX patterns, and specific patterns before or after a swallow (EX-S, IN-S, S-IN, S-EX). The primary model was created to calculate a pooled estimate of EX-EX and non-EX-EX respiratory phase patterns in individuals with PD and healthy controls, and the pooled difference between the groups. Meta-analysis was run using extracted data reflecting EX-EX respiratory phase patterns from 24 trials, and non-EX-EX patterns from 15 trials. The second model was to determine the pooled estimate of specific respiratory phase patterns between individuals with PD and healthy controls, and the pooled difference between the groups. Meta-analysis was run using extracted data reflecting EX-S from 4 trials, IN-S from 9 trials, S-EX from 6 trials, and S-IN from 8 trials. The third model was to determine the impact of bolus type (thin liquids) on respiratory phase patterns. Meta-analysis was run using extracted data reflecting EX-EX respiratory phase patterns from 21 trials, and non-EX-EX patterns from 15 trials. From these models, we converted the logit event rates to percentages. A sensitivity analysis was done by bolus type to test if results were highly influenced by a single study. To test for the possibility of bias due to publication of selective or significant results by journals, a publication bias analysis was performed. Small sample corrections in the model accounting for dependent data were incorporated, and an Egger’s regression test was performed.
RESULTS
Study selection
We identified 12,118 articles in the initial literature search. The first author (RR) conducted a title and abstract screen utilizing the inclusion and exclusion criteria, resulting in a yield of 175 full text articles that were further assessed for eligibility. Brief coding was completed, and nine articles met the inclusion criteria which then underwent full coding and data extraction [82, 83, 85, 87, 88, 91–93, 96]. In February 2022, a repeat literature search was conducted, which identified 883 articles published in these databases since March 2021. Fifty-seven full-text articles were assessed for eligibility, and two additional articles met the inclusion criteria [99, 100]. In February 2023, a third literature search was conducted, identifying 759 articles published in these databases since February 2022. Fifty-one full text articles were screened for eligibility, and no new articles met the inclusion criteria. A total of eleven articles were included in this systematic review [82, 83, 85, 87, 88, 91–93, 96, 99, 100]. Figure 1 outlines the PRISMA flow chart. Interrater reliability exceeded 80% for all screening and extraction, and complete agreement was achieved for decisions regarding inclusion or exclusion of studies.
Fig. 1
The figure showcases the screening process using Preferred Reporting Items for Systematic Reviews and Meta-Analysis (PRISMA) for determining the eleven articles included in this systematic review.
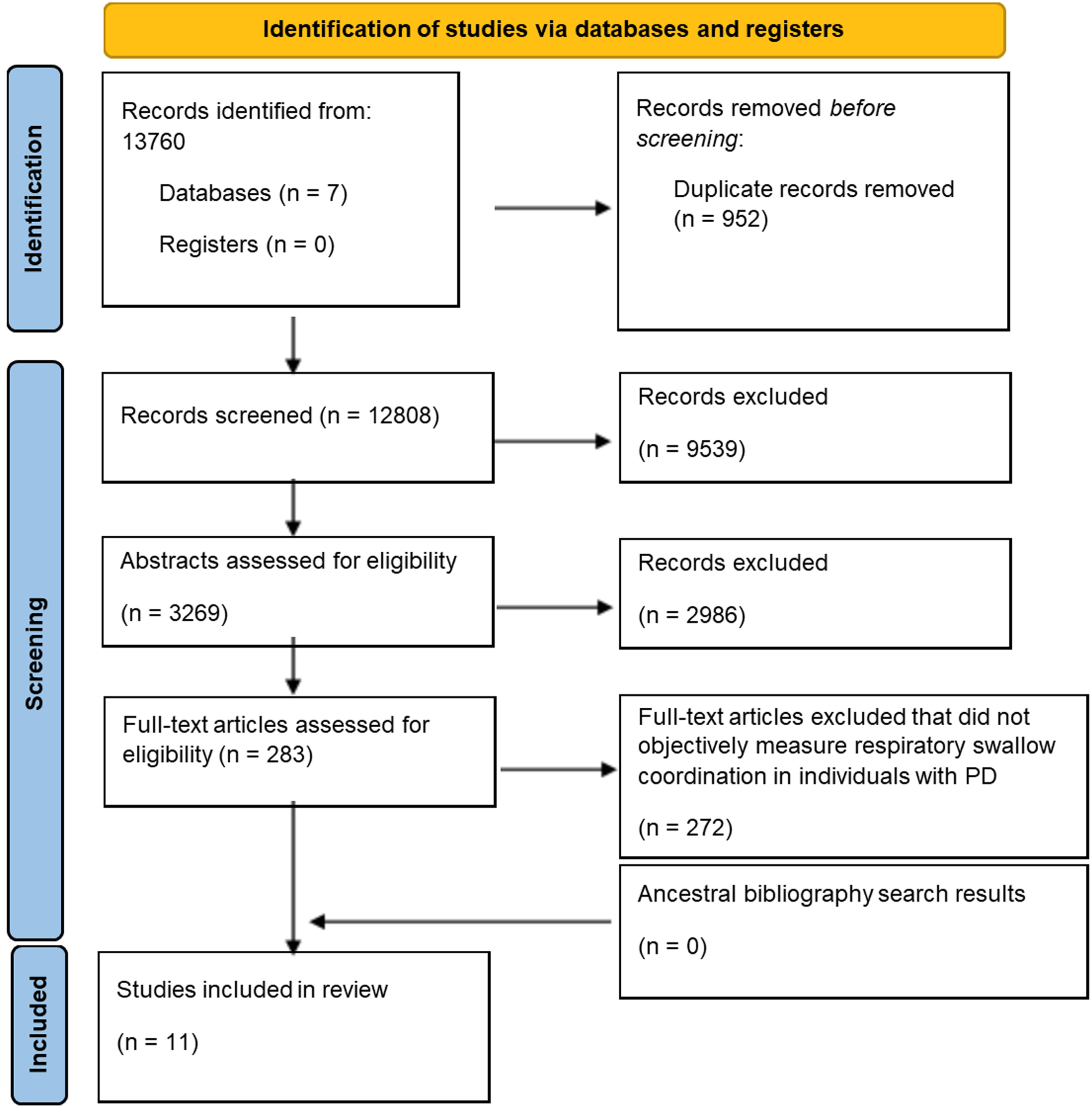
Study characteristics
Participant demographics
Of the eleven studies included in this review, the number of participants included in each study ranged from 1 to 42 (Mean = 22.63; Median = 25). The mean age of the participants was 68.61 years with an age range reported in nine of eleven (81.8%) studies [82, 83, 85, 88, 91, 92, 96, 99, 100] ranging from 40 to 88 years. Participant demographics and details are outlined in Table 1.
Table 1
Participant demographics
Studies | Number of participants with PD | Mean age (y)±Standard deviation (SD); Age range (y) | Sex | Mean disease duration (y)±Standard deviation (SD); Disease duration range (y) | Mean Hoehn-Yahr scale scores±Standard deviation Hoehn-Yahr scale scores; Range Hoehn-Yahr scale scores | Pharmacological dopamine use during study |
Curtis, 2020 pages 74–142 [82] | 24 | 69.5±9.9; 49 to 88 | Male: 17 Female: 7 | Not provided by study | 2±1.022; 1 to 4 | No information provided |
Curtis, 2020 pages 171–232 [83] | 12 | 66.83; 49 to 84 | Male: 10 Female: 2 | 7.42; 2 to 16 | 2.42; 1 to 4 | “On” stage |
Wang et al., 2017 [85] | 42; Control subjects recruited | 64.7±8.6; Not provided by study | Male: 27 Female: 15 | 8.95±4.86, 1 to 19 | 1.68±0.67; 1 to 3 | “On” stage |
Pinnington et al., 2000 [87] | 12; Control subjects recruited | 68; Not provided by study | Male: 7 Female: 5 | 11; Not provided by study | Not provided by study; 2 to 5 | “On” stage |
Lim et al., 2008 [88] | 10 | 63.5; 51 to 69 | Male: 6 Female: 4 | 8.5; 2 to 16 | 2.4; 1 to 4 | “On” stage |
Troche et al., 2011 [91] | 39 | 67.8±9.28; Not provided by study | Male: 29 Female: 10 | Not provided by study | Not provided by study; 2 to 4 during “on” stage | “On” stage |
Gross et al., 2008 [92] | 25; Control subjects recruited | 71±9.86; 53 to 84 | Male: 25 Female: 0 | Not provided by study | 2.4; 2 to 3 | “On” stage |
Curtis et al., 2020 [93] | 1 | 81; not applicable | Male: 1 Female: 0 | 8.5; Not applicable | Not applicable; score of 3 | “On” stage |
Curtis &Troche, 2020 [96] | 25 | 70.5; 40 to 87 | Male: 19 Female: 6 | Since diagnosis: 6.7±3.5; 8 to 16 | Not provided by study | No information provided |
Curtis et al., 2022 [99] | 33 | 69.8±10.6; Not provided by study | Male: 26 Female: 7 | Since diagnosis: 6.7±3.5; 8 to 16 | Not provided by study; | “On” stage |
Wang et al., 2018 [100] | 26 | 62.12±8.52; Not provided by study | Male: 19 Female: 7 | 8.5±4.65; 1 to 19 | 1.84±0.811; 1 to 3 | “On” stage |
Risk of bias
Risk of bias was assessed using the modified NIH Quality Assessment Tool for Observational Cohort and Cross-Sectional studies [104] with scores ranging from 0 to 9, and 9 being the highest score. All studies clearly stated the research question, and all studies recruited from the same or similar populations including the severity of PD. Ten of the eleven (90.9%) studies [82, 83, 85, 87, 91–93, 96, 99, 100] clearly defined the study population. One of eleven (9.1%) studies [92] provided justification related to sample size. Variables were clearly defined and statistically evaluated in all studies. However, insufficient or unclear information for blinding of raters was provided in six of eleven (54.5%) studies [85, 87, 88, 96, 99, 100].
Results of individual studies and syntheses
Methods for recording respiratory-swallow coordination
All of the studies used variable instrumental methods and measures to assess the coordination of respiration and swallowing. Methods for recording respiratory-swallow signals from each study are outlined in Table 2.
Table 2
Methods for recording respiratory-swallow coordination, swallowing, and respiration.
Studies | Instrumentation utilized to measure respiratory-swallow coordination, swallowing and respiration |
Curtis, 2020 pages 74–142 [82] | Respiratory inductive plethysmography; Nasal airflow recording via nasal airflow cannula; Submental surface electromyography; Spirometry with face mask; Perceived respiratory sensation testing via inspiratory resistive loading, respiratory strength via MicroRPM respiratory manometer; Signals input directly into a PowerLab data acquisition system, digitized, and recorded at 2kHz; Lab Chart data analysis software sync all signals and stored them for analyses; FEES; Patient reported outcome measures, Unified Parkinson’s Disease Rating Scale. |
Curtis, 2020 pages 171–232 [83] | Respiratory inductive plethysmography via Respitrace system; Nasal airflow monitoring via nasal airflow cannula; Spirometry with face mask; Submental surface electromyography; FEES; Patient reported outcome measures; Unified Parkinson’s Disease Rating Scale. |
Wang et al., 2017 [85] | Non-invasive electrophysiological swallowing and respiration assessment was based on BIOPAC MP100 system; Sensors used to detect bio-signals of swallowing and respiration; Nasal cannula for airflow monitoring; Force sensing resistor (FSR) sensors for laryngeal excursion; Submental surface electromyography; Patient reported outcome measures; Unified Parkinson’s Disease Rating Scale. |
Pinnington et al., 2000 [87] | Non-invasive method - Exeter Dysphagia Assessment Technique (EDAT) recorded; Simultaneous respiratory synchronization with swallowing; Bi-directional airflow recorded through nasal cannula; Swallow sounds recorded by microphone held against throat; All signals recorded by chart recorder; Analyzed subsequently by paper or disk record; Patient reported outcome measures; Unified Parkinson’s Disease Rating Scale. |
Lim et al., 2008 [88] | EMG surface electrodes placed midline under the chin; Nasal cannula attached to patient and connected to Digital Swallowing Workstation v.3.0.1; Lung function using closed loop spirometry; Assessment of swallowing using a nasoendoscope; Timed test of swallowing; Patient reported outcome measures; Unified Parkinson’s Disease Rating Scale. |
Troche et al., 2011 [91] | Videofluoroscopy - Philips radiographic/fluoroscopic unit; Nasal respiratory flow captured using a 7-foot nasal cannula coupled to Swallow Signals lab; Respiratory tracing captured at 250 Hz used to generate digital display of both respiratory phase and duration of apneic pause during swallowing; Respiratory events interpreted from Kay elemetrics signal lab output; Patient reported outcome measures. |
Gross et al., 2008 [92] | Respiratory inductance plethysmography; Kaypentax swallowing station with swallowing signals lab; Nasal cannula that connected to transducer in the Swallowing signals lab; Submental surface electromyography; Patient reported outcome measure; Unified Parkinson’s Disease Rating Scale. |
Curtis et al., 2020 [93] | Simultaneous respiratory plethysmography; Nasal thermistor; Submental surface electromyography; Spirometry; Signals input directly into a PowerLab data acquisition system, digitized, and recorded at 2kHz; Lab Chart data analysis software sync all signals and stored them for analyses; FEES; respiratory strength via MicroRPM respiratory manometer; Patient reported outcome measures; Unified Parkinson’s Disease Rating Scale. |
Curtis &Troche, 2020 [96] | Respiratory inductive plethysmography (RIP) via the Respitrace system; Elastic Respitrace bands around ribcage and abdomen; Signals were input directly to a PowerLab data acquisition system, digitized, and recorded at 2 kHz; Video capture of the flexible endoscopic evaluation of swallowing was also recorded and inputted into the same PowerLab system; FEES; LabChart data analysis software was used to synchronize the RIP signals with the FEES video recording; Patient reported outcome measures. |
Curtis et al., 2022 [99] | Simultaneous respiratory plethysmography and FEES; Respiratory inductive plethysmography using Respitrace system; Elastic Respitrace bands around ribcage and abdomen; Input directly into PowerLab data acquisition system, digitized, and recorded at 2 kHz; Sync with FEES using external camera with LabChart video capture module; Patient reported outcome measures. |
Wang et al., 2018 [100] | Electrophysiological monitoring system (BIOPAC MP 100 system); AcqKnowledge software for data recording and analysis; Simultaneously recorded swallowing and respiration biosignals, including sEMG, nasal airflow and thyroid cartilage excursion; Patient reported outcome measures; Unified Parkinson’s Disease Rating Scale. |
Measures of respiratory-swallow coordination
In this systematic review, RSC measures focusing on respiratory phase patterning, respiratory cessation duration, and lung volume at swallow initiation in individuals with PD were examined. The impact of RSC and potential linkages to swallowing impairments and airway protection were reviewed.
Respiratory phase
Ten of eleven (90.9%) studies [82, 83, 85, 87, 91–93, 96, 99, 100] reported varied respiratory phase patterns in individuals with PD, differing from the EX-EX phase pattern observed in healthy controls [26, 39–74]. Results from each study are outlined in Table 3.
Table 3
Summary of respiratory phase patterns during swallowing for each individual study
Studies | Respiratory phase patterns; total number of swallows; bolus characteristics |
Curtis, 2020 pages 74–142 [82] | EX-S (Expiration prior to cessation): 47.90%, Range: 9 to 84.8% S-EX (Expiration post cessation): 71.80%, Range: 31 to 96.9% EX-EX: 30.2%, Range: 6 to 69.7% Total number of swallows: 729 Bolus type: 10 trials of preferred bolus volume of thin liquid and 23 trials across 11 swallowing conditions |
Curtis, 2020 pages 171–232 [83] | EX-EX: Probe (delayed baseline): 22.50%, Probe (Pre-RST): 25%, Generalization testing (delayed baseline): 27.30%, Generalization testing (Pre-RST): 30.70% Total number of swallows: Probe: 120 each, Generalization testing: 276 each. Bolus type: Probe task: preferred bolus volume of safest thin liquid consistency; Generalization task: - 23 trials across 11 swallowing conditions. |
Wang et al., 2017 [85] | EX-EX: PD group: 30%, control group: 52% Non-EX-EX: PD group: 70%, control group: 48% Total number of swallows: 265 Bolus type: 1 mL, 3 mL, 5 mL, 10 mL, and 20 mL of thin liquid bolus |
Pinnington et al., 2000 [87] | EX-S: PD: 83%, control group: 88% IN-S: PD: 10%, control group: 12% RC-S: PD: 7% S-EX: PD: 80%, control group: 99% S-IN: PD: 18% S-RC: PD: 2% Total number of swallows: 120 Bolus type: 10 trials of 5 mL thin liquid bolus |
Lim et al., 2008 [88] | S-EX: “off” phase of dopamine: 91%, “on” phase of dopamine: 100% Total number of swallows: 100 each Bolus type: 10 trials of 8 mL of thin liquid bolus |
Troche et al., 2011 [91] | EX-S: 70.10% IN-S: 29.90% S-EX: 86.40% S-IN: 13.60% Total number of swallows: 670 Bolus type: 10 trials of 5 mL thin liquid bolus and one 3 oz sequential thin liquid swallow by cup |
Gross et al., 2008 [92] | IN-S: PD: Pudding: 16.60%, Cookie: 13.75%; healthy: Pudding: 9.56%, Cookie: 1.86% S-IN: PD: Pudding: 25.53%, Cookie: 24.76%; healthy: Pudding: 7.39%, Cookie: 9.34% Total number of swallows: PD: Pudding: 235, Cookie: 210/211 Bolus type: 10 trials of semisolid (pudding) and 9 trials of 2.5 g solid (cookie) portions |
Curtis et al., 2020 [93] | Probe measures: EX-EX: Probe: 23.40%, Generalization: 28.20% IN-EX: Probe: 66.60%, Generalization: 71.80% EX-IN: Probe: 3.40%, Generalization: 0% IN-IN: Probe: 6.60%, Generalization: 0% Total number of swallows: Probe: 20, Generalization: 14 Bolus type: 10 trials of cup sips of thin liquid and non-cued saliva swallows; generalization: liquids and solids |
Curtis &Troche, 2020 [96] | IN-S: 21.3% S-IN: 8% EX-EX: 70.7% IN-EX: 22.7% EX-IN: 6.7% IN-IN: 0% Total number of swallows: 75 Bolus type: Three trials of 5 mL thin liquid administered by syringe |
Curtis et al., 2022 [99] | IN-S: 55.20% S-IN: 19% EX-EX: 33.10% IN-EX: 48.40% EX-IN: 11% IN-IN: 6.70% Total number of swallows: 163 Bolus type: Five trials of 5 mL thin liquid by 30 mL medicine cup |
Wang et al., 2018 [100] | EX-EX: PD: 1 mL: 22%, 3 mL: 29%, 5 mL: 33%, 10 mL: 38%, 20 mL: 22%, total: 30% EX-EX: Healthy: 1 mL: 59%, 3 mL: 48%, 5 mL: 53%, 10 mL: 53%, 20 mL: 44%, total: 52% Non-EX-EX: PD: 1 mL: 78%, 3 mL: 71%, 5 mL: 67%, 10 mL: 62%, 20 mL: 78%, total: 70% Non-EX-EX: Healthy: 1 mL: 41%, 3 mL: 52%, 5 mL: 47%, 10 mL: 47%, 20 mL: 56, total: 48% Total number of swallows: PD: 248, healthy: 231 Bolus type: 3 trials of each bolus volume of thin liquid (1 mL, 3 mL, 5 mL, 10 mL, 20 mL) |
EX-EX: expiration-swallow-expiration; IN-EX: inspiration-swallow-expiration; EX-IN: expiration-swallow-inspiration; IN-IN: inspiration-swallow-inspiration; EX-S: expiration before a swallow; IN-S: inspiration before a swallow; S-EX: expiration after a swallow; S-IN: inspiration after a swallow; non-EX-EX: non-expiration-swallow-expiration.
Meta-analyses of respiratory phase patterns
Pooled estimates of EX-EX vs non-EX-EX in PD and healthy group
For individuals with PD, EX-EX patterns were reported by seven studies [82, 83, 85, 93, 96, 99, 100] from 19 trials, and the pooled percentage estimate is 40.8%. Non-EX-EX patterns were reported by two studies [85, 100] from 10 trials, and the pooled percentage estimate is 59.9%. These results show that individuals with PD have respiratory phase patterns consisting of greater non-EX-EX patterns, irrespective of bolus consistency or volume. Results are outlined in Table 4 and Figs. 2 and 3.
Table 4
Pooled estimates and differences in percentage of respiratory phase patterns between individuals with PD and healthy controls by bolus type
Bolus type | Respiratory phase patterns | Pooled percentage estimate of PD patients | Pooled percentage estimate of healthy controls | Pooled estimated difference in percentage between PD patients and healthy controls | Pooled estimated difference in logit between PD patients and healthy controls (95% CI) | p | No. of trials | Reported by no. of studies |
All | EX-EX | 40.8% | 50.6% | –9.9% | –0.40 (–0.53, –0.27) | 0.001 | PD: 19; controls: 5 | PD: 7; controls: 1 |
Thin | EX-EX | 40.8% | 50.6% | –9.8% | –0.40 (–0.56, –0.23) | 0.004 | PD: 16; controls: 5 | PD: 6; controls: 1 |
All | Non-EX-EX | 59.9% | 49.4% | 10.5% | 0.43 (0.21, 0.65) | 0.026 | PD: 10; controls: 5 | PD: 2; controls: 1 |
Thin | Non-EX-EX | 59.9% | 49.4% | 10.5% | 0.43 (0.21, 0.65) | 0.026 | PD: 10; controls: 5 | PD: 2; controls: 1 |
All | EX-S | 58.4% | 70.4% | –12.0% | –0.53 (–1.43, 0.38) | 0.130 | PD: 3; controls: 1 | PD: 3; controls: 1 |
All | IN-S | 35.5% | 22.4% | 13.1% | 0.65 (–0.75, 2.05) | 0.160 | PD: 6; controls: 3 | PD: 4; controls: 2 |
All | S-EX | 76.6% | 88.0% | –11.5% | –0.81 (–2.53, 0.91) | 0.215 | PD: 5; controls: 1 | PD: 4; controls: 1 |
All | S-IN | 31.9% | 26.0% | 6.0% | 0.29 (–0.08, 0.67) | 0.095 | PD: 6; controls: 2 | PD: 4; controls: 1 |
Fig. 2
Pooled estimate of EX-EX respiratory phase pattern by groups (in proportion).
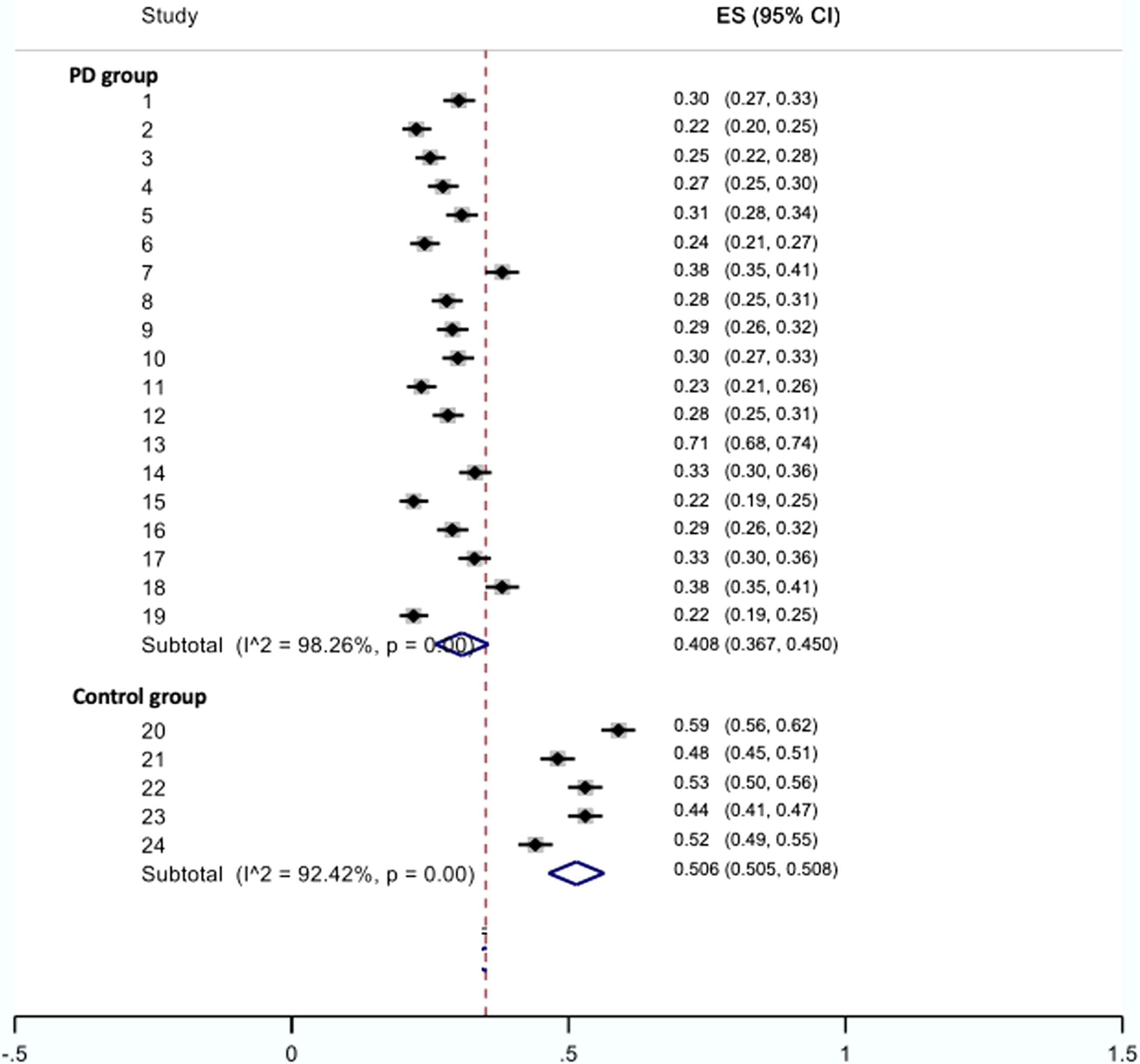
Fig. 3
Pooled estimate of non-EX-EX respiratory phase pattern by groups (in proportion).
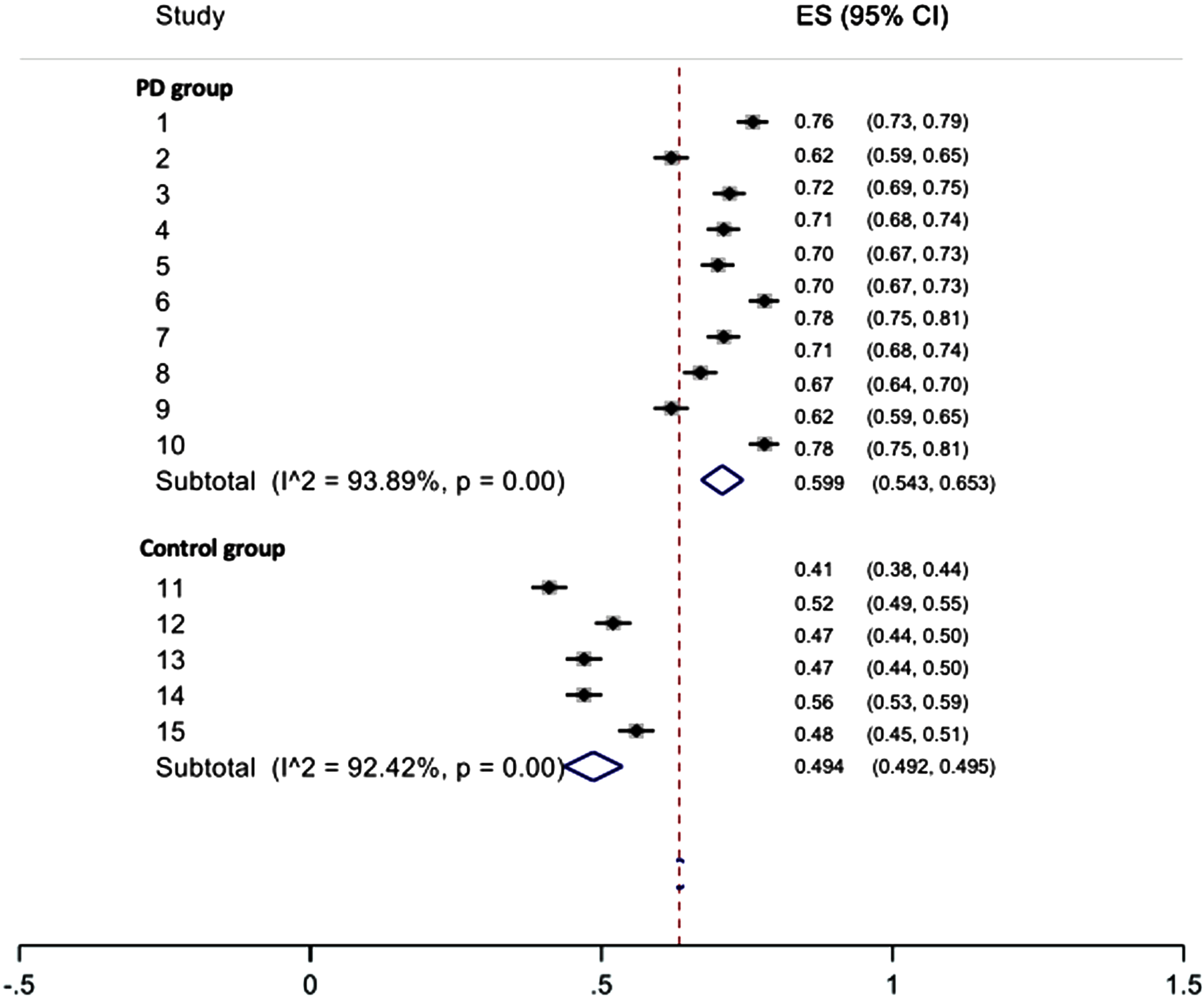
For healthy controls, EX-EX were reported by only one study [85] from 5 trials, and the pooled percentage estimate is 50.6%. Non-EX-EX patterns were reported by one study [85] from 5 trials, and the pooled percentage estimate is 49.4%.
Pooled difference of EX-EX vs non-EX-EX in PD and healthy group and impact of bolus type
The PD group’s EX-EX percentage was 9.9% lower than the control group (p = 0.001) for all bolus types. Pooled estimated difference for EX-EX was similar for thin liquid only (–9.8%, p = 0.004). The PD group’s non-EX-EX percentage was 10.5% higher than the control group (p = 0.026) for all bolus types. Pooled estimated difference for non-EX-EX was similar for thin liquid only (10.5%, p = 0.026). Results are summarized in Table 4.
Sensitivity analysis and publication bias
A sensitivity analysis was completed by restricting the analysis to thin liquids only, and no change in results was observed. This indicates that no single study had extreme percentage-weights to highly influence the estimates. Publication bias was assessed by Egger’s regression tests for asymmetry (for EX-EX patterns: t = 4.65, p = 0.0001; for non-EX-EX patterns: t = –7.16, p < 0.0001). This indicates that there may be potential publication bias in the meta-analysis findings.
Respiratory cessation
Ten of eleven (90.9%) studies measured respiratory cessation as a RSC outcome measure [82, 83, 85, 87, 91–93, 96, 99, 100]. Six of ten (60%) studies [82, 83, 87, 93, 96, 99] reported longer respiratory cessation duration in individuals with PD when compared to healthy controls. In contrast, two of the ten (20%) studies [85, 92] reported no significant difference in respiratory cessation duration between PD and control groups. Two of the ten (20%) studies [91, 100] reported inconclusive or unclear findings of respiratory cessation duration in individuals with PD. Individual characteristics of each study are outlined in Table 5.
Table 5
Measures of respiratory cessation and lung volume at swallow initiation for each individual study
Studies | Respiratory cessation | Lung volume at swallow initiation |
Curtis, 2020 (pages 74–142) [82] | Average - 3.55±1.34 s Range –1.49 to 7.39 s | 11.5±5.6% Vital capacity (VC) Range –0% VC to 23.1% VC |
Curtis, 2020 (pages 171–232) [83] | Average during probe measures - 3.24 s at delayed baseline and 1.68 s pre-intervention Average during Generalization testing -4.36 s at delayed baseline and 4.14 s pre-intervention | Average during probe measures - 10.8% VC at delayed baseline and 13% VC pre-intervention Average during Generalization testing -12.8% VC at delayed baseline and 14.6% VC pre-intervention |
Pinnington et al., 2000 [87] | PD - 0.69±0.17 s Healthy - 0.42±0.11 s | Not measured |
Curtis et al., 2020 [93] | Average during probe measures - 3.17±2.29 s (Range –0.79 to 9.30 s) Average during Generalization testing - 5.58±2.58 s (Range –1.37 to 11.68 s) | Average during probe measures - 66.0±12.3% VC (Range –44.7 to 99.3% Vital capacity) Average during Generalization testing - 67.5±10% VC (Range –53.6 to 95.4% Vital capacity) |
Curtis &Troche, 2020 [96] | Average - 1.74±1.11 s; (Percentile ranges –25% –1.03 s, 50% –1.39 s, 75% –1.81 s | 34.6±41.2% Tidal breathing above end expiratory level |
Curtis et al., 2022 [99] | Average - 2.96±2.78 s Avg coefficient of variation –41.4% (SD = 20.1%); Percentile ranges –25% –1.21 s, 50% –1.88 s, 75% –3.68 s | 83.3±81.8% Tidal volume relative to end expiratory level of rest breathing; 16.9±16.0% vital capacity relative to end expiratory level of rest breathing (SD = 16.0); Avg coefficient of variation –12.9% (SD = 8.2%) |
Gross et al., 2008 [92] | PD group - Average for swallows occurring during expiration - 0.643±0.133 (Range –0.409 to 1.300 s) Average for swallows occurring during inspiration -0.665±0.182 (Range –0.417 to 1.325 s) Control group - Average for swallows occurring during expiration - 0.649±0.133 (Range –0.377 to 1.357 s) Average for swallows occurring during inspiration -0.887±0.274 (Range –0.576 to 1.744 s) | Low tidal volume (no value provided) |
Wang et al., 2017 [85] | No value provided | Not measured |
Wang et al., 2018 [100] | No value provided | Not measured |
Troche et al., 2011 [91] | No value provided | Not measured |
Lim et al., 2008 [88] | Not measured | Not measured |
SD: standard deviation.
Varying durations of respiratory cessation were reported by studies included in this review. Studies that reported longer durations of respiratory cessation associated with swallowing events exhibited an average of 2.6 s (range: 0.69–5.58 s), compared to healthy or control groups (range: 0.5–1.5 s) [74].
Lung volume at swallow initiation
Six of eleven (54.5%) studies [82, 83, 92, 93, 96, 99] evaluated lung volume at swallow initiation (LVSI) with varying conclusions. Results of lung volume at swallow initiation ranged from 0% vital capacity to 99.3% vital capacity. Individual characteristics of each study are outlined in Table 5.
Impact on swallowing efficiency
Five of eleven (45.5%) studies [82, 83, 91, 93, 99] linked RSC outcome measures to the presence of swallowing impairment or reduced airway protection. Four of eleven (36.4%) studies [85, 87, 96, 100] implicated a relationship between RSC outcomes and swallowing function and airway protection. One of eleven (9.1%) studies [92] reported inconclusive results. In addition, one of eleven (9.1%) studies [88] correlated RSC with pharmacological dopaminergic intervention.
Variable methodology and results were found in studies that linked RSC outcome measures to the presence of swallowing impairment or reduced airway protection [82, 83, 91, 93, 99]. Troche et al. (2011) reported significant positive relationships between respiratory events, respiratory cessation duration, and penetration-aspiration scale scores (PAS) [107] assessed by videofluoroscopy [91]. Similarly, Curtis (2020; pages 74–142) reported that RSC measures such as respiratory phase patterning, respiratory cessation duration, and lung volume at swallow initiation were altered, and these changes were linked with the severity of vallecular and pyriform residue, and the presence of penetration-aspiration assessed by Flexible Endoscopic Evaluation of Swallowing (FEES) in an participants with PD [82]. Likewise, Curtis et al. (2021) reported that participants demonstrated PAS scores assessed by FEES ranging from 1–8, with a score of 8, representing silent aspiration, was most frequent in 27.2% of participants [99]. Swallows in these participants were frequently characterized by IN-EX patterns with initiation near the end-inspiratory level of tidal breathing, and a median respiratory pause duration of approximately 2 s [99].
Intervention studies that examined changes in RSC showed similar results to the observational studies. These respiratory-swallow interventions trained individuals with PD to perform the typical EX-EX respiratory pattern. In a single subject treatment study, Curtis et al. (2020) reported descriptive analyses revealing improvements from baseline measures in PAS scores assessed by FEES, vallecular and piriform residue assessed by the 5-point Yale Pharyngeal Residue Severity Rating scale [108] and Dynamic Imaging Grade of Swallowing Toxicity (DIGEST) [109] following completion of respiratory-swallow training [93]. In another experiment Curtis (2020; pages 171–232) reported that 58.3% of participants had moderate dysphagia as per Dynamic Imaging Grade of Swallowing Toxicity for FEES (DIGEST-FEES) [83]. Significant reductions in the frequency of abnormal PAS scores (p < 0.0005), vallecular residue (p = 0.001), piriform residue (p = 0.001), laryngeal vestibule residue (p < 0.0005), vocal fold residue (p = 0.002), and subglottic residue (p = 0.010) immediately post respiratory-swallow training and during the 1-month follow up assessment were reported. Patient reported outcome measures of swallowing using the Functional Oral Intake Scale (FOIS) showed significant improvement post respiratory-swallow training [83].
DISCUSSION
We completed a systematic review of the literature resulting in identification of 13,760 articles of which eleven articles met the inclusion criteria [82, 83, 85, 87, 88, 91–93, 96, 99, 100]. Results of this review supported the presence of atypical RSC in individuals with PD, with a meta-analysis reporting an estimated 60% of non-EX-EX and 40% of EX-EX respiratory phase patterns surrounding swallowing, irrespective of bolus type. This differs from the predominant occurrence of swallows bracketed by expiration in healthy adults [26, 39–74].
Methods of data acquisition and analysis
Variable measures of evaluation to assess RSC in individuals with PD were utilized in the studies included in this review. Five of eleven (45.5%) studies [82, 83, 93, 96, 99] used Flexible Endoscopic Evaluation of Swallowing (FEES) in attempt to objectively assess swallowing physiology simultaneously during respiratory-swallow measurements. FEES is a minimally invasive procedure, with a scope passed through the nasopharynx and positioned at a point posterior to the epiglottis where the laryngeal structures can be clearly visualized. Swallowing physiology is analyzed upon oral intake of measured boluses of food and liquid by the patient [110]. The use of FEES during respiratory-swallow measurements presents some challenges. A prevalent complaint during the administration of FEES is patient discomfort [111]. Secondly, there is partial obstruction of the nasopharynx during FEES due to insertion of the scope through the nose. These challenges may negatively disrupt the natural, synergistic relationship between respiration and swallowing, impacting the incidence of naturally occurring swallows. When performing FEES in individuals with PD, an approximate increase in lung volume at swallow initiation by 3% vital capacity as a function of the laryngoscope was reported [82]. The clinical impact of this effect was unknown [82]. Use of videofluoroscopic procedures during respiratory-swallow measurements alleviates some of the physiological load associated with the indwelling endoscope used in FEES and may lead to a more accurate representation of a patient’s typical respiratory-swallow pattern. Additionally, use of varying methodologies utilized to detect a swallow, such as identification of endoscopic white-out on the FEES, respiratory pause on nasal plethysmography signals, and placement of digital tags manually during respiratory data acquisition [96, 99] may have also confounded direct comparison of results.
Measures of respiratory-swallow coordination
For this review, RSC measures included respiratory phase patterning, respiration cessation duration, and lung volume at swallow initiation in individuals with PD.
Respiratory phase
This review and meta-analysis support that individuals with PD exhibit an estimated 60% of non-EX-EX and 40% of EX-EX respiratory phase patterns surrounding swallowing, irrespective of bolus type. In some cases, we were unable to directly compare all studies due to differences in the operational definitions and labeling of phase patterns.
The high occurrence of atypical respiratory phase patterns in individuals with PD may be attributed not only to PD, but also to concurrent clinical factors such as the known age-related changes to swallowing [26, 71] and respiration [112, 113]. These factors, when combined with PD, may consequently increase the risk of penetration or aspiration of liquids or solids during a swallow.
Previous review studies confirmed that the EX-EX respiratory phase pattern remains predominant in adults across the age continuum of 18 to 83 years [74]. It has been shown in other disease populations that initiation of swallowing during the expiratory phase of respiration at mid to low lung volume provides a neutral position of the tongue base and larynx, creating an ideal physiologic set point for airway protection and bolus clearance movements that occur during pharyngeal swallowing [114]. In contrast, this meta-analysis reports an estimated 50% of non-EX-EX and 50% of EX-EX respiratory phase patterns in the control group of healthy older adults, with mean age of 63 years [85]. However, these findings are derived from a single study [85] and should be interpreted with caution. Due to this limitation, the estimates for respiratory phase patterns in healthy controls from this review cannot be compared or generalized.
Respiratory cessation
This review supports that individuals with PD exhibit longer respiratory cessation durations during swallowing when compared to healthy controls. Studies included in this review implemented varying terminologies, definitions, and methods to measure respiratory cessation in individuals with PD, which may have contributed to variability of results. Terms utilized were ‘respiratory pause duration’ [82, 83, 99], ‘swallowing respiratory pause’ [85, 100], ‘pharyngeal part’ or ‘deglutition apnoea’ [87], ‘swallowing apnea’[91], ‘duration of deglutitive apnea’ [92], and ‘swallow apnea duration’ [93, 96]. The term “apnea” can be associated with presence of a disease or deficit [115]. As such, a more physiologically appropriate term to use in studies of RSC and the inhibition in respiration resulting from swallowing is ‘respiratory cessation’ or ‘respiratory pause’ [79, 116].
If shown in future studies using clearly defined methods and measures, the prevalence of longer respiratory cessation durations during swallowing in individuals with PD suggests that longer periods of cessation may be a compensatory strategy for prolonged airway closure and increased airway clearance [82]. Longer respiratory cessation may enable increased airway protection related to impaired movements of swallowing or altered RSC. It should be noted that this potential compensatory strategy may also result in dyspnea or shortness of breath in patients with PD and co-existing respiratory disorders.
Lung volume at swallow initiation
This review supports that individuals with PD initiate swallows at lung volumes that are different from typical lung volumes at swallowing initiation reported in healthy adults [26, 39, 41, 57, 60, 61, 63, 67–69]. Interpretation and conclusions are difficult, however, because of the wide variations in the nature of respiratory methods and values to report lung volume at swallowing initiation. For example, some studies reported lung volume at swallow initiation as a percentage of vital capacity (% VC) relative to end expiratory level of tidal breathing [82, 83, 93]. Others used a percentage of the participants’ average tidal breathing range (% TB) [96], or as a percentage of the participant’s average tidal volume (% TV) relative to end-expiratory level [92, 99]. Further, varying methods used to analyze the lung volume data and small sample sizes limit the ability to draw conclusions regarding the relevance of the lung volume data on swallowing physiology in patients with PD.
Individuals with PD exhibit significantly reduced chest wall expansion, upper airway obstruction and muscular dysfunction resulting in lower forced vital capacity (VC), forced expiratory volume in 1 s (FEV1), FEV1/VC, and peak expiratory flow rate (PEFR) compared to age and sex matched controls [15, 16, 36–38, 117]. Initiation of a swallow at atypical lung volumes may be related to these underlying changes in pulmonary function in patients with PD. If future studies with adequate sample sizes and controls allowing direct comparisons support the alterations in lung volume at swallow initiation in patients with PD, these lung volumes may contribute to reduced airway protection and bolus clearance based on information obtained in healthy adults. That is, initiation of swallowing at typical lung volumes provides significant airway and biomechanical advantages related to laryngeal closure and bolus clearance through the upper aerodigestive tract [41].
Limitations
Multiple variables, in addition to the variation in data recording and measurement, may have contributed to the inconsistency of RSC findings in this review. Nuances of the study protocols, such as swallowing instruction or cueing, mode of bolus delivery, and bolus characteristics have been shown to result in different respiratory swallow pattern and lung volume at swallow initiation [41]. Additionally, variations in participant demographics, severity and symptoms associated with PD for each patient, reported deficits in swallowing physiology, and use of pharmacological or dopaminergic intervention may have directly impacted respiratory-swallow measurements. Finally, despite thorough and widespread review of the literature targeting RSC and PD, relevant studies could have been missed. The potential of publication bias in the meta-analysis due to the limited and variable published studies, suggests that findings should be interpreted with caution until more studies are completed.
Conclusions
This systematic review appears to support that respiratory-swallow patterning, respiratory pause duration, and lung volume at swallow initiation differ when comparing patients with PD to healthy controls [26, 39–74]. These altered components of RSC may play a collective role in the high prevalence of dysphagia and aspiration in this patient population. However, results from this review should be interpreted with caution due to sample size limitations and high degree of variability in swallowing physiology and respiratory-swallow signal data acquisition, measurement, and analysis methods. Future research examining the nature and impact of RSC on swallowing physiology and airway protection, that use consistent, comparable, and reproducible methods and metrics in individuals with PD and healthy controls is warranted. Such studies will clarify our understanding of the relevance of RSC on swallowing function and airway protection in patients with PD, and the role of training typical coordination patterns on swallowing safety and efficiency observed in other patient groups with dysphagia.
ACKNOWLEDGMENTS
Ms. Rabab Rangwala: doctoral student stipend from Northwestern University. Ms. Raneh Saadi: doctoral student stipend from Northwestern University. Dr. Lee: salary from Northwestern University. Dr. Reedy: salary from Northwestern University. Dr. Kantarcigil: salary from Northwestern University. Dr. Roberts: salary from Northwestern University. Dr. Martin-Harris: salary from Northwestern University and Edward Hines, Jr. VA Hospital; NIH/NIDCD, 2K24DC12801-07 grant; Modified Barium Swallow Impairment Profile (MBSImP) royalties from Northern Speech Services through Medical University of South Carolina Foundation for Research Development.
CONFLICT OF INTEREST
The authors have no conflict of interest to report.
DATA AVAILABILITY
The data supporting the findings of this study are available within the article and/or its supplementary material.
SUPPLEMENTARY MATERIAL
[1] The supplementary material is available in the electronic version of this article: https://dx.doi.org/10.3233/JPD-230057.
REFERENCES
[1] | Pringsheim T , Jette N , Frolkis A , Steeves TDL ((2014) ) The prevalence of Parkinson’s disease: A systematic review and meta-analysis. Mov Disord 29: , 1583–1590. |
[2] | Wirdefeldt K , Adami HO , Cole P , Trichopoulos D , Mandel J ((2011) ) Epidemiology and etiology of Parkinson’s disease: A review of the evidence. Eur J Epidemiol 26: , S1–S58. |
[3] | Yang W , Hamilton JL , Kopil C , Beck JC , Tanner CM , Albin RL , Ray Dorsey E , Dahodwala N , Cintina I , Hogan P , Thompson T ((2020) ) Current and projected future economic burden of Parkinson’s disease in the U.S. NPJ Parkinsons Dis 6: , 15. |
[4] | Davie CA ((2008) ) A review of Parkinson’s disease. Br Med Bull 86: , 109–127. |
[5] | Kalia LV , Lang AE ((2015) ) Parkinson’s disease. Lancet 386: , 896–912. |
[6] | Blandini F , Nappi G , Tassorelli C , Martignoni E ((2000) ) Functional changes of the basal ganglia circuitry in Parkinson’s disease. Prog Neurobiol 62: , 63–88. |
[7] | Lang AE , Lozano AM ((1998) ) Parkinson’s disease. Second of two parts. N Engl J Med 339: , 1130–1143. |
[8] | Magrinelli F , Picelli A , Tocco P , Federico A , Roncari L , Smania N , Zanette G , Tamburin S ((2016) ) Pathophysiology of motor dysfunction in Parkinson’s disease as the rationale for drug treatment and rehabilitation. Parkinsons Dis 2016: , 9832839. |
[9] | Gibb WR , Lees AJ ((1988) ) The relevance of the Lewy body to the pathogenesis of idiopathic Parkinson’s disease. J Neurol Neurosurg Psychiatry 51: , 745–752. |
[10] | Coates C , Bakheit AM ((1997) ) Dysphagia in Parkinson’s disease. Eur Neurol 38: , 49–52. |
[11] | Kalf JG , de Swart BJM , Bloem BR , Munneke M ((2012) ) Prevalence of oropharyngeal dysphagia in Parkinson’s disease: A meta-analysis. Parkinsonism Relat Disord 18: , 311–315. |
[12] | Fuh JL , Lee RC , Wang SJ , Lin CH , Wang PN , Chiang JH , Liu HC ((1997) ) Swallowing difficulty in Parkinson’s disease. Clin Neurol Neurosurg 99: , 106–112. |
[13] | Mu L , Sobotka S , Chen J , Su H , Sanders I , Adler CH , Shill HA , Caviness JN , Samanta JE , Beach TG ; Arizona Parkinson’s Disease Consortium ((2012) ) Altered pharyngeal muscles in Parkinson disease. J Neuropathol Exp Neurol 71: , 520–530. |
[14] | Suttrup I , Warnecke T ((2016) ) Dysphagia in Parkinson’s disease. Dysphagia 31: , 24–32. |
[15] | D’Arrigo A , Floro S , Bartesaghi F , Casellato C , Sferrazza Papa GF , Centanni S , Priori A , Bocci T ((2020) ) Respiratory dysfunction in Parkinson’s disease: A narrative review. ERJ Open Res 6: , 00165–02020. |
[16] | Vincken WG , Gauthier SG , Dollfuss RE , Hanson RE , Darauay CM , Cosio MG ((1984) ) Involvement of upper-airway muscles in extrapyramidal disorders. A cause of airflow limitation. N Engl J Med 311: , 438–442. |
[17] | Izquierdo-Alonso JL , Jiménez-Jiménez FJ , Cabrera-Valdivia F , Mansilla-Lesmes M ((1994) ) Airway dysfunction in patients with Parkinson’s disease. Lung 172: , 47–55. |
[18] | Sabaté M , González I , Ruperez F , Rodríguez M ((1996) ) Obstructive and restrictive pulmonary dysfunctions in Parkinson’s disease. J Neurol Sci 138: , 114–119. |
[19] | Onodera H , Okabe S , Kikuchi Y , Tsuda T , Itoyama Y ((2000) ) Impaired chemosensitivity and perception of dyspnoea in Parkinson’s disease. Lancet 356: , 739–740. |
[20] | Herer B , Arnulf I , Housset B ((2001) ) Effects of levodopa on pulmonary function in Parkinson’s Disease. Chest 119: , 387–393. |
[21] | De Pandis MF , Starace A , Stefanelli F , Marruzzo P , Meoli I , De Simone G , Prati R , Stocchi F ((2002) ) Modification of respiratory function parameters in patients with severe Parkinson’s disease. Neurol Sci 23: , S69–S70. |
[22] | Weiner P , Inzelberg R , Davidovich A , Nisipeanu P , Magadle R , Berar-Yanay N , Carasso RL ((2002) ) Respiratory muscle performance and the perception of dyspnea in Parkinson’s disease. Can J Neurol Sci 29: , 68–72. |
[23] | Sathyaprabha TN , Kapavarapu PK , Pall PK , Thennarasu K , Raju T ((2005) ) Pulmonary functions in Parkinson’s disease. Indian J Chest Dis Allied Sci 47: , 251–257. |
[24] | Seccombe LM , Giddings HL , Rogers PG , Corbett AJ , Hayes MW , Peters MJ , Veitch EM ((2011) ) Abnormal ventilatory control in Parkinson’s disease—further evidence for non-motor dysfunction. Respir Physiol Neurobiol 179: , 300–304. |
[25] | Baille G , Perez T , Devos D , Deken V , Defebvre L , Moreau C ((2018) ) Early occurrence of inspiratory muscle weakness in Parkinson’s disease. Plos One 13: , e0190400. |
[26] | Martin-Harris B , Brodsky MB , Michel Y , Ford CL , Walters B , Heffner J ((2005) ) Breathing and swallowing dynamics across the adult lifespan. Arch Otolaryngol Head Neck Surg 131: , 762–770. |
[27] | Monteiro L , Souza-Machado A , Pinho P , Sampaio M , Nóbrega AC , Melo A ((2014) ) Swallowing impairment and pulmonary dysfunction in Parkinson’s disease: The silent threats. J Neurol Sci 339: , 149–152. |
[28] | Beyer MK , Herlofson K , Årsland D , Larsen JP ((2001) ) Causes of death in a community-based study of Parkinson’s disease: Causes of death in Parkinson’s disease. Acta Neurol Scand 103: , 7–11. |
[29] | Fall PA , Saleh A , Fredrickson M , Olsson JE , Granérus AK ((2003) ) Survival time, mortality, and cause of death in elderly patients with Parkinson’s disease. A 9-year follow-up: Mortality in Parkinson’s disease. Mov Disord 18: , 1312–1316. |
[30] | Martinez-Ramirez D , Almeida L , Giugni JC , Ahmed B , Higuchi M , Little CS , Chapman JP , Mignacca C , Shukla AW , Hess CW , Hegland KW , Okun MS ((2015) ) Rate of aspiration pneumonia in hospitalized Parkinson’s disease patients: A cross-sectional study. BMC Neurol 15: , 104. |
[31] | Morgante L , Salemi G , Meneghini F , Di Rosa AE , Epifanio A , Grigoletto F , Ragonese P , Patti F , Reggio A , Di Perri R , Savettieri G ((2000) ) Parkinson Disease survival: A population-based study. Arch Neurol 57: , 507–512. |
[32] | Jean A ((2001) ) Brain stem control of swallowing: Neuronal network and cellular mechanisms. Physiol Rev 81: , 929–969. |
[33] | Braak H , Tredici KD , Rüb U , de Vos RAI , Jansen Steur ENH , Braak E ((2003) ) Staging of brain pathology related to sporadic Parkinson’s disease. Neurobiol Aging 24: , 197–211. |
[34] | Hunter PC , Crameri J , Austin S , Woodward MC , Hughes AJ ((1997) ) Response of parkinsonian swallowing dysfunction to dopaminergic stimulation. J Neurol Neurosurg Psychiatry 63: , 579–583. |
[35] | Braak H , Braak E ((2000) ) Pathoanatomy of Parkinson’s disease. J Neurol 247: , II3–II10. |
[36] | Torsney K , Forsyth D ((2017) ) Respiratory dysfunction in Parkinson’s disease. J R Coll Physicians Edinb 47: , 35–39. |
[37] | Hovestadt A , Bogaard JM , Meerwaldt JD , van der Meche FG , Stigt J ((1989) ) Pulmonary function in Parkinson’s disease. J Neurol Neurosurg Psychiatry 52: , 329–333. |
[38] | Santos RBD , Fraga AS , Coriolano MDGWS , Tiburtino BF , Lins OG , Esteves ACF , Asano NMJ ((2019) ) Respiratory muscle strength and lung function in the stages of Parkinson’s disease. J Bras Pneumol 45: , e20180148. |
[39] | Martin BJ , Logemann JA , Shaker R , Dodds WJ ((1994) ) Coordination between respiration and swallowing: Respiratory phase relationships and temporal integration. J Appl Physiol 76: , 714–723. |
[40] | Paydarfar D , Gilbert RJ , Poppel CS , Nassab PF ((1995) ) Respiratory phase resetting and airflow changes induced by swallowing in humans. J Physiol 483: , 273–288. |
[41] | McFarland DH , Martin-Harris B , Fortin AJ , Humphries K , Hill E , Armeson K ((2016) ) Respiratory-swallowing coordination in normal subjects: Lung volume at swallowing initiation. Respir Physiol Neurobiol 234: , 89–96. |
[42] | Ayuse T , Ayuse T , Ishitobi S , Kurata S , Sakamoto E , Okayasu I , Oi K ((2006) ) Effect of reclining and chin-tuck position on the coordination between respiration and swallowing. J Oral Rehabil 33: , 402–408. |
[43] | Balasubramanium RK , Bhat JS ((2012) ) Respiratory swallow coordination in healthy individuals. Int J Adv Speech Hear Res 1: , 1–9. |
[44] | Boden K , Hardemark Cedborg AI , Eriksson LI , Hedstrom HW , Kuylenstierna R , Sundman E , Ekberg O ((2009) ) Swallowing and respiratory pattern in young healthy individuals recorded with high temporal resolution. Neurogastroenterol Motil 21: , 1163–e101. |
[45] | Dozier TS , Brodsky MB , Michel Y , Walters BC Jr, Martin-Harris B ((2006) ) Coordination of swallowing and respiration in normal sequential cup swallows. Laryngoscope 116: , 1489–1493. |
[46] | Fontecave-Jallon J , Baconnier P ((2016) ) A simple mathematical model of spontaneous swallow effects on breathing based on new experimental data. Annu Int Conf IEEE Eng Med Biol Soc 2016: , 4260–4263. |
[47] | Gurgor N , Arici S , Kurt Incesu T , Secil Y , Tokucoglu F , Ertekin C ((2013) ) An electrophysiological study of the sequential water swallowing. J Electromyogr Kinesiol 23: , 619–626. |
[48] | Hirst LJ , Ford GA , John Gibson G , Wilson JA ((2002) ) Swallow-induced alterations in breathing in normal older people. Dysphagia 17: , 152–161. |
[49] | Hardemark Cedborg AI , Sundman E , Boden K , Hedstrom HW , Kuylenstierna R , Ekberg O , Eriksson LI ((2009) ) Co-ordination of spontaneous swallowing with respiratory airflow and diaphragmatic and abdominal muscle activity in healthy adult humans. Exp Physiol 94: , 459–68. |
[50] | Hiss SG , Treole K , Stuart A ((2001) ) Effects of age, gender, bolus volume, and trial on swallowing apnea duration and swallow/respiratory phase relationships of normal adults. Dysphagia 16: , 128–135. |
[51] | Kelly BN , Huckabee ML , Cooke N ((2006) ) The coordination of respiration and swallowing for volitional and reflexive swallows-a pilot study. J Med SPEECH Lang Pathol 14: , 67. |
[52] | Kelly BN , Huckabee ML , Jones RD , Carroll GJ ((2007) ) The influence of volition on breathing-swallowing coordination in healthy adults. Behav Neurosci 121: , 1174–1179. |
[53] | Kelly BN , Huckabee M , Jones RD , Frampton CMA ((2007) ) Integrating swallowing and respiration: Preliminary results of the effect of body position. J Med Speech-Lang Pathol 15: , 347–355. |
[54] | Klahn MS , Perlman AL ((1999) ) Temporal and durational patterns associating respiration and swallowing. Dysphagia 14: , 131–138. |
[55] | Lederle A , Hoit JD , Barkmeier-Kraemer J ((2012) ) Effects of sequential swallowing on drive to breathe in young, healthy adults. Dysphagia 27: , 221–227. |
[56] | Leslie P , Drinnan MJ , Ford GA , Wilson JA ((2005) ) Swallow respiratory patterns and aging: Presbyphagia or dysphagia? J Gerontol Biol Sci Med Sci 60: , 391–395. |
[57] | Martin-Harris B , Brodsky MB , Price CC , Michel Y , Walters B ((2003) ) Temporal coordination of pharyngeal and laryngeal dynamics with breathing during swallowing: Single liquid swallows. J Appl Physiol (1985) 94: , 1735–1743. |
[58] | Matsuo K , Hiiemae KM , Gonzalez-Fernandez M , Palmer JB ((2008) ) Respiration during feeding on solid food: Alterations in breathing during mastication, pharyngeal bolus aggregation, and swallowing. J Appl Physiol (1985) 104: , 674–681. |
[59] | Matsuo K , Palmer JB ((2015) ) Coordination of oro-pharyngeal food transport during chewing and respiratory phase. Physiol Behav 142: , 52–56. |
[60] | McFarland DH , Lund JP , Gagner M ((1994) ) Effects of posture on the coordination of respiration and swallowing. J Neurophysiol 72: , 2431–2437. |
[61] | McFarland DH , Lund JP ((1995) ) Modification of mastication and respiration during swallowing in the adult human. J Neurophysiol 74: , 1509–1517. |
[62] | Nilsson H , Ekberg O , Olsson R , Kjellin O , Hindfelt B ((1996) ) Quantitative assessment of swallowing in healthy adults. Dysphagia 11: , 110–116. |
[63] | Nishino T , Yonezawa T , Honda Y ((1985) ) Effects of swallowing on the pattern of continuous respiration in human adults. Am Rev Respir Dis 132: , 1219–1222. |
[64] | Palmer JB , Hiiemae KM ((2003) ) Eating and breathing: Interactions between respiration and feeding on solid food. Dysphagia 18: , 169–178. |
[65] | Perlman AL , He X , Barkmeier J , Leer EV ((2005) ) Bolus location associated with videofluoroscopic and respirodeglutometric events. J Speech Lang Hear Res 48: , 21–33. |
[66] | Pinto CF , Balasubramanium RK , Acharya V ((2017) ) Nasal airflow monitoring during swallowing: Evidences for respiratory-swallowing incoordination in individuals with chronic obstructive pulmonary disease. Lung India 34: , 247–250. |
[67] | Preiksaitis HG , Mills CA ((1996) ) Coordination of breathing and swallowing: Effects of bolus consistency and presentation in normal adults. J Appl Physiol (1985) 81: , 1707–1714. |
[68] | Preiksaitis HG , Mayrand S , Robins K , Diamant NE ((1992) ) Coordination of respiration and swallowing: Effect of bolus volume in normal adults. Am J Physiol 263: , R624–R630. |
[69] | Smith J , Wolkove N , Colacone A , Kreisman H ((1989) ) Coordination of eating, drinking and breathing in adults. Chest 96: , 578–582. |
[70] | Wang CM , Li HY , Lee LA , Shieh WY , Lin SW ((2016) ) Non-invasive assessment of swallowing and respiration coordination for the OSA patient. Dysphagia 31: , 771–780. |
[71] | Wang CM , Chen JY , Chuang CC , Tseng WC , Wong AM , Pei YC ((2015) ) Aging-related changes in swallowing, and in the coordination of swallowing and respiration determined by novel non-invasive measurement techniques. Geriatr Gerontol Int 15: , 736–744. |
[72] | Wheeler Hegland KM , Huber JE , Pitts T , Sapienza CM ((2009) ) Lung volume during swallowing: Single bolus swallows in healthy young adults. J Speech Lang Hear Res 52: , 178–187. |
[73] | Wheeler Hegland K , Huber JE , Pitts T , Davenport PW , Sapienza CM ((2011) ) Lung volume measured during sequential swallowing in healthy young adults. J Speech Lang Hear Res 54: , 777–786. |
[74] | Hopkins-Rossabi T , Curtis P , Temenak M , Miller C , Martin-Harris B ((2019) ) Respiratory phase and lung volume patterns during swallowing in healthy adults: A systematic review and meta-analysis. J Speech Lang Hear Res 62: , 868–882. |
[75] | Charbonneau I , Lund JP , McFarland DH ((2005) ) Persistence of respiratory-swallowing coordination after laryngectomy. J Speech Lang Hear Res 48: , 34–44. |
[76] | Martin BJW (1991) The Influence of Deglutition on Respiration. Doctoral Dissertation, Northwestern University. |
[77] | Martin-Harris B , Kantarcigil C , Reedy EL , McFarland DH (2022) Cross-system integration of respiration and deglutition: Function, treatment, and future directions. Dysphagia, doi: 10.1007/s00455-022-10538-x. |
[78] | Wang CM , Shieh WY , Chen JY , Wu YR ((2015) ) Integrated non-invasive measurements reveal swallowing and respiration coordination recovery after unilateral stroke. Neurogastroenterol Motil 27: , 1398–1408. |
[79] | Martin-Harris B , McFarland D , Hill EG , Strange CB , Focht KL , Wan Z , Blair J , McGrattan K ((2015) ) Respiratory-swallow training in patients with head and neck cancer. Arch Phys Med Rehabil 96: , 885–893. |
[80] | Brodsky MB , McFarland DH , Dozier TS , Blair J , Ayers C , Michel Y , Gillespie MB , Day TA , Martin-Harris B ((2009) ) Respiratory-swallow phase patterns and their relationship to swallowing impairment in patients treated for oropharyngeal cancer. Head Neck 32: , 481–489. |
[81] | Curtis JA (2020) Mechanisms of Respiratory-Swallow Coordination and the Effects of Skill Training on Swallowing Rehabilitation in Parkinson’s Disease: Chapter 1: Introduction. Doctoral Thesis, Columbia University, pp. 1-73. |
[82] | Curtis JA (2020) Mechanisms of Respiratory-Swallow Coordination and the Effects of Skill Training on Swallowing Rehabilitation in Parkinson’s Disease: Chapter 2: Characterizing Respiratory-Swallow Coordination in Parkinson’s Disease and Its Effect on Swallowing Safety and Efficiency. Doctoral Thesis, Columbia University, pp. 74-142. |
[83] | Curtis JA (2020) Mechanisms of Respiratory-Swallow Coordination and the Effects of Skill Training on Swallowing Rehabilitation in Parkinson’s Disease: Chapter 5: Examining the Effects of Respiratory-Swallow Coordination Training on Swallowing rehabilitation and Motor Learning in Parkinson’s Disease. Doctoral Thesis, Columbia University, pp. 171-232. |
[84] | Yagi N , Nagami S , Lin MK , Yabe T , Itoda M , Imai T , Oku Y ((2017) ) A noninvasive swallowing measurement system using a combination of respiratory flow, swallowing sound, and laryngeal motion. Med Biol Eng Comput 55: , 1001–1017. |
[85] | Wang CM , Shieh WY , Weng YH , Hsu YH , Wu YR ((2017) ) Non-invasive assessment determine the swallowing and respiration dysfunction in early Parkinson’s disease. Parkinsonism Relat Disord 42: , 22–27. |
[86] | Argolo N , Sampaio M , Pinho P , Melo A , Nobrega AC ((2013) ) Do swallowing exercises improve swallowing dynamic and quality of life in Parkinson’s disease? Neurorehabilitation 32: , 949–955. |
[87] | Pinnington LL , Muhiddin KA , Ellis RE , Playford ED ((2000) ) Non-invasive assessment of swallowing and respiration in Parkinson’s disease. J Neurol 247: , 773–777. |
[88] | Lim A , Leow L , Huckabee ML , Frampton C , Anderson T ((2008) ) A pilot study of respiration and swallowing integration in Parkinson’s disease: “On” and “Off” Levodopa. Dysphagia 23: , 76–81. |
[89] | Golabbakhsh M , Rajaei A , Derakhshan M , Sadri S , Taheri M , Adibi P ((2014) ) Automated acoustic analysis in detection of spontaneous swallows in Parkinson’s disease. Dysphagia 29: , 572–577. |
[90] | Jenks J , Pitts LL ((2019) ) Effects of an intensive exercise-based swallowing program for persons with Parkinson’s disease and complex medical history: A single-case experiment. Am J Speech Lang Pathol 28: , 1268–1274. |
[91] | Troche MS , Huebner I , Rosenbek JC , Okun MS , Sapienza CM ((2011) ) Respiratory-swallowing coordination and swallowing safety in patients with Parkinson’s disease. Dysphagia 26: , 218–224. |
[92] | Gross RD , Atwood CW , Ross SB , Eichhorn KA , Olszewski JW , Doyle PJ ((2008) ) The coordination of breathing and swallowing in Parkinson’s disease. Dysphagia 23: , 136–145. |
[93] | Curtis JA , Dakin AE , Troche MS ((2020) ) Respiratory-swallow coordination training and voluntary cough skill training: A single-subject treatment study in a person with Parkinson’s disease. J Speech Lang Hear Res 63: , 472–486. |
[94] | Yagi N , Oku Y , Nagami S , Yamagata Y , Kayashita J , Ishikawa A , Domen K , Takahashi R ((2017) ) Inappropriate timing of swallow in the respiratory cycle causes breathing-swallowing discoordination. Front Physiol 8: , 676. |
[95] | Silverman EP , Carnaby G , Singletary F , Hoffman-Ruddy B , Yeager J , Sapienza C ((2016) ) Measurement of voluntary cough production and airway protection in Parkinson disease. Arch Phys Med Rehabil 97: , 413–420. |
[96] | Curtis JA , Troche MS ((2020) ) Effects of verbal cueing on respiratory-swallow patterning, lung volume initiation, and swallow apnea duration in Parkinson’s disease. Dysphagia 35: , 460–470. |
[97] | Ertekin C ((2014) ) Electrophysiological evaluation of oropharyngeal dysphagia in Parkinson’s disease. J Mov Disord 7: , 31–56. |
[98] | Hegland KW , Troche M , Brandimore A ((2019) ) Relationship between respiratory sensory perception, speech, and swallow in Parkinson’s disease. Mov Disord Clin Pr 6: , 243–249. |
[99] | Curtis JA , Huber JE , Dakin AE , Troche MS ((2022) ) Effects of bolus holding on respiratory–swallow coordination in Parkinson’s disease. Am J Speech Lang Pathol 31: , 705–721. |
[100] | Wang CM , Shieh WY , Ho CS , Hu YW , Wu YR ((2018) ) Home-based orolingual exercise improves the coordination of swallowing and respiration in early Parkinson disease: A quasi-experimental before-and-after exercise program study. Front Neurol 9: , 624. |
[101] | Page MJ , McKenzie JE , Bossuyt PM , Boutron I , Hoffmann TC , Mulrow CD , Shamseer L , Tetzlaff JM , Akl EA , Brennan SE , Chou R , Glanville J , Grimshaw JM , Hróbjartsson A , Lalu MM , Li T , Loder EW , Mayo-Wilson E , McDonald S , McGuinness LA , Stewart LA , Thomas J , Tricco AC , Welch VA , Whiting P , Moher D ((2021) ) The PRISMA 2020 statement: An updated guideline for reporting systematic reviews. BMJ 372: , n71. |
[102] | McWeeny S , Choe J , Norton E (2021) SnowGlobe: An Iterative Search Tool for Systematic Reviews and Meta-Analyses. 10.17605/OSF.IO/U25RN |
[103] | Ouzzani M , Hammady H , Fedorowicz Z , Elmagarmid A ((2016) ) Rayyan—a web and mobile app for systematic reviews. Syst Rev 5: , 210. |
[104] | NIH Quality Assessment Tool for Observational Cohort and Cross-Sectional Studies, https://www.nhlbi.nih.gov/health-topics/study-quality-assessment-tools |
[105] | Hedges LV , Tipton E , Johnson MC ((2010) ) Robust variance estimation in meta-regression with dependent effect size estimates. Res Synth Methods 1: , 39–65. |
[106] | Fisher Z , Tipton E , Zhipeng H (2017) robumeta: Robust variance meta-regression. https://github.com/zackfisher/robumeta |
[107] | Rosenbek JC , Robbins JA , Roecker EB , Coyle JL , Wood JL ((1996) ) A penetration-aspiration scale. Dysphagia 11: , 93–98. |
[108] | Neubauer PD , Rademaker AW , Leder SB ((2015) ) The Yale Pharyngeal Residue Severity Rating Scale: An anatomically defined and image-based tool. Dysphagia 30: , 521–528. |
[109] | Hutcheson KA , Barrow MP , Barringer DA , Knott JK , Lin HY , Weber RS , Fuller CD , Lai SY , Alvarez CP , Raut J , Lazarus CL , May A , Patterson J , Roe JWG , Starmer HM , Lewin JS ((2017) ) Dynamic Imaging Grade of Swallowing Toxicity (DIGEST): Scale development and validation. Cancer 123: , 62–70. |
[110] | Langmore SE , Schatz K , Olsen N ((1988) ) Fiberoptic endoscopic examination of swallowing safety: A new procedure. Dysphagia 2: , 216–219. |
[111] | Cohen MA , Setzen M , Perlman PW , Ditkoff M , Mattucci KF , Guss J ((2003) ) The safety of flexible endoscopic evaluation of swallowing with sensory testing in an outpatient otolaryngology setting. Laryngoscope 113: , 21–24. |
[112] | Sharma G , Goodwin J ((2006) ) Effect of aging on respiratory system physiology and immunology. Clin Interv Aging 1: , 253–260. |
[113] | Janssens JP , Pache JC , Nicod LP ((1999) ) Physiological changes in respiratory function associated with ageing. Eur Respir J 13: , 197–205. |
[114] | Pearson WG , Taylor BK , Blair J , Martin-Harris B ((2016) ) Computational analysis of swallowing mechanics underlying impaired epiglottic inversion: Mechanics of impaired epiglottic inversion. Laryngoscope 126: , 1854–1858. |
[115] | Martin-Harris B ((2008) ) Clinical implications of respiratory–swallowing interactions. Curr Opin Otolaryngol Head Neck Surg 16: , 194–199. |
[116] | Hopkins-Rossabi T , Armeson KE , Zecker SG , Martin-Harris B ((2021) ) Respiratory-swallow coordination and swallowing impairment in head and neck cancer. Head Neck 43: , 1398–1408. |
[117] | Owolabi L , Nagoda M , Babashani M ((2016) ) Pulmonary function tests in patients with Parkinson’s disease: A case-control study. Niger J Clin Pract 19: , 66–70. |