The Role of Microelectrode Recording in Deep Brain Stimulation Surgery for Parkinson’s Disease: A Systematic Review and Meta-Analysis
Abstract
Background:
STN-DBS is a cornerstone in the treatment of advanced Parkinson’s disease (PD). The traditional approach is to use an awake operative technique with microelectrode recording (MER). However, more centers start using an asleep MRI-guided technique without MER.
Objective:
We systematically reviewed the literature to compare STN-DBS surgery with and without MER for differences in clinical outcome.
Methods:
We systematically searched PubMed, Embase, MEDLINE, and Web of Science databases for randomized clinical trials and consecutive cohort studies published between 01-01-2000 and 26-08-2021, that included at least 10 PD patients who had received bilateral STN-DBS.
Results:
2,129 articles were identified. After abstract screening and full-text review, 26 studies were included in the final analysis, comprising a total of 34 study groups (29 MER and 5 non-MER). The standardized mean difference (SMD) in change in motor symptoms between baseline (OFF medication) and 6–24 months follow-up (OFF medication and ON stimulation) was 1.64 for the MER group and 1.87 for non-MER group (p = 0.59). SMD in change in levodopa equivalent daily dose (LEDD) was 1.14 for the MER group and 0.65 for non-MER group (p < 0.01). Insufficient data were available for comparative analysis of PDQ-39 and complications.
Conclusion:
The change in motor symptoms from baseline to follow-up did not differ between studies that used MER and those that did not. The postoperative reduction in LEDD from baseline to follow-up was greater in the MER-group. In the absence of high-quality studies comparing both methods, there is a clear need for a well-designed comparative trial.
INTRODUCTION
Parkinson’s disease (PD) is one of the most common neurodegenerative diseases, with an incidence that has doubled over the past 25 years [1]. Bilateral deep brain stimulation (DBS) of the subthalamic nucleus (STN) has been a well-established treatment for PD for several decades and has become a cornerstone in the treatment of advanced stages of PD. Despite the established benefits, an enormous heterogeneity exists in the way DBS-surgery is performed across centers [2]. Traditionally, the surgical procedure is performed awake and under local anesthesia allowing for intraoperative microelectrode recording (MER) and test stimulation with clinical testing for therapeutic benefits and adverse effects.
At the time when STN-DBS first emerged, the STN could not be visualized directly. Targeting had to be done indirectly based on stereotactic atlas coordinates and indirect anatomical landmarks that could be visualized by ventriculography. Therefore, there was a clear need for intraoperative verification of the correct target by MER and intraoperative test stimulation to assess the beneficial therapeutic response and to screen for stimulation side effects. Awake DBS with MER and intraoperative test stimulation became the standard of care and still is the preferred method in most centers around the world that perform DBS-surgery.
MER involves insertion of microelectrodes into each side of the brain to electrophysiologically identify and refine the location and border of the desired target to accurately determine the location of the permanent DBS electrode. This method typically requires one to five passes through each side of the brain and is often combined with intraoperative stimulation and clinical testing to screen for relief of motor symptoms such as rigidity, tremor, and akinesia, and to determine the threshold for stimulation-related side effects. This requires the patient to be awake during the procedure. The testing needs to be done in a practically defined OFF-state, so the anti-parkinsonian medication must be ceased for up to 24 hours prior to surgery. Consequently, a profound OFF-state in the night prior to surgery and in particular during the operation is typically accompanied by considerable discomfort.
A concern is that the traditional indirect targeting approach does not account for inter- and intra-individual variation in location, size and orientation of the STN. Advances in MRI techniques have made it possible to directly visualize the STN in a reliable manner. As a result, some centers have started to adopt a direct targeting approach where the intended target is defined on tailored MRI sequences and where the final electrode position is verified with an immediate postoperative MRI or CT. This approach has shown to be an alternative method for the more conventional approach, especially since the position of the final electrode within the MRI-defined STN is the most important predictor of long-term outcome [3]. Potential advantages of this approach could be that MER and intraoperative testing are no longer required, allowing for the entire procedure to be performed under general anesthesia (GA) and thereby improving patient comfort and reducing operative time and costs. Intermediate solutions have also been considered. Specifically, some studies show that MER can be used under GA or procedural sedation [4–6].
Although operative methods with and without the use of MER have both been integrated in daily clinical practice, there are no good quality comparative studies. We conducted a systematic review with a pooled analysis of the available DBS literature to search for possible differences in motor outcome, levodopa dose equivalence, complications, and quality of life between DBS surgery with and without the use of MER.
METHODS
The systematic review was performed in accordance with PRISMA (preferred reporting items for systematic reviews and meta-analyses) and MOOSE (meta-analysis of observational studies in epidemiology) guidelines [7, 8].
Search strategy and selection criteria
We systematically searched the PubMed, Embase, MEDLINE, and Web of Science databases from January 1, 2000, to August 26, 2021, using a comprehensive search strategy that was developed in consultation with a medical librarian. Search terms included “Parkinson’s disease”, “deep brain stimulation”, “subthalamic nucleus”, and “Unified Parkinson’s disease rating scale (UPDRS)”. Details of the full search strategy are provided in the Supplementary Material. The date limits of the search strategy reflect the period in which bilateral STN-DBS had already become a common and well-established treatment in the advanced management of PD. All titles and abstracts were assessed by two independent researchers (RSV and MG). In case there was a conflict between the two researchers whether to include a study, a third researcher (AKS) assessed the title and abstract and made the final decision. Studies were included in the full text review if they met all the following criteria: at least one group of the study population received bilateral STN-DBS for PD, the article was published in English language, the study was either a randomized controlled trial (RCT) or a consecutive cohort and the study population consisted of at least 10 patients with a follow up of at least 6 months and not more than 24 months. The upper limit of 24 months follow up was chosen to exclude the factor of disease progression. Studies that were not clear in describing their operative method were excluded. Studies that had any pre-operative, intraoperative or postoperative intervention that could influence the outcome measures, were only included if there was a control group that received surgical treatment only. In case of studies that used the same dataset for posthoc analyses, only the original study was included. The search strategy and items for data extraction were predefined and agreed upon by all authors.
Outcome measures
The primary outcome of this study was the change in the motor part (part III) of the UPDRS from pre-operative baseline (medication OFF state) to at least six months and not more than 24 months of postoperative follow up (medication OFF and stimulation ON). Secondary outcome measures were the reduction in levodopa equivalent daily dose (LEDD) from pre-operative baseline to at least 6 months and not more than 24 months of postoperative follow up and incidence of adverse effects and complications. The inclusion of articles for the meta-analysis was done separately for all outcome measures.
Data extraction and assessment of bias
All selected abstracts were evaluated in a full-text review and data extraction was performed by two independent researchers (MG and AKS). A list of variables that were extracted from each study is provided in the Supplementary Material. Studies were excluded from the review if the reported amount of data was insufficient to contribute to at least one of the outcome measures mentioned below. If multiple articles reported results from the same data source, the article with the most complete reporting of results was considered. If an article reported multiple groups with different DBS targets (e.g., internal segment of the globus pallidus and STN), only the data from the STN-DBS group were considered. In case a larger RCT did not provide sufficient data to be included in the analysis, an email was sent to the corresponding author to attempt to further complete the missing data. Due to the extensive search and the large number of articles, this could only be done for the larger trials to try to give the meta-analysis more power. Quality assessment of all individual articles was performed using the Newcastle-Ottawa Scale [9]. Funnel plot visualization and Egger’s Regression Test for Funnel Plot Asymmetry were used to assess for publication bias and small-study effects for the MER and the non-MER groups separately.
Data analysis
All data were analyzed descriptively in R [10], using the packages metaphor [11] and ggplot2 [12] for visualization. Due to the heterogeneity of the included studies, a meta-analysis was conducted for each of the outcome measures separately using restricted maximum likelihood (REML) in a random-effects model. Estimates of the two meta-analyses were compared using a Wald-type test. A p-value of <0.05 was considered statistically significant.
RESULTS
Our search identified a total of 2,129 unique articles. 276 of the screened abstracts qualified for a full-text review of which 30 were included in the analysis (Fig. 1). 242 studies were excluded based on the established in- and exclusion criteria. Two studies were excluded because full text was not available. Another two studies were excluded because they performed a posthoc analysis of previously published data. A total of 34 patient groups were identified, 29 in the MER group (26 studies), 5 in the non-MER group (4 studies). The included studies are described in Table 1.
Fig. 1
Flow diagram summarizing the literature review and the process of selection for the articles ultimately included in the meta-analysis.
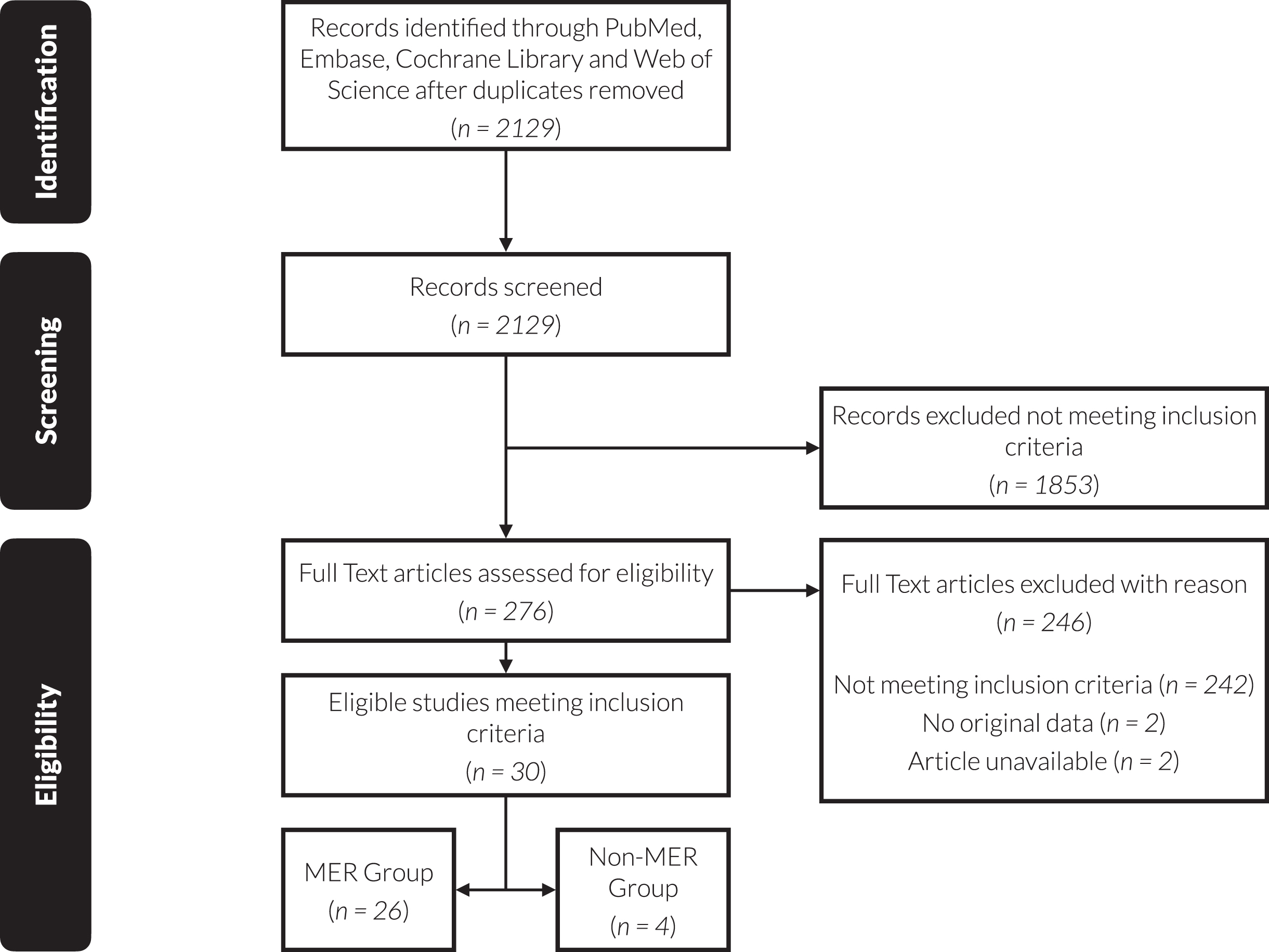
Table 1
Study characteristics
Author | Year | Use of | Study | Number of | Number Male | Mean Disease | Mean Age | Mean UPDRS- | Mean UPDRS- | Mean LEDD | Mean LEDD | Follow-Up |
MER | Design | Patients | Patients (%) | Duration (y) | at Surgery | III Pre- OP | III Post-OP | Pre-OP | Post-OP | (mo) | ||
Hung et al. [20] | 2013 | Yes | RC | 120 | N.A. | 9.8 | 59.3±11.1 | 45.6±14.7 | 27.1±11.1 | 779.1±389.5 | 425.1±258.4 | 12 |
Simuni et al. [21] | 2002 | Yes | PC | 12 | 10 (83%) | 12.0 | 58.0±11.0 | 43.5±3.6 | 23.0±2.2 | N.A. | N.A. | 12 |
Capecci et al. [22] | 2005 | Yes | PC | 23 | 12 (52%) | 12.8 | 59.5±7.5 | 38.3±11.6 | 17.9±11.7 | 987.9±427.0 | 708.0±311.0 | 12 |
Chiou et al. [23] | 2016 | Yes | RC | 72 | 48 (67%) | 7.9 | 61.1±9.7 | 40.5±15.6 | 30.5±11.7 | N.A. | N.A. | 6 |
Rabie et al. [24] | 2016 | Yes | RC | 20 | 6 (30%) | 11.7 | 61.1±8.7 | 40.0±12.4 | 19.7±7.1 | 1570.8±662.9 | 721.4±312.4 | 12 |
Lefranc et al. [5] | 2017 | Yes | RC | 10 | 5 (50%) | 12.6 | 63.1±10.0 | 33.1±5.4 | 20.0±10.5 | 1247.7±579.8 | 716.8±320.1 | 12 |
Lefranc et al. [5] | 2017 | Yes | RC | 13 | 9 (69%) | 12.1 | 62.8±7.1 | 16.7±5.4 | 18.0±7.2 | 1585.1±496.4 | 519.2±282.7 | 12 |
Deuschl et al. [25] | 2006 | Yes | RCT | 78 | 50 (64%) | 13.0 | 60.5±7.4 | 48.0±12.3 | 31.8±16.3 | 1176.0±517.0 | 597.0±381 | 6 |
Lyons et al. [26] | 2005 | Yes | RC | 59 | 44 (75%) | 11.9 | 59.5±9.8 | 41.3±9.8 | 24.3±11.2 | N.A. | N.A. | 12 |
Zibetti et al. [27] | 2007 | Yes | PC | 36 | 22 (61%) | 16.7 | 61.2±6.2 | 54.5±14.5 | 25.8±9.9 | 1023.0±428.9 | 405.9±306.3 | 12 |
Castelli et al. [28] | 2006 | Yes | PC | 65 | 38 (58%) | 15.1 | 60.5±6.5 | 54.4±13.4 | 27.2±11.7 | 1010.5±419.9 | 447.1±284.8 | 12 |
Zhang et al. [29] | 2006 | Yes | RC | 17 | N.A. | 11.3 | 63.8±7.8 | 49.2±19.3 | 38.1±29.7 | N.A. | N.A. | 12 |
Zhang et al. [30] | 2021 | Yes | RC | 57 | 34 (60%) | 10.1 | 64.1±8 | 60.0±23.0 | 30.0±17.0 | 831.0±453.0 | 475.0±220.5 | 6 |
Lemaire et al. [31] | 2016 | Yes | RC | 53 | 30 (57%) | 10.9 | 60.9±7.4 | 29.6±9.8 | 22.9±10.2 | 1182.3±411.0 | 1051.3±566.0 | 12 |
Chan et al. [32] | 2016 | Yes | PC | 25 | 17 (68%) | 13.0 | 55.0±6.0 | 45.0±18.6 | 19.3±14.1 | 1368.4±1014.0 | 496.3±491.0 | 12 |
Ryu et al. [33] | 2017 | Yes | RC | 28 | 7 (25%) | 11.4 | 56.9±7.7 | 44.6±11.6 | 26.6±11.0 | 1268.1±468.1 | 830.6±331.4 | 12 |
Rahmani et al. [34] | 2018 | Yes | RC | 35 | 22 (63%) | 12.0 | 54.7±8.5 | 46.0±14.2 | 22.2±11.3 | 1237.6±577.4 | 511.9±368.1 | 12 |
Jiang et al. [35] | 2019 | Yes | PC | 33 | 16 (48%) | 10.0 | N.A. | 40.0±11.3 | 27.8±7.1 | 848.0±373.7 | 616.4±331.6 | 12 |
Altug et al. [36] | 2014 | Yes | PC | 19 | 9 (47%) | 12.6 | 55.1±9.1 | 29.2±13.7 | 10.0±6.7 | 648.7±446.9 | 209.2±191.8 | 6 |
Tai et al. [37] | 2010 | Yes | PC | 12 | 9 (75%) | 11.8 | 62.5±12.8 | 41.7±13.5 | 17.7±9.4 | 954.2±522.0 | 495.8±210.5 | 12 |
Tai et al. [37] | 2010 | Yes | PC | 12 | 9 (75%) | 11.2 | 60.4±9.4 | 41.7±13.5 | 17.7±9.4 | 954.2±522.0 | 495.8±210.5 | 12 |
Chen et al. [6] | 2011 | Yes | RC | 19 | N.A. | 10.5 | 53.8±13.4 | 46.1±13.1 | 23.2±7.4 | 849.9±313.8 | 411.6±274.1 | 12 |
Chen et al. [6] | 2011 | Yes | RC | 33 | N.A. | 9.3 | 59.9±8.8 | 47.7±17.8 | 25.2±10.5 | 972.4±441.7 | 531.1±291.7 | 12 |
Tandra et al. [38] | 2020 | Yes | PC | 40 | N.A. | 7.3 | 55.5±4.9 | 61.1±10.8 | 39.6±10.0 | N.A. | N.A. | 6 |
Fluchere et al. [39] | 2014 | Yes | RC | 213 | 150 (70%) | 12.0 | 61.0±7.0 | 33.6±13.3 | 13.2±9.1 | 1173.0±495.0 | 636.0±376.0 | 12 |
Shin et al. [40] | 2020 | Yes | RC | 49 | 18 (37%) | 15.9 | 59.5±N.A. | 39.7±10.7 | 21.6±9.8 | 1181.8±420.8 | 931.8±418.0 | 12 |
Li et al. [41] | 2015 | Yes | RC | 195 | 105 (54%) | 6.8 | 58.2±10.0 | 68.3±12.1 | 20.2±12.1 | 802.0±407.0 | 534.0±327.0 | 12 |
Lhommee et al. [42] | 2012 | Yes | PC | 63 | 40 (63%) | 10.5 | 57.8 | 36.4±12.8 | 20.3±13.7 | 1306.0±475.0 | 400.0±386.0 | 12 |
Schuepbach et al. [43] | 2013 | Yes | RCT | 124 | 94 (76%) | 7.3 | 52.9±6.6 | 33.2±N.A. | 15.6±N.A. | 935.6±454.3 | 572.3±485.8 | 12 |
Aviles-Olmos et al. [44] | 2014 | No | PC | 41 | 27 (66%) | 12.9 | 56.2±8.4 | 50.3±15.8 | 22.9±12.8 | 1471.0±515.0 | 950.0±573.0 | 12 |
Foltynie et al. [45] | 2011 | No | PC | 79 | 49 (62%) | 13.4 | 57.3±7.7 | 51.5±14.9 | 23.8±11.2 | N.A. | N.A. | 12 |
Nakajima et al. [46] | 2011 | No | RC | 14 | 8 (57%) | 13.8 | 56.1±6.5 | 57.9±16.6 | 27.3±11.8 | 1505.0±764.0 | 764.0±435.0 | 12 |
Nakajima et al. [46] | 2011 | No | RC | 68 | 45 (66%) | 15.2 | 57.5±7.0 | 48.2±15.7 | 23.7±11.8 | 1138.0±680.0 | 805.0±596.0 | 12 |
Moran et al. [13] | 2020 | No | RC | 152 | N.A. | 11 | 60.0±9.0 | 39.0±11.0 | 20.5±9.5 | 1230.0±1107.0 | 793.0±830.0 | 12 |
Mean age at surgery, Mean UPDRS-III and Mean LEDD are displayed as mean±standard deviation. UPDRS-III, Unified Parkinson’s Disease Rating Scale part III; LEDD, Levodopa equivalent daily dose; RCT, randomized controlled trial, PC, prospective cohort; RC, retrospective cohort; N.A., not available.
Primary outcome: UPDRS-III
Our predefined primary outcome is the standardized mean difference between the preoperative UPDRS-III assessment in the OFF condition and the postoperative assessment with the stimulator turned on (medication OFF) 6–24 months after surgery. No significant difference was found between the MER and non-MER groups (MER 1.64 (CI 1.33, 1.96), non-MER 1.87 (CI 1.69, 2,05); p 0.59) (Fig. 2).
Fig. 2
Forest plot of the standardized mean difference of the UPDRS-III of A) MER group and B) non-MER group.
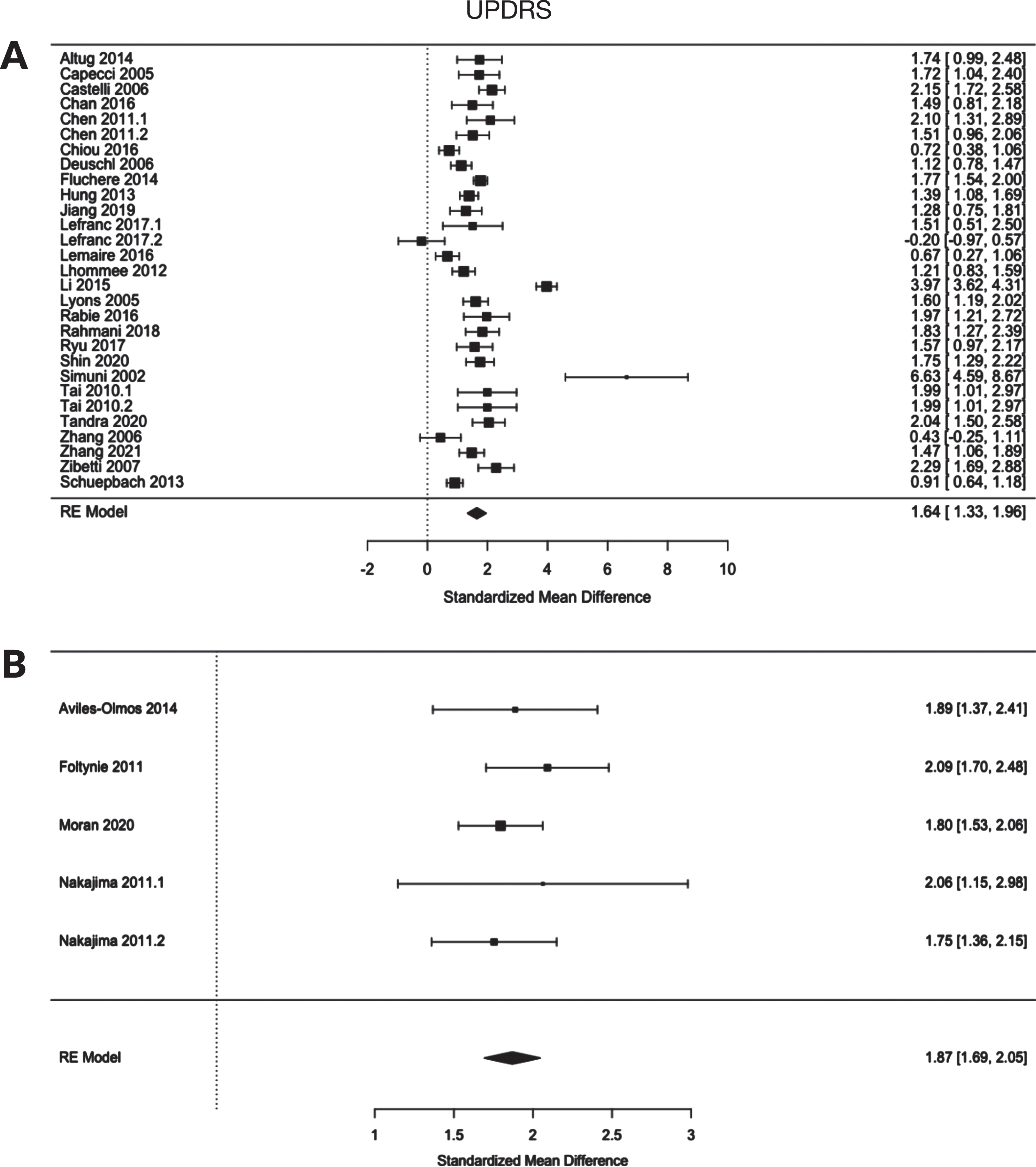
Secondary outcomes: LEDD, PDQ-39
21 studies with 24 groups in the MER group and 3 studies with 4 groups in de non-MER group reported on the LEDD. Here, we found a significant difference in the standardized mean difference (MER 1.14 (CI 0.95, 1.32), non-MER 0.65 (CI 0.37, 0.93); p < 0.01) (Fig. 3). For a comparative analysis of the quality of life (PDQ-39) no sufficient data were available. Only two studies in the non-MER group reported a PDQ-39 total or summary index score.
Fig. 3
Forest plot of the standardized mean difference of the LEDD of A) MER group and B) non-MER group.
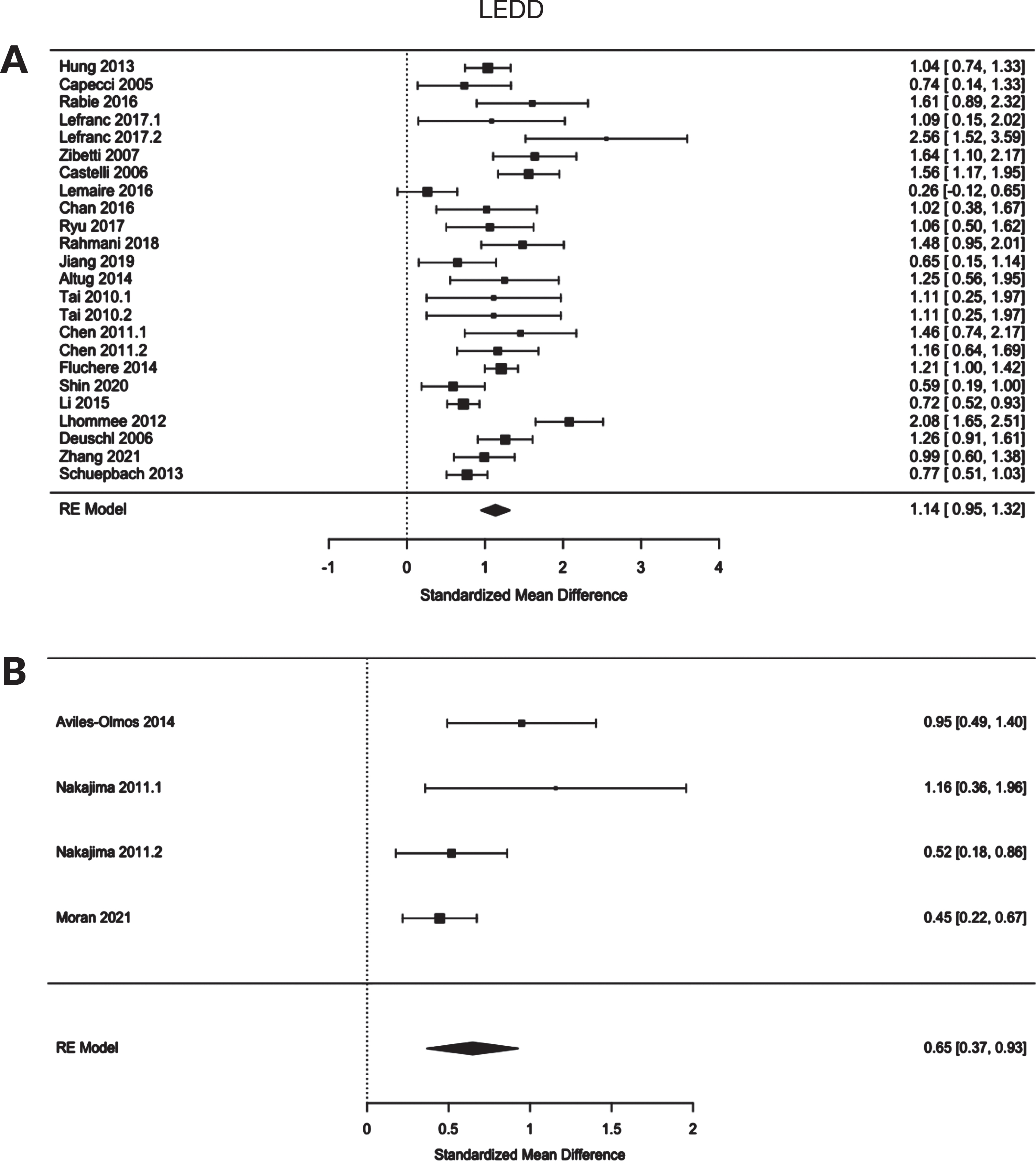
Adverse events
Complications were not reported consistently. There is no consensus on the definition of surgery-related adverse events, e.g., intracranial hemorrhage. Some studies reported only symptomatic hemorrhages, others reported all hemorrhages that occurred. Several studies lack any report of adverse events at all. Hence, no reliable analysis could be performed.
Risk of bias and quality assessment
Most included studies were non-randomized uncontrolled observational studies. Their quality was assessed using a modified Newcastle-Ottawa scale (the items “selection of the non-exposed cohort” and “comparability of cohorts” were not appropriate for assessing the quality of uncontrolled studies) (Supplementary Table 1). The main weakness of the studies is the lack of description whether the position of the leads was verified postoperatively by CT or MRI and whether the clinical assessment of our primary outcome measure (UPDRS-III) was performed in a blinded fashion. In addition, some studies had a high number of discontinuations at follow up with no reason given. Included RCTs were assessed according to Cochrane Handbook for Systematic Reviews of Interventions (Supplementary Table 2).
Publication bias was assessed using Egger’s regression test for funnel plot asymmetry performed on our primary outcome measure. This revealed significant publication bias in the MER group (p = 0.0084), but not in the non-MER group (p = 0.5236) (Supplementary Figures 1 and 2).
DISCUSSION
This is the first meta-analysis on the use of MER in STN-DBS-surgery for PD. Our main findings were as follows. First, descriptive and structured analysis of all included studies in this systematic review, shows that there was no difference in the change in UPDRS part III from pre-operative baseline to follow up at 6–24 months (medication OFF and stimulation ON) between the cohorts in which DBS-surgery was performed with MER and the cohorts in which DBS-surgery was performed without MER.
Second, the postoperative reduction in LEDD from baseline to 6–24 months follow-up was greater for the MER-group. However, there was no difference in the pre-operative LEDD between the two groups. The largest study in the non-MER group had a relatively small change in LEDD compared to the other studies in this group [13]. This could have been related to different local protocols for stimulation and reduction of medication. Therefore, the effect of the relatively small change in LEDD in this single study made the difference in LEDD between the MER and non-MER group more significant. Hypothetically, this could mean that, although there is no statistically significant difference in change in UPDRS part III, the MER-group may have a potential benefit in terms of reduced levodopa-induced side effects such as dyskinesias, wearing OFF, and others.
Finally, not enough studies were identified to provide sufficient data for a meta-analysis to compare the quality of life (PDQ-39) and adverse events other than hemorrhage.
All studies in which DBS-surgery was performed without MER, used an MRI-guided technique with direct postoperative verification of lead position based on an immediate postoperative MRI or CT. Only one study directly compared a surgical approach with and without the use of MER [14]. However, this study was underpowered and only reported medians with a confidence interval for UPDRS-III, LEDD and PDQ-39 and was therefore excluded from analysis.
Clinical relevance
This meta-analysis shows that there is no difference in clinical outcome between a surgical technique with and without MER. The success of both surgical approaches is highly dependent on the expertise available at any particular center. An MRI-guided approach without MER requires tailored MRI sequences that visualize the target and that are meticulously corrected for MRI distortion. Furthermore, it requires a surgical workflow that includes immediate postoperative imaging to verify the final electrode position. On the other hand, an MER-guided approach requires the expertise of a dedicated and experienced neurophysiologist to interpret the MER signals, a neurologist must be present in the operating theatre to perform intraoperative testing and patients must be awake and remain in the OFF-medication state during lead placement. Although some studies suggest that the use of MER leads to an improved accuracy of lead placement, other studies suggest that MER and the number of microelectrode passes are associated with an increased risk of intracerebral hemorrhage and cognitive decline [15–17]. Advances in MRI techniques have led to a better visualization of the STN, and more is known about the correlation between electrode position within the MRI-defined STN and long-term clinical outcome [3]. Because of these technological advances and the growing knowledge of functional anatomy, the role of MER in today’s DBS surgery is changing. Although the use of MER is still considered the gold standard, increasingly more centers are moving to an MRI-guided and image-verified approach.
Awake vs. asleep
A possible advantage of a procedure without MER, is that the procedure can be performed while the patient is asleep. A procedure under GA and without discontinuation of anti-parkinsonian medication, will afford much greater patient comfort and hence a better patient experience. However, LaHue et al. [18] show that 12% of the patients who underwent MRI-guided surgery under GA would have preferred to have surgery while being awake. Interestingly, they also showed that only 27% of the patients who underwent awake surgery would have preferred to be operated on while asleep. This suggests that there are certain subgroups of patients that prefer to be operated awake, while other subgroups prefer to be operated asleep. This is very much in keeping with the concept of personalized medicine, including a shared decision-making approach where patients are involved in making the choice for the type of surgical procedure. Nevertheless, several studies show that an MER-guide procedure could be performed safely under procedural sedation and GA, even though anesthetic agents have shown to alter firing patterns of the STN [4–6]. Importantly, while most of the debate in the literature is about asleep versus awake surgery, an equally relevant question is how the final electrode position is verified. Using the traditional awake procedure, this would be the electrophysiological signature of the STN in combination with the result of test stimulation and intraoperative clinical testing. Using an MRI-guided procedure, the final electrode position can be verified by an immediate postoperative MRI or CT. Furthermore, anatomical or stereotactical malposition can be corrected within the same operative session.
Cost-effectiveness
In terms of cost-effectiveness, the use of MER more than doubles the costs of bilateral STN-DBS. This significant increase in costs is mainly due to a longer operating time, the equipment required and the greater amount of personnel involved during the operation [19].
Limitations
This meta-analysis has several limitations. Despite the extensive literature search, only a relatively small number of studies was included in the meta-analysis. Unfortunately, there was a clear lack of high-quality studies providing robust data on methodology, operative procedure, outcome measures, adverse events, and follow-up. In- or exclusion criteria were strictly adhered to. For example, studies that were unclear about the use of MER or did not explicitly describe whether their cohort was consecutive, were excluded from the analysis. Studies that met the inclusion criteria, but only reported one or two of the outcome measures, were only included in the analysis of that particular outcome measure. Only RCTs and consecutive cohorts were included in the analysis to minimize selection bias. Another limitation is that the number of studies that used MER was considerably larger than the number of studies that did not use MER. This ratio may very well reflect daily clinical practice, as MER-guided DBS-surgery remains the most commonly used surgical technique worldwide [2]. Because there were no studies that directly compared both treatment groups, a paired meta-analysis was not an option. The variety in patient selection, target localization, operative method, hardware used, postoperative electrode verification, programming and adjusting stimulation and medication appears to be considerable. This most certainly has implications for the results in both treatment groups and how they compare to each other. In particular, the non-MER group is susceptible to this critique of the variations of approach across centers, since the size of the non-MER group is smaller. Another factor that may have affected the results is the publication bias in the MER group.
Future perspectives
An MRI-guided approach is associated with an outcome that is at least not inferior to the outcome of the traditional surgical approach with MER. This notion is in line with the current paradigm shift towards MRI-guided programming of DBS. Since electrode designs and stimulation options become more advanced, the number of possible stimulation settings are almost infinite. The conventional monopolar review to initiate stimulation appears to be too time-consuming to obtain all the potential benefit out all these stimulation options. Furthermore, the role of MER in today’s DBS-surgery should be further investigated by properly designed prospective comparative trials, with a back-to-back comparison to an MRI-guided approach. This is important first and foremost in light of patient comfort during surgery, but also from an economical perspective since the use of MER is associated with higher costs and a financial burden for the healthcare system.
CONFLICT OF INTEREST
The authors report no conflicts of interest and have nothing to disclose related to the research in this manuscript.
SUPPLEMENTARY MATERIAL
[1] The supplementary material is available in the electronic version of this article: https://dx.doi.org/10.3233/JPD-223333.
REFERENCES
[1] | Dorsey ER , Bloem BR ((2018) ) The Parkinson pandemic-a call to action. JAMA Neurol 75: , 9–10. |
[2] | Abosch A , Timmermann L , Bartley S , Rietkerk HG , Whiting D , Connolly PJ , Lanctin D , Hariz MI ((2013) ) An international survey of deep brain stimulation procedural steps. Stereotact Funct Neurosurg 91: , 1–11. |
[3] | Wodarg F , Herzog J , Reese R , Falk D , Pinsker MO , Steigerwald F , Jansen O , Deuschl G , Mehdorn HM , Volkmann J ((2012) ) Stimulation site within the MRI-defined STN predicts postoperative motor outcome. Mov Disord 27: , 874–879. |
[4] | Bos MJ , de Korte-de Boer D , Alzate Sanchez AM , Duits A , Ackermans L , Temel Y , Absalom AR , Buhre WF , Roberts MJ , Janssen MLF ((2021) ) Impact of procedural sedation on the clinical outcome of microelectrode recording guided deep brain stimulation in patients with Parkinson’s disease. J Clin Med 10: , 1557. |
[5] | Lefranc M , Zouitina Y , Tir M , Merle P , Ouendo M , Constans JM , Godefroy O , Peltier J , Krystkowiak P ((2017) ) Asleep robot-assisted surgery for the implantation of subthalamic electrodes provides the same clinical improvement and therapeutic window as awake surgery. World Neurosurg 106: , 602–608. |
[6] | Chen SY , Tsai ST , Lin SH , Chen TY , Hung HY , Lee CW , Wang WH , Chen SP , Lin SZ ((2011) ) Subthalamic deep brain stimulation in Parkinson’s disease under different anesthetic modalities: A comparative cohort study. Stereotact Funct Neurosurg 89: , 372–380. |
[7] | Moher D , Liberati A , Tetzlaff J , Altman DG , Group P ((2009) ) Preferred reporting items for systematic reviews and meta-analyses: The PRISMA statement. BMJ 339: , b2535. |
[8] | Stroup DF , Berlin JA , Morton SC , Olkin I , Williamson GD , Rennie D , Moher D , Becker BJ , Sipe TA , Thacker SB ((2000) ) Meta-analysis of observational studies in epidemiology: A proposal for reporting. Meta-analysis Of Observational Studies in Epidemiology (MOOSE) group. JAMA 283: , 2008–2012. |
[9] | Wells GA , The Newcastle-Ottawa Scale (NOS) for assessing the quality of nonrandomised studies in meta analyses, http://www.ohri.ca/programs/clinicalepidemiology/oxford.asp. |
[10] | Team RC, A language and environment for statistical computing., R Foundation for Statistical Computing, https://www.R-project.org/. |
[11] | Viechtbauer W ((2010) ) Conducting meta-analyses in R with the metafor package. J Stat Softw 36: , 1–48. |
[12] | Wickham H (2009) Ggplot2: Elegant Graphics for Data Analysis. |
[13] | Moran CH , Pietrzyk M , Sarangmat N , Gerard CS , Barua N , Ashida R , Whone A , Szewczyk-Krolikowski K , Mooney L , Gill SS ((2020) ) Clinical outcome of “asleep” deep brain stimulation for Parkinson disease using robot-assisted delivery and anatomic targeting of the subthalamic nucleus: A series of 152 patients. Neurosurgery 88: , 165–173. |
[14] | Engelhardt J , Caire F , Damon-Perriere N , Guehl D , Branchard O , Auzou N , Tison F , Meissner WG , Krim E , Bannier S , Benard A , Sitta R , Fontaine D , Hoarau X , Burbaud P , Cuny E ((2021) ) A phase 2 randomized trial of asleep versus awake subthalamic nucleus deep brain stimulation for Parkinson’s disease. Stereotact Funct Neurosurg 99: , 230–240. |
[15] | Zrinzo L , Foltynie T , Limousin P , Hariz MI ((2012) ) Reducing hemorrhagic complications in functional neurosurgery: A large case series and systematic literature review. J Neurosurg 116: , 84–94. |
[16] | Hertel F , Zuchner M , Weimar I , Gemmar P , Noll B , Bettag M , Decker C ((2006) ) Implantation of electrodes for deep brain stimulation of the subthalamic nucleus in advanced Parkinson’s disease with the aid of intraoperative microrecording under general anesthesia. . Neurosurgery 59: , E1138; discussion E1138. |
[17] | Montgomery EB Jr ((2012) ) Microelectrode targeting of the subthalamic nucleus for deep brain stimulation surgery. Mov Disord 27: , 1387–1391. |
[18] | LaHue SC , Ostrem JL , Galifianakis NB , San Luciano M , Ziman N , Wang S , Racine CA , Starr PA , Larson PS , Katz M ((2017) ) Parkinson’s disease patient preference and experience with various methods of DBS lead placement. Parkinsonism Relat Disord 41: , 25–30. |
[19] | McClelland S 3rd ((2011) ) A cost analysis of intraoperative microelectrode recording during subthalamic stimulation for Parkinson’s disease. Mov Disord 26: , 1422–1427. |
[20] | Hung H-Y , Tsai S-T , Lin S-H , Jiang J-L , Chen S-Y ((2013) ) Uneven benefits of subthalamic nucleus deep brain stimulation in Parkinson’s disease— A 7-year cross-sectional study. Tzu Chi Med J 25: , 239–245. |
[21] | Simuni T , Jaggi JL , Mulholland H , Hurtig HI , Colcher A , Siderowf AD , Ravina B , Skolnick BE , Goldstein R , Stern MB , Baltuch GH ((2002) ) Bilateral stimulation of the subthalamic nucleus in patients withParkinson disease: A study of efficacy and safety. JNeurosurg 96: , 666–672. |
[22] | Capecci M , Ricciuti RA , Burini D , Bombace VG , Provinciali L , Iacoangeli M , Scerrati M , Ceravolo MG ((2005) ) Functional improvement after subthalamic stimulation in Parkinson’s disease: A non-equivalent controlled study with 12-24 month follow up. J Neurol Neurosurg Psychiatry 76: , 769–774. |
[23] | Chiou SM ((2016) ) Benefits of subthalamic stimulation for elderly parkinsonian patients aged 70 years or older. Clin Neurol Neurosurg 149: , 81–86. |
[24] | Rabie A , Verhagen Metman L , Fakhry M , Eassa AY , Fouad W , Shakal A , Slavin KV ((2016) ) Improvement of advanced Parkinson’s disease manifestations with deep brain stimulation of the subthalamic nucleus: A single institution experience. Brain Sci 6: , 58. |
[25] | Deuschl G , Schade-Brittinger C , Krack P , Volkmann J , Schafer H , Botzel K , Daniels C , Deutschlander A , Dillmann U , Eisner W , Gruber D , Hamel W , Herzog J , Hilker R , Klebe S , Kloss M , Koy J , Krause M , Kupsch A , Lorenz D , Lorenzl S , Mehdorn HM , Moringlane JR , Oertel W , Pinsker MO , Reichmann H , Reuss A , Schneider GH , Schnitzler A , Steude U , Sturm V , Timmermann L , Tronnier V , Trottenberg T , Wojtecki L , Wolf E , Poewe W , Voges J , German Parkinson Study Group Neurostimulation Section ((2006) ) A randomized trial of deep-brain stimulation for Parkinson’s disease. N Engl J Med 355: , 896–908. |
[26] | Lyons KE , Pahwa R ((2005) ) Long-term benefits in quality of life provided by bilateral subthalamic stimulation in patients with Parkinson disease. J Neurosurg 103: , 252–255. |
[27] | Zibetti M , Torre E , Cinquepalmi A , Rosso M , Ducati A , Bergamasco B , Lanotte M , Lopiano L ((2007) ) Motor and nonmotor symptom follow-up in parkinsonian patients after deep brain stimulation of the subthalamic nucleus. Eur Neurol 58: , 218–223. |
[28] | Castelli L , Perozzo P , Zibetti M , Crivelli B , Morabito U , Lanotte M , Cossa F , Bergamasco B , Lopiano L ((2006) ) Chronic deep brain stimulation of the subthalamic nucleus for Parkinson’s disease: Effects on cognition, mood, anxiety and personality traits. Eur Neurol 55: , 136–144. |
[29] | Zhang JG , Zhang K , Ma Y , Hu WH , Yang AC , Chu JS , Wu ST , Ge M , Zhang Y , Wang ZC ((2006) ) Follow-up of bilateral subthalamic deep brain stimulation for Parkinson’s disease. Acta Neurochir Suppl 99: , 43–47. |
[30] | Zhang F , Wang F , Li W , Wang N , Han C , Fan S , Li P , Xu L , Zhang J , Meng F ((2021) ) Relationship between electrode position of deep brain stimulation and motor symptoms of Parkinson’s disease. BMC Neurol 21: , 122. |
[31] | Lemaire JJ , Pereira B , Derost P , Vassal F , Ulla M , Morand D , Coll G , Gabrillargues J , Marques A , Debilly B , Coste J , Durif F ((2016) ) Subthalamus stimulation in Parkinson disease: Accounting for the bilaterality of contacts. Surg Neurol Int 7: , S837–S847. |
[32] | Chan DT , Zhu CX , Lau CK , Poon TL , Cheung FC , Lee M , Taw B , Hung KN , Choi P , AuYeung M , Chan G , Cheung YF , Chan AY , Yeung JH , Mok VC , Poon WS , Hong Kong Movement Disorder Society ((2016) ) Subthalamic nucleus deep brain stimulation for Parkinson disease in Hong Kong: A prospective territory-wide 2-year follow-up study. World Neurosurg 93: , 229–236. |
[33] | Ryu HS , Kim MS , You S , Kim MJ , Kim YJ , Kim J , Kim K , Chung SJ ((2017) ) Comparison of pallidal and subthalamic deep brain stimulation in Parkinson’s disease: Therapeutic and adverse effects. J Mov Disord 10: , 80–86. |
[34] | Rahmani M , Benabdeljlil M , Bellakhdar F , Faris MEA , Jiddane M , Bayad KE , Boutbib F , Razine R , Gana R , Hassani MRE , Fatemi NE , Fikri M , Sanhaji S , Tassine H , Balrhiti IEA , Hadri SE , Kettani NE , Abbadi NE , Amor M , Moussaoui A , Semlali A , Aidi S , Benhaddou EHA , Benomar A , Bouhouche A , Yahyaoui M , Khamlichi AE , Ouahabi AE , Maaqili RE , Tibar H , Arkha Y , Melhaoui A , Benazzouz A , Regragui W ((2018) ) Deep brainstimulation in moroccan patients with Parkinson’s disease: Theexperience of Neurology Department of Rabat. FrontNeurol 9: , 532. |
[35] | Jiang JL , Chen SY , Tsai ST ((2019) ) Quality of life in patients with Parkinson’s disease after subthalamic stimulation: An observational cohort study for outcome prediction. Ci Ji Yi Xue Za Zhi 31: , 107–112. |
[36] | Altug F , Acar F , Acar G , Cavlak U ((2014) ) The effects of brain stimulation of subthalamic nucleus surgery on gait and balance performance in Parkinson disease. A pilot study. Arch Med Sci 10: , 733–738. |
[37] | Tai CH , Wu RM , Lin CH , Pan MK , Chen YF , Liu HM , Lu HH , Tsai CW , Tseng SH ((2010) ) Deep brain stimulation therapy for Parkinson’s disease using frameless stereotaxy: Comparison with frame-based surgery. Eur J Neurol 17: , 1377–1385. |
[38] | Tandra S , Ramavath B , Kandadai RM , Jabeen SA , Kannan MA , Borgohain R ((2020) ) Functional outcome of bilateral subthalamic nucleus-deep brain stimulation in advanced Parkinson’s disease patients: A prospective study. Ann Indian Acad Neurol 23: , 54–58. |
[39] | Fluchere F , Witjas T , Eusebio A , Bruder N , Giorgi R , Leveque M , Peragut JC , Azulay JP , Regis J ((2014) ) Controlled general anaesthesia for subthalamic nucleus stimulation in Parkinson’s disease. J Neurol Neurosurg Psychiatry 85: , 1167–1173. |
[40] | Shin HW , Kim MS , Kim SR , Jeon SR , Chung SJ ((2020) ) Long-term effectsof bilateral subthalamic deep brain stimulation on posturalinstability and gait difficulty in patients with Parkinson’sdisease. J Mov Disord 13: , 127–132. |
[41] | Li J , Zhang Y , Li Y ((2015) ) Long-term follow-up of bilateral subthalamic nucleus stimulation in Chinese Parkinson’s disease patients. Br J Neurosurg 29: , 329–333. |
[42] | Lhommee E , Klinger H , Thobois S , Schmitt E , Ardouin C , Bichon A , Kistner A , Fraix V , Xie J , Aya Kombo M , Chabardes S , Seigneuret E , Benabid AL , Mertens P , Polo G , Carnicella S , Quesada JL , Bosson JL , Broussolle E , Pollak P , Krack P ((2012) ) Subthalamic stimulation in Parkinson’s disease: Restoring the balance of motivated behaviours. Brain 135: , 1463–1477. |
[43] | Schuepbach WM , Rau J , Knudsen K , Volkmann J , Krack P , Timmermann L , Halbig TD , Hesekamp H , Navarro SM , Meier N , Falk D , Mehdorn M , Paschen S , Maarouf M , Barbe MT , Fink GR , Kupsch A , Gruber D , Schneider GH , Seigneuret E , Kistner A , Chaynes P , Ory-Magne F , Brefel Courbon C , Vesper J , Schnitzler A , Wojtecki L , Houeto JL , Bataille B , Maltete D , Damier P , Raoul S , Sixel-Doering F , Hellwig D , Gharabaghi A , Kruger R , Pinsker MO , Amtage F , Regis JM , Witjas T , Thobois S , Mertens P , Kloss M , Hartmann A , Oertel WH , Post B , Speelman H , Agid Y , Schade-Brittinger C , Deuschl G , EARLYSTIM Study Group ((2013) ) Neurostimulation for Parkinson’s disease with early motor complications. N Engl J Med 368: , 610–622. |
[44] | Aviles-Olmos I , Kefalopoulou Z , Tripoliti E , Candelario J , Akram H , Martinez-Torres I , Jahanshahi M , Foltynie T , Hariz M , Zrinzo L , Limousin P ((2014) ) Long-term outcome of subthalamic nucleus deep brain stimulation for Parkinson’s disease using an MRI-guided and MRI-verified approach. J Neurol Neurosurg Psychiatry 85: , 1419–1425. |
[45] | Foltynie T , Zrinzo L , Martinez-Torres I , Tripoliti E , Petersen E , Holl E , Aviles-Olmos I , Jahanshahi M , Hariz M , Limousin P ((2011) ) MRI-guided STN DBS in Parkinson’s disease without microelectrode recording: Efficacy and safety. J Neurol Neurosurg Psychiatry 82: , 358–363. |
[46] | Nakajima T , Zrinzo L , Foltynie T , Olmos IA , Taylor C , Hariz MI , Limousin P ((2011) ) MRI-guided subthalamic nucleus deep brain stimulation without microelectrode recording: Can we dispense with surgery under local anaesthesia? Stereotact Funct Neurosurg 89: , 318–325. |